- 1Centre d'Etude des Transformations des Activités Physiques et Sportives (CETAPS) - EA 3832, Faculty of Sport Sciences, University of Rouen, Rouen, France
- 2School of Exercise and Nutrition Science, Queensland University of Technology, Brisbane, QLD, Australia
- 3Movement Neuroscience Program, Institute of Health and Biomedical Innovation, Queensland University of Technology, Brisbane, QLD, Australia
- 4Centre for Sports Engineering Research, Sheffield Hallam University, Sheffield, United Kingdom
In this review of research on climbing expertise, we focus on different measures of climbing performance, including spatiotemporal measures related to fluency and activity states (i.e., discrete actions), adopted by climbers for achieving overall performance goals of getting to the end of a route efficiently and safely. Currently, a broad range of variables have been reported, however, many of these fail to capture how climbers adapt to a route whilst climbing. We argue that spatiotemporal measures should be considered concurrently with evaluation of activity states (such as reaching or exploring) in order gain a more comprehensive picture of how climbers successfully adapt to a route. Spatial and temporal movement measures taken at the hip are a traditional means of assessing efficiency of climbing behaviors. More recently, performatory and exploratory actions of the limbs have been used in combination with spatiotemporal indicators, highlighting the influence of limb states on climbing efficiency and skill transfer. However, only a few studies have attempted to combine spatiotemporal and activity state measures taken during route climbing. This review brings together existing approaches for observing climbing skill at performance outcome (i.e., spatiotemporal assessments) and process (i.e., limb activity states) levels of analysis. Skill level is associated with a spatially efficient route progression and lower levels of immobility. However, more difficult hold architecture designs require significantly greater mobility and more complex movement patterning to maintain performance. Different forms of functional, or goal-supportive, movement variability, including active recovery and hold exploration, have been implicated as important adaptations to physiological and environmental dynamics that emerge during the act of climbing. Indeed, recently it has also been shown that, when climbing on new routes, efficient exploration can improve the transfer of skill. This review provides new insights into how climbing performance and related actions can be quantified to better capture the functional role of movement variability.
Introduction
Traditionally, skilled climbing is characterized by the efficiency of spatial and temporal patterns that emerge at the center of mass (COM) during the act of climbing (Billat et al., 1995; Cordier et al., 1996). Temporal assessment quantifies the number and nature of stoppages relative to continuous climbing, indicating the amount of time spent in isometric contraction (Billat et al., 1995; White and Olsen, 2010). Spatial indicators highlight the efficiency of a climber's trajectory across the surface, estimating the ability to perceive an efficient “pathway” through the route (Cordier et al., 1993; Boschker and Bakker, 2002). Finally, combined spatiotemporal measures, such as the minimization of jerk, globally indicate how smoothly climbing movements are linked together (Seifert et al., 2014b). Importantly, evaluating performance along spatial and temporal variables can address different mechanisms underpinning skilled climbing (Cordier et al., 1996). For example, initial and rapid improvement in performance is believed to be primarily influenced by the rapidly adapting visual-motor system (Pezzulo et al., 2010). Alternatively, a climber may improve performance by linking movements in a more periodic fashion. These sorts of improvement occur over longer time-scales, such as the months and years required for musculoskeletal system adaptation (Vigouroux and Quaine, 2006).
More recently, activity states such as reaching and grasping have been distinguished as having exploratory (information gathering) or performatory (body progressing) qualities, providing an estimate of the intentions underpinning an individual's actions during climbing (Pijpers et al., 2006). For example, changing task constraints, such as height from the ground during climbing practice (Pijpers et al., 2006), does not physically modify climbing affordances. Where climbing affordances are defined as opportunities for qualitatively distinct actions that support climbing such as hold reachability, grasp-ability, stand on-ability and specific climbing movements (Boschker et al., 2002). However, increasing climbing height can interact with an individual's emotional state. This can alter the discrete actions used during climbing, transiently, on the basis of altered intentions brought about by an increased state of anxiety. In this case, changes in emotional states can influence intentions toward information pick-up for remaining fixed to the wall, as opposed to achieving vertical progression. Inferences of climbers' intentions are generally based on behavioral data. For example, when an individual reduces the distance they are willing to reach for grasping holds, or they increase their use of exploratory actions, this suggests the climber is primarily concerned with stability as opposed to efficient progression (Pijpers et al., 2006; Seifert et al., 2014c).
A limitation in the extant literature is poor understanding of how an individual's specific activity state can influence climbing efficiency and being able to combine these measures can be highly informative (Orth et al., 2016). Indeed, approaches that have considered these variables in combination have uncovered important insights into the functional or goal-supportive characteristics of movement variability (Fryer et al., 2012; Seifert et al., 2015). For instance, Pijpers et al. (2006) implied that exploratory behavior reflects poor performance. More recent studies have combined the analysis of exploratory actions with spatiotemporal performance outcomes, revealing that exploratory actions can be related to an improvement in performance through practice (Seifert et al., 2015). Thus, this review draws together studies that have reported on performance and discrete limb actions in climbing tasks to evaluate how, in combination, these analyses can explain successful and efficient climbing. The review is structured into three parts. First, we examine the existing state of the art on how spatial and temporal outcomes are used to quantify skilled climbing. Next, data pertaining to activity states are considered with respect to their functionality for the individual. Finally, hypotheses are presented for how activity states combined with spatial-temporal outcomes can indicate specific intentions of climbers during the act of climbing.
Search Methodology
Medline and SPORTDiscus databases were searched for published primary sources. Keywords related to climbing (rock climbing, ice climbing, mountain climbing, boulder climbing, artificial climbing, top-rope climbing, lead-rope climbing, mixed climbing, indoor climbing, outdoor climbing, route climbing, slope climbing) were pooled (via Boolean operation “OR”) and combined (via Boolean operation “AND”) with keywords related to skilled behavior (skill, transfer, perform, ability, expert, novice, beginner, intermediate, advanced, elite, dynamic, force, kinematics, kinetics, perception, action, cognition, behavior, center of mass, trajectory, movement, movement pattern, recall, gaze, vision, coordination, motor, feet, hand, foot, grasp, reach, pattern, intervention, pedagogy, feedback, constraint, coach, learn, practice, applied, train, fluency, fluidity, smoothness, jerk, activity state, classification, intention, exploration, strategy) and also pooled via Boolean operation “OR.” Results were limited to human participants, written in the English language, and, Medline and SPORTDiscuss databases searched from their earliest available record up to November 2016. Google Scholar was then used to scrutinize the related articles and referencing studies. Reference lists of all eligible studies were then manually inspected.
Articles were restricted to those written in the English language. Restrictions were also made on the participant sample, study design and outcomes measures. Specifically, for inclusion, studies were required to report sample characteristics so that ability level could be estimated as either beginner, intermediate, advanced, elite or upper elite (Draper et al., 2011a). Study designs were limited to experimental or technical reports that involved climbing a surface graded for difficulty (Draper et al., 2011a). Furthermore, studies where the task goal did not, implicitly or otherwise, require getting to the end of the route were excluded. For example, if the task required participants to adopt a static posture or perform isolated reach and grasp actions, it was excluded since such task constraints do not impose a route finding problem. Outcomes were restricted to at least one measure to quantify spatial, temporal patterns of the COM or limbs, or, activity state during actual climbing. Appraisal of article quality was evaluated in terms of potential contribution to understanding how activity states influence performance efficiency along spatial-temporal measures. Eligible experiments were then identified to a standardized form which was then used to extract relevant study data (see Table 1). These included: experimental design, sample characteristics, interventions (including detailed characteristics of route design properties), task characteristics, independent variables and levels, outcome measures, and comparisons and interaction effects.
Results
Using the search methodology, the Medline database yielded 1,099 titles and abstracts. These were screened yielding 35 relevant articles, which were identified and their full texts retrieved. Relevant studies were then screened using the standardized inclusion criteria and 13 eligible studies identified. Using the same search methodology, the SPORTDiscsuss database was searched. This analysis yielded 2,201 results from which titles and abstracts were screened, and 59 relevant articles identified. After duplicate removal, full texts were retrieved and eligibility was assessed using the standardized inclusion criteria, identifying 15 studies this way. The related articles, citing articles and reference lists of 7,400 eligible studies were searched using Google Scholar. 94 relevant studies were subsequently identified for eligibility screening. After duplicates were removed, an additional 13 eligible studies were identified this way. The article search was stopped at this point. From this pool of 41 studies, 21 fulfilled the eligibility criteria. These are summarized to Table 1 and form the basis of the discussion below.
Spatial and Temporal Measures of Skilled Adaptation to Route Properties in Climbing
Data on skilled climbing behavior can reflect coordination of actions to route properties, providing insights on the quality of movement adaptations. A number of studies have incorporated spatial and temporal measures into a single outcome to quantify climbing fluency. These have generally involved the analyses of the climbers' COM projection, to estimate velocity (Cordier et al., 1996; Sibella et al., 2007), acceleration (Cordier et al., 1996; Sibella et al., 2007), jerk (Seifert et al., 2014b), and phase portrait patterning (Cordier et al., 1996). Among these, linked to the number sub-movements used in carrying out an action (Elliott et al., 2010), jerk coefficients on hip movements provide the most straightforward indication of capacity to co-adapt spatial-temporal demands of performance (Seifert et al., 2014b). For example, Seifert et al. (2014b) calculated jerk coefficients on three dimensional hip translation and rotation accelerations. Here, jerk coefficients improved with practice on a route that involved use of different types of grasping techniques (overhand grasping and pinch grips), compared to no significant change on a route that required use of a single type of action (overhand grasping) (Seifert et al., 2014b).
Whilst expertise in climbing involves highly adaptive and proficient performance along both spatial and temporal dimensions in combination, current understanding of skill and practice effects has been primarily approached by considering each dimension separately (Cordier et al., 1996; Sibella et al., 2007).
Spatial Indicators of Climbing Fluency
Spatial indicators relate to analyses of displacement on a surface. Existing approaches include computation of the geometric index of entropy (GIE, see equation 1 below) (Cordier et al., 1993, 1994a,b; Boschker and Bakker, 2002; Pijpers et al., 2003; Sibella et al., 2007; Sanchez et al., 2010; Seifert et al., 2015; Watts et al., 2016; Orth et al., 2017), climb distance (Green and Helton, 2011; Seifert et al., 2013b, 2014c; Green et al., 2014), average movement distance (Nieuwenhuys et al., 2008), COM-to-wall distance (Zampagni et al., 2011), and planar displacement of the COM (Zampagni et al., 2011). Interpreting the quality of displacement with respect to a route is the main reason GIE has enjoyed widespread application (Cordier et al., 1993, 1994a,b; Boschker and Bakker, 2002; Pijpers et al., 2003; Sibella et al., 2007; Sanchez et al., 2010; Seifert et al., 2017).
Specifically, GIE is given for a given trajectory x : [O, T] → R3, letting Δx be the trajectory length (Equation 1) and Δc(x) the convex hull parameter. The GIE is given by:
According to Cordier et al. (1994b) the GIE can assess the amount of fluency of a curve. The higher the entropy value, the higher the irregularity of the climbing trajectory, whereas the lower the entropy value, the more regular is the climbed trajectory. GIE has a number of advantages over reported spatial variables, such as the average movement distance (Nieuwenhuys et al., 2008), in that it is based on theoretically generalizable principles (Cordier et al., 1994b), readily interpreted with respect to climbing activity, and, is effective for detecting skill (Cordier et al., 1993), practice (Cordier et al., 1993), route (Seifert et al., 2017) and technique effects (Boschker and Bakker, 2002; Sibella et al., 2007). Furthermore, data collection to perform an entropy calculation is highly feasible involving use of a single camera (Sanchez et al., 2010). Figure 1 shows how entropy is calculated (Figure 1A) and with respect to how changing the length of an analyzed trajectory with the convex hull affects outcomes.
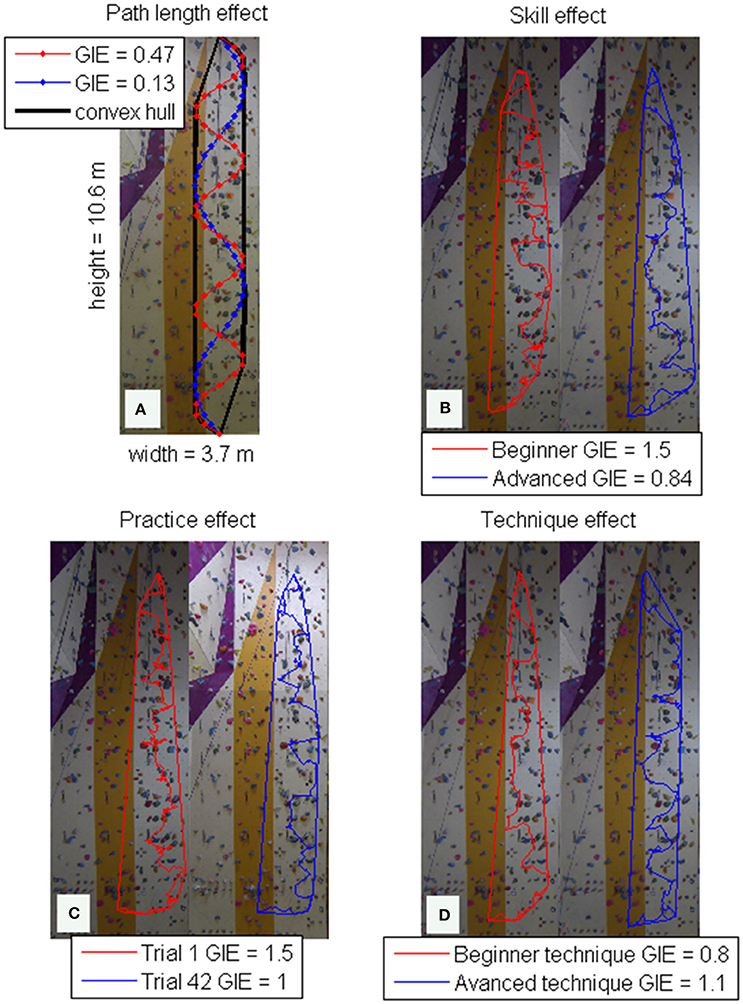
Figure 1. (A) Shows that the shorter the path length within a given convex hull, the lower the geometric index of entropy (GIE). (B) The blue line is data from an advanced climber, who shows a more straight forward trajectory (and thus lower GIE) compared to the beginners climbed trajectory (red line). (C) After practice (the blue line representing a climbed trajectory after 42 trials of practice) typically do not show periods of searching as shown in the first trial of practice (red line on the left). (D) When the technique used is more complex, GIE also increases. Here an individual was asked to climb the same route either with the front of the body remained facing the wall (red line) or with the side of the body facing the wall (blue line). The more advanced technique required an increase in movement complexity.
In climbing tasks, entropy outcomes are particularly increased when route difficulty is hard relative to the ability level of the climber, sometimes referred to as functional task difficulty (Guadagnoli and Lee, 2004), and, when the route has not yet been practiced (Cordier et al., 1994b). For example, when the functional difficulty of a route is increased, by modifying the number of choices embedded into it, entropy increases even in experienced climbers (Seifert et al., 2015). Practice effects have also been reported, with performance after repeated practice generally converging to an asymptote level at a rate dependent on the initial skill level of the climbers. Typically, the higher the initial skill level, the more rapid an asymptote is reached (Cordier et al., 1993, 1994a,b). Intriguingly, Boschker and Bakker (2002) found that prior knowledge about advanced inter-limb coordination patterns can improve entropy in beginners, allowing them to improve performance faster through practice (Seifert et al., 2013b).
Notably in Boschker and Bakker (2002), practice of less advanced techniques also resulted in entropy values similar to those observed when advanced actions were used, suggesting that the route designs may not have required more advanced technique for improved performance. Sanchez et al. (2010) also raised the concern, that, when elite climbers were compared on routes close to the limits of their ability level, no relationship between the climbers' performance GIE was shown. This may have been because the climbers had not practiced physically on the wall before testing began, and, the difficulty was close to the climbers' ability limits (Sanchez et al., 2010, p. 360). Implications of these studies suggest that, through observing repeated practice, larger learning effects can be expected when route difficulty is closer to a climber's ability level (Cordier et al., 1993). It is worth emphasizing, however, that these findings also indicate that in some cases a higher entropy may not necessarily indicate poor performance (Davids et al., 2014).
Aside from cases where task and skill interaction effects make entropy difficult to interpret, the variable is limited in other ways. Currently the application of GIE is limited to a single plane of analysis and important anterior-posterior plane translations (Sibella et al., 2007; Zampagni et al., 2011; Russell et al., 2012; Robert et al., 2013) or rotations around any given axis are missed (Seifert et al., 2014b, 2015). Of additional concern, is that if a climber is “blocked” at certain points in the climb, the GIE magnitude will only be influenced if there is an increase in the length of the trajectory during this time. For example. this can occur when postural readjustments are made. If no movement at the hip occurs during a stoppage, however, GIE magnitude will not be affected (Watts et al., 2016). Thus, changes in constraints, such as the use of a top-rope (a rope is secured to the top of the route prior to performance) vs. lead roping (where the climber needs to secure the rope to multiple fixed points during climbing for safety), may not lead to significant differences in entropy because they do not require a significant reorganization of the pathway taken through the route (cf. Hardy and Hutchinson, 2007). Nonetheless, GIE is a highly usable method, where limitations are made up for in ease of data acquisition and interpretation.
Temporal Indicators of Fluency
Temporal measures interpreted with respect to continuity of climbing performance include the: (i) relationship between static and dynamic movements at the hips (Cordier et al., 1994a; Billat et al., 1995; Nieuwenhuys et al., 2008; White and Olsen, 2010; Seifert et al., 2013b, 2014c); (ii) relationship between hold grasping and moving between holds (Pijpers et al., 2005, 2006; Nieuwenhuys et al., 2008; White and Olsen, 2010); (iii) plateau durations at the hips (Seifert et al., 2013b, 2014c); (iv) within-route climb time (Sanchez et al., 2010; Draper et al., 2011b; Seifert et al., 2013a); (v) time spent in three-hold support (Sibella et al., 2007); and (vi), movement frequency (Cordier et al., 1996).
Quantifying the amount of time spent in different climbing-specific activity states provides one of the better temporal indications of the climbers' adaptations to route properties. For example, the degree of mobility is sensitive to local changes in the route's difficulty level, including crux and rest points (Sanchez et al., 2010), and can detect differences between individuals who fall or complete the route (Draper et al., 2011b). The most predominant approach to estimate performance in the temporal dimension is the computation immobility to mobility ratio, calculated by determining how long, with respect to the total climb time, an individual's COM or limbs remain in a stationary state relative to its moving state.
According to Billat et al. (1995) time spent immobile reflects time under isometric contraction, subsequently incurring an energy cost. However, since depending on the nature of the hand holds, this time can either increase fatigue in the finger muscles (Vigouroux and Quaine, 2006) or provide an opportunity to allow these muscles to recover (Sanchez et al., 2012), the characteristics of the route design needs to be addressed (for an innovative modeling approach see, Tosi et al., 2011). Indeed, it has been shown that periods of immobility can reflect strategic actions with respect to demands on the physiological system imposed by route design (Billat et al., 1995; White and Olsen, 2010). For example, different gripping techniques provide the possibility to vary the arm angle, which might afford more or less rest while grasping a hold and remaining immobile (Amca et al., 2012). This is also true in terms of the overall posture that climbers can adopt. For example, when sitting away from the wall with arms extended, passive forces can be exploited for remaining on the wall at a reduced energy cost (Zampagni et al., 2011; Russell et al., 2012).
Alternatively, White and Olsen (2010) also speculated that high immobility at the hip, in the case of bouldering, reflects an inability to perceive how to move through a route continuously, reducing performance in the activity. Sanchez et al. (2012) provided some evidence for this argument, showing that more experienced climbers spent longer periods at rest locations within routes when not given an opportunity to view the route from the ground. This finding suggests that immobility can indicate visual exploration of upcoming holds. Thus, individuals might benefit from periods of immobility at the hips and longer periods of reaching because exploratory actions might help to determine more effective pathways through the route (Nieuwenhuys et al., 2008; Sanchez et al., 2010; Seifert et al., 2015). Indeed, typically beginners show high levels of immobility, suggesting a lack of effective pick-up of information for perceiving climbing opportunities for route progression (Pijpers et al., 2005, 2006).
A key disadvantage of immobility is that classifying an individual as immobile is commonly undertaken by frame-by-frame analysis of an operator. For example, criteria for mobility have included statements like: “progress of the hips was observed” (Billat et al., 1995) whereas, criteria for static climbing have included: “no discernible movement in pelvic girdle” (White and Olsen, 2010). In an ice-climbing study, an automatic approach was taken by Seifert et al. (2014c) using a definition based on a movement threshold. In this case, immobility was considered when, along the vertical axis, pelvis displacement was less than 0.15 m for durations longer than 30 s. This approach, however, required manual digitisation of the hips and was limited to analysis of vertical displacement actions of ice-climbers. Similar problems arise when manually coding limb states, where a limb is determined as moving between holds (mobile) or is in contact with a support surface (immobile) (Pijpers et al., 2006; White and Olsen, 2010). Thus, since immobility is generally determined as the lack of displacement over time, directly using velocity is a possible solution suggested here. Specifically, for a trajectory x : [O, T] → R3, we find the threshold based immobility to mobility ratio as:
Of additional concern when using immobility is that the (ir)regularity in the temporal dynamics of movements are not considered (Seifert et al., 2013b). For example, a climber could remain immobile at a single location on the wall, with the remaining climb time measured as mobile. Cordier et al. (1996), addressed this concern using a spectral dimension analysis of the last five practice trials (of 10) and showed that temporal movement dynamics of experts were periodic, since they displayed vertical displacement of the hips at regular intervals of 3 s. Furthermore, phase portrait analyses of each group revealed that skilled individuals displayed more regular movement characteristics (stable dynamics), whereas, intermediate climbers exhibited less predictable dynamics. These findings suggested that advanced climbers achieved a stable “coupling” between their coordination repertoire and the environmental features. The temporal analyses used with reference to their GIE analysis (Cordier et al., 1993, 1996), showed that, whilst the intermediate climbers achieved similar levels of GIE efficiency relative to the advanced group, they still required more training to improve efficient temporal dynamics. Indeed, the major limitation of spatial and temporal measures is that, although they provide important information in isolation, interpreting the nature of movement adaptions during climbing can be enhanced by considering these outcomes in combination (Draper et al., 2011a; Magiera et al., 2013; Seifert et al., 2013a; Laffaye et al., 2014).
Multi-Variate Approaches to Understanding Climbing Fluency
Thus, we now consider in more detail how combined measures of spatial-temporal indicators of performance can improve interpretation of climbing performance behaviors using exemplary data (Orth et al., 2014). In Figure 2, both immobility (using Equations 3–5) and GIE (Equations 1, 2) are calculated on a climbed trajectory at three sections of a beginner level route (French rating scale of difficulty = 5a). It is shown that, depending on which section of the route the climber is in, the relationship between GIE and immobility can be inversed. Indeed, spatial and temporal properties of behavior are probably co-adapted depending on the constraints on performance (Billat et al., 1995). For example, when required to use complex movements, such as when using dynamic moves, a high degree of mobility is probably also important. Conversely, when using less dynamic movements, a low level of mobility may help maintain a degree of stability, particularly when needing to keep the COM close to the wall (Fuss et al., 2013). If co-adaptation between GIE and IMR do support efficient climbing, a clear hypothesis is that immobility and movement complexity are co-adapted to maintain performance in terms of smoothness or jerk (Seifert et al., 2014b, 2015).
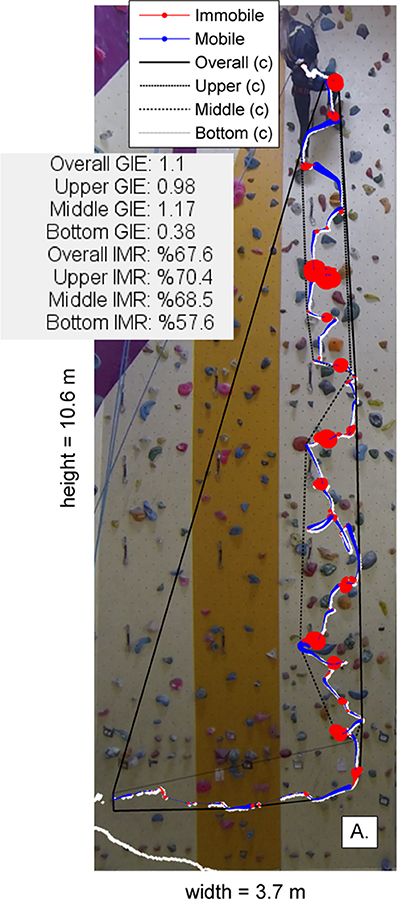
Figure 2. The relationship between entropy and immobility as a function of wall position. Radius of each point was scaled to increase in proportion to the duration spent in a given state (thus the larger the dot, the longer the individual was in the given state of mobility (i.e., blue line) or immobility (redline). c, convex hull. Also note that upper means the top convex hull. Middle means the middle convex hull, bottom means bottom convex hull, and overall means the total convex hull. GIE, geometric index of entropy; IMR, immobility to mobility; m, meters.
An important limitation in understanding the results related to performance fluency such as, Jerk, GIE, and IMR, is that, without a consideration of the climbers intentions during periods of immobility or increased entropy, these data may be mistakenly concluded as dysfunctional (Seifert et al., 2014b). The study by Fryer et al. (2012) illustrates this point nicely. In this study more experienced climbers exhibited a greater percentage of time spent immobile, compared to less experienced individuals. After carrying out an activity analysis into the types of actions undertaken during rest, it was found that the more experienced climbers were actively resting during immobility, either applying chalk to their hands or shaking their hands. In this example, without additional data from the activity analysis, it may have been erroneously concluded that the climbers were stopping more due to the greater physiological demand imposed by the route. In actual fact, the data highlighted the climbers' self-management of their internal states, relative to their exploitation of opportunities for rest in the climbing route, an important skill-dependent performance behavior (Fryer et al., 2012). This case exemplifies how interpreting activity states of climbers can provide mechanistic insights on fluency measures (Seifert et al., 2013b, 2015).
The Role of Activity States in Climbing for Understanding Performance
It is generally assumed that the task goal corresponds to the intentions of the individual where in climbing, the goals of the task are to: (a) not fall; (b) get to the end of a route, and (c), use an efficient pathway and movement patterning that reduces prolonged pauses (Orth et al., 2016). However, importantly, intentions can be influenced by skill (Rietveld and Kiverstein, 2014), which are reflected in adaptations that emerge with respect to dynamic constraints (Balagué et al., 2012; Davids et al., 2015). Thus, estimates of the intentions of individuals during performance can help place performance outcomes more accurately in line with what an individual was trying to achieve.
Seifert et al. (2014c), for example, showed that expert ice-climbers went about achieving their intentions to maintain energy and economy by focusing perception and action toward specific intentions. Actions were related to the perception of information for the usability of existing holes in the ice fall in so far that they tended to seek holds that did not require them to swing their ice tool. In contrast, the intentions of inexperienced climbers pertained to stability, where perceptions were focused on information related to the size of holes in the ice surface. In this case actions were motivated for achieving deep, secure, anchorages during ascent. Indeed, inexperienced climbers displayed significantly longer periods of immobility at the hips, higher amounts of swinging actions prior to making a definitive anchorage with their ice-tools, and tended to adopt a “X-like” body position with the arms and legs spread out for stability. Whilst the inexperienced climbers showed poor performance in terms of temporal fluency, their exploratory actions were in correspondence to the key intention to avoid falling. Thus, the distinction between exploratory and performatory actions is fundamental to understanding climber intentions and the functionality of their actions during performance and learning.
Performatory Actions
According to Pijpers et al. (2006), performatory actions are meant to reach a specific goal and include: moving a hand or foot from one hold to the next to use it as support for further climbing actions (Pijpers et al., 2006; Nieuwenhuys et al., 2008; White and Olsen, 2010); using a hold to move the entire body vertically or ascend the route (Sanchez et al., 2012; Seifert et al., 2013a, 2014c); using a hold to support recovery actions (Fryer et al., 2012; Sanchez et al., 2012); and, making visual fixations during movement at the hips (Nieuwenhuys et al., 2008). Theoretically, performatory actions correspond to actions that are intended for progression. If performatory actions are effective they should improve fluency, by reducing the amount of time spent immobile and contributing to ongoing progression through the route. For example, a climber might skip holds, use a more difficult movement (Sibella et al., 2007) or use less advanced actions (Boschker and Bakker, 2002) which might result in more or less fluid climbing performance.
Exploratory Actions
Exploratory actions, on the other hand, are primarily information gathering movements (Pijpers et al., 2006) where the type of information important to support perception of movement opportunities on a surface (i.e., affordances) can pertain to modalities such as haptic, auditory, visual and kinesthetic (Smyth and Waller, 1998; Seifert et al., 2014c). Exploratory actions have included: when climbers explore whether a hold is within reach (Pijpers et al., 2006); when a hold is touched without being used as a support (Pijpers et al., 2006; Nieuwenhuys et al., 2008; Sanchez et al., 2012; Seifert et al., 2013b, 2014b,c); when an anchorage is weighted to test its fallibility (Seifert et al., 2014c); when tools are used to swing without a definite anchorage (Seifert et al., 2011, 2013a, 2014c); and when a visual fixation occurs whilst an individual is immobile (Nieuwenhuys et al., 2008). An increase in exploratory indices is generally associated with poorer performance on measures of fluency (Orth et al., 2016). For example, if a climber stops because they cannot perceive an effective path through the route (Cordier et al., 1993; Sanchez et al., 2012), this would be associated with a higher frequency of hold exploration (Pijpers et al., 2006) and possibly an increased GIE (Cordier et al., 1994b).
However it is important the functionality of exploration, since as exploration reduces, fluency can improve (Seifert et al., 2013c, 2014b), suggesting an important relationship between exploration and performance improvement through practice. For example, Seifert et al. (2015) recently showed how exploration remained elevated under transfer conditions after a period of variable practice (i.e., where each training session involved practice on one of three different routes). In this study, implications were that potential mechanisms underpinning the positive transfer in climbing were related to the efficient use of exploration.
Variability in Activity States and Their Functionality
In this final section, we explore some of the implications of linking different activity states with performance outcomes (summarized in Table 2) with predictions for future work. Specifically, we attempt to explain the goals or intentions underpinning behavioral variability related to both activity state and spatial-temporal measures. Indeed, a key outcome of this review has been the identification of a broad range of activity states that have been reported in the literature as potentially important for performance during climbing. As clarified in Table 2, key activity states include: immobility; postural regulation; grasping; grip change; active recovery; reaching; reaching and withdrawing; traction; and, chaining movements in succession.
Typically, total immobility is a sign of poor performance (e.g., being “blocked”). However, functional movement variability can be identified. Postural exploration is probably particularly relevant for beginners, as this may allow an individual to determine more efficient positions and new body-wall orientations that may be important for more advanced movements (Seifert et al., 2015). Another possibility discussed has been that the individual may benefit from immobility by visually exploring upcoming holds, perhaps indicated by the amount of fixations made and their relative distance to the individual during immobility (Sanchez et al., 2012).
Exploration can also include reaching to touch a hold but not grasping it or using it to support the body weight (Seifert et al., 2015). This is probably important for perceiving accurate body-scaled actions (Pijpers et al., 2007). Perhaps, as different techniques, such as dynamic moves (Fuss et al., 2013), become part of an individual's action capabilities, this boundary of reachability may distinguish individuals of different skill levels. Making adjustments in how a hold is grasped prior to using it is also a form of exploration in terms of its “grasp-ability” affordance. Prior to applying force to a hold climbers can be seen, in some cases, to make adjustments to how they position their hand on a hold. Such exploratory actions may be important to improve the amount of friction that can be applied to the hold (Fuss et al., 2013), or, to enable a qualitatively different way of using the hold such as in cases where multiple edge orientations are available (Seifert et al., 2014b).
Finally, It has been argued that exploration can support perception of affordances or opportunities for new climbing moves (Seifert et al., 2013c). This may be observed by examining how climbing actions differ through practice. For example, over repeated attempts, different route pathways, body orientations or grasping patterns might be used, reflecting exploration emerging during learning. Thus, during interventions, the nature of learning behavior, in so far that it can be related to the progression toward higher levels of performance (or fluency), may be better understood by evaluating the level at which exploration emerges.
A substantial challenge, in future research is in measuring exploration at different levels of analysis with respect to performance, both, in technically manageable and theoretically consistent ways (Seifert et al., 2014d; Orth et al., 2016; Schmidt et al., 2016). For instance, whilst, performatory and exploratory actions are predominantly assessed by considering overt action at the limbs, such characteristics are distinguishable across other levels, such as overall organization of the body (Russell et al., 2012; Seifert et al., 2014a), postural regulation (Boulanger et al., 2016), visual search (Nieuwenhuys et al., 2008) and at more refined levels of control at hand-hold interaction (Fuss and Niegl, 2008). Identification of these movement features is a clear research challenge for future work.
In particular the role of exploration for improving transfer is worth more attention. Indeed, any on-sight climb (where a climber attempts to climb a route they have never physically practiced) might be conceptualized as a skill transfer problem, requiring adaptations during performance to unfamiliar surface properties and in dynamic environments (such as outdoors). Assuming positive transfer (Carroll et al., 2001; Issurin, 2013) is supported by the ability to skillfully seek efficient route pathways and climbing opportunities, interventions aiming to improve performance on new routes should consider the functional role of exploration during practice.
Conclusion
This review has demonstrated the importance of relating fluency and activity measures for understanding climbing actions and performance outcomes. Whilst numerous variables have been reported across the extant literature, many of these fail to capture how climbers adapt to a route whilst climbing. We have argued that there should be an emphasis on considering spatiotemporal measures concurrent with the evaluation of climbing specific activity states. Depending on the level of detail, such states can include: immobility; postural regulation; grasping; grip change; active recovery; reaching; reaching and withdrawing; traction; and, chaining movements in succession. In doing so, a more comprehensive picture of how climbers successfully adapt to a given route can be taken. In particular, the climber's intentions should be easier to estimate. For example, by combining these data, it is possible to more accurately determine whether an individual is stopping in order to recover or because he/she cannot perceive opportunities for progressing. We have also highlighted limitations in traditional performance measures (i.e., entropy and immobility). If activity analysis is not feasible, the main recommendation is that entropy and immobility should be concurrently assessed with respect to jerk. In doing so, the efficiency with which a climber is able to co-adapt movement complexity with required mobility can be addressed.
For future research, there is a major lack of understanding for how climbers transfer their skills to new routes and warrants more innovative approaches. Skill transfer is an essential part of climbing and indeed physical activity and sports in general. We anticipate that more successful climbers are more effective in how they explore new routes. Thus, characterizing how exploration is functional to climbers, and how they learn to explore effectively, such as based on practice constraints that require exploration, is a key problematic for future work.
Practical Summary
Findings in this review have a number of practical implications.
• Skilled performance in climbing can be broadly characterized in terms of how fluently an individual reaches the end of the route. Measures of jerk (at the body's center of mass) currently provide the best global indicators of fluency. Skilled climbers maintain fluency under changing constraints by adapting both how fast they climb (their level of mobility) and the complexity of their movements. The clearest indication of these adaptive behaviors is in experiments involving modification of hold size, the orientation of holds and the number of ways a hold can be used. In cases where holds require more complex body positions, experienced climbers will increase both how fast they climb and how complex their movements are.
• In this review we stressed that skilled climbers explore efficiently—this allows them to adapt strategies, while climbing, to help maintain performance. Indeed, efficient exploration would seem essential for effective performance under on-sight conditions. Constraints that support learning that improves on-sight performance, may be achieved by promoting exploration during practice. Recommendations are that the transition toward skilled behavior involves learning efficient exploration across different levels (i.e., using hands/feet, limb, varied hip orientations and visual inspection). Learning design should support each learner's current needs (such as maintaining stability or improved performance). Practitioners have an abundance of strategies at their disposal—what is essential is that movement variability, such as exploration, can be viewed as functional to the individual.
• These findings also provide practitioners with a way of assessing learning. Currently, automatic tracking procedures of the climber's hips and limbs en route presents an opportunity to assess learning. For instance, the rate of learning on new routes may be indexed as the rate at which jerk stabilizes or the rate that exploration subsides. In facilitating learning, the practitioner should constrain information during practice that can be meaningful to the climber for future performance and practice contexts. We suggest designing practice tasks to influence affordance perception. That is, to manipulate constraints so that the individual is challenged during practice to seek out movement opportunities relevant to completing a route fluently. Consistent with the findings in this review, early in learning or under challenging circumstances, affordance perception provides a means of remaining fixed to the surface. Later in learning, a climber perceives affordances for linking movements in a more periodic and fluent manner, supporting efficient and effective performance.
Author Contributions
DO, GK, KD, and LS conceived, performed and wrote the review in collaboration.
Funding
This project received the support of the CPER/GRR1880 Logistic, Mobility and Numeric and Injury Prevention Program, Institute of Health and Biomedical Innovation, Queensland University of Technology and funding from the French National Agency of Research (reference: ANR-13-JSH2-0004 DynaMov) and the French Federation of Alpine Club (FFCAM).
Conflict of Interest Statement
The authors declare that the research was conducted in the absence of any commercial or financial relationships that could be construed as a potential conflict of interest.
References
Amca, A. M., Vigouroux, L., Aritan, S., and Berton, E. (2012). Effect of hold depth and grip technique on maximal finger forces in rock climbing. J. Sports Sci. 30, 669–677. doi: 10.1080/02640414.2012.658845
Balagué, N., Hristovski, R., and Aragonés, D. (2012). Nonlinear model of attention focus during accumulated effort. Psychol. Sport Exerc. 13, 591–597. doi: 10.1016/j.psychsport.2012.02.013
Billat, V., Palleja, P., Charlaix, T., Rizzardo, P., and Janel, N. (1995). Energy specificity of rock climbing and aerobic capacity in competitive sport rock climbers. J. Sports Med. Phys. Fitness 35, 20–24.
Boschker, M. S., and Bakker, F. C. (2002). Inexperienced sport climbers might perceive and utilize new opportunities for action by merely observing a model. Percept. Mot. Skills 95, 3–9. doi: 10.2466/pms.2002.95.1.3
Boschker, M. S., Bakker, F. C., and Michaels, C. F. (2002). Memory for the functional characteristics of climbing walls: perceiving affordances. J. Mot. Behav. 34, 25–36. doi: 10.1080/00222890209601928
Boulanger, J., Seifert, L., Hérault, R., and Coeurjolly, J. F. (2016). Automatic sensor-based detection and classification of climbing activities. Sens. J. IEEE 16, 742–749. doi: 10.1109/JSEN.2015.2481511
Carroll, T. J., Riek, S., and Carson, R. G. (2001). Neural adaptations to resistance training. Sports Med. 31, 829–840. doi: 10.2165/00007256-200131120-00001
Cordier, P., Dietrich, G., and Pailhous, J. (1996). Harmonic analysis of a complex motor behavior. Hum. Mov. Sci. 15, 789–807. doi: 10.1016/S0167-9457(96)00023-1
Cordier, P., Mendès-France, M., Bolon, P., and Pailhous, J. (1993). Entropy, degrees of freedom, and free climbing: a thermodynamic study of a complex behavior based on trajectory analysis. Int. J. Sport Psychol. 24, 370–378.
Cordier, P., Mendès-France, M., Bolon, P., and Pailhous, J. (1994a). Thermodynamic study of motor behaviour optimization. Acta Biotheor. 42, 187–201. doi: 10.1007/BF00709490
Cordier, P., Mendès-France, M., Pailhous, J., and Bolon, P. (1994b). Entropy as a global variable of the learning process. Hum. Mov. Sci. 13, 745–763. doi: 10.1016/0167-9457(94)90016-7
Davids, K., Araújo, D., Seifert, L., and Orth, D. (2015). “Expert performance in sport: an ecological dynamics perspective,” in Routledge Handbook of Sport Expertise, eds J. Baker and D. Farrow (London: Routledge), 130–144.
Davids, K., Hristovski, R., Araújo, D., Serre, N. B., Button, C., and Passos, P. (eds.). (2014). Complex Systems in Sport. London: Routledge.
Draper, N., Canalejo, J. C., Fryer, S., Dickson, T., Winter, D., Ellis, G., et al. (2011a). Reporting climbing grades and grouping categories for rock climbing. Isokinet. Exerc. Sci. 19, 273–280. doi: 10.3233/IES-2011-0424
Draper, N., Dickson, T., Fryer, S., and Blackwell, G. (2011b). Performance differences for intermediate rock climbers who successfully and unsuccessfully attempted an indoor sport climbing route. Int. J. Perform. Anal. Sport 11, 450–463. doi: 10.1080/24748668.2011.11868564
Elliott, D., Hansen, S., Grierson, L. E., Lyons, J., Bennett, S. J., and Hayes, S. J. (2010). Goal-directed aiming: two components but multiple processes. Psychol. Bull. 136:1023. doi: 10.1037/a0020958
Fryer, S., Dickson, T., Draper, N., Eltom, M., Stoner, L., and Blackwell, G. (2012). The effect of technique and ability on the VO2–heart rate relationship in rock climbing. Sports Technol. 5, 143–150. doi: 10.1080/19346182.2012.755538
Fuss, F. K., and Niegl, G. (2008). Instrumented climbing holds and performance analysis in sport climbing. Sports Technol. 1, 301–313. doi: 10.1080/19346182.2008.9648487
Fuss, F. K., Weizman, Y., Burr, L., and Niegl, G. (2013). Assessment of grip difficulty of a smart climbing hold with increasing slope and decreasing depth. Sports Technol. 6, 122–129. doi: 10.1080/19346182.2013.854800
Green, A. L., Draper, N., and Helton, W. S. (2014). The impact of fear words in a secondary task on complex motor performance: a dual-task climbing study. Psychol. Res. 78, 557–565. doi: 10.1007/s00426-013-0506-8
Green, A. L., and Helton, W. S. (2011). Dual-task performance during a climbing traverse. Exp. Brain Res. 215, 307–313. doi: 10.1007/s00221-011-2898-2
Guadagnoli, M. A., and Lee, T. D. (2004). Challenge point: a framework for conceptualizing the effects of various practice conditions in motor learning. J. Mot. Behav. 36, 212–224. doi: 10.3200/JMBR.36.2.212-224
Hardy, L., and Hutchinson, A. (2007). Effects of performance anxiety on effort and performance in rock climbing: a test of processing efficiency theory. Anxiety Stress Coping 20, 147–161. doi: 10.1080/10615800701217035
Issurin, V. B. (2013). Training transfer: scientific background and insights for practical application. Sports Med. 43, 675–694. doi: 10.1007/s40279-013-0049-6
Laffaye, G., Collin, J. M., Levernier, G., and Padulo, J. (2014). Upper-limb power test in rock-climbing. Int. J. Sports Med. 35, 670–675. doi: 10.1055/s-0033-1358473
Magiera, A., Roczniok, R., Maszczyk, A., Czuba, M., Kantyka, J., and Kurek, P. (2013). The structure of performance of a sport rock climber. J. Hum. Kinet. 36, 107–117. doi: 10.2478/hukin-2013-0011
Nieuwenhuys, A., Pijpers, J. R., Oudejans, R. R., and Bakker, F. C. (2008). The influence of anxiety on visual attention in climbing. J. Sport Exer. Psychol. 30, 171–185. doi: 10.1123/jsep.30.2.171
Orth, D., Button, C., Davids, K., and Seifert, L. (2017). “What current research tells us about skill acquisition in climbing,” in The Science of Climbing and Mountaineering, eds L. Seifert, P. Wolf, and A. Schweizer (Oxon: Routledge), 196–209.
Orth, D., Davids, K., and Seifert, L. (2014). Hold design supports learning and transfer of climbing fluency. Sports Technol. 7, 159–165. doi: 10.1080/19346182.2014.968167
Orth, D., Davids, K., and Seifert, L. (2016). Coordination in climbing: effect of skill, practice and constraints manipulation. Sports Med. 46, 255–268. doi: 10.1007/s40279-015-0417-5
Pezzulo, G., Barca, L., Bocconi, A. L., and Borghi, A. M. (2010). When affordances climb into your mind: advantages of motor simulation in a memory task performed by novice and expert rock climbers. Brain Cogn. 73, 68–73. doi: 10.1016/j.bandc.2010.03.002
Pijpers, J. R., Oudejans, R. R., and Bakker, F. C. (2005). Anxiety-induced changes in movement behaviour during the execution of a complex whole-body task. Q. J. Exp. Psychol. A 58, 421–445. doi: 10.1080/02724980343000945
Pijpers, J. R., Oudejans, R. R., and Bakker, F. C. (2007). Changes in the perception of action possibilities while climbing to fatigue on a climbing wall. J. Sports Sci. 25, 97–110. doi: 10.1080/02640410600630894
Pijpers, J. R., Oudejans, R. R., Bakker, F. C., and Beek, P. J. (2006). The role of anxiety in perceiving and realizing affordances. Ecol. Psychol. 18, 131–161. doi: 10.1207/s15326969eco1803_1
Pijpers, J. R., Oudejans, R. R., Holsheimer, F., and Bakker, F. C. (2003). Anxiety–performance relationships in climbing: a process-oriented approach. Psychol. Sport Exerc. 4, 283–304. doi: 10.1016/S1469-0292(02)00010-9
Rietveld, E., and Kiverstein, J. (2014). A rich landscape of affordances. Ecol. Psychol. 26, 325–352. doi: 10.1080/10407413.2014.958035
Robert, T., Rouard, A., and Seifert, L. (2013). Biomechanical analysis of the strike motion in ice-climbing activity. Comput. Methods Biomech. Biomed. Engin. 16, 90–92. doi: 10.1080/10255842.2013.815890
Russell, S. D., Zirker, C. A., and Blemker, S. S. (2012). Computer models offer new insights into the mechanics of rock climbing. Sports Technol. 5, 120–131. doi: 10.1080/19346182.2012.749831
Sanchez, X., Boschker, M. S. J., and Llewellyn, D. J. (2010). Pre-performance psychological states and performance in an elite climbing competition. Scand. J. Med. Sci. Sports 20, 356–363. doi: 10.1111/j.1600-0838.2009.00904.x
Sanchez, X., Lambert, P., Jones, G., and Llewellyn, D. J. (2012). Efficacy of pre-ascent climbing route visual inspection in indoor sport climbing. Scand. J. Med. Sci. Sports 22, 67–72. doi: 10.1111/j.1600-0838.2010.01151.x
Schmidt, A., Orth, D., and Seifert, L. (2016). “Collection of visual data in climbing experiments for addressing the role of multi-modal exploration in motor learning efficiency,” in Paper Presented at the In International Conference on Advanced Concepts for Intelligent Vision Systems. (Italy). doi: 10.1007/978-3-319-48680-2_59
Seifert, L., Boulanger, J., Orth, D., and Davids, K. (2015). Environmental design shapes perceptual-motor exploration, learning, and transfer in climbing. Front. Psychol. 6:1819. doi: 10.3389/fpsyg.2015.01819
Seifert, L., Coeurjolly, J. F., Hérault, R., Wattebled, L., and Davids, K. (2013b). Temporal dynamics of inter-limb coordination in ice climbing revealed through change-point analysis of the geodesic mean of circular data. J. Appl. Stat. 40, 2317–2331. doi: 10.1080/02664763.2013.810194
Seifert, L., Dovgalecs, V., Boulanger, J., Orth, D., Hérault, R., and Davids, K. (2014a). Full-body movement pattern recognition in climbing. Sports Technol. 7, 166–173. doi: 10.1080/19346182.2014.968250
Seifert, L., L'Hermette, M., Komar, J., Orth, D., Mell, F., Merriaux, P., et al. (2014d). Pattern recognition in cyclic and discrete skills performance from inertial measurement units. Proc. Eng. 72, 196–201. doi: 10.1016/j.proeng.2014.06.033
Seifert, L., Orth, D., Boulanger, J., Dovgalecs, V., Hérault, R., and Davids, K. (2014b). Climbing skill and complexity of climbing wall design: assessment of jerk as a novel indicator of performance fluency. J. Appl. Biomech. 30, 619–625. doi: 10.1123/jab.2014-0052
Seifert, L., Orth, D., Button, C., Brymer, E., and Davids, K. (2017). “An ecological dynamics framework for the acquisition of perceptual–motor skills in climbing,” in Extreme Sports Medicine, ed F. Feletti (Switzerland: Springer International Publishing), 365–382.
Seifert, L., Orth, D., Herault, R., and Davids, K. (2013c). “Affordances and grasping action variability during rock climbing,” in Studies in Perception and Action: Seventeenth International Conference on Perception and Action, eds T. J. Davis, P. Passos, M. Dicks, and J. A. Weast-Knapp (New York, NY: Psychology Press), 114–118.
Seifert, L., Wattebled, L., Herault, R., Poizat, G., Adé, D., Gal-Petitfaux, N., et al. (2014c). Neurobiological degeneracy and affordance perception support functional intra-individual variability of inter-limb coordination during ice climbing. PLoS ONE 9:e89865. doi: 10.1371/journal.pone.0089865
Seifert, L., Wattebled, L., L'Hermette, M., Bideault, G., Herault, R., and Davids, K. (2013a). Skill transfer, affordances and dexterity in different climbing environments. Hum. Mov. Sci. 32, 1339–1352. doi: 10.1016/j.humov.2013.06.006
Seifert, L., Wattebled, L., L'Hermette, M., and Herault, R. (2011). Inter-limb coordination variability in ice climbers of different skill level. Educ. Phys. Train. Sport 1, 63–68.
Sibella, F., Frosio, I., Schena, F., and Borghese, N. A. (2007). 3D analysis of the body center of mass in rock climbing. Hum. Mov. Sci. 26, 841–852. doi: 10.1016/j.humov.2007.05.008
Smyth, M. M., and Waller, A. (1998). Movement imagery in rock climbing: patterns of interference from visual, spatial and kinaesthetic secondary tasks. Appl. Cogn. Psychol. 12, 145–157. doi: 10.1002/(SICI)1099-0720(199804)12:2<145::AID-ACP505>3.0.CO;2-Z
Tosi, P., Ricci, L., Rosponi, A., and Schena, F. (2011). A theory of energy cost and speed of climbing. AIP Adv. 1:032169. doi: 10.1063/1.3646533
Vigouroux, L., and Quaine, F. (2006). Fingertip force and electromyography of finger flexor muscles during a prolonged intermittent exercise in elite climbers and sedentary individuals. J. Sports Sci. 24, 181–186. doi: 10.1080/02640410500127785
Watts, P. B., Drum, S. N., Kilgas, M. A., and Phillips, K. C. (2016). Geometric entropy for lead vs top-rope rock climbing. Int. J. Exerc. Sci. 9, 168–174.
White, D. J., and Olsen, P. D. (2010). A time motion analysis of bouldering style competitive rock climbing. J. Strength Cond. Res. 24, 1356–1360. doi: 10.1519/JSC.0b013e3181cf75bd
Keywords: affordances, exploration, functional movement variability, rock climbing, motor skill, skill transfer
Citation: Orth D, Kerr G, Davids K and Seifert L (2017) Analysis of Relations between Spatiotemporal Movement Regulation and Performance of Discrete Actions Reveals Functionality in Skilled Climbing. Front. Psychol. 8:1744. doi: 10.3389/fpsyg.2017.01744
Received: 09 April 2017; Accepted: 20 September 2017;
Published: 06 October 2017.
Edited by:
Sergio Machado, Salgado de Oliveira University, BrazilReviewed by:
Bettina E. Bläsing, Bielefeld University, GermanyXavier Sanchez, Halmstad University, Sweden
Copyright © 2017 Orth, Kerr, Davids and Seifert. This is an open-access article distributed under the terms of the Creative Commons Attribution License (CC BY). The use, distribution or reproduction in other forums is permitted, provided the original author(s) or licensor are credited and that the original publication in this journal is cited, in accordance with accepted academic practice. No use, distribution or reproduction is permitted which does not comply with these terms.
*Correspondence: Ludovic Seifert, bHVkb3ZpYy5zZWlmZXJ0QHVuaXYtcm91ZW4uZnI=