- 1Research Center for Education and Mind Sciences, College of Education, National Tsing Hua University, Hsinchu, Taiwan
- 2Department of Educational Psychology and Counseling, College of Education, National Tsing Hua University, Hsinchu, Taiwan
- 3Graduate Institute of Mind, Brain and Consciousness, Taipei Medical University, Taipei, Taiwan
- 4Brain and Consciousness Research Center, Shuang Ho Hospital-Taipei Medical University, Taipei, Taiwan
- 5Department of Education, National Taipei University of Education, Taipei, Taiwan
- 6Department of Biological Science and Technology, National Yang Ming Chiao Tung University, Hsinchu, Taiwan
- 7Center for Intelligent Drug Systems and Smart Bio-devices (IDS2B), National Yang Ming Chiao Tung University, Taipei, Taiwan
- 8Graduate Institute of Behavioral Sciences, Chang Gung University, Taoyuan, Taiwan
- 9Department of Child Psychiatry, Chang Gung Memorial Hospital at Linkou, Taoyuan, Taiwan
- 10Graduate Institute of Biomedical Engineering, Chang Gung University, Taoyuan, Taiwan
- 11Department of Neurology, Chang Gung Memorial Hospital at Linkou, Taoyuan, Taiwan
- 12Institute for Neural Computation and Institute of Engineering in Medicine, University of California, San Diego, San Diego, CA, United States
Objectives: Mindfulness-based stress reduction has been proven to improve mental health and quality of life. This study examined how mindfulness training and various types of mindfulness practices altered brain activity.
Methods: Specifically, the spectral powers of scalp electroencephalography of the mindfulness-based stress reduction (MBSR) group (n=17) who underwent an 8-week MBSR training—including mindful breathing and body-scan—were evaluated and compared with those of the waitlist controls (n=14).
Results: Empirical results indicated that the post-intervention effect of MBSR significantly elevated the resting-state beta powers and reduced resting-state delta powers in both practices; such changes were not observed in the waitlist control. Compared with mindful breathing, body-scanning resulted in an overall decline in electroencephalograms (EEG) spectral powers at both delta and low-gamma bands among trained participants.
Conclusion: Together with our preliminary data of expert mediators, the aforementioned spectral changes were salient after intervention, but mitigated along with expertise. Additionally, after receiving training, the MBSR group’s mindfulness and emotion regulation levels improved significantly, which were correlated with the EEG spectral changes in the theta, alpha, and low-beta bands. The results supported that MBSR might function as a unique internal processing tool that involves increased vigilant capability and induces alterations similar to other cognitive training.
Introduction
For Mindfulness refers to the mental state of being fully open and having attentional and nonjudgmental awareness of one’s internal and external experiences in the present moment (Kabat-Zinn, 1994). At present, mindfulness meditation has attracted global attention because of its benefits to practitioners’ mental health (Brown and Ryan, 2003). Mindfulness practices have been discovered to induce brain structure alterations (Fox et al., 2014), associated with improved working memory and attention (Van den Hurk et al., 2010; Mrazek et al., 2013). Mindfulness meditation can improve a practitioner’s self-regulation capability by increasing positive affect, life satisfaction, and wellbeing (Brown and Ryan, 2003; Garland et al., 2015) and reducing depression, anxiety (Brown and Ryan, 2003; Davidson et al., 2003), stress (Irving et al., 2009), and even insomnia (Goldstein et al., 2019). Among various types of mindfulness interventions, mindfulness-based stress reduction (MBSR) is a standardized and secularized training program designed to improve mindfulness and coping abilities (Kabat-Zinn, 1994). MBSR programs typically span 8weeks of weekly training, include one full-day workshop (Kabat-Zinn, 1994), and involve continuous mindfulness practices, such as mindful breathing, body-scan, and sitting meditation. Studies have demonstrated that after an 8-week training period, MBSR is generally effective in reducing depression and anxiety and promoting mental health (Fjorback et al., 2011). Although abundant evidence supports the role of MBSR in improving subjective perceptions, the brain mechanisms underlying MBSR remain to be investigated.
Neuroscience Evidence of Mindfulness
Mindfulness-based neuroscience studies have generally adopted a longitudinal approach instead of targeting situational practice effects. Objective measures of brain functions, such as electroencephalography (EEG) and functional magnetic resonance imaging (fMRI), have generally been adopted to test the efficacy of mindfulness interventions. For example, in a study of the EEG power of the experienced Rinpoche, with meditation experience of >10,000h, gamma power enhancement was evident even during a resting state (Lutz et al., 2004), and this effect was sustained even during non-rapid-eye-movement (NREM) sleep (Ferrarelli et al., 2013). For meditation novices, EEG measures following an 8-week MBSR program have been widely associated with convergent and consistent outcomes. Researchers have found MBSR practitioners tend to exhibit stronger beta power in the frontal lobe during mindfulness practice than during the resting state (Gao et al., 2016). Similarly, MBSR practitioners exhibited elevated alpha power in the occipital and right temporal lobes (Ahani et al., 2014). Theta band power was reported to increase in the central, parietal, occipital, and left and right temporal lobes after the MBSR intervention by Ahani et al. (2014). Furthermore, MBSR practitioners exhibited lower delta power in the central–parietal area after MBSR intervention (Gao et al., 2016), and patients with chronic insomnia were also found to have lower delta power in the central lobe during NREM sleep after MBSR intervention (Goldstein et al., 2019). Overall, MBSR intervention is generally believed to enhance high-frequency EEG power (i.e., beta and gamma); however, its effect on low-frequency EEG power (i.e., theta and delta) remains uncertain.
Such neurophysiological evidence concerning MBSR is consistent with the evidence on stress reduction and cognitive improvement (Davidson et al., 2003). For example, the beta power in the frontal and temporal lobes of participants without stress stimuli were higher than that of the participants with stress stimuli (Hayashi et al., 2009), suggesting a negative relationship between beta power and stress level. As for the low-frequency bands, healthy adult participants with a high perceived stress level had higher delta and theta activity in the frontal, central, and parietal lobes, compared with those who had a low perceived stress level (Luijcks et al., 2015). Another study on stress revealed that participants exhibited lower theta power under acute stressful conditions (Gärtner et al., 2014). Furthermore, another study highlighted how cognitive tasks elevated gamma power in comparison with the control conditions (Fitzgibbon et al., 2004), and a study on vigilance suggested that highly vigilant states corresponded to delta power suppression (Smallwood and Schooler, 2015). Overall, EEG evidence generally reveals that stress reduction is positively correlated to low-frequency-band power and negatively correlated to high-frequency-band power, whereas cognitive performance and vigilance state are positively correlated to high-frequency-band power and negatively correlated to low-frequency-band power. EEG spectral powers can serve as objective functional markers of cognitive enhancement and stress reduction.
The Difference Between Mindfulness Breathing and Body-Scan
In addition to the post-intervention effect of an 8-week MBSR program, the situational practice effect of such programs has recently attracted the attention of mindfulness researchers seeking to identify the variations between distinct mindfulness practices. The MBSR program involves a series of mindfulness practices (Kabat-Zinn, 1994; Davidson et al., 2003), such as mindful breathing, body-scan, compassion meditation, and open-monitoring. Davidson described the distinct practices associated with various cognitive effects in his book (Goleman and Davidson, 2017). Among the practices, mindful breathing and body-scan were most frequently used in previous studies (Ahani et al., 2014; Wahbeh et al., 2014; Isbel et al., 2019), and both of them are associated with interceptive perceptions. However, the distinction between mindful breathing and body-scan can lead to different interoceptive effects on behavior and brain mechanisms. The fixed attention and relaxation in mindful breathing may differ from the attentional shifts to and from various body parts during body-scan. Recent studies have assessed the diverse effects of mindfulness practices using questionnaires and behavioral measures. One study demonstrated that people who practiced breath-focused meditation had a more nonjudgmental attitude toward themselves, showed more self-compassion, and experienced less emotional regulation difficulty. In contrast, those who practiced body-scan showed increased capabilities to describe their feelings and reduced rumination tendencies (Sauer-Zavala et al., 2013), suggesting that mindful breathing and body-scan affect different brain functions. Some studies have indicated that body-scan yields more positive outcomes for practitioners than does breathing. For example, body-scan practice leads to a major increase in body awareness and a decrease in thought contents, whereas breathing practice engenders a comparatively less intense change (Kok and Singer, 2017). Another study on veterans with post-traumatic stress disorder disclosed that participants who practiced body-scan exhibited greater mindfulness improvement than their breathing group counterparts (Colgan et al., 2016). Studies with self-rating scales have established that body-scan seems to provide more promising benefits than mindful breathing. On the other hand, neuroimaging studies on mindfulness generally examined mindfulness as a self-focused attention process, usually achieved through mindful breathing (Ferrarelli et al., 2013; Matiz et al., 2019). Shifted attention process, or body-scan practice, was rarely addressed before. The brain mechanisms targeted by the two mindfulness practices remain elusive at the current stage of research. Therefore, we adopted EEG measurement to examine the functional distinctions between mindful breathing and body-scan practices.
Working Hypothesis
Collectively, the EEG spectral alterations associated with various mindfulness practices remain elusive, and whether the mindful breathing and body-scan practices take effect are yet to be tested. We first proposed that the functional distinction between these two mindfulness practices lies in the spatio-spectral disparity of the EEG, meaning that frontal power elevation follows body-scan, and parietal power reduction follows mindful breathing after the 8-week MBSR intervention. Second, we proposed that the functional distinction between practices is amplified by the post-intervention effect (not observed in novices). Accordingly, we designed an EEG experiment to assess the neurophysiological changes in terms of both situational mindfulness practice and post-intervention effects. In addition, we conducted the same protocol with a waitlist control group without MBSR training to enable cross-group comparison.
Materials and Methods
Participants
Forty-three volunteers were invited to participate in the MBSR training and register for an 8-week MBSR course in the fall of 2019. Before the course started, all volunteers were required to undergo one of the three orientation sessions to understand the details of the procedure, compensation, potential risks, and the contributions of this study. Potential participants were screened by applying the following exclusion criteria: being outside the age range of 20 to 80years old; having prior experience of mindfulness meditation; having a metabolic illness, any history of mental illness, neural illness, or epilepsy; being a smoker or drug addict; and having any bodily metallic implant, claustrophobia, or pregnancy. Ten people who did not meet the criteria were excluded, and eventually 33 (4 men and 29 women) people aged between 29 and 68years participated in the study (mean=47.46, SD=8.79; 18 participants in the MBSR group and 15 participants in the waitlist control group). The participants were new to mindfulness training before the study, and they received a free 8-week MBSR course for electing to participate. All of the participants provided written informed consent prior to participation. This study was reviewed and approved by the Taipei Medical University Joint Institute Review Board (TMU-JIRB, project number N201905049).
Intervention: MBSR and Waitlist Control
Each participant completed the EEG–fMRI experiments twice. For the MBSR group, two experiments were scheduled before and after the 8-week MBSR training (pre-test and post-test). For the waitlist control group, two scans were performed 8weeks apart (same pre-test and post-test); however, they were prohibited from accessing and receiving information regarding mindfulness practice during this period. The MBSR group participated in an 8-week standardized MBSR course, as proposed by Santorelli et al. (2017). The MBSR course was instructed by a licensed MBSR instructor. MBSR classes were conducted weekly for 2.5h for 8weeks in addition to a one-day mindfulness workshop. These weekly meetings involved the development of various mindfulness skills, dialogue and reflection on mindfulness home practice, and practice segments. The participants performed types of mindfulness practice, such as a sitting meditation entailing breathing, mindful listening, body-scan, and mindful yoga. Participants were also assigned daily homework comprising both formal and informal meditation activities. Formal activities, including body-scan practice, sitting meditation, mindful yoga, mountain/lake meditation, or loving kindness meditation, required 45min to complete each day. The participants were asked to complete a practice and information sheet. The participants in the waitlist control group were instructed to maintain their usual life activities but not engage with any mindfulness-related information during the 8weeks. After the post-test experiment, the waitlist control group participants underwent an MBSR program for compensation.
Experimental Procedure
The study procedure is presented in Figure 1. In the orientation sessions, the study was explained to the participants; in particular, the use of the fMRI machine was detailed, as this study employed the EEG–fMRI simultaneous scanning technique (however, only EEG and behavioral information are included in this report). Thereafter, the participants finished the informed consent form and a questionnaire battery. The questionnaires were the Five Facet Mindfulness Questionnaire (FFMQ; Baer et al., 2008)—Taiwanese version, the Difficulties in Emotion Regulation Scale (DERS; Gratz and Roemer, 2004), and demographic questionnaires. The questionnaire battery took approximately 30min to complete.
After the orientation sessions and within 2months before the MBSR course, the researchers made an appointment for the participants to come into the laboratory for the pre-test experiment. The participants were introduced to the tasks that they would perform during the scanning while an EEG cap was being set up. In the scanning section, participants laid down in the MRI machine to perform four tasks. A mirror setting was installed with projectors for visual presentations. The first task was the resting state (resting 1): Participants were instructed not to think of anything specific with their eyes closed, not to move their heads, and not to fall asleep. The second task was the mindful breathing (breathing): Participants were instructed to pay attention to their natural breath and focus on the sensation near their noses during respiration. Whenever they realized that they were getting distracted, they were instructed to press a button on the right hand. The third task was the body-scan (body-scan): Participants were instructed to perceive the most salient body sensations and shift focus between various body parts during the session. Similar to the breathing task, they were instructed to press a button when they were distracted. Finally, an additional resting state (resting 2), identical to resting 1, was conducted to evaluate whether the brain status was returned to baseline after the mindful practices. Every task lasted 5min, and if participants opened their eyes during a task, they would see a white fixation cross. The fixation image was black with a white cross in the center (see Supplementary Material). We used E-Prime 2.0.10 (Psychology Software Tools, Pittsburgh, PA, United States) for instruction presentation and response recording. After the pre-test experiment, the MBSR group participated in an 8-week MBSR course while the waitlist control group went about their usual life. Within 3months after the completion of the MBSR course, all participants from both groups participated in the post-test experiments that were identical to the pre-test sessions. From pre-test sections to post-test sections, the mean time duration of all participants was 92.56days (SD=20.77days).
It is noted that our task sequence had no counter-balance design. The consideration behind will be discussed in the limitation section.
Questionnaires
The Five Facet Mindfulness Questionnaire
Is a self-reported assessment for measuring mindfulness level (Baer et al., 2008). The FFMQ concerns five aspects of mindfulness, namely, observing, describing, being self-aware, having a nonjudgmental attitude toward inner experience, and being nonreactive to inner experience. Sample questions of the FFMQ are “I notice the smells and aromas of things” (observing), “I am good at finding words to describe my feelings” (describing), “I find myself doing things without paying attention (reversed)” (self-awareness), “I think some of my emotions are bad or inappropriate and I should not feel them (reversed)” (nonjudgmental), and “I perceive my feelings and emotions without having to react to them” (nonreactive). The FFMQ has 39 items, of which 19 are reversed. Items are rated on a 5-point Likert scale, ranging from 1 (never or very rarely true) to 5 (very often or always true), and a total score from 39 to 195 can be obtained after answering the whole questionnaire. The FFMQ has good reliability (α=0.72 to .92; Baer et al., 2008). In this study, we employed a Taiwanese version of the FFMQ that was in Chinese (Huang et al., 2015), and its reliability was also satisfactory (α =0.91 and α =0.96 in the pre-test and post-test, respectively).
The Difficulties in Emotion Regulation Scale
Is a self-reported measurement tool for examining the level of difficulty experienced by people in addressing their negative emotions and producing desirable outcomes (Gratz and Roemer, 2004). The DERS concerns six aspects of emotion regulation difficulties, namely, nonacceptance of emotional responses, difficulties engaging in goal-directed behaviors, impulse control difficulties, lack of emotional awareness, limited access to emotion regulation strategies, and lack of emotional clarity. Sample items are “When I’m upset, I feel guilty for feeling that way” (nonacceptance), “When I’m upset, I have difficulty getting work done” (goals), “When I’m upset, I lose control over my behaviors” (impulse), “I pay attention to how I feel (reversed)” (awareness), “When I’m upset, I believe that I will remain that way for a long time” (strategies), and “I am confused about how I feel” (clarity). The DERS has 36 items, of which 11 are reversed. Items are rated on a 5-point Likert scale, ranging from 1 (almost never) to 5 (almost always), and the total score obtained ranges from 36 to 180. The DERS has good reliability (α=0.80 to .89). This study employed a Taiwanese version of the DERS that was translated into Chinese through the back-translation procedure, and the reliability analysis yielded α=0.96 and α=0.95 for the pre-test and post-test, respectively.
EEG Measurement and Analysis
In each experiment, simultaneous EEG–fMRI signals were recorded for each functional scan using a 3T PRISMA MRI scanner (Siemens, Erlangen, Germany). The 32-channel EEG data were recorded using an MR-compatible system (Brain Products GmbH, Gilching, Germany) that was positioned according to the international 10/20 system. The built-in impedance in each electrode was 5 kΩ, and abrasive electrode paste (Abralyt HiCl) was used to reduce the electrode–skin impedance. The EEG signal was recorded synchronously with the MR trigger using Brain Vision Recorder (Brain Products) with a 5k-Hz sampling rate and a 0.5μv voltage resolution (reference at FCz). A band-pass filter was set with cutoff frequencies of 250Hz and 0.0159Hz, and an additional 60-Hz notch filter was employed. Here, we have reported only the EEG outcomes, as the MRI results were designed to be reported separately.
Recorded EEG data were re-sampled to 50,000Hz and then corrected for gradient-induced artifacts as Becker et al. (2005) suggested. Ballistocardiographic artifacts were corrected using the adaptive average subtraction method, and the R-peak intervals were estimated from the electrocardiogram electrode through Analyzer 2.1 (Brain Products) after the data were down-sampled to 250Hz. The EEG data were then filtered with a 0.2–40Hz band-pass FIR filter. Thereafter, an independent component analysis (ICA, with Infomax method) to eliminate artifacts caused by electrooculogram (EOG), electromyogram (EMG), and electrocardiogram (ECG) artifacts and the remaining MRI-induced artifacts. The artifact-free data were referenced to an average electrode across whole scalps, as recommended by Allen et al. (2004) and Davidson (1998). The processed data were converted into frequency domain representations using short-time Fourier transformation and Welch’s periodogram method. Specifically, each 5-min EEG signal (75,000 points) was divided into 256-point segments using the Hanning window with 128 points overlapped. Each segment was zero-padded to 512 points, followed by a 512-point fast Fourier transformation. All the resultant spectra were subsequently log-transformed (10log10, results in dB; Allen et al., 2004) and averaged over segments. Finally, average EEG band powers were calculated in the frequency ranges of 1–4Hz, 4–8Hz, 8–13Hz, 13–20Hz, 20–30Hz, and 30–40Hz, representing delta, theta, alpha, low-beta, high-beta, and low-gamma band powers, respectively (Deuschl and Eisen, 1999; Rangaswamy et al., 2002; Teplan, 2002; Hauswald et al., 2015; Abhang et al., 2016; Grent-'t-Jong et al., 2018). These signal processes were conducted using the EEGLAB toolbox 13.6.5b (Swartz Center for Computational Neuroscience, University of California San Diego; Delorme and Makeig, 2004) with Matlab R2019a. The calculation of power spectrum density (PSD; in “dB”) also followed the suggestion of Delorme and Makeig (2004). This study filtered out frequencies above 40Hz since MR gradient-induced artifacts would severely contaminate the spectra over 40Hz (Solana et al., 2014). Therefore, the EEG band of highest frequency in this study was low-gamma band (30–40Hz).
Statistical Analysis
To ensure that all parameters (EEG band power and FFMQ and DERS scores) were fitted for parametric analyses, Kolmogorov–Smirnov tests were performed to examine normality. The chi-square test and t-test were conducted to examine whether demographic features, FFMQ, and DERS levels were different between the MBSR group and the waitlist control group before the MBSR intervention. Given that our working hypothesis is particularly to examine the effects of MBSR intervention, multiple paired t-tests were performed to examine whether a significant difference existed in terms of FFMQ and DERS in both groups before and after the MBSR intervention.
To examine whether EEG power spectra changed before and after the MBSR intervention, a paired-sample t-test was performed for every 0.5-Hz frequency bin in all four tasks (resting 1, breathing and body-scan, resting 2) in both groups and for both channels, Fz and Pz. In order to examine individual variations between EEG activities and behavioral measures, correlation analysis was then performed to reveal whether a correlation existed between the change of EEG band power before and after the MBSR intervention and the change of FFMQ and DERS scores in both groups. The change of EEG power was calculated by subtracting the pre-test EEG band power from the post-test EEG band power (post-test–pre-test), and the change in FFMQ and DERS scores was calculated similarly.
Results
Normality Test
Table 1 presents the mean FFMQ and DERS scores rated by both the MBSR and control groups during the pre-test and post-test sessions. The MBSR group (N=18) scored 114.94±16.99 on the FFMQpre, 142.56±23.24 on the FFMQpost, 97.78±20.72 on the DERSpre, and 84.72±23.21 on the DERSpost. The control group (N=15) scored 116.80±16.45 on the FFMQpre, 115.87±14.65 on the FFMQpost, 84.87±22.53 on the DERSpre, and 98.80±16.12 on the DERSpost. All these scores validated the assumption of normality (Kolmogorov–Smirnov test: ps>0.05). In terms of resting-state EEG activity, the logarithmic powers of the delta, theta, alpha, low-beta, high-beta, and low-gamma bands over channels Fz and Pz recorded in the two sessions for both groups also validated the assumption of normality (Kolmogorov–Smirnov test: ps>0.05). The only exception was the post-test low-gamma score for channel Pz in the waitlist control group (p=0.032). Because our analysis focused on the main effects of the MBSR intervention group, parametric tests were employed in the subsequent analyses.
Demographic and Behavioral Analysis
Thirty-three participants (29 women and 4 men, aged 47.67±8.79years) were recruited in this study, of whom 15 (12 women and 3 men, aged 46.67±8.03years) served as the control group and 18 (17 women and 1 man, aged 48.50±9.52years) served as the MBSR group. As evident in the demographic data and questionnaire results presented in Table 1, no significant difference was apparent in terms of gender (χ2 [1]=1.60, p=0.206), age (t [31]=0.59, p=0.559), and educational level (t [31]=−0.90, p=0.377) between the waitlist control and MBSR groups.
A between-group analysis showed that the differences between the two groups in both questionnaire responses were significant in the post-test session (FFMQ: t [31]=5.32, p<0.001; DERS: t [31]=−2.08, p=0.046) but not in the pre-test session (FFMQ: t [31]=−0.32, p=0.753; DERS: t [31]=0.39, p=0.702). A within-group analysis showed that after the MBSR intervention, the MBSR group exhibited a significant increase in the FFMQ score (t [17]=5.32, p<0.001) and a decrease in the DERS score (t [17]=−2.14, p=0.047) from the scores before the intervention, whereas the FFMQ (t [14]=−0.27, p=0.796) and DERS (t [14]=0.76, p=0.458) scores of the waitlist control group remained unchanged.
EEG Comparison Between Pre-test and Post-test Sessions
After an 8-week mindfulness training, the effect of mindfulness practices on resting-state EEG activity was examined. Figures 2, 3 illustrate the comparisons of PSD between the pre-test and post-test for Fz and Pz electrodes, respectively; the black asterisks indicate the significant differences in EEG powers identified at certain frequency bins. Student’s t-test was applied for every 0.5-Hz frequency bin ranging from 0Hz to 40Hz (0–0.2Hz was filtered out during preprocessing), followed by false discovery rate correction for conducting multiple comparisons across frequency bins and channels (Keselman et al., 2002).
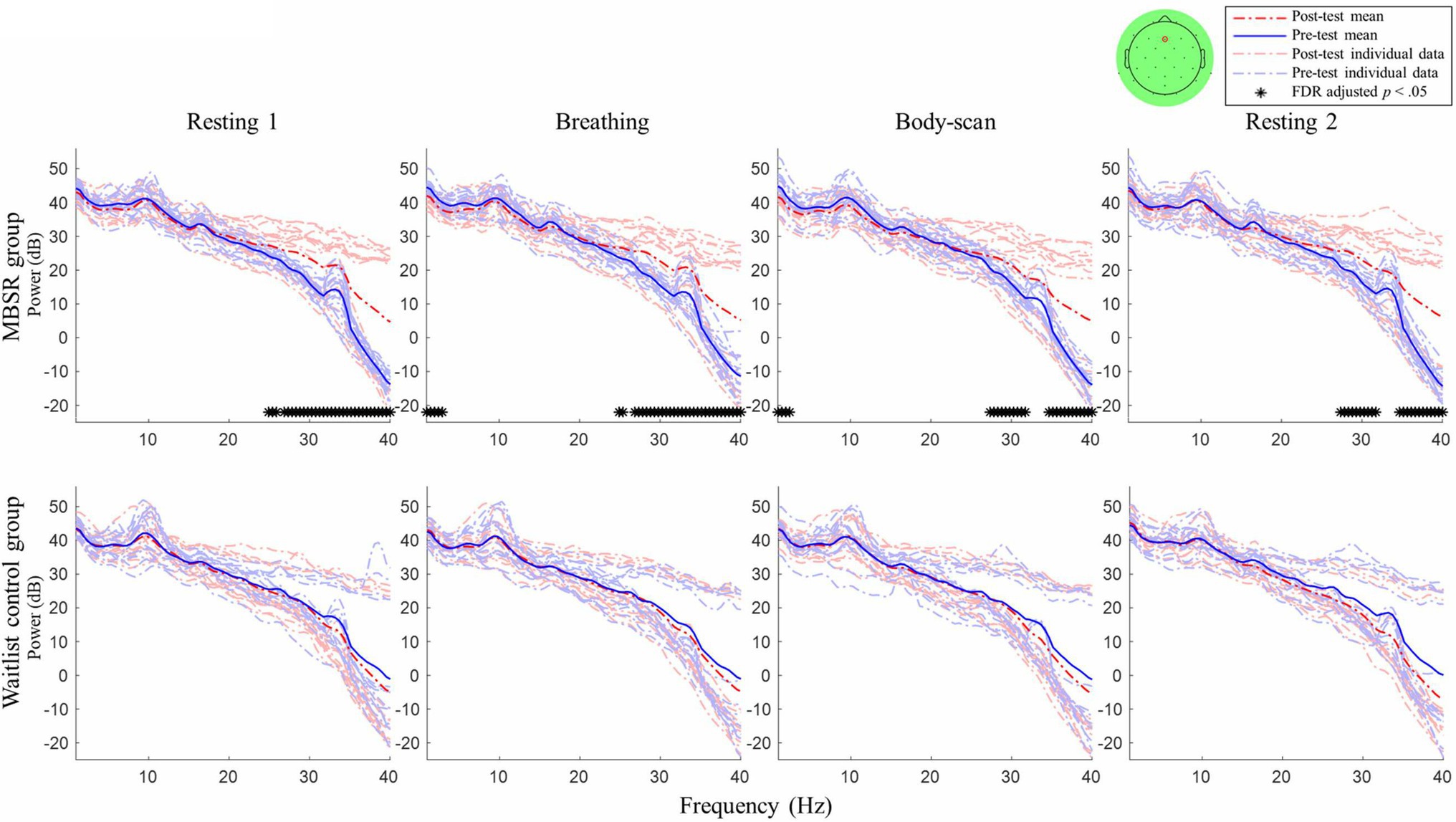
Figure 2. Post–pre-test PSD t-test for channel Fz between the mindfulness-based stress reduction (MBSR) group and waitlist control. MBSR group n=17, waitlist control group n=14.
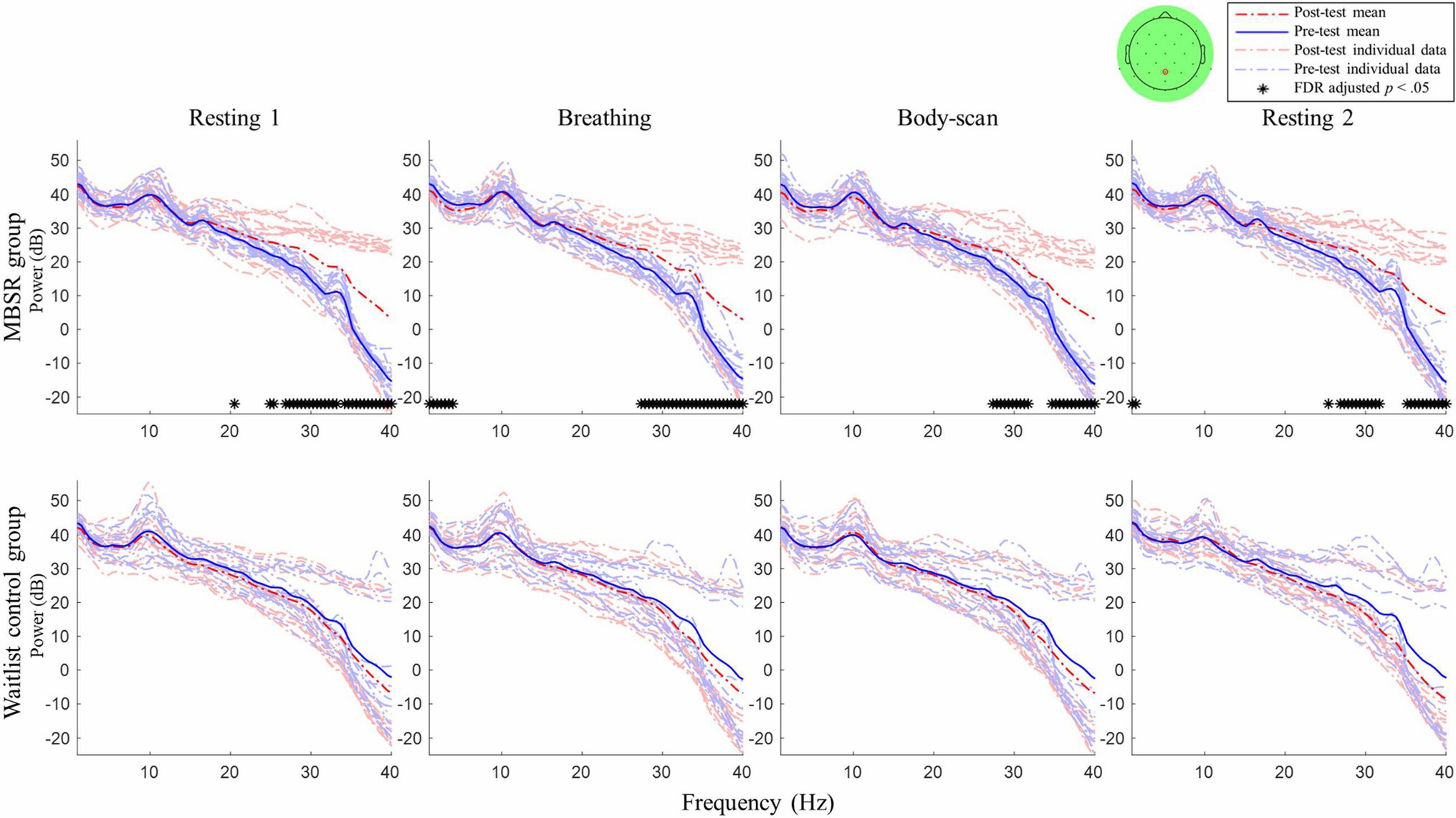
Figure 3. Post–pre-test PSD t-test for channel Pz between the MBSR group and waitlist control. MBSR group n=17, waitlist control group n=14.
For the MBSR group, compared with the pre-test session, the EEG activity of Fz and Pz sites measured in the post-test session revealed the following: (1) in resting 1, high-beta and low-gamma powers significantly increased (Fz: 24.5–40Hz except 26Hz, ts≥2.56, ps<0.05; Pz: 20–20.5Hz and 24.5–40Hz, except 25.5, 26, and 35.5Hz, ts≥2.41, ps<0.05); (2) in breathing, high-beta and low-gamma powers significantly increased (Fz: 24.5–40Hz except 25.5–26Hz, ts≥2.31, ps<0.05; Pz: 27–40Hz, ts≥2.70, ps<0.05) and delta powers significantly decreased (Fz: 0.2–2Hz, ts≥−2.72, ps<0.05; Pz: 0.2–3Hz, ts≥−2.61, ps<0.05); (3) in body-scan, high-beta and low-gamma powers significantly increased (Fz: 27–31.5Hz, ts≥2.83, ps<0.05; 34.5–40Hz, ts≥2.74, ps<0.05; Pz: 27–31.5Hz, ts≥3.05, ps<0.05; 34.5–40Hz, ts≥2.94, ps<0.05) and delta power significantly decreased only at Fz (0.2–1.5Hz, ts≥−2.76, ps<0.05); and (4) in resting 2, high-beta and low-gamma powers significantly increased (Fz: 27–31.5Hz, ts≥2.80, ps<0.05; 34.5–40Hz, ts≥2.85, ps<0.05; Pz: 25–31.5Hz except 25.5 and 26Hz, ts≥2.99, ps<0.05; 35–40Hz, ts≥3.56, ps<0.05) and delta powers significantly decreased only at Pz (0.2–0.5Hz, ts≥−2.80, ps<0.05). For the waitlist control group, no significant difference was observed in EEG power between the post-test and pre-test sessions in all four tasks (lower panel of Figures 2, 3, ps>0.05).
Figure 4 presents the spatial distribution of the EEG spectral power differences between the post-test and pre-test of the MBSR group in the four tasks. Paired-sample t-tests were applied to all channels to compare the difference between the post-test and pre-test conditions. The channel locations that do not exhibit significant differences (ps>0.05) were marked as zero difference and depicted in green in the topoplots. The results showed that the high-beta and low-gamma bands had significant spectral differences across the whole scalp in resting 1 and resting 2. There were also some small-scale differences in the delta and low-beta bands in the lateral frontal, left parietal, and right temporal areas in resting 1 and resting 2. The delta band had significant EEG power differences in the frontal and parietal areas, and the high-beta and low-gamma bands had significant power differences across the whole scalp in the breathing and body-scan tasks. There were also significant differences in the theta band in the frontal and occipital areas in the breathing and body-scan tasks. For the breathing task, there are small-scale differences in the alpha and low-beta bands in the right temporal area. For the body-scan task, there is a small-scale difference in the alpha in the right temporal area, and a frontal power asymmetry (right>left) in the low-beta band.
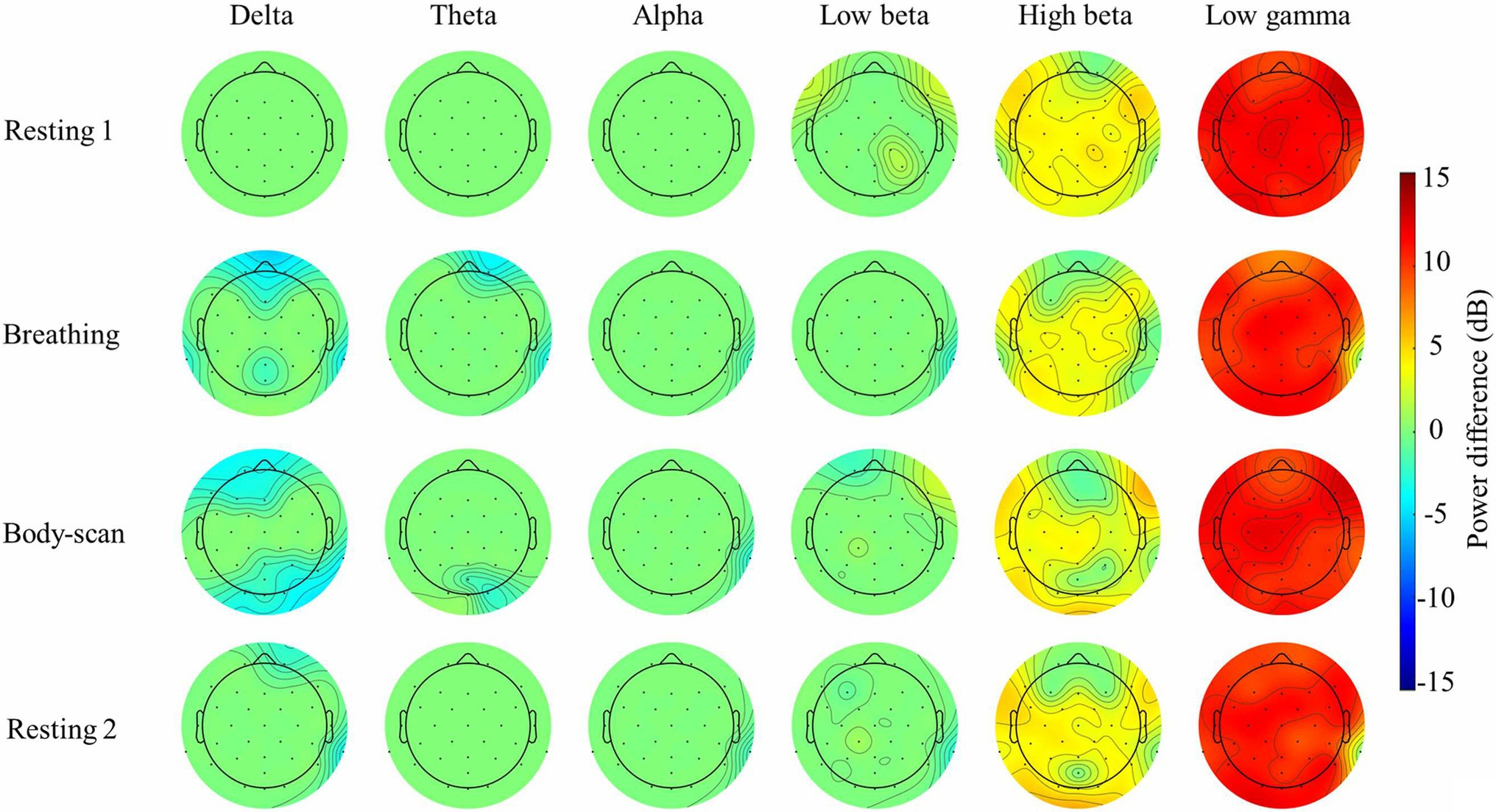
Figure 4. Topoplot analysis of the Electroencephalograms (EEG) spectral power of the MSBR group in the breathing and body-scan tasks, and the paired t-test comparison between post-test and pre-test. Power difference = Post-test–pre-test power. Channel locations with zero power differences represent no significant difference (paired-sample t-test, FDR adjusted ps>0.05, depicted in green)
EEG Correlates of Mindfulness Practice
This study further investigated the momentary state effect of acquired mindfulness skills by examining the EEG activity. To this end, the EEG powers during breathing and body-scan were referenced to that during resting 1. Figure 5 shows the spectral comparisons of breathing, body-scan, and resting 2 in the post-test session. Compared with resting 1, the powers of delta, low-beta, high-beta, and low-gamma bands significantly decreased during body-scan at both Fz (0.2–2.5Hz, ts≥−2.55, ps<0.05; 15–17.5Hz, ts≥−2.92, ps<0.05; 19.5–20.5Hz, ts≥−2.59, ps<0.05; 23.5–24.5Hz, ts≥−2.54, ps<0.05; 28.5–37Hz, ts≥−2.59, ps<0.05) and Pz (0.2–4Hz, ts≥−3.08, ps<0.05; 13–16.5Hz, ts≥−3.00, ps<0.05; 29.5–37.5Hz, ts≥−2.44, ps<0.05). However, no significant power change was found in breathing and resting 2 (ps>0.05). The EEG power during body-scan was also referenced to the power during breathing, and no significant difference was found (ps>0.05). For the waitlist control group that did not engage in any mindfulness practice, no significant differences were found in the EEG power between every task pair (ps>0.05).
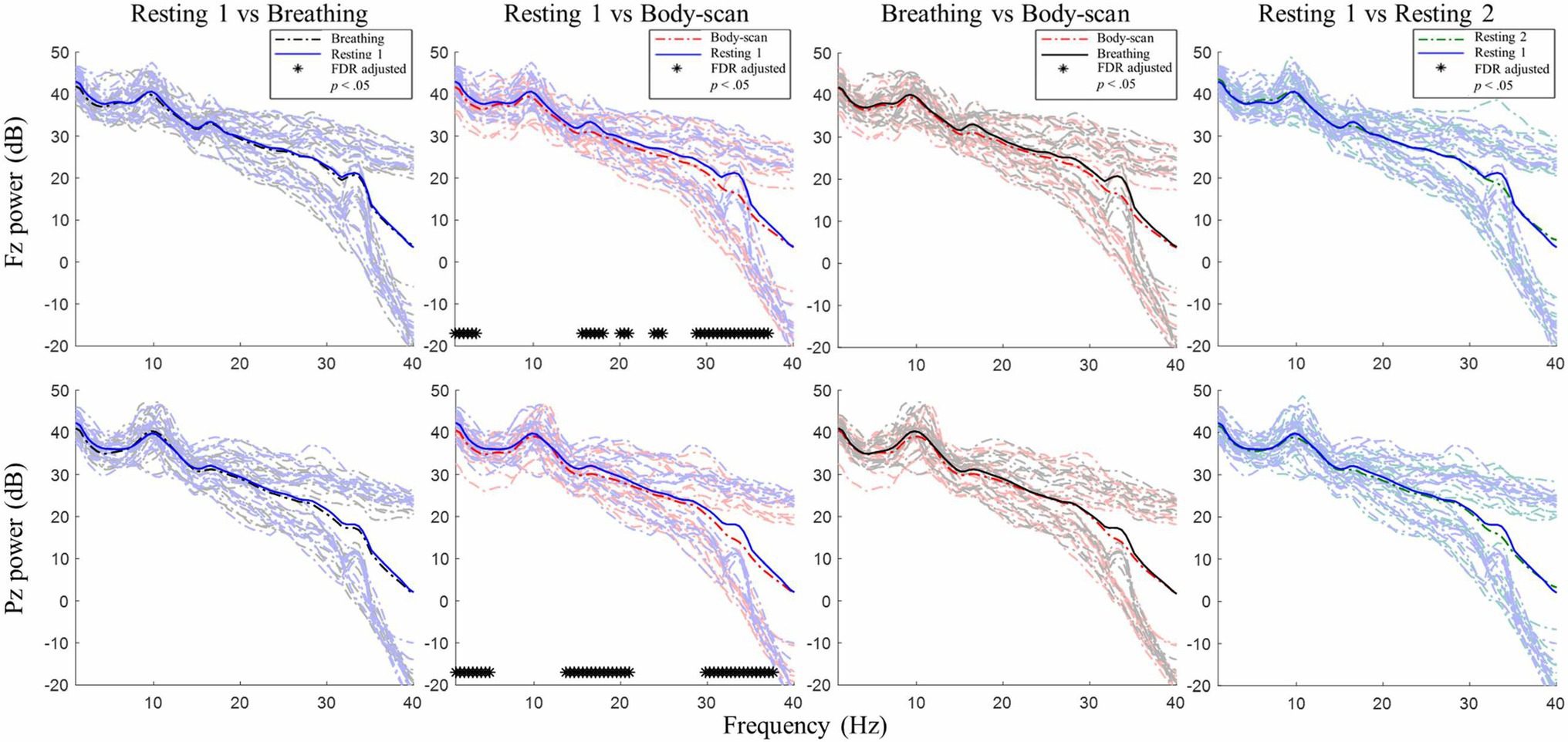
Figure 5. Post-test PSD t-test among the four tasks in the MBSR group. n =18. No significant difference was found in the post-test for the waitlist control group. No significant difference was found in the pre-test for either group.
EEG Correlates of Mindfulness and Behavioral Measures
Correlation analysis was performed to examine the potential correlations between EEG activities and behavioral outcomes. Table 2 presents the correlation coefficients between the post-test–pre-test differences of EEG power in the four tasks and those in the behavioral measures. For the MBSR group, Δlow-beta power in resting 1 for the Pz site was found to have a negative correlation with ΔDERS (b=−0.50, p=0.040), Δtheta in breathing for the Pz site had a marginally significant negative correlation with ΔFFMQ (b=−0.48, p=0.053), Δlow-beta in body-scan for the Fz site had a marginally significant negative correlation with ΔFFMQ (b=−0.48, p=0.054), Δalpha in resting 2 for both Fz (b=−0.64, p=0.006) and Pz sites (b=−0.51, p=0.036) showed a negative correlation with ΔFFMQ, and Δtheta in resting 2 for the Pz site had a marginally significant negative correlation with ΔFFMQ (b=−0.47, p=0.058).
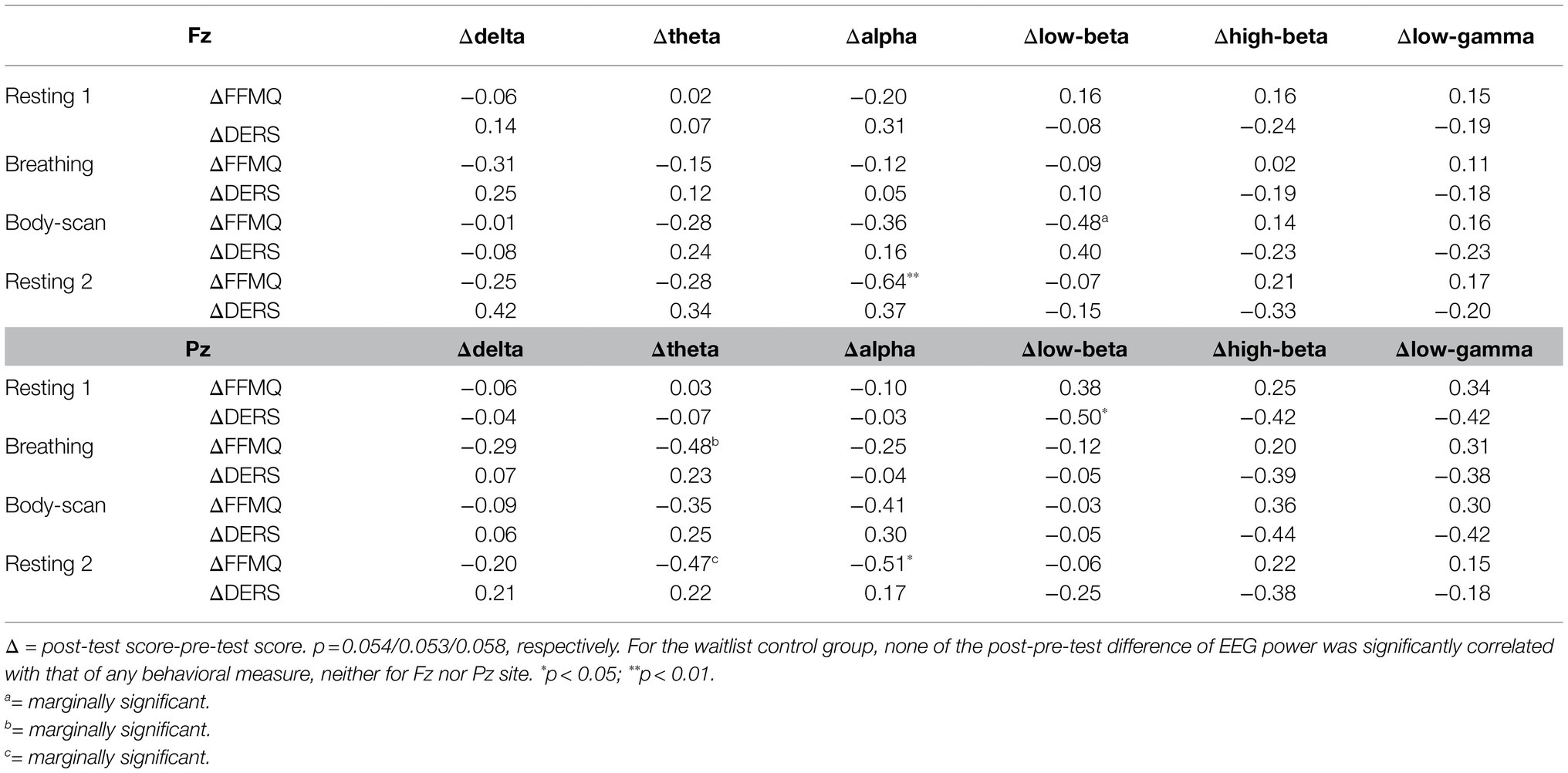
Table 2. Correlation coefficients among the changes of EEG wave bands and behavioral measures in MBSR group.
The correlation coefficients between the changes in the EEG band power and behavioral subscales in the MBSR group are shown in Supplementary Material (Supplementary Tables 2, 3). With regard to FFMQ subscales, alpha and theta spectral changes were significantly correlated with observing, describing during resting 1, and nonreactivity subscales during resting 2 in both Fz and Pz (ps<0.05). In the DERS subscales, delta spectral changes in Fz were significantly associated with goals (p=0.037) during resting 2, whereas low-beta and high-beta spectral changes in Pz were significantly associated with awareness and clarity during resting 1 (ps<0.05), respectively.
Discussion
Summary of Results
Table 1 presents the MBSR intervention effects on the FFMQ and DERS scores, indicating that participants demonstrated augmented levels of trait mindfulness and emotional regulation. The EEG spectral results (Figures 2, 3) showed that the 8-week intervention led to an increase in high-frequency EEG activities across all conditions and a decrease in low-frequency EEG activities during the breathing and body-scan practices. As observed in the MBSR group at the follow-up visit in week 8 (Figure 5), body-scan suppressed EEG power across all frequencies. Additionally, the mindfulness-induced changes in theta, alpha, and low-beta band powers significantly correlated with the changes in FFMQ or DERS scores.
Intervention Effect of MBSR
The differences between the post-test and pre-test results in resting 1 indicated the post-intervention effect of the MBSR practice on EEG activity. Previous studies have reported that relaxation exercises induced decreased beta and gamma band power (Stinson and Arthur, 2013) and increased theta band power (Field et al., 2010). The current study’s EEG results and previous research on MBSR (Lutz et al., 2004; Cahn et al., 2010) have demonstrated that, unlike relaxation exercises, mindfulness practices yield increased high-frequency EEG activity. This post-intervention neuro-electrical change was sustained in our study irrespective of which mindfulness task the participants engaged in. In the MBSR group, the post–pre-test EEG power comparisons of resting 1, breathing, body-scan, and resting 2 revealed the same pattern, namely, elevated high-frequency (high-beta and low-gamma) EEG power in the post-test EEG scan. The results further supported our argument that the increase in high-frequency EEG power is a post-intervention effect of mindfulness practice. Although mindfulness practice is known to provide the same relaxation effect as other relaxation exercises (Davidson et al., 2003), this study suggested that mindfulness practice differs from relaxation exercises.
The results of mindfulness practice were rather similar to the EEG findings on beta and gamma neurofeedback training (a training aimed at improving beta or gamma power) that showed that 10days of beta and gamma neurofeedback training led to improved episodic memory among healthy adults (Keizer et al., 2010). A similar association between long-term cognitive training and gamma power elevation was also found among the elderly population (Staufenbiel et al., 2014) and patients with Alzheimer’s disease (Van Deursen et al., 2008), schizophrenia (Molina et al., 2020), and attention-deficit/hyperactivity disorder (Yordanova et al., 2001). Furthermore, the results were consistent with the neuroimaging findings that highly focused participants with 6weeks of mindfulness training showed better cognitive performance in the Stroop test, in addition to exhibiting higher dorsolateral prefrontal cortex (dlPFC) activation (Allen et al., 2012). With results showing elevated high-frequency EEG power, mindfulness practice is likely similar in effect to cognitive training.
After the MBSR intervention, the low-frequency (delta) activity at Fz decreased during breathing and body-scan (Figure 2) and that at Pz decreased during breathing (Figure 3). Previous studies on different mindfulness styles (i.e., Vipassana and Qigong) have suggested that compared with non-meditators, practitioners’ delta activity increased in the prefrontal area during the resting state (Tei et al., 2009; Cahn et al., 2010). However, another study on MBSR reported that practitioners’ frontal delta power decreased (Gao et al., 2016), indicating that different mindfulness practices may lead to different EEG results. Our data supported the results of Gao et al. (2016) that MBSR practitioners’ low-frequency EEG power decreases and high-frequency EEG power increases. The current study further suggested that such EEG power changes could be introduced with 8weeks of training.
Some have argued that the delta oscillation of EEG is related to mental task performance because delta oscillation represents people’s attention to their internal process (Harmony et al., 1996; Harmony, 2013). Knyazev (2007) further suggested that delta and alpha oscillations in the prefrontal area may contribute to a reciprocal inhibitory mechanism that can manipulate people’s motivation and attention and moderate people’s mental task performance. Harmony (2013) suggested that the increased delta oscillation in mindfulness practitioners represents the inhibition of the prefrontal cortex in addition to the reduction of emotional and cognitive engagement. MBSR practitioners, however, are instructed to not inhibit their emotions or cognition but to accept and merely observe their inner thoughts just as they are (Davidson et al., 2003). This practice of acceptance may explain the current result that delta power decreased during mindfulness practice after 8weeks of training. This argument is further legitimized by the result that only the MBSR-trained participants, and not the waitlist control group, demonstrated the delta power drop. This is because attentional yet nonjudgmental acceptance of oneself requires continuous and effort-intensive practices to master.
Additionally, this delta power suppression suggested that the practices might involve a stage of high vigilance (Smallwood and Schooler, 2015). A previous study on mind-wandering (Braboszcz and Delorme, 2011) showed that when people were distracted from a task and started mind-wandering, their delta and theta power increased, whereas their alpha and beta power dropped. The EEG results of mind-wandering were exactly the opposite of our mindfulness results of decreased delta power. The contradictory EEG results support the argument that mindfulness is a process of disciplining the mind and stopping mind-wandering (mindlessness; Davidson et al., 2003; Mrazek et al., 2012). Furthermore, another study suggested that mindfulness programs can reduce mind-wandering episodes (Schooler et al., 2014). This claim is supported by the results that participating in 8weeks of mindfulness training promotes working memory capacity, which is the key to maintaining focus in a cognitively demanding and vigilance-requiring situation (Jha et al., 2010). Even 8min of mindful breathing was found to reduce people’s attentional error in a vigilance task (Mrazek et al., 2012). Therefore, EEG spectral whitening (low-frequency power drop and high-frequency power elevation) represents a mental state with less mind-wandering and higher mindfulness.
Previous neuroimaging studies on mindfulness have suggested frontal and parietal areas are mostly related to mindfulness-induced neuro changes. For example, an fMRI study on long-term mindfulness practitioners reported the increased functional connectivity among the (PCC; Brewer et al., 2011). A similar finding was also reported in the post-MBSR intervention participants (Kral et al., 2019). Meanwhile, the fMRI data collected in this study showed that the activation difference between breathing and body-scan in the pre-test was notable in frontal and parietal spatial distribution (Guu et al., 2020). The current EEG data agreed with the previous fMRI results that the changes in mindfulness-induced neuro-activation were evident in the frontal and parietal areas (Figure 4). The results in Figure 4 further suggested that some other brain areas, including the occipital and temporal areas, also exhibit comparable spectral changes. Also, there was a frontal power asymmetry in the low-beta band in body-scan. We expect that further studies on the frontal EEG power asymmetry will be legitimate in the exploration of mindfulness (also see Isbel et al., 2019).
It is noted that four participants in the MBSR group did not show EEG power difference between the post-test and pre-test, even though the general group power elevation was significant (see Figures 2, 3). The four participants reported higher FFMQ (mean difference=24.25, SD=12.37; group mean difference=27.61, SD=22.02) and half reported lower DERS (mean difference=−5.25, SD=26.54; group mean difference=−13.06, SD=25.87). Although both the FFMQ and DERS levels of the four participants changed in the manner intended by the MBSR intervention, the magnitude of these changes was lower than that of the MBSR group average. A potential explanation for this phenomenon was that the MBSR intervention was not effective in some of the participants, and therefore, no neurophysiological change was introduced. The same phenomenon was also found in a previous mindfulness study on the bereaved individuals (Huang et al., 2021) that some of them had their mindfulness levels decreased after the 8-week mindfulness intervention. The phenomenon suggested that mindfulness training did not guarantee success for everyone. Further studies are required to figure out the reason underneath to improve the efficacy of the mindfulness training courses.
Situational Practice Effect After the 8-Week Training
Another focus of this study was to examine whether different mindfulness practices contribute to participants’ neuro-electrical responses differently. Compared with resting 1, the MBSR-trained participants showed significantly decreased EEG activities across delta, low-beta, and parts of high-beta and low-gamma bands at both Fz and Pz when practicing body-scan. During the body-scan practice, practitioners were instructed to be aware of their introspective body sensations. As suggested in a previous study (Mirams et al., 2013), body-scan exercise can improve people’s somatic signal detection clarity. The EEG changes associated with the body-scan task found in this study reflected how the body-scan exercise is specific and concrete for practitioners. By contrast, no significant power difference was found when practicing mindful breathing (Figure 5), suggesting that body-scan leads to more significant EEG change among the participants than breathing.
Notably, the preliminary data collected from five mindfulness practitioners with more than 2years of MBSR experience (i.e., the expert group; Figure 6) further revealed that experts’ EEG power was consistently lower than that of the MBSR practitioners with 8weeks of experience, irrespective of whether they were resting, practicing mindful breathing, or body-scan practice. In general, the high-frequency power of the MBSR practitioners elevated and the low-frequency power decreased after the 8-week MBSR training, but all EEG power decreased along with the expertise of mindfulness. Although the expert group had few participants, a general drop in EEG power across low-, middle-, and high-frequency bands could be a neuro-electrical feature of long-term MBSR practice.
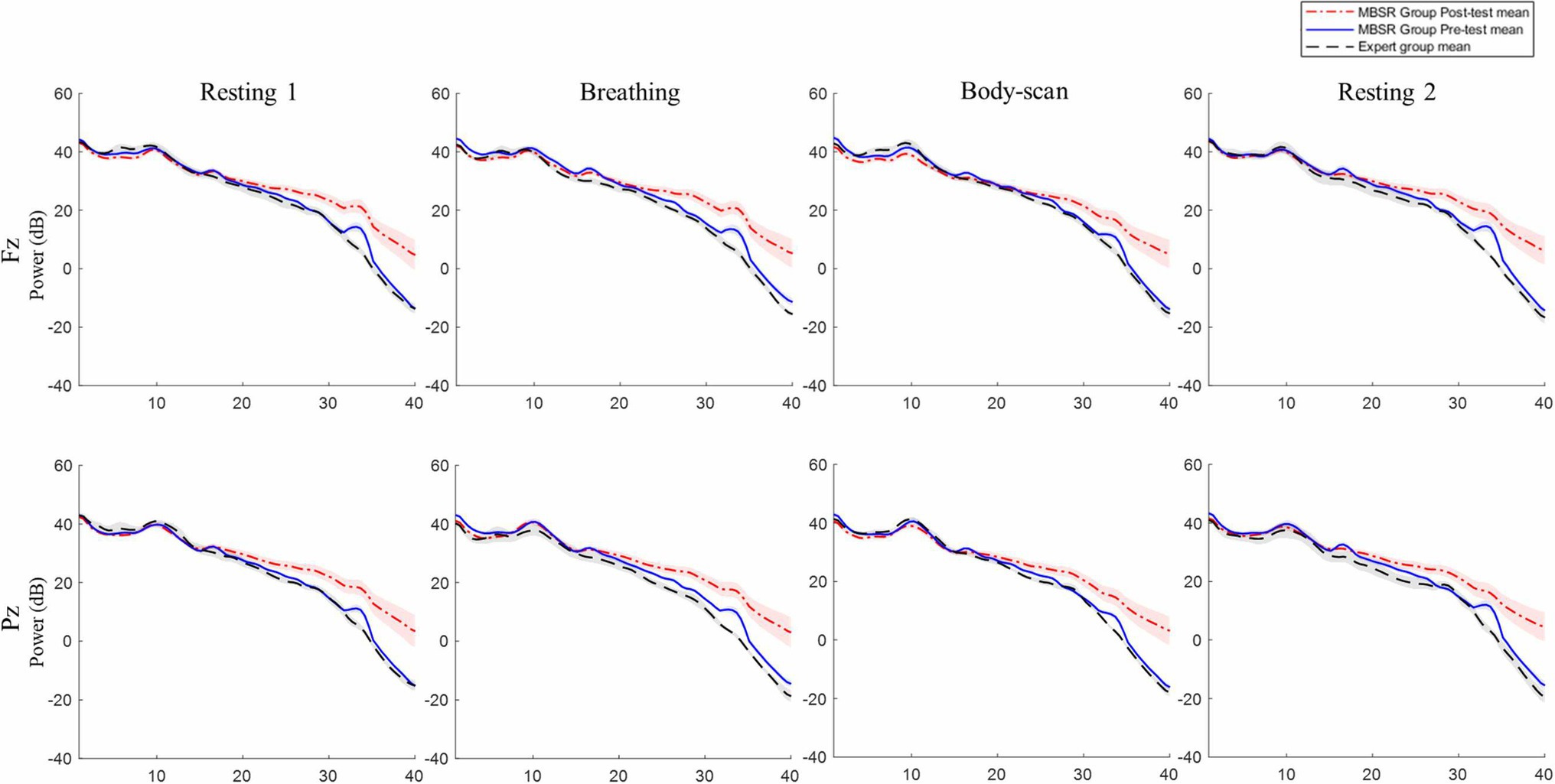
Figure 6. MBSR group and expert EEG power comparison. MBSR group n=17, expert group n=5. Shading represents standard error of mean. No significant difference was found between expert group and the MBSR group.
Correlations Between the Change of EEG and Behavioral Indexes
The differences in the theta, alpha, and low-beta band powers between the post-test and pre-test were correlated with the change in the FFMQ or DERS scores (as shown in Table 2). In our major findings concerning behavior and EEG biomarkers, we found significant correlations between behavioral indexes and theta, alpha, and low-beta power. These results were consistent with Ahani et al.’ (2014) finding that improvement in mindfulness levels was characterized by increases in the theta, alpha, and beta activities. Another study on emotional regulation also highlighted that frontal bilateral alpha activity and the parietal delta/beta ratio predicted people’s spontaneous emotion regulation level (Tortella-Feliu et al., 2014). This leads to the following question: Why was a major EEG power difference observed in high- and low-frequency bands in our data despite the clear correlation between EEG power and behavioral indexes observed in the theta, alpha, and beta bands? A possible reason could be that the major differences in neurophysiological changes after 8-weeks of mindfulness practice can be observed through delta, high-beta, and low-gamma spectral power and that the biomarker of mindfulness is found in alpha. A previous study on experienced Zen meditators showed that the hours of meditation practice and weekly frequency were negatively correlated with alpha power but not gamma power (Pasquini et al., 2015). Our results further suggest that mindfulness and its positive outcomes involve a complex mechanism. The EEG signals represent the mixtures of these contributing factors. Future EEG biomarker studies on mindfulness may also include the factor of cognitive ability to clarify such a complex mechanism.
Limitation
It is noted that the task sequence was suggested by licensed and experienced MBSR instructors. Even though we are well aware that counter-balance is valuable in terms of causal interpretation, we treasure the ecological validity of mindfulness practice. In MBSR training, practitioners start learning mindfulness from breathing, then advance to body-scan afterward. It is unlikely that practitioners can practice body-scan without settling their mind with breathing first. In order to ensure that the experimental conditions were faithful to natural MBSR practice as much as possible, the participants were always asked to practice breathing and then body-scan in both scanning sessions.
There may also be some concern about the relatively small sample size of this study. We admitted that this can be a shortcoming of this study, and the major difficulty of data collection came from the limited MRI scanning sessions we can enrolled. We argued that our results are convincing because the results are still significant with small sample size, and the trends are consistent with our predictions based on the previous works on mindfulness.
Another limitation of this study is the frequency range of EEG data. Due to the contamination of MR gradient-induced artifacts, the current study examined the EEG data only up to 40Hz, leading not to make a fully compatible interpretation between the current results and the other studies involving high-gamma activity. The following studies on this topic may examine mindfulness practitioners’ EEG signals outside of an MRI scanner to address this limitation.
We were aware that there is a psychometric discussion on the diverse nature of mindfulness assessment (Grossman, 2011). Although FFMQ is not the one and only one mindfulness assessment developed, FFMQ is one of the most used and sophisticated mindfulness assessments (Carpenter et al., 2019). Especially, the validity of FFMQ on the assessment of MBSR intervention outcomes has wildly been supported (Lamothe et al., 2016; Vibe et al., 2017). Still, further studies may examine the validity of other mindfulness questionnaires on MSBR outcomes and the correlation differences between the questionnaires and EEG band powers.
The current result is only a glimpse of the neuro-evidence of mindfulness practitioners. Other perspectives and evidence, including EEG connectivity analysis and MRI joint analysis, can be used and may reveal the bigger picture of mindfulness neuro-mechanism in the following studies.
Data Availability Statement
The original contributions presented in the study are included in the article/Supplementary Material, and further inquiries can be directed to the corresponding authors.
Ethics Statement
The studies involving human participants were reviewed and approved by the Taipei Medical University Joint Institute Review Board. The patients/participants provided their written informed consent to participate in this study.
Author Contributions
H-YN: conceptualization, data curation, formal analysis, investigation, visualization, and writing—original draft. CW: funding acquisition, conceptualization, project administration, supervision, and writing—review and editing. F-YH: supervision, methodology, and resources—MBSR instruction. Y-TC and S-FG: data curation. C-MH, Y-PC, and C-FH: supervision and methodology. T-PJ: supervision, conceptualization, methodology, and writing—review and editing. C-HC: supervision, conceptualization, methodology, formal analysis, and writing—review and editing. All authors contributed to the article and approved the submitted version.
Funding
This work was supported by the Center for Intelligent Drug Systems and Smart Bio-devices (IDS2B) from the Featured Areas Research Center Program within the framework of the Higher Education Sprout Project by the Ministry of Education (MOE) and by the Ministry of Science and Technology of Taiwan (project numbers: MOST 108-2321-B-038-005-MY2 and MOST 109-2636-E-007-022). No funding source had involved in any of the research procedures.
Conflict of Interest
The authors declare that the research was conducted in the absence of any commercial or financial relationships that could be construed as a potential conflict of interest.
Publisher’s Note
All claims expressed in this article are solely those of the authors and do not necessarily represent those of their affiliated organizations, or those of the publisher, the editors and the reviewers. Any product that may be evaluated in this article, or claim that may be made by its manufacturer, is not guaranteed or endorsed by the publisher.
Acknowledgments
This manuscript was uploaded to the preprint server for biology “BioRxiv” (Ng et al., 2021).
Supplementary Material
The Supplementary Material for this article can be found online at: https://www.frontiersin.org/articles/10.3389/fpsyg.2021.748584/full#supplementary-material
References
Abhang, P. A., Gawali, B. W., and Mehrotra, S. C. (2016). Introduction to EEG-and Speech-Based Emotion Recognition. Cambridge, MA: Academic Press.
Ahani, A., Wahbeh, H., Nezamfar, H., Miller, M., Erdogmus, D., and Oken, B. (2014). Quantitative change of EEG and respiration signals during mindfulness meditation. J. Neuroeng. Rehabil. 11, 1–11. doi: 10.1186/1743-0003-11-87
Allen, J. J. B., Coan, J. A., and Nazarian, M. (2004). Issues and assumptions on the road from raw signals to metrics of frontal EEG asymmetry in emotion. Biol. Psychol. 67, 183–218. doi: 10.1016/j.biopsycho.2004.03.007
Allen, M., Dietz, M., Blair, K. S., van Beek, M., Rees, G., Vestergaard-Poulsen, P., et al. (2012). Cognitive-affective neural plasticity following active-controlled mindfulness intervention. J. Neurosci. 32, 15601–15610. doi: 10.1523/JNEUROSCI.2957-12.2012
Baer, R. A., Smith, G. T., Lykins, E., Button, D., Krietemeyer, J., Sauer, S., et al. (2008). Construct validity of the five facet mindfulness questionnaire in meditating and nonmeditating samples. Assessment 15, 329–342. doi: 10.1177/1073191107313003
Becker, R., Ritter, P., Moosmann, M., and Villringer, A. (2005). Visual evoked potentials recovered from fMRI scan periods. Hum. Brain Mapp. 26, 221–230. doi: 10.1002/hbm.20152
Braboszcz, C., and Delorme, A. (2011). Lost in thoughts: neural markers of low alertness during mind wandering. NeuroImage 54, 3040–3047. doi: 10.1016/j.neuroimage.2010.10.008
Brewer, J. A., Worhunsky, P. D., Gray, J. R., Tang, Y. Y., Weber, J., and Kober, H. (2011). Meditation experience is associated with differences in default mode network activity and connectivity. Proc. Natl. Acad. Sci. 108, 20254–20259. doi: 10.1073/pnas.1112029108
Brown, K. W., and Ryan, R. M. (2003). The benefits of being present: mindfulness and its role in psychological well-being. J. Pers. Soc. Psychol. 84, 822–848. doi: 10.1037/0022-3514.84.4.822
Cahn, B. R., Delorme, A., and Polich, J. (2010). Occipital gamma activation during Vipassana meditation. Cogn. Process. 11, 39–56. doi: 10.1007/s10339-009-0352-1
Carpenter, J. K., Conroy, K., Gomez, A. F., Curren, L. C., and Hofmann, S. G. (2019). The relationship between trait mindfulness and affective symptoms: a meta-analysis of the five facet mindfulness questionnaire (FFMQ). Clin. Psychol. Rev. 74:101785. doi: 10.1016/j.cpr.2019.101785
Colgan, D. D., Christopher, M., Michael, P., and Wahbeh, H. (2016). The body scan and mindful breathing among veterans with PTSD: type of intervention moderates the relationship between changes in mindfulness and post-treatment depression. Mindfulness 7, 372–383. doi: 10.1007/s12671-015-0453-0
Davidson, R. J. (1998). Anterior electrophysiological asymmetries, emotion, and depression: conceptual and methodological conundrums. Psychophysiology 35, 607–614. doi: 10.1017/S0048577298000134
Davidson, R. J., Kabat-Zinn, J., Schumacher, J., Rosenkranz, M., Muller, D., Santorelli, S. F., et al. (2003). Alterations in brain and immune function produced by mindfulness meditation. Psychosom. Med. 65, 564–570. doi: 10.1097/01.PSY.0000077505.67574.E3
Delorme, A., and Makeig, S. (2004). EEGLAB: An open source toolbox for analysis of single-trial EEG dynamics including independent component analysis. J. Neurosci. Methods 134, 9–21. doi: 10.1016/j.jneumeth.2003.10.009
Deuschl, G., and Eisen, A. (1999). Recommendations for the practice of clinical neurophysiology: Guidelines of the International Federation of Clinical Neurophysiology. Electroencephalogr. Clin. Neurophysiol. 52, 1–304.
Ferrarelli, F., Smith, R., Dentico, D., Riedner, B. A., Zennig, C., Benca, R. M., et al. (2013). Experienced mindfulness meditators exhibit higher parietal-occipital EEG gamma activity during NREM sleep. PLoS One 8:e73417. doi: 10.1371/journal.pone.0073417
Field, T., Diego, M., and Hernandez-Reif, M. (2010). Tai chi/yoga effects on anxiety, heartrate, EEG and math computations. Complement. Ther. Clin. Pract. 16, 235–238. doi: 10.1016/j.ctcp.2010.05.014
Fitzgibbon, S. P., Pope, K. J., Mackenzie, L., Clark, C. R., and Willoughby, J. O. (2004). Cognitive tasks augment gamma EEG power. Clin. Neurophysiol. 115, 1802–1809. doi: 10.1016/j.clinph.2004.03.009
Fjorback, L. O., Arendt, M., Ørnbøl, E., Fink, P., and Walach, H. (2011). Mindfulness-based stress reduction and mindfulness-based cognitive therapy - A systematic review of randomized controlled trials: systematic review of mindfulness RCTs. Acta Psychiatr. Scandinavica 124, 102–119. doi: 10.1111/j.1600-0447.2011.01704.x
Fox, K. C. R., Nijeboer, S., Dixon, M. L., Floman, J. L., Ellamil, M., Rumak, S. P., et al. (2014). Is meditation associated with altered brain structure? A systematic review and meta-analysis of morphometric neuroimaging in meditation practitioners. Neurosci. Biobehav. Rev. 43, 48–73. doi: 10.1016/j.neubiorev.2014.03.016
Gao, J., Fan, J., Wu, B. W. Y., Zhang, Z., Chang, C., Hung, Y.-S., et al. (2016). Entrainment of chaotic activities in brain and heart during MBSR mindfulness training. Neurosci. Lett. 616, 218–223. doi: 10.1016/j.neulet.2016.01.001
Garland, E. L., Geschwind, N., Peeters, F., and Wichers, M. (2015). Mindfulness training promotes upward spirals of positive affect and cognition: multilevel and autoregressive latent trajectory modeling analyses. Front. Psychol. 6, 15. doi: 10.3389/fpsyg.2015.00015
Gärtner, M., Rohde-Liebenau, L., Grimm, S., and Bajbouj, M. (2014). Working memory-related frontal theta activity is decreased under acute stress. Psychoneuroendocrinology 43, 105–113. doi: 10.1016/j.psyneuen.2014.02.009
Goldstein, M. R., Turner, A. D., Dawson, S. C., Segal, Z. V., Shapiro, S. L., Wyatt, J. K., et al. (2019). Increased high-frequency NREM EEG power associated with mindfulness-based interventions for chronic insomnia: preliminary findings from spectral analysis. J. Psychosom. Res. 120, 12–19. doi: 10.1016/j.jpsychores.2019.02.012
Goleman, D., and Davidson, R. J. (2017). Altered Traits: Science Reveals how Meditation Changes your Mind, Brain, and Body. UK: Penguin Books.
Gratz, K. L., and Roemer, L. (2004). Multidimensional assessment of emotion regulation and dysregulation: development, factor structure, and initial validation of the difficulties in emotion regulation scale. J. Psychopathol. Behav. Assess. 26, 41–54. doi: 10.1023/B:JOBA.0000007455.08539.94
Grent-'t-Jong, T., Gross, J., Goense, J., Wibral, M., Gajwani, R., Gumley, A. I., et al. (2018). Resting-state gamma-band power alterations in schizophrenia reveal E/I-balance abnormalities across illness-stages. elife 7:e37799. doi: 10.7554/eLife.37799
Grossman, P. (2011). Defining mindfulness by how poorly I think I pay attention during everyday awareness and other intractable problems for psychology's (re)invention of mindfulness: comment on Brown et al. (2011). Psychol. Assess. 23, 1034–1040. doi: 10.1037/a0022713
Guu, S. F., NG, H. Y., Cheng, Y. T., Wu, C., Liu, C. Y., Huang, C. M., et al. (2020). Changes of Networks Connectivity Under Mindfulness Practices Among Novice Mindfulness Practitioners Organization for Human Brain Mapping Annual Conference 2020, Online conference.
Harmony, T. (2013). The functional significance of delta oscillations in cognitive processing. Front. Integr. Neurosci. 7:83. doi: 10.3389/fnint.2013.00083
Harmony, T., Fernández, T., Silva, J., Bernal, J., Díaz-Comas, L., Reyes, A., et al. (1996). EEG delta activity: an indicator of attention to internal processing during performance of mental tasks. Int. J. Psychophysiol. 24, 161–171. doi: 10.1016/S0167-8760(96)00053-0
Hauswald, A., Übelacker, T., Leske, S., and Weisz, N. (2015). What it means to be zen: marked modulations of local and interareal synchronization during open monitoring meditation. NeuroImage 108, 265–273. doi: 10.1016/j.neuroimage.2014.12.065
Hayashi, T., Okamoto, E., Nishimura, H., Mizuno-Matsumoto, Y., Ishii, R., and Ukai, S. (2009). Beta activities in EEG associated with emotional stress. Int. J. Intell. Comput. Med. Sci. Image. Process. 3, 57–68. doi: 10.1080/1931308X.2009.10644171
Huang, F. Y., Hsu, A. L., Chao, Y. P., Shang, C. M. H., Tsai, J. S., and Wu, C. W. (2021). Mindfulness-based cognitive therapy on bereavement grief: alterations of resting-state network connectivity associate with changes of anxiety and mindfulness. Hum. Brain Mapp. 42, 510–520. doi: 10.1002/hbm.25240
Huang, F. Y., Wu, C. W., Bhikshu, H. M., Shih, G. H., Chao, Y. P., and Dai, C. T. (2015). Validation of the Taiwanese version of the five facet mindfulness questionnaire (T-FFMQ). Psychol. Test. 62, 231–260.
Irving, J. A., Dobkin, P. L., and Park, J. (2009). Cultivating mindfulness in health care professionals: a review of empirical studies of mindfulness-based stress reduction (MBSR). Complement. Ther. Clin. Pract. 15, 61–66. doi: 10.1016/j.ctcp.2009.01.002
Isbel, B., Lagopoulos, J., Hermens, D. F., and Summers, M. J. (2019). Mindfulness induces changes in anterior alpha asymmetry in healthy older adults. Mindfulness 10, 1381–1394. doi: 10.1007/s12671-019-01106-w
Jha, A. P., Stanley, E. A., Kiyonaga, A., Wong, L., and Gelfand, L. (2010). Examining the protective effects of mindfulness training on working memory capacity and affective experience. Emotion 10, 54–64. doi: 10.1037/a0018438
Keizer, A. W., Verschoor, M., Verment, R. S., and Hommel, B. (2010). The effect of gamma enhancing neurofeedback on the control of feature bindings and intelligence measures. Int. J. Psychophysiol. 75, 25–32. doi: 10.1016/j.ijpsycho.2009.10.011
Keselman, H. J., Cribbie, R., and Holland, B. (2002). Controlling the rate of type I error over a large set of statistical tests. Br. J. Math. Stat. Psychol. 55, 27–39. doi: 10.1348/000711002159680
Knyazev, G. G. (2007). Motivation, emotion, and their inhibitory control mirrored in brain oscillations. Neurosci. Biobehav. Rev. 31, 377–395. doi: 10.1016/j.neubiorev.2006.10.004
Kok, B. E., and Singer, T. (2017). Phenomenological fingerprints of four meditations: differential state changes in affect, mind-wandering, meta-cognition, and interoception before and after daily practice across 9 months of training. Mindfulness 8, 218–231. doi: 10.1007/s12671-016-0594-9
Kral, T. R. A., Imhoff-Smith, T., Dean, D. C., Grupe, D., Adluru, N., Patsenko, E., et al. (2019). Mindfulness-based stress reduction-related changes in posterior cingulate resting brain connectivity. Soc. Cogn. Affect. Neurosci. 14, 777–787. doi: 10.1093/scan/nsz050
Lamothe, M., Rondeau, E., Malboeuf-Hurtubise, C., Duval, M., and Sultan, S. (2016). Outcomes of MBSR or MBSR-based interventions in health care providers: a systematic review with a focus on empathy and emotional competencies. Complement. Ther. Med. 24, 19–28. doi: 10.1016/j.ctim.2015.11.001
Luijcks, R., Vossen, C. J., Hermens, H. J., van Os, J., and Lousberg, R. (2015). The influence of perceived stress on cortical reactivity: a proof-of-principle study. PLoS One 10:e0129220. doi: 10.1371/journal.pone.0129220
Lutz, A., Greischar, L. L., Rawlings, N. B., Ricard, M., and Davidson, R. J. (2004). Long-term meditators self-induce high-amplitude gamma synchrony during mental practice. Proc. Natl. Acad. Sci. 101, 16369–16373. doi: 10.1073/pnas.0407401101
Matiz, A., Crescentini, C., Fabbro, A., Budai, R., Bergamasco, M., and Fabbro, F. (2019). Spontaneous eye movements during focused-attention mindfulness meditation. PLoS One 14:e0210862. doi: 10.1371/journal.pone.0210862
Mirams, L., Poliakoff, E., Brown, R. J., and Lloyd, D. M. (2013). Brief body-scan meditation practice improves somatosensory perceptual decision making. Conscious. Cogn. 22, 348–359. doi: 10.1016/j.concog.2012.07.009
Molina, J. L., Thomas, M. L., Joshi, Y. B., Hochberger, W. C., Koshiyama, D., Nungaray, J. A., et al. (2020). Gamma oscillations predict pro-cognitive and clinical response to auditory-based cognitive training in schizophrenia. Transl. Psychiatry 10, 1–10. doi: 10.1038/s41398-020-01089-6
Mrazek, M. D., Franklin, M. S., Phillips, D. T., Baird, B., and Schooler, J. W. (2013). Mindfulness training improves working memory capacity and GRE performance while reducing mind wandering. Psychol. Sci. 24, 776–781. doi: 10.1177/0956797612459659
Mrazek, M. D., Smallwood, J., and Schooler, J. W. (2012). Mindfulness and mind-wandering: finding convergence through opposing constructs. Emotion 12, 442–448. doi: 10.1037/a0026678
Ng, H. H.-Y., Wu, C. W., Huang, F.-Y., Cheng, Y.-T., Guu, S.-F., Huang, C.-M., et al. (2021). Mindfulness training alters resting-state EEG dynamics in novice practitioners via mindful breathing and body-scan. BioRxiv. doi: 10.1101/2021.04.16.439387 [Epub ahead of print]
Pasquini, H. A., Tanaka, G. K., Basile, L. F. H., Velasques, B., Lozano, M. D., and Ribeiro, P. (2015). Electrophysiological correlates of long-term Soto zen meditation. Biomed. Res. Int. 2015:598496. doi: 10.1155/2015/598496
Rangaswamy, M., Porjesz, B., Chorlian, D. B., Wang, K., Jones, K. A., Bauer, L. O., et al. (2002). Beta power in the EEG of alcoholics. Biol. Psychiatry 52, 831–842. doi: 10.1016/S0006-3223(02)01362-8
Santorelli, S. F., Kabat-Zinn, J., Blacker, M., Meleo-Meyer, F., and Koerbel, L. (2017). Mindfulness-Based Stress Reduction (MBSR) Authorized Curriculum Guide Center for Mindfulness in Medicine, Health Care, and Society (CFM), Worcester, MA: University of Massachusetts Medical School.
Sauer-Zavala, S. E., Walsh, E. C., Eisenlohr-Moul, T. A., and Lykins, E. L. B. (2013). Comparing mindfulness-based intervention strategies: differential effects of sitting meditation, body scan, and mindful yoga. Mindfulness 4, 383–388. doi: 10.1007/s12671-012-0139-9
Schooler, J. W., Mrazek, M. D., Franklin, M. S., Baird, B., Mooneyham, B. W., Zedelius, C., et al. (2014). The middle way: finding the balance between mindfulness and mind-wandering. Psychol. Learn. Motiv. 60, 1–33. doi: 10.1016/B978-0-12-800090-8.00001-9
Smallwood, J., and Schooler, J. W. (2015). The science of mind wandering: empirically navigating the stream of consciousness. Annu. Rev. Psychol. 66, 487–518. doi: 10.1146/annurev-psych-010814-015331
Solana, A. B., Hernandez-Tamames, J. A., Manzanedo, E., Garcia-Alvarez, R., Zelaya, F. O., and del Pozo, F. (2014). Gradient induced artifacts in simultaneous EEG-fMRI: effect of synchronization on spiral and EPI k-space trajectories. Magn. Reson. Imaging 32, 684–692. doi: 10.1016/j.mri.2014.03.008
Staufenbiel, S. M., Brouwer, A. M., Keizer, A. W., and Van Wouwe, N. C. (2014). Effect of beta and gamma neurofeedback on memory and intelligence in the elderly. Biol. Psychol. 95, 74–85. doi: 10.1016/j.biopsycho.2013.05.020
Stinson, B., and Arthur, D. (2013). A novel EEG for alpha brain state training, neurobiofeedback and behavior change. Complement. Ther. Clin. Pract. 19, 114–118. doi: 10.1016/j.ctcp.2013.03.003
Tei, S., Faber, P. L., Lehmann, D., Tsujiuchi, T., Kumano, H., Pascual-Marqui, R. D., et al. (2009). Meditators and non-meditators: EEG source imaging during resting. Brain Topogr. 22, 158–165. doi: 10.1007/s10548-009-0107-4
Tortella-Feliu, M., Morillas-Romero, A., Balle, M., Llabrés, J., Bornas, X., and Putman, P. (2014). Spontaneous EEG activity and spontaneous emotion regulation. Int. J. Psychophysiol. 94, 365–372. doi: 10.1016/j.ijpsycho.2014.09.003
Van den Hurk, P. A. M., Giommi, F., Gielen, S. C., Speckens, A. E. M., and Barendregt, H. P. (2010). Greater efficiency in attentional processing related to mindfulness meditation. Q. J. Exp. Psychol. 63, 1168–1180. doi: 10.1080/17470210903249365
Van Deursen, J. A., Vuurman, E., Verhey, F. R. J., Van Kranen-Mastenbroek, V., and Riedel, W. J. (2008). Increased EEG gamma band activity in Alzheimer’s disease and mild cognitive impairment. J. Neural Transm. 115, 1301–1311. doi: 10.1007/s00702-008-0083-y
Vibe, M., Bjørndal, A., Fattah, S., Dyrdal, G. M., Halland, E., and Tanner-Smith, E. E. (2017). Mindfulness-based stress reduction (MBSR) for improving health, quality of life and social functioning in adults: a systematic review and meta-analysis. Campbell Syst. Rev. 13, 1–264. doi: 10.4073/csr.2017.11
Wahbeh, H., Lane, J. B., Goodrich, E., Miller, M., and Oken, B. S. (2014). One-on-one mindfulness meditation trainings in a research setting. Mindfulness 5, 88–99. doi: 10.1007/s12671-012-0155-9
Yordanova, J., Banaschewski, T., Kolev, V., Woerner, W., and Rothenberger, A. (2001). Abnormal early stages of task stimulus processing in children with attention-deficit hyperactivity disorder–evidence from event-related gamma oscillations. Clin. Neurophysiol. 112, 1096–1108. doi: 10.1016/S1388-2457(01)00524-7
Keywords: mindfulness, mindfulness-based stress reduction, electroencephalograms, spectral power, five facet mindfulness questionnaire, difficulties in emotion regulation scale
Citation: Ng H-YH, Wu CW, Huang F-Y, Cheng Y-T, Guu S-F, Huang C-M, Hsu C-F, Chao Y-P, Jung T-P and Chuang C-H (2021) Mindfulness Training Associated With Resting-State Electroencephalograms Dynamics in Novice Practitioners via Mindful Breathing and Body-Scan. Front. Psychol. 12:748584. doi: 10.3389/fpsyg.2021.748584
Edited by:
Ulrich Kirk, University of Southern Denmark, DenmarkReviewed by:
Hironori Nakatani, The University of Tokyo, JapanSeyed Mohammad Mahdi Moshirian Farahi, Carleton University, Canada
Copyright © 2021 Ng, Wu, Huang, Cheng, Guu, Huang, Hsu, Chao, Jung and Chuang. This is an open-access article distributed under the terms of the Creative Commons Attribution License (CC BY). The use, distribution or reproduction in other forums is permitted, provided the original author(s) and the copyright owner(s) are credited and that the original publication in this journal is cited, in accordance with accepted academic practice. No use, distribution or reproduction is permitted which does not comply with these terms.
*Correspondence: Yi-Ping Chao, eWlwaW5nQG1haWwuY2d1LmVkdS50dw==; Chun-Hsiang Chuang, Y2guY2h1YW5nQG14Lm50aHUuZWR1LnR3