- 1Department of Physical Education, Suzhou University of Science and Technology, Suzhou, China
- 2School of Physical Education, Chengdu Sports University, Chengdu, China
Background: Although the acute effects of exercise on executive function are extensively documented in the field of exercise psychology, a thorough assessment of neuroelectric brain activity that is underlying executive function following acute exercise is absent. This systematic review investigated the effects of acute exercise on event-related potentials through their amplitude and latency.
Methods: Six electronic databases were searched from their inception to April 15, 2024. This review analyzed the influence of variables such as exercise dosage, test duration, population characteristics, and physical fitness on event-related potential components and executive function. The proportions of positive and null or negative effects across all studies were systematically assessed.
Results: In total, 52 studies were included in the analysis. The results revealed that 45 (86.5%) of the 52 studies focused on inhibitory control, with moderate-intensity aerobic exercise lasting 16–35 min demonstrating a positive effect on event-related potential components. Nine event-related potential components were examined, with P3 (observed in 43 studies), N2 (17 studies), and N1 (5 studies) being the most frequently reported. Thirty-seven studies (86%) demonstrated that exercise enhanced P3 wave amplitude, whereas 13 studies (27.1%) observed a reduction in latency. Eight studies (53.3%) indicated an increase in N2 wave amplitude following exercise, whereas two studies (13.3%) reported a decrease in latency.
Conclusion: Moderate-intensity acute aerobic exercise lasting 16–35 min demonstrates a positive impact on both executive function performance and event-related potential components, with effects lasting approximately 30 min. The optimal intervention dosage for resistance exercise, mind–body exercise, and other types of exercise necessitates further investigation in future studies.
Introduction
Maintaining optimal cognitive performance is essential for promoting human well-being. In recent decades, there has been substantial interest in the correlation between cognitive function and physical exercise. Engaging in an active lifestyle or participating in regular physical exercise is correlated with enhanced brain health (Erickson et al., 2019). Research indicates that both acute and chronic exercise can significantly enhance cognitive function, mitigate cognitive decline in the elderly, and positively influence academic performance among children and adolescents. Chronic and acute exercise have distinct influences on cognitive functions and neurophysiological responses. Chronic exercise, such as regular aerobic training, enhance executive function through sustained neuroplasticity (Aly and Kojima, 2020; Kamijo and Takeda, 2009). In contrast, acute exercise focuses on transient neurophysiological changes, such as increased arousal and neurotransmitter availability. This review specifically examines the acute effects of exercise on executive function, as measured by ERP components.
Executive function is a higher-order cognitive process characterized by active regulation of non-automatic behaviors to achieve consciously set goals (Diamond, 2013). Core executive function consists of three distinguishable subdomains: inhibitory control, working memory, and cognitive flexibility (Diamond, 2020). Specifically, inhibitory control refers to the ability to actively suppress dominant stimuli or interfering information and filter out irrelevant cues (Ren et al., 2021); working memory is defined as the capacity to temporarily maintain and manipulate relevant information (Miyake et al., 2000); and cognitive flexibility is the ability to flexibly shift between mental frameworks, operations, or conceptual representations (Kofler et al., 2019). In 2003, Colcombe et al. demonstrated that the effects of exercise on cognitive function are selective, particularly with respect to executive function (Colcombe and Kramer, 2003). Since then, the enhancement of executive function through exercise has emerged as a significant area of research. Historically, the field has primarily relied on behavioral performance metrics (e.g., response accuracy and reaction time) as indicators of cognitive function. While these metrics assess whether exercise is cognitively beneficial, they are less effective at detecting subtle changes in cognitive processes. In recent years, advanced neuroscientific techniques, including functional near-infrared spectroscopy (Cai et al., 2023), functional magnetic resonance imaging (fMRI) (Yin et al., 2022), and event-related potentials (ERP) (Zhao et al., 2022), have been extensively utilized to investigate the relationship between exercise and cognitive functions. Among these techniques, ERP offers high temporal resolution and yields insights into covert cognitive processes that occur between stimulus presentation and response execution. It is particularly effective for elucidating the neurobiological mechanisms underlying motor induction that may not be apparent from behavioral performance alone. As cognitive neuroscience has advanced, an increasing number of studies have utilized ERP to examine how acute exercise enhances executive function, thereby addressing the limitations of behavioral indicators. Chang et al., demonstrated that 20 min of power cycling resulted in reduced Stroop reaction times, increased P3 amplitude, and decreased N450 amplitude (Chang et al., 2017). Bae et al. reported that after 30 min of running, the P3 amplitude at Pz was significantly greater and the latency was notably shorter under exercise conditions compared to control conditions (Bae and Masaki, 2019).
Numerous ERP components have been investigated in studies examining the effects of acute exercise on executive function. Commonly utilized components include P3, N2, and N450, which are employed in research on various aspects of executive function, including inhibition, working memory, and cognitive flexibility. Although previous reviews have focused exclusively on P3 (Kao et al., 2020a,b; Kao et al., 2022a; Pedroso et al., 2017), other ERP components remain inadequately summarized and lack a cohesive understanding. The primary objective of this study was to systematically review the immediate effects of acute exercise on neurophysiological representations of executive function. Secondary objectives were to investigate the moderating effects of exercise intensity, duration, timing of assessment, and individual differences.
Methods
Search strategy
This systematic review was conducted following the guidelines of the Preferred Reporting Items for Systematic Reviews and Meta-Analyses extension for scoping review (PRISMA-ScR) (Tricco et al., 2018). The literature search strategy was constructed based on the PICO framework (Population, Intervention, Comparison, Outcome) to systematically identify eligible studies. In this study, P refers to humans, I refers to acute exercise intervention, C refers to rest, reading or another kind of exercise, O refers to ERP components, behavioral indices of executive functions. We searched six electronic databases (PubMed, Cochrane Library, Web of Science, Scopus, Wangfang, and the National Knowledge Infrastructure of China) from inception to October 15, 2024. To ensure a thorough exploration, we also reviewed the reference lists of systematic reviews published in the previous 3 years and conducted a manual search for additional relevant studies. The research strategy implemented in this review is described as follows:
#1 acute exercise OR a single bout exercise OR physical exercise OR physical activity OR aerobic exercise OR resistance exercise OR strength exercise OR stretching OR mind-body exercise OR flexibility exercise OR coordinative training OR multicomponent exercise
#2 cognition OR executive function OR executive control
#3 event-related potentials OR ERP OR electroencephalography
#4 #1 AND #2 AND #3
Inclusion and exclusion criteria
The inclusion criteria for this study consisted of three key aspects: (1) the study must be an experimental investigation focusing on acute exercise interventions for cognitive function; and (2) the outcome measures must include both executive function and ERP components.
The exclusion criteria comprised two main aspects: (1) the intervention protocol included confounding factors associated with non-exercise interventions; (2) the study type included meta-analyses, reviews, case reports, conference papers, commentaries, and other non-original research articles; and (3) data could not be extracted from the studies, even after contacting the authors.
Risk of bias assessment
Two independent reviewers (S.L. and W.M.L.) evaluated the quality of the selected studies. Both reviewers had previously received training in evaluating the risk of bias in research studies. Initially, there was strong agreement among the reviewers in assigning risk of bias scores, resulting in a concordance rate of 85.8%. Discrepancies between the reviewers were addressed through a consensus meeting. The methodological quality of the studies was assessed using an adapted version of the PEDro checklist, as previously employed by researchers (Kao et al., 2022b).
The criteria considered in this review included: (1) random allocation; (2) concealed allocation; (3) comparability at baseline; (4) blinded assessment; (5) between-group comparison; (6) outcome point estimates; (7) exercise specifications; and (8) evaluation of ERP components. Reviewers awarded a score of 0 if a study failed to meet a criterion or if the information was unclear, and a score of 1 if the criterion was met. Studies that utilized within-subject designs received a score of 1 for the criteria of random allocation, concealed allocation, baseline comparability, and between-group comparison.
Study selection process
Two reviewers were tasked with screening the articles. First, titles and abstracts were examined to identify studies that met the inclusion criteria. Second, the full text of the selected studies was reviewed to determine final inclusion. Disagreements between reviewers were resolved through meetings involving both reviewers and field experts.
Data collection and extraction process
The final studies included in the review were rigorously examined, and the following data were extracted and recorded in the database: (a) the name of the first author and the publication year; (b) sample size, age, sex, and other relevant characteristics of the study population; (c) experimental protocol; (d) ERP components and tasks utilized; and (e) the primary findings.
Data synthesis
The descriptive information for all included studies was summarized in Table 1. In cases where meta-analyses were not feasible or suitable, the effects of the studies were summarized based on the recommendation to synthesize and present the findings of the review (Mckenzie and Brennan, 2021). The proportions of positive and null/negative effects across all studies were calculated.
Event-related potential
ERP refers to brain potentials recorded from the scalp surface, obtained by averaging responses to specific stimuli during cognitive processing. ERP reflects neurophysiological changes occurring in the brain during cognitive tasks, thus the term “cognitive potential.” Walter discovered the first cognitively relevant ERP component, the Contingent Negative Variation (CNV), which marked the beginning of modern ERP research. The discovery of the P300 (P3) component by Sutton et al. (1965) established ERP as a significant topic in cognitive psychology. ERP is a non-invasive technique that offers high temporal resolution at the millisecond level, facilitating a detailed investigation of the “black box” of brain function and enabling the assessment of complex cognitive processes. An increasing number of studies have employed ERP techniques to investigate the underlying mechanisms linking acute exercise to cognitive function.
An ERP component is a neural signal that originates from a specific neuroanatomical region when the brain performs a particular computational operation and can be recorded from the scalp. Determining the nature of the psychological or neural processes reflected by an ERP component is a central issue in ERP research. Traditionally, ERP components are classified based on their polarity and latency. For instance, the P300 component reflects a positive wave observed at approximately 300 ms. Classical ERP components include P1, N1, P2, N2, and P3, with P1, N1, and P2 being exogenous components influenced by the stimulus, while N2 and P3 are endogenous components related to the individual’s cognitive state (Zhao, 2007). Consequently, P3 and N2 are the most frequently utilized components in studying executive function within the context of acute exercise interventions (Table 2).
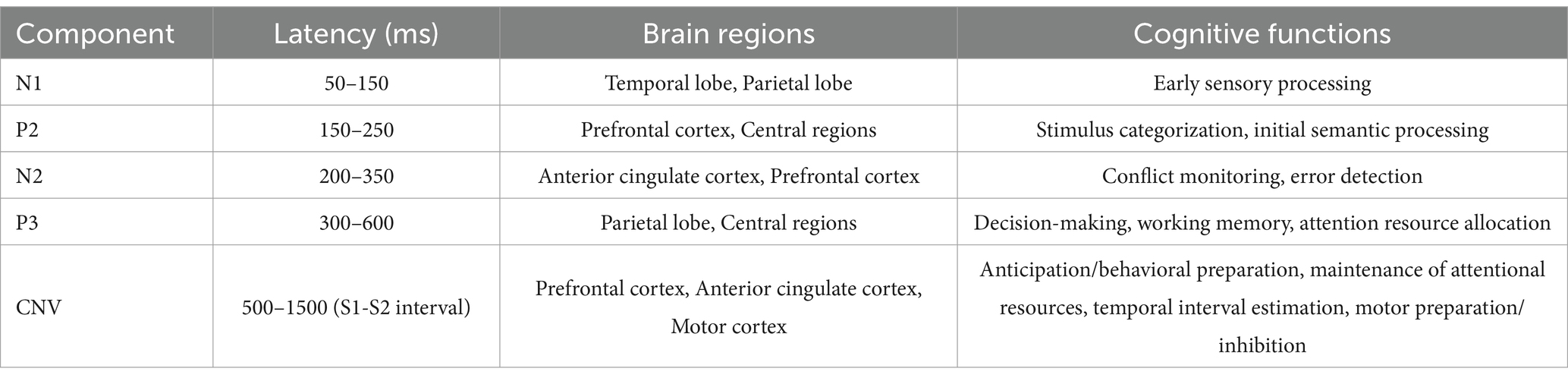
Table 2. Summary of event-related potential components: latency, associated brain regions, and cognitive functions.
The P3 component is a key ERP component for studying executive function in acute exercise interventions and is subdivided into P3b and P3a. P3b was first identified by Sutton during Oddball experiments involving rare stimuli, typically recorded as a positive deflection occurring between 300 and 800 ms (Sutton et al., 1965). P3b has been observed in both the frontal and parietal lobes, with the maximum amplitude recorded in the parietal lobe. Although the precise interpretation of P3 remains controversial, P3b is often associated with situational updating, stimulus evaluation, and resource allocation (Coles and Rugg, 1995). Halgren et al. found that P3-like signals can also be recorded from the hippocampal structures and the amygdala (Halgren et al., 1980). The hypothesis that the hippocampus is one of the sources of P3 is widely accepted among scholars. P3a is a component of P3 elicited by novel, contingent stimuli, with the maximum amplitude observed in the posterior frontal lobe, significantly more anterior than that of P3b.
The N2 component is characterized by a maximum negative deflection occurring in the frontal-central region approximately 150 to 350 ms post-stimulation (Kopp et al., 2010). N2 can be divided into subcomponents known as N2a, N2b, and N2c (Pritchard et al., 1991). Early studies employing the go/no-go task paradigm reported that N2 is more pronounced in the no-go condition than in the go condition. This finding suggests that N2 is associated with inhibition and error-monitoring processes. Additionally, under more challenging task conditions that require greater cognitive control, the amplitude of N2 is increased (Folstein and Van Petten, 2008).
The N1 component represents the initial stage of the attentional allocation process (Chacko et al., 2020) and peaks between 130 and 200 ms following stimulus onset. It is associated with visual stimulus discrimination and selective attention processing (Chacko et al., 2020). The N1 component has been found to originate from extrastriate and various posterior parietal areas (Di Russo et al., 2002).
The CNV was discovered in 1964 and is recognized as the first component identified in contemporary ERP research (Walter et al., 1964). The CNV is a stable, slow, negative waveform that is typically observed as peaks in the central and prefrontal regions. Negative potential deflections occur between warning stimuli and command stimuli, as well as during the presentations of warning stimuli, command stimuli, and the execution of commands. The first negative phase reflects the processing of the warning stimulus, while the second negative phase represents the preparatory potential associated with the individual’s readiness to respond to the target stimulus (Falkenstein et al., 2003).
Characteristics of included studies
A total of 5,837 relevant articles were retrieved during the search process. After removing 1,255 duplicates, 4,582 articles were retained following title and abstract screening. Subsequently, 87 full-text articles were reviewed, leading to the inclusion of 52 studies in the final review (Figure 1). Of these, 45 articles investigated inhibition, 3 articles examined working memory, and 4 articles explored cognitive flexibility (Table 3). Most studies employed a within-subjects crossover design, whereas only a few utilized randomized controlled designs. A total of nine ERP components were investigated, with the most frequently observed being P3 (43 studies), N2 (17 studies), and N1 (5 studies). Thirty-seven studies (86%) indicated that exercise increased P3 wave amplitude, whereas 13 studies (27.1%) reported shorter latency. Eight studies (53.3%) demonstrated an increase in N2 wave amplitude following exercise, whereas two studies (13.3%) observed shorter latency. Furthermore, two studies (40%) reported an increase in N1 amplitude after exercise, while another two studies (40%) found no significant change, and one study observed a decrease in amplitude. The remaining ERP components were reported less frequently, with each occurring in fewer than three studies.
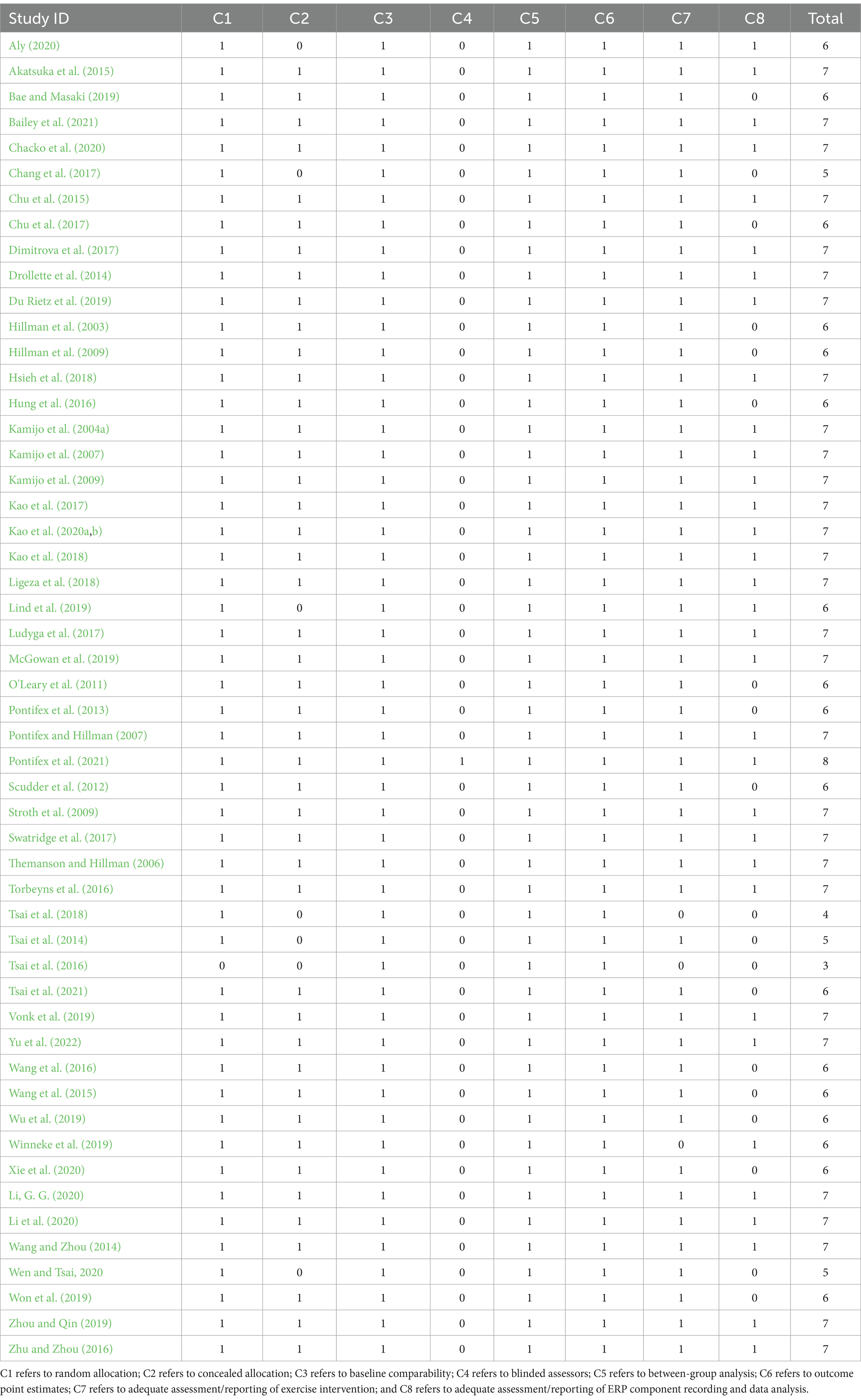
Table 3. Detailed risk of bias quality assessment of each criterion for all studies included (n = 52).
The quality of the studies was assessed, revealing a generally low risk of bias, with except for blinded assessors. The majority (96%) of the studies included in this systematic review were classified as high quality according to the PEDro checklist, indicating a low risk of bias in the findings.
As shown in Figure 2, the publication years of the included literature range from 2003 to 2023, with an average annual publication volume of 2.3. This indicates that research on the effects of acute exercise on cognitive function using ERP techniques remains in its early stages. Over the past decade, there has been an exponential increase in research on the effects of acute exercise on cognitive function, with 60% of these studies concentrating on executive function (Pontifex et al., 2019). However, there are relatively fewer studies employing ERP techniques to investigate the impact of acute exercise on executive function.
Effects of acute exercise on executive function as measured by event-related potentials
Timing of cognitive testing
Acute exercise refers to a single session of physical activity (Cai et al., 2020). Research on the effects of acute exercise on executive function has gained increasing attention in recent years. Recent experimental studies (Chang et al., 2019; Cui et al., 2020; McSween et al., 2021) and meta-analyses (Chen et al., 2020; Pontifex et al., 2019) indicate that acute exercise positively impacts executive function. The effects of acute exercise on cognitive function are transient, making it critical to explore the duration of these effects. Based on the chronological sequence of exercise and cognitive testing, cognitive tests are categorized into three phases: during exercise, immediate post-exercise, and delayed post-exercise. Immediate post-exercise refers to ERP testing conducted within 15 min after exercise, while delayed post-exercise refers to testing performed 20 to 55 min post-exercise. It has been concluded that 15 min post-exercise is the optimal time window for ERP testing (Chang et al., 2012).
Based on Chang’s categorization, 24 (46.2%) of the included studies conducted cognitive tests immediately following exercise, examining ERP components, including P3, N2, N1, P2, N140, N450 and CNV. All of these studies reported an positive effect on at least one ERP component. For example, Chang et al. (2015) found that 20 min of moderate-intensity cycling resulted in an increased P3 amplitude, a decreased N450 amplitude, and a shortened N450 latency post-exercise, while the N1 and N2 components remained unaffected. Xie et al. (2020) conducted 20 min of high-intensity interval cycling with young obese individuals and found that the amplitude of the late positive potential (LPP) increased, whereas the P3 amplitude remained unchanged.
Twenty-one (40.4%) studies assessed cognitive tasks 20 min post-exercise (Figure 3). Only Themanson’s study observed that individuals with higher fitness exhibited larger error-related negativity (ERN) and P300 (Pe) amplitudes; however, it found no relationship between changes in ERP components and acute exercise. The remaining literature indicated that acute exercise improved executive function and ERP components across the corresponding paradigms to varying degrees.
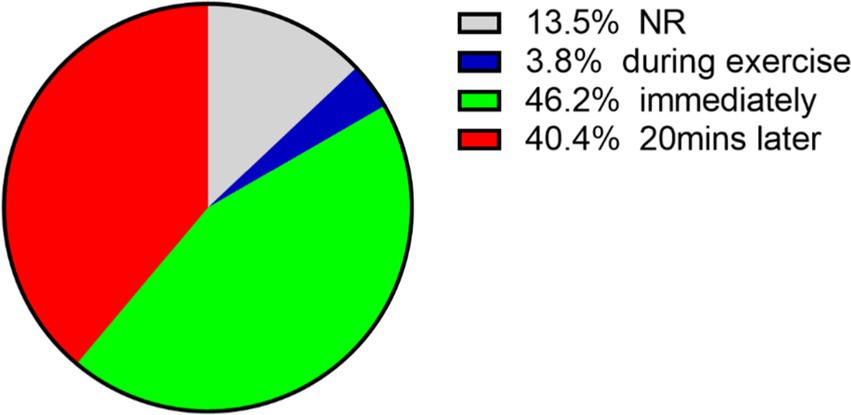
Figure 3. Time of cognitive test in the included literature. Note: Two studies included multiple testing times.
Two (3.8%) studies tested ERP during exercise with inconsistent findings. Pontifex and Hillman (2007) investigated ERP during moderate-intensity cycling (60% of maximal heart rate) and observed a decrease in N1 and N2 amplitudes in parietal regions, accompanied by an increase in P2 and P3 amplitudes in the frontal lobes. This suggests that the allocation of attentional resources to gross body movements during exercise may be linked to inefficiencies in neural resource allocation, potentially leading to reduced interference control. In contrast, Torbeyns et al. (2016) employed a power bicycle at a self-selected speed for 30 min and administered the Stroop task concurrently with exercise. The study found no difference in accuracy under exercise conditions; however, N2 amplitude and P3 latency were notably affected, demonstrating positive effects on inhibitory function. The discrepancies between the studies may be attributed to several factors: Torbeyns included sedentary individuals engaging in moderate to high-intensity exercise for an average of 2.5 h per week, whereas Pontifex included university students with no restrictions on their exercise habits. The intensity of the exercises varied, with Pontifex employing 60% of maximal heart rate and Torbeyns utilizing a self-selected speed. Relatively low exercise intensity may be insufficient to impair executive function in individuals with regular exercise habits. According to a meta-analysis by Lambourne and Tomporowski (2010), cognitive function tends to be impaired during exercise but shows improvement afterward. The transient frontal function decline theory posits that strong activation of motor and sensory systems during exercise may compromise higher cognitive functions in the prefrontal cortex (Dietrich, 2003). During cognitive tasks performed concurrently with exercise, both the exercise and cognitive tasks compete for limited cerebral blood oxygen and glucose, which may temporarily interfere with frontal lobe function and result in diminished performance on executive function tasks.
In summary, test timing is a critical factor influencing ERP outcomes. Our findings suggest that the effect of exercise on enhancing executive function may last for approximately 40 min; however, the optimal measurement time remains to be determined. The mechanism underlying the immediate effects of acute exercise on cognitive function may be associated with changes in physiological arousal. According to the Yerkes-Dodson inverted U-shaped model, an optimal level of arousal enhances cognitive performance (Yerkes and Dodson, 1908). The arousal level induced by acute exercise is temporary and gradually diminishes post-exercise, returning to baseline levels approximately 20 min after cessation of exercise. The literature reviewed generally supports this principle. The choice of ERP components can significantly influence the observed effects of acute exercise interventions, as these components reflect distinct cognitive processes. Similarly, variations in electrode placement can lead to inconsistent recordings of neural activity, potentially obscuring the true effects of exercise.
Different subdomains of executive function
Differences may exist in the effects of acute exercise interventions on the various subdomains of executive function. Some studies have reported significantly greater effects of acute exercise on inhibition compared to working memory and cognitive flexibility (Chen et al., 2011), whereas others have observed more substantial intervention effects on working memory than on cognitive flexibility and inhibition (Weng et al., 2015; Cai et al., 2020). Conversely, other research has found no significant differences in the effects across these three subdomains (Verburgh et al., 2014).
Inhibition represents a crucial component of executive function. Pontifex et al. (2019) found that 60% of the literature on acute exercise interventions targeting cognitive function focused on executive function, with 41% specifically examining the effects of inhibition. The results of the present study align with previous findings, as 46 of the included papers (Figure 4) investigated inhibition (88.5%), with the primary ERP components being P3, N2, and CNV, which exhibited increased amplitudes and shortened latencies. Test paradigms for inhibition, such as the Flanker, Go/No-Go, and Stroop tasks, demonstrate that acute exercise enhances attentional resources, aids in the classification and evaluation of executive function stimuli, and improves both conflict inhibition and response inhibition.
Only two studies in the included literature utilized acute aerobic exercise as an intervention for working memory. Both studies employed a within-subject design to assess N-back and AX-CPT performance, as well as ERP components, in college students under both resting and moderate-intensity aerobic exercise conditions. The results indicated a significant increase in P3 amplitude following aerobic exercise, whereas P3 latency, as well as N2 amplitude and latency, did not exhibit significant changes (Kao et al., 2020a,b; Scudder et al., 2012). These findings suggest that aerobic exercise may enhance goal maintenance processes and more effectively inhibit external neural activity, thereby allocating additional attentional resources for goal updating and modification. Three studies in the included literature examined the switching function and found that switching costs were significantly lower in the exercise condition compared to the control condition, with a larger P3 amplitude observed (Bae and Masaki, 2019; Hung et al., 2016; Wu et al., 2019).
As noted in the review, ERP research on acute exercise has primarily focused on inhibition, yielding relatively consistent results. However, there are comparatively fewer studies on working memory and cognitive flexibility, and the findings in these domains have been inconsistent. The predominance of inhibition research stems from its methodological maturity, urgent clinical demands, and early theoretical advancements, whereas the inherent complexity of working memory and cognitive flexibility, coupled with measurement challenges and indirect application value, have contributed to relative research stagnation.
Different participants
The literature predominantly focused on young individuals, comprising 39 studies, including two on drug addiction, one on obesity, and one on criminal behavior. Five studies were conducted on older adults, including one on mild cognitive impairment, while three addressed both older and younger populations. Additionally, five studies involved children and adolescents, with three specifically focusing on children diagnosed with attention-deficit/hyperactivity disorder (ADHD). Overall, the research subjects predominantly consist of young individuals, with fewer studies involving older adults, children, and adolescents. Recent research has increasingly focused on specific populations, including those with ADHD, addiction issues, criminal behavior, and obesity.
Age may act as a moderating factor influencing the relationship between acute exercise and ERP components. Ludyga et al. (2016) meta-analysis concluded that older adults and children exhibited significantly greater enhancements in executive function following acute exercise compared to adolescents and adults. Hillman et al. (2009) study found that 20 min of running at 60% of maximal heart rate significantly improved inhibition in children, as evidenced by higher accuracy on the Flanker task and increased P3 amplitude in the exercise condition. Drollette et al. (2014) categorized 40 children into high- and low-inhibition groups based on their Flanker task performance and had them engage in 20 min of jogging at 60–70% of maximal heart rate. Both groups exhibited shorter P3 latencies and lower N2 amplitudes compared to the sedentary condition, indicating that acute exercise enhances efficiency in stimulus categorization and reduces response conflict. However, P3 amplitude increased in the low-inhibition group, whereas no significant change was observed in the high-inhibition group. This study is the first to report the effects of acute exercise on N2 components, revealing that a decrease in N2 amplitude indicates enhanced brain efficiency and reduced conflict. This implies that children with lower inhibition experienced greater benefits from acute exercise. Similar results were observed using fMRI, indicating significant increases in frontal and parietal activation and reductions in reaction time before and after exercise (Chen et al., 2011). Pontifex et al. (2013) reported a 4% increase in Flanker task accuracy, a 38% increase in P3 amplitude, and a decrease in P3 latency following 20 min of running at 65–75% of maximal heart rate in individuals with ADHD compared to the control condition.
Kamijo et al. (2007) discovered that moderate-intensity aerobic exercise (50% maximal oxygen uptake) increased P3 amplitude in young adults, while both low-intensity (30% maximal oxygen uptake) and moderate-intensity exercises resulted in reduced P3 latency in both older and younger adults. This indicates that low-intensity acute exercise may also confer benefits for executive function in older adults. Similarly, Dimitrova et al. (2017) found that 20 min of moderate-intensity exercise (60% of reserve heart rate) improved Stroop task performance in both young and elderly individuals. Notably, the amplitude of the ERP component associated with incongruent tasks increased more significantly in elderly participants.
In summary, the current study indicates that acute exercise significantly impacts both the latency and amplitude of ERP components in younger individuals, while older individuals show a greater sensitivity to changes in latency alone. This discrepancy may be attributed to electrophysiological variations across different age groups.
Exercise prescription
The results indicated that 42 out of 52 studies focused on inhibitory control, with moderate-intensity aerobic exercise lasting between 16 and 35 min demonstrating a positive effect on P3 and N2 components. The review suggests that current ERP research methods are somewhat limited and recommends incorporating advanced techniques, such as source localization and frequency-domain analysis. Furthermore, it highlights the need to investigate working memory, cognitive flexibility, and other executive functions in greater depth, as well as to further explore the “dose” effect of exercise through additional experimental studies. Understanding how the dose of exercise affects executive function is crucial. Previous meta-analyses and systematic reviews have investigated the impact of exercise dose on the improvement of executive function (Chang et al., 2012; Cai et al., 2020). To further elucidate the relationship between physical exercise doses and ERP components, the American College of Sports Medicine (2018) “Guidelines for Exercise Testing and Prescription” (10th edition) were utilized to categorize exercise intensity and type (American College of Sports Medicine, 2018). Physical exercise types are categorized as aerobic, resistance, flexibility, and balance exercises.
For ease of interpretation, the frequency of physical activity doses is represented by a heat map (Figure 5), which depicts the relationship between exercise intensity and duration. Moderate intensity was the most frequently reported in the included literature (71.7%), followed by high intensity (58.7%); low intensity was the least used (13%) (11 studies contained more than 2 experimental groups). These findings align with the inverted U-shaped model, which is supported by numerous studies. Kamijo et al. (2004b) found that moderate-intensity exercise increased CNV and P3 amplitude, indicating that acute exercise induces optimal arousal and enhances attentional resource allocation to executive function tasks. However, both low- and moderate-intensity exercises were found to shorten P3 latency in young and older adults alike (Kamijo and Takeda, 2009). Chang et al. (2017) observed that moderate-intensity exercise increased P3 amplitude in the context of the Stroop task and shortened N450 latency. Ligeza et al. (2018) reported that moderate-intensity exercise increased N2 amplitude in the Flanker task relative to both sedentary conditions and high-intensity interval exercise. It has also been suggested that the inverted U-shaped model may vary depending on the type of cognitive task, with tasks of lower complexity potentially benefiting more from high-intensity physical activity (Mcmorris et al., 2016). Chang and Etnier (2009) suggested that high-intensity resistance exercise enhanced information processing, whereas moderate-intensity resistance exercise more effectively enhanced aspects of cognitive control. High-intensity exercise has a transient negative impact on prefrontal-dominated executive function by elevating blood lactate levels, and is more sensitive to young people and has less impact on the elderly (Coco et al., 2020). In addition, individual differences (such as those with physically active individuals) can also affect the improvement of exercise on ERP components and cognitive function (Massimino et al., 2023).
Exercise duration and intensity are intrinsically interconnected indicators of physical activity. Exercise intensity is inversely related to duration; as intensity increases, the maximum duration achievable decreases. Acute exercise-induced changes in executive function may require a minimum exercise duration. The literature predominantly employs durations of 16–35 min (94.3%), while durations of 15 min or less, as well as 36–45 min, are less common, comprising only about 5% of studies. Chang et al. (2012) found that a minimum of 11 min of exercise was required to produce changes in cognitive function, with no improvement observed for exercises lasting 10 min or less. A previous study by our team found that neither exercise lasting less than 10 min nor that lasting more than 20 min improved executive function in middle-aged and older adults; only exercise lasting between 11 and 20 min significantly enhanced executive function (Cai et al., 2020). In this study, we observed that acute exercise lasting between 16 and 25 min was associated with an increase in P3 amplitude, whereas only one instance of exercise lasting 26–35 min showed a decrease in P3 amplitude. This suggests that the exercise durations utilized in the included studies are appropriate. However, it should be noted that 10 and 20 min merely represent key time thresholds, reflecting the characteristics of the included studies, and only offer a general estimate of the effect of exercise duration. The optimal exercise duration necessitates further investigation through additional experiments.
Aerobic exercise predominated in the included literature, with cycling and running being the most commonly utilized forms, while soccer, video games, Tai Chi, and resistance exercises were employed less frequently. Tsai and Wang (2015) found that open-skill exercises resulted in smaller switching costs, reduced reaction times, and increased P3 amplitudes compared to those of closed-skill and control groups. Tsai et al. (2018) reported that both aerobic and resistance exercises improved behavioral performance and increased P3 amplitudes in older adult. O'Leary et al. (2011) found that aerobic exercise increased P3 amplitude; however, sports games and electronic games did not demonstrate similar benefits.
In summary, most studies primarily utilized aerobic exercises, such as running and cycling, while other types of exercise were used to a lesser extent. Variations in exercise modalities may engender divergent results, given their differential engagement of distinct physiological systems and cognitive mechanisms. Moderate-intensity exercise, lasting between 16 and 25 min, was found to improve ERP components, such as P3 and N2.
Mediation of cardiovascular fitness
The level of cardiovascular fitness may influence the relationship between acute exercise and ERP components, with the “cardiovascular function hypothesis” serving as a classical mechanism to explain how physical activity enhances cognitive function (Etnier et al., 2006). Many studies have identified cardiovascular fitness as a crucial variable affecting cognitive function within the framework of this hypothesis. Tsai et al. (2016) categorized participants into two groups based on their cardiovascular fitness levels and conducted a 30-min run at 60% of maximal oxygen uptake. The study found that only individuals with higher cardiovascular fitness experienced smaller switching costs and larger P3 amplitudes following acute exercise. This supports the notion that the neural mechanisms underlying the effects of acute exercise may depend on an individual’s level of cardiovascular fitness. Stroth et al. (2009) found that after 20 min of running at 60% of maximal oxygen uptake, adolescents with high cardiovascular fitness exhibited larger CNV amplitudes and smaller N2 amplitudes, whereas P3 amplitude was not significantly associated with either acute exercise or cardiovascular fitness. Themanson and Hillman (2006) demonstrated that after 30 min of running at 60% of maximal heart rate, young individuals with high cardiovascular fitness exhibited larger ERN amplitudes, while Pe amplitudes were not significantly associated with acute exercise. This suggests that cardiovascular fitness may influence executive function performance and electrophysiological indices more than acute exercise alone. Improved cardiovascular fitness may lead to increased cerebral blood flow and elevated levels of brain-derived neurotrophic factors, which in turn manifest as changes in both the amplitude and latency of ERP components. Therefore, cardiovascular fitness should be considered an important variable in study design.
Limitations and conclusion
This paper examines the effects of acute exercise on ERP components associated with executive function tasks. ERP, which reflects brain neuroelectric activity occurring between stimulus presentation and response, offers a unique approach for elucidating how acute exercise enhances executive function. Combining ERP with behavioral performance improves the understanding of the mechanisms underlying the relationship between physical activity and cognition. However, this review has several limitations: (1) language bias (English/Chinese studies only); (2) heterogeneity in exercise protocols limiting dose–response analysis; and (3) insufficient data synthesis methods for ERP components.
The present study found that acute exercise has a positive impact on executive function and ERP components, with benefits becoming evident not during exercise but rather during the period following exercise cessation. However, the optimal measurement window may not be strictly limited to 15 min, and a broader time frame could be more effective. Therefore, investigating the duration of cognitive benefits derived from exercise should be a focus of future research. Most studies utilized aerobic exercises, such as cycling and running, while fewer studies explored resistance exercise, Tai Chi, and football. Future research should encourage a diverse range of physical activities to meet the exercise needs of various populations. This approach may enhance physical fitness levels and elevate cognitive demands. Regarding exercise dosage, most studies employed moderate-intensity exercise, while others incorporated high-intensity interval training. Given the significant variation in exercise intensity, there is currently no consensus on the optimal intensity for physical activity. This issue necessitates resolution through enhanced experimental design.
Future studies are encouraged to adopt more diverse interventions for different populations, such as the dual-task protocol of exercise combined with cognitive training. Exercise may improve cognition by releasing the brain-derived neurotrophic factors, while cognitive training can directly enhance executive functions. It seems that the association of active exercise and cognitive training in a dual task protocol could enhance the improvement on executive functions (Deodato et al., 2024).
Data availability statement
The original contributions presented in the study are included in the article/supplementary material, further inquiries can be directed to the corresponding author.
Author contributions
ZC: Writing – review & editing, Writing – original draft. LS: Writing – review & editing, Formal analysis. WW: Writing – review & editing, Data curation. LM: Formal analysis, Writing – review & editing. YR: Writing – review & editing, Data curation. MW: Writing – original draft.
Funding
The author(s) declare that financial support was received for the research and/or publication of this article. This research was supported by the Jiangsu Province Social Science Fund (23TYB011), the National Social Science Fund of China (22BTY076) and the Ministry of Education, Humanities and Social Science Fund of China (23YJC890023).
Conflict of interest
The authors declare that the research was conducted in the absence of any commercial or financial relationships that could be construed as a potential conflict of interest.
Generative AI statement
The authors declare that no Gen AI was used in the creation of this manuscript.
Publisher’s note
All claims expressed in this article are solely those of the authors and do not necessarily represent those of their affiliated organizations, or those of the publisher, the editors and the reviewers. Any product that may be evaluated in this article, or claim that may be made by its manufacturer, is not guaranteed or endorsed by the publisher.
References
Akatsuka, K., Yamashiro, K., Nakazawa, S., Mitsuzono, R., and Maruyama, A. (2015). Acute aerobic exercise influences the inhibitory process in the go/no-go task in humans. Neurosci. Lett. 600, 80–84. doi: 10.1016/j.neulet.2015.06.004
Aly, M. (2020). Acute moderate-intensity exercise generally enhances neural resources related to perceptual and cognitive processes: a randomized controlled ERP study. Ment. Health Phys. Act. 19:100363. doi: 10.1016/j.mhpa.2020.100363
Aly, M., and Kojima, H. (2020). Relationship of regular physical activity with neuroelectric indices of interference processing in young adults. Psychophysiol, 57, e13674. doi: 10.1111/psyp.13674
Bae, S., and Masaki, H. (2019). Effects of acute aerobic exercise on cognitive flexibility required during task-switching paradigm. Front. Hum. Neurosci. 13:260. doi: 10.3389/fnhum.2019.00260
Bailey, B. W., Muir, A. M., Bartholomew, C. L., Christensen, W. F., Carbine, K. A., Marsh, H., et al. (2021). The impact of exercise intensity on neurophysiological indices of food-related inhibitory control and cognitive control: a randomized crossover event-related potential (ERP) study. Neuroimage 237:118162. doi: 10.1016/j.neuroimage.2021.118162
Cai, Z. D., Jiang, W. T., Wang, Q., Song, D. H., and Wang, X. (2020). Systematic review and meta-analysis of the effect of acute physical exercise on executive function in middle-aged and elderly people. China Sport Sci. Technol. 56, 45–57. doi: 10.16470/j.csst.2020147
Cai, Z. D., Shu, Z., Wang, X., and Jiang, W. T. (2023). Effects of acute elastic band exercise on working memory in the oldest-old: evidence from fNIRS. China Sport Sci. Technol. 59, 46–53. doi: 10.16470/j.csst.2023009
Chacko, S. C., Quinzi, F., De Fano, A., Bianco, V., Mussini, E., Berchicci, M., et al. (2020). A single bout of vigorous-intensity aerobic exercise affects reactive, but not proactive cognitive brain functions. Int. J. Psychophysiol. 147, 233–243. doi: 10.1016/j.ijpsycho.2019.12.003
Chang, Y. K., Alderman, B. L., Chu, C. H., Wang, C. C., Song, T. F., and Chen, F. T. (2017). Acute exercise has a general facilitative effect on cognitive function: a combined ERP temporal dynamics and BDNF study. Psychophysiology 54, 289–300. doi: 10.1111/psyp.12784
Chang, Y.-K., Chen, F.-T., Kuan, G., Wei, G.-X., Chu, C.-H., Yan, J., et al. (2019). Effects of acute exercise duration on the inhibition aspect of executive function in late middle-aged adults. Front. Aging Neurosci. 11:227. doi: 10.3389/fnagi.2019.00227
Chang, Y.-K., and Etnier, J. L. (2009). Exploring the dose-response relationship between resistance exercise intensity and cognitive function. J. Sport Exerc. Psychol. 31, 640–656. doi: 10.1123/jsep.31.5.640
Chang, Y. K., Labban, J. D., Gapin, J. I., and Etnier, J. L. (2012). The effects of acute exercise on cognitive performance: a meta-analysis. Brain Res. 1453, 87–101. doi: 10.1016/j.brainres.2012.02.068
Chang, Y. K., Chu, C. H., Wang, C. C., Wang, Y. C., Song, T. F., Tsai, C. L., et al. (2015). Dose–response relation between exercise duration and cognition. Medi. Science Sports Exerc., 47, 159–165.
Chen, F.-T., Etnier, J. L., Chan, K.-H., Chiu, P.-K., Hung, T.-M., and Chang, Y.-K. (2020). Effects of exercise training interventions on executive function in older adults: a systematic review and meta-analysis. Sports Med. 50, 1451–1467. doi: 10.1007/s40279-020-01292-x
Chen, A. G., Yin, H. C., Yan, J., and Yang, Y. (2011). Effects of acute aerobic exercise of different intensity on executive function. Acta Psychol. Sin. 43, 1055–1062. doi: 10.3724/SP.J.1041.2011.01055
Chu, C.-H., Alderman, B. L., Wei, G.-X., and Chang, Y.-K. (2015). Effects of acute aerobic exercise on motor response inhibition: an ERP study using the stop-signal task. J. Sport Health Sci. 4, 73–81. doi: 10.1016/j.jshs.2014.12.002
Chu, C.-H., Kramer, A. F., Song, T.-F., Wu, C.-H., Hung, T.-M., and Chang, Y.-K. (2017). Acute exercise and neurocognitive development in preadolescents and young adults: an ERP study. Neural Plast. 2017, 1–13. doi: 10.1155/2017/2631909
Coco, M., Buscemi, A., Guerrera, C. S., Di Corrado, D., Cavallari, P., Zappalà, A., et al. (2020). Effects of a bout of intense exercise on some executive functions. Int. J. Environ. Res. Public Health, 17, 898. doi: 10.3390/ijerph17030898
Colcombe, S., and Kramer, A. F. (2003). Fitness effects on the cognitive function of older adults: a meta-analytic study. Psychol. Sci. 14, 125–130. doi: 10.1111/1467-9280.t01-1-01430
Coles, Michael G. H., and Rugg, Michael D. (1995). Event-related brain poten-tials: an introduction. Oxford, England: Blackwell.
Cui, J., Zou, L., Herold, F., Yu, Q., Jiao, C., Zhang, Y., et al. (2020). Does cardiorespiratory fitness influence the effect of acute aerobic exercise on executive function? Front. Hum. Neurosci. 14:569010. doi: 10.3389/fnhum.2020.569010
Deodato, M., Granato, A., Buoite Stella, A., Martini, M., Marchetti, E., Lise, I., et al. (2024). Efficacy of a dual task protocol on neurophysiological and clinical outcomes in migraine: a randomized control trial. Neurol. Sci. 45, 4015–4026. doi: 10.1007/s10072-024-07611-8
Di Russo, F., Martínez, A., Sereno, M. I., Pitzalis, S., and Hillyard, S. A. (2002). Cortical sources of the early components of the visual evoked potential. Hum. Brain Mapp. 15, 95–111. doi: 10.1002/hbm.10010
Diamond, A. (2013). Executive functions. Annu. Rev. Psychol. 64, 135–168. doi: 10.1146/annurev-psych-113011-143750
Diamond, A. (2020). Executive functions. Handb. Clin. Neurol. 173, 225–240. doi: 10.1016/b978-0-444-64150-2.00020-4
Dietrich, A. (2003). Functional neuroanatomy of altered states of consciousness: the transient hypofrontality hypothesis. Conscious. Cogn. 12, 231–256. doi: 10.1016/S1053-8100(02)00046-6
Dimitrova, J., Hogan, M., Khader, P., O’Hora, D., Kilmartin, L., Walsh, J. C., et al. (2017). Comparing the effects of an acute bout of physical exercise with an acute bout of interactive mental and physical exercise on electrophysiology and executive functioning in younger and older adults. Aging Clin. Exp. Res. 29, 959–967. doi: 10.1007/s40520-016-0683-6
Drollette, E. S., Scudder, M. R., Raine, L. B., Moore, R. D., Saliba, B. J., Pontifex, M. B., et al. (2014). Acute exercise facilitates brain function and cognition in children who need it most: an ERP study of individual differences in inhibitory control capacity. Dev. Cogn. Neurosci. 7, 53–64. doi: 10.1016/j.dcn.2013.11.001
Du Rietz, E., Barker, A. R., Michelini, G., Rommel, A. S., Vainieri, I., Asherson, P., et al. (2019). Beneficial effects of acute high-intensity exercise on electrophysiological indices of attention processes in young adult men. Behav. Brain Res. 359, 474–484. doi: 10.1016/j.bbr.2018.11.024
Erickson, K. I., Hillman, C., Stillman, C. M., Ballard, R. M., Bloodgood, B., Conroy, D. E., et al. (2019). Physical activity, cognition, and brain outcomes: a review of the 2018 physical activity guidelines. Med. Sci. Sports Exerc. 51, 1242–1251. doi: 10.1249/MSS.0000000000001936
Etnier, J. L., Nowell, P. M., Landers, D. M., and Sibley, B. A. (2006). A meta-regression to examine the relationship between aerobic fitness and cognitive performance. Brain Res. Rev. 52, 119–130. doi: 10.1016/j.brainresrev.2006.01.002
Falkenstein, M., Hoormann, J., Hohnsbein, J., and Kleinsorge, T. (2003). Short-term mobilization of processing resources is revealed in the event-related potential. Psychophysiology 40, 914–923. doi: 10.1111/1469-8986.00109
Folstein, J. R., and Van Petten, C. (2008). Influence of cognitive control and mismatch on the N2 component of the ERP: a review. Psychophysiology 45, 152–170. doi: 10.1111/j.1469-8986.2007.00602.x
Halgren, E., Squires, N. K., Wilson, C. L., Rohrbaugh, J. W., Babb, T. L., and Crandall, P. H. (1980). Endogenous potentials generated in the human hippocampal formation and amygdala by infrequent events. Science 210, 803–805. doi: 10.1126/science.7434000
Hillman, C. H., Pontifex, M. B., Raine, L. B., Castelli, D. M., Hall, E. E., and Kramer, A. F. (2009). The effect of acute treadmill walking on cognitive control and academic achievement in preadolescent children. Neuroscience 159, 1044–1054. doi: 10.1016/j.neuroscience.2009.01.057
Hillman, C. H., Snook, E. M., and Jerome, G. J. (2003). Acute cardiovascular exercise and executive control function. Inter. J. Psychophysio. 48, 307–314. doi: 10.1016/S0167-8760(03)00080-1
Hsieh, S. -S., Huang, C. -J., Wu, C. -T., Chang, Y. K., and Hung, T. M. (2018). Acute exercise facilitates the N450 inhibition marker and P 3 attention marker during stroop test in young and older adults. J. Clin. Med. 7:391. doi: 10.3390/jcm7110391
Hung, C. -L., Huang, C. -J., Tsai, Y. -J., Chang, Y. -K., and Hung, T. -M. (2016). Neuroelectric and behavioral effects of acute exercise on task switching in children with attention-deficit/hyperactivity disorder. Front. Psychol. 7:1589. doi: 10.3389/fpsyg.2016.01589
Kamijo, K., Hayashi, Y., Sakai, T., Yahiro, T., Tanaka, K., and Nishihira, Y. (2009). Acute effects of aerobic exercise on cognitive function in older adults. J. Gerontol. Ser. B Psychol. Sci. Soc. Sci. 64B, 356–363. doi: 10.1093/geronb/gbp030
Kamijo, K., Nishihira, Y., Hatta, A., Kaneda, T., Wasaka, T., Kida, T., et al. (2004a). Differential influences of exercise intensity on information processing in the central nervous system. Eur. J. Appl. Physiol. 92, 305–311. doi: 10.1007/s00421-004-1097-2
Kamijo, K., Nishihira, Y., Hatta, A., Kaneda, T., Kida, T., Higashiura, T., et al. (2004b). Changes in arousal level by differential exercise intensity. Clinical Neurophysiol, 115, 2693–2698.
Kamijo, K., Nishihira, Y., Higashiura, T., and Kuroiwa, K. (2007). The interactive effect of exercise intensity and task difficulty on human cognitive processing. Int. J. Psychophysiol. 65, 114–121. doi: 10.1016/j.ijpsycho.2007.04.001
Kamijo, K., and Takeda, Y. (2009). General physical activity levels influence positive and negative priming effects in young adults. Clin. Neurophysiol. 120, 511–519. doi: 10.1016/j.clinph.2008.11.022
Kao, S.-C., Cadenas-Sanchez, C., Shigeta, T. T., Walk, A. M., Chang, Y.-K., Pontifex, M. B., et al. (2020a). A systematic review of physical activity and cardiorespiratory fitness on P3b. Psychophysiology 57:e13425. doi: 10.1111/psyp.13425
Kao, S. -C., Chen, F.-T., Moreau, D., Drollette, E. S., Amireault, S., Chu, C.-H., et al. (2022b). Acute effects of exercise engagement on neurocognitive function: a systematic review and meta-analysis on P3 amplitude and latency. Int. Rev. Sport Exerc. Psychol., 1–43. doi: 10.1080/1750984x.2022.2155488
Kao, S. C., Chen, F. T., Moreau, D., Drollette, E., Amireault, S., Chu, C. H., et al. (2022a). Acute exercise effects on neurocognitive function: a Meta-analysis on P3 amplitude and latency. Med. Sci. Sports Exerc. 54:593. doi: 10.1249/01.mss.0000882500.95594.bb
Kao, S.-C., Drollette, E. S., Ritondale, J. P., Khan, N., and Hillman, C. H. (2018). The acute effects of high-intensity interval training and moderate-intensity continuous exercise on declarative memory and inhibitory control. Psychol. Sport Exerc. 38, 90–99. doi: 10.1016/j.psychsport.2018.05.011
Kao, S. -C., Wang, C. -H., and Hillman, C. H. (2020b). Acute effects of aerobic exercise on response variability and neuroelectric indices during a serial n-back task. Brain Cogn. 138:105508. doi: 10.1016/j.bandc.2019.105508
Kao, S. -C., Westfall, D. R., Soneson, J., Gurd, B., and Hillman, C. H. (2017). Comparison of the acute effects of high-intensity interval training and continuous aerobic walking on inhibitory control. Psychophysiology 54, 1335–1345. doi: 10.1111/psyp.12889
Kofler, M. J., Irwin, L. N., Soto, E. F., Groves, N. B., Harmon, S. L., and Sarver, D. E. (2019). Executive functioning heterogeneity in pediatric ADHD. J. Abnorm. Child Psychol. 47, 273–286. doi: 10.1007/s10802-018-0438-2
Kopp, B., Rist, F., and Mattler, U. (2010). N200 in the flanker task as a neurobehavioral tool for investigating executive control. Psychophysiology 33, 282–294. doi: 10.1111/j.1469-8986.1996.tb00425.x
Lambourne, K., and Tomporowski, P. (2010). The effect of exercise-induced arousal on cognitive task performance: a meta-regression analysis. Brain Res. 1341, 12–24. doi: 10.1016/j.brainres.2010.03.091
Li, G. G. (2020). Effects of a single medium-intensity aerobic exercise and e-sports game on inhibition control under different working memory loads. Doctor. Wuhan, China: Wuhan Sports University.
Li, X. J., Yin, H. C., Cui, L., Wang, Y., Li, J., Shen, Q. Q., et al. (2020). Effect of acute Bafa Wubu of tai Chi on inhibition function: an ERP study. J. Tianjin Univ. Sport 35, 29–35. doi: 10.13297/j.cnki.issn1005-0000.2020.01.006
Ligeza, T. S., Maciejczyk, M., Kałamała, P., Szygula, Z., and Wyczesany, M. (2018). Moderate-intensity exercise boosts the N2 neural inhibition marker: a randomized and counterbalanced ERP study with precisely controlled exercise intensity. Biol. Psychol. 135, 170–179. doi: 10.1016/j.biopsycho.2018.04.003
Lind, R. R., Beck, M. M., Wikman, J., Malarski, K., Krustrup, P., Lundbye-Jensen, J., et al. (2019). Acute high-intensity football games can improve children’s inhibitory control and neurophysiological measures of attention. Scand. J. Med. Sci. Sports 29, 1546–1562. doi: 10.1111/sms.13485
Ludyga, S., Brand, S., Gerber, M., Weber, P., Brotzmann, M., Habibifar, F., et al. (2017). An event-related potential investigation of the acute effects of aerobic and coordinative exercise on inhibitory control in children with ADHD. Dev. Cogn. Neurosci. 28, 21–28. doi: 10.1016/j.dcn.2017.10.007
Ludyga, S., Gerber, M., Brand, S., Holsboer-Trachsler, E., and Pühse, U. (2016). Acute effects of moderate aerobic exercise on specific aspects of executive function in different age and fitness groups: a meta-analysis. Psychophysiology 53, 1611–1626. doi: 10.1111/psyp.12736
Massimino, S., Rinella, S., Guerrera, C. S., Di Corrado, D., Astuto, R., Sorbello, A., et al. (2023). Self-efficacy and the digit ratio in a group of sports university students. J. Funct. Morphol. Kinesiol., 8, 97. doi: 10.3390/jfmk8030097
Miyake, A., Friedman, N. P., Emerson, M. J., and Pontifex, M. B. (2000). The unity and diversity of executive functions and their contributions to complex "frontal lobe" tasks: a latent variable analysis. Cogn. Psychol. 41, 49–100. doi: 10.1006/cogp.1999.0734
McGowan, A. L., Chandler, M. C., Brascamp, J. W., et al. (2019). Pupillometric indices of locus-coeruleus activation are not modulated following single bouts of exercise. Int. J. Psychophysiol. 140, 41–52. doi: 10.1016/j.ijpsycho.2019.04.004
Mckenzie, Joanne E, and Brennan, Sue E. (2021). Synthesizing and presenting findings using other methods: Cochrane handbook for systematic reviews of interventions. Hoboken, NJ: Wiley.
Mcmorris, T., Turner, A., Hale, B. J., and Sproule, J. (2016). Beyond the catecholamines hypothesis for an acute exercise-cognition interaction: a neurochemical perspective. San Diego, CA: Academic Press.
McSween, M. P., McMahon, K. L., Maguire, K., Coombes, J. S., Rodriguez, A. D., Erickson, K. I., et al. (2021). The acute effects of different exercise intensities on associative novel word learning in healthy older adults: a randomized controlled trial. J. Aging Phys. Act. 29, 793–806. doi: 10.1123/japa.2020-0093
American College of Sports Medicine (2018). ACSM’s guidelines for exercise testing and prescription. 10th Edn. New York: Lippincott Williams & Wilkins.
O'Leary, K. C., Pontifex, M. B., Scudder, M. R., Brown, M. L., and Hillman, C. H. (2011). The effects of single bouts of aerobic exercise, exergaming, and videogame play on cognitive control. Clin. Neurophysiol. 122, 1518–1525. doi: 10.1016/j.clinph.2011.01.049
Pedroso, R. V., Fraga, F. J., Ayan, C., Cancela Carral, J. M., Scarpari, L., Santos-Galduroz, R. F., et al. (2017). Effects of physical activity on the P300 component in elderly people: a systematic review. Psychogeriatrics 17, 479–487. doi: 10.1111/psyg.12242
Pontifex, M. B., and Hillman, C. H. (2007). Neuroelectric and behavioral indices of interference control during acute cycling. Clin. Neurophysiol. 118, 570–580. doi: 10.1016/j.clinph.2006.09.029
Pontifex, M. B., McGowan, A. L., Chandler, M. C., Gwizdala, K. L., Parks, A. C., Fenn, K., et al. (2019). A primer on investigating the after effects of acute bouts of physical activity on cognition. Psychol. Sport Exerc. 40, 1–22. doi: 10.1016/j.psychsport.2018.08.015
Pontifex, M. B., Parks, A. C., Delli Paoli, A. G., Schroder, H. S., and Moser, J. S. (2021). The effect of acute exercise for reducing cognitive alterations associated with individuals high in anxiety. Int. J. Psychophysiol. 167, 47–56. doi: 10.1016/j.ijpsycho.2021.06.008
Pontifex, M. B., Saliba, B. J., Raine, L. B., et al. (2013). Exercise improves behavioral, neurocognitive, and scholastic performance in children with attention-deficit/hyperactivity disorder. J. Pediatr. 162, 543–551. doi: 10.1016/j.jpeds.2012.08.036
Pritchard, W., Shappell, S., and Brandt, M. (1991). Psychophysiology of N200/N400: A review and classification scheme. Adv. Psychophysiol. 4, 43–106.
Ren, F. -F., Chen, F. -T., Zhou, W. -S., et al. (2021). Effects of Chinese mind-body exercises on executive function in middle-aged and older adults: a systematic review and meta-analysis. Front. Psycho. 12:656141. doi: 10.3389/fpsyg.2021.656141
Scudder, M. R., Drollette, E. S., Pontifex, M. B., and Hillman, C. H. (2012). Neuroelectric indices of goal maintenance following a single bout of physical activity. Biol. Psychol. 89, 528–531. doi: 10.1016/j.biopsycho.2011.12.009
Stroth, S., Kubesch, S., Dieterle, K., Ruchsow, M., Heim, R., Kiefer, M., et al. (2009). Physical fitness, but not acute exercise modulates event-related potential indices for executive control in healthy adolescents. Brain Res. 1269, 114–124. doi: 10.1016/j.brainres.2009.02.073
Sutton, S., Braren, M., Zubin, J., and John, E. R. (1965). Evoked-potential correlates of stimulus uncertainty. Science 150, 1187–1188. doi: 10.1126/science.150.3700.1187
Swatridge, K., Regan, K., Staines, W. R., Roy, E., and Middleton, L. E. (2017). The acute effects of aerobic exercise on cognitive control among people with chronic stroke. J. Stroke Cerebrovasc. Dis. 26, 2742–2748. doi: 10.1016/j.jstrokecerebrovasdis.2017.06.050
Themanson, J. R., and Hillman, C. H. (2006). Cardiorespiratory fitness and acute aerobic exercise effects on neuroelectric and behavioral measures of action monitoring. Neuroscience 141, 757–767. doi: 10.1016/j.neuroscience.2006.04.004
Torbeyns, T., de Geus, B., Bailey, S., De Pauw, K., Decroix, L., Van Cutsem, J., et al. (2016). Cycling on a bike desk positively influences cognitive performance. PLoS One 11:e0165510. doi: 10.1371/journal.pone.0165510
Tricco, A. C., Lillie, E., Zarin, W., O’Brien, K. K., Colquhoun, H., Levac, D., et al. (2018). PRISMA extension for scoping reviews (PRISMA-ScR): checklist and explanation. Ann. Intern. Med. 169, 467–473. doi: 10.7326/M18-0850
Tsai, C. L., Ukropec, J., Ukropcova, B., and Pai, M. C. (2018). An acute bout of aerobic or strength exercise specifically modifies circulating exerkine levels and neurocognitive functions in elderly individuals with mild cognitive impairment. Neuroimage Clin. 17, 272–284. doi: 10.1016/j.nicl.2017.10.028
Tsai, C. L., Wang, C. H., Pan, C. Y., Chen, F. C., Huang, T. H., Chou, F. Y., et al. (2014). Executive function and endocrinological responses to acute resistance exercise. Front. Behav. Neurosci. 8:262. doi: 10.3389/fnbeh.2014.00262
Tsai, C. L., Pan, C. Y., Chen, F. C., Wang, C. -H., and Chou, F. -Y. (2016). Effects of acute aerobic exercise on a task-switching protocol and brain-derived neurotrophic factor concentrations in young adults with different levels of cardiorespiratory fitness. Exp. Physiol. 101, 836–850. doi: 10.1113/EP085682
Tsai, C. -L., Pan, C. -Y., Tseng, Y. -T., Chen, F. -C., Chang, Y. -C., Wang, T. -C., et al. (2021). Acute effects of high-intensity interval training and moderate-intensity continuous exercise on BDNF and irisin levels and neurocognitive performance in late middle-aged and older adults. Behav. Brain Res. 413:113472. doi: 10.1016/j.bbr.2021.113472
Tsai, C. -L., and Wang, W. -L. (2015). Exercise-mode-related changes in task-switching performance in the elderly. Front. Behav. Neurosci. 9:56. doi: 10.3389/fnbeh.2015.00056
Verburgh, L., Königs, M., Scherder, E. J. A., and Oosterlaan, J. (2014). Physical exercise and executive functions in preadolescent children, adolescents and young adults: a meta-analysis. Br. J. Sports Med. 48, 973–979. doi: 10.1136/bjsports-2012-091441
Vonk, M., Wikkerink, S., Regan, K., and Middleton, L. E. (2019). Similar changes in executive function after moderate resistance training and loadless movement. PLoS One 14:e0212122. doi: 10.1371/journal.pone.0212122
Walter, W. G., Cooper, R., Aldridge, V. J., Mccallum, W. C., and Winter, A. L. (1964). Contingent negative variation: an electric sign of Sensori-motor association and expectancy in the human brain. Nature 203, 380–384. doi: 10.1038/203380a0
Wang, D., Zhou, C., and Chang, Y. -K. (2015). Acute exercise ameliorates craving and inhibitory deficits in methamphetamine: an ERP study. Physiol. Behav. 147, 38–46. doi: 10.1016/j.physbeh.2015.04.008
Wang, D., Zhou, C., Zhao, M., Wu, X., and Chang, Y. -K. (2016). Dose-response relationships between exercise intensity, cravings, and inhibitory control in methamphetamine dependence: an ERPs study. Drug Alcohol Depend. 161, 331–339. doi: 10.1016/j.drugalcdep.2016.02.023
Wang, Y. Y., and Zhou, C. L. (2014). The dose-response relationship between acute exercise intensity and inhibitory control ——case from ERP study. China Sport Sci. 34, 42–49. doi: 10.16469/j.css.2014.11.001
Wen, H. J., and Tsai, C. L. (2020). Effects of acute aerobic exercise combined with resistance exercise on neurocognitive performance in obese women. Brain Sci. 10:767. doi: 10.3390/brainsci10110767
Weng, T. B., Pierce, G. L., Darling, W. G., and Voss, M. W. (2015). Differential effects of acute exercise on distinct aspects of executive function. Med. Sci. Sports Exerc. 47, 1460–1469. doi: 10.1249/MSS.0000000000000542
Winneke, A. H., Hübner, L., Godde, B., and Voelcker-Rehage, C. (2019). Moderate cardiovascular exercise speeds up neural markers of stimulus evaluation during attentional control processes. J. Clin. Med. 8:1348. doi: 10.3390/jcm8091348
Won, J., Alfini, A. J., Weiss, L. R., Michelson, C. S., Callow, D. D., Ranadive, S. M., et al. (2019). Semantic memory activation after acute exercise in healthy older adults. J. Int. Neuropsychol. Soc. 25, 557–568. doi: 10.1017/s1355617719000171
Wu, C. -H., Karageorghis, C. I., Wang, C. -C., Chu, C. -H., Kao, S. -C., Hung, T. -M., et al. (2019). Effects of acute aerobic and resistance exercise on executive function: an ERP study. J. Sci. Med. Sport 22, 1367–1372. doi: 10.1016/j.jsams.2019.07.009
Xie, C., Alderman, B. L., Meng, F., Ai, J., Chang, Y. -K., Li, A., et al. (2020). Acute high-intensity interval exercise improves inhibitory control among young adult males with obesity. Front. Psychol. 11:1291. doi: 10.3389/fpsyg.2020.01291
Yerkes, R. M., and Dodson, J. D. (1908). The relation of strength of stimulus to rapidity of habit-formation. J. Comp. Neurol. Psychol. 18, 459–482. doi: 10.1002/cne.920180503
Yin, L. Q., Tang, C. F., Luo, W. Q., Tao, X., Li, F. L., and Li, J. (2022). Effect of multi-mode exercise on cognitive function and neuroplasticity in the elderly with MCI. China Sport Sci 42, 78–87+97. doi: 10.16469/j.css.202201007
Yu, C. -C., Chen, C. -Y., Muggleton, N. G., Ko, C. -H., and Liu, S. (2022). Acute exercise improves inhibitory control but not error detection in male violent perpetrators: an ERPs study with the emotional stop signal task. Front. Hum. Neurosci. 16:796180. doi: 10.3389/fnhum.2022.796180
Zhao, J., Liu, X. B., Li, J., Zheng, Z., and Jin, R. J. (2022). Event-related potential for cognitive function research: a visual analysis. Chin J. Rehabil. Theory Pract. 28, 69–78. doi: 10.3969/j.issn.1006-9771.2022.01.011
Zhou, F., and Qin, C. (2019). Acute moderate-intensity exercise generally enhances attentional resources related to perceptual processing. Front. Psychol. 10:2547. doi: 10.3389/fpsyg.2019.02547
Zhu, F. S., and Zhou, C. L. (2016). Effects of acute and moderate-intensity aerobic exercises on inhibiting ability of college students: evidence from behavior and physiology. Chin J. Sports Med. 35, 940–971. doi: 10.16038/j.1000-6710.2016.10.007
Glossary
ADHD - attention-deficit/hyperactivity disorder
AE - aerobic exercise
AR - Accuracy rate
C - control group
CNV - Contingent Negative Variation
CPT-OX - cued continuous performance task
D - duration
EIH - higher fitness group
ERN - error-related negativity
ERP - event-related potentials
fMRI - functional magnetic resonance imaging
HIIE - high intensity interval exercise
HR - heart rate
HRmax - maximum heart rate
HRR - heart rate reserve
I - intensity
LPP - late positive potential
MCI - mild cognitive impairment
MA - methamphetamine addict
MICE - moderate intensity continuous exercise
N - sample size
NR - no report
PRISMA - Preferred Reporting Items for Systematic Reviews and Meta-Analyses
RE - resistance exercise
RF - resting
RM - repetition maximum
RPE - rating of perceived exertion
RT - reaction time
SP - sustained potential
SRF - small-sided real football game
SWF - small-sided walking football games
VO2max - maximum oxygen consumption
Keywords: cognition, executive function, acute exercise, event-related potential, EEG
Citation: Cai Z, Shi L, Wu W, Meng L, Ru Y and Wu M (2025) A scoping review of effects of acute exercise on executive function: evidence from event-related potentials. Front. Psychol. 16:1599861. doi: 10.3389/fpsyg.2025.1599861
Edited by:
Alessandra Galmonte, University of Trieste, ItalyReviewed by:
Claudia Savia Guerrera, Department of Biomedical and Biotechnological Science, ItalyManuela Deodato, University of Trieste, Italy
Copyright © 2025 Cai, Shi, Wu, Meng, Ru and Wu. This is an open-access article distributed under the terms of the Creative Commons Attribution License (CC BY). The use, distribution or reproduction in other forums is permitted, provided the original author(s) and the copyright owner(s) are credited and that the original publication in this journal is cited, in accordance with accepted academic practice. No use, distribution or reproduction is permitted which does not comply with these terms.
*Correspondence: Moulin Wu, d3Vtb3VsaW5AdXN0cy5lZHUuY24=