- 1Department of Genomics, Institute of Plant Genetics, Polish Academy of Sciences, Poznań, Poland
- 2Institute for Resistance Research and Stress Tolerance, Julius Kühn Institute, Federal Research Centre for Cultivated Plants, Quedlinburg, Germany
- 3Department of Genetics and Plant Breeding, Plant Breeding and Acclimatization Institute – National Research Institute, Błonie, Poland
- 4Department of Biotechnology, Institute of Plant Genetics, Polish Academy of Sciences, Poznań, Poland
Leaf rust caused by Puccinia triticina Eriks belongs to the most important fungal pathogens of wheat (Triticum aestivum L.) and triticale (× Triticosecale). Effective resistance to leaf rust is both, cost-effective and environmentally safe. Many wild Aegilops species carry unknown resistances against fungal diseases and are characterized by a high genetic variability. The main goal of this work was to examine the resistance of (Aegilops tauschii × Secale cereale) × Triticosecale hybrids to leaf rust in inoculation tests with different races of P. triticina. Hybrid plants were selected for the presence of 2D chromosome/s in the triticale background using fluorescence and genomic in situ hybridization. The presence of leaf rust resistance genes was confirmed with closely linked molecular markers, i.e., Xgdm35 and Xgwm296. 14 genotypes of BC2F4 – BC2F6 hybrid plants with the monosomic addition of chromosome 2D (M2DA) were analyzed together with nine control lines. Resistance was determined at the macroscopic and microscopic level at the seedling and adult plant stage (flag leaf). In general, results revealed limited resistance of hybrid plants at the seedling stage, followed by an increase of the resistance level at later stages of plant development. This indicates that respective hybrid plants may exhibit APR resistance conferred by Lr22a introgressed from Ae. tauschii. On the basis of the macroscopic and microscopic analysis, this kind of resistance turned out to be additive and race-specific. We selected four monosomic 2D addition triticale genotypes highly resistant to P. triticina infection at the two main stages of plant development. From the selected genotypes, we obtained 26 doubled haploid lines among which two lines with doubled additional chromosomes 2D of Ae. tauschii can be used for further breeding to increase leaf rust resistance of cultivated triticale.
Introduction
Leaf rust caused by Puccinia triticina Eriks, is one of the most destructive and important foliar diseases of cereals, especially because of the wide distribution and potential to develop rapidly under optimal environmental conditions (Kolmer, 1996; Dean et al., 2012; Chen et al., 2013). Yield losses caused by P. triticina range between 10 up to 70% (Roelfs et al., 1992; Huerta-Espino et al., 2011). Infections result in a reduction of kernels per ear, lower kernel weight and degradation in grain quality. In order to avoid yield losses and reduced quality, host resistance is both cost-effective and environmentally safe (Assefa and Fehrmann, 2000; Kalia et al., 2017).
Genetic resistance to leaf rust can be determined as seedling resistance or adult plant resistance (APR). Seedling resistance, also defined as major gene, race-specific or qualitative resistance confers a high level of resistance mostly caused by a hypersensitive response. The APR is described as a susceptible reaction at the seedling stage, followed by an increased level of resistance in further stages of plant development. APR can be either race-specific (e.g., Lr12) or race-non-specific (e.g., Lr34) and is usually measured on the flag leaf (Dyck and Kerber, 1985; Park and McIntosh, 1994; McIntosh et al., 1995).
Wild Aegilops species constitute a rich source of genetic variability of agronomically important traits (Frankel and Bennett, 1970), especially resistance to diseases (leaf/brown rust, yellow rust, stripe rust and powdery mildew) (Valkoun et al., 1985; Gill et al., 1986; Cox et al., 1992; Assefa and Fehrmann, 2004). Due to the close genetic relationship with cereals, goat grasses have been used successfully in breeding programs conducted in order to improve the triticale (× Triticosecale Wittm.) and wheat (Triticum aestivum L.) using conventional crossing and recombination methods (Schneider et al., 2008; Apolinarska et al., 2010).
A number of leaf rust resistance genes were transferred from Ae. tauschii to cultivated wheat. Ae. tauschii is the source of seedling resistance genes Lr21 (1D), Lr32 (3D), Lr39 (2D), Lr42 (1D), and APR gene Lr22a (2D) (Rowland and Kerber, 1974; Kerber, 1987; Cox et al., 1994; Raupp et al., 2001). Lr22a confers resistance at the adult plant stage comparable to the resistance conferred by seedling resistance genes, contrary to the slow-rusting type APR determined by genes, e.g., Lr34 (Lagudah et al., 2006). Furthermore, the absence of virulence to Lr22a is partially explained by its lack of exposure to pathogen, thus this gene can be used for pyramiding of leaf rust resistance (McIntosh et al., 1995; Hiebert et al., 2007). In the United States, there are no reports of cultivars carrying this gene (Kolmer, personal communication). Using TACCA (targeted chromosome-based cloning via long-range assembly) Thind et al. (2017) cloned the broad-spectrum of Lr22a and found that this gene encodes an intracellular immune receptor homologous to the RPM1 protein of Arabidopsis thaliana. Another resistance gene on the short arm of chromosome 2D is Lr39 which has been transferred already to modern cultivars, however, virulent races were observed already in some regions of the world (Kolmer and Hughes, 2017).
The combination of both seedling and APR genes on chromosome 2D together with genes determining agronomically important traits (Jia et al., 2013), indicates that this chromosome is best suited for improving Triticeae species by wide crosses. Ae. tauschii × Secale cereale amphiploids, as a bridge between wild and cultivated species, constitute a successful way to transfer the D-genome chromatin into triticale. Utilization of this amphiploids allowed Kwiatek et al. (2015) to transfer the 3D chromosome carrying Lr32 from Ae. tauschii into triticale, whereas Majka et al. (2016) obtained monosomic addition lines of triticale carrying chromosome 2D of Ae. tauschii (M2DA), which let to changes in plant height, spike morphology and an increased resistance to Blumeria graminis.
The acceleration of breeding progress is one of the crucial matters for crop improvement. Doubled haploids (DHs) directly leading to homozygous plants, considerably shortened the breeding process in many inbreeding plant species (Murovec and Bohanec, 2012). In triticale DHs are usually obtained by androgenesis induced in in vitro anther cultures and are routinely used in triticale breeding (Ślusarkiewicz-Jarzina and Ponitka, 1997, 2003; Ślusarkiewicz-Jarzina et al., 2017).
The main goal of this work was to examine the resistance of (Ae. tauschii × S. cereale) × Triticosecale hybrids to leaf rust. For that purpose, inoculation with variable isolates of P. triticina carrying different virulence patterns was performed, to get information on genes transferred from Ae. tauschii. In order to obtain stable triticale lines with introgression of D-genome chromatin and with resistance to leaf rust, DHs of selected resistant hybrids were produced and analyzed. To achieve this macroscopic and microscopic analyses of infection were conducted complemented by molecular marker analyses and cytogenetic examination of hybrid plants.
Materials and Methods
Plant Material and Growing Conditions
Seeds of Aegilops tauschii Coss. (D51; 2n = 2x = 14; DD), Secale cereale L. (Strzekecinskie; 2n = 2x = 14, RR) and × Triticosecale Wittm. (Bogo; 2n = 6x = 42; AABBRR) originating from the collection of the Institute of Plant Genetics, PAS, were used in this study. The Ae. tauschii × S. cereale amphiploids (2n = 4x = 28; DDRR) were obtained using embryo rescue by Sulinowski and Wojciechowska in the IPG PAS (data unpublished). The F1 (Ae. tauschii × S. cereale) × triticale hybrids were obtained by crossing of triticale cv. Bogo with Ae. tauschii × S. cereale amphiploids as a pollinator. Backcrosses with the triticale as a male parent were used to achieve the following generations (BC1F1 and BC2F1) and further selfed to produce the BC2F4 to BC2F7 hybrid plants. Seeds of T. aestivum L. (Thatcher and Borenos, 2n = 6x = 42; AABBDD), near-isogenic lines (NILs) of wheat cv. Thatcher, i.e., Tc + Lr22a and Tc + Lr39 originated from the collection of the Julius Kuehn Institute (JKI) and the Plant Breeding and Acclimatization Institute – National Research Institute (PBAI-NRI). Cultivar Thatcher and Borenos were used as standards susceptible to leaf rust. Cultivation was conducted at 80% ± 10% humidity, at 20°C ± 2°C and a light intensity higher than 300 ± 15 mmol under daylight conditions (16 h) on the level of the soil surface.
Chromosome Preparation and Labeling of Probes
Germination, metaphase accumulation, and fixation procedures were carried out according to Kwiatek et al. (2016a). The chromosome preparations were made from root tips using the squash method according to Hasterok et al. (2006) with minor modifications. Three repetitive sequences were used for FISH: pTa-535, pTa-86 and pTa-k374 which were amplified and labeled according to Kwiatek et al. (2016b). Probe pTa-535 was labeled using tetramethyl-5-dUTP-rhodamine (Roche), pTa-86 was labeled with digoxigenin-11-dUTP (Roche) and pTa-k374 with Atto647N (Jena BioScience). Total genomic DNA was extracted from fresh leaves of Ae. tauschii (DD) and triticale ‘Bogo’ (AABBRR) using GeneMATRIX Plant and Fungi DNA Purification Kit (EURx). In GISH experiments, genomic DNA from Ae. tauschii was labeled with Atto647N (Jena BioScience). Blocking DNA from triticale was sheared to fragments of 5–10 kb by boiling for 30–45 min and used at a ratio of 1:50 (probe:block).
Fluorescent and Genomic in situ Hybridization (FISH and GISH)
FISH and GISH procedures were performed according to Kwiatek et al. (2016a) with minor modifications. Three probes (pTa-535, pTa-86, pTa-k347) were subjected to FISH on the same chromosome preparations. The hybridization mixture (20 μl per slide) contained 90 ng of each probe in the presence of salmon sperm DNA, 50% formamide, 2 × SSC, and 10% dextran sulfate, and was denatured at 70°C for 10 min and stored on ice for 5 min. Chromosomal DNA was denatured in the presence of the hybridization mixture at 70°C for 3 min on a heating table (Medax) and allowed to hybridize overnight at 37°C. Digoxigenin detection was made using anti-digoxigenin-fluorescein antibody (Roche). After documentation of the FISH sites, the slides were washed according to the procedure of Heslop-Harrison (2000) with minor modifications (2 × 15 min in 4 × SSC + 0.2% Tween at 37°C and 1 × 5 min in 2 × SSC, at room temperature). GISH, with total genomic DNA of Ae. tauschii as a probe and DNA of triticale as a block, was made with the same conditions after reprobing. Ten metaphases per slide were analyzed and documented with the Olympus BX61 automatic epifluorescence microscope supplied with an Olympus XM10 CCD camera. Image processing was carried out using the Olympus Cell-F imaging software (version 3.1; Olympus Soft Imaging Solutions) and PaintShop ProX5 software (version 15.0.0.183; Corel Corporation). The identification of particular chromosomes was made in reference to previous reports of Cuadrado and Jouve (1994) and Komuro et al. (2013).
PCR Amplification of Molecular Markers
Genomic DNA was extracted from fresh leaves of single genotypes of (Ae. tauschii × S. cereale) × Triticosecale hybrid plants as well as positive and negative controls: Ae. tauschii, triticale cv. Bogo, Ae. tauschii × S. cereale amphiploid, wheat cv. Thatcher and NILs: Tc + Lr22a and Tc + Lr39, using GeneMATRIX Plant and Fungi DNA Purification Kit (EURx). Two molecular markers localized on chromosome 2D were used: Xgdm35 and Xgwm296 (Roder et al., 1998; Pestsova et al., 2000). PCR reactions were performed according to Majka et al. (2017) with appropriate annealing temperature (52–60°C). Amplification products were separated in agarose gels (Sigma-Aldrich), stained, visualized and photographed according to Majka et al. (2017).
Isolates of P. triticina, Inoculation of Plants and Infection Ratings
For the analyses of hybrids resistance to leaf rust infection, three isolates of P. triticina from the JKI collection were used. Isolate 1 one was the aggressive isolate (wxr77), has a high germination rate and generates the highest number of uredospores per pustule on susceptible genotypes in comparison to all other isolates used for experiments. The isolate is avirulent against Lr22a at the adult stage and develops uredospore pustules delayed at the seedling stage. Furthermore isolate 1 is avirulent to Lr39. Isolate 2 was virulent for Lr22a at all stages and isolate 3 was virulent to Lr39 (resulting in infection rate from 2 to 3) at all stages of plant development. In order to find virulence/avirulence pattern useful for plant genotypes tested at PBAI-NRI we include for initial screening 10 isolates originating from wheat (Pt13-4-1, Pt14-82-1, Pt14-84-1, Pt14-85-1, Pt15-82, Pt15-89, Pt15-94-1, Pt15-100, Pt15-101, Pt15-103) and one from triticale (Pt11WT12). These isolates were chosen from the PBAI-NRI collection based on host origin and diverse response of Thatcher NILs containing Lr22a and Lr39 genes (data not shown). Initial screening of isolates was performed at seedling and adult plant stage on five genotypes: Ae. tauschii, triticale cv. Bogo, wheat cv. Thatcher and two NILs of that cultivar with leaf rust resistance genes Tc + Lr22a and Tc + Lr39.
For the analysis of the level of resistance (JKI), five plants of each genotype were planted in pots with a diameter of 5.5 cm in four replications in soil for germination (Archut-Fruhstorfer Erde, Hawita). Seedlings (first leaf fully expanded) were inoculated 12 days after germination with 10mg of leaf rust uredospores mixed with 10 mg of dry powdered clay per pot using a settling tower (Isolates 1–3) (Hoogkamp et al., 1998). In the experiments with isolates from PBAI-NRI collection, 10-day-old seedlings (3–5 per genotype) grown in 8 × 13-cell horticultural plastic tray (30 cm × 60 cm) filled with pindstrup mix special substrate (Pindstrup) were inoculated with 80 mg of uredospores, using the spraying method (Roelfs et al., 1992). In all tests, inoculated plants were kept in a humid glass chamber at 25°C for incubation for the first 24 h and then, shifted to a muslin cloth chamber under 24°C/20°C (16-h day/8-h night) regime.
After tests at seedling stage all genotypes planned for inoculation at adult plant stage (flag leaf) were grown at 4°C/8 weeks for vernalization and preservation of plants at the same plant growth stage as possible. After this period each seedling was planted to individual larger pot (20 cm × 20 cm × 30 cm depth) filled with pindstrup mix special substrate and kept at glasshouse (at least 16 h light and temperature below 28°C) until testing flag leaf. Nevertheless, because of different maturity, the genotypes were tested in two series, so plants had flag leaf developed at the same stage. Plants with fully developed flag leaves were moved back to plant growth chamber using temperature and humidity for inoculation and incubation period as described earlier. However, flag leaves of adult plants were inoculated using uredospores in rate 200 mg/50 ml water/24 pots.
Disease reaction (both, seedlings and flag leaves) was recorded for all accessions and different isolates at 8–10 days after the inoculation using 0–4 scale described by McIntosh et al. (1995). This rating system allows the classification as “immune” (rated as “0”), “very resistant” (rated as “;” or “0.5”), “resistant” (rated as “1”), “moderately resistant” (rated as “2”) “moderately resistant to moderately susceptible” (rated as “3”) and “susceptible” (rated as “4”) to leaf rust. In parallel genotypes were vernalized for 8 weeks (4°C) before infection at the flag leaf stage in order to analyze resistance reaction of adult plants.
Staining Procedures and Microscopy
Leaves of inoculated plants were collected at 48 hai (hours after inoculation), 72 hai, 96 hai, and 168 hai by cutting leaves to segments of 1.5 cm length. Segments were incubated in reaction tubes (2 ml) in 1.5 ml of the 3,3′ Diaminobenzidine (DAB) solution for 16 h at room temperature. Fungal cell walls were stained with Calcofluor White M2R (Rohringer et al., 1977) according to Serfling et al. (2016). Samples were washed with sterile water, transferred to a microscope slide and embedded in a glycerol/water solution (1:1 v/v). Microscopy of leaf cells and fungal structures was performed using a Zeiss Axioskop 50 microscope supplied with the AxiocamMRc camera connected with the software package Axiovision 4 (Carl Zeiss). Fungal structures were observed using the filter set 02 (excitation filter G 365, beam splitter FT 395, and barrier filter LP 420), autofluorescence within plant tissue was observed using the filter set 05 (excitation filter BP 400-440, beam splitter FT 460, barrier filter LP 470). These conditions enabled the visualization of pathogen development, i.e., germinating spores, appressoria and haustorial mother cells (HMCs). This procedure also allowed the detection of host cell micronecrosis autofluorescence adjacent to HMC. For experiments in which fungal structures (hmc) were counted, four leaf segments from the middle of the youngest leaf of four seedlings from four different plants of the same genotype were microscopically analyzed. Within each replication 10 infection sites (germinated uredospore and appressorium generated) were counted, thus counts of 40 infection sites (4 × 10) were used for the analysis.
Doubled Haploid Production
Tillers from the monosomic addition lines of triticale with chromosome 2D were cut at the uninuclear stage of the microspore development and cold treated at 4°C for 6 days in the mineral salt medium N6 (Chu et al., 1975) in the dark before plating the anthers. Anthers were cultured on the C17 medium (Wang and Chen, 1983) with 90 g/l maltose (instead of sucrose), 2.0 mg/l 2,4-D and 0.5 mg/l KIN in the dark at 28°C for 4 weeks. The androgenic embryos developed from the microspores were transferred to the regeneration medium 190-2 with 1.0 mg/l KIN, 0.5 mg/l NAA and 30 g/l saccharose (Zhuang and Xu, 1983). Organogenesis was induced at 22°C in the continuous light for 12 h per day. The ploidy levels of green plants were determined by flow cytometry. The identified haploid plants were subjected to colchicine treatment (0.1% aqueous solution with 4% DMSO and 25 mg/l GA3) for 6 h in light at 25°C. After the treatments, plants were washed, potted, vernalized for 6 weeks at 4°C and moved to the greenhouses where they were grown to maturity.
Statistical Analysis
The macroscopic and microscopic observations data were subjected to statistical analysis. Two-way analysis of variance (ANOVA) with a homogenous groups of genotypes (hybrids and controls) and stage of development (seedling and adult plants) as classification factors and Fisher’s least significant difference test (LSD) were made using STATISTICA 10 software (StatSoft, Tulsa, OK, United States). Fisher’s LSD of samples at 5% was used.
Results
Identification of Monosomic 2D Addition Hexaploid Triticale Plants
The chromosome composition of (Ae. tauschii × S. cereale) × triticale cv. Bogo hybrids was verified using FISH and GISH. In FISH analyses three BAC clones were mapped: pTa-k374 (28S rDNA), pTa-535 (pAs1 related), pTa-86 (pSc119) (Figure 1a), and in GISH D-genome probe (DNA of Ae. tauschii) and blocking DNA from triticale were applied (Figure 1b). Because of the diversity of genes and according to previous results (Majka et al., 2016), hybrid plants were selected for the presence of 2D chromosome/s in triticale background. Fourteen genotypes of BC2F4 – BC2F6 hybrid plants with the monosomic addition of chromosome 2D (M2DA) were used. Additionally, two genotypes of BC2F7 without Lr genes, but carrying one 2D chromosome and pair of 2D chromosomes were chosen as susceptible controls. As a complement, seven control lines were applied: Ae. tauschii, Ae. tauschii × S. cereale amphiploid form, triticale cv. Bogo, wheat cv. Borenos and Thatcher as well as two NILs: Tc + Lr22a and Tc + Lr39 (Supplementary Tables S1, S2). Plant material was divided into three groups. The first group was designated for the macroscopic and microscopic analysis of the leaf rust resistance performed in JKI, the second group for the macroscopic analysis of the leaf rust resistance performed in PBAI-NRI and a third group of hybrid plants for the DH production (IPG PAS).
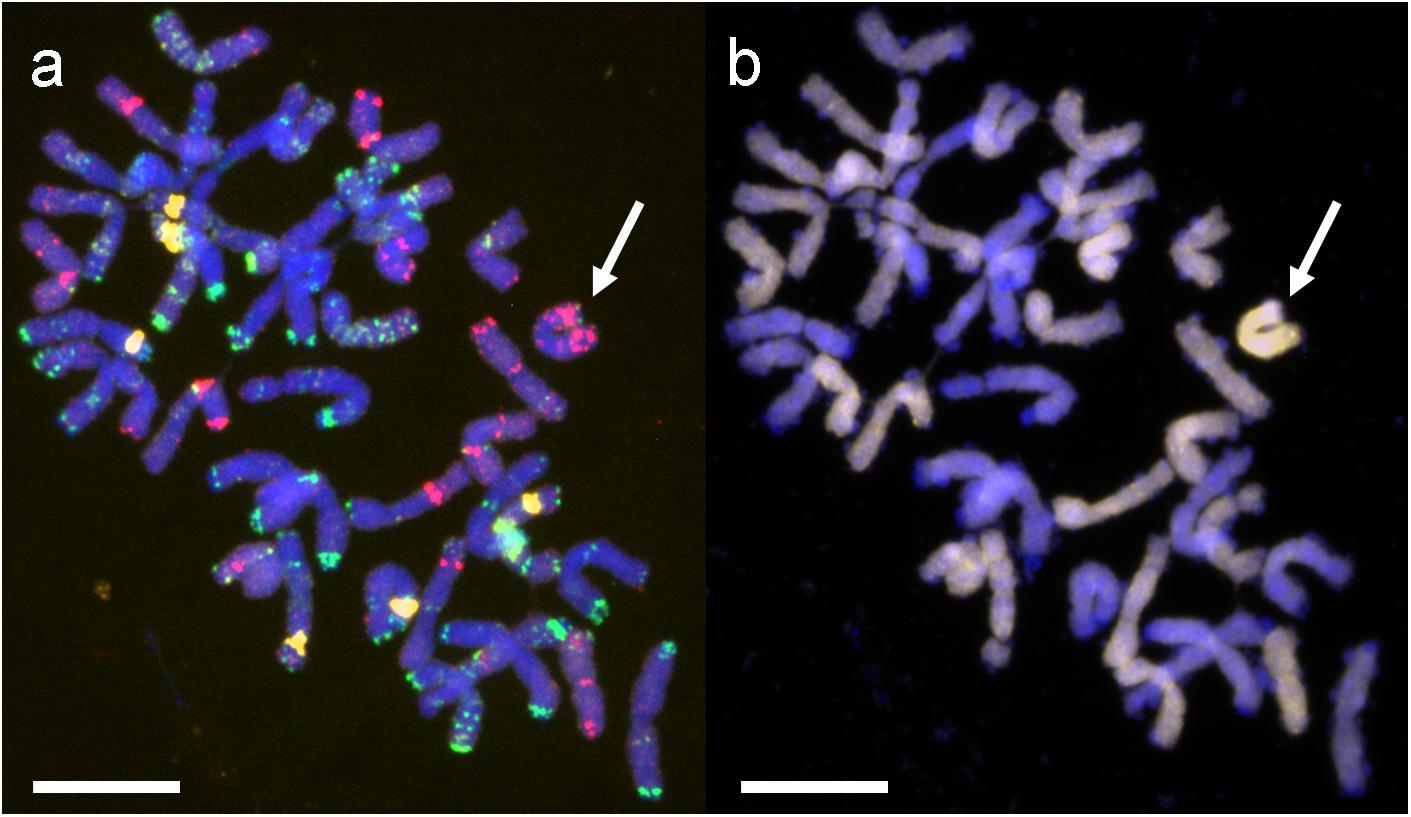
FIGURE 1. Mitotic chromosomes of hybrid genotype 1 (BC2F4) (Ae. tauschii × S. cereale) × Triticosecale cv. Bogo analyzed using (a) FISH with pTa-535 (red), pTa-86 (green) and pTa-k374 (28S rDNA) (yellow) probes, (b) GISH with total genomic DNA probe of Ae. tauschii (D, yellow) and triticale (ABR, blocking DNA). Arrows indicate the introgressed chromosome 2D. Scale bars 10 μm.
PCR Analysis of Markers Related to Leaf Rust Resistance Genes
In this study, the presence of two molecular markers associated with the leaf rust resistance genes, i.e., Xgwm296 and Xgdm35 (Lr22a and Lr39, respectively) localized on chromosome 2D was verified (Roder et al., 1998; Pestsova et al., 2000). Results obtained for Xgdm35 marker revealed that the allele linked to resistance (PCR product of 180 bp) was present in all analyzed genotypes of hybrid plants and control plants: Ae. tauschii, amphiploid form Ae. tauschii × S. cereale and Tc + Lr39. We observed products of different size (indicative for susceptibility) in two genotypes of hybrid plants with one 2D chromosome and a pair of 2D chromosomes which were selected as control plants without resistance genes as well as for triticale Bogo and Tc + Lr22a. The size of the bands differed between Ae. tauschii and NILs of T. aestivum cv. Thatcher with Lr39. The presence of Lr22 was determined with Xgwm296 marker. The banding pattern specific for resistance allele Lr22a (120 + 150 bp) was present in all analyzed genotypes of hybrid plants and control plants: Ae. tauschii, amphiploid form Ae. tauschii × S. cereale and Tc + Lr22a.
Selection of the Isolates
To select appropriate isolate with virulence/avirulence pattern useful for plant genotypes tested at PBAI-NRI, 11 isolates of P. triticina from the PBAI-NRI collection were tested at seedling (first leaf) and adult plant stage (flag leaf) (Table 1 and Figure 2). From among isolates tested, Pt11WT12 was chosen for further studies, since this isolate was the only virulent to triticale cv. Bogo at both plant growth stages and could differentiate Thatcher lines with Lr22a and Lr39.
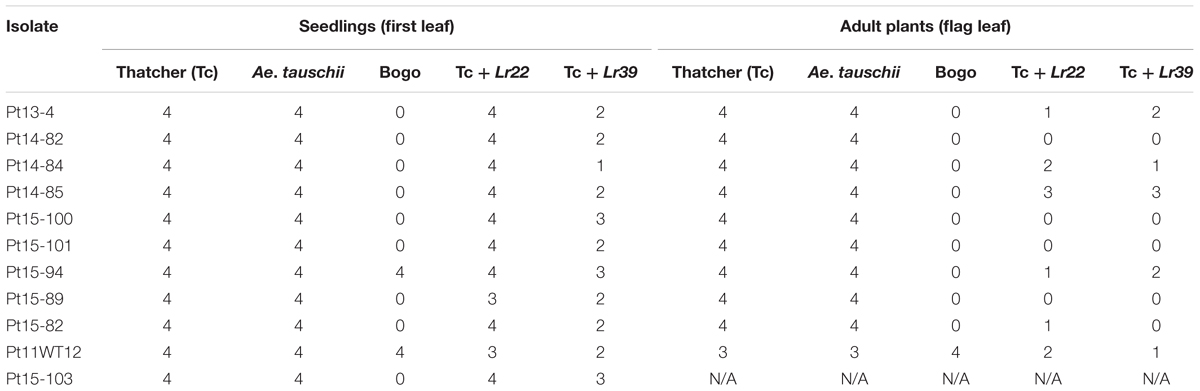
TABLE 1. Reaction of plant genotypes (scale 0–4, description in “Materials and Methods” section) at seedling and adult plant stage to inoculation with 11 isolates of P. triticina.
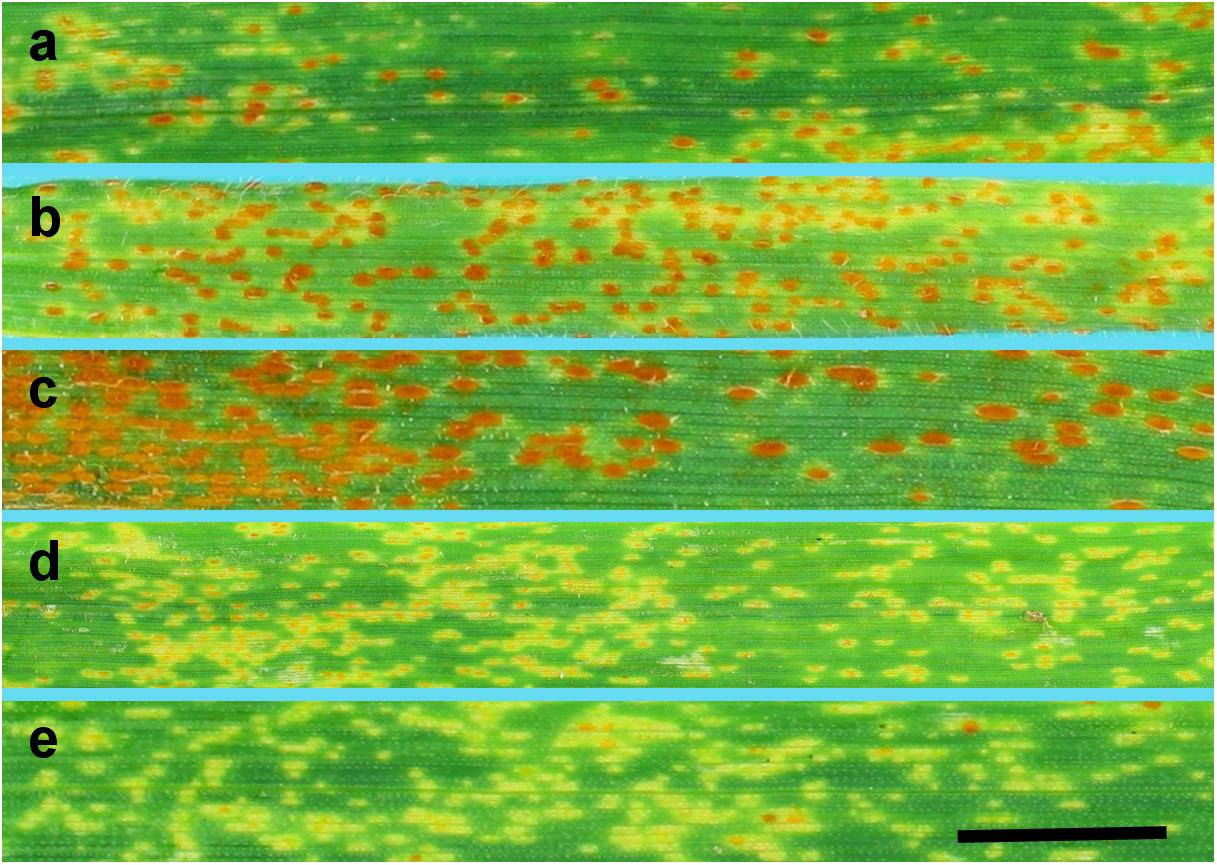
FIGURE 2. Macroscopic observation of the infection rates (8 days) after inoculation of control plants with Pt11WT12 (isolate 4) at the adult plant stage (flag leaf). Wheat cv. Thatcher (rated as “3”) (a), Ae. tauschii (rated as “3”) (b), triticale cv. Bogo (rated as “4”) (c), Tc + Lr22a (rated as “2”) (d), Tc + Lr39 (rated as “1”) (e). Scale bar 1 cm.
Macroscopic Analysis of Leaf Rust Infection
The macroscopic evaluation of infection rates was performed 8 days after inoculation for three isolates from the JKI collection (Isolates 1–3), and one isolate from the collection of PBAI-NRI (Isolate 4) for seedlings and adult plants (Supplementary Tables S1, S2). On the basis of two-way ANOVA we observed significant differences at P-value lower than 0.05, between classification factors, i.e., a homogenous groups of genotypes (hybrids and controls) and stage of development (seedling and adult plants). In case of homogenous groups of genotypes we observed significant differences for all four isolates. We identified significant differences between stage of development for isolates 1, 2, and 3. ANOVA did not show significant differences in the interaction between homogenous groups of genotypes and stage of development. The infection rate of isolates 1 and 2 was higher at the seedling stage in comparison with the adult plant stage (mean 0.98–0.43 for isolate 1 and 1.05–0.76 for isolate 2 in hybrid plants). The same was observed for isolate 3 at the adult plant stage (mean 0.69–1.79 in hybrid plants). Isolate 4 was the most aggressive (mean 3.02 for hybrids), whereas isolate 1 was the least aggressive for all genotypes (mean 0.70 for hybrids) (Figure 3). The selection of the most resistant genotypes on the basis of macroscopic rates, was difficult, because of the similarities of infection rates obtained. Only in case of isolate 4, four genotypes with a lower rate (3 instead of 4) at both analyzed stages of development were selected: Hybrid genotype 1 (Figure 4g), Hybrid genotype 2, Hybrid genotype 11 and Hybrid genotype 14. The reduced infection rate was identified also for Ae. tauschii, amphiploids (Ae tauschii × S. cereale) and the two NILs (Supplementary Tables S1, S2). Ae. tauschii plants were characterized by high infection rates at both stages and for all used isolates (Supplementary Tables S1, S2). For amphiploid forms, we identified a similar level of infection in comparison to the mean infection rate obtained for hybrid plants, however, in case of isolate 4, amphiploids were less infected (Figure 3 and Supplementary Tables S1, S2). The rating of triticale cv. Bogo revealed no significant differences to the hybrids. We observed no dependence between generations of hybrid plants and resistance. However, among four outstanding genotypes with the higher resistance, two of them were from the earliest generation BC2F4. Infection rates established for adult control plants were lower in comparison to the infection rates evaluated at the seedling stage for this group (Figure 3 and Supplementary Tables S1, S2).
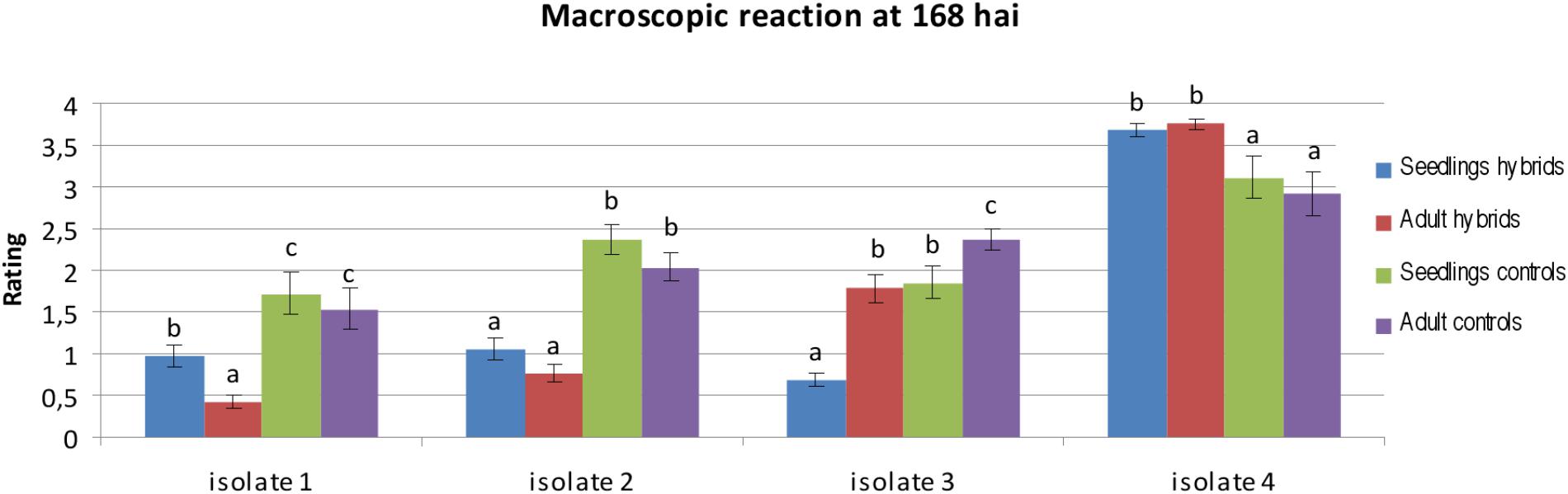
FIGURE 3. Statistical analysis of macroscopic reaction of hybrids carrying leaf rust resistance genes (hybrids) and control plants (controls) inoculated with single spore isolates at 168 hai at the seedling and adult plant stage. The bars represent a mean value (over replications) and error bars represent standard errors. The letters indicate homogenous groups of means that do not differ significantly at a significance level of 0.05 (Fisher’s LSD-test).
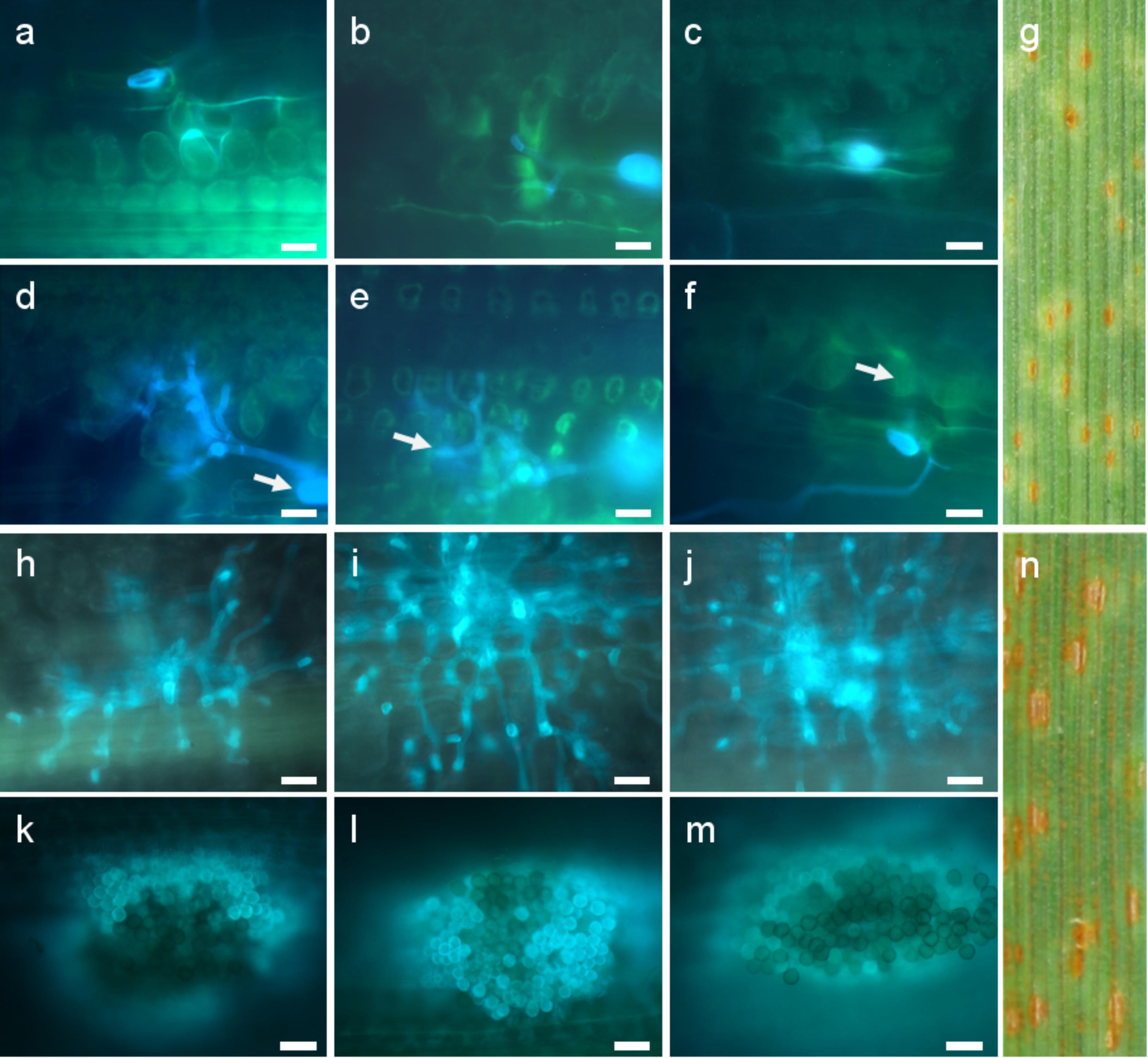
FIGURE 4. Development of fungal structures in the resistant hybrid genotype 1 (a–f) and the susceptible hybrid genotype 12 (h–m) after inoculation with isolate 1 (a,d,h,k), isolate 2 (b,e,i,l), and isolate 3 (c,f,j,m) at the seedling stage. Macroscopic symptoms on leaf segments 8 days after inoculation with isolate 4 (g,n) and microscopic observations 72 hai (a,b,c,h,i,j) and 168 hai (d,e,f,k,l,m) are compared. Arrows indicate substomatal vesicle (d), haustorial mother cell (e), and autofluorescence around infection sites (f). Scale bars 20 μm.
Microscopic Analysis of Fungal Structures Development
The infection level was determined on the basis of the number of HMCs at 72 hai and colonies with uredospore pustules per mm-2, 7 days after inoculation. Resistance level was estimated for seedlings and adult plants (Supplementary Tables S1, S2, respectively).
On the basis of two-way ANOVA we observed significant differences at P-value lower than 0.05, between classification factors, i.e., a homogenous groups of genotypes (hybrids and controls) and stage of development (seedling and adult plants). In case of homogenous groups of genotypes we observed significant differences for all three isolates. We identified significant differences between stage of development for isolates 1 and 2. ANOVA show significant differences in the interaction between homogenous groups of genotypes and stage of development for isolates 2 and 3. Obtained results revealed that hybrids and control plants at the adult plant stage were less infected than seedlings in case of isolates 1 and 2 (mean 0.00 to 1.81 for isolate 1 and 1.71 to 2.60 for isolate 2 in hybrid plants), whereas after inoculation with isolate 1 we identified no infection on the hybrid plants (Supplementary Table S1 and Figure 5). Hybrid genotype 1 (Figures 4a–f), Hybrid genotype 2, Hybrid genotype 11, and Hybrid genotype 14 were very resistant and Hybrid genotype 12 (Figures 4h–n) very susceptible to all three isolates at both stages of development (Supplementary Tables S1, S2). Two of the most resistant genotypes were from the earliest generation – BC2F4. Triticale cv. Bogo and the amphiploid Ae. tauschii × S. cereale were very resistant in contrast to Ae. tauschii and both susceptible standard wheat cultivars, i.e., Thatcher and Borenos. The number of HMC and uredospores in two genotypes with introgression of 2D chromatin but without resistance genes were similar to those obtained for triticale. Tc + Lr22a plants were more infected at the seedling stage than at the adult plant stage, especially in case of inoculation with isolate 2 (Supplementary Tables S1, S2). What is more, adult control plants were less infected than seedlings for all isolates (Figure 5).
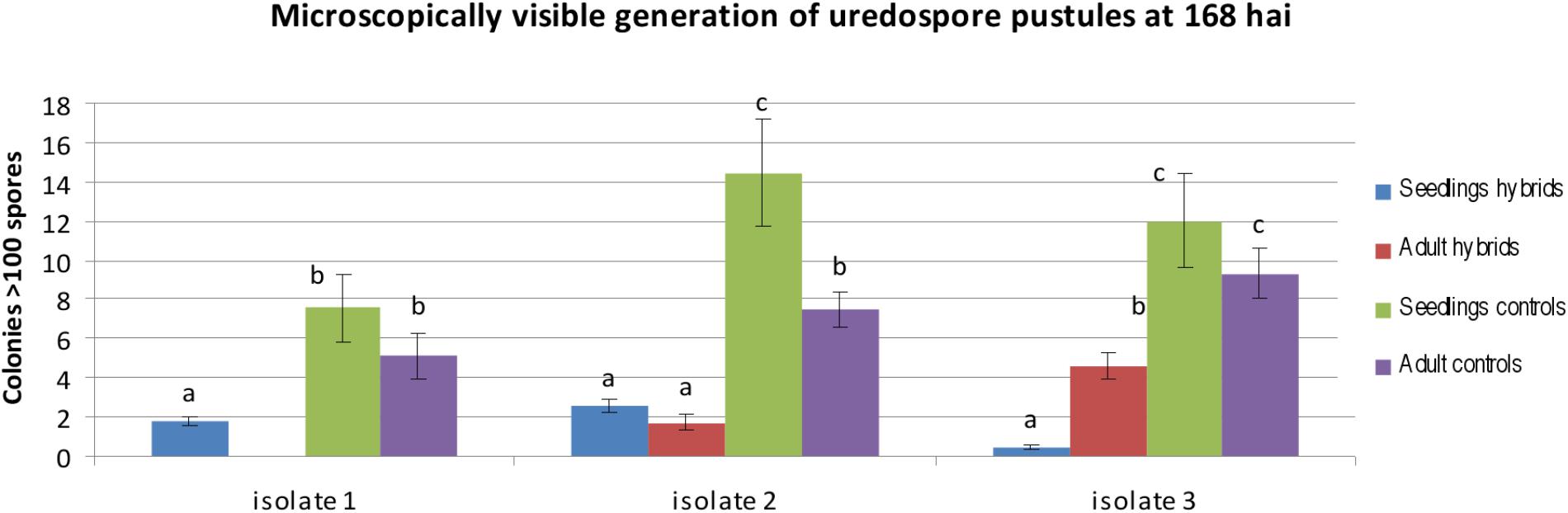
FIGURE 5. Statistical analysis of microscopically visible generation of uredospore pustules of hybrids carrying leaf rust resistance genes (hybrids) and control plants (controls) inoculated with single spore isolates at 168 hai at the seedling and adult plant stage. The bars represent a mean value (over replications) and error bars represent standard errors. The letters indicate homogenous groups of means that do not differ significantly at a significance level of 0.05 (Fisher’s LSD-test).
Production of DH Lines
A total of 5,634 anthers collected from three M2DA triticale plants (Hybrid genotype 1, 2, and 11) were used to produce 2,271 androgenic structures. The lowest number of androgenic structures (427) was obtained from the anthers of plant M2DA_1 (Hybrid genotype 1), followed by 796 androgenic structures obtained from plant M2DA_2 (Hybrid genotype 2), whereas the highest number of those structures (1,048) was obtained from anthers of plant M2DA_3 (Hybrid genotype 11). Overall, 61 haploid plants were obtained, of which 36 were generated from plant M2DA_3. After the colchicine treatment, 26 DH plants were generated, which were self-pollinated and harvested (Table 2). Flow cytometry experiments confirmed the ploidy level of androgenic plants, derived DH plants and control triticale cv. “Bogo.” The efficiency of androgenesis is presented in Table 2, whereas numbers of spikes and seeds of DH lines are presented in Table 3. We obtained 26 lines of DH among which all plants of two lines were characterized by the presence of two 2D chromosomes in the triticale background. One line was derived from the Hybrid genotype 1 and second line from the Hybrid genotype 2.

TABLE 2. The effectiveness of androgenesis induction and doubled haploid production from three monosomic 2D addition plants of triticale.
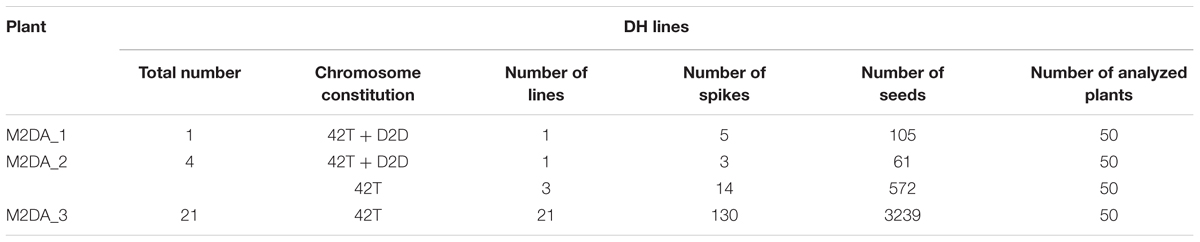
TABLE 3. Characterization of androgenesis induction and doubled haploid production from three monosomic 2D addition plant of triticale.
Discussion
Ae. tauschii is an excellent source of genes that could be used in breeding of Triticeae species, especially determining resistance to fungal diseases like leaf rust (Valkoun et al., 1985; Gill et al., 1986; Dhaliwal et al., 1991). The main goal of this work was to examine the resistance of (Ae. tauschii × S. cereale) × Triticosecale hybrids to leaf rust in inoculation tests with isolates of P. triticina at two stages of plant development. Macroscopic and microscopic analysis of infection were combined with molecular markers associated with leaf rust resistance genes and cytogenetic analysis of hybrid plants. Selected resistant genotypes were stabilized by the production of double haploids.
Allocation of chromosome 2D of Ae. tauschii (D51) in triticale background leads to the expression of genes determining resistance to powdery mildew, semi-dwarfism and spike morphology (Majka et al., 2016), whereas Kwiatek et al. (2015) reported a positive transfer of 3D chromosomes with Lr32 from Ae. tauschii into triticale cv. Bogo. The use of this wild species as a source of leaf rust resistance genes was confirmed by Majka et al. (2017). Besides the fact that triticale cv. Bogo was determined as a cultivar very resistant to leaf rust infection (Grzesik and Strzembicka, 2003), this resistance may break down in the next few years. In this respect, genotypes that carry complex disease resistance are most valuable. Thus, pyramidization of triticale resistance with seedling and APR resistance from Ae. tauschii would play a key role for the obtaining durable resistance in this species.
The exact resistance of triticale hybrids with the introgression of 2D chromosomes was determined in the inoculation tests, which were performed on seedlings and adult plants. The selection of isolates according to macroscopic observations of infection rates, showed that triticale cv. Bogo is very resistant (rate 0) to leaf rust (10/11 isolates) at both stages of development (Table 1) in accordance with previous reports (Grzesik and Strzembicka, 2003). Grzesik and Strzembicka (2003) revealed that in greenhouse and field trials, this cultivar was highly resistant to both isolates (65a/95 and 81c/95) used for inoculation. Only one isolate (isolate 4; Pt11WT12) undoubtedly was virulent to triticale cv. Bogo (rate 4) (Table 1). Inoculations of Ae. tauschii with P. triticina isolates demonstrated a high level of infection. It was very surprising because this species was believed as a source of leaf rust resistance, but in exceptional cases, like dry and cold winters, this species may not have seedling resistance (Kalia et al., 2017). In this work, the susceptibility of Ae. tauschii to all analyzed isolates of P. triticina may result from differences between the origin of this species and the origin of the isolates. It was reported that the Ae. tauschii reaction to P. triticina is variable with place of host origin (Assefa and Fehrmann, 2000). On the other hand, leaf rust symptoms have never been observed in natural stands of Aegilops species including Ae. tauschii (Anikster et al., 2005; Liu et al., 2014; Gultyaeva et al., 2016). However, infections can develop after inoculation with defined isolates of wheat leaf rust (Anikster et al., 2005), what is in accordance with results obtained in this study.
It was assumed that macroscopic observations of infection rates were not sufficient to determine the exact resistance of hybrid plants. However, general observations were consistent with the microscopic analyses, which were more informative and allowed the visualization of the pathogen structures. The histological investigations of host–pathogen interactions revealed that all analyzed genotypes were infected to a higher level at the seedling stage. Furthermore, a race-specific resistance of the obtained hybrids was observed. Isolate 1 (wxr77) was the least aggressive for all analyzed genotypes, especially at the adult plant stage. It was reported that with the exception of Lr47 this isolate was virulent against Lr genes located on the A-genome, i.e., Lr10, Lr11, Lr20, Lr28, Lr37, Lr49 (Serfling et al., 2016). Isolates 2 and 3 were determined as virulent for Lr22a and Lr39, respectively, which was confirmed in present study. According to macroscopic and microscopic results we observed that hybrid plants inoculated at the seedling stage with isolate 3 were less infected in comparison to inoculation with isolates 1 and 2 (Figures 3, 5). Similarly, hybrid plants inoculated at the adult plant stage with isolate 2 were characterized by less disease severity in comparison to results obtained with isolate 3. These findings indicate appropriate selection of the P. triticina isolates and that observed resistance is conferred by both genes introgressed with chromosome 2D. Variations in the resistance were observed also in both Thatcher NILs inoculated with the same isolates. The effectiveness of leaf rust resistance varies, depending on the combination of a particular race-specific Lr gene and a corresponding P. triticina avirulence gene (Bolton et al., 2008). According to Kalia et al. (2017) accessions of Ae. tauschii exhibiting high APR were found in Azerbaijan, followed by Iran and Afghanistan. The origin of Ae. tauschii accession D51 used in this study to obtain amphiploids and hybrid plants with triticale is unknown (Majka et al., 2017). Nevertheless, accessions with high APR are likely to carry either race-specific APR gene like Lr22a or a combination of two or more minor genes (Hiebert et al., 2007). It is also known that Lr22a is expressed only at the adult plant stage, however, the degree of resistance is comparable to Lr genes determining high resistance at the seedling stage in contrast to genes like Lr34, which confer the slow-rusting type APR (Hiebert et al., 2007). Our results revealed the decreased reaction of hybrid plants at the seedling stage, followed by the increase of resistance in further stages of development giving hint that obtained hybrid plants may especially exhibit APR resistance conferred by Lr22a introgressed from Ae. tauschii. On the basis of the macroscopic and microscopic analyses, this resistance can be determined as additive and race-specific to four selected isolates what is in accordance with previous assumptions (Hiebert et al., 2007; Kalia et al., 2017). So far, the resistance conferred by Lr22a was found in only three Canadian cultivars of spring wheat: AC Minto, 5500 HR and 5600 HR or breeding lines with AC Minto in their pedigree. Lr22a was introgressed from the AC Minto into susceptible Swiss cultivars CH Campala and CH Rubli with no associated negative effects (Fossati, personal communication). Because of the low exposure to P. triticina, this gene can be used for pyramiding of leaf rust resistance (Hiebert et al., 2007). The resistance conferred by this gene applied to triticale is reported for the first time in this study.
Seedling resistance gene Lr39 is linked to the marker Xgdm35 and the 180 bp fragment obtained for Ae. tauschii is reported to be indicative for resistance (Singh et al., 2012), whereas Xgwm296 is localized 2.9 cM from Lr22a (Hiebert et al., 2007). In this study, we confirmed the presence of both markers in all fourteen analyzed hybrid genotypes demonstrating their effectiveness in marker-assisted selection. Hence the simultaneous transfer of APR and seedling resistances genes, i.e., Lr22a and Lr39 into breeding lines reduces the vulnerability to a breakdown by virulent races within the leaf rust population which was reported for Lr39 by Kolmer and Anderson (2011) and other seedling resistances already.
According to macroscopic and microscopic results, we observed no dependence between generation of hybrid plants and resistance. Among four of the most resistant genotypes, two of them were from the earliest generation BC2F4. It is worth to mention, that DH lines obtained from genotypes 1 and 2 were characterized by the presence of two chromosomes of 2D, whereas genotype 11 is not. The effectiveness of in vitro cultures was determined as the number of plants compared to the number of anthers (Table 2). The number of green haploid plants ranged from eight for M2DA_1 (0.5 per 100 anthers) to 36 for M2DA_3 (1.6 per 100 anthers), and was low in comparison to previous reports regarding DHs production of triticale, which resulted from 0.4 to 7.9 green plants per 100 anthers (Ślusarkiewicz-Jarzina and Ponitka, 1997, 2003; Ponitka et al., 1999).
Conclusion
In conclusion, we selected four monosomic 2D addition triticale genotypes highly resistant to P. triticina infection at all stages of development. The level of resistance was determined in inoculation tests combined with macroscopic and microscopic observations of leaf rust infection. From the selected genotypes, we obtained 26 DH lines among which two lines with doubled additional chromosomes 2D of Ae. tauschii can be used for further breeding work to increase the agronomic value and the resistance against leaf rust at the seedling and adult plant stages of cultivated triticale.
Author Contributions
MM initiated project, designed the study, performed the FISH/GISH and molecular marker analysis, and wrote the manuscript. MM, MK, and HW obtained the hybrid plants. AŚ-J produced the DH plants. MM, AS, PC, and FO performed inoculation tests and analyzed the data. All authors agreed to be accountable for all aspects of the work in ensuring that questions related to the accuracy or integrity of any part of the work are appropriately investigated and resolved. All authors contributed and approved the final manuscript.
Funding
This work was funded by the National Science Centre (PRELUDIUM8-2014/15/N/NZ9/00448).
Conflict of Interest Statement
The authors declare that the research was conducted in the absence of any commercial or financial relationships that could be construed as a potential conflict of interest.
Acknowledgments
We gratefully acknowledge the technical assistance of Mrs. Jolanta Belter, Mrs. Hanna Pudelska, Mr. Grzegorz Czajowski, and Mr. Nico Pastor-Kaeppner.
Supplementary Material
The Supplementary Material for this article can be found online at: https://www.frontiersin.org/articles/10.3389/fpls.2018.01418/full#supplementary-material
References
Anikster, A. C., Manisterski, J., Long, D. L., and Leonard, K. J. (2005). Resistance to leaf rust, stripe rust, and stem rust in the Aegilops species in Israel. Plant Dis. 89, 303–308. doi: 10.1094/PD-89-0303
Apolinarska, B., Wiśniewska, H., and Wojciechowska, B. (2010). Aegilops-rye amphiploids and substitution rye used for introgression of genetic material into rye (Secale cereale L.). J. Appl. Genet. 51, 413–420. doi: 10.1007/BF03208871
Assefa, S., and Fehrmann, H. (2000). Resistance to wheat leaf rust in Aegilops tauschii Coss. and inheritance of resistance in hexaploid wheat. Genet. Resour. Crop Evol. 47, 135–140. doi: 10.1023/A:1008770226330
Assefa, S., and Fehrmann, H. (2004). Evaluation of Aegilops tauschii Coss. for resistance to wheat stem rust and inheritance of resistance genes in hexaploid wheat. Genet. Resour. Crop Evol. 51, 663–669. doi: 10.1023/B:GRES.0000024657.20898.ed
Bolton, M. D., Kolmer, J. A., and Garvin, D. F. (2008). Wheat leaf rust caused by Puccinia triticina. Mol. Plant Pathol. 9, 563–576. doi: 10.1111/j.1364-3703.2008.00487.x
Chen, W., Liu, T., and Gao, L. (2013). Suppression of stripe rust and leaf rust resistances in interspecific crosses of wheat. Euphytica 192, 339–346. doi: 10.1007/s10681-012-0854-2
Chu, C. C., Wang, C. S., Sun, C. S., Hus, J.-C., Ying, K. C., Chu, C. Y., et al. (1975). Establishment of an efficient medium for anther culture of rice through comparative experiments on the nitrogen sources. Sci. Sin. 18, 659–668.
Cox, T. S., Raupp, W. J., and Gill, B. S. (1994). Leaf rust-resistance genes Lr41, Lr42, and Lr43 transferred from Triticum tauschii to common wheat. Crop Sci. 34, 339–343. doi: 10.2135/cropsci1994.0011183X003400020005x
Cox, T. S., Raupp, W. J., Wilson, D. L., Gill, B. S., Leath, S., Bockus, W. W., et al. (1992). Resistance to foliar diseases in a collection of T. tauschii germplasm. Plant Dis. 76, 1061–1064. doi: 10.1094/PD-76-1061
Cuadrado, A., and Jouve, N. (1994). Mapping and organization of highly repeated DNA sequences by means of simultaneous and sequential FISH and C-banding in 6x-triticale. Chromosome Res. 2, 331–338. doi: 10.1007/BF01552727
Dean, R., Van Kan, J. A. L., Pretorius, Z. A., Hammond-Kosack, K. E., Di Pietro, A., Spanu, P. D., et al. (2012). The top 10 fungal pathogens in molecular plant pathology. Mol. Plant Pathol. 13, 414–430. doi: 10.1111/j.1364-3703.2011.00783.x
Dhaliwal, H. S., Singh, H., Gupta, S., Bagga, P. S., and Gill, K. S. (1991). Evaluation of Aegilops and wild Triticum species for resistance to leaf rust (Puccinia recondita f. sp. tritici) of wheat. Int. J. Trop. Agric. 9, 118–122.
Dyck, P. L., and Kerber, E. R. (1985). “Resistance of the race-specific type,” in ‘The Cereal Rusts, Vol. II’, eds A. P. Roelfs and W. R. Bushnell (London: Academic Press), 469–500.
Frankel, O. H., and Bennett, E. (1970). “Genetic resources in plants - their exploration and conservation,” in International Biological Programme Handbook No. 11, eds O. H. Frankel and E. Bennett (Edinburgh: Blackwell).
Gill, B. S., Raupp, W. J., Sharma, H. C., Browder, L. E., Hatchett, J. H., Harvey, T. L., et al. (1986). Resistance in Aegilops squarrosa to wheat leaf rust, wheat powdery mildew, greenbug, and Hessian fly. Plant Dis. 70, 553–556. doi: 10.1094/PD-70-553
Grzesik, H., and Strzembicka, A. (2003). Resistance of some winter triticale varieties to leaf rust (Puccinia recondita f. sp. tritici). Biul. Hodowli Aklimatyzacji Roślin 230, 171–175.
Gultyaeva, E. I., Shaydayuk, E. L., Goncharov, N. P., Akhumetova, A., Abdullaev, M. H., and Kosman, E. (2016). Virulence of Puccinia triticina on Triticum and Aegilops species. Australas. Plant Pathol. 45, 155–163. doi: 10.1007/s13313-016-0395-6
Hasterok, R., Dulawa, J., Jenkins, G., Leggett, M., and Langdon, T. (2006). Multisubstrate chromosome preparations for high throughput comparative FISH. BMC Biotechnol. 6:20. doi: 10.1186/1472-6750-6-20
Heslop-Harrison, J. S. (2000). Comparative genome organization in plants: from sequence and markers to chromatin and chromosomes. Plant Cell 12, 617–635. doi: 10.1105/tpc.12.5.617
Hiebert, C. W., Thomas, J. B., Somers, D. J., McCallum, B. D., and Fox, S. L. (2007). Microsatellite mapping of adult-plant leaf rust resistance gene Lr22a in wheat. Theor. Appl. Genet. 115, 877–884. doi: 10.1007/s00122-007-0604-3
Hoogkamp, T. J., Chen, H. W. Q., and Niks, R. E. (1998). Specificity of prehaustorial resistance to Puccinia hordei and to two inappropriate rust fungi in barley. Phytopathology 88, 856–861. doi: 10.1094/PHYTO.1998.88.8.856
Huerta-Espino, J., Singh, R. P., Germán, S., McCallum, B. D., Park, R. F., Chen, W. Q., et al. (2011). Global status of wheat leaf rust caused by Puccinia triticina. Euphytica 179, 143–160. doi: 10.1007/s10681-011-0361-x
Jia, J., Zhao, S., Kong, X., Li, Y., Zhao, G., He, W., et al. (2013). Aegilops tauschii draft genome sequence reveals a gene repertoire for wheat adaptation. Nature 496, 91–95. doi: 10.1038/nature12028
Kalia, B., Wilson, D. L., Bowden, R. L., Singh, R. P., and Gill, B. S. (2017). Adult plant resistance to Puccinia triticina in a geographically diverse collection of Aegilops tauschii. Genet. Resour. Crop Evol. 64, 913–926. doi: 10.1007/s10722-016-0411-2
Kerber, E. R. (1987). Resistance to leaf rust in hexaploid wheat: Lr32 a third gene derived from Triticum tauschii. Crop Sci. 27, 204–206. doi: 10.2135/cropsci1987.0011183X002700020013x
Kolmer, J. A. (1996). Genetics of resistance to wheat leaf rust. Annu. Rev. Phytopathol. 34, 435–455. doi: 10.1146/annurev.phyto.34.1.435
Kolmer, J. A., and Anderson, J. A. (2011). First detection in North America of virulence in wheat leaf rust (Puccinia triticina) to seedling plants of wheat with Lr21. Plant Dis. 95, 1032. doi: 10.1094/PDIS-04-11-0275
Kolmer, J. A., and Hughes, M. E. (2017). Physiologic specialization of Puccinia triticina on wheat in the United States in 2015. Plant Dis. 101, 1968–1973. doi: 10.1094/PDIS-02-17-0200-SR
Komuro, S., Endo, R., Shikata, K., and Kato, A. (2013). Genomic and chromosomal distribution patterns of various repeated DNA sequences in wheat revealed by a fluorescence in situ hybridization procedure. Genome 56, 131–137. doi: 10.1139/gen-2013-0003
Kwiatek, M., Belter, J., Majka, M., and Wiśniewska, H. (2016a). Allocation of the S-genome chromosomes of Aegilops variabilis Eig. carrying powdery mildew resistance in triticale ( × Triticosecale Wittmack). Protoplasma 253, 329–343. doi: 10.1007/s00709-015-0813-6
Kwiatek, M., Majka, M., Majka, J., Belter, J., Suchowilska, E., Wachowska, U., et al. (2016b). Intraspecific polymorphisms of cytogenetic markers mapped on chromosomes of Triticum polonicum L. PLoS One 11:e0158883. doi: 10.1371/journal.pone.0158883
Kwiatek, M., Majka, M., Wiśniewska, H., Apolinarska, B., and Belter, J. (2015). Effective transfer of chromosomes carrying leaf resistance genes from Aegilops tauschii Coss. into hexaploid triticale (X Triticosecale Witt.) using Ae. tauschii × Secale cereale amphiploid form. J. Appl. Genet. 56, 163–168. doi: 10.1007/s13353-014-0264-3
Lagudah, E. S., McFadden, H., Singh, R. P., Huerta-Espino, J., Bariana, H. S., and Spielmeyer, W. (2006). Molecular genetic characterization of the Lr34/Yr18 slow rusting resistance gene region in wheat. Theor. Appl. Genet. 114, 21–30. doi: 10.1007/s00122-006-0406-z
Liu, M., Rodrigue, N., and Kolmer, J. (2014). Population divergence in the wheat leaf rust fungus Puccinia triticina is correlated with wheat evolution. Heredity 112, 443–453. doi: 10.1038/hdy.2013.123
Majka, M., Kwiatek, M., Belter, J., and Wiśniewska, H. (2016). Characterization of morphology and resistance to Blumeria graminis of winter triticale monosomic addition lines with chromosome 2D of Aegilops tauschii. Plant Cell Rep. 35, 2125–2135. doi: 10.1007/s00299-016-2023-x
Majka, M., Kwiatek, M. T., Majka, J., and Wiśniewska, H. (2017). Aegilops tauschii accessions with geographically diverse origin show differences in chromosome organization and polymorphism of molecular markers linked to leaf rust and powdery mildew resistance genes. Front. Plant Sci. 8:1149. doi: 10.3389/fpls.2017.01149
McIntosh, R. A., Wellings, C. R., and Park, R. F. (1995). Wheat Rusts: An Atlas of Resistance Genes. Melbourne: CSIRO Publishing. doi: 10.1007/978-94-011-0083-0
Murovec, J., and Bohanec, B. (2012). “Haploids and doubled haploids in plant breeding,” in Plant Breeding, ed. I. Y. Abdurakhmonov (Rijeka: INTECH).
Park, R. F., and McIntosh, R. A. (1994). Adult plant resistances to Puccinia recondita f.sp. tritici in wheat. N. Z. J. Crop Horticult. Sci. 22, 151–158. doi: 10.1080/01140671.1994.9513819
Pestsova, E., Ganal, M. W., and Roder, M. S. (2000). Isolation and mapping of microsatellite markers specific for the D genome of bread wheat. Genome 43, 689–697. doi: 10.1139/g00-042
Ponitka, A., Ślusarkiewicz-Jarzina, A., Wêdzony, M., Barcińska, I., and Woźna, J. (1999). The influence of various in vitro culture conditions on androgenetic embryo induction and plant regeneration from hexaploid triticale ( × Triticosecale Wittm.). J. Appl. Genet. 40, 165–174.
Raupp, W. J., Sukhwinder-Singh, Brown-Guedira, G. L., and Gill, B. S. (2001). Cytogenetic and molecular mapping of the leaf rust resistance gene Lr39 in wheat. Theor. Appl. Genet. 102, 347–352. doi: 10.1007/s001220051652
Roder, M. S., Korzun, V., Wendehake, K., Plaschke, J., Tixier, M.-H., Leroy, P., et al. (1998). A microsatellite map of wheat. Genetics 149, 2007–2023.
Roelfs, A. P., Singh, R. P., and Saari, E. E. (1992). Rust Diseases of Wheat: Concepts and Methods of Disease Management. Mexico: CIMMYT.
Rohringer, R., Kim, W. K., Samborski, D. J., and Howes, N. K. (1977). Calcofluor: an optical brightener for fluorescence microscopy of fungal plant parasites in leaves. Phytopathology 67, 808–810. doi: 10.1094/Phyto-67-808
Rowland, G. G., and Kerber, E. R. (1974). Telocentric mapping in hexaploid wheat of genes for leaf rust resistance and other characters derived from Aegilops squarrosa. Can. J. Genet. Cytol. 16, 137–144. doi: 10.1139/g74-013
Schneider, A., Molnar, I., and Molnar-Lang, M. (2008). Utilization of Aegilops (goatgrass) species to widen the genetic diversity of cultivated wheat. Euphytica 163, 1–19. doi: 10.1007/s10681-007-9624-y
Serfling, A., Templer, S. E., Winter, P., and Ordon, F. (2016). Microscopic and molecular characterization of the prehaustorial resistance against wheat leaf rust (Puccinia triticina) in Einkorn (Triticum monococcum). Front. Plant Sci. 7:1668. doi: 10.3389/fpls.2016.01668
Singh, S., Chahal, G. S., Singh, P. K., and Gill, B. S. (2012). Discovery of desirable genes in the germplasm pool of Aegilops tauschii Coss. Indian J. Genet. 72, 271–277.
Ślusarkiewicz-Jarzina, A., and Ponitka, A. (1997). Effect of genotype and media composition on embryoid induction and plant regeneration from anther culture in triticale. J. Appl. Genet. 38, 253–258.
Ślusarkiewicz-Jarzina, A., and Ponitka, A. (2003). Efficient production of spontaneous and induced doubled haploid triticale plants derived from anther culture. Cereal Res. Commun. 31, 289–296.
Ślusarkiewicz-Jarzina, A., Pudelska, H., Woźna, J., and Pniewski, T. (2017). Improved production of doubled haploids of winter and spring triticale hybrids via combination of colchicine treatments on anthers and regenerated plants. J. Appl. Genet. 58, 287–295. doi: 10.1007/s13353-016-0387-9
Thind, K. T., Wicker, T., Simkova, H., Fossati, D., Moullet, O., Brabant, C., et al. (2017). Rapid cloning of genes in hexaploid wheat using cultivar-specific long-range chromosome assembly. Nat. Biotech. 35, 793–796. doi: 10.1038/nbt.3877
Valkoun, J., Hammer, K., Kucerova, D., and Bartos, P. (1985). Disease resistance in the genus Aegilops L.– stem rust, leaf rust, stripe rust, and powdery mildew. Kulturpflanze 33, 133–153.
Wang, P., and Chen, Y. (1983). Preliminary study on prediction of height of pollen H2 generation in winter wheat grown in the field. Acta Agron. Sin. 9, 283–284. doi: 10.1007/s13353-016-0387-9
Keywords: adult plant resistance, Aegilops tauschii, doubled haploids, leaf rust, marker-assisted selection, Puccinia triticina, seedling resistance, triticale
Citation: Majka M, Serfling A, Czembor P, Ślusarkiewicz-Jarzina A, Kwiatek MT, Ordon F and Wiśniewska H (2018) Resistance of (Aegilops tauschii × Secale cereale) × Triticosecale Hybrids to Leaf Rust (Puccinia triticina) Determined on the Macroscopic and Microscopic Level. Front. Plant Sci. 9:1418. doi: 10.3389/fpls.2018.01418
Received: 17 May 2018; Accepted: 06 September 2018;
Published: 26 September 2018.
Edited by:
Luigi Cattivelli, Consiglio per la ricerca in agricoltura e l’analisi dell’economia agraria (CREA), ItalyReviewed by:
Pablo Daniel Olivera, University of Minnesota Twin Cities, United StatesSambasivam Periyannan, Commonwealth Scientific and Industrial Research Organisation (CSIRO), Australia
Copyright © 2018 Majka, Serfling, Czembor, Ślusarkiewicz-Jarzina, Kwiatek, Ordon and Wiśniewska. This is an open-access article distributed under the terms of the Creative Commons Attribution License (CC BY). The use, distribution or reproduction in other forums is permitted, provided the original author(s) and the copyright owner(s) are credited and that the original publication in this journal is cited, in accordance with accepted academic practice. No use, distribution or reproduction is permitted which does not comply with these terms.
*Correspondence: Maciej Majka, mmaj@igr.poznan.pl
†Present address: Michał Tomasz Kwiatek, Department of Genetics and Plant Breeding, Poznań University of Life Sciences, Poznań, Poland