- 1Instituto de la Grasa (IG-CSIC), Seville, Spain
- 2Estación Experimental de Aula Dei (EEAD-CSIC), Zaragoza, Spain
In higher plants, the stearoyl-acyl carrier protein desaturase (SAD) catalyzes the first desaturation step leading to oleic acid, which can be further desaturated to linoleic and α-linolenic acids. Therefore, SAD plays an essential role in determining the overall content of unsaturated fatty acids (UFA). We have investigated how SAD genes expression and UFA composition are regulated in olive (Olea europaea) mesocarp tissue from Picual and Arbequina cultivars in response to different abiotic stresses. The results showed that olive SAD genes are transcriptionally regulated by temperature, darkness and wounding. The increase in SAD genes expression levels observed in Picual mesocarp exposed to low temperature brought about a modification in the UFA content of microsomal membrane lipids. In addition, darkness caused the down-regulation of SAD genes transcripts, together with a decrease in the UFA content of chloroplast lipids. The differential role of olive SAD genes in the wounding response was also demonstrated. These data point out that different environmental stresses can modify the UFA composition of olive mesocarp through the transcriptional regulation of SAD genes, affecting olive oil quality.
Introduction
Unsaturated fatty acids not only serve as a major source of reserve energy in the form of triacylglycerols, but also constitute complex lipids that are essential components of cellular membranes. In plants, an increasing number of studies has also proposed UFA and their derivatives as signaling molecules, which are involved in the response to biotic and abiotic stresses (Kachroo and Kachroo, 2009).
In higher plants, de novo fatty acid biosynthesis starts in the plastid by successive addition of two carbon atoms from acetyl-CoA, mainly leading to the synthesis of palmitoyl-acyl carrier protein (palmitoyl-ACP) and stearoyl-ACP (Harwood, 2005). These products, which can be desaturated in different cellular compartments, are the source of most of the fatty acids present in plant lipids. The first desaturation takes place in the plastid by the action of the Δ9 stearoyl-ACP desaturase (SAD), which produces oleoyl-ACP, using ferredoxin as electron donor. The oleoyl-ACP is then cleaved by specific thioesterases to free fatty acids, which are then incorporated into glycerolipids, where can be further desaturated to linoleic and α-linolenic acid by membrane-bound fatty acid desaturases, that differ in their cellular localization, lipid substrates, and electron donor system (Shanklin and Cahoon, 1998). The microsomal oleate desaturase (FAD2) and linoleate desaturase (FAD3) are located in the endoplasmic reticulum, use phospholipids as acyl substrates and NADH, NADH-cytochrome b5 reductase and cytochrome b5 as electron donor system. On the other hand, the plastidial oleate desaturase (FAD6) and linoleate desaturase (FAD7/8) are located in the plastid, use glycolipids as acyl carriers and NAD(P)H, ferredoxin-NAD(P) reductase and ferredoxin as electron donor system (Supplementary Figure S1).
Plant fatty acid desaturases are regulated by different environmental and physical stresses. Temperature is one of the main environmental factors affecting fatty acid desaturases. The plants ability to adjust membrane lipid fluidity in response to temperature by changing the levels of UFA is allowed by the regulated activity of fatty acid desaturases (Iba, 2002; Upchurch, 2008). Several mechanisms have been described to explain how temperature regulates fatty acids desaturation, including transcriptional (Kargiotidou et al., 2008) and post-transcriptional regulation (Matsuda et al., 2005), and through the effect of temperature on oxygen availability (Rolletschek et al., 2007). Light is a second environmental factor regulating fatty acid desaturation. An increase in polyunsaturated fatty acids have been reported in cucumber cotyledons (Murphy and Stumpf, 1979), oat leaves (Ohnishi and Yamada, 1983), and Arabidopsis callus cultures (Brockman et al., 1990) exposed to light. Regarding the molecular mechanism by which light regulates fatty acid desaturase, transcriptional (Kargiotidou et al., 2008) and post-translational (Collados et al., 2006) regulation have been reported. Plant fatty acid desaturases are also affected by wounding or pathogen attack. In fact, plants have evolved multiple mechanism to defend themselves against pathogens. It has been widely described the key role of polyunsaturated fatty acids in plants defense response, mainly as precursor of signal molecules such as jasmonic acid (Farmer, 1994). Accordingly, the transient induction of fatty acid desaturases in response to wounding has been observed in several plants (Hamada et al., 1996; Nishiuchi et al., 1997).
Olive (Olea europaea L.) is one of the first plants to be cultivated for oil production, and olive oil is the one with most impact in the Mediterranean region either at the economic, social and cultural levels (Baldoni et al., 2009). Virgin olive oil is a natural fruit juice, highly enriched in oleic acid (55–83%), while linoleic acid accounts for 3.5–21%, and linolenic acid for less than 1%. The relative contents of these UFA depends mainly on the olive cultivar, but also on pedoclimatic and culture conditions (Beltrán et al., 2004), affecting the nutritional (Perona et al., 2005) and technological properties (Aparicio et al., 1999) of the oil, and, therefore, the olive oil quality. In olive, three genes encoding SAD have been reported (Haralampidis et al., 1998; Parvini et al., 2016). SAD2 was highly expressed in mesocarp and seed, whereas the transcript of SAD3 was mainly detected in young drupes and leaves. In contrast, SAD1 expression levels remained low in all tissues studied. In addition, SAD2 gene has been suggested as the main contributor to oleic acid synthesis in olive mesocarp (Parvini et al., 2016). Regarding membrane desaturases, two genes encoding microsomal (Hernández et al., 2005) and one plastidial (Banilas et al., 2005; Hernández et al., 2011) oleate desaturases have been described, identifying FAD2-2 as the main gene responsible for the linoleic acid accumulation in olive mesocarp (Hernández et al., 2009). Finally, four genes encoding linoleate desaturases have been reported, two microsomal (Banilas et al., 2007; Hernández et al., 2016) and two plastidial (Poghosyan et al., 1999; Hernández et al., 2016), with FAD7-1 and FAD7-2 genes being suggested to contribute mostly to the linolenic acid present in the olive mesocarp (Hernández et al., 2016).
Early studies using olive callus cultures revealed that FAD2 is regulated by temperature and light intensity, while FAD7 is affected by high temperature (Hernández et al., 2008). More recently, transcriptional analysis conducted on olive mesocarp exposed to low and high temperatures demonstrated the role of FAD2, FAD6, and FAD7 genes in regulating UFA levels in order to maintain the fluidity of the biological membranes (Hernández et al., 2011; Matteucci et al., 2011; D’Angeli et al., 2013). In the same way, RNAseq analysis has shown that FAD2-2 gene increased its expression in olive leaves in response to cold (Leyva-Pérez et al., 2015). Furthermore, Hernández et al. (2011) observed a decrease in oleate desaturase genes expression levels when the olive fruit were incubated under darkness conditions. On the other hand, a transient induction of oleate desaturases, together with a slight increase in linoleic acid and the appearance of palmitolinoleic acid, have been reported in olive mesocarp subjected to wounding (Hernández et al., 2011) (Supplementary Figure S1). In addition, Padilla et al. (2012, 2014) described the transient induction of 13- and 9-lipoxygenases, and 13-hydroperoxide lyase in mechanically damaged olive mesocarp. These results showed the involvement of olive oleate desaturases in plant defense response providing the substrates to the different lipoxygenases pathways, including that of jasmonic acid synthesis (Weber, 2002). Not only that, a transcriptomic approach has also been performed to study the molecular interaction between the olive fruit fly (Bactrocera oleae) and tolerant and susceptible olive cultivars (Corrado et al., 2012; Grasso et al., 2017).
However, with the exception of a recent study on the effect of irrigation in desaturase gene expression of olive mesocarp (Hernández et al., 2018), there is still scarce information about the regulation of SAD genes in response to environmental stresses in plant tissues and, even more, in the case of the mesocarp of oil fruits. This tissue possesses the remarkable characteristic of having a high proportion of active chloroplasts together with a high amount of oil (Sánchez, 1994). In addition, SAD is of particular interest because is a key determinant of the overall level of fatty acid desaturation (Shanklin and Somerville, 1991), since this enzyme carries out the first desaturation step leading to oleic acid, which can be further desaturated to linoleic acid and α-linolenic acid. Therefore, it has a significant effect on the fluidity and rigidity of membrane system and the relationship of this to the adaption of plants to various environmental conditions.
For these reasons, we have studied in this work the transcriptional regulation of SAD genes in olive fruit remaining in branches incubated under different abiotic stresses, together with its impact at the metabolite level on the UFA content in different lipid classes of mesocarp tissue from Picual and Arbequina cultivars.
Materials and Methods
Plant Material and Stress Treatments
Olive (Olea europaea L. cv. Picual and Arbequina) trees were grown in the experimental orchard of Instituto de la Grasa, Seville (Spain), with drip irrigation and fertirrigation from the time of complete flowering to fruit ripening.
Four olive branches carrying 100 olive fruit at 28 WAF (turning stage) each one, were collected from different olive trees located in the same field, and transferred to growth chamber where they were incubated at 25°C with a 12 h light/12 h dark cycle, with a light intensity of 300 μmol m−2 s−1. These incubation parameters attempted to simulate physiological conditions of the tree, and were considered the standard conditions. No significant alterations in the fatty acid composition or SAD genes expression levels were observed in the mesocarp tissue when olive fruits were incubated under the above mentioned standard conditions (Supplementary Figure S2). For stress treatments, a new set of four olive branches with the same characteristics as previously mentioned were collected from the different olive trees and transferred to growth chamber, where the described standard conditions were altered to achieve the stress conditions to be studied. To examine the effect of temperature, the olive branches containing the fruits were incubated at 15°C for the low temperature experiment and 35°C for the high temperature one, at the same standard light intensity. For darkness treatment, light was turned off maintaining the same standard temperature. To study the effect of wounding, the whole surface of the olive fruit was mechanically damaged at zero time exerting pressure using tweezers with serrated tips, so that mesocarp tissue was affected. Each experiment corresponding to a different treatment was carried out at a different day of the same week, to ensure that the olive fruits of the different experiments were in the same stage (28 WAF). Zero time was designated 2 h after the beginning of the light period in every experiment, in order to maintain the natural photoperiod day/night of the olive fruit. At the indicated times, 10 olive fruits were taken from each olive branch, then 1–2 g of olive mesocarp was collected from 5 different olive fruits for RNA isolation, and 1.5 g was collected from the other 5 different olive fruits for lipid analysis. Therefore, in each experiment we used approximately 60 olive fruits from three olive branches, one branch for each biological replicate. In all experiments we incubated four branches, so that in case that we had a problem with a branch, to ensure we had another branch with olives and we could continue with the experiment. Olive mesocarp samples were frozen in liquid nitrogen and stored at −80°C.
Total RNA Extraction and cDNA Synthesis
Total RNA isolation was carried out according to Hernández et al. (2005) using 1–2 g of frozen olive fruit mesocarp tissue collected from at least five different olive fruit per each of the three biological replicates. Briefly, the frozen olive mesocarp was ground in a pre-cooled mortar with liquid nitrogen and homogenized with the extraction buffer, containing Tris-HCl, NaCl, Na2EDTA and SDS, and 2-mercaptoethanol. Afterward, nucleic acids were extracted with phenol/chloroform twice, and precipitated with NaAc and ethanol. The nucleic acid pellet was resuspended in DEPC treated water and LiCl was added to precipitate the RNA. The pellet was washed twice with 70% ethanol and resuspended in 25 μl DEPC-water. RNA quality verification, removal of contaminating DNA and cDNA synthesis were performed as described by Hernández et al. (2009), using the TURBO DNA-free kit (Ambion, United States) and SuperScript III First-Strand Synthesis System (Invitrogen, Carlsbad, CA, United States) with oligo (dT)20.
Quantitative Real Time-PCR (qRT-PCR)
Gene expression analysis was performed by quantitative real time PCR (qRT-PCR) using a CFX Connect real-time PCR System and iTaq Universal SYBR Green Supermix (Bio-Rad, Hercules, CA, United States). Primers for gene-specific amplification were described by Parvini et al. (2016) and are shown in Supplementary Table S1. Reaction mix (10 μL per well) contained 1X iTaq-QPCR Master Mix, 100 nM forward and reverse primers, and 2 μL of cDNA of appropriate dilution, which was selected according to the primers amplification efficiency. The thermal cycling conditions included an initial denaturation step of 95°C for 10 min, followed by 40 cycles of 95°C for 30 s, 60°C for 1 min, and 72°C for 30 s. The melting reaction from 55°C through 95°C, at 0.1°C s−1, following the final step of the PCR, was used to examine the specificity of the PCR amplification and the presence of primer dimers. Additionally, the purity of the PCR products were also checked by agarose gel electrophoresis. PCR efficiencies (E) of all primers were calculated using dilution curves with eight dilution points, twofold dilution, and the equation E = [10(−1/slope)] – 1. For normalization of the data, the olive ubiquitin2 gene (OeUBQ2, AF429430) was used as an endogenous reference. The qRT-PCR data were calibrated relative to the corresponding gene expression level at zero time for each treatment and cultivar, following the relative quantification by the 2−ΔΔCt method (Livak and Schmittgen, 2001). The data are presented as means ± SD of the three biological replicates, each having two technical replicates per 96 well plate.
Lipid Extraction and Fatty Acid Analysis
For lipid analysis, 1.5 g of frozen olive mesocarp tissue collected from at least five different olive fruits per each of the three biological replicate, were used. Olive fruit mesocarp tissue was firstly treated with isopropanol at 70°C for 30 min to inactivate endogenous lipase activity. Extraction of lipids was carried out according to Hara and Radin (1978), followed by their separation by thin layer chromatography as described by Hernández et al. (2008). Acid-catalyzed transmethylation of the different lipid preparations was performed to obtain the corresponding fatty acid methyl esters (Garcés and Mancha, 1993), which were analyzed by gas chromatography (Román et al., 2012). The internal standard used to calculate the lipid and fatty acid content in the samples was heptadecanoic acid. Results are expressed in μg of the sum of UFA per mg of FW, and are presented as means ± SD of three biological replicates.
Results and Discussion
Olive SAD Genes Expression and Unsaturated Fatty Acids Composition Are Regulated by Temperature
To investigate how temperature regulates UFA synthesis in olive mesocarp, olive branches from Picual and Arbequina cultivars holding olive fruits at 28 WAF, which correspond to turning stage, were incubated at low (15°C) and high (35°C) temperature with a 12 h light/12 h dark cycle, for 24 h. Lipid and SAD genes expression analysis were carried out using olive mesocarp at different times after incubation at the aforementioned conditions.
The effect of low temperature (15°C) on the UFA content and SAD transcript levels was different in the two cultivars studied. Low temperatures reduced the UFA content at the beginning of incubation in both cultivars, and then recovered practically to the initial levels at 6 h of incubation. These levels were maintained in Arbequina mesocarp until the end of the experiment. However, in Picual mesocarp the UFA levels increased during the 24 h period, reaching about 20% more than at the beginning of the treatment (Figure 1A). These results correlated well with the expression levels of SAD genes in mesocarp tissue exposed to the cold treatment. In this way, while Arbequina SAD transcripts remained practically constant throughout the treatment, in Picual mesocarp the expression levels of the three SAD genes showed a transient and significant increase during the first 3 h of incubation, with SAD1 undergoing a 10-fold increase in gene expression compared to SAD2 and SAD3 with 6- and 3-fold increase, respectively (Figure 1B). The effect of incubation at 15°C observed in Picual mesocarp is the general response to low temperature changes for desaturases, since there are numerous reports describing that low temperature causes an increase in the UFA content (Los and Murata, 1998). With only two exceptions in lima bean (Zhang et al., 2011) and avocado (García-Rojas et al., 2012), an induction of SAD genes by cold stress has been reported in different plant species. For instance, Wang et al. (2013) described that in Ginkgo biloba leaves, SAD mRNA levels increased transiently, reaching a maximum at 6 h after incubation at 4 or 15°C, and then decreased after 24 h, analogous to what we observed in the Picual olive mesocarp. Similarly, a transient induction of SAD gene was observed in tea leaves incubated at 4 or −5°C for 24 h (Ding et al., 2016). In a like manner, an increase in SAD transcript in response to low temperature has been described in avocado fruit (Madi et al., 2003), potato leaves (Vega et al., 2004; De Palma et al., 2008), rape hypocotyl (Tasseva et al., 2004), and soybean seed (Byfield and Upchurch, 2007), which was also accompanied by an increment in the UFA content. The role of SAD gene in plant cold stress has also been demonstrated in transplastomic tobacco plants expressing a wild potato SAD gene, that exhibited increased UFA content and improved cold tolerance (Craig et al., 2008). Furthermore, the rice cold inducible transcription factor Osmyb4, which is involved in the cold stress response, has been shown to transactivate the wild potato SAD gene promoter (Vannini et al., 2004).
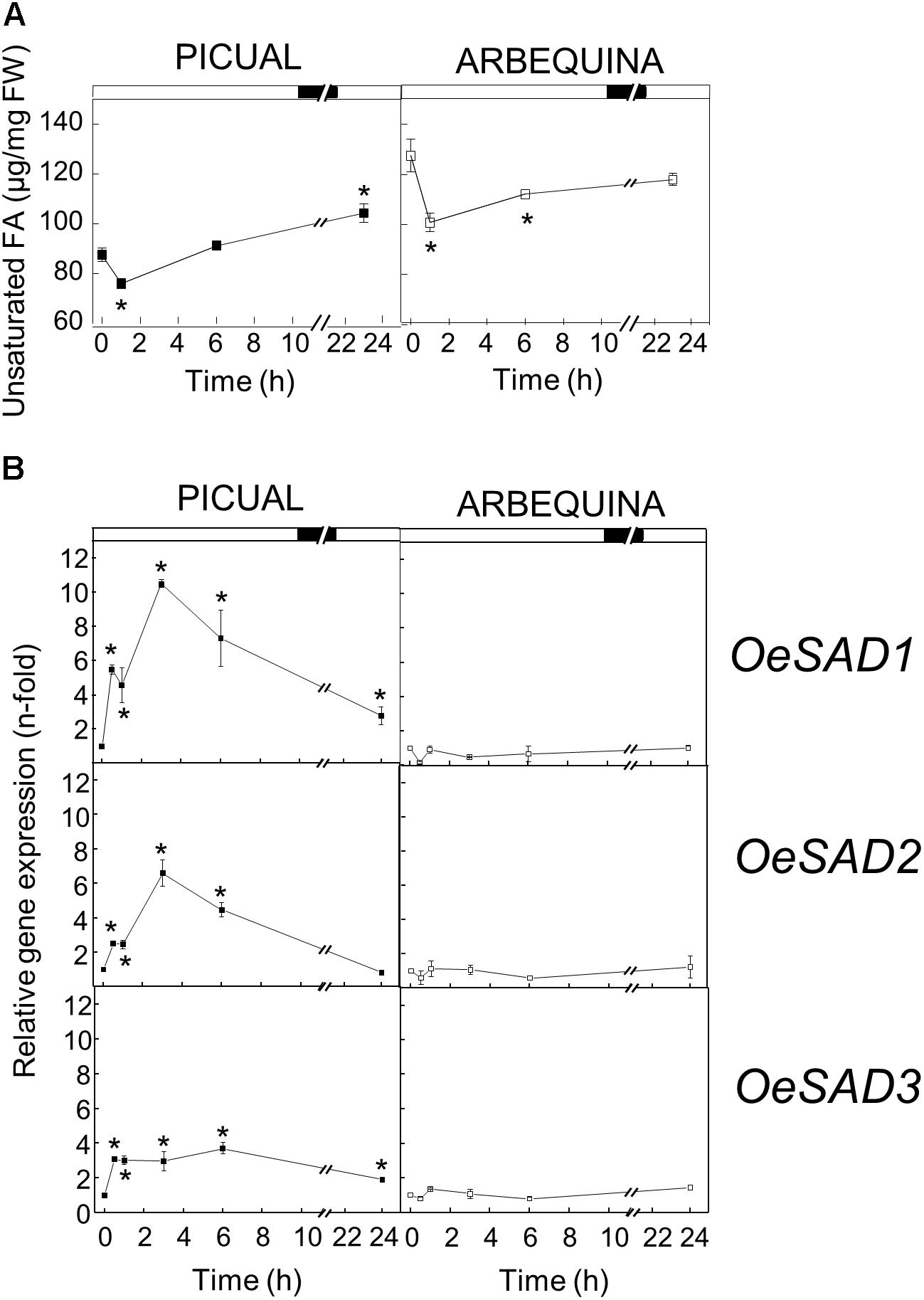
Figure 1. Effect of low temperature on the unsaturated fatty acids content (A) and the relative expression levels of olive SAD1, SAD2, and SAD3 genes (B) in the mesocarp tissue from cultivars Picual and Arbequina. Branches with about 100 olive fruit (28 WAF) were incubated using standard conditions except that the temperature was 15°C. At the indicated times, fatty acid composition was analyzed by gas chromatography, and relative expressions levels were determined by qRT-PCR using the expression level of the corresponding gene at zero time as calibrator. Data are presented as means ± SD of three biological replicates. ∗Indicates significantly different to time 0 h (p < 0.05) by two-way ANOVA with a Bonferroni post-test.
To gain further insight about the cold stress response observed in olive mesocarp from Picual cultivar, we decided to analyze the UFA content in the different lipids classes from this tissue incubated at 15°C for 24 h, to investigate whether the detected increase in SAD genes expression leads to an increase in the UFA content in membrane lipids. We observed that the UFA content in triacylglycerol decreased throughout the incubation at 15°C, while UFA levels increased significantly in the membrane phospholipids (Table 1). In addition, we observed an increase in all of the UFA, being oleic acid the one that increases the most (Supplementary Table S2). These data suggested that low temperature incubation induced a mobilization of UFA from storage lipids into microsomal membrane lipids.
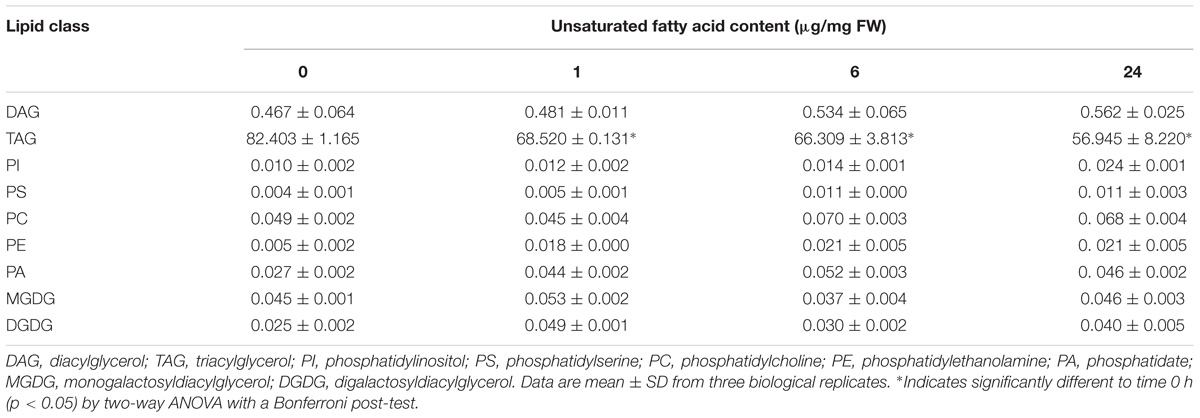
Table 1. Effect of low temperature on the unsaturated fatty acids content of lipid classes from Picual mesocarp tissue.
We have previously reported an induction of FAD2 genes in mesocarp tissue incubated at 15°C for 24 h, although non-significant differences were detected in the linoleic acid content of microsomal and plastidial membranes (Hernández et al., 2011). In that study, we suggested that the timescale could be too short to observe effects, although the existence of post-transcriptional regulatory mechanism could not be discarded. In this work (Supplementary Table S2), we noticed that the increase in oleic acid in membrane phospholipids took place from the beginning of the cold treatment (1 h after incubation), whereas a very slight increase of linoleic acid was detected in phosphatidic acid, phosphatidylserine, phosphatidylcholine, and phosphatidylethanolamine at longer times (6–24 h after incubation), suggesting that low temperature induced the synthesis of oleic acid in the short–term, while the increase of linoleic acid occurs at longer incubations periods. These results indicate that the observed changes in the transcript levels of SAD genes caused by low temperature are accompanied by the adjustment of UFA content of microsomal membrane lipids and modulation of membrane fluidity in olive mesocarp cv. Picual.
The fact that low temperatures did not increase either SAD expression levels or the UFA levels in Arbequina mesocarp could be related with a lower cold tolerance of this cultivar with respect to Picual. In this sense, Vega et al. (2004) reported that the expression of SAD gene increased during cold acclimation only in Solanum commersonii, a species capable of cold acclimation, and not in the cultivated non-cold acclimating Solanum tuberosum species, although the latter had a greater amount of constitutive SAD gene expression, indicating that the changes in transcript accumulation observed in S. commersonii may be related to its capacity to cold acclimate. However, these data contrast slightly with those found by De Palma et al. (2008), which provided evidence that the freezing tolerant S. commersonii plants have a higher constitutive transcript levels of SAD gene. Nevertheless, these results altogether suggest that SAD transcript accumulation plays a key role in cold tolerance. This assumption was further confirmed by Li et al. (2015), who reported that SAD overexpression caused an increase in membrane linoleic acid content, which improved the cold acclimation capacity of transgenic potato plants.
With respect to high temperature, when we incubated the olive branches with turning olive fruits at 35°C for 24 h, we observed a decreased in the UFA content 1 h after the incubation, to subsequently recover to the initial values throughout the incubation period in both cultivars, reaching even higher values than the initial ones after 24 h of incubation (Figure 2A). The changes observed in UFA content are mainly detected in TAG for both cultivars (Supplementary Tables S3, S4). These modifications in UFA levels did not correlate well with the SAD genes expression pattern detected in mesocarp tissue exposed to high temperature. We observed in Figure 2B that the expression levels of the three SAD genes decreased during the incubation at 35°C, although SAD genes expression patterns were different in both cultivars. Specifically, in cv. Picual, SAD1 and SAD2 transcripts slightly increased at 0.5 and 1 h of incubation, the three genes expression levels were similar to initial values at 3 h, and then decreased considerably after 24 h of incubation. However, in cv. Arbequina the three SAD transcripts decreased from the beginning of the treatment. The downregulation of SAD genes has been previously reported by Wang et al. (2013) in leaves of ginkgo grown at 35 or 45°C, and by Lu et al. (2013) in Pinellia ternata leaves incubated at 35°C for 24 h. In addition, when soybean plants were incubated under warm conditions, the SAD-A and SAD-B genes expression levels decreased in the seeds, but with negligible effect on the seed stearate content (Byfield and Upchurch, 2007). The lack of correlation between the effect of high temperature on SAD genes expression levels and the UFA content could be explained by several factors. In particular, the high temperature regulation of oleic acid synthesis may be mediated by post-transcriptional mechanism. Several cases of temperature-related post-transcriptional mechanisms have been reported for the oleate desaturases. In sunflower seeds, it has been described that changes in temperature bring about shifts in the very low endogenous oxygen concentration, which affect FAD2 activity reversibly, without having an effect on gene transcription (Rolletschek et al., 2007). Besides, Tang et al. (2005) identified two domains that appear to be important in mediating the temperature-dependent instability of the soybean FAD2-1A isoform when expressed in yeast. However, to date, no mechanism of post-transcriptional regulation by temperature has been described for SAD enzymes.
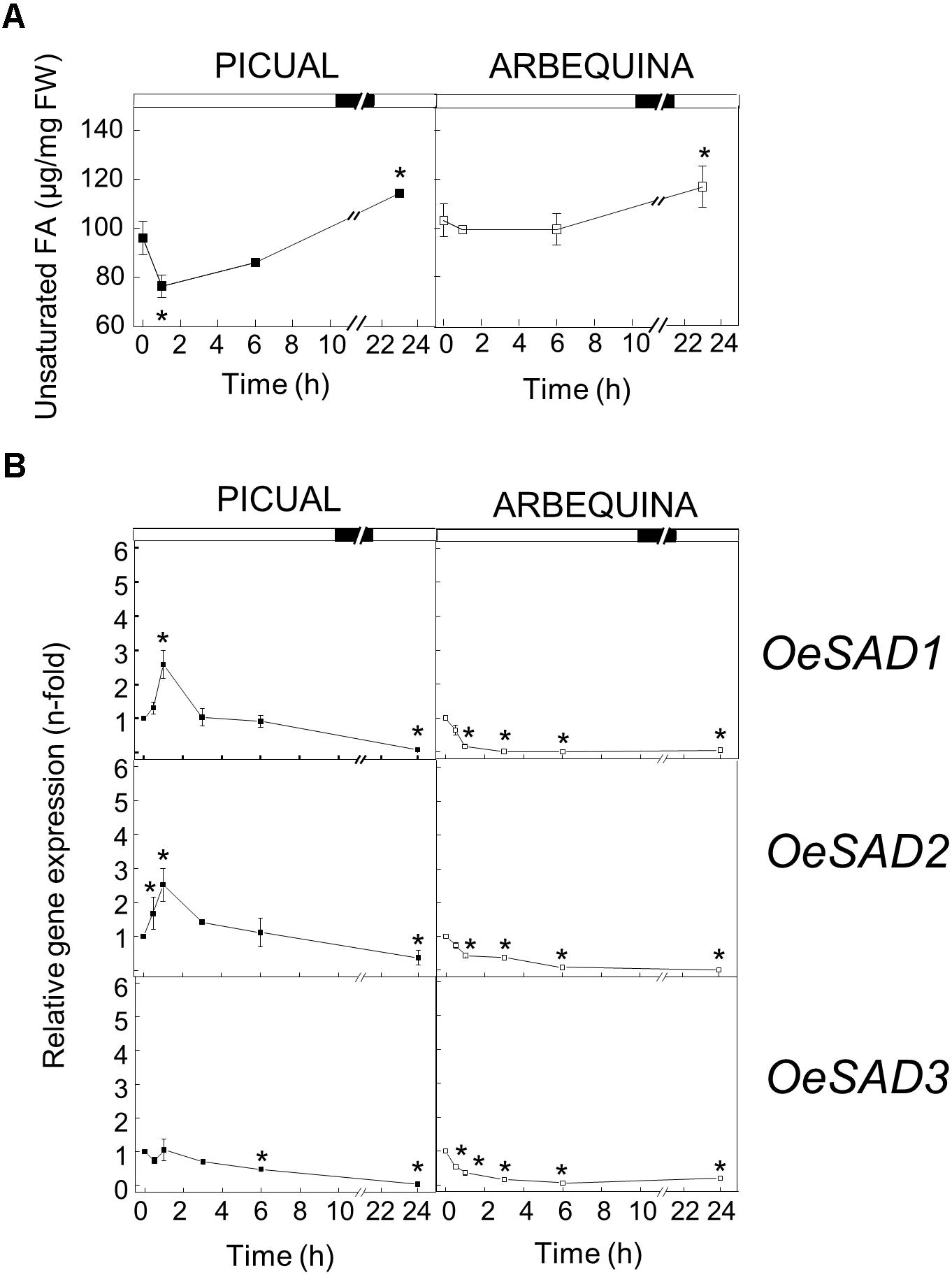
Figure 2. Effect of high temperature on the unsaturated fatty acids content (A) and the relative expression levels of olive SAD1, SAD2, and SAD3 genes (B) in the mesocarp tissue from cultivars Picual and Arbequina. Branches with about 100 olive fruit (28 WAF) were incubated using standard conditions except that the temperature was 35°C. At the indicated times, fatty acid composition was analyzed by gas chromatography, and relative expressions levels were determined by qRT-PCR using the expression level of the corresponding gene at zero time as calibrator. Data are presented as means ± SD of three biological replicates. ∗Indicates significantly different to time 0 h (p < 0.05) by two-way ANOVA with a Bonferroni post-test.
Regulation of Olive SAD Transcript Levels and Unsaturated Fatty Acids Content by Darkness
To test whether darkness alters the UFA content and SAD transcript levels in olive mesocarp, olive branches from Picual and Arbequina cultivars holding olive fruits at 28 WAF (turning stage) were incubated at 25°C in the darkness, for 24 h. Although we did not observe any significant difference in the total UFA content during the incubation period, a decrease in the three SAD genes expression levels was detected in both cultivars (Figure 3). SAD1 and SAD3 transcripts levels decreased considerably in both cultivars from the beginning of the treatment, so that after 24 h of incubation reached a reduction of 90-fold in comparison to the initial levels. However, the decrease in SAD2 expression levels was about 50-fold after 3 h of incubation, maintaining these levels in Picual cultivar and recovering the initial values in the case of Arbequina (Figure 3B).
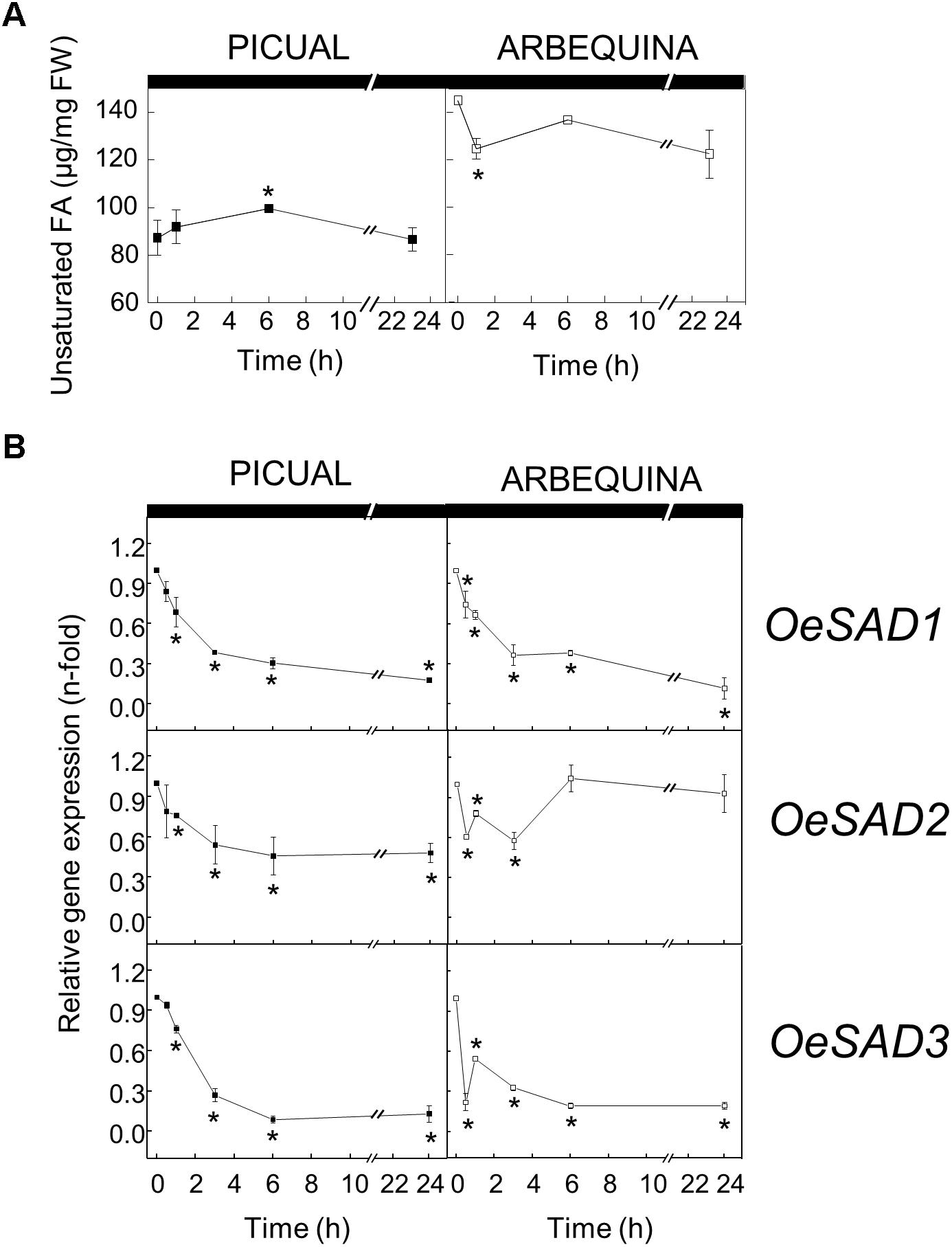
Figure 3. Effect of darkness on the unsaturated fatty acids content (A) and the relative expression levels of olive SAD1, SAD2, and SAD3 genes (B) in the mesocarp tissue from cultivars Picual and Arbequina. Branches with about 100 olive fruit (28 WAF) were incubated at 25°C under darkness conditions. At the indicated times, fatty acid composition was analyzed by gas chromatography, and relative expressions levels were determined by qRT-PCR using the expression level of the corresponding gene at zero time as calibrator. Data are presented as means ± SD of three biological replicates. ∗Indicates significantly different to time 0 h (p < 0.05) by two-way ANOVA with a Bonferroni post-test.
Several evidences indicate that light may play a regulatory role for plant desaturases, although data are scarce in this area. A light-dependent transcriptional regulation of oleate desaturase genes has been described in olive mesocarp (Hernández et al., 2011), similarly to that reported by Kargiotidou et al. (2008) in cotton cotyledons, where FAD2 expression was reduced after a 24 h incubation in the dark. In the same way, FAD7 and FAD8 are also transcriptionally regulated by light in different plants (Nishiuchi et al., 1995; Horiguchi et al., 1996; Collados et al., 2006). In general, it appears that increasing light conditions are associated with an enhancement in desaturases genes expression, with the converse also holding true in darkness conditions. Although this is the first time that the effect of darkness on SAD genes expression levels is reported in plants, the regulatory role of light on Δ9 desaturase genes has been studied before in other photosynthetic organism. Kis et al. (1998) described that in the cyanobacteria Synechocystis PCC 6803 desaturases genes were strongly induced by light, except for the Δ9 desaturase, that was not significantly affected. On the other hand, Ma et al. (2018) observed that the expression levels of SAD gene from the green microalgae Haematococcus pluvialis were significantly upregulated by light, which correlated well with an increase in oleic acid. These results are in agreement with the decrease in SAD genes expression levels observed in olive mesocarp in darkness conditions, although we did not observe a decrease in the total UFA content.
To further investigate the effect of darkness in oleic acid synthesis, we decided to analyze the UFA content of the different lipid classes in olive mesocarp subjected to darkness conditions, in order to elucidate whether the decrease in the expression levels of SAD genes affects the UFA content of a specific lipid. Interestingly, we only observed a decrease in the UFA content of the galactolipids, MGDG and DGDG, in both cultivars (Figure 4), although with different behavior. While in Picual mesocarp, the UFA content decreased considerably during the 24 h of incubation under darkness conditions, in Arbequina mesocarp, the UFA levels were reduced after 1 h of incubation, and then recovered, almost reaching the levels detected at the beginning of the treatment. This effect of the dark on the pattern of UFA content in galactolipids correlated quite well with that of SAD2 expression levels in both cultivars and not with SAD1 and SAD3, which suggests, that the reduction of SAD2 transcript due to the dark incubation could be the responsible for the decrease in UFA in galactolipids. In fact, we noticed that the decrease detected in the UFA content of MGDG and DGDG during the dark incubation of olive mesocarp is mainly due to a reduction in oleic acid content (Table 2). Therefore, we can conclude that the down-regulation of SAD2 gene during the dark incubation is the responsible for the reduction in the UFA content in the main chloroplast lipids, MGDG and DGDG. Furthermore, this light-dependent regulation detected in olive mesocarp is cultivar-dependent, since the restoration of SAD2 gene expression levels and UFA content in galactolipids after 24 h of incubation observed in Arbequina cultivar, was not detected in Picual mesocarp. The fact that the effect of darkness was noticeable on chloroplast-localized lipids is not unexpected. Gemmrich (1982) observed in Ricinus communis cultures, that the light-induced changes in lipid composition were associated with thylakoid formation. Strong light induces ultrastructural changes in chloroplasts, so that the area of thylakoid system on chloroplast sections increases, there is an accumulation of chloroplast-localized lipids, like MGDG, and the unsaturation index of fatty acids is elevated, being the relative content of linolenic acid the one that increases the most (Kislyuk et al., 2013).
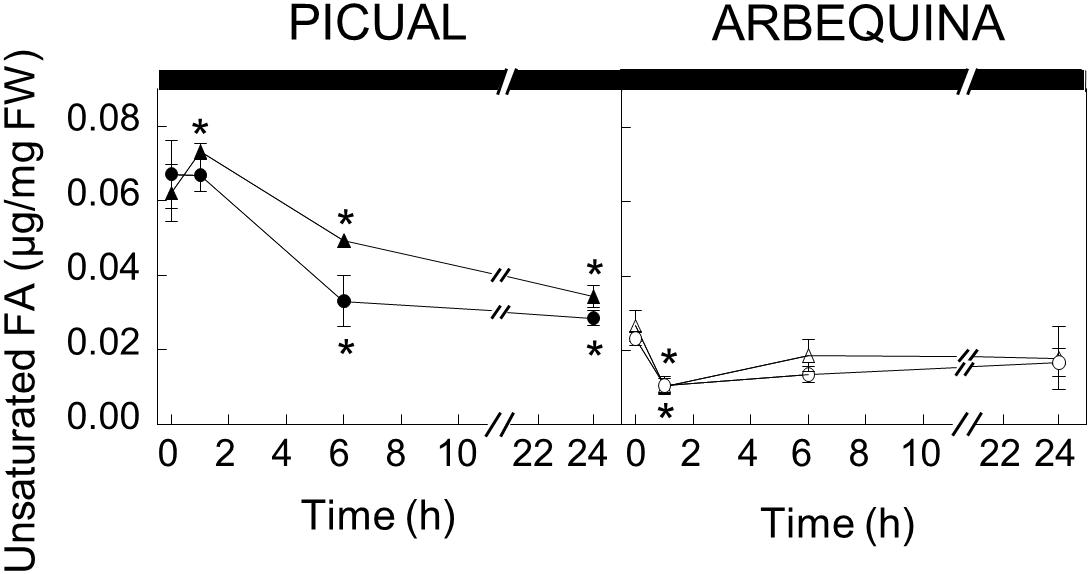
Figure 4. Effect of darkness on the galactolipids unsaturated fatty acid content in the mesocarp tissue from cultivars Picual and Arbequina. Branches with about 100 olive fruit (28 WAF) were incubated at 25°C under darkness conditions. At the indicated times, fatty acid composition of galactolipids were analyzed by gas chromatography a Triangles, monogalactosyldiacylglycerol; circles, digalactosyldiacylglycerol. Data are presented as means ± SD of three biological replicates. ∗Indicates significantly different to time 0 h (p < 0.05) by two-way ANOVA with a Bonferroni post-test.
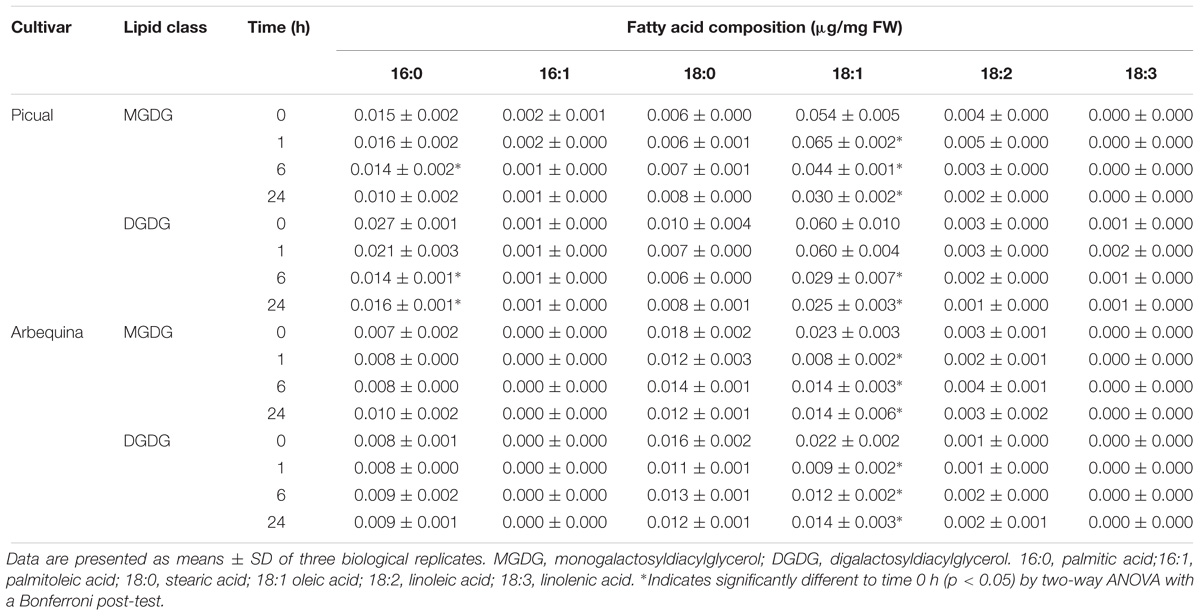
Table 2. Effect of darkness on the fatty acid composition of galactolipids from Picual and Arbequina mesocarp tissue.
Differential Transcriptional Regulation of Olive SAD Genes in Response to Wounding
The involvement of a Δ9 stearate desaturase in defense mechanisms was firstly demonstrated by Xing and Chin (2000), who reported that the expression of a yeast Δ9 desaturase in eggplant enhanced its resistance to Verticillium dahliae. To investigate possible changes in the UFA composition and SAD genes expression of olive mesocarp in response to wounding, olive branches from Picual and Arbequina cultivars holding olive fruits at 28 WAF (turning stage) were incubated using standard conditions except that olive fruit were mechanically damaged at zero time. A slight increase in the UFA content was observed when lipid analysis was performed at different times of incubation after wounding, although with some cultivar differences. While in cv. Picual the increase in UFA content after wounding was slow and progressive, in Arbequina mesocarp the UFA content increases rapidly after 1 h of incubation, continues rising until 6 h and then, the levels were maintained until the end of the incubation (Figure 5A).
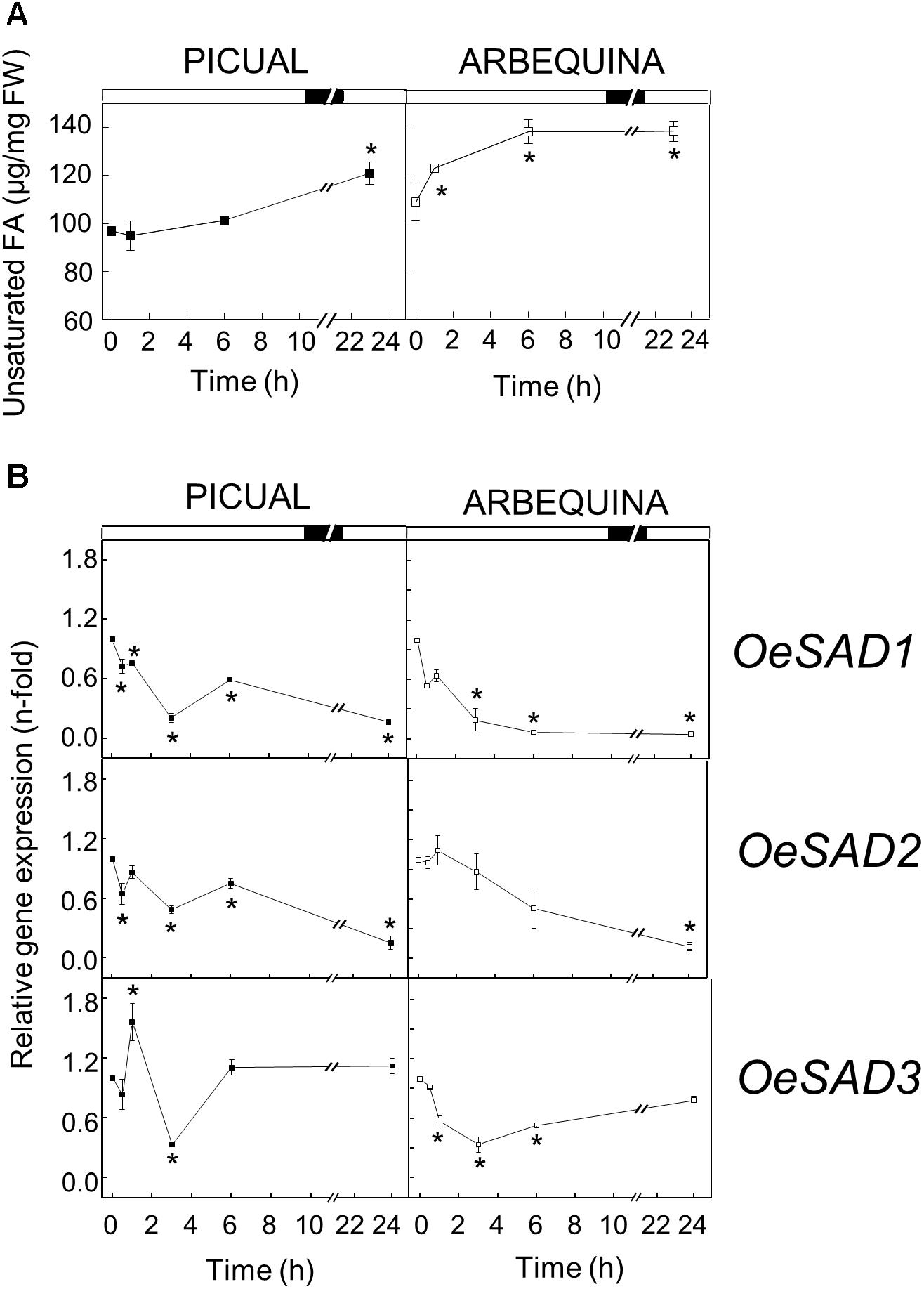
Figure 5. Effect of wounding on the unsaturated fatty acids content (A) and the relative expression levels of olive SAD1, SAD2 and SAD3 genes (B) in the mesocarp tissue from cultivars Picual and Arbequina. Branches with about 100 olive fruit (28 WAF) were incubated using standard conditions except that the olive fruit were mechanically damaged at zero time. At the indicated times, fatty acid composition was analyzed by gas chromatography, and relative expressions levels were determined by qRT-PCR using the expression level of the corresponding gene at zero time as calibrator. Data are presented as means ± SD of three biological replicates. ∗Indicates significantly different to time 0 h (p < 0.05) by two-way ANOVA with a Bonferroni post-test.
In contrast, except in the case of Picual SAD3 gene, olive SAD genes transcript levels decreased after wounding in both cultivars (Figure 5B). It has been demonstrated that a mutation in the Arabidopsis gene ssi2/fab2, which encodes a SAD, resulted in the reduction of oleic acid levels, causing the constitutive defense response in the mutant plant (Kachroo and Kachroo, 2009; Xia et al., 2009). Analogous results were observed in OsSSI2-knockdown plants in rice (Jiang et al., 2009), and in GmSAD-silenced soybean (Kachroo et al., 2008). In addition, overexpression of the TaSSI2 in ssi2 Arabidopsis mutant plants resulted in restoration of oleic acid and, thereby, rescued other ssi2-associated phenotypes (Song et al., 2013). Further studies revealed that the reduced oleic acid levels triggered the transcriptional up-regulation of pathogenesis-related genes, the genes governing synthesis of salicylic acid, and nitric oxide responsive nuclear genes, thus activating disease resistance (Venugopal et al., 2009; Mandal et al., 2012). In this sense, we also detected in olive mesocarp a discrete reduction of 1.24% in Picual and 2.88% in Arbequina in oleic acid levels after wounding (Table 3). Since olive SAD2 has been reported to be the main gene contributing to the oleic acid content in olive mesocarp (Parvini et al., 2016), the down-regulation of olive SAD1 and SAD2 genes observed in response to wounding could be responsible for the decrease in the oleic acid proportion and, consequently, trigger the defense response.
Unlike SAD1 and SAD2 genes, SAD3 increased its transcript levels transiently after 1 h in cv. Picual, and returned to initial values at 24 h of treatment (Figure 5B). Remarkably, SAD3 gene has been previously shown to be induced in olive leaves infected by Spilocaea oleagina (Benítez et al., 2005), and Verticillium dahliae and Fusarium spp. (Trabelsi et al., 2017). Similar results to olive SAD3 gene induction were observed in yellow lupine, avocado and tea. Zaborowska et al. (2002) reported an increase in SAD gene transcripts in yellow lupine nodules from 12 days after infection with Bradyrhizobium sp. (Lupinus). In the same way, SAD expression levels increased in response to wounding in avocado fruit (Madi et al., 2003) and tea leaves (Ding et al., 2016). Interestingly, we observed that the reduction in the oleic acid levels was accompanied by an increase in palmitolinoleic and linoleic acids at 24 h after wounding in both cultivars (Table 3). We have reported before this increase in dienoic fatty acids induced by wounding, showing that FAD2 genes are involved in the wounding response of olive fruit mesocarp, and causing an increase in the content of palmitolinoleic and linoleic acids in microsomal lipids (Hernández et al., 2011). In the same study, we also suggested that the synthesis of palmitolinoleic acid is a consequence of the simultaneous induction of SAD and FAD2 genes in olive fruit mesocarp in response to wounding. Since, in this work, we have observed a specific induction of the SAD3 gene (Figure 5B), it is tempting to speculate that the SAD3 isoform is the one involved in this response mechanism. These dienoic fatty acids probably serve as precursors of a different set of oxylipins involved in plant defense, generated by the lipoxygenase pathway (Weber, 2002).
Conclusion
In the present study, we have demonstrated that low temperature transcriptionally regulates SAD genes from olive mesocarp in a cultivar-dependent manner, leading to a modification of the UFA content in Picual microsomal membrane lipids, in order to maintain membrane fluidity in the mesocarp tissue. On the contrary, in the case of high temperature SAD genes expression levels did not correlate well with the UFA content in olive mesocarp. Our results have also shown that the decrease of SAD gene transcripts caused by darkness in olive mesocarp was accompanied by a reduction in the UFA content of chloroplast lipids. In addition, the differential transcriptional regulation of SAD genes after wounding seems to have a crucial role in the olive defense response, not only by reducing oleic acid levels, which triggers the transcriptional up-regulation of defense related genes, but also by promoting the increase of dienoic fatty acids, that serve as precursors of oxylipins. Taken together, the data presented in this work point out that the different environmental stresses can modify the content of oleic acid and its polyunsaturated derivatives in the olive mesocarp through the transcriptional regulation of SAD genes, affecting olive oil quality.
Data Availability
All datasets generated for this study are included in the manuscript and/or the Supplementary Files.
Author Contributions
MH managed and performed the stress experiments, carried out RNA isolation and cDNA synthesis, and drafted the manuscript. MS performed the qRT-PCR and lipid analysis. MA revised the study and the manuscript. JM-R conceived and designed the study and contributed to manuscript revision. All authors discussed, commented and approved the final version of the manuscript.
Funding
This work was supported by the Spanish Ministry of Economy and Competitiveness (Research Grants AGL2014-55300-R and AGL2017-87871-R). MH was the recipient of a contract from the JAE-Postdoctoral CSIC Program (Spain).
Conflict of Interest Statement
The authors declare that the research was conducted in the absence of any commercial or financial relationships that could be construed as a potential conflict of interest.
Acknowledgments
We acknowledge support of the publication fee by the CSIC Open Access Publication Support Initiative through its Unit of Information Resources for Research (URICI).
Supplementary Material
The Supplementary Material for this article can be found online at: https://www.frontiersin.org/articles/10.3389/fpls.2019.00251/full#supplementary-material
Abbreviations
DGDG, digalactosyldiacylglycerol; FAD2, microsomal oleate desaturase; FAD3, microsomal linoleate desaturase; FAD6, plastidial oleate desaturase; FAD7/8, plastidial linoleate desaturase; MGDG, monogalactosyldiacylglycerol; SAD, stearoyl-acyl carrier protein desaturase; UFA, unsaturated fatty acids; WAF, weeks after flowering.
References
Aparicio, R., Roda, L., Albi, M. A., and Gutiérrez, F. (1999). Effect of various compounds on virgin olive oil stability measured by rancimat. J. Agric. Food Chem. 47, 4150–4155. doi: 10.1021/jf9812230
Baldoni, L., Cultrera, N. G., Mariotti, R., Ricciolini, C., Arcioni, S., Vendramin, G. G., et al. (2009). A consensus list of microsatellite marker for olive genotyping. Mol. Breed. 24, 213–231. doi: 10.1007/s11032-009-9285-8
Banilas, G., Moressis, A., Nikoloudakes, N., and Hatzopoulos, P. (2005). Spatial and temporal expressions of two distinct oleate desaturases from olive (Olea europaea L.). Plant Sci. 168, 547–555. doi: 10.1016/j.plantsci.2004.09.026
Banilas, G., Nikiforiadis, A., Makariti, I., Moressis, A., and Hatzopoulos, P. (2007). Discrete roles of a microsomal linoleate desaturase gene in olive identified by spatiotemporal transcriptional analysis. Tree Physiol. 27, 481–490. doi: 10.1093/treephys/27.4.481
Beltrán, G., del Río, C., Sánchez, S., and Martínez, L. (2004). Influence of harvest date and crop yield on the fatty acid composition of virgin olive oils from cv. Picual. J. Agric. Food Chem. 52, 3434–3440. doi: 10.1021/jf049894n
Benítez, Y., Botella, M. A., Trapero, A., Alsalimiya, M., Caballero, J. L., Dorado, G., et al. (2005). Molecular analysis of the interaction between Olea europaea and the biotrophic fungus Spilocaea oleagina. Mol. Plant Pathol. 6, 425–438. doi: 10.1111/J.1364-3703.2005.00290.X
Brockman, J. A., Norman, H. A., and Hildebrand, D. F. (1990). Effects of temperature, light and a chemical modulator on linolenate biosynthesis in mutant and wild type Arabidopsis calli. Phytochemistry 29, 1447–1453. doi: 10.1016/0031-9422(90)80098-2
Byfield, G. E., and Upchurch, R. G. (2007). Effect of temperature on delta-9 stearoyl-ACP and microsomal omega-6 desaturase gene expression and fatty acid content in developing soybean seeds. Crop Sci. 47, 1698–1704. doi: 10.2135/cropsci2006.04.0213
Collados, R., Andreu, V., Picorel, R., and Alfonso, M. (2006). A light-sensitive mechanism differently regulates transcription and transcript stability of ω3 fatty-acid desaturases (FAD3, FAD7 and FAD8) in soybean photosynthetic cell suspensions. FEBS Lett. 580, 4934–4940. doi: 10.1016/j.febslet.2006.07.087
Corrado, G., Alagna, F., Rocco, M., Renzone, G., Varricchio, P., Coppola, V., et al. (2012). Molecular interactions between the olive and the fruit fly Bactrocera oleae. BMC Plant Biol. 12:86. doi: 10.1186/1471-2229-12-86
Craig, W., Lenzi, P., Scotti, N., De Palma, M., Saggese, P., Carbone, V., et al. (2008). Transplastomic tobacco plants expressing a fatty acid desaturase gene exhibit altered fatty acid profiles and improved cold tolerance. Transgenic Res. 17, 769–782. doi: 10.1007/s11248-008-9164-9
D’Angeli, S., Falasca, G., Matteucci, M., and Altamura, M. M. (2013). Cold perception and gene expression differ in Olea europaea seed coat and embryo during drupe cold acclimation. New Phytol. 197, 123–138. doi: 10.1111/j.1469-8137.2012.04372.x
De Palma, M., Grillo, S., Massarelli, I., Costa, A., Balogh, G., Vigh, L., et al. (2008). Regulation of desaturase gene expression, changes in membrane lipid composition and freezing tolerance in potato plants. Mol. Breed. 21, 15–26. doi: 10.1007/s11032-007-9105-y
Ding, Z. T., Shen, J. Z., Pan, L. L., Wang, Y. U., Li, Y. S., Wang, Y., et al. (2016). CsSAD: a fatty acid desaturase gene involved in abiotic resistance in Camellia sinensis (L.). Genet. Mol. Res. 15:15017512. doi: 10.4238/gmr.15017512
Farmer, E. E. (1994). Fatty acid signaling in plants and their associated microorganisms. Plant Mol. Biol. 26, 1423–1437. doi: 10.1007/BF00016483
Garcés, R., and Mancha, M. (1993). One-step lipid extraction and fatty acid methyl esters preparation from fresh plant tissues. Anal. Biochem. 211, 139–143. doi: 10.1006/abio.1993.1244
García-Rojas, M., Gudenschwager, O., Defilippi, B. G., and Gónzalez-Agüero, M. (2012). Identification of genes possibly related to loss of quality in late-season ‘Hass’ avocados in Chile. Postharvest Biol. Technol. 73, 1–7. doi: 10.1016/j.postharvbio.2012.05.007
Gemmrich, A. R. (1982). “Effect of light on lipid metabolism of tissue cultures,” in Biochemistry and Metabolism of Plant Lipids, eds J. F. G. Wintermans and P. J. C. Kuiper (Amsterdam: Elsevier), 213–216.
Grasso, F., Coppola, M., Carbone, F., Baldoni, L., Alagna, F., Perrotta, G., et al. (2017). The transcriptional response to the olive fruit fly (Bactrocera oleae) reveals extended differences between tolerant and susceptible olive (Olea europaea L.) varieties. PLoS One 12:e0183050. doi: 10.1371/journal.pone.0183050
Hamada, T., Nishiuchi, T., Kodama, H., Nishimura, M., and Iba, K. (1996). cDNA cloning of a wounding-inducible gene encoding a plastid omega-3 fatty acid desaturase from Tobacco. Plant Cell Physiol. 37, 606–611. doi: 10.1093/oxfordjournals.pcp.a028988
Hara, A., and Radin, N. S. (1978). Lipid extraction of tissues with a low-toxicity-solvent. Anal. Biochem. 90, 420–426. doi: 10.1016/0003-2697(78)90046-5
Haralampidis, K., Milioni, D., Sánchez, J., Baltrusch, M., Heinz, E., and Hatzopoulos, P. (1998). Temporal and transient expression of stearoyl-ACP carrier protein desaturase gene during olive fruit development. J. Exp. Bot. 49, 1661–1669. doi: 10.1093/jexbot/49.327.1661
Harwood, J. L. (2005). “Fatty acid biosynthesis,” in Plant Lipids, ed. D. J. Murphy (Oxfork: Blackwell Publishing), 27–101.
Hernández, M. L., Guschina, I. A., Martínez-Rivas, J. M., Mancha, M., and Harwood, J. L. (2008). The utilization and desaturation of oleate and linoleate during glycerolipid biosynthesis in olive (Olea europaea L.) callus cultures. J. Exp. Bot. 59, 2425–2435. doi: 10.1093/jxb/ern121
Hernández, M. L., Mancha, M., and Martínez-Rivas, J. M. (2005). Molecular cloning and characterization of genes encoding two microsomal oleate desaturases (FAD2) from olive. Phytochemistry 66, 1417–1426. doi: 10.1016/j.phytochem.2005.04.004
Hernández, M. L., Padilla, M. N., Mancha, M., and Martínez-Rivas, J. M. (2009). Expression analysis identifies FAD2-2 as the olive oleate desaturase gene mainly responsible for the linoleic acid content in virgin olive oil. J. Agric. Food Chem. 57, 6199–6206. doi: 10.1021/jf900678z
Hernández, M. L., Padilla, M. N., Sicardo, M. D., Mancha, M., and Martínez-Rivas, J. M. (2011). Effect of different environmental stresses on the expression of oleate desaturase genes and fatty acid composition in olive fruit. Phytochemistry 72, 178–187. doi: 10.1016/j.phytochem.2010.11.026
Hernández, M. L., Sicardo, M. D., and Martínez-Rivas, J. M. (2016). Differential contribution of endoplasmic reticulum and chloroplast ω-3 fatty acid desaturase genes to the linolenic acid content of olive (Olea europaea) fruit. Plant Cell Physiol. 57, 138–151. doi: 10.1093/pcp/pcv159
Hernández, M. L., Velázquez-Palmero, D., Sicardo, M. D., Fernández, J. E., Diaz-Espejo, A., and Martínez-Rivas, J. M. (2018). Effect of a regulated deficit irrigation strategy in a hedgerow ‘Arbequina’ olive orchard on the mesocarp fatty acid composition and desaturase gene expression with respect to olive oil quality. Agric. Water Manag. 204, 100–106. doi: 10.1016/j.agwat.2018.04.002
Horiguchi, G., Iwakawa, H., Kodama, H., Kawakami, N., Nishimura, M., and Iba, K. (1996). Expression of a gene for plastid ω-3 fatty acid desaturase and changes in lipid and fatty acid compositions in light- and dark-grown wheat leaves. Physiol. Plant. 96, 275–283. doi: 10.1111/j.1399-3054.1996.tb00214.x
Iba, K. (2002). Acclimative response to temperature stress in higher plants: approaches of gene engineering for temperature tolerance. Annu. Rev. Plant Biol. 53, 225–245. doi: 10.1146/annurev.arplant.53.100201.160729
Jiang, C. J., Shimono, M., Maeda, S., Inoue, H., Mori, M., Hasegawa, M., et al. (2009). Suppression of the rice fatty-acid desaturase gene OsSSI2 enhances resistance to blast and leaf blight diseases in rice. Mol. Plant Microbe Interact. 22, 820–829. doi: 10.1094/MPMI-22-7-0820
Kachroo, A., Fu, D. Q., Havens, W., Navarre, D., Kachroo, P., and Ghabrial, S. A. (2008). An oleic acid-mediated pathway induces constitutive defense signalling and enhanced resistance to multiple pathogens in soybean. Mol. Plant Microbe Interact. 21, 564–575. doi: 10.1094/MPMI-21-5-0564
Kachroo, A., and Kachroo, P. (2009). Fatty acid-derived signals in plant defense. Annu. Rev. Phytopathol. 47, 153–176. doi: 10.1146/annurev-phyto-080508-081820
Kargiotidou, A., Deli, D., Galanopoulou, D., Tsaftaris, A., and Farmaki, T. (2008). Low temperature and light regulate delta 12 fatty acid desaturases (FAD2) at a transcriptional level in cotton (Gossypium hirsutum). J. Exp. Bot. 59, 2043–2056. doi: 10.1093/jxb/ern065
Kis, M., Zsiros, O., Farkas, T., Wada, H., Nagy, F., and Gombos, Z. (1998). Light-induced expression of fatty acid desaturase genes. Proc. Natl. Acad. Sci. U.S.A. 95, 4209–4214. doi: 10.1073/pnas.95.8.4209
Kislyuk, I. M., Bubolo, L. S., Bykov, O. D., Kamentseva, I. E., Kotlova, E. R., and Vinogradskaya, M. A. (2013). Strong light elevates thermotolerance of photosynthetic apparatus and the content of membranes and polar lipids in wheat leaves. Russ. J. Plant Physiol. 60, 611–622. doi: 10.1134/S1021443713050099
Leyva-Pérez, M., Valverde-Corredor, A., Valderrama, R., Jiménez-Ruiz, J., Muñoz-Mérida, A., Trelles, O., et al. (2015). Early and delayed long-term transcriptional changes and short-term transient responses during cold acclimation in olive leaves. DNA Res. 22, 1–11. doi: 10.1093/dnares/dsu033
Li, F., Bian, C. S., Xu, J. F., Pang, W. F., Liu, J., Duan, S. G., et al. (2015). Cloning and functional characterization of SAD genes in potato. PLoS One 10:e0122036. doi: 10.1371/journal.pone.0122036
Livak, K. J., and Schmittgen, T. D. (2001). Analysis of relative gene expression data using real-time quantitative PCR and the 2−ΔΔCt method. Methods 25, 402–408. doi: 10.1006/meth.2001
Los, D. A., and Murata, N. (1998). Structure and expression of fatty acid desaturases. Biochim. Biophys. Acta 1394, 3–15. doi: 10.1016/S0005-2760(98)00091-5
Lu, H., Xue, T., Zhang, A., Sheng, W., Zhu, Y., Chang, L., et al. (2013). Construction of an SSH library of Pinellia ternata under heat stress, and expression analysis of four transcripts. Plant Mol. Biol. Rep. 31, 185–194. doi: 10.1007/s11105-012-0488-5
Ma, R., Thomas-Hall, S. R., Chua, E. T., Alsenani, F., Eltanahy, E., Netzel, M. E., et al. (2018). Gene expression profiling of astaxanthin and fatty acid pathways in Haematococcus pluvialis in response to different LED lighting conditions. Bioresour. Technol. 250, 591–602. doi: 10.1016/j.biortech.2017.11.094
Madi, L., Wang, X., Kobiler, I., Lichter, A., and Prusky, D. (2003). Stress on avocado fruits regulates Δ9-stearoyl ACP desaturase expression, fatty acid composition, antifungal diene level and resistance to Colletotrichum gloeosporioides attack. Physiol. Mol. Plant Pathol. 62, 277–283. doi: 10.1016/S0885-5765(03)00076-6
Mandal, M. K., Chandra-Shekara, A. C., Jeong, R. D., Yu, K., Zhu, S., Chanda, B., et al. (2012). Oleic acid-dependent modulation of NITRIC OXIDE ASSOCIATED1 protein levels regulates nitric oxide-mediated defense signalling in Arabidopsis. Plant Cell 24, 1654–1674. doi: 10.1105/tpc.112.096768
Matsuda, O., Sakamoto, H., Hashimoto, T., and Iba, K. (2005). A temperature-sensitive mechanism that regulates post-translational stability of a plastidial omega-3 fatty acid desaturase (FAD8) in Arabidopsis leaf tissues. J. Biol. Chem. 280, 3597–3604. doi: 10.1074/jbc.M407226200
Matteucci, M., D’Angeli, S., Errico, S., Lamanna, G., Perrotta, G., and Altamura, M. M. (2011). Cold affects the transcription of fatty acid desaturases and oil quality in the fruit of Olea europaea L. genotypes with different cold hardiness. J. Exp. Bot. 62, 3403–3420. doi: 10.1093/jxb/err013
Murphy, D. J., and Stumpf, P. K. (1979). Light-dependent induction of polyunsaturated fatty acid biosynthesis in greening cucumber cotyledons. Plant Physiol. 63, 328–335. doi: 10.1104/pp.63.2.328
Nishiuchi, T., Hamada, T., Kodama, H., and Iba, K. (1997). Wounding changes the spatial expression pattern of the Arabidopsis plastid omega-3 fatty acid desaturase gene (FAD7) through different signal transduction pathways. Plant Cell 9, 1701–1712. doi: 10.1105/tpc.9.10.1701
Nishiuchi, T., Nakamura, T., Abe, T., Kodama, H., Nishimura, M., and Iba, K. (1995). Tissue-specific and light-responsive regulation of the promoter region of the Arabidopsis thaliana chloroplast ω3 fatty-acid desaturase gene (FAD7). Plant Mol. Biol. 29, 599–609. doi: 10.1007/BF00020987
Ohnishi, J., and Yamada, M. (1983). Glycerolipid synthesis in Avena leaves during greening of etiolated seedlings IV. Effect of light on fatty acid desaturation. Plant Cell Physiol. 24, 1553–1557. doi: 10.1093/oxfordjournals.pcp.a076678
Padilla, M. N., Hernández, M. L., Sanz, C., and Martínez-Rivas, J. M. (2012). Molecular cloning, functional characterization and transcriptional regulation of a 9-lipoxygenase gene from olive. Phytochemistry 74, 58–68. doi: 10.1016/j.phytochem.2011.11.006
Padilla, M. N., Hernández, M. L., Sanz, C., and Martínez-Rivas, J. M. (2014). Stress-dependent regulation of 13-lipoxygenase and 13-hydroperoxide lyase in olive fruit mesocarp. Phytochemistry 102, 80–88. doi: 10.1016/j.phytochem.2014.01.024
Parvini, F., Sicardo, M. D., Hosseini-Mazinani, M., Martínez-Rivas, J. M., and Hernández, M. L. (2016). Transcriptional analysis of stearoyl-acyl carrier protein desaturase genes from olive (Olea europaea) in relation to the oleic acid content of the virgin olive oil. J. Agric. Food Chem. 64, 7770–7781. doi: 10.1021/acs.jafc.6b02963
Perona, J. S., Rodríguez-Rodríguez, R., and Ruiz-Gutiérrez, V. (2005). Effects of oleic acid rich oils on aorta lipids and lipoprotein lipase activity of spontaneously hypertensive rats. J. Agric. Food Chem. 53, 7330–7336. doi: 10.1021/jf051375c
Poghosyan, Z. P., Haralampidis, K., Martsinkowskaya, A. I., Murphy, D. J., and Hatzopoulos, P. (1999). Developmental regulation and spatial expression of a plastidial fatty acid desaturase from Olea europaea. Plant Physiol. Biochem. 37, 109–119. doi: 10.1016/S0981-9428(99)80072-2
Rolletschek, H., Borisjuk, L., Sánchez-García, A., Gotor, C., Romero, L. C., Martínez-Rivas, J. M., et al. (2007). Temperature-dependent endogenous oxygen concentration regulates microsomal oleate desaturase in developing sunflower seeds. J. Exp. Bot. 58, 3171–3181. doi: 10.1093/jxb/erm154
Román, A., Andreu, V., Hernández, M. L., Lagunas, B., Picorel, R., Martínez-Rivas, J. M., et al. (2012). Contribution of the different omega-3 fatty acid desaturase genes to the cold response in soybean. J. Exp. Bot. 63, 4973–4982. doi: 10.1093/jxb/err313
Sánchez, J. (1994). Lipid photosynthesis in olive fruit. Prog. Lipid Res. 33, 97–104. doi: 10.1016/0163-7827(94)90012-4
Shanklin, J., and Cahoon, E. B. (1998). Desaturation and related modifications of fatty acids. Annu. Rev. Plant Physiol. Plant Mol. Biol. 49, 611–641. doi: 10.1146/annurev.arplant.49.1.611
Shanklin, J., and Somerville, C. (1991). Stearoyl-acyl-carrier-protein desaturase from higher plants is structurally unrelated to the animal and fungal homologs. Proc. Natl. Acad. Sci. U.S.A. 88, 2510–2514. doi: 10.1073/pnas.88.6.2510
Song, N., Hu, Z., Li, Y., Li, C., Peng, F., Yao, Y., et al. (2013). Overexpression of a wheat stearoyl-ACP desaturase (SACPD) gene TaSSI2 in Arabidopsis ssi2 mutant compromise its resistance to powdery mildew. Gene 524, 220–227. doi: 10.1016/j.gene.2013.04.019
Tang, G. Q., Novitzky, W. P., Griffin, H. C., Huber, S. C., and Dewey, R. E. (2005). Oleate desaturase enzymes of soybean: evidence of regulation through differential stability and phosphorylation. Plant J. 44, 433–446. doi: 10.1111/j.1365-313X.2005.02535.x
Tasseva, G., De-Virville, J. D., Cantrel, C., Moreau, F., and Zachowski, A. (2004). Changes in the endoplasmic reticulum lipid properties in response to low temperature in Brassica napus. Plant Physiol. Biochem. 42, 811–822. doi: 10.1016/j.plaphy.2004.10.001
Trabelsi, R., Sellami, H., Gharbi, Y., Cheffi, M., Chaari, A., Baucher, M., et al. (2017). Response of olive tree (Olea europaea L. cv. Chemlali) to infection with soilborne fungi. J. Plant Dis. Prot. 124, 153–162. doi: 10.1007/s41348-016-0062-8
Upchurch, R. G. (2008). Fatty acid unsaturation, mobilization, and regulation in the response of plants to stress. Biotechnol. Lett. 30, 967–977. doi: 10.1007/s10529-008-9639-z
Vannini, C., Locatelli, F., Bracale, M., Magnani, E., Marsoni, M., Osnato, M., et al. (2004). Overexpression of the rice Osmyb4 gene increases chilling and freezing tolerance of Arabidopsis thaliana plants. Plant J. 37, 115–127. doi: 10.1046/j.1365-313X.2003.01938.x
Vega, S. E., Del-Rio, A. H., Bamberg, J. B., and Palta, J. P. (2004). Evidence for the up-regulation of stearoyl-ACP (Δ9) desaturase gene expression during cold acclimation. Am. J. Potato Res. 81, 125–135. doi: 10.1007/BF02853610
Venugopal, S. C., Jeong, R.-D., Mandal, M. K., Zhu, S., Chandra-Shekara, A. C., Xia, Y., et al. (2009). Enhanced disease susceptibility 1 and salicylic acid act redundantly to regulate resistance gene-mediate signaling. PLoS Genet. 5:e1000545. doi: 10.1371/journal.pgen.1000545
Wang, H., Cao, F., Zhang, W., Wang, G., and Yu, W. (2013). Cloning and expression of stearoyl-ACP desaturase and two oleate desaturases genes from Ginkgo biloba L. Plant Mol. Biol. Rep. 31, 633–648. doi: 10.1007/s11105-012-0525-4
Weber, H. (2002). Fatty acid-derived signals in plants. Trends Plant Sci. 7, 217–224. doi: 10.1016/S1360-1385(02)02250-1
Xia, Y., Gao, Q.-M., Yu, K., Lapchyk, L., Navarre, D., Hildebrand, D., et al. (2009). An intact cuticle in distal tissues is essential for the induction of systemic acquired resistance in plants. Cell Host Microbe 5, 151–165. doi: 10.1016/j.chom.2009.01.001
Xing, J., and Chin, C. (2000). Modification of fatty acids in eggplant affects its resistance to Verticillium dahliae. Physiol. Mol. Plant Pathol. 56, 217–225. doi: 10.1006/pmpp.2000.0268
Zaborowska, Z., Starzycki, M., Femiak, I., Swiderski, M., and Legocki, A. B. (2002). Yellow lupine gene encoding stearoyl-ACP desaturase organization, expression and potential application. Acta Biochim. Pol. 49, 29–42.
Keywords: Olea europaea, olive, abiotic stress, gene expression, unsaturated fatty acids, stearoyl-ACP desaturase
Citation: Hernández ML, Sicardo MD, Alfonso M and Martínez-Rivas JM (2019) Transcriptional Regulation of Stearoyl-Acyl Carrier Protein Desaturase Genes in Response to Abiotic Stresses Leads to Changes in the Unsaturated Fatty Acids Composition of Olive Mesocarp. Front. Plant Sci. 10:251. doi: 10.3389/fpls.2019.00251
Received: 09 November 2018; Accepted: 15 February 2019;
Published: 05 March 2019.
Edited by:
Andrea Chini, Centro Nacional de Biotecnología (CNB), SpainReviewed by:
Qing Liu, Commonwealth Scientific and Industrial Research Organisation (CSIRO), AustraliaMaria Cecilia Rousseaux, Centro Regional de Investigaciones Científicas y Transferencia Tecnológica de La Rioja (CRILAR CONICET), Argentina
Marjorie A. Lienard, Broad Institute, United States
Copyright © 2019 Hernández, Sicardo, Alfonso and Martínez-Rivas. This is an open-access article distributed under the terms of the Creative Commons Attribution License (CC BY). The use, distribution or reproduction in other forums is permitted, provided the original author(s) and the copyright owner(s) are credited and that the original publication in this journal is cited, in accordance with accepted academic practice. No use, distribution or reproduction is permitted which does not comply with these terms.
*Correspondence: M. Luisa Hernández, mlhdez@cica.es; lhernandez@ig.csic.es