Single-Phase Concentrated Solid-Solution Alloys: Bridging Intrinsic Transport Properties and Irradiation Resistance
- Materials Science and Technology Division, Oak Ridge National Laboratory, Oak Ridge, TN, United States
Single-phase concentrated solid-solution alloys (SP-CSAs), including high entropy alloys (HEAs), are compositionally complex but structurally simple, and provide a playground of tailoring material properties through modifying their compositional complexity. The recent progress in understanding the compositional effects on the energy and mass transport properties in a series of face-centered-cubic SP-CSAs is the focus of this review. Relatively low electrical and thermal conductivities, as well as small separations between the interstitial and vacancy migration barriers have been generally observed, but largely depend on the alloying constituents. We further discuss the impact of such intrinsic transport properties on their irradiation response; the linkage to the delayed damage accumulation, slow defect aggregation, and suppressed irradiation induced swelling and segregation has been presented. We emphasize that the number of alloying elements may not be a critical factor on both transport properties and the defect behaviors under ion irradiations. The recent findings have stimulated novel concepts in the design of new radiation-tolerant materials, but further studies are demanded to enable predictive models that can quantitatively bridge the transport properties to the radiation damage.
Introduction
High entropy alloys (HEAs) have gained increasing research attention since proposed in 2004 (Cantor et al., 2004; Yeh et al., 2004; Guo and Liu, 2011; Senkov et al., 2011; Lucas et al., 2012). Different from conventional alloys (e.g., steels and Ni-based superalloys), HEAs contain multiple alloying elements (usually more than four) in equal or near equal concentrations but form simple structures. The outstanding properties for potential structural and mechanical applications have attracted the majority of investigations on HEAs, including good thermal stability, high fracture toughness, good creep behavior, as well as strong resistance to wear, oxidation and corrosion, as been comprehensively reviewed in literatures such as Zhang et al. (2014) and Miracle and Senkov (2017). Another important research focus on HEAs is their transport properties. For example, the so-called “sluggish diffusion” effect, a mass transport property, has been considered one of the core effects of HEAs, responsible to the outstanding high temperature properties (Tsai et al., 2013; Dabrowa et al., 2016; Vaidya et al., 2016). In addition, interesting electrical transport properties such as superconductivity (Koželj et al., 2014) and quantum critical behavior (Sales et al., 2016, 2017) have been discovered, and the HEAs have been considered promising in functional applications such as thermoelectric materials (Shafeie et al., 2015; Fan et al., 2016) and soft ferromagnetic materials (Zhang et al., 2013). Furthermore, recent studies have linked the mass and energy transport properties to the enhanced irradiation resistance (Zhang et al., 2015; Lu et al., 2016b; Zhang Y. et al., 2017). Different from the traditional design strategies of irradiation tolerant alloys that usually introduce extrinsic defect sinks, such as grain boundary, secondary phases, and nano particles (Ukai and Fujiwara, 2002; Was, 2007; Garner, 2012), HEAs allow the focus on the effects of intrinsic properties determined by the major alloying elements on irradiation response, as considered a new path of designing radiation resistant alloys.
Although the early studies of HEAs have mainly targeted on the alloys containing five or more elements, evidence has suggested that their excellent properties do not necessarily require the large number of alloying elements. For example, the mechanical properties (e.g., strength, ductility, and fracture toughness) of ternary alloy NiCoCr, a so-called medium entropy alloy, have been reported superior to those of NiCoFeCrMn (Gludovatz et al., 2016). In addition, sluggish diffusion have also been found not merely related to the number of elements (Zhang C. et al., 2017; Jin et al., 2018). Thus, the studies of HEAs have been gradually broadened to a more general family—single-phase concentrated solid-solution alloys (SP-CSAs).
Ni-containing SP-CSAs in a face-centered cubic (FCC) structure form a family of alloys that enables varying the number, type, and concentration of alloying elements in a wide range without changing the structure, and thus an ideal model system for studying the effects of compositional complexity (Wu et al., 2014; Miracle and Senkov, 2017). This review summarizes the recent progress in understanding the compositional effects on the intrinsic transport properties, and its connection to the irradiation response: Section Electrical and Thermal Transport Properties summarizes the recent results on electrical resistivity and thermal conductivity in SP-CSAs, and compared them with some other transition metal alloys. In section Mass Transport Properties: Atomic Diffusion Behavior, Diffusion and Defect Dynamics in SP-CSAs are discussed and the concept of “sluggish diffusion” is clarified. In section Irradiation Resistance, experimental results of ion irradiation induced defect evolution at low, room and elevated temperatures in SP-CSAs are reviewed. In section Linkage Between the Transport Properties and Irradiation Response, present understanding on how such irradiation response is correlated to their intrinsic transport properties are discussed.
Electrical and Thermal Transport Properties
Electrical Resistivity
Extensive measurements have been performed in the early years on the electrical resistivity of binary alloys, the simplest SP-CSAs, with a number of elemental combinations, see e.g., Ho et al. (1983). Later on, Ni-Fe-Cr system has been one of the most studied ternary systems, probably due to their highly tunable magnetic phases and their importance in many commercial alloys, e.g., stainless steels (Banerjee and Raychaudhuri, 1994; Nath and Majumdar, 1996; Chakraborty and Majumdar, 1998). High electrical resistivity was proposed as feature of HEA in its early development stage in 2006, with potential application of high frequency communication materials (Wu et al., 2006; Yeh, 2006). Nonetheless, NiCoFeCrAlx was among the first HEAs with systematic electrical resistivity measurements reported a few years after then (Chou et al., 2009; Kao et al., 2011). In its FCC phase, the electrical resistivity increases with Al concentrations, in the range of ~100–150 μΩ cm. Recently, the electrical resistivity of a series of Ni-containing FCC SP-CSAs from binary to quinary has been measured, as shown in Figure 1 (Jin et al., 2016d). The addition of Cr was shown having the most significant impact on the residual resistivity: the alloys containing Cr have one order magnitude higher resistivity than those without Cr. The NiCoFeCrAlx fall into the same resistivity range with those Cr-containing alloys. The NiCoFe-based alloys containing other alloying elements, e.g., Ti, and Si, have been observed to reach higher electrical resistivity only when significant amounts of intermetallics phases are formed (Zhang and Fu, 2012; Zuo et al., 2014), which is beyond the scope of SP-CSAs.
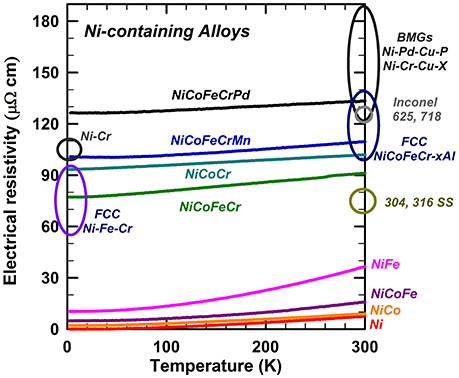
Figure 1. Electrical resistivity of Ni-containing FCC SP-CSAs, compared with several conventional alloy systems and BMGs. Data adapted from Mooij (1972), Chakraborty and Majumdar (1998), Yamasaki et al. (2005), Chou et al. (2009), Kao et al. (2011), Lee et al. (2016), and Jin et al. (2016d).
As shown in Figure 1, single-phase HEAs (i.e., containing four or more principal elements) do not necessarily have significantly higher electrical resistivities than the conventional alloys or the SP-CSAs containing less alloying elements: their electrical resistivity is indeed slightly higher than the 304 and 316 stainless steels, but close to Inconel 625, 718, and even the binary Ni-20Cr alloys (Mooij, 1972; Chakraborty and Majumdar, 1998; Lee et al., 2016). It also needs to be noted that single-phase HEAs usually have smaller electrical resistivities than the common bulk metallic glasses (BMGs) containing Ni and multiple transition metals (Yamasaki et al., 2005).
The complete picture of underlying physics is still challenging for CSAs due to the integrated impact from chemical, magnetic, and displacement disorder. The large difference in the (residual) resistivity between the NiCoFeCrMn subsets with and without Cr has been interpreted with the calculated Bloch spectral function (BSF) using ab initio Korring-Kohn-Rostoker coherent potential approximation (KKR-CPA) calculations (Jin et al., 2016d; Sales et al., 2016). For the alloys that that only contain Ni, Co and Fe, the d-band smearing is limited in the minority spin channel; its majority spin channel, with large (or infinite) electron mean free path, providing a short circuit and an overall low resistivity. In contrast, both minority and majority states are smeared in the alloys containing Cr, giving rise to the much larger overall smearing and large resistivity.
Thermal Conductivity
The thermal conductivity in the NiCoFeCr-based HEAs and its subsets has been systematically measured (Jin et al., 2016d, 2017). As shown in Figure 2, two groups can be clearly identified. All tested SP-CSAs containing Cr, from binary to quinary, have similar thermal conductivity values across the temperature range. Their room temperature thermal conductivities are ~10–15 W/m·K, which is close to that for the Ni-Fe-Cr based conventional commercial alloys such as stainless steels and Inconel (Lee et al., 2016). It should also be noted that the SP-CSAs have in general higher thermal conductivities than that for BMGs, which have the values of ~4–8 W/m·K at room temperature (Yamasaki et al., 2005). For the alloys that only contain Ni, Co, and Fe, the thermal conductivities are much higher, and considerably depend on specific compositions. Similar to the case of electrical resistivity, the number of alloying elements has little impact on the thermal conductivities. For example, the thermal conductivity of binary Ni-20Cr is similar to that of quinary NiCoFeCrMn, but much lower than the ternary NiCoFe.
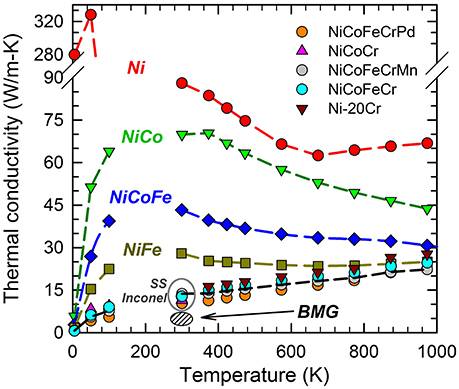
Figure 2. Thermal conductivity of Ni-containing FCC SP-CSAs, compared with several commercial alloys and BMGs. Data adapted from Yamasaki et al. (2005), Lee et al. (2016), and Jin et al. (2016d, 2017).
Thermal conductivity is composed of electronic (κe) and lattice (κg) contributions, among which the electronic thermal conductivity is usually estimated from the electrical resistivity value based on the Wiedemann-Franz relationship, κe = LT/ρ, where L is the Lorenz constant, T is the temperature, and ρ is the electrical resistivity, since the direct measurement of this portion is usually not feasible (Klemens and Williams, 1986). In pure transition metals, the electronic contribution is dominant, i.e., the lattice contribution is negligible. In contrast, the fraction of lattice contribution is greatly enhanced in SP-CSAs. The fraction of lattice contribution to the total thermal conductivity is shown in Figure 3, for a series of Ni-containing SP-CSAs. The lattice and electronic contributions are comparable for most NiCr- alloys (Chou et al., 2009; Jin et al., 2016d, 2017). Again, Ni-20Cr binary alloy has basically the same behavior, compared with the other quinary alloys.
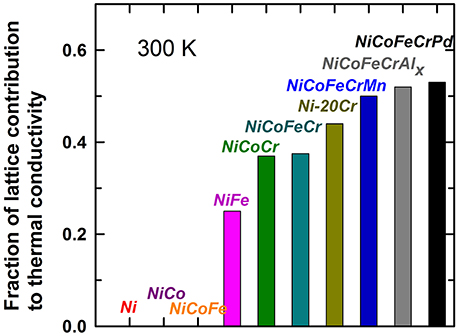
Figure 3. Fraction of lattice contribution to thermal conductivity in several Ni-containing FCC SP-CSAs at 300 K. Values calculated based on the data in Chou et al. (2009), Kao et al. (2011), and Jin et al. (2016d, 2017).
Although the accuracy of Wiedemann-Franz relationship remains arguable, the results in Figure 3 at least reveals that the lattice thermal conductivity is an important transport property worth investigating. While directly determining the lattice thermal conductivity is non-trivial, it can be achieved from the phonon spectra since it is inversely proportional to phonon bandwidth, which is affected by the mass and force constant fluctuation. A recent theoretical work employing ab initio calculations has systematically studied the impact of these two kinds of disorders in a series of BCC equiatomic alloys, from binary to quinary (refractory HEAs) (Körmann et al., 2017). In this series of calculations, the mass fluctuations have played a dominant role, although the impact from force constant fluctuation could not be neglected. They have further indicated that the specific alloy combinations, rather than the configurational entropy, control the vibration properties. Coupling between different disorder sources and scattering mechanisms have been found from calculations based on Lennard-Jones potentials (Caro et al., 2015); it would be interesting to learn the role of force constant fluctuations on phonon broadening and further the lattice thermal conductivity in the subsets of NiCoFeCrMn system, since the atomic mass difference in this family of alloys is very small compared with that in the BCC HEAs. Moreover, experimental measurement on the phonon broadening in HEAs is desired to acquire experimental lattice thermal conductivity.
Electrical and thermal conductivities of HEAs are further related to their thermoelectric properties, with the figure of merit (ZT) calculated from the Seebeck coefficient (S), electrical resistivity (ρ), and total thermal conductivity (κ) from the equation ZT = S2ρ−1κ−1T. For example, low lattice thermal conductivities above room temperature in BiSbTe1.5Se1.5 (Fan et al., 2016) and PbSnTeSe (Fan et al., 2017), ~0.47 and 0.6 Wm−1K−1, respectively, have been reported to contribute to their relatively high ZT values. Both studies have ascribed such low κg values to the severe lattice distortion, while further evidences may be necessary to validate this proposed mechanism. The thermoelectric properties of NiCoFeCrAlx, have been studied (Shafeie et al., 2015). Increasing Al concentration up to x = 3 has been observed to enhance the absolute values of Seebeck coefficient but reduce the electrical conductivity above room temperature. The power factor and ZT reach maximum at x = 2.0 and 2.25, where the alloys no longer maintain single-phase solid solution, and the ZT values still remain in the low regime.
Mass Transport Properties: Atomic Diffusion Behavior
“Sluggish diffusion” has been considered one of the four “core effects” in HEAs, with the three others being “high entropy,” “severe lattice distortion,” and “cocktail effects” (Miracle and Senkov, 2017), and has been usually referred to the hypothesis that atomic diffusion is retarded in HEAs compared with in pure metals and traditional alloys. This idea was proposed at the invention of the HEAs (Yeh et al., 2004; Yeh, 2006), accounting for its high temperature thermal stability. The first experimental support to this hypothesis has become available in 2013 through interdiffusion experiments in the NiCoFeCrMn systems (Tsai et al., 2013). By comparing the diffusion coefficients and activation energies between the HEA system, pure metals, and conventional alloys, a positive relation between the normalized activation energy and the number of alloying elements has been proposed. It needs to be noted that all comparisons were made with respect to homologous temperature in Tsai et al. (2013), i.e., the difference in their melting temperatures was normalized. This viewpoint was later supported by several revisits to the data analysis, and another measurement on the NiCoFeCrAl system (Beke and Erdélyi, 2016; Dabrowa et al., 2016). The first tracer diffusion experiment regarding the diffusion in HEAs has been reported in 2016 (Vaidya et al., 2016), pointing out that although the diffusion in NiCoFeCrMn is indeed slower than in NiCoFeCr under the same homologous temperature, it is faster with respect to the same absolute temperature. The same point has also been mentioned in a recent review on the HEAs concepts that the diffusion coefficient in NiCoFeCrMn0.5 is actually higher than the austenitic steels at the same absolute temperatures (Miracle and Senkov, 2017).
A recent interdiffusion experiment has further shown that, more alloying elements do not necessarily retard diffusion process, even with respect to homologous temperatures (Jin et al., 2018). Figure 4 shows the normalized activation energies for a series of Ni containing SP-CSAs (Tsai et al., 2013; Vaidya et al., 2016; Jin et al., 2018). The left panel shows the data from interdiffusion experiments, and the right panel shows those from tracer diffusions. The same color indicates the same number of alloying elements. Different from the original proposal for the sluggish diffusion (Tsai et al., 2013), no direct correlation between the number of elements and the diffusion parameter (even normalized to the melting temperatures) can be observed. For example, the normalized activation energy for the quinary NiCoFeCrPd is smaller than most other alloys containing less alloying elements. Furthermore, it needs to be emphasized that the uncertainties of diffusion parameters from different diffusion experiments or data analysis procedures can be inevitably large, as about one order for the diffusion coefficient and 15% for the activation energy (Million et al., 1981; Dabrowa et al., 2016; Miracle and Senkov, 2017; Jin et al., 2018). To this end, the difference in diffusion kinetics with respect to the number of alloying elements is further weakened.
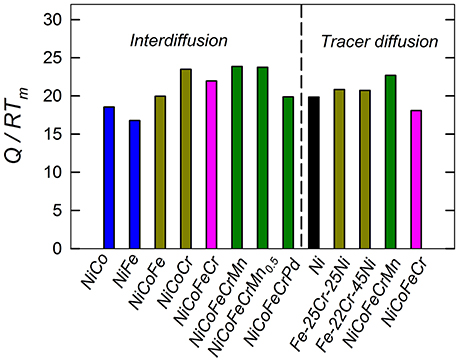
Figure 4. Normalized diffusion activation energy in several Ni-containing CSAs. Data adapted from Tsai et al. (2013), Vaidya et al. (2016), and Jin et al. (2018).
Other than the difficulties on accurate experimental quantification, the chemical disorder induced loss of translational invariance brings in much challenge for theoretical description of the defect transport behaviors in concentrated alloys. Defect behavior depends on its local environment, which is different from atom to atom due to the random distribution of chemical species. Unlike in pure metals, the defect energies are no longer single values but wide distributions in CSAs. Consequently, correct understanding the defect transport properties require large sampling effort, which is challenging especially to the first principles calculations (Zhao et al., 2017b).
Despite such challenges, theoretical calculations have been made recently to unveil the defect energies in a series of NiCoFeCrMn sub-systems, including NiCo, NiFe, Ni-20Cr, NiCoCr, and NiCoFeCr (Zhao et al., 2017a,b, 2018). The migration barriers for different elements in NiCoFeCr are shown in Figures 5A,B as a representative case (Zhao et al., 2018). The compositional effects on the interstitial and vacancy migration are qualitatively different. Compared with pure Ni, the interstitials in the CSAs have overall greater migration barriers (note again that the defect energies are distributions but not single values), and lower diffusion coefficients (Osetsky et al., 2016; Zhao et al., 2017a). On the contrary, the migration barriers of vacancies in NiFe, NiCoCr, and NiCoFeCr are generally much smaller than in pure Ni, especially through those preferable diffusion channels such as Fe in NiFe, and Cr in both NiCoCr and NiCoFeCr (Osetsky et al., 2016; Zhao et al., 2018) indicating a faster vacancy migration. One of the dominant physical origins has been proposed as the elemental-specific deformation flexibility of d-electrons (Zhao et al., 2018).
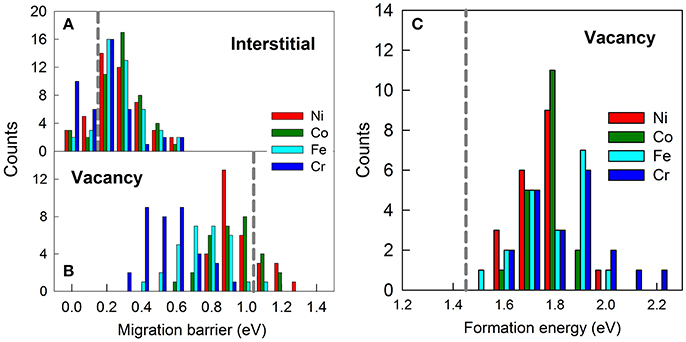
Figure 5. Migration barriers of (A) interstitials and (B) vacancies, as well as (C) vacancy formation energies in NiCoFeCr. The dash lines indicate the values for pure Ni. Data adapted from Zhao et al. (2018).
The much faster vacancy migration revealed by the ab initio calculations seem to be “contradict” to the thermal diffusion experiments (through vacancy mediated diffusion mechanism), at the first glance, that the experimental diffusion activation energy in the CSAs is generally not lower than in pure Ni although the number of element may not be a controlling factor; the faster diffusion in CSAs has not been observed in the thermal diffusion experiments. This “discrepancy” can be attributed to that the activation energy derived from the diffusion experiments is the addition of both vacancy migration barrier and the vacancy formation energy (Janotti et al., 2004), and the vacancy formation energy in the CSAs is higher than in pure Ni that compensates the lower migration barrier, as shown in Figure 5C. The direct quantification of the diffusion activation energy considering both contributions through calculations has not been available, probably because both energies are wide distributions and the summation is not trivial.
In addition to the ab initio based investigations, phenomenological methods such as the calculation of phase diagrams (CALPHAD) have been used to understand and, more importantly, to predict the diffusion behavior in multicomponent system using the database parameters obtained from its constituent lower-order systems (Zhang C. et al., 2017). The Ni tracer diffusion coefficient in all possible Ni-containing subsystems of NiCoFeCrMn has been calculated, based on a series of assessment and validation on the mobility database of the binary and ternary alloys in this family. The calculated diffusion coefficients have little dependent on the number of alloying elements, even with respect to the homologous temperature. The qualitative comparison with interdiffusion experiments has shown a good agreement, which may suggest that the diffusion kinetics of HEAs can be reasonably extrapolated from general concentrated solid-solution alloys containing less alloying elements (Jin et al., 2018).
Irradiation Resistance
Early systematic investigations on the irradiation response of concentrated alloys, while not equiatomic and not necessarily single-phase solid-solution, have been targeted primarily on the Fe-Cr-Ni based alloys, which are the prototypes of varieties of commercial alloys used or potentially used for nuclear engineering (e.g., stainless steels and Inconel series). For example, irradiation-induced swelling in about twenty Fe-Cr-Ni-based commercial alloys, pure Ni, and four Fe-15Cr-Ni model alloys with different Ni, Fe concentrations has been measured in Johnston et al. (1974). The concerns have been focused on the impacts of the concentration of these three alloying elements, additional dilute alloying elements, and the microstructures. These works have been comprehensively summarized in a number of review articles and textbooks e.g., Garner (2012) and Was (2007), and will not be further discussed in the present review. Here we focus on the recent investigations of the impact of principal alloying elements on irradiation response in this new set of FCC SP-CSAs with various number, type, and concentration of alloying elements that arises from the past decade. The most studied high and medium entropy alloy systems regarding irradiation effects in the past few years have been the FCC transition metal alloys, primarily the NiCoFeCr-M (M = Mn, Al, Cu, and Pd) and the binary and ternary subsystems of NiCoFeCr. The BCC structured alloys have been much less studied, although the studies on the thin film Zr-Hf-Nb near-equiatomic ternary alloys (while not single-phase) under MeV electron irradiation have been among the earliest experimental investigations of the phase stability that argue the high irradiation resistance of HEAs (Nagase et al., 2012, 2013).
Response to the Irradiation at Room and Low Temperatures
NiCoFeCr and its SP-CSA subsets in single crystalline form have been experimentally studied in response to Au and Ni ion irradiations at room temperature (Zhang et al., 2015, 2016; Granberg et al., 2016; Jin et al., 2016a,b; Lu et al., 2016a; Velişa et al., 2017a). All the studied alloys have shown lower overall lattice distortion compared with pure Ni in the relatively low dose regime, as characterized using ion channeling techniques. Among the alloys, the irradiation response of NiCo has been found the closest to pure Ni, followed by NiFe and NiCoFe that have shown similar behavior. The two Cr containing alloys, NiCoCr and NiCoFeCr, have shown the lowest disorder level, suggesting the highest irradiation resistance. The delay of damage accumulation in the compositionally complex alloys has also been demonstrated to occur at low temperature. At 16 K, where long-range thermal migration of defects is frozen, the damage accumulation is delayed from Ni, NiFe, to NiCoFeCr, and the maximum disorder level is decreased in the same sequence (Velişa et al., 2017b). These data brought up an implication of the importance of Cr addition, stimulating another experiments targeting on verifying this issue (Velişa et al., 2017a). Limited by the Cr concentration in the Ni-containing FCC SP-CSAs, non-equiatomic Ni-20Cr and NiFe-20Cr have been compared with corresponding Ni-20Fe and Ni-60Fe. Unlike the difference found between NiCoFe and NiCoCr, no significant difference was found between Ni-20Fe and Ni-20Cr, and between Ni-60Fe and NiFe-20Cr, from channeling measurements.
The damage accumulation process characterized by ion channeling has been further investigated with combined transmission electron microscopy (TEM), diffuse X-ray scattering, and nanoindentation. For example, ion channeling data have shown that the damage accumulation process is delayed with increasing Fe concentration in the binary Ni-xFe alloys up to 60 at%, the microstructure features of which have been further revealed by TEM observations: the average defect size (especially for interstitial loops) decreases greatly with increasing Fe concentration (Figures 6b,c), although the defect density is correspondingly increased (Jin et al., 2016b). The combined effects of the increasing density and decreasing size have been considered responsible to the invariance of irradiation hardening with varying Fe concentration, as characterized using nanoindentation (Jin et al., 2016b). The decreased defect size from pure Ni has also been observed using diffuse X-ray scatterings, even for NiCo as the closest case: the averaged size is reduced from 6.8 to 4.6 nm and from 2.3 to 1.5 nm for interstitial and vacancy type defects, respectively (Olsen et al., 2016). Moreover, the presence of monovacancies has been reported, through positron annihilation experiments, in NiCoFeCr under Ni ion irradiations even to a high dose of ~100 dpa (Abhaya et al., 2016).
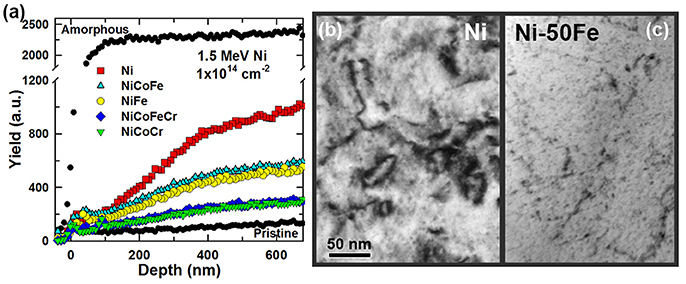
Figure 6. (a) Ion channeling spectra of several Ni-containing FCC SP-CSAs irradiated with 1.5 MeV Ni ions to the fluence of 1 × 1014 cm−2, adapted from Zhang et al. (2016). Reproduced with the permission of the copyright holder (Cambridge University Press). (b,c) are the TEM images of Ni and NiFe, respectively, irradiated with 1.5 MeV Ni ions to the fluence of 5 × 1015 cm−2. Adapted from Jin et al. (2016b). Reproduced with the permission of the copyright holder (Elsevier).
The phase stability of FCC SP-CSAs at room temperature has been examined in both NiCoFeCr (Abhaya et al., 2016) and NiCoFeCrAl0.1 (Xia et al., 2016) under high dose irradiations up to ~100 dpa. No observable secondary phase was observed in both studies. Little swelling was reported, as expected at this irradiation temperature.
Response to the Irradiations at Elevated Temperatures
Comparisons on the irradiation induced swelling up to 53 dpa have been made between Ni and a series of Ni-containing FCC SP-CSAs in single crystalline form at 500°C using step-height measurements (Jin et al., 2016c). Pure Ni has shown >6% overall swelling at this condition. In contrast to the conventional austenitic stainless steels, which are usually subject to higher swelling than pure Ni (Was, 2007), all the tested FCC SP-CSAs have exhibited lower swelling. The swelling of NiCo is about half of pure Ni, showing the weakest reduction among the alloys. Alloying with Fe and Cr can both further reduce the swelling of NiCo but to different extents: NiCoCr only moderately suppresses the swelling, but little swelling was observed in NiCoFe. The number of alloying elements is again not a critical factor: the swelling in both binary NiFe and quaternary NiCoFeCr is similar, and in between the two ternary alloys. Little swelling was observed in the quinary HEA, NiCoFeCrMn.
In addition to the overall swelling values, the void formation has been observed under cross-sectional TEM observation (Lu et al., 2016b; Yang et al., 2017). Two qualitatively different types of depth distributions have been observed among these materials. In pure Ni and NiCo, see Figure 7a as an example, the large voids are located at in the first 1300 nm, corresponding well to the predicted major displacement regime. In the other SP-CSAs, however, majority of the voids (although small) are distributed deeper than 1300 nm, beyond the displacement regime, as shown in Figure 7b for NiCoFeCr as a representative case. Further observations on the dislocation distribution have indicated that the interstitial-type dislocations are located deeper than the void regime in pure Ni and NiCo; for the other SP-CSAs the situation is reversed.
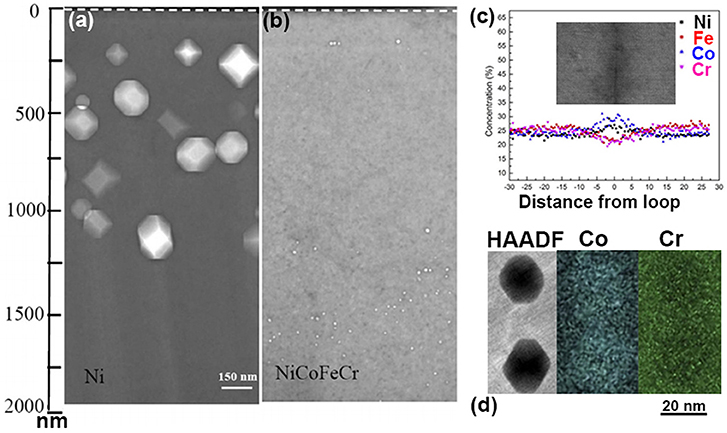
Figure 7. Cross-sectional TEM images for the void distributions in (a) Ni and (b) NiCoFeCr, and elemental distribution around (c) dislocations and (d) voids in NiCoFeCr, after 3 MeV Ni ion irradiations at 500°C. Adapted from Lu et al. (2016b) and Lu et al. (2017).
The temperature dependence of microstructure evolution under ion irradiations at elevated temperatures has been performed in FeNiMnCr0.66 to 10 dpa at 400–700°C (Kumar et al., 2016), and in NiCoFeCrAl0.1 to 31 dpa at 250–650°C (Yang et al., 2018). Unlike in Lu et al. (2016b), no voids have been observed in both cases at all the test temperatures. For the observable defect clusters, the density decreases but the size increases with increasing irradiation temperature (Kumar et al., 2016; Yang et al., 2018). However, the temperature dependence in the FeNiMnCr0.66 is less significant compared with the conventional Fe-Cr-Ni alloys. Moreover, considerably higher loop density and lower loop size have been reported in FeNiMnCr0.66, compared with those in the conventional Fe-Ni-Cr alloys (Kumar et al., 2016). Furthermore, higher fraction of faulted loops have been observed in the more compositionally complex alloys, indicating that increasing compositional complexity can extend the incubation period and delay loop growth (Lu et al., 2017). Note that irradiation temperature is a critical parameter for high temperature irradiation induced effects, e.g., swelling, and the temperature dependence varies with alloy compositions. The experimental studies till present primarily have focused either on one HEA at different irradiation temperatures (Kumar et al., 2016; Yang et al., 2018) or on the effects of compositional complexity at single irradiation temperature (Jin et al., 2016c; Lu et al., 2016b). How the number and species of principal alloying elements affect the temperature dependence of irradiation response in SP-CSAs requires future systematic studies.
The phase stability under elevated temperature irradiation has been another major topic studied for this series of SP-CSAs. No secondary phases have been observed in any of the above materials under ion irradiations (including NiFe, NiCoFe, NiCoFeCr, NiCoFeCrMn, FeNiMnCr0.66, and NiCoFeCrAl0.1) at all conditions, suggesting a great phase stability. However, the electron-irradiated NiCoFeCrMn and NiCoFeCrPd thin foils (TEM samples) have shown L10 (NiMn)-type ordering decomposition and < 001>-oriented spinodal decomposition between Co/Ni and Pd, respectively, even at a low dose of 1 dpa (He et al., 2017). While not being single-phase by itself, the FCC solid-solution phase portion in NiCoFeCrCu nano-crystalline sample also maintains high phase stability against electron irradiation at temperatures up to 500°C (Nagase et al., 2015).
The radiation-induced segregation (RIS) has been reported much suppressed in FeNiMnCr0.66, as compared with the Fe-Cr-Ni and Fe-Cr-Mn austenitic alloys (Kumar et al., 2016). Furthermore, a trend of reducing RIS level has been observed with increasing compositional complexity, from NiFe, NiCoFe, NiCoFeCr, to NiCoFeCrMn (Lu et al., 2017). For all the observed RIS cases, as representatively shown in Figures 7c,d, Ni and Co tend to enrich, but Cr, Fe, and Mn prefer to deplete near the defects (grain boundaries, loops, and voids) (He et al., 2017; Lu et al., 2017; Yang et al., 2018).
Linkage Between the Transport Properties and Irradiation Response
Ion irradiation generally involves atomic displacement and thermal spikes. Therefore, the (energy and mass) transport properties may affect the irradiation response of materials. Since external defect sinks appear minimum in the SP-CSAs, they provide a way to directly bridge the intrinsic transport properties to the irradiation response.
One of the contributions to the delayed damage accumulation in SP-CSAs under room temperature irradiation has been proposed as the reduced thermal conductivity (Zhang et al., 2015). The physics picture has been intuitively described as follows: the reduced thermal conductivity can slow down the dissipation of the deposited heat, lengthen the thermal spike, which enhances the lifetime of thermally enhanced recombination stage (Ullah et al., 2016; Zhang Y. et al., 2017). Experimental support of this hypothesis has been seen from the correlation between thermal conductivity and damage level of the Ni-containing equiatomic SP-CSAs under low dose room temperature irradiations (e.g., comparing Figures 2, 6a). Both thermal conductivity and irradiation-induced damage level decreases from Ni, NiCo, NiFe/NiCoFe, to NiCoFeCr/NiCoCr. This hypothesis has also been supported by the two-temperature model in molecular dynamics simulation, showing that reduced thermal conductivity can potentially contribute to the quenching and annealing of the damage, leading to decreased number of point defects (Zarkadoula et al., 2016). However, the impact of thermal conductivity has also been reported not dominant, in a simpler comparison, for example, although Ni-20Fe has much higher thermal conductivity than Ni-20Cr, they seem to have similar damage accumulation rate from the view of ion channeling, which suggests other controlling factors (Velişa et al., 2017a).
The defect evolution during irradiation contains stages of defect production from collision cascades, in-cascade vacancy-interstitial recombination, and the subsequent prolonged migration process that causes the aggregation or annihilation of defects. Thus, the mass transport properties, i.e., defect diffusion kinetics can critically affect the eventual irradiation response of materials. As discussed in section Mass Transport Properties: Atomic Diffusion Behavior, the most apparent feature among the mass transport properties observed in SP-CSAs is the reduced separation, or even overlapping, of the migration barriers between interstitial and vacancies, compared with pure metals or dilute alloys in which interstitials usually migrates much faster than vacancies (Zhao et al., 2018). In other words, the interstitial diffusion is retarded while the vacancy migration is promoted.
The impact of sluggish interstitial diffusion has been observed from several perspectives. First, as discussed for the cases of Ni-xFe, FeNiMnCr0.66, etc., the size of interstitial defect clusters in the SP-CSAs is much smaller than in pure metals and conventional alloys, due to the slow aggregation of interstitials (Jin et al., 2016b; Kumar et al., 2016). Second, the irradiation induced damage range in the single-crystalline complex alloys is reduced: cross-sectional TEM images have shown that the damage range in NiFe is shallower than that in pure Ni (Lu et al., 2016a).
Further combined with the fast vacancy migration, the close migration barriers between interstitials and vacancies have also shown strong impact of irradiation response. First, it has been considered to reduce of surviving defects in SP-CSAs under room and low temperature irradiations, by reducing the separation of interstitial and vacancy populations, enhancing the their interactions, and further promoting the defect recombination (Velişa et al., 2017b; Zhang Y. et al., 2017). Second, this mechanism has been considered to attribute to the qualitative different void distribution and the reduced swelling in SP-CSAs (see Figures 7a,b): Interstitials migrate fast in Ni and NiCo, leaving the vacancies behind in the matrix oversaturated and aggregated into large voids. In concentrated alloys, on the contrary, much more vacancies and interstitials recombine in the displacement regime, suppressing the formation of voids, and small amount of fast migrating vacancies reach beyond the end of irradiation depth, forming small voids (Lu et al., 2016b).
The investigations on both irradiation response and transport properties of SP-CSAs with multiple principal elements (HEAs) are at the frontier of materials science. While their correlations have been presented, identifications of the roles of each aspect of compositional complexity have not been available. For example, clarifying the effects of the number of alloying elements is challenging, since although considerable differences have been observed between HEAs and the compositionally simpler alloys, few of the reported properties (both transport properties and irradiation response) monotonously change with the number of elements. Moreover, analyzing the role of each constituent becomes more difficult with increasing number of elements, since each added element interacts with all species in the original alloys and also affects their mutual interaction. Therefore, future experimental and theoretical investigations with careful control of variants among the three aspects of compositional complexity, number, type, and concentration of alloying elements, are demanded to better understand how modification of compositional complexity can affect the irradiation response through tailoring the transport properties (Figure 8).
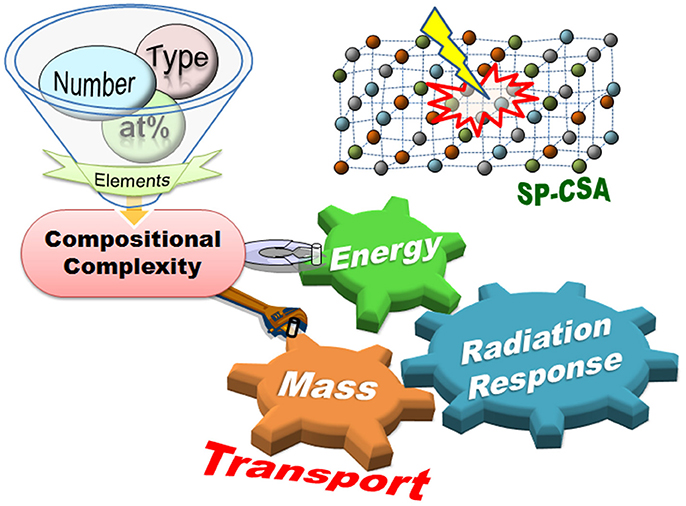
Figure 8. Schematic drawing of the concept of enhancing irradiation resistance from modifying the energy and mass transport properties with the control of compositional complexity.
Summaries
Reduced electronic and thermal conductivity, retarded interstitial diffusion, easier vacancy migration, and harder vacancy formation have been observed in recent studies in a novel class of alloys, SP-CSAs. These intrinsic transport properties have been found to significantly impact their irradiation response, delay the damage accumulation, and suppress the interstitial aggregation and volume swelling. Bridging the intrinsic transport properties to the irradiation response has pushed forward the understanding on mechanisms of energy dissipation and defect evolution at the atomic and electronic level. The demonstrated capability of controlling irradiation response in SP-CSAs by modifying intrinsic transport properties without changing the microstructure may stimulate new alloy design strategies for the next generation nuclear structural alloys. However, although descriptive correlations between intrinsic transport properties and the irradiation response have been proposed, a predictive model that can quantitatively evaluate the compositional effects on defect evolution in SP-CSAs has not been available, and other factors than the transport properties such as the stacking fault energy and local atomic stress can also affect the irradiation response simultaneously; Further theoretical and experimental investigations are demanded to fill such knowledge gaps to further extract the potential of this attractive alloy family on energy applications.
Author Contributions
KJ and HB together finished this review, including analyzing, writing, and figures.
Conflict of Interest Statement
The authors declare that the research was conducted in the absence of any commercial or financial relationships that could be construed as a potential conflict of interest.
Acknowledgments
The work was supported as part of the Energy Dissipation to Defect Evolution (EDDE), an Energy Frontier Research Center funded by the U.S. Department of Energy, Office of Science, Basic Energy Sciences. The authors thank Dr. Shijun Zhao at the Oak Ridge National Laboratory for fruitful discussions and suggestions.
References
Abhaya, S., Rajaraman, R., Kalavathi, S., David, C., Panigrahi, B. K., and Amarendra, G. (2016). Effect of dose and post irradiation annealing in Ni implanted high entropy alloy FeCrCoNi using slow positron beam. J. Alloy Compond. 669, 117–122. doi: 10.1016/j.jallcom.2016.01.242
Banerjee, S., and Raychaudhuri, A. K. (1994). Electrical resistivities of γ-phase FexNi80–xCr20 alloys. Phys. Rev. B 50, 8195–8206. doi: 10.1103/PhysRevB.50.8195
Beke, D. L., and Erdélyi, G. (2016). On the diffusion in high-entropy alloys. Mater. Lett. 164, 111–113. doi: 10.1016/j.matlet.2015.09.028
Cantor, B. I., Chang, T. H., Knight, P., and Vincent, A. J. B. (2004). Microstructural development in equiatomic multicomponent alloys. Mater. Sci. Eng. A 375–377, 213–218. doi: 10.1016/j.msea.2003.10.257
Caro, M., Béland, L. K., Samolyuk, G. D., Stoller, R. E., and Caro, A. (2015). Lattice thermal conductivity of multi-component alloys. J. Alloy. Comp. 648, 408–413. doi: 10.1016/j.jallcom.2015.06.035
Chakraborty, S., and Majumdar, A. K. (1998). Electron transport studies in Ni-rich γ-NiFeCr alloys. J. Mag. Mag. Mater. 186, 357–372. doi: 10.1016/S0304-8853(98)00053-5
Chou, H., Chang, Y., Chen, S., and Yeh, J. (2009). Microstructure, thermophysical and electrical properties in AlxCoCrFeNi (0≤x≤2) high-entropy alloys. Mater. Sci. Eng. B 163, 184–189. doi: 10.1016/j.mseb.2009.05.024
Dabrowa, J., Kucza, W., Cieślak, G., Kulik, T., Danielewski, M., and Yeh, J.-W. (2016). Interdiffusion in the FCC-structured Al-Co-Cr-Fe-Ni high entropy alloys: experimental studies and numerical simulations. J. Alloy Comp. 674, 455–462. doi: 10.1016/j.jallcom.2016.03.046
Fan, Z., Wang, H., Wu, Y., Liu, X. J., and Lu, Z. P. (2016). Thermoelectric high-entropy alloys with low lattice thermal conductivity. RSC Advances 6, 52164–52170. doi: 10.1039/C5RA28088E
Fan, Z., Wang, H., Wu, Y., Liu, X., and Lu, Z. (2017). Thermoelectric performance of PbSnTeSe high-entropy alloys. Mater. Res. Lett. 5, 187–194. doi: 10.1080/21663831.2016.1244116
Garner, F. A. (2012). Radiation damage in austenitic steels, compreh. Nucl. Mater. 4, 33–95. doi: 10.1016/B978-0-08-056033-5.00065-3
Gludovatz, B., Hohenwarter, A., Thurston, K. V., Bei, H., Wu, Z., George, E. P., et al. (2016). Exceptional damage-tolerance of a medium-entropy alloy CrCoNi at cryogenic temperatures. Nat. Commun. 7:10602. doi: 10.1038/ncomms10602
Granberg, F., Nordlund, K., Ullah, M. W., Jin, K., Lu, C., Bei, H., et al. (2016). Mechanism of radiation damage reduction in equiatomic multicomponent single phase alloys. Phys. Rev. Lett. 116:135504. doi: 10.1103/PhysRevLett.116.135504
Guo, S., and Liu, C. T. (2011). Phase stability in high entropy alloys: formation of solid-solution phase or amorphous phase. Prog. Nat. Sci. 21, 433–446. doi: 10.1016/S1002-0071(12)60080-X
He, M.-R., Wang, S., Shi, S., Jin, K., Bei, H., Yasuda, K., et al. (2017). Mechanisms of radiation-induced segregation in CrFeCoNi-based single-phase concentrated solid solution alloys. Acta Mater. 126, 182–193. doi: 10.1016/j.actamat.2016.12.046
Ho, C. Y., Ackerman, M. W., Wu, K. Y., Havill, T. N., Bogaard, R. H., Matula, R. A., et al. (1983). Electrical resistivity of ten selected binary alloy systems. J. Phys. Chem. Ref. Data 12:183. doi: 10.1063/1.555684
Janotti, A., Krčmar, M., Fu, C. L., and Reed, R. C. (2004). Solute Diffusion in metals: larger atoms can move faster. Phys. Rev. Lett. 92:085901. doi: 10.1103/PhysRevLett.92.085901
Jin, K., Bei, H., and Zhang, Y. (2016a). Ion irradiation induced defect evolution in Ni and Ni-based FCC equiatomic binary alloys. J. Nucl. Mater. 471, 193–199. doi: 10.1016/j.jnucmat.2015.09.009
Jin, K., Guo, W., Lu, C., Ullah, M. W., Zhang, Y., Weber, W. J., et al. (2016b). Effects of Fe concentration on the ion-irradiation induced defect evolution and hardening in Ni-Fe solid solution alloys. Acta Mater. 121, 365–373. doi: 10.1016/j.actamat.2016.09.025
Jin, K., Lu, C., Wang, L. M., Qu, J., Weber, W. J., Zhang, Y., et al. (2016c). Effects of compositional complexity on the ion-irradiation induced swelling and hardening in Ni-containing equiatomic alloys. Scripta Mater. 119, 65–70. doi: 10.1016/j.scriptamat.2016.03.030
Jin, K., Mu, S., An, K., Porter, W. D., Samolyuk, G. D., Stocks, G. M., et al. (2017). Thermophysical properties of Ni-containing single-phase concentrated solid solution alloys. Mater. Des. 117, 185–192. doi: 10.1016/j.matdes.2016.12.079
Jin, K., Sales, B. C., Stocks, G. M., Samolyuk, G. D., Daene, M., Weber, W. J., et al. (2016d). Tailoring the physical properties of Ni-based single-phase equiatomic alloys by modifying the chemical complexity. Sci. Rep. 6:20159. doi: 10.1038/srep20159
Jin, K., Zhang, C., Zhang, F., and Bei, H. (2018). Influence of compositional complexity on interdiffusion in Ni-containing concentrated solid-solution alloys. Mater. Res. Lett. 6, 293–299. doi: 10.1080/21663831.2018.1446466
Johnston, W. G., Rosolowski, J. H., Turkalo, A. M., and Lauritzen, T. (1974). An experimental survey of swelling in commercial Fe-Cr-Ni alloys bombarded with 5 MeV Ni Ions. J. Nucl. Mater. 54, 24–40. doi: 10.1016/0022-3115(74)90073-7
Kao, Y., Chen, S., Chen, T., Chu, P., Yeh, J., and Lin, S. (2011). Electrical, magnetic, and Hall properties of AlxCoCrFeNi high-entropy alloys. J. Alloy. Comp. 509, 1607–1614. doi: 10.1016/j.jallcom.2010.10.210
Klemens, P. G., and Williams, R. K. (1986). Thermal conductivity of metals and alloys. Int. Metal. Rev. 31, 197–215. doi: 10.1179/095066086790324294
Körmann, F., Ikeda, Y., Grabowski, B., and Sluiter, M. H. F. (2017). Phonon broadening in high entropy alloys. NPJ Comp. Mater. 3:36. doi: 10.1038/s41524-017-0037-8
Koželj, P., Vrtnik, S., Jelen, A., Jazbec, S., Jagličić, Z., Maiti, S., Feuerbacher, M., et al. (2014). Discovery of a superconducting high-entropy alloy. Phys. Rev. Lett. 113:107001. doi: 10.1103/PhysRevLett.113.107001
Kumar, N. A. P. K., Li, C., Leonard, K. J., Bei, H., and Zinkle, S. J. (2016). Microstructural stability and mechanical behavior of FeNiMnCr high entropy alloy under ion irradiation. Acta Materialia 113, 230–244. doi: 10.1016/j.actamat.2016.05.007
Lee, J. I., Oh, H. S., and Park, E. S. (2016). Manipulation of σy/κ ratio in single phase FCC solid-solutions. Appl. Phys. Lett. 109:061906. doi: 10.1063/1.4960809
Lu, C., Jin, K., Beland, L. K., Zhang, F., Yang, T., Qiao, L., et al. (2016a). Direct observation of defect range and evolution in ion-irradiated single crystalline Ni and Ni binary alloys. Sci. Rep. 6:19994. doi: 10.1038/srep19994
Lu, C., Niu, L., Chen, N., Jin, K., Yang, T., Xiu, P., et al. (2016b). Enhancing radiation tolerance by controlling defect mobility and migration pathways in multicomponent single-phase alloys. Nat. Commun. 7:13564. doi: 10.1038/ncomms13564
Lu, C., Yang, T., Jin, K., Gao, N., Xiu, P., Zhang, Y., et al. (2017). Radiation-induced segregation on defect clusters in single-phase concentrated solid-solution alloys. Acta Mater. 127, 98–107. doi: 10.1016/j.actamat.2017.01.019
Lucas, M. S., Wilks, G. B., Mauger, L., Muñoz, J. A., Senkov, O. N., Michel, E., et al. (2012). Absence of long-range chemical ordering in equimolar FeCoCrNi. Appl. Phys. Lett. 100:251907. doi: 10.1063/1.4730327
Million, B., Ružičková, J., Velíšek, J., and Vreštál, J. (1981). Diffusion processes in the Fe-Ni system. Mater. Sci. Eng. 50, 43–52. doi: 10.1016/0025-5416(81)90084-7
Miracle, D. B., and Senkov, O. N. (2017). A critical review of high entropy alloys and related concepts. Acta Mater. 122, 448–511. doi: 10.1016/j.actamat.2016.08.081
Mooij, J. H. (1972). Structural and electrical properties of sputtered CrNi films. J. Vac. Sci. Tech. 9:446. doi: 10.1116/1.1316651
Nagase, T., Anada, S., Rack, P. D., Noh, J. H., Yasuda, H., Mori, H., et al. (2012). Electron-irradiation-induced structural change in Zr–Hf–Nb alloy. Intermetallics 26, 122–130. doi: 10.1016/j.intermet.2012.02.015
Nagase, T., Anada, S., Rack, P. D., Noh, J. H., Yasuda, H., Mori, H., et al. (2013). MeV electron-irradiation-induced structural change in the bcc phase of Zr–Hf–Nb alloy with an approximately equiatomic ratio. Intermetallics 38, 70–79. doi: 10.1016/j.intermet.2013.02.009
Nagase, T., Rack, P. D., Noh, J. H., and Egami, T. (2015). In-situ TEM observation of structural changes in nano-crystalline CoCrCuFeNi multicomponent high-entropy alloy (HEA) under fast electron irradiation by high voltage electron microscopy (HVEM). Intermetallics 59, 32–42. doi: 10.1016/j.intermet.2014.12.007
Nath, T. K., and Majumdar, A. K. (1996). Resistivity saturation in substitutionally disordered γ-Fe80–xNixCr20 (14≤x≤30) alloys. Phys. Rev. B 53, 12148–12159. doi: 10.1103/PhysRevB.53.12148
Olsen, R. J., Jin, K., Lu, C., Beland, L. K., Wang, L., Bei, H., et al. (2016). Investigation of defect clusters in ion-irradiated Ni and NiCo using diffuse X-ray scattering and electron microscopy. J. Nucl. Mater. 469, 153–161. doi: 10.1016/j.jnucmat.2015.11.030
Osetsky, Y. N., Béland, L. K., and Stoller, R. E. (2016). Specific features of defect and mass transport in concentrated fcc alloys. Acta Mater. 115, 364–371. doi: 10.1016/j.actamat.2016.06.018
Sales, B. C., Jin, K., Bei, H., Nichols, J., Chisholm, M. F., May, A. F., et al. (2017). Quantum critical behavior in the asymptotic limit of high disorder in the medium entropy alloy NiCoCr0.8. npj Quant. Mater. 2:33. doi: 10.1038/s41535-017-0042-7
Sales, B. C., Jin, K., Bei, H., Stocks, G. M., Samolyuk, G. D., May, A. F., et al. (2016). Quantum critical behavior in a concentrated ternary solid solution. Sci. Rep. 6:26179. doi: 10.1038/srep26179
Senkov, O. N., Wilks, G. B., Scott, J. M., and Miracle, D. B. (2011). Mechanical properties of Nb25Mo25Ta25W25 and V20Nb20Mo20Ta20W20 refractory high entropy alloys. Intermetallics 19, 698–706. doi: 10.1016/j.intermet.2011.01.004
Shafeie, S., Guo, S., Hu, Q., Fahlquist, H., Erhart, P., and Palmqvist, A. (2015). High-entropy alloys as high-temperature thermoelectric materials. J. Appl. Phys. 118:184905. doi: 10.1063/1.4935489
Tsai, K. Y., Tsai, M. H., and Yeh, J. W. (2013). Sluggish diffusion in Co–Cr–Fe–Mn–Ni high-entropy alloys. Acta Mater. 61, 4887–4897. doi: 10.1016/j.actamat.2013.04.058
Ukai, S., and Fujiwara, M. (2002). Perspective of ODS alloys application in nuclear environments. J. Nucl. Mater. 307–311, 749–757. doi: 10.1016/S0022-3115(02)01043-7
Ullah, M. W., Aidhy, D. S., Zhang, Y., and Weber, W. J. (2016). Damage accumulation in ion-irradiated Ni-based concentrated solid-solution alloys. Acta Materialia 109, 17–22. doi: 10.1016/j.actamat.2016.02.048
Vaidya, M., Trubel, S., Murty, B. S., Wilde, G., and Divinski, S. V. (2016). Ni tracer diffusion in CoCrFeNi and CoCrFeMnNi high entropy alloys. J. Alloy Comp. 688, 994–1001. doi: 10.1016/j.jallcom.2016.07.239
Velişa, G., Ullah, M. W., Xue, H., Jin, K., Crespillo, M. L., Bei, H., et al. (2017a). Irradiation-induced damage evolution in concentrated Ni-based alloys. Acta Mater. 135, 54–60. doi: 10.1016/j.actamat.2017.06.002
Velişa, G., Wendler, E., Zhao, S., Jin, K., Bei, H., Weber, W. J., et al. (2017b). Delayed damage accumulation by athermal suppression of defect production in concentrated solid solution alloys. Mater. Res. Lett. 6, 136–141. doi: 10.1080/21663831.2017.1410863
Was, G. S. (2007). Fundamentals of Radiation Materials Science: Metals and Alloys. Berlin; Heidelberg; New York, NY: Springer.
Wu, W.-H., Yang, C. C., and Yeh, J. W. (2006). Industrial development of high-entropy alloys. Ann. Chim. Sci. Mat. 31, 737–747. doi: 10.3166/acsm.31.737-747
Wu, Z., Bei, H., Otto, F., Pharr, G. M., and George, E. P. (2014). Recovery, recrystallization, grain growth and phase stability of a family of FCC-structured multi-component equiatomic solid solution alloys. Intermetallics 46, 131–140. doi: 10.1016/j.intermet.2013.10.024
Xia, S., Gao, M. C., Yang, T., Liaw, P. K., and Zhang, Y. (2016). Phase stability and microstructures of high entropy alloys ion irradiated to high doses. J. Nucl. Mater. 480, 100–108. doi: 10.1016/j.jnucmat.2016.08.017
Yamasaki, M., Kagao, S., and Kawamura, Y. (2005). Thermal diffusivity and conductivity of Zr55Al10Ni5Cu30 bulk metallic glass. Scripta Mater. 53, 63–67. doi: 10.1016/j.scriptamat.2005.03.021
Yang, T., Xia, S., Guo, W., Hu, R., Poplawsky, J. D., Sha, G., et al. (2018). Effects of temperature on the irradiation responses of Al 0.1 CoCrFeNi high entropy alloy. Scripta Mater. 144, 31–35. doi: 10.1016/j.scriptamat.2017.09.025
Yang, T.-N., Lu, C., Jin, K., Crespillo, M. L., Zhang, Y., Wang, L., et al. (2017). The effect of injected interstitials on void formation in self-ion irradiated nickel containing concentrated solid solution alloys. J. Nucl. Mater. 488, 328–337. doi: 10.1016/j.jnucmat.2017.02.026
Yeh, J. W. (2006). Recent progress in high entropy alloys. Ann. Chim. Sci. Mat. 31, 633–648. doi: 10.3166/acsm.31.633-648
Yeh, J. W., Chen, S. K., Lin, S. J., Gan, J. Y., Chin, T. S., Shun, T. T., et al. (2004). Nanostructured high-entropy alloys with multiple principal elements: novel alloy design concepts and outcomes. Adv. Eng. Mater. 6, 299–303. doi: 10.1002/adem.200300567
Zarkadoula, E., Samolyuk, G., Xue, H., Bei, H., and Weber, W. J. (2016). Effects of two-temperature model on cascade evolution in Ni and NiFe. Scripta Mater. 124, 6–10. doi: 10.1016/j.scriptamat.2016.06.028
Zhang, C., Zhang, F., Jin, K., Bei, H., Chen, S., Cao, W., et al. (2017). Understanding of the elemental diffusion behavior in concentrated solid solution alloys. J. Phase Equilib. Diffus. 38, 434–444. doi: 10.1007/s11669-017-0580-5
Zhang, K. B., and Fu, Z. Y. (2012). Effects of annealing treatment on properties of CoCrFeNiTiAlx multi-component alloys. Intermetallics 28, 34–39. doi: 10.1016/j.intermet.2012.03.059
Zhang, Y., Jin, K., Xue, H., Lu, C., Olsen, R. J., Beland, L. K., et al. (2016). Influence of chemical disorder on energy dissipation and defect evolution in advanced alloys. J. Mater. Res. 31, 2363–2375. doi: 10.1557/jmr.2016.269
Zhang, Y., Stocks, G. M., Jin, K., Lu, C., Bei, H., Sales, B. C., et al. (2015). Influence of chemical disorder on energy dissipation and defect evolution in concentrated solid solution alloys. Nat. Commun. 6:8736. doi: 10.1038/ncomms9736
Zhang, Y., Zhao, S., Weber, W. J., Nordlund, K., Granberg, F., and Djurabekova, F. (2017). Atomic-level heterogeneity and defect dynamics in concentrated solid-solution alloys. Curr. Opin. Solid State Mater. Sci. 21, 221–237. doi: 10.1016/j.cossms.2017.02.002
Zhang, Y., Zuo, T. T., Tang, Z., Gao, M. C., Dahmen, K. A., Liaw, P. K., et al. (2014). Microstructures and properties of high-entropy alloys. Prog. Mater. Sci. 61, 1–93. doi: 10.1016/j.pmatsci.2013.10.001
Zhang, Y., Zuo, T., Cheng, Y., and Liaw, P. K. (2013). High-entropy alloys with high saturation magnetization, electrical resistivity, and malleability. Sci. Rep. 3:1455. doi: 10.1038/srep01455
Zhao, S., Egami, T., Stocks, G. M., and Zhang, Y. (2018). Effect of d electrons on defect properties in equiatomic NiCoCr and NiCoFeCr concentrated solid solution alloys. Phys. Rev. Mater. 2:013602. doi: 10.1103/PhysRevMaterials.2.013602
Zhao, S., Osetsky, Y., and Zhang, Y. (2017a). Preferential diffusion in concentrated solid solution alloys: NiFe, NiCo and NiCoCr. Acta Mater. 128, 391–399. doi: 10.1016/j.actamat.2017.01.056
Zhao, S., Weber, W. J., and Zhang, Y. (2017b). Unique challenges for modeling defect dynamics in concentrated solid-solution alloys. JOM 69, 2084–2091. doi: 10.1007/s11837-017-2461-0
Keywords: solid-solution alloys, high entropy alloys, thermal conductivity, electrical resistivity, radiation damage, diffusion
Citation: Jin K and Bei H (2018) Single-Phase Concentrated Solid-Solution Alloys: Bridging Intrinsic Transport Properties and Irradiation Resistance. Front. Mater. 5:26. doi: 10.3389/fmats.2018.00026
Received: 25 January 2018; Accepted: 12 April 2018;
Published: 30 April 2018.
Edited by:
Yong Yang, City University of Hong Kong, Hong KongReviewed by:
Michael Gao, National Energy Technology Laboratory (DOE), United StatesHaihui Ruan, Hong Kong Polytechnic University, Hong Kong
This material is published by permission of UT-Battelle, LLC, for the US Department of Energy under Contract No. DE-AC05-00OR22725. The US Government retains for itself, and others acting on its behalf, a paid-up, non-exclusive, and irrevocable worldwide license in said article to reproduce, prepare derivative works, distribute copies to the public, and perform publicly and display publicly, by or on behalf of the Government. This is an Open Access article and currently distributed under the terms of the Creative Commons Attribution License, which permits the use, distribution and reproduction of published articles, provided that the original authors and source are credited, and subject to any copyright notices concerning any third-party content.
*Correspondence: Ke Jin, jink@ornl.gov
Hongbin Bei, beih@ornl.gov