- 1State Key Laboratory of Inorganic Synthesis & Preparative Chemistry, College of Chemistry, Jilin University, Changchun, China
- 2Key Laboratory of Polyoxometalate Science of Ministry of Education, Department of Chemistry, Northeast Normal University, Changchun, China
Porous organic frameworks (POFs) are a new family of porous materials, which are characterized by high chemical and thermal stabilities, structural design-abilities, chemical functionalities, high porosities, etc. Designable skeletons of POFs lead to different applications in many fields. Herein, we report a porous aromatic framework PAF-72 synthesized via ionothermal reaction. The inherently nitrogen contained skeleton of PAF-72 is beneficial for introducing catalytic active center Ruthenium, which is verified by ICP, TGA, and TEM element mapping. Besides, Ru/PAF-72 shows excellent activity in hydrogen generation from hydrolysis of ammonia borane and good cyclic performance.
Introduction
Hydrogen is the most abundant element on earth and the molecular hydrogen gas, H2, is one of the cleanest energies we can utilize (Schlapbach and Zuttel, 2001). The product of combustion is water, which is also environmentally friendly. However, the storage and transportation remain as the main problems for hydrogen energy, which restrict its development considerably. Hydrogen storage materials, such as chemical hydride, adsorption of hydrogen storage material, and metal hydride have been widely studied in recent years (Züttel, 2003; Graetz, 2009). Ammonia borane (AB), which has high stability and high hydrogen content (19.6 wt% and 146 g L−1), makes it as a potential and ideal hydrogen storage material (Peng and Chen, 2008; Rossin and Peruzzini, 2016). Hydrogen can be released from AB by pyrolysis or hydrolysis process. Pyrolysis usually happens at high temperature conditions with accompanying by-products (Hu et al., 1978; Frueh et al., 2011). In contrast, hydrolysis happens under mild conditions, and metal catalysts can accelerate the procedure (Fu et al., 2018; Chen et al., 2019). The aggregation of metal catalysts is a main problem that can decrease the activity of catalysis and cyclic utilization (Zhang et al., 2018). So, it is helpful to disperse the metal catalyst in porous frameworks and anchor them in a skeleton (Qin et al., 2018).
From the last decade, porous organic frameworks (POFs), which are usually constructed from wholly organic building units, have enjoyed a good development because of their high stability, structural design-abilities, chemical functionalities, and high porosities (Zhu and Ren, 2015; Das et al., 2017; Tan and Tan, 2017). Current investigations have demonstrated that POFs are promising materials in many fields, such as gas storage, separation, heterogeneous catalysis, and sensors. Since the polymers of intrinsic microporosity (PIM) was reported by Budd et al. (2004), the covalent organic frameworks (COFs) (Cote et al., 2005; El-Kaderi et al., 2007), conjugated microporous polymers (CMP) (Jiang et al., 2007; Li et al., 2011), covalent triazine-based frameworks (CTFs) (Kuhn et al., 2008), and porous aromatic frameworks (PAFs) (Ben et al., 2009; Peng et al., 2011; Li et al., 2018) have enriched the type of POF materials.
Ru/Carbon reported by Liang et al. shows excellent efficiency for hydrolysis of AB with high turnover frequency (TOF) value of 429 mol (H2) min−1 mol−1 (Liang et al., 2012). However, Ru/Carbon shows low stability indicated by recycling tests. As an outstandingly qualified catalyst, it should have excellent recyclability. Normally, the issue of the aggregation of catalysts or the exfoliation of catalysts is the decisive factor. In this paper, we present the design and synthesis of PAF-72 with small pore size and N-decorated skeleton, which is employed as a scaffold to anchor Ru for hydrolysis AB under mild conditions. 9,10-dicyanoanthracene (DCA) containing nitrile group was used as a building unit to construct PAF material (PAF-72) via ionothermal reaction. Adequate characterizations have been carried out to confirm the structure feature and pore properties of the PAF-72. Additionally, the porous property was explored by N2, CO2, and CH4 sorptions. Furthermore, we presented the Ru/PAF-72 for hydrogen generation from hydrolysis of ammonia borane (AB). Because of the N-decorated skeleton and suitable pore size, it is effective to avoid the falling off and uniting of Ru. Therefore, Ru/PAF-72 shows an excellent catalytic activity and recycling performance.
Experimental
Synthesis of PAF-72
Synthesis of PAF-72 was conducted under ionothermal condition (Figure 1A). Typically, DCA (228 mg, 1 mmol), and anhydrous ZnCl2 (136 mg, 1 mmol) were mixed and grinded in the glove box. Then the mixture was transferred into a Pyrex ampoule (1 × 12 cm). The ampoule was flame-sealed under vacuum and was placed in a muffle furnace at 400°C for 40 h. After cooling down to room temperature, the ampoule was opened carefully and the crude product was stirred in H2O (10 mL) and refreshed three times to remove ZnCl2, and then washed with 1M HCl (3 × 10 mL), THF (3 × 10 mL), and dichloromethane (10 mL) to purify the product. Finally, the powder was dried overnight in an oven at 100°C to give PAF-72 as a black particle (98% yield).
Synthesis of RuCl3 and PAF-72 Mixture
PAF-72 was degassed at 120°C for 12 h before use. RuCl3·nH2O (10 mg) was dissolved in methanol (2 mL) and then PAF-72 (10 mg) was added into the solution. The mixture was stirred 12 h for incorporating thoroughly. After filtration, the product was wash by methanol completely to remove the RuCl3·nH2O at surface. Finally, the powder was dried overnight in oven at 100°C.
Synthesis of Ru/PAF-72 Catalysts
Ru/PAF-72 was synthesized in situ by reducing the RuCl3 using AB in aqueous solution.
Catalytic Hydrolysis of AB
Catalytic testing of Ru/PAF-72 for hydrolytic dehydrogenation of AB was carried out at 298 K under atmospheric pressure. Ru/PAF-72 (10 mg) was placed in a two-necked glass container and aqueous AB solution (2 mL) was injected via a syringe. The mixture was mixed by using a magnetic stirrer, and the generated volume of H2 was measured by a water-filled burette. For durability tests, the reactions were repeated 10 times. The first cycle refers to the reduction of Ru3+ by AB and simultaneous AB hydrolysis reaction. When the hydrolysis process was completed, Ru/PAF-72 was filtered to separate from the mixture for the next cycle.
Results and Discussion
The structure was confirmed firstly by comparison of the FTIR spectra of PAF-72 and DCA. As shown in Figure 1B, the disappearance of nitrile group characteristics peak at 2,218 cm−1 of DCA demonstrated the successful involvement of DCA in the ionothermal reaction, and the appearance of peaks at 1,585 and 1,203 cm−1 indicated that the triazine ring was synthesized as desired. The XPS spectrum of Zn element showed no residual Zn element in PAF-72 (Figure S1).
Powder X-ray diffraction (PXRD) was carried out to discover the crystallinity of Figure S2. There were no intense peaks but only a broad peak which shows that PAF-72 is amorphous. This might be caused by the benzene of anthracene becoming twisted, which causes that the adjacent layers to be not completely parallel. Scanning electron microscopy (SEM) shows that the PAF-72 is in irregular blocks with nanometer dimensions (Figure 2A). Transmission electron microscopy (TEM) also indicates the amorphous texture of PAF-72 (Figure 2B). As shown in Figure 2C, the Ru nanoparticles in Ru/PAF-72 are dispersed well in the skeleton. Based on the statistics of nano measurements, the size of Ru nanoparticles is ~1–2 nm (Figure 2D). The image of TEM mapping also indicates that Ru is dispersed clearly (Figure 3).
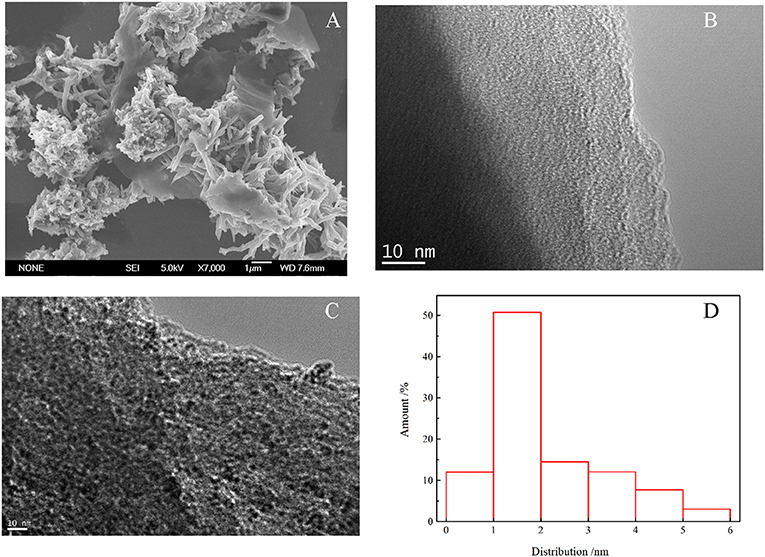
Figure 2. (A) SEM of PAF-72, (B) TEM of PAF-72, (C) TEM of Ru/PAF-72, and (D) Ru particles diameter distribution.
The existence of Ru influences N element in the skeleton from X-ray photoelectron spectroscopy (XPS) spectrum. PAF-72 exhibits N 1 s signal at 400.10 eV, which is shifted into 399.53 eV after loading Ru (Figure 4A). The Ru content in PAF-72 is 2.2 wt% via inductive coupled plasma (ICP) studies. The structure stability of PAF-72 and Ru content are also studied by thermogravimetric analysis (TGA) (Figure 4B). Because of the existence of Ru in Ru/PAF-72, there is about 3.4 wt% oxide residue, which is corresponding to 2.58 wt% Ru.
The porosities of PAF-72 and Ru/PAF-72 were studied by N2 sorption measurement at 77 K (Figure 5A). At low relative pressure, there is a sharp increase for both PAF-72 and Ru/PAF-72, indicating that they are microporous textures. At high relative pressure, a steady linear increase is observed. Calculated from the Brunauer-Emmett-Teller (BET) model, the apparent surface area of PAF-72 is 582 m2 g−1. The pore size distribution is calculated from nonlocal density functional theory (NLDFT). It gives two narrow peaks at 0.7 and 1.4 nm (Figure 5B). In addition, CO2 and CH4 sorptions of PAF-72 at 273 and 298 K were measured (Figures S3–S8). The CO2 uptake at 273 and 298 K is 36.9 and 25.2 cm3·g−1, respectively (Figure S3). The BET surface area of Ru/PAF-72 is 332 m2 g−1 and the pore width converges at 0.5 and 1.1 nm. The decrease of BET surface area and pore width indicate that pore volume is occupied by Ru nanoparticles.
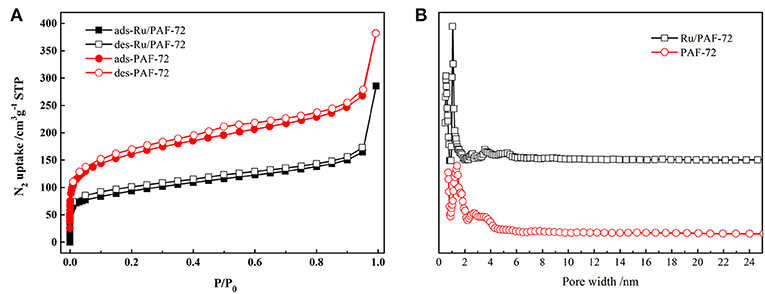
Figure 5. (A) N2 sorption isotherms and (B) pore width distributions of PAF-72 (red) and Ru/PAF-72 (black).
The hydrolysis of AB was tested after Ru was proved to be loaded into PAF-72. Firstly, at 298 K, different amount of AB is used for hydrolysis to confirm the best concentration of AB after recording the hydrolysis and calculating the TOF (Figure 6A). With the increase concentration of AB, the reaction speed enhances, and the maximum TOF is 294 mol (H2) min−1 mol−1 (Ru) when the amount of AB is 60 mg (Table S1). Besides, the temperature can also influence the reaction speed. The total hydrolysis time decreased with the increase of reaction temperature (Figure 6B). Furthermore, Ru/PAF-72 shows very excellent stable and continuous catalytic ability even after 10th cyclic measurement (Figure 7). By comparison, carbon black loaded Ru material shows great performance during the first hydrolytic process, but during the fourth time the total hydrolysis time increases, clearly indicating that carbon black is not a good scaffold to anchor metal (Figure S9).
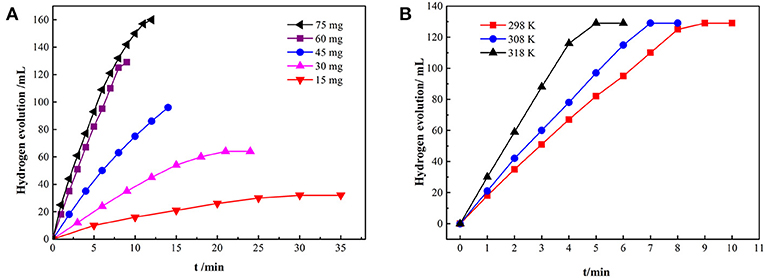
Figure 6. The volume of hydrogen for the Ru/PAF-72 catalytic hydrolysis of AB with different concentrations of AB (A) and at different temperature (B).
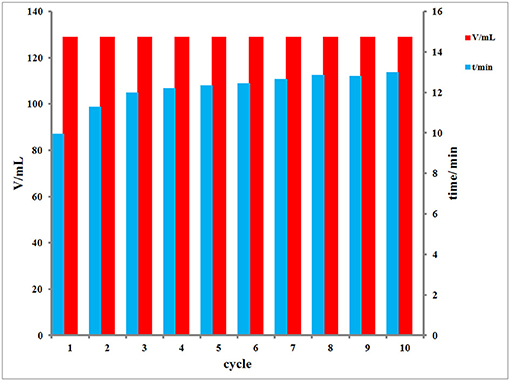
Figure 7. The volume and time for the Ru/PAF-72 catalytic hydrolysis of AB from 1st to 10th cycles at 298 K.
In summary, PAF-72 was synthesized successfully via ionothermal reaction. The properties of PAF-72 were well-investigated and the porosity was explored by N2, CO2, and CH4 sorptions. Furthermore, Ru/PAF-72 exhibits favorable catalytic abilities for hydrolyzing AB. Such a POF material containing a nitrogen skeleton promises great potential as a scaffold for metal catalysts.
Data Availability
All datasets generated for this study are included in the manuscript/Supplementary Files.
Author Contributions
GZ and HR conceived the idea, designed, and supervised the experiment. PC designed and carried out the experiments and performed the data interpretation. PC and HR drafted the manuscript. All authors contributed to the writing of the manuscript.
Funding
This work was supported by National Natural Science Foundation of China (NSFC grant nos. 21531003, 91622106), 111 program, National Basic Research Program of China (973 Program, grant no. 2014CB931804).
Conflict of Interest Statement
The authors declare that the research was conducted in the absence of any commercial or financial relationships that could be construed as a potential conflict of interest.
Supplementary Material
The Supplementary Material for this article can be found online at: https://www.frontiersin.org/articles/10.3389/fmats.2019.00223/full#supplementary-material
References
Ben, T., Ren, H., Ma, S., Cao, D., Lan, J., Jing, X., et al. (2009). Targeted synthesis of a porous aromatic framework with high stability and exceptionally high surface area. Angew. Chem. Int. Ed. Engl. 48, 9457–9460. doi: 10.1002/anie.200904637
Budd, P. M., Ghanem, B. S., Makhseed, S., McKeown, N. B., Msayib, K. J., and Tattershall, C. E. (2004). Polymers of intrinsic microporosity (PIMs): robust, solution-processable, organic nanoporous materials. Chem. Commun. 2, 230–231. doi: 10.1039/b311764b
Chen, M., Xiong, R., Cui, X., Wang, Q., and Liu, X. (2019). SiO2-encompassed Co@N-doped porous carbon assemblies as recyclable catalysts for efficient hydrolysis of ammonia borane. Langmuir. 35, 671–677. doi: 10.1021/acs.langmuir.8b03921
Cote, A. P., Benin, A. I., Ockwig, N. W., O'Keeffe, M., Matzger, A. J., and Yaghi, O. M. (2005). Porous, crystalline, covalent organic frameworks. Science 310, 1166–1170. doi: 10.1126/science.1120411
Das, S., Heasman, P., Ben, T., and Qiu, S. (2017). Porous organic materials: strategic design and structure-function correlation. Chem. Rev. 117, 1515–1563. doi: 10.1021/acs.chemrev.6b00439
El-Kaderi, H. M., Hunt, J. R., Mendoza-Cortes, J. L., Cote, A. P., Taylor, R. E., O'Keeffe, M., et al. (2007). Designed synthesis of 3D covalent organic frameworks. Science 316, 268–272. doi: 10.1126/science.1139915
Frueh, S., Kellett, R., Mallery, C., Molter, T., Willis, W. S., King'ondu, C., et al. (2011). Pyrolytic decomposition of ammonia borane to boron nitride. Inorg. Chem. 50, 783–792. doi: 10.1021/ic101020k
Fu, F., Wang, C., Wang, Q., Martinez-Villacorta, A. M., Escobar, A., Chong, H., et al. (2018). Highly selective and sharp volcano-type synergistic Ni2Pt@ZIF-8-catalyzed hydrogen evolution from ammonia borane hydrolysis. J. Am. Chem. Soc. 140, 10034–10042. doi: 10.1021/jacs.8b06511
Graetz, J. (2009). New approaches to hydrogen storage. Chem. Soc. Rev. 38, 73–82. doi: 10.1039/b718842k
Hu, M. G., Geanangel, R. A., and Wendlandt, W. W. (1978). The thermal decomposition of ammonia borane. Thermochim. Acta 23, 249–255. doi: 10.1016/0040-6031(78)85066-7
Jiang, J. X., Su, F., Trewin, A., Wood, C. D., Campbell, N. L., Niu, H., et al. (2007). Conjugated microporous poly(aryleneethynylene) networks. Angew. Chem. Int. Ed. Engl. 46, 8574–8578. doi: 10.1002/anie.200701595
Kuhn, P., Antonietti, M., and Thomas, A. (2008). Porous, covalent triazine-based frameworks prepared by ionothermal synthesis. Angew. Chem. Int. Ed. Engl. 47, 3450–3453. doi: 10.1002/anie.200705710
Li, A., Sun, H.-X., Tan, D.-Z., Fan, W.-J., Wen, S.-H., Qing, X.-J., et al. (2011). Superhydrophobic conjugated microporous polymers for separation and adsorption. Energy Environ. Sci. 4, 2062–2065. doi: 10.1039/c1ee01092a
Li, M., Ren, H., Sun, F., Tian, Y., Zhu, Y., Li, J., et al. (2018). Construction of porous aromatic frameworks with exceptional porosity via building unit engineering. Adv. Mater. 30:e1804169. doi: 10.1002/adma.201804169
Liang, H., Chen, G., Desinan, S., Rosei, R., Rosei, F., and Ma, D. (2012). In situ facile synthesis of ruthenium nanocluster catalyst supported on carbon black for hydrogen generation from the hydrolysis of ammonia-borane. Int. J. Hydrogen Energy 37, 17921–17927. doi: 10.1016/j.ijhydene.2012.09.026
Peng, B., and Chen, J. (2008). Ammonia borane as an efficient and lightweight hydrogen storage medium. Energy Environ. Sci. 1, 479–483. doi: 10.1039/b809243p
Peng, Y., Ben, T., Xu, J., Xue, M., Jing, X., Deng, F., et al. (2011). A covalently-linked microporous organic-inorganic hybrid framework containing polyhedral oligomeric silsesquioxane moieties. Dalton Trans. 40, 2720–2724. doi: 10.1039/c0dt01268h
Qin, R., Liu, P., Fu, G., and Zheng, N. (2018). Strategies for stabilizing atomically dispersed metal catalysts. Small Methods 2:1700286. doi: 10.1002/smtd.201700286
Rossin, A., and Peruzzini, M. (2016). Ammonia-borane and amine-borane dehydrogenation mediated by complex metal hydrides. Chem. Rev. 116, 8848–8872. doi: 10.1021/acs.chemrev.6b00043
Schlapbach, L., and Zuttel, A. (2001). Hydrogen-storage materials for mobile applications. Nature 414, 353–358. doi: 10.1038/35104634
Tan, L., and Tan, B. (2017). Hypercrosslinked porous polymer materials: design, synthesis, and applications. Chem. Soc. Rev. 46, 3322–3356. doi: 10.1039/c6cs00851h
Zhang, H., Liu, G., Shi, L., and Ye, J. (2018). Single-atom catalysts: emerging multifunctional materials in heterogeneous catalysis. Adv. Energy Mater. 8:1701343. doi: 10.1002/aenm.201701343
Zhu, G., and Ren, H. (2015). Porous Organic Frameworks: Design, Synthesis and Their Advanced Applications. ChangChun: Springer. doi: 10.1007/978-3-662-45456-5
Keywords: porous organic framework, ionothermal reaction, ammonia borane, Ruthenium, catalytic hydrolysis
Citation: Cui P, Ren H and Zhu G (2019) Ruthenium Inlaying Porous Aromatic Framework for Hydrogen Generation From Ammonia Borane. Front. Mater. 6:223. doi: 10.3389/fmats.2019.00223
Received: 30 July 2019; Accepted: 29 August 2019;
Published: 13 September 2019.
Edited by:
Zhonghua Xiang, Beijing University of Chemical Technology, ChinaCopyright © 2019 Cui, Ren and Zhu. This is an open-access article distributed under the terms of the Creative Commons Attribution License (CC BY). The use, distribution or reproduction in other forums is permitted, provided the original author(s) and the copyright owner(s) are credited and that the original publication in this journal is cited, in accordance with accepted academic practice. No use, distribution or reproduction is permitted which does not comply with these terms.
*Correspondence: Hao Ren, cmVuaGFvQGpsdS5lZHUuY24=