- 1School of Environmental Science and Engineering, Shanghai Jiao Tong University, Shanghai, China
- 2Shanghai Institute of Pollution Control and Ecological Security, Shanghai, China
Metal oxides exhibit excellent barrier properties against moisture and oxygen, which are regarded as the powerful candidate for boosting the stability of perovskite nanocrystals (NCs). Here, we develop a simple method to coat perovskite NCs with PbO by peroxides post-treatment. Firstly, the didodecyl dimethyl ammonium sulfide (DDAS) was used to modify the CsPbBr3 NCs (DDAS-CsPbBr3), and then sequentially treated with benzoyl peroxide (BPO). By this way, the self-metal source (lead) can be easily oxidized, leading to the quick growth of lead oxide (PbO) on the surface of individual CsPbBr3 NCs. Under illumination with a 450 nm LED light (175 mW/cm2), the PbO coated CsPbBr3 NCs maintained at ~90% of the initial intensity for 20 h of operations; in contrast, the emission from the CsPbBr3 NCs completely quenched.
Introduction
All inorganic cesium lead halide (CsPbX3, X = Cl, Br, and I) perovskite nanocrystals (NCs) are promising materials for optoelectronic applications (Zhang et al., 2018; Juang et al., 2019; Papagiorgis et al., 2019), especially for light emitting because of their optically tunable band gap and excellent photoluminescence quantum yields (PLQY). In spite of the excellent merits of the perovskite NCs in various fields, the applications of perovskite NCs are impeded by several issues with regard to their stability. Perovskite NCs are highly sensitive to polar solvents due to their inherent ionic nature. Surface ligands and even structural integrity may be lost in the polar solvents, such as ethyl alcohol and water (Kim et al., 2015; Li X. et al., 2017). In addition, environmental stresses, such as light, oxygen, and moisture, could make harmful effects on their performance, because these external conditions accelerate the rates of oxidation and hydration, which lead to the photoluminescence quenching (Zhang et al., 2018; Zhang et al., 2018; Wei et al., 2019). Therefore, poor stability under operational conditions remains a great challenge for practical applications (Yang et al., 2016). To date, a variety of strategies have been developed toward stable perovskite NCs. For example, strong binding ligands, such as didodecyl dimethyl ammonium bromide (Li Z. et al., 2017) and 2,2′-Iminodi benzoic acid (Pan J. et al., 2017) were introduced to enhance the stability of perovskite NCs. Incorporation into stable materials, such as silica (Liu et al., 2018), silica/alumina monolith (Li Z. et al., 2017), polymer (Wu et al., 2019), and graphene oxide matrix (Pan A. et al., 2017) are another major direction to stabilize perovskite NCs. Unfortunately, the existing insulating barrier layer and relatively large size of the resultant integrated composites make them not well-suitable for many optoelectronic and bio-imaging applications. As well-known, coating oxide is a powerful approach to improve the stability of conventional quantum dots (QDs). However, the direct growing of oxides on CsPbBr3 NCs is quite challenging because of the lattice mismatch of two kinds of materials.
Metal, for example, steel or aluminum, which are immersed into the strong oxidizers, such as nitric acid and sulfate acid, a very dense oxidation layer can be formed quickly, which could prevent rusting of steel products and improve its service life by blocking the intrusion of water vapor, oxygen and other corrosive substances (Uhlig and O'Connor, 1955; Jovanovic and Hackerman, 1998; Robin et al., 2008). In the field of QDs, oxidant-hydrogen peroxides have been using to improve the luminescent properties of quantum dots. Lee et al. treated CdS QDs with hydrogen peroxide and formed CdSO4 on the surface of QDs, which increased the radiation recombination rate of CdS QDs and improved their luminous efficiency (Lee et al., 2012). Klyuev et al. prepared CdS QDs in gelatin, followed by hydrogen peroxide treatment, and found that the QDs size became smaller and CdO appeared on the surface (Klyuev et al., 2017). Liu et al. used hydrogen peroxide as an etchant to prepare QDs of different particle sizes (Liu et al., 2008). Under mild oxidative conditions, the yellow-emitting CdSe QDs (546 nm) can be converted into blue-emitting CdSe QDs (466 nm), which provides a simple and fast method for preparing small-diameter CdSe QDs. However, there is no report that the related oxidant improves the stability of CsPbBr3 NCs by oxidation.
Inspired by the fact that oxidants promote the formation of oxide protective films on metal surfaces, we treated CsPbBr3 NCs with oxidizing agents, which is expected to improve their stability. However, hydrogen peroxide is insoluble in the CsPbBr3 NCs toluene solution. Therefore, it is necessary to select a suitable oxidizing agent, which has good solubility in toluene. Benzoyl peroxide (BPO) is a strong oxidant that contains weak oxygen-oxygen linkages that release reactive oxygen when heated. In the polymerization reaction, polymerization is induced as an initiator (Raeesi et al., 2017). At the same time, low concentrations of BPO solutions are commonly used in clinical medicine, and their oxidative properties can effectively treat acne and powder (Thiboutot et al., 2008). In addition, BPO has good solubility in toluene, and it can form a uniform system with CsPbBr3 NCs toluene solution, which is beneficial to the oxidation reaction. Therefore, BPO is chosen here as an oxidant to treat CsPbBr3 NCs, and PbO is formed on the surface, which resists the corrosion of water vapor and oxygen, and endows the CsPbBr3 NCs with excellent phototability.
Materials and Methods
Chemicals
Cesium carbonate(Cs2CO3, 98%), lead bromide(PbBr2, 98%), sodium sulfide nonahydrate (Na2S·9H2O, 98%), oleylamine(OAm, 90%), didodecyl dimethylammonium bromide (DDAB, Aladdin, 98%), and 1-octadecene (ODE, Aladdin, 90%) were purchased from Aladdin Reagent Co. Oleic acid(OA, 90%) was purchased from Sigma-Aldrich Co. Benzoyl peroxide(BPO, C14H10O4, 98%) was purchased from Beijing Bailingwei Technology Co., Ltd. Methyl acetate(98%) was purchased from Sinopharm Chemical Reagent Co., Ltd.
Preparation of Cs-oleate
Ten millimolar Cs2CO3 20 mL OA, and 20 mL ODE were loaded into a 100 mL three-necked flask, then was degassed at 120°C for 30 min. Subsequently, the temperature was raised to 150°C under the N2 protection and a transparent solution was formed, and then the heat source was removed to allow the temperature of the system to fall to room temperature. The concentration of Cs-oleate (CsOA) was 0.5 mmol/mL, which was stored as a stock solution.
Preparation of CsPbBr3 Nanocrystals
Two millimolar of PbBr2, 20 mL of ODE, 5 mL of OA, and 5 mL of OAm were placed in a 100 mL three-necked flask, and the reaction system was dried under vacuum at 120°C for 30 min, during which N2 was introduced several times. Then, the temperature was raised to 180°C under the N2 protection state, and a preheated (70°C) 1 mL of CsOA solution was quickly injected immediately after the formation of the transparent solution. After 10 s, the flask was placed in an ice water bath to be cooled. The CsPbBr3 NCs were extracted and washed with toluene and methyl acetate, and then the CsPbBr3 NCs were dissolved in 20 mL of toluene and stored as a stock solution. The concentration is about 15 mg/mL.
Preparation of DDAS Solution
0.75 mmol of DDAB and 15 mL of toluene were mixed and then treated with sonication for 20 min, marking as solution A. 0.75 mmol of Na2S·9H2O was dissolved into 15 mL of distilled via the similar method, marking as solution B. Then, A solution was mixed with the B solution and sonicated for 0.5 h. The mixed solution was centrifuged at 10,000 rpm for 1 min, and the upper layer of toluene solution was taken to obtain a DDAS solution at a concentration of 0.05 mmol/mL, which was stored as stock solution.
Preparation of BPO Toluene Solution
One millimolar of BPO was add into 10 mL of the toluene, and then was sonicated for 1 h. The BPO toluene solution (0.1 mmol/mL) was obtained.
BPO Peroxidation of DDAS Treatment of CsPbBr3 NCs
Firstly, the CsPbBr3 NCs were treated with BPO toluene solution at 70°C for 1 h (DDAS-CsPbBr3 NCs). Subsequently, the BPO was injected by adjusting the speed of the syringe pump, the mount depended on the concentration of CsPbBr3 NCs, and the reaction was proceeded at 70°C. After 2 h, the solution was cooled down to room temperature, and then washed three times with methyl acetate and toluene, and stored in toluene (BPO-DDAS-CsPbBr3).
Photostability Test
The absorbance of NCs was adjusted to 1.8 at 450 nm, and the same volume of solution was added to the closed cuvette. We performed photostability tests of NCs on a blue LED module with 0.175 W/cm2 light intensity (peak at 450 nm, Philips Fortimo).The fluorescence spectrum of the sample was collected at a certain time interval, the PL ratio was obtained by calculating the ratio of the area of the corresponding fluorescence spectrum with the initial fluorescence spectrum.
Results and Discussion
BPO Direct Peroxidation of CsPbBr3 NCs
Initially, BPO toluene solution was directly added to a CsPbBr3 NCs solution using a syringe pump for oxidation. As shown in Figure 1, as the reaction time prolonged, the color of the CsPbBr3 NCs solution gradually changed from green to pale yellow, accompanying by fluorescence quenching (Figure S1B). In addition, it was observed that there existed the aggregation of CsPbBr3 NCs after BPO treatment in the Figure 2, which indicated that ligands deriving from the CsPbBr3 NCs were peeled. Thus, some CsPbBr3 NCs grew into the larger nanocrystals, which led to the variation of the morphology. Meanwhile, a new phase corresponding to the structure (JCPDS 25-0211) was observed after BPO treatment (Figure S2), this phenomenon manifested that CsPbBr3 NCs underwent the phase change.
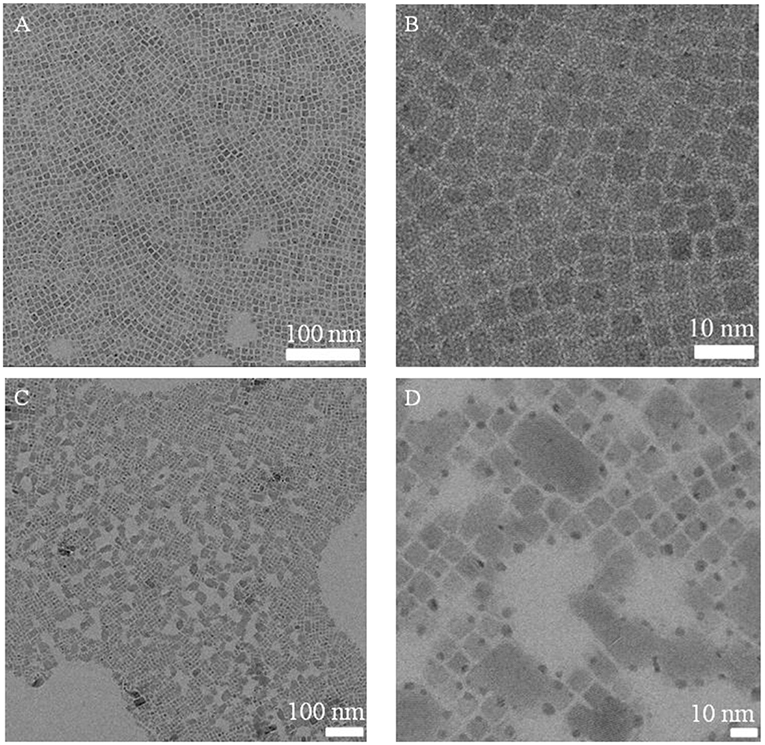
Figure 2. TEM images of CsPbBr3 NCs (A,B) and BPO oxidized CsPbBr3 NCs (C,D, 120 min) with different magnifications.
BPO Peroxidation DDAS Treated CsPbBr3 NCs
When BPO was directly applied to oxidize CsPbBr3 NCs, although photostability is slightly improved (Figure S1A), the fluorescence intensity is reduced. Therefore, it is urgent to increase the tolerance of CsPbBr3 NCs for the BPO. As we known, the unpurified CsPbBr3 NCs contain a large number of ligands in their solution and have good protection for NCs. Thus, the unpurified CsPbBr3 NCs were oxidized by BPO and their photostability was tested. As shown in Figure S3, the unpurified CsPbBr3 NCs were oxidized, and their photostability was improved, but the extent of improvement was very limited. Therefore, it is necessary to further improve the tolerance of CsPbBr3 NCs for the BPO.
Related literature reported that DDAS could improve the stability of CsPbBr3 NCs (Li Z. et al., 2017). Therefore, DDAS is used to treat CsPbBr3 NCs (DDAS-CsPbBr3) firstly, which improves their resistance to BPO oxidation treatment and maintains excellent photoluminescence of CsPbBr3 NCs. As shown in Figure 5, the fluorescence intensity of CsPbBr3 NCs was enhanced by DDAS treatment for 1 h (Figure S4), which did not affect the fluorescence efficiency of CsPbBr3 NCs. Subsequently, the BPO toluene solution was injected, and CsPbBr3 NCs were oxidized at 70°C for 2 h. The solution was cooled down to the room temperature, then purified the BPO oxidized CsPbBr3 NCs (BPO-DDAS-CsPbBr3). As shown in Figure S5, the fluorescence intensity and peak position of the CsPbBr3 NCs almost underwent no change during the oxidation process, and the excellent luminescence properties are maintained, and the solution appears bright green (Figure 3).
Characterization of BPO-DDAS-CsPbBr3 NCs
As shown in Figure 4A, the BPO-DDAS-CsPbBr3 NCs showed good photostability. Among them, 0.050 mmol of DDAS treated CsPbBr3 NCs showed the best photostability after BPO oxidation. After the illumination of 20 h, the fluorescence intensity is 90% of the initial value, while the CsPbBr3 NCs were almost completely quenched. At the same time, DDAS can also improve the photostability of CsPbBr3 NCs. In addition, the effect of BPO concentration on the photostability of NCs was investigated. In Figure 4B, although 0.125 mmol of BPO treated DDAS-CsPbBr3 NCs improved their stability, they were much lower than 0.250 mmol of BPO oxidized DDAS-CsPbBr3 NCs, perhaps due to insufficient oxidation of 0.125 mmol of BPO. In addition, when the BPO was 0.375 mmol, the DDAS-CsPbBr3 NCs were excessively oxidized; destroying the structure of the NCs, and the solution showed a pale yellow color and became turbid (Figure 5).
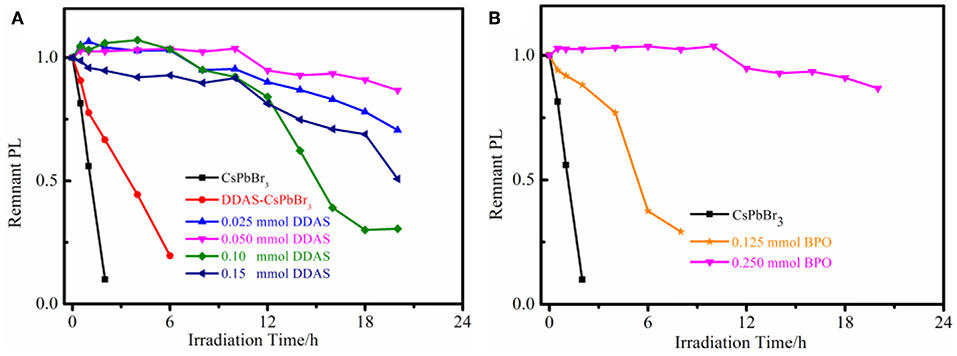
Figure 4. (A) Photostability of the CsPbBr3 NCs, DDAS-CsPbBr3 NCs (0.050 mmol DDAS treatment), and 0.250 mmol BPO oxidized DDAS-CsPbBr3 NCs with different DDAS treatment. (B) Photostability of the different BPO oxidized DDAS-CsPbBr3 NCs (0.050 mmol DDAS treatment).
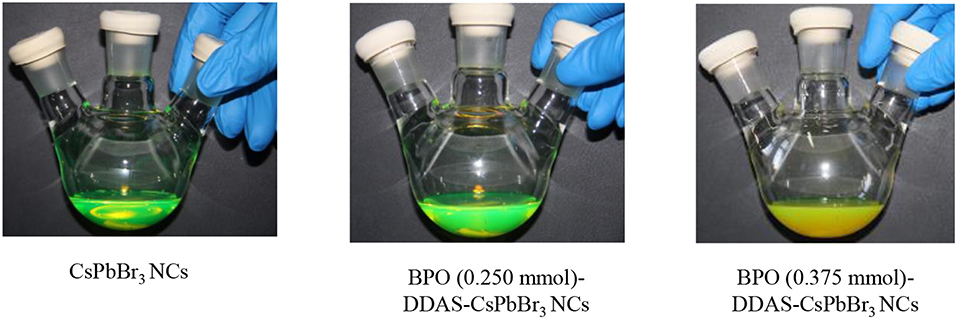
Figure 5. Photographs of CsPbBr3 NCs, BPO (0.250 mmol)-DDAS-CsPbBr3 NCs, and BPO (0.375 mmol)-DDAS-CsPbBr3 NCs.
At the same time, the morphological changes of CsPbBr3NCs were characterized by TEM. As shown in Figure 6, the morphology and size of CsPbBr3 NCs did not change significantly after DDAS treatment and BPO oxidation. The average particle size was 8.2, 8.4, and 8.5 nm, respectively. This indicates that the DDAS treatment improves the resistance of CsPbBr3 NCs for the BPO treatment, and they are not etched by proper BPO during oxidation. The photostability is improved while maintaining the initial morphology and excellent luminescence performance. In addition, we measured the lattice spacing of the CsPbBr3 NCs, DDAS-CsPbBr3 NCs, and BPO-DDAS-CsPbBr3 NCs. As shown in Figure S6, lattice spacing of all the samples is about 0.30 nm corresponding to the (200) crystal faces of the CsPbBr3 NCs. These results confirm that the BPO treated CsPbBr3 NCs do not have significant tendency of lattice change. At the same time, the PLQY of CsPbBr3 NCs is 70%, the DDAS treatment is increased to 81%, and the PLQY of BPO-DDAS-CsPbBr3NCs is 78%. It is proved once again that DDAS does improve the tolerance of CsPbBr3 NCs for the BPO treatment.
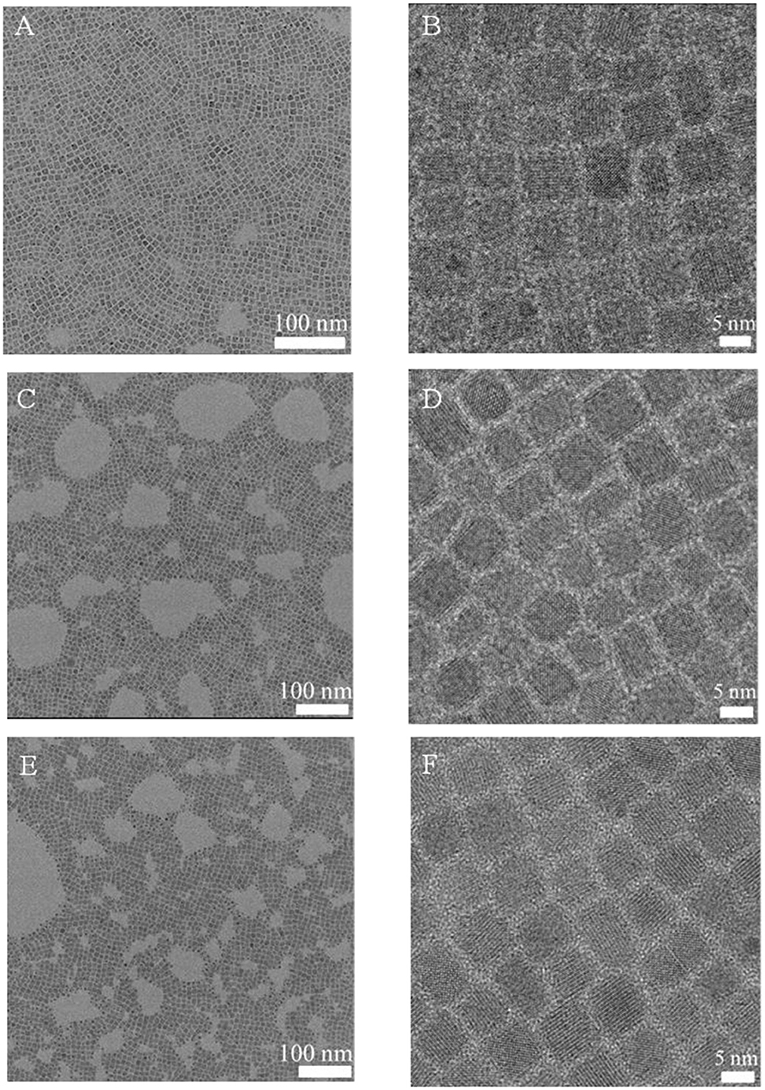
Figure 6. TEM images of CsPbBr3 NCs (A,B), DDAS-CsPbBr3 NCs (C,D), and BPO-DDAS-CsPbBr3 NCs (E,F) with different magnification.
In addition, as shown in Figure S7, the fluorescence peak position of the BPO-DDAS-CsPbBr3 NCs is redshifted by 2 nm compared to the CsPbBr3 NCs. However, as shown in Figure 6, the size of CsPbBr3 NCs are about 8.2 nm, which really exceed in the Bohr radius (Butkus et al., 2017). Therefore, the redshift of the peak position was not caused by the change in the particle size. This may be due to the fact that the electrons or holes of the CsPbBr3 NCs escape into the formed oxide, resulting in the above phenomenon and the same phenomenon is observed in the CdO-coated CdSe/CdZnS quantum dots (Cho et al., 2017).
The CsPbBr3 NCs solution, DDAS-CsPbBr3 NCs solution, and BPO-DDAS-CsPbBr3 NCs were added dropwise to the quartz glass plate, and then dried to form a film for the XRD test. The spectrum are shown in Figure 7A, wherein the (200) crystal plane of the samples were normalized. All the samples corresponded to the cubic perovskite structure (JCPDS 54-0752), with all crystal planes of the perovskite structure appearing, i.e., (100), (110), (200), (210), (211), and (220) crystal planes. Taking the (200) crystal plane as the reference standard, the three samples had similar half-widths, indicating that the particle size of the CsPbBr3 NCs did not change significantly.
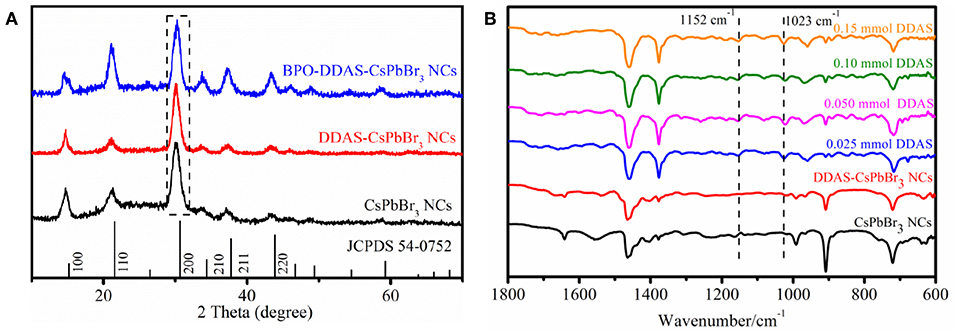
Figure 7. (A) XRD patterns of CsPbBr3 NCs, DDAS-CsPbBr3 NCs, and BPO-DDAS-CsPbBr3 NCs. (B) FTIR spectra of the CsPbBr3 NCs, DDAS-CsPbBr3 NCs (0.050 mmol DDAS treatment), and 0.0250 mmol BPO oxidized DDAS-CsPbBr3 NCs with different DDAS treatment.
As shown in Figure 7B, the FITR of all BPO-DDAS-CsPbBr3 NCs showed a new absorption peak at 1,023 cm−1 compared to CsPbBr3 NCs and DDAS-CsPbBr3 NCs, corresponding to the vibration mode of Pb-O (Alagar et al., 2012; Nouri et al., 2017). It is indicated that PbO is formed on the surface of DDAS-CsPbBr3 NCs after BPO oxidation, which resists the corrosion of water vapor and oxygen to quantum dots, giving the CsPbBr3 NCs the excellent photostability. Furthermore, the absorption peak at 1,152 cm−1 corresponds to the asymmetric stretching vibration mode of (Lane, 2007; Periasamy et al., 2009), which indicates that S2− form the DDAS is oxidized to .
In order to further confirm that PbO is formed on the surface of DDAS-CsPbBr3 NCs after BPO oxidation, XPS spectra were also performed to analyze the surface change. As shown in Figure 8A, the Pb 4f spectra of DDAS-CsPbBr3 NCs and BPO-DDAS-CsPbBr3 NCs can be deconvoluted into two peaks, respectively. The binding energy at142.9 eV (142.8 eV) and 138.6 eV (138.0 eV) can be assigned to the Pb atoms of CsPbBr3 NCs (Cai et al., 2018). In addition, the peaks at 143.1 eV and 138.2 eV could be attributed to the presence of Pb-S (Kong et al., 2017), which derived from the DDAS-CsPbBr3 NCs. The peaks located at 142.9 and 138.1 eV were associated with the Pb-O (Rondon and Sherwood, 1998). But, it is difficult to distinguish from the spectra of Pb-O and the Pb-S, because it seems that their spectra are close to each other. Meanwhile, the peak at 532.1 eV is assigned to the C = O group arising from the residual OA of the BPO-DDAS-CsPbBr3 NCs in Figure 8B (Zherebetskyy et al., 2014). Furthermore, the O 1s peak of BPO-DDAS-CsPbBr3 NCs was deconvoluted into two peaks with the binding energies of 532.1 and 530.8 eV. The peak at 530.8 eV is possibly attributed to oxygen in the Pb-O of PbO (Huang et al., 2017), which confirmed that the PbO was formed on the surface of DDAS-CsPbBr3 NCs after BPO oxidation. Therefore, on the basis of aforementioned analyses, it can be concluded that the improved stability of CsPbBr3 NCs is attributed to the protective layer of PbO.
Conclusions
In summary, DDAS is firstly used to treat CsPbBr3 NCs, following by BPO oxidation treatment to generate PbO on the surface of CsPbBr3 NCs. The compact PbO acts as robust protective layer to prevent the NCs from the attack of oxygen and moisture. Therefore, CsPbBr3 NCs exhibit stronger photostability under illumination. We believe that this strategy represents a universal approach to improving the stability of NCs in optoelectronic applications.
Data Availability Statement
All datasets generated for this study are included in the article/Supplementary Material.
Author Contributions
ML and ZL contributed to the CsPbBr3 NCs synthesis. LK and WZ contributed to the imaging of NCs. LL designed the experiments.
Funding
This work was supported by the Guangdong Province's 2018-2019 Key R&D Program (2019B010924001), National Natural Science Foundation of China (NSFC 21773155), and Shanghai Sailing Program (19YF1422200).
Conflict of Interest
The authors declare that the research was conducted in the absence of any commercial or financial relationships that could be construed as a potential conflict of interest.
Supplementary Material
The Supplementary Material for this article can be found online at: https://www.frontiersin.org/articles/10.3389/fmats.2019.00306/full#supplementary-material
References
Alagar, M., Theivasanthi, T., and Raja, A. K. (2012). Chemical synthesis of nano-sized particles of lead oxide and their characterization studies. J. Appl. Sci. 12, 398–401. doi: 10.3923/jas.2012.398.401
Butkus, J., Vashishtha, P., Chen, K., Gallaher, J. K., Prasad, S. K. K., Metin, D. Z., et al. (2017). The evolution of quantum confinement in CsPbBr3 perovskite nanocrystals. Chem. Mater. 29, 3644–3652. doi: 10.1021/acs.chemmater.7b00478
Cai, Y., Wang, L., Zhou, T., Zheng, P., Li, Y., and Xie, R. J. (2018). Improved stability of CsPbBr3 perovskite quantum dots achieved by suppressing interligand proton transfer and applying a polystyrene coating. Nanoscale 45, 21441–21450. doi: 10.1039/C8NR06607H
Cho, J., Jung, Y. K., Lee, J. K., and Jung, H. S. (2017). Surface coating of gradient alloy quantum dots with oxide layer in white-light-emitting diodes for display backlights. Langmuir 33, 13040–13050. doi: 10.1021/acs.langmuir.7b03335
Huang, S., Li, Z., Wang, B., Zhu, N., Zhang, C., and Kong, L. (2017). Morphology evolution and degradation of CsPbBr3 nanocrystals under blue light-emitting diode illumination. ACS Appl. Mater. Interfaces 8, 7249–7258. doi: 10.1021/acsami.6b14423
Jovanovic, V. M., and Hackerman, N. (1998). Electrochemical passivation of iron in , , and Solutions. J. Phys. Chem. B 102, 9855–9860. doi: 10.1021/jp982602z
Juang, S. S., Lin, P. Y., Lin, Y. C., Chen, Y. S., Shen, P. S., Guo, Y. L., et al. (2019). Energy harvesting under dim-light condition with dye-sensitized and perovskite solar cells. Front. Chem. 7:209. doi: 10.3389/fchem.2019.00209
Kim, Y., Yassitepe, E., Voznyy, O., Comin, R., Walters, G., Gong, X., et al. (2015). Efficient luminescence from perovskite quantum dot solids. ACS Appl. Mater. Interfaces 7, 25007–25013. doi: 10.1021/acsami.5b09084
Klyuev, V. G., Volykhin, D. V., and Ivanova, A. A. (2017). Influence of hydrogen peroxide on the stability and optical properties of CdS quantum dots in gelatin. J. Lumin. 183, 519–524. doi: 10.1016/j.jlumin.2016.11.069
Kong, L., Li, Z., Huang, X., Huang, S., Sun, H., Liu, M., et al. (2017). Efficient removal of Pb (II) from water using magnetic Fe3S4/reduced graphene oxide composites. J. Mater. Chem. A 36, 19333–19342. doi: 10.1039/C7TA05389D
Lane, M. D. (2007). Mid-infrared emission spectroscopy of sulfate and sulfate-bearing minerals. Am. Mineral. 92, 1–18. doi: 10.2138/am.2007.2170
Lee, W., Kim, H., Jung, D. R., Kim, J., Nahm, C., Lee, J., et al. (2012). An effective oxidation approach for luminescence enhancement in CdS quantum dots by H2O2. Nanoscale Res. Lett. 7:672. doi: 10.1186/1556-276X-7-672
Li, X., Cao, F., Yu, D., Chen, J., Sun, Z., Shen, Y., et al. (2017). All inorganic halide perovskites nanosystem: synthesis, structural features, optical properties and optoelectronic applications. Small 13:1603996. doi: 10.1002/smll.201603996
Li, Z., Kong, L., Huang, S., and Li, L. (2017). Highly luminescent and ultrastable CsPbBr3 perovskite quantum dots incorporated into a silica/alumina monolith. Angew. Chem. Int. Ed. 56, 8134–8138. doi: 10.1002/anie.201703264
Liu, L., Peng, Q., and Li, Y. (2008). An eEffective oxidation route to blue emission CdSe quantum dots. Inorg. Chem. 47, 3182–3187. doi: 10.1021/ic702203c
Liu, Z., Zhang, Y., Fan, Y., Chen, Z., Tang, Z., Zhao, J., et al. (2018). Toward highly luminescent and stabilized silica-coated perovskite quantum dots through simply mixing and stirring under room temperature in air. ACS Appl. Mater. Interfaces 10, 13053–13061. doi: 10.1021/acsami.7b18964
Nouri, M., Alizadeh, P., and Tavoosi, M. (2017). The relationship between structural and optical properties of GeO2-PbO glasses. J. Adv. Mater. Process. 5, 3–10.
Pan, A., Jurow, M. J., Qiu, F., Yang, J., Ren, B., Urban, J. J., et al. (2017). Nanorod suprastructures from a ternary graphene oxide-polymer-csPbX3 perovskite nanocrystal composite that display high environmental stability. Nano Lett. 17, 6759–6765. doi: 10.1021/acs.nanolett.7b02959
Pan, J., Shang, Y., Yin, J., De Bastiani, M., Peng, W., Dursun, I., et al. (2017). Bidentate ligand-passivated CsPbI3 perovskite nanocrystals for stable near-unity photoluminescence quantum yield and efficient red light-emitting diodes. J. Am. Chem. Soc. 140, 562–565. doi: 10.1021/jacs.7b10647
Papagiorgis, P. G., Manoli, A., Alexiou, A., Karacosta, P., Karagiorgis, X., Papaparaskeva, G., et al. (2019). Robust hydrophobic and hydrophilic polymer fibers sensitized by inorganic and hybrid lead halide perovskite nanocrystal emitters. Front. Chem. 7:87. doi: 10.3389/fchem.2019.00087
Periasamy, A., Muruganand, S., and Palaniswamy, M. (2009). Vibrational studies of Na2SO4, K2SO4, NaHSO4 and KHSO4 crystals. Rasayan J. Chem. 2, 981–989.
Raeesi, M., Mirabedini, S. M., and Farnood, R. R. (2017). Preparation of microcapsules containing benzoyl peroxide initiator with gelatin-gum arabic/polyurea-formaldehyde shell and evaluating their storage stability. ACS Appl. Mater. Interfaces 9, 20818–20825. doi: 10.1021/acsami.7b03708
Robin, R., Miserque, F., and Spagnol, V. (2008). Correlation between composition of passive layer and corrosion behavior of high Si-containing austenitic stainless steels in nitric acid. J. Nucl. Mater. 375, 65–71. doi: 10.1016/j.jnucmat.2007.10.016
Rondon, S., and Sherwood, P. M. (1998). Core level and valence band spectra of PbO by XPS. Surf. Sci. Spectra 2, 97–103. doi: 10.1116/1.1247866
Thiboutot, D., Zaenglein, A., Weiss, J., Webster, G., Calvarese, B., and Chen, D. (2008). An aqueous gel fixed combination of clindamycin phosphate 1.2% and benzoyl peroxide 2.5% for the once-daily treatment of moderate to severe acne vulgaris: assessment of efficacy and safety in 2813 patients. J. Am. Acad. Dermatol. 59, 792–800. doi: 10.1016/j.jaad.2008.06.040
Uhlig, H. H., and O'Connor, T. L. (1955). Nature of the passive film on iron in concentrated nitric acid. J. Electrochem. Soc. 102, 562–572. doi: 10.1149/1.2429913
Wei, Y., Cheng, Z., and Lin, J. (2019). An overview on enhancing the stability of lead halide perovskite quantum dots and their applications in phosphor-converted LEDs. Chem. Soc. Rev. 48, 310–350. doi: 10.1039/C8CS00740C
Wu, H., Wang, S., Cao, F., Zhou, J., Wu, Q., Wang, H., et al. (2019). Ultrastable inorganic perovskite nanocrystals coated with a thick long-chain polymer for efficient white light-emitting diodes. Chem. Mater. 31, 1936–1940. doi: 10.1021/acs.chemmater.8b04634
Yang, G., Fan, Q., Chen, B., Zhou, Q., and Zhong, H. (2016). Reprecipitation synthesis of luminescent CH3NH3 PbBr3/NaNO3 nanocomposites with enhanced stability. J. Mater. Chem. C, 4, 11387–11391. doi: 10.1039/C6TC04069A
Zhang, F., Xiao, C., Li, Y., Zhang, X., Tang, J., Chang, S., et al. (2018). Gram-scale synthesis of blue-emitting CH3NH3PbBr3 quantum dots through phase transfer strategy. Front. Chem. 6:444. doi: 10.3389/fchem.2018.00444
Zhang, Q., and Yin, Y. (2018). All-inorganic metal halide perovskite nanocrystals: opportunities and challenges. ACS Cent. Sci. 4, 668–679. doi: 10.1021/acscentsci.8b00201
Keywords: nanocrystal, lead halide perovskites, oxidization, metal oxide, photostability
Citation: Liu M, Li Z, Zheng W, Kong L and Li L (2019) Improving the Stability of CsPbBr3 Perovskite Nanocrystals by Peroxides Post-treatment. Front. Mater. 6:306. doi: 10.3389/fmats.2019.00306
Received: 23 August 2019; Accepted: 14 November 2019;
Published: 29 November 2019.
Edited by:
Peter Reiss, Commissariat à l'Energie Atomique et aux Energies Alternatives (CEA), FranceCopyright © 2019 Liu, Li, Zheng, Kong and Li. This is an open-access article distributed under the terms of the Creative Commons Attribution License (CC BY). The use, distribution or reproduction in other forums is permitted, provided the original author(s) and the copyright owner(s) are credited and that the original publication in this journal is cited, in accordance with accepted academic practice. No use, distribution or reproduction is permitted which does not comply with these terms.
*Correspondence: Liang Li, bGlhbmdsaTExN0BzanR1LmVkdS5jbg==