- Swiss Plasma Center, École Polytechnique Fédérale de Lausanne, Lausanne, Switzerland
Surface characterization of plasma-treated seeds has made significant progress over the last decade. Most papers in the literature use scanning electron microscopy (SEM) and contact angle goniometry to investigate surface modifications. However, very few papers address the chemical modifications to the seed coat after plasma treatment. Here, a summary of the methods used to analyze plasma-treated seeds is presented, such as SEM, contact angle goniometry, energy-dispersive X-ray spectroscopy (EDX), X-ray photoelectron spectroscopy (XPS), atomic force microscopy (AFM), and attenuated total reflection Fourier transform infrared spectroscopy (ATR-FTIR). The results obtained on Arabidopsis thaliana Col-0 seeds and the limitations of these techniques are discussed. An experiment was designed in order to compare the relative advantages and limitations of these surface analysis techniques by investigating the separate effects of plasma, heat, and ozone on A. thaliana seeds.
Introduction
Biological applications of low temperature, non-thermal plasmas have recently become widespread from medicine, environmental remediation, and sterilization, to agriculture. The interest in plasma agriculture continuously increases as scientists demonstrate the potential of improving plant growth parameters such as germination, crop yield, and disease resistance when seeds are treated with plasmas (Attri et al., 2020). However, it remains unclear which plasma properties are responsible for these effects on plant growth. To gain a better understanding, surface characterization of plasma-treated seeds has the potential to provide both structural and chemical information.
Surface morphology information can be obtained using scanning electron microscopy (SEM). Seed coat erosion in plasma-treated seeds is the most common effect observed by SEM (Stolárik et al., 2015; Junior et al., 2016; Ambrico et al., 2017; da Silva et al., 2017; Guo et al., 2017; Li et al., 2017; Wang et al., 2017; Gao et al., 2019) but this does not necessarily occur in every instance. For example, Mildaziene et al. (2016) noticed etching only on the seed surface facing the plasma, while other authors did not remark any (Sera et al., 2010; Bormashenko et al., 2012; Kitazaki et al., 2014). Cui et al. (2019) showed SEM images of Arabidopsis seeds after various plasma treatment times and observed detached epidermis with increasing exposure. However, adhesive tape was used to hold the seeds during the plasma treatment which may or may not have affected the results. This inconsistency in seed coat erosion may be partly due to the seed type as described by Molina et al. (2020), who noticed that nasturtium seeds were less prone to etching than wheat seeds under the same experimental conditions (Molina et al., 2018). SEM can also be used to observe surface roughness where Pawłat et al. (2018) interpreted the more creased appearance with an increase in micro-roughness. Alternatively, atomic force microscopy (AFM) can be used to observe and measure poration or surface roughness. Volkov et al. (2019) used AFM to show increase in poration on pumpkin seeds after plasma treatment and Holc et al. (2019) found that plasma removed the wax layer via etching from the garlic tissue.
In addition to SEM, energy-dispersive X-ray spectroscopy (EDX) has been used on plasma-treated seeds, for example, by Cui et al. (2019) on Arabidopsis seeds. They observe an increase of O concentration from approximately 38% to 45% and a decrease of C concentration from 62% to 55% in the untreated and plasma-treated seeds, respectively, indicating the oxidation of carbon. Using cartography images, Gómez-Ramírez et al. (2017), and Molina et al. (2018, 2020), observed a migration of potassium from the interior to the exterior in cross-sections of quinoa, nasturtium and wheat seeds after dry air plasma, and helium plasma treatment, respectively. Molina et al. (2020) explained the initial movement of potassium as being due to the polar-containing groups on the seed coat. Gómez-Ramírez et al. (2017) pointed out that, with the addition of water vapor, the potassium and nitrogen-containing groups decreased, presumably because they were absorbed by the seed and thereby improving seed germination.
Similar trends have been confirmed by X-ray photoelectron spectroscopy (XPS), generally revealing an altered C/O ratio, increased potassium and sometimes increased nitrogen concentration after plasma treatment. Depending on the seed type and plasma setup, the changes can range from a 5% to 30% increase in oxygen. Specifically, there is an increase in C-O, O-C = C bonds at the cost of C-C or C-H bonds. Gómez-Ramírez et al. (2017), Molina et al. (2018, 2020), and Holc et al. (2019) all demonstrated this with quinoa, nasturtium, wheat grains and garlic, respectively. Molina participated in two separate studies where nitrogen increased in quinoa, whereas this did not occur in wheat grains. However, different plasmas were used, where quinoa was subjected to an air plasma and wheat grains to helium plasma with impurities. In both circumstances, the seed surfaces were oxidized. Similarly, the oxidation of hydrocarbons was also detected on plasma-treated thermally modified wood by Talviste et al. (2019) using a diffuse coplanar surface barrier discharge (DCSBD) air plasma. Therefore, it seems that oxidative etching plays a role in plasma-seed interactions whether the plasma is removing the top layer to expose layers that are more oxygen-rich, or by oxidizing the surface directly.
X-ray photoelectron spectroscopy can investigate the depth of the sample within the first few nanometers whereas attenuated total reflection Fourier transform infrared spectroscopy (ATR-FTIR) has a higher penetration depth in the μm range and therefore can analyze several layers. Kitazaki et al. (2012) did not observe any differences due to plasma treatment on radish seeds, however, other authors have similar findings to the XPS results. Zahoranová et al. (2018) found an increase in C=O and decrease in hydrocarbons after treating maize seeds and suggested that lipids were removed from the seed surface. Švubová et al. (2020) did not see such a pronounced effect but saw a slight decrease in the C-H bond stretching of lipids, suggesting lipid oxidation. Despite omitting wavenumbers below 1,500 cm–1 which were difficult to assign to specific functional groups, Wang et al. (2017) suggested the seed is able to absorb water due to improved hydrophilicity via oxidative etching and the partial degradation of cellulose. This enhanced water absorption is supported by Yamauchi et al. (2012) who witnessed the degradation of cellulose on seed surfaces. Holc et al. (2019) and Molina et al. (2020) suggested two scenarios: plasma may be removing the hydrophobic layers and exposing the cellulose and other hydrocarbons, or remodeling the hydrophobic wax through heat and/or chemical reactions.
The measurement of contact angle is a straightforward method to determine if the seed surface is more hydrophilic and therefore changing the water uptake rate. Many authors over the last decade observed increased hydrophilicity following Bormashenko et al. (2012) who improved the wettability of lentil, beans and wheat seeds with an air plasma treatment. For example, Velichko et al. (2019) compared an argon plasma jet in open air with an atmospheric air dielectric barrier discharge (DBD) in terms of germination and contact angle of plasma-treated wheat grains. Both methods decreased the contact angle although it was more strongly reduced by the air DBD. Likewise, Junior et al. (2016) observed increased hydrophilicity in Mimosa seeds with a helium plasma jet in air and Hoppanová et al. (2020) observed increased hydrophilicity in cereal seeds with a DBD in open air. da Silva et al. (2017) demonstrated that air plasmas increase the hydrophilicity due to the absorption of radicals such as OH and N2+. Conversely, it is possible to increase the hydrophobic properties by changing the gas source to carbon tetrafluoride (CF4) or octadecafluorodecalin (ODFD) (Volin et al., 2000), although this is achieved by deposition rather than by modifying intrinsic properties.
Contact angle measurements are important for understanding whether the effects are due to increased water uptake. Arguably, an XPS analysis provides the chemical composition and surface modifications such as oxidation that would increase the sample wettability so it may not be necessary to do contact angle measurements. However, XPS may not be available to many scientists so it is understandable if many use the sessile drop method for straightforward qualitative and sometimes quantitative results. Clearly, this is best done on a flat surface and may not be possible with all seed types due to a range of shapes and sizes as in our case, i.e., if 1 μl of water is the minimum volume, a seed like Arabidopsis is too small, or if the seed surface is very rough, it is not possible to obtain quantitative measurements.
The relative merits of these various surface analysis techniques are now investigated by using them as diagnostics in a model experiment. The aim in the following experimental investigation is to gain insight into which physical phenomena are relevant in the plasma treatment that would have an effect on the seed coat surface using a model plant organism, Arabidopsis thaliana Col-0. Changes to the seed coat and other downstream processes may be due to ozone (chemical modifications to the seed surface), heat (mechanical modifications of wax) or plasma (mechanical and chemical modifications to the seed surface). Therefore, ozone and heat are applied as separate treatments and compared to the plasma treatment in an effort to identify the individual effect of each component on the seed. Based on these results, we show that it seems to be a synergy of the plasma effects, and not its individual components, which is responsible for any modifications to the seeds. Finally, through this experience, certain surface analysis techniques can be preferentially recommended as they provide more fruitful results than other techniques.
Experiment Description
Commercially available surface dielectric barrier discharges (SDBD) by VOSOCO with alumina dielectric and printed electrodes in a striped or honeycomb geometry were used for the plasma treatment (Figures 1A,B, respectively).
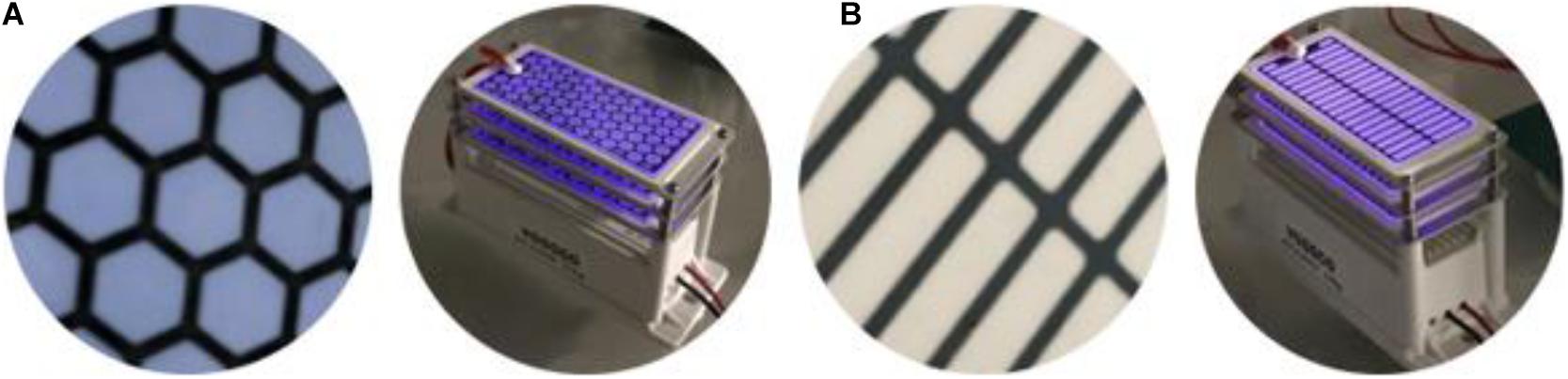
Figure 1. A detailed view and an overall view of a surface dielectric barrier discharge (DBD) plasma source with (A) honeycomb electrodes; and (B) stripe electrodes.
The SDBD was powered by a 12 kHz sine wave with 8.8 kV peak-to-peak voltage, for a power of ∼75 W over a stack of three alumina plates, each of 105 mm × 50 mm area. Treatments were performed in an open-air environment with no gas flow (ambient temperature, pressure, humidity ∼40%). A plasma treatment time of 3 min was chosen because the first visible plasma effects on the seed surface, seed coat erosion were observed for this case (Figure 2). To identify the effect of the individual components, heat and ozone treatments were carried out separately in the absence of a plasma. The heat treatment parameters were chosen to give a similar temperature rise of the dielectric/electrodes with plasma on. The temperature reached 80°C after 3 min, but to ensure sufficient heat transfer from the hot plate to the seed, the treatment time was extended to 10 min. The ozone treatment parameters were based on measurements with plasma on using an ozone analyzer UV-100 (EcoSensors). The ozone generator, developed by Sterilux, was an enclosed compartment with three, 172 nm V-UV lamps (Thill and Spaltenstein, 2020). Since it could only reach 200 ppm of ozone, the treatment time was also extended to 10 min. Arguably, because the plasma treatment was carried out in open air, the overall ozone concentration was not so important provided that a similar ozone concentration was maintained at the seed surface. All treatments were performed directly on the seeds initially spread uniformly over the SDBD.
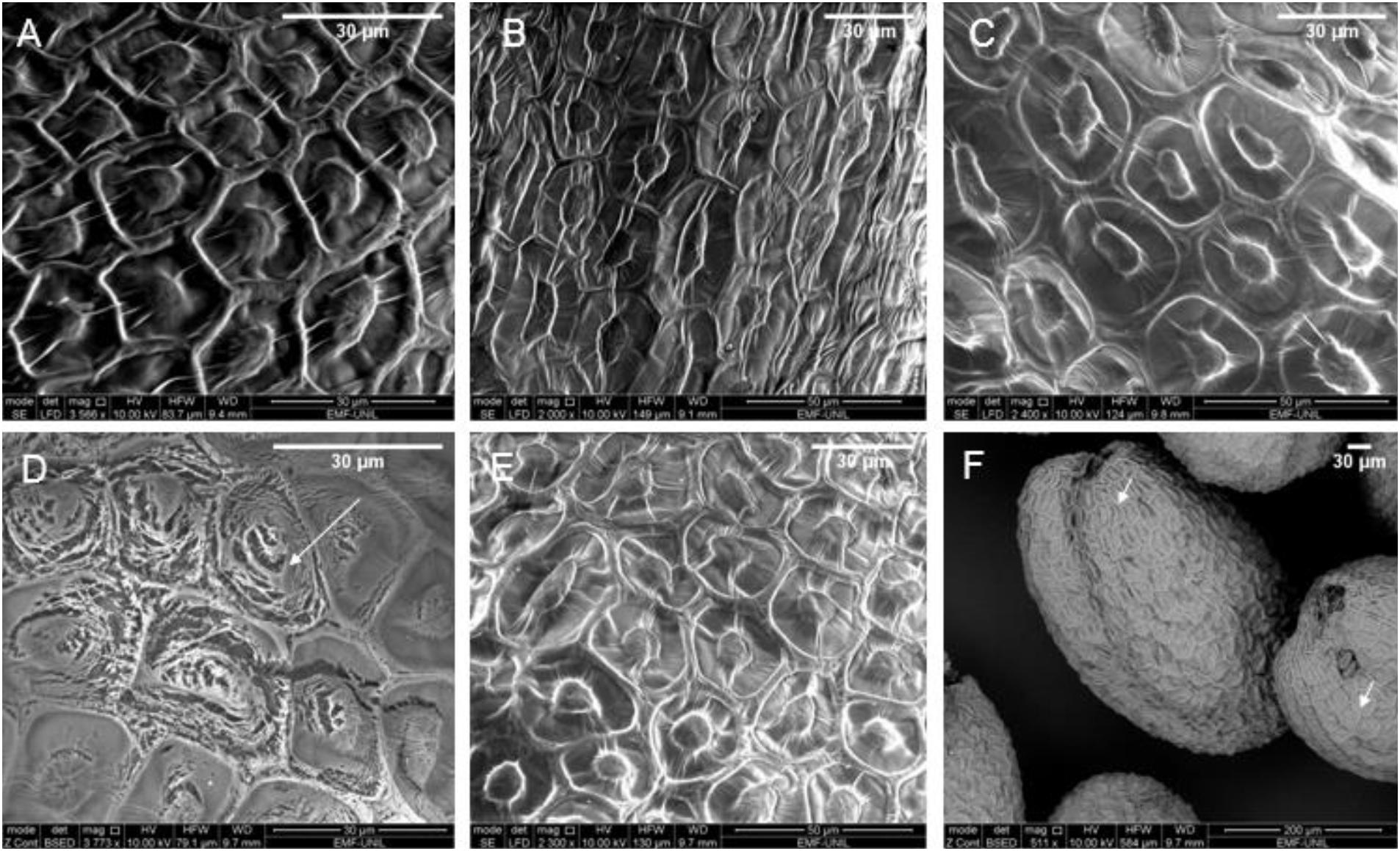
Figure 2. Scanning electron microscopy (SEM) images of Arabidopsis seeds which are (A) untreated, (B) heat-treated for 10 min at 80°C, (C) plasma-treated for 3 min using honeycomb electrode dielectric barrier discharge (DBD) configuration, (D) plasma-treated for 3 min with stripe electrode DBD configuration; the white arrow points to the eroded area (E) ozone-treated for 10 min at 200 ppm, and (F) ozone-treated for 10 min at 200 ppm of multiple seeds, white arrows point to biological diversity in seed coat patterns. Images at a lower magnification are shown in the Supplementary Figure 1.
Results and Comparison of Surface Analysis Techniques
Seed erosion was observed after plasma treatment, but not with heat nor ozone alone, as shown in Figure 2 by the white arrows. Erosion was particularly observed with the stripe electrode configuration and to a smaller degree with the honeycomb electrodes. These results reinforced the previously reported observations of seed coat erosion in some, but not all, cases. Molina et al. (2018, 2020) showed differences in seed coat erosion based on different seed types, but in our study we used the same seed type with different plasmas. This highlights that seed type is not the only parameter to consider whether there will be erosion and that the type of plasma treatment also needs to be considered as another parameter. As pointed out by Mildaziene et al. (2016), this may depend on the seed position and whether it is in direct contact with the surface or close to the plasma. It was observed that the plasma in the hexagonal geometry of the honeycomb electrode (see Figure 1A) positioned the seeds in the center of the hexagons, perhaps due to electrostatic forces or air flow, during the plasma treatment. This distancing from the plasma can possibly explain the lack of erosion whereas, for the stripe electrodes, the seeds were more evenly distributed, thereby interacting with the plasma, resulting in erosion.
Furthermore, caution should be taken when interpreting information from SEM images because plant genetics (natural variation) and dehydration of the seeds can influence the seed coat pattern. As shown in Figure 2F, the white arrows point out variations in the seed coat in the same seed sample despite undergoing the same ozone treatment simultaneously. Therefore, observed changes on different seeds could be attributed to biological variation rather than the applied treatment. This emphasizes the necessity of checking the seed sample prior to use and if possible, observing the same single seed before and after treatment.
The samples need to be handled carefully to avoid overexposing the sample to the SEM electron beam because the electron beam can cause damage to the sample and may be confused as an effect of plasma treatment. Furthermore, since SEM analysis is performed in vacuum, moisture is removed (unless operating under low vacuum conditions where not all moisture is removed). This then may not exactly reproduce the same experimental set up and, as a result, there may be cracking in the seed coat. However, this can be verified to some extent by comparison with the control seeds if all seeds have similar moisture content. Alternatively, AFM can produce topography images without surface damage and the morphology can be measured in x, y, and z, but it was not technically feasible to image A. thaliana seeds due to their deep contours and seed roundness. AFM remains as an option for flat seed types such as pumpkin seeds, whereas SEM can be used on all seed types in a qualitative manner (Volkov et al., 2019).
Overall, SEM can provide detailed surface topology information within seconds or minutes but is otherwise limited in explaining the effect of plasma on seeds. Authors such as Mildaziene et al. (2017) observed positive growth effects using vacuum and electromagnetic fields without visibly changing the seed coat structure using SEM. This suggests that SEM is not sufficiently specific since it remains unclear whether erosion is necessary to alter plant growth. Thus, it is arguably more interesting to focus on the seed coat chemistry, which may be more explicit concerning downstream processes that change the growth parameters (Los et al., 2019). Analyzing the chemical changes on the seed coat after plasma treatment can be done with ATR-FTIR, EDX, or XPS.
Attenuated total reflection Fourier transform infrared spectroscopy analysis was performed but the data is only shown in the Supplementary Figure 2 since it did not reveal any relevant information between the untreated and treated seeds. With our sample, it was difficult to ensure that sufficient pressure was applied to ensure proper contact and a strong signal without crushing the seed. When the appropriate conditions were identified, there were no detectable differences between the untreated seeds, and the heat-, ozone-, or plasma-treated seeds in the ratio between the C-H stretch around 3,100–2,850 cm–1 and C = O stretch around 1,800–1,650 cm–1. Cui et al. (2019) showed an increase in the O-H stretch with increasing plasma treatment time. However, in our case, it was clear that incrementally increasing the pressure was responsible for the O-H stretch around 3,400–3,200 cm–1, suggesting that compressing the seed may release its water content. Furthermore, Šerá et al. (2021) also had a reference spectra with more water content and in one among three species of pine seeds, there was no obvious difference. Therefore, it may be important before the analysis to dehydrate the seeds to have consistent results. In our case, the expected oxidation of the hydrocarbons was perhaps not observed because ATR-FTIR penetrates into the bulk material, whereas XPS measures only the first nanometers of the sample, where plasma is expected to make surface changes. Therefore, for newcomers to the plasma agriculture field, it is recommended to work with specialists to interpret the overlapping bands. When done properly, it is interesting to see how the plasma etches through the tissue layers (Molina et al., 2020). Although ATR-FTIR does not damage the surface, finding the correct amount of pressure may be simpler for hard seed types like maize and pine but more difficult for delicate, small seeds like Arabidopsis. Although Cui et al. (2019) also performed ATR-FTIR on Arabidopsis, the authors selectively showed only the O-H stretch change due to plasma treatment, but in our instance, the change was due to increased pressure. Instead, under our conditions, similar information by the ATR-FTIR can be obtained with XPS on delicate seeds if simply checking for oxidation or nitration.
With EDX, it is possible to retrieve the distribution of the elements. However, considering that plasma treatment affects the surface and not the bulk material, this technique may not necessarily provide any information about the seed interior unless a cross-section is taken as done by Gómez-Ramírez et al. (2017) and Molina et al. (2018, 2020). Furthermore, difficulties may be encountered in performing absolute measurements, partly due to the curved surface of the seed, and in identifying the source of any changes in element concentration i.e., carbon may be taken up from the adhesive paper on the sample holder. Therefore, EDX data are not shown here but are given in the Supplementary Figure 3. The EDX maps did not reveal any obvious changes other than perhaps an increase in potassium in heat-, ozone- and plasma-treated seeds compared to the untreated seeds, but this information was not enough to confidently draw a conclusion. Nevertheless, an important aspect of this analysis revealed that nickel was unexpectedly detected on the seeds after plasma treatments when initially using different DBDs, which were gold-plated copper electrodes on Kapton or epoxy dielectric. This indicates that one should be attentive to nanoparticle contamination of the biological sample from the electrodes (Kasote et al., 2019; An et al., 2020). To avoid this contamination, the DBDs were changed to the plasma devices described in this paper, and XPS was used as an alternative to obtain absolute values and compare them to the EDX measurements (Supplementary Figure 3).
The elements analyzed were carbon, nitrogen, oxygen, potassium, magnesium, calcium, sulfur, and silicon. However, only the first four are shown since the others remained mostly unchanged.
In line with Gómez-Ramírez et al. (2017), Molina et al. (2018, 2020), and Holc et al. (2019), the oxidation of carbon, shown by the decrease in C-H bonds and increase in O=C-O, C=O, C-O, and increased potassium peaks were the obvious changes in seeds after plasma treatment. Here, the oxygen content increased between 15% and 30% depending on the plasma device in agreement with the 5% to 30% increase in oxygen observed by other authors. Additionally, there is a qualitative increase in C-O, O-C=C bonds at the expense of C-C or C-H bonds in the C1s spectra (Figure 3). These changes, however, were not observed with the ozone or heat treatments.
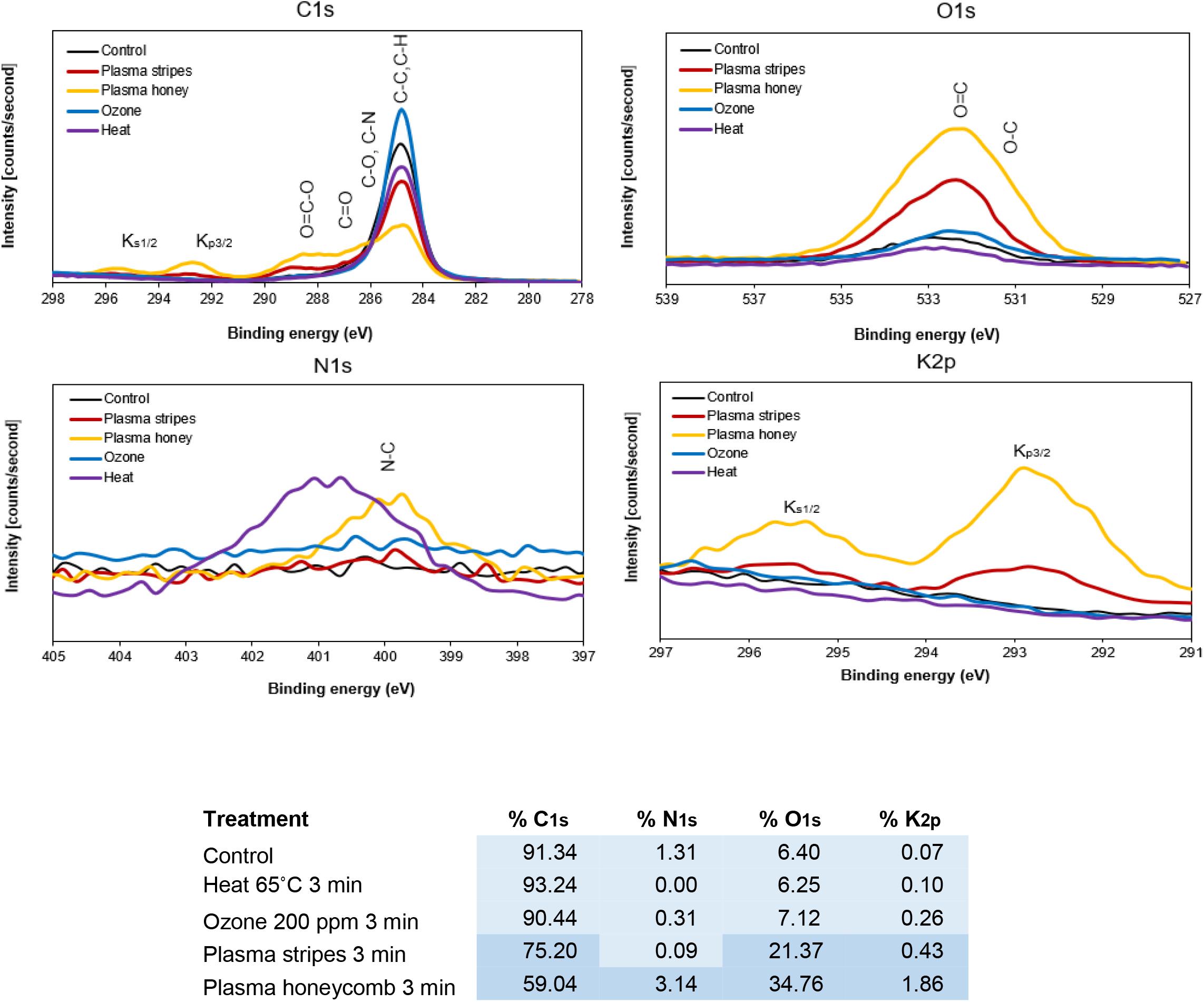
Figure 3. X-ray photoelectron spectroscopy (XPS) spectra with atomic concentration table of carbon, nitrogen, oxygen, and potassium in untreated, heat-treated, ozone-treated, and plasma-treated (stripe and honeycomb electrodes) Arabidopsis seeds.
There are therefore two clear conclusion: First, that the XPS surface analysis technique gives the most significant and unambiguous results; and second, that plasma in both stripes and honeycomb configurations is responsible for oxidizing the seed surface. Concerning heat or ozone, these individual treatments may not be sufficiently reactive during the 3-min treatment time to cause changes to the seed surface, however, they have the potential to do so with longer treatment time. Likewise, higher potassium concentrations were mainly in plasma-treated seeds; possibly indicating that the intensity of plasma treatment leads to damage. Interestingly, there was little to no erosion found with the honeycomb configuration, suggesting that either correlation is not causation or that there is a time window to capture and measure increasing levels of potassium which was missed for the stripes configuration. For example, Švubová et al. (2020) did not observe surface damage despite detecting chemical changes.
Considering that all treatments were done in open air, the concentration of ozone was difficult to determine and therefore may not be precise. However, it is plausible that the surface would become more reactive due to plasma’s well-known ability for etching and sputtering, explaining the lack of obvious changes with ozone treatment alone. The etching mechanism may indeed, as others have postulated, remove lipid layers to facilitate oxidation (Zahoranová et al., 2018; Švubová et al., 2020). Although ozone has a role in seed treatments and the potential to oxidize surfaces, it may not be sufficiently long enough to induce changes.
This suggests that the combination of heat and ozone within the plasma or, indeed, other plasma properties altogether such as electric fields or ions, are responsible for yielding these surface changes on the seed coat, rather than the individual components such as heat or ozone acting alone.
Conclusion
In this study, we analyzed and compared untreated A. thaliana Col-0 seeds to seeds treated with heat, ozone, or plasma, using SEM, EDX, ATR-FTIR, and XPS. SEM revealed erosion, and XPS revealed surface oxidation only in plasma-treated seeds. This suggests that the synergy of different plasma components or another component of plasma is responsible for change instead of the individual heat or ozone treatments, and this may be due to the etching ability of plasma. Overall, in these experiments, it can be concluded that XPS was the most useful surface analysis technique on A. thaliana.
Data Availability Statement
The original contributions presented in the study are included in the article/Supplementary Material, further inquiries can be directed to the corresponding author/s.
Author Contributions
AW was responsible for study conception, analysis, and writing the manuscript. AH and IF read and approved the manuscript. All authors contributed to the article and approved the submitted version.
Conflict of Interest
The authors declare that the research was conducted in the absence of any commercial or financial relationships that could be construed as a potential conflict of interest.
Funding
This work was supported by an ad hoc grant of the Swiss Federal Budgetary Framework 2017–2020 for the ETH Domain.
Acknowledgments
The authors would like to thank Pierre Mettraux for the XPS sample characterization and Dr. Claes Olsson for his support and advice. The authors also would like to thank Antonio Mucciolo at the UNIL Electron Microscopy Facility for the SEM images.
Supplementary Material
The Supplementary Material for this article can be found online at: https://www.frontiersin.org/articles/10.3389/fmats.2021.642099/full#supplementary-material
Supplementary Figure 1 | SEM images of Arabidopsis seeds which are (A) untreated, (B) heat-treated for 10 min at 80°C, (C) plasma-treated for 3 min using honeycomb electrode DBD configuration, (D) plasma-treated for 3 min with stripe electrode DBD configuration; the white arrows point to eroded areas (E) ozone-treated for 10 min at 200 ppm, showing the biological diversity in seed coat patterns (F) ozone-treated for 10 min at 200 ppm of multiple seeds.
Supplementary Figure 2 | ATR-FTIR spectra of Arabidopsis seeds (A) spectra of untreated seeds under increasing pressure demonstrating changes are only due to increase in pressure (B) spectra of untreated, heat-treated, ozone-treated, plasma-treated seeds demonstrating no relevant changes.
Supplementary Figure 3 | EDX maps of Arabidopsis seeds which are (A) untreated, (B) heat-treated for 10 min at 80°C, (C) ozone-treated for 10 min at 200 ppm, (D) plasma-treated for 3 min using honeycomb electrode DBD configuration, (E) plasma-treated for 3 min with stripe electrode DBD configuration.
References
Ambrico, P. F., Šimek, M., Morano, M., Angelini, R. M. D. M., Minafra, A., and Trotti (2017). Reduction of microbial contamination and improvement of germination of sweet basil (Ocimum basilicum L.) seeds via surface dielectric barrier discharge. J. Phys. D: Appl. Phys. 50:305401.
An, J., Hu, P., Li, F., Wu, H., Shen, Y., White, J. C., et al. (2020). Emerging investigator series: molecular mechanisms of plant salinity stress tolerance improvement by seed priming with cerium oxide nanoparticles. Environ. Sci.: Nano 7, 2214–2228. doi: 10.1039/D0EN00387E
Attri, P., Ishikawa, K., Okumura, T., Koga, K., and Shiratani, M. (2020). Plasma agriculture from laboratory to farm: A review. Processes 8:1002.
Bormashenko, E., Grynyov, R., Bormashenko, Y., and Drori, E. (2012). Cold radiofrequency plasma treatment modifies wettability and germination speed of plant seeds. Sci. Rep. 2, 1–8. doi: 10.1038/srep00741
Cui, D., Yin, Y., Wang, J., Wang, Z., Ding, H., Ma, R., et al. (2019). Research on the Physio-Biochemical Mechanism of Non-Thermal Plasma-Regulated Seed Germination and Early Seedling Development in Arabidopsis. Front. Plant Sci. 10:1322. doi: 10.3389/fpls.2019.01322
da Silva, A. R. M., Farias, M. L., da Silva, D. L. S., Vitoriano, J. O., de Sousa, R. C., and Alves-Junior, C. (2017). Using atmospheric plasma to increase wettability, imbibition and germination of physically dormant seeds of Mimosa Caesalpiniafolia. Coll. Surf. B: Biointerf. 157, 280–285.
Gao, X., Zhang, A., Héroux, P., Sand, W., Sun, Z., Zhan, J., et al. (2019). Effect of Dielectric Barrier Discharge Cold Plasma on Pea Seed Growth. J. Agricult. Food Chem. 67, 10813–10822. doi: 10.1021/acs.jafc.9b03099
Gómez-Ramírez, A., López-Santos, C., and Cantos, M. (2017). Surface chemistry and germination improvement of Quinoa seeds subjected to plasma activation. Sci. Rep. 7:5924. doi: 10.1038/s41598-017-06164-5
Guo, Q., Wang, Y., and Zhang, H. (2017). Alleviation of adverse effects of drought stress on wheat seed germination using atmospheric dielectric barrier discharge plasma treatment. Sci. Rep. 7:16680. doi: 10.1038/s41598-017-16944-8
Holc, M., Primc, G., Iskra, J., Titan, P., Kovač, J., Mozetič, M., et al. (2019). Effect of Oxygen plasma on Sprout and root growth, surface morphology and yield of garlic. Plants 8:462. doi: 10.3390/plants8110462
Hoppanová, L., Medvecká, V., Dylíková, J., Hudecová, D., Kaliňáková, B., Kryštofová, S., et al. (2020). Low-temperature plasma applications in chemical fungicide treatment reduction. Acta Chim. Slov. 13, 26–33. doi: 10.2478/acs-2020-0005
Junior, C. A., de Oliveira Vitoriano, J., Da Silva, D. L. S., de Lima Farias, M., and de Lima Dantas, N. B. (2016). Water uptake mechanism and germination of Erythrina velutina seeds treated with atmospheric plasma. Sci. Rep. 6, 1–7. doi: 10.1038/srep33722
Kasote, D. M., Lee, J. H., Jayaprakasha, G. K., and Patil, B. S. (2019). Seed priming with iron oxide nanoparticles modulate antioxidant potential and defense-linked hormones in watermelon seedlings. ACS Sustain. Chem. Eng. 7, 5142–5151. doi: 10.1021/acssuschemeng.8b06013
Kitazaki, S., Koga, K., Shiratani, M., and Hayashi, N. (2012). Growth enhancement of radish sprouts induced by low pressure O2 radio frequency discharge plasma irradiation. Japan. J. Appl. Phys. 51:01AE01.
Kitazaki, S., Sarinont, T., Koga, K., Hayashi, N., and Shiratani, M. (2014). Plasma induced long-term growth enhancement of Raphanus sativus L. using combinatorial atmospheric air dielectric barrier discharge plasmas. Curr. Appl. Phys. 14, S149–S153.
Li, Y., Wang, T., Meng, Y., Qu, G., Sun, Q., Liang, D., et al. (2017). Air Atmospheric Dielectric Barrier Discharge Plasma Induced Germination and Growth Enhancement of Wheat Seed. Plasm. Chem. Plasm. Proc. 117237, 1621–1634. doi: 10.1007/s11090-017-9835-5
Los, A., Ziuzina, D., Boehm, D., Cullen, P. J., and Bourke, P. (2019). Investigation of mechanisms involved in germination enhancement of wheat (Triticum aestivum) by cold plasma: Effects on seed surface chemistry and characteristics. Plasm. Proc. Poly. 16:1800148. doi: 10.1002/ppap.201800148
Mildaziene, V., Pauzaite, G., Malakauskiene, A., Zukiene, R., Nauciene, Z., Filatova, I., et al. (2016). Response of perennial woody plants to seed treatment by electromagnetic field and low-temperature plasma. Bioelectromagnetics 37, 536–548. doi: 10.1002/bem.22003
Mildaziene, V., Pauzaite, G., Naucienė, Z., Malakauskiene, A., Rasa Zukiene, Januskaitiene, I., et al. (2017). Pre-sowing seed treatment with cold plasma and electromagnetic field increases secondary metabolite content in purple coneflower (Echinacea purpurea) leaves; Pre-sowing seed treatment with cold plasma and electromagnetic field increases secondary metabolite content in purple coneflower (Echinacea purpurea) leaves. Hoboken, NY: Wiley. doi: 10.1002/ppap.201700059
Molina, R., Lalueza, A., López-Santos, C., Ghobeira, R., Cools, P., Morent, R., et al. (2020). Physicochemical surface analysis and germination at different irrigation conditions of DBD plasma-treated wheat seeds. Plasm. Proc. Poly. 2020:e2000086. doi: 10.1002/ppap.202000086
Molina, R., López-Santos, C., and Gómez-Ramírez, A. (2018). Influence of irrigation conditions in the germination of plasma treated Nasturtium seeds. Sci. Rep. 8:16442. doi: 10.1038/s41598-018-34801-0
Pawłat, J., Starek, A., Sujak, A., Terebun, P., Kwiatkowski, M., Budzeń, M., et al. (2018). Effects of atmospheric pressure plasma jet operating with DBD on Lavatera thuringiaca L. seeds’ germination. PLoS One 13:0194349. doi: 10.1371/journal.pone.0194349
Šerá, B., Šerý, M., Zahoranová, A., and Tomekova, J. (2021). Germination Improvement of Three Pine Species (Pinus) After Diffuse Coplanar Surface Barrier Discharge Plasma Treatment. Plas. Chem. Plasm. Proc. 41, 211–226. Doi: 10.1007/s11090-020-10128-5
Sera, B., Spatenka, P., Sery, M., Vrchotova, N., and Hruskova, I. (2010). Influence of plasma treatment on wheat and oat germination and early growth. IEEE Transac. Plasm. Sci. 38:29632968. doi: 10.1109/TPS.2010.2060728
Stolárik, T., Henselová, M., Martinka, M., Novák, O., Zahoranová, A., and Ċernák, M. (2015). Effect of Low-Temperature Plasma on the Structure of Seeds, Growth and Metabolism of Endogenous Phytohormones in Pea (Pisum sativum L.). Plasm. Chem. Plasm. Proc. 35, 659–676. doi: 10.1007/s11090-015-9627-8
Švubová, R., Kyzek, S., Medvecká, V., Slováková, L’, Gálová, E., and Zahoranová, A. (2020). Novel insight at the effect of cold atmospheric pressure plasma on the activity of enzymes essential for the germination of pea (Pisum sativum L. cv. Prophet) seeds. Plasm. Chem. Plasm. Proc. 40, 1221–1240.
Talviste, R., Galmiz, O., Stupavská, M., Tučeková, Z., Kaarna, K., and Kováčik, D. (2019). Effect of DCSBD plasma treatment on surface properties of thermally modified wood. Surf. Interf. 16, 8–14. Doi: 10.1016/j.surfin.2019.04.005
Thill, S. A., and Spaltenstein, M. (2020). Toward Efficient Low-Temperature Ozone Gas Sterilization of Medical Devices. Ozone: Sci. Eng. 42, 386–398. doi: 10.1080/01919512.2019.1704217
Velichko, I., Gordeev, I., Shelemin, A., Nikitin, D., Brinar, J., Pleskunov, P., et al. (2019). Plasma Jet and Dielectric Barrier Discharge Treatment of Wheat Seeds. Plasm. Chem. Plasm. Proc. 39, 913–928. doi: 10.1007/s11090-019-09991-8
Volin, J. C., Denes, F. S., Young, R. A., and Park, S. M. (2000). Modification of seed germination performance through cold plasma chemistry technology. Crop Sci. 40, 1706–1718. doi: 10.2135/cropsci2000.4061706x
Volkov, A. G., Hairston, J. S., Patel, D., Gott, R. P., and Xu, K. G. (2019). Cold plasma poration and corrugation of pumpkin seed coats. Bioelectrochemistry 128, 175–185.
Wang, X. Q., Zhou, R. W., Groot, G., De, Bazaka, K., Murphy, A. B., et al. (2017). Spectral characteristics of cotton seeds treated by a dielectric barrier discharge plasma. Sci. Rep. 7, 1–9. doi: 10.1038/s41598-017-04963-4
Yamauchi, Y., Kuzuya, M., Sasai, Y., and Kondo, S. I. (2012). Surface treatment of natural polymer by plasma technique-Promotion of seed germination. J. Photopoly. Sci. Technol. 25, 535–538.
Zahoranová, A., Hoppanová, L., Šimončicová, J., Tucekova, Z., Medvecka, V., Hudecova, D., et al. (2018). Effect of Cold Atmospheric Pressure Plasma on Maize Seeds: Enhancement of Seedlings Growth and Surface Microorganisms Inactivation. Plasm. Chem. Plasm. Proc. 38, 969–988. Doi: 10.1007/s11090-018-9913-3
Keywords: plasma, seeds, surface modification, SEM, EDX, XPS, ATR-FTIR
Citation: Waskow A, Howling A and Furno I (2021) Advantages and Limitations of Surface Analysis Techniques on Plasma-Treated Arabidopsis thaliana Seeds. Front. Mater. 8:642099. doi: 10.3389/fmats.2021.642099
Received: 15 December 2020; Accepted: 31 March 2021;
Published: 21 April 2021.
Edited by:
Paolo Francesco Ambrico, Istituto per la Scienza e Tecnologia dei Plasmi – Consiglio Nazionale delle Ricerche (CNR), ItalyReviewed by:
Hock Jin Quah, University of Science Malaysia, MalaysiaGiorgio S. Senesi, Institute for Science and Technology of Plasmas, National Research Council, Italy
Copyright © 2021 Waskow, Howling and Furno. This is an open-access article distributed under the terms of the Creative Commons Attribution License (CC BY). The use, distribution or reproduction in other forums is permitted, provided the original author(s) and the copyright owner(s) are credited and that the original publication in this journal is cited, in accordance with accepted academic practice. No use, distribution or reproduction is permitted which does not comply with these terms.
*Correspondence: Alexandra Waskow, YWxleGFuZHJhLndhc2tvd0BlcGZsLmNo