- 1Higher Institution Centre of Excellence (HICoE), UM Power Energy Dedicated Advanced Centre (UMPEDAC), Level 4, Wisma R&D, University of Malaya, Kuala Lumpur, Malaysia
- 2Department of Electrical Engineering, University of Lahore, Islamabad Campus, Islamabad, Pakistan
- 3Department of Unmanned Vehicle Engineering, Sejong University, Seoul, South Korea
- 4Nust Business School, National University of Science and Technology, Islamabad, Pakistan
- 5Department of Materials Science and Engineering, Pak-Austria Fachhochschule Institute of Applied Sciences and Technology, Haripur, Pakistan
- 6Department of Mechanical Engineering, Institute of Space Technology, Islamabad, Pakistan
Solar light absorber surface is probably one of the most important components in solar still that dictates the distillate yield. In this work, a systematic study is conducted to investigate the effect of particle size and concentration of titanium oxide (TiO2) in black paint in increasing the solar still absorber surface temperature. The various available particle sizes, i.e., 20, 150, and 400 nm, are mixed in black paint with varying concentrations and are applied on the absorber plate. XRD is used for phase identification of as-received powders. UV-Vis spectroscopy is used to examine light absorption properties. Finally, extensive indoor testing (using an improvised solar simulator) and outdoor testing are conducted to optimize the concentration. An increase in surface temperature is observed with the introduction of TiO2 nanoparticles in black paint. Furthermore, the increase in particle size leads to an increase in temperature. The highest surface temperatures of 104.86°C, 105.42°C, and 106.32°C are recorded for specimens with particles sizes 20 nm (at 15 wt% concentration), 150 nm (at 10 wt% concentration), and 400 nm (at 7 wt% concentration), respectively. Furthermore, the highest temperature of 69.69°C is recorded for TiO2-400 nm specimens under outdoor conditions, which is 15.97% higher than that of the bare aluminum plate. The increase in surface temperature may be due to high UV absorption. Moreover, an increase in particle size leads to high light-scattering ability, further improving the light-harvesting ability.
Introduction
Day by day, the requirement for drinking water keeps increasing as the demand for freshwater goes up (Chandrashekara and Yadav, 2017). Fresh drinking water is a foundation of life and an essential building block for sustained human development (Chandrashekara and Yadav, 2017; Sharshir et al., 2017). The demand is higher due to the claim for water from the various surface water sources used for various developments (Naveenkumar et al., 2020). The lack of portable water is elevating due to important factors such as global warming, exhaustion of groundwater level, and contamination due to poor water management (Biswas and Tortajada, 2019; Naveenkumar et al., 2020). Although around 70% of the earth is covered with water, 67.4% is saline water and only 2.6% water is drinkable. From this 2.6%, only 0.9% of fresh water is easily accessible (Srithar and Rajaseenivasan, 2018). The direct utilization of water from existing water reserves should be eliminated because it consists of many debris, biological impurities, and a higher quantity of total dissolved solids (Kabeel et al., 2019b; Naveenkumar et al., 2020).
In this context, seawater desalination has been regarded as the most feasible approach due to its vast availability (Ali et al., 2018). Large-scale desalination includes distillation methods such as thermal desalination, i.e., multistage flash desalination, vapor compression, and desalination, UV filtration, and membrane technology (Huang et al., 2020). Small-scale desalination techniques include electrodialysis, reverse osmosis, and desalination using photovoltaic cells (Heidary et al., 2018; Huang et al., 2020). Although the methods guarantee high desalination yields, the associated installation, maintenance, and operational costs are prohibitively high (Khanafer and Vafai, 2018).
In this regard, water distillation operation using solar still is a promising technique due to its operational simplicity, less running cost, and environmental-friendly operation (Abujazar et al., 2017). Solar still is essentially a sealed box-like structure with a transparent glass top cover and structural decoration, accordingly (Kabeel et al., 2020). The water inside the box is heated by direct sunlight. The water vapors in contact with the backside of the top glass cover become liquid and are collected for drinking purposes (Alotaibi et al., 2017). Although the technique is very simple and lucrative, it suffers low productivity per square active area. The low productivity is directly related to the low temperature inside the solar still. Various design modifications and the use of light absorption materials have been tried with varying results (Kabeel et al., 2019a).
One of the main components contributing a lot to solar still production efficiency is the base plate material, which acts as a solar radiation absorber and provides the required energy to water (Hong et al., 2019). Recently, the coating of sunlight absorption nanomaterials on the absorbed plate (generally aluminum or copper) has been suggested with promising claims (Deshmukh and Thombre, 2017). In a recent study, TiO2 nanoparticles have been mixed with black paint and coated onto the absorber plate (Sathyamurthy et al., 2019). A 1.5°C increase in water temperature has been reported for TiO2-modified black paint compared to bare black paint. This increase in temperature leads to a 6.1% increase in solar distillate yield. Carbon nanotubes and graphene have also been employed to achieve maximum temperature (Abdelal and Taamneh, 2017; Baticados et al., 2020).
In another study, TiO2 along with phase change materials (PCMs) has been used. The claimed increase in yield was 88.46%, which is attributed to energy absorption and storage (Rufuss et al., 2018). The use of TiO2-based nano-fluids with PCMs has also been suggested for improved yield and ease of operations (Shareef et al., 2019). In another study, the nanocomposite of TiO2 and Cr2O3 has been investigated. The efficiency of the system was claimed to be 57.16 and 36.69% in the summer and winter seasons, respectively (Shanmugan and Essa, 2020). Another experimental study investigated the fumed SiO2 nanoparticle in black paint at varying concentrations (i.e., 10–40%) which is coated on the absorber plate of stepped solar still for augmenting the freshwater yield. A 10.2 and 12.3% increase in the temperature of water vapor and absorber plate, respectively, has been reported by adding the optimized concentration, i.e., 20%, in black paint (Sathyamurthy et al., 2020). In another study, it has been proven that the solar still interior coated with white-colored paint improved the distillate yield by 6.8% compared to conventionally used black paint (Tenthani et al., 2012). In another study, cuprous oxide (Cu2O) nanoparticles in black paint have been used to increase the absorber temperature. A maximum temperature of around 70°C has been reported in outdoor conditions (Kabeel et al., 2017). Other nanoparticles with different morphologies, i.e., sphere-shaped Cu2O, plate-like MoO3, and wedge-shaped ZnO, have been employed with increased surface temperature and water yield (Arunkumar et al., 2020).
Herein, we conducted detailed indoor and outdoor studies of variations in temperatures of the absorber plate concerning the size and concentration of TiO2. Previous studies only discussed the effect of the concentration of TiO2 nanoparticles. Moreover, no studies under controlled environments have been presented. In this study, a detailed and systematic investigation is performed to understand the effect of both the concentration and size of TiO2 nanoparticles. Furthermore, the experimental studies under both simulated indoor and outdoor conditions revealed in-depth effects of both the concentration and size of TiO2 nanoparticles.
Methods
Research Design
The study is designed to systematically investigate the improvement in temperature of the solar still absorber plate. Supplementary Figure 1 shows the schematic diagram of the research design. Various sizes and concentrations of TiO2 are tried in black paint formulation, and the increase in temperature is recorded in simulated indoor conditions and real-time outdoor conditions. As is well known, the increased absorption ability of the solar spectrum leads to an increase in the efficiency of the solar still. The highest temperatures achieved by the absorber will lead to an increased inefficiency, where qev is the heat energy and H is the total solar radiation that falls upon the surface.
Experimental
Figure 1 shows the schematic process diagram of specimen preparation. Black paint was considered the base fluid to paint onto the absorber plate, and TiO2 nanoparticles of sizes 20 nm (Sigma-Aldrich), 150 nm (Sigma-Aldrich), and 400 nm (supplied by Faculty of Sciences, University of Malaya) in various weight percent, i.e., 0.5, 1, 3, 5, 7, 10, 15, and 20 wt%, as nano-fillers were used. In brief, the TiO2 nanoparticles were added to 5 ml of isopropyl alcohol, sonicated for 1 h, and stirred for 1 h to achieve proper dispersion. This suspension was blended with 10 ml dark paint (KCC black paint, acrylic-based binder with carbon black pigment) and hand mixed. The prepared mixtures, i.e., black paint/TiO2 nanoparticles, were painted onto aluminum sheets using a paintbrush and dried at room temperature for 24 h. A portable alcometer (model S) was used to measure the thickness of the coating. It is worth mentioning here that five samples for each temperature reading were prepared and tested under indoor simulated conditions, and the mean values are presented throughout the study. Moreover, no repetitions were conducted at outdoor conditions because the outdoor conditions are intermittent and cannot be controlled. Furthermore, the thermocouples were placed at the center of the specimens and covered with ceramic wool to avoid direct exposure of thermocouples to irradiation.
Indoor Setup and Experimental Procedure
The indoor experiment was carried out at the solar thermal laboratory, UMPEDAC, University of Malaya. There are many components and instruments used in this experiment to fulfill the required indoor conditions. For providing required irradiations, an improvised solar simulator comprising 120 halogen bulbs (brand: OSRAM) with power, voltage, and current capacity of 90 W, 12 V, and 7.5 A, respectively, is used. The solar simulator is controlled by three variable control AC-power-supply transformers to control radiations from 100 W/m2 to 1,200 W/m2. Furthermore, few other standard measuring instruments are used to record the data. Some key instruments and sensors used are pyranometer (brand: LI-COR, LI200R), data logger [brand: dataTaker (DT80)], and thermocouples (K-type). The measuring and accuracy range of all the instruments and sensors is given in Supplementary Table 1. It should be mentioned here that it took 30 min to reach the maximum and stable temperature for all the specimens. After 30 min, the simulator was shut down to achieve a cooling curve. The arrangement of the indoor experiment is shown in Supplementary Figure 2.
Outdoor Setup and Experimental Procedure
The outdoor experimental setup was installed on the Solar Garden of Higher Institution Centre of Excellence, UM Power Energy Dedicated Advanced Centre (UMPEDAC), Kuala Lumpur, Malaysia. Experiments were carried out from 08.00 am to 02:00 pm on various days. Supplementary Figure 3 shows the outdoor experimental arrangements. Similarly, standard devices and instruments were used to record the data, and the measuring and accuracy range of all the instruments and sensors is already given in Supplementary Table 1. Sensors were placed in the systems at the necessary locations for recording the data. Other meteorological data, such as wind speed and humidity, were measured using the weather station installed at Solar Garden. All the thermocouples were covered with ceramic wool from the top to avoid direct sunlight. Furthermore, the optimum angle was calculated using Cooper’s equation to intercept the maximum radiations throughout the day:
where
Results and Discussion
XRD (D8 Discover, Bruker) was performed to identify the phases present. Figure 2 shows the XRD patterns of all the TiO2 nanoparticles as received. The peaks at 2θ are in high agreement with JCPDS number 84-1286. The peaks at 2θ values of 25.3, 37.82, and 48.02 correspond to the anatase TiO2 phase with corresponding indices as (101), (004), and (200). The diffraction patterns were also matched with JCPDS card no. 21-1272 (for anatase TiO2) and JCPDS card no. 21-1276 to confirm the rutile phase; however, some rutile phase has been identified among all the XRD patterns. It can be concluded with confidence that the dominant TiO2 phase in the powder is anatase.
Figure 3 shows the UV-Vis spectra (UV-2600i, Shimadzu) of as-received particles. A sharp absorption in the UV region was identified, followed by a reduction in absorption. The second gradual absorption was identified in the near-infrared to the far region with onset starting after 900 nm of the solar spectrum. This high absorption in the UV and infrared regions indicates high sunlight absorption properties which helped improve the surface temperature of the absorber plate.
Kuala Lumpur Weather Profile
The variation of maximum and minimum ambient temperatures, relative humidity, wind speed and direction, precipitation, and cloud cover across the year from December 2019 to 2020 is shown in Supplementary Figure 4. It can be seen that the profile is almost consistent within an average of 25°C throughout the year. However, maximum and minimum temperatures of 34 and 23°C are also seen in March. Kuala Lumpur is located in the equatorial region with two monsoon seasons in a year, namely, northeast and southwest.
Indoor Analysis
For the indoor experiment, three rounds of experiments were carried out for different particle sizes of TiO2 (20, 150, and 400 nm) with varying irradiation from 100 to 1,000 W/m2. However, for the analysis purpose, the effect of temperature on the absorber plates with the increment of 200, 600, 800, and 1,000 W/m2 and rate of drop in temperature is considered. The results of different nanoparticles at varying radiations are discussed in sub-sections.
TiO2-20 nm
Figure 4 illustrates the effect of 200, 600, and 1,000 W/m2 on the absorber plate temperature in 30 min intervals and also shows the rate of temperature drop in the absorber plate coated with TiO2-20 nm nanoparticles in black paint in 15 min intervals. As illustrated in Figures 4A–C, the increase in concentration leads to an increase in surface temperature, which can be due to an increase in the amount of UV radiation absorption, which is the characteristic of TiO2. The steady increase in temperature with concentration increment was observed till the concentration reached 15 wt%. A maximum temperature of 104.86°C at 1,000 W/m2 was recorded for specimens containing 15 wt% TiO2 of 20 nm particle size. Further increase in concentration did not improve the surface temperature. This reduction in temperature can be explained based on excessive convective losses of heat due to increased thermal conductivity. Figures 4D–F show the cooling curves of the specimens. It can be observed that an increase in concentration leads to higher cooling rates. The optimum cooling rate was observed for specimens containing 15 wt% TiO2 of 20 nm size. The same trend of temperature vs time was observed for 600 and 1,000 W/m2 irradiation levels.
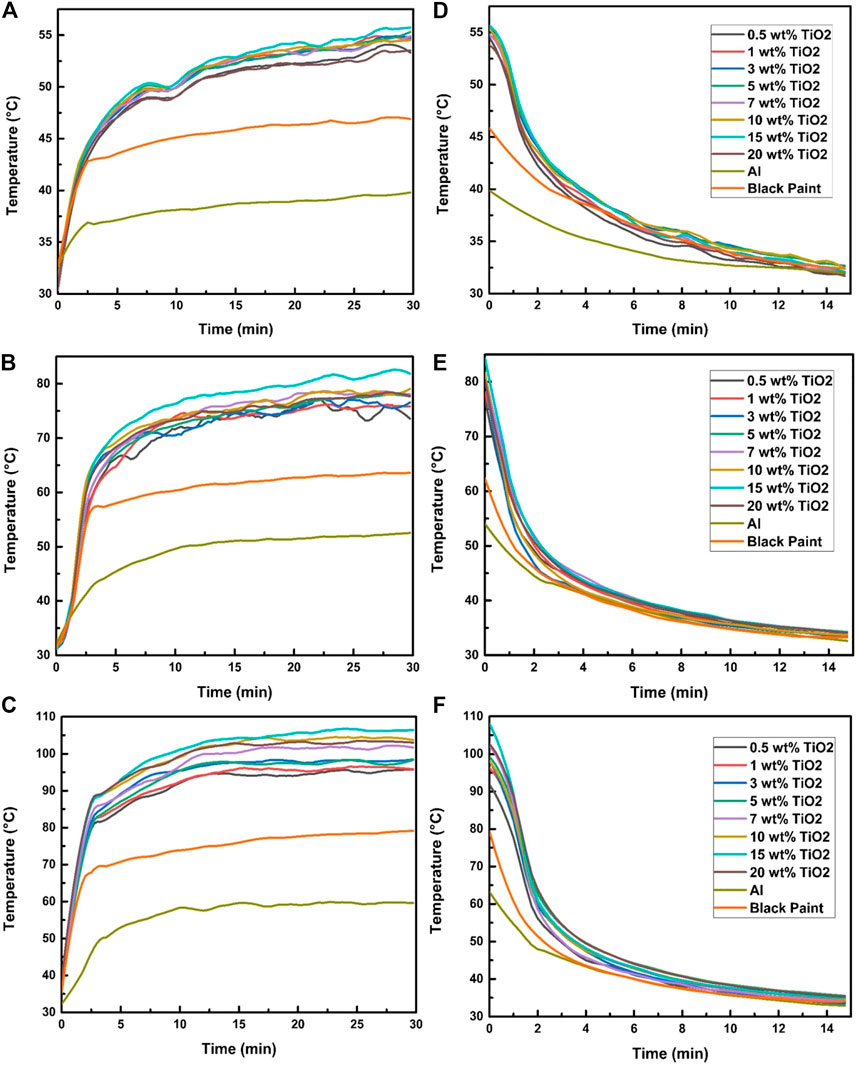
FIGURE 4. Effect of TiO2-20 nm on the absorber temperature at irradiation exposure of (A) 200 W/m2, (B) 600 W/m2, and (C) 1,000 W/m2 and (D–F) temperature drop profile.
Consequently, indoor results conclude that the thermal absorptivity of TiO2 nanoparticles increases from low (0.5%) to high (15%) concentrations, and after 15%, it decreases again.
TiO2-150 nm
Figure 5 illustrates the effect of 200, 600, and 1,000 W/m2 on the absorber plate temperature in 30 min intervals and also shows the rate of temperature drop in the absorber plate coated with TiO2-150 nm nanoparticles in black paint in 15 min intervals. As illustrated in Figures 5A–C, the results show that the steadiness of temperatures of the absorber plates is undoubtedly noticed for the time of 30 min. A further significant finding is that the rate of decrease in temperature may negatively affect the performance of solar absorbers, as shown in Figures 5D–F. The temperature drop effect was observed for about 15 min after completing each irradiation exposure.
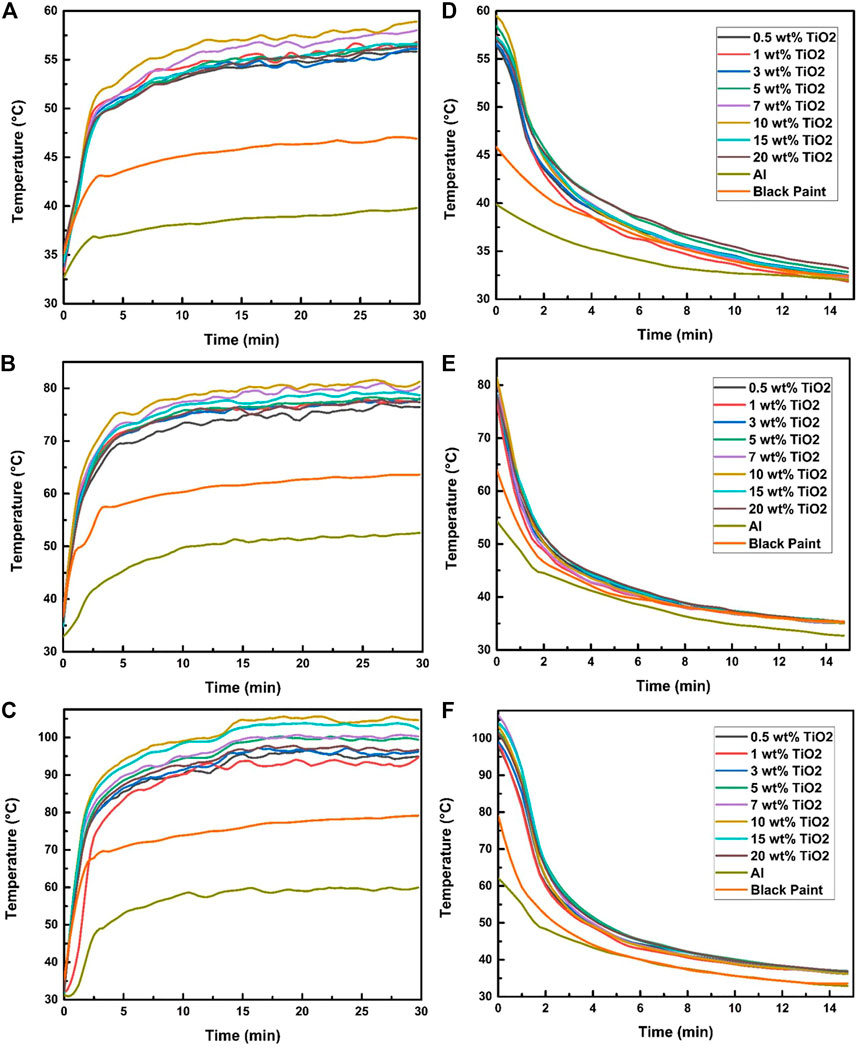
FIGURE 5. Effect of TiO2-150 nm on the absorber temperature at irradiation exposure of (A) 200 W/m2, (B) 600 W/m2, and (C) 1,000 W/m2 and (D–F) temperature drop profile.
Similarly, it can be observed from Figure 5A that, after 30 min of proper exposure of selected samples to 200 W/m2 irradiation, the average highest temperature was found to be 59.5°C in a 10% TiO2 plate, and at that time, the maximum temperature of black paint and bare aluminum was 44.2 and 37°C, respectively. Therefore, these practical results show that the impregnating 10% TiO2 nanoparticles of 150 nm in black paint increased approximately the temperature by more than 30%, respectively, compared to that of plain black paint. Moreover, it was also observed that the 10% TiO2 absorber plate properly maintained the decreasing temperature rate better than others, as presented in Figures 5D–F. Similarly, the experiment results of 600 and 1000 W/m2 showed that 10% concentration had the highest absorption and maintaining temperature. It was seen that, at 1000 W/m2, the temperature of 10% TiO2 increased to the average maximum temperature of 105.42°C, while the temperature in the black plate only raised to 79.23°C. A summary of the indoor analysis for TiO2-150 nm at 1,000 W/m2 at all concentrations is given in Table 3.
TiO2-400 nm
Figure 6 illustrates the effect of 200, 600, and 1,000 W/m2 on the absorber plate temperature in 30 min intervals and also shows the rate of temperature drop in the absorber plate coated with TiO2-400 nm nanoparticles in black paint in 15 min intervals. As illustrated in Figures 6A–C, the results show that the steadiness of temperatures of the absorber plates is undoubtedly noticed for the time of 30 min. A further significant finding is that the rate of decrease in temperature may negatively affect the performance of solar absorbers as shown in Figures 6D–F. The temperature drop effect was observed for about 15 min after completing each irradiation exposure.
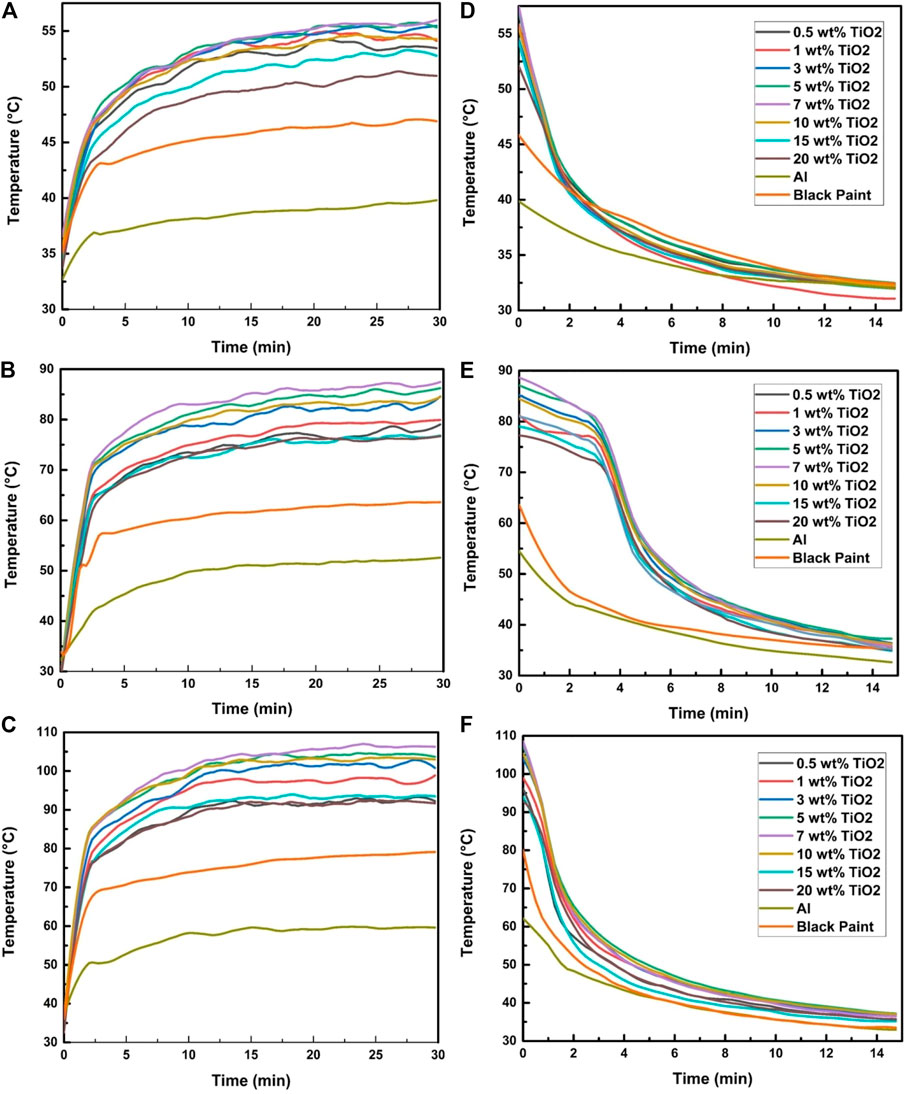
FIGURE 6. Effect of TiO2-400 nm on the absorber temperature at irradiation exposure of (A) 200 W/m2, (B) 600 W/m2, and (C) 1,000 W/m2 and (D–F) temperature drop profile.
It can be observed from Figure 6A that, after 30 min of proper exposure of selected samples to 200 W/m2 irradiation, the average highest temperature was found to be 55.9°C in a 7% TiO2 plate, and at that time, the maximum temperature of black paint and bare aluminum was 44.2°C and 37°C, respectively. Moreover, it was also observed that the 7 wt% TiO2 absorber plate properly maintained the decreasing temperature rate better than others, as presented in Figures 6D,E,F. Similarly, the experiment results of 600 and 1,000 W/m2 showed that 7 wt% concentration had the highest absorption and maintaining temperature. It was seen that, at 1,000 W/m2, the temperature of 7 wt% TiO2 increased to the average maximum temperature of 106.32°C, while the temperature in the black plate only raised to 79.23°C. It is worth mentioning here that the legends for left and right side graphs in Figures 4, 5, 6 are the same. The authors showed legends in only right-hand side graphs to avoid poor visibility.
The maximum temperatures of specimens containing 15 wt% TiO2 of 20 nm, 10 wt% TiO2 of 150 nm, and 7 wt% TiO2 of 400 nm were recorded to be 104.86°C, 105.42°C, and 106.32°C, respectively. The comparative analysis of the concentration and size of TiO2 particles suggests an increasing trend in temperature with increases in both concentration and size. This behavior can be explained through the light-scattering phenomenon. As the small-sized particles (especially 20 nm) are unable to scatter the light due to their much smaller size compared to the wavelength of UV irradiation, light-harvesting remains minimal. The particles bigger in size effectively absorb the UV light and increase the bulk surface temperature. A detailed summary of maximum temperatures under indoor conditions at all the concentrations and sizes is presented in Table 1.
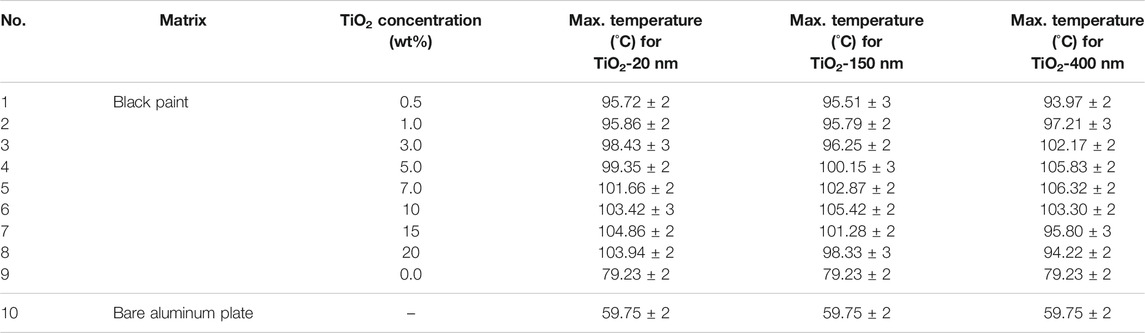
TABLE 1. Summary of indoor maximum temperature for TiO2 with varying concentrations and sizes at 1,000 W/m2.
Outdoor Analysis
The weather of Malaysia experiences an equatorial climate illustrated by hot and humid weather throughout the whole year. The southwest monsoon takes place from April to September, and the northeast monsoon occurs from October to March. The southwest monsoon highlights a drier climate with less precipitation, in contrast to the northeast monsoon, which brings more rainfall. For the outdoor experiment, three rounds of experiments were carried out for different particle sizes of TiO2 (20, 150, and 400 nm) on different days and weather conditions. The results of different nanoparticles at varying radiations are discussed in sub-sections. The outdoor experiments were conducted from 8:00 am to 2:00 pm in December.
Supplementary Figures 5,6,7 show the temperature vs time relation of specimens with varying concentrations of TiO2 and weather parameters such as temperature, wind speed, humidity, and % cloud along with solar irradiation levels for 20, 150, and 400 nm TiO2, respectively. The summary of maximum recorded temperatures for each specimen is listed in Table 2 with respect to the size at 1,000 W/m2 irradiation. An increase in TiO2 concentration in black paint leads to an increase in surface temperature; moreover, increased particle size also leads to the increase in temperature as can be seen in Table 2. This is due to light absorption characteristics of TiO2 in the UV region and light-scattering ability of large-sized particles. A maximum temperature of 71.06°C for specimens containing 15 wt TiO2 (20 nm) was recorded, which is 8.14% higher than that of the bare aluminum plate and 4.26% higher than that of the only black paint–coated aluminum plate. The maximum temperature of 74.77°C for specimens containing 10 wt TiO2 (150 nm) was recorded, which is 12.82% higher than that of the bare aluminum plate and 6.98% higher than that of the only black paint–coated aluminum plate. And a maximum temperature of 69.69°C for specimens containing 7 wt TiO2 (400 nm) was recorded, which is 15.97% higher than that of the bare aluminum plate and 10.21% higher than that of the only black paint–coated aluminum plate. The trend is strictly the same as that under the indoor conditions. The temperature variations are due to uncontrolled outer weather conditions.
Comparative Analysis
Table 3 shows the comparative percent increase in the temperature of specimens with optimum concentration under indoor conditions at 1,000 W/m2 and outdoor conditions. The percent increase in temperature is consistent for indoor and outdoor conditions. But a marked difference in controlled indoor and outdoor conditions is observed. For instance, the percent increase in temperature for specimens containing 20 nm-sized TiO2 particles under controlled and persistent 1,000 W/m2 irradiation is 75.52%, whereas the percent increase in outdoor conditions is just 8.14%. This behavior is due to high convective losses due to wind and solar intermittence under uncontrolled outdoor conditions. The difference between indoor controlled conditions and outdoor conditions for specimens containing optimum concentration and black paint–coated samples is much less than the difference for highest performing specimens and bare aluminum plates under indoor and outdoor conditions. This is because of the much less thermal conductivity of black paint compared to the bare aluminum plate.
Future Aspects
This work can be extended in the future by fabricating a novel design of solar still to improve the conventional solar still performance. Furthermore, performance analysis can be done to investigate the influence of utilizing optimum nanoparticles on the absorber of novel solar still and can be compared with a conventional solar still at the same operating conditions. The future aim should be to enhance the solar still productivity by adding the nanomaterials to the absorber paint by increasing the heat transfer rate and water temperature due to the existence of nanoparticles. Also, to ensure the stability of nanoparticles under the solar radiation and water flowing over the absorber plate, the surfaces can be prepared by some novel techniques.
Conclusion
The current research paper presents a systematic investigation effect of particle size and concentration of TiO2 on the surface temperature of the absorber plate. This research aimed to enhance the performance of the absorber of solar still to increase distillate output. Various particle sizes, i.e., 20, 150, and 400 nm, were investigated with concentrations varying from 1 to 20%. XRD was employed for phase identification, whereas UV-Vis spectroscopy was used to observe the optical absorption of nanoparticles under investigation. A simple solution mixing method was employed to incorporate nanoparticles in black paint and applied on aluminum plates manually. Indoor tests were performed in a controlled environment using the solar simulator. Furthermore, outside testing was also conducted to compare the performance in real time. An increase in surface temperature was recorded by increasing the particle size and concentration. The highest temperature of 106.4°C was observed for specimens containing 400 nm TiO2 with 7 wt% concentration under controlled indoor conditions, which is approximately 77.97% higher than that of the bare aluminum plate. The increase in temperature can be associated with optimum thermal conduction and the high scattering ability of large-sized particles. Furthermore, the highest temperature for 20 nm TiO2 in black paint was observed to be 104.86°C at 15 wt% concentration (which is 75.52% higher than that of bare aluminum) and 105.42°C for 150 nm TiO2 at 10 wt% concentration (which is 76.26% higher than that of bare aluminum) in indoor controlled conditions. The same trend was observed for outdoor experiments where the maximum percent increase for 20, 150, and 400 nm at 15 wt%, 10 wt%, and 7 wt%, respectively, was recorded to be 8.14, 12.87, and 15.97%, respectively, compared to that of the bare aluminum plate. Further increase in concentration leads to a reduction in surface temperature due to increased thermal conduction which in turn caused excessive heat losses due to convection as observed in cooling curves.
Data Availability Statement
The prepared samples to support the findings of this study are available from the corresponding authors upon reasonable request.
Author Contributions
HS and LK wrote the first draft of the manuscript. AZ and MUA suggested the theoretical aspects of the study and participated in the revision of the manuscript. UG and TZ participated in data processing to customize the results. SB corrected the manuscript. MSA and JS designed the study. All the authors approved the final manuscript.
Funding
The authors would like to acknowledge the financial assistance of Japan International Cooperation Agency for AUN/SEED-Net on Collaboration Education Program UM CEP 1901, Japan ASEAN Collaborative Education Program (JACEP). The authors would also thank the technical and financial assistance of UM Power Energy Dedicated Advanced Centre (UMPEDAC) and the Higher Institution Centre of Excellence (HICoE) Program Research Grant, UMPEDAC - 2020 (MOHE HICOE - UMPEDAC), Ministry of Education Malaysia, TOP100 UMPEDAC, RU003-2020, University of Malaya.
Conflict of Interest
The authors declare that the research was conducted in the absence of any commercial or financial relationships that could be construed as a potential conflict of interest.
Publisher’s Note
All claims expressed in this article are solely those of the authors and do not necessarily represent those of their affiliated organizations, or those of the publisher, the editors, and the reviewers. Any product that may be evaluated in this article, or claim that may be made by its manufacturer, is not guaranteed or endorsed by the publisher.
Acknowledgments
The authors would like to acknowledge the financial assistance of Japan International Cooperation Agency for AUN/SEED-Net on Collaboration Education Program UM CEP 1901, Japan ASEAN Collaborative Education Program (JACEP). The authors would also thank the technical and financial assistance of UM Power Energy Dedicated Advanced Centre (UMPEDAC) and the Higher Institution Centre of Excellence (HICoE) Program Research Grant, UMPEDAC - 2020 (MOHE HICOE - UMPEDAC), Ministry of Education Malaysia, TOP100 UMPEDAC, RU003-2020, University of Malaya.
Supplementary Material
The Supplementary Material for this article can be found online at: https://www.frontiersin.org/articles/10.3389/fmats.2021.683490/full#supplementary-material
References
Abdelal, N., and Taamneh, Y. (2017). Enhancement of pyramid solar still productivity using absorber plates made of carbon fiber/CNT-modified epoxy composites. Desalination 419, 117–124. doi:10.1016/j.desal.2017.06.012
Abujazar, M. S. S., Fatihah, S., and Kabeel, A. E. (2017). Seawater desalination using inclined stepped solar still with copper trays in a wet tropical climate. Desalination 423, 141–148. doi:10.1016/j.desal.2017.09.020
Ali, A., Tufa, R. A., Macedonio, F., Curcio, E., and Drioli, E. (2018). Membrane technology in renewable-energy-driven desalination. Renew. Sust. Energ. Rev. 81, 1–21. doi:10.1016/j.rser.2017.07.047
Alotaibi, S., Ibrahim, O. M., Luo, S., and Luo, T. (2017). Modeling of a continuous water desalination process using directional solvent extraction. Desalination 420, 114–124. doi:10.1016/j.desal.2017.07.004
Arunkumar, T., Murugesan, D., Viswanathan, C., Neri, G., and Denkenberger, D. (2020). Effect of CuO, MoO3 and ZnO nanomaterial coated absorbers for clean water production. SN Appl. Sci. 2 (10), 1–19. doi:10.1007/s42452-020-03504-5
Baticados, E. J. N., Capareda, S. C., Liu, S., and Akbulut, M. (2020). Advanced Solar Still Development: Improving Distilled Water Recovery and Purity via Graphene-Enhanced Surface Modifiers. Front. Environ. Sci 8, 531049. doi:10.3389/fenvs.2020.531049
Biswas, A. K., and Tortajada, C. (2019). Water Crisis and water wars: Myths and realities. England: Taylor & Francis.
Chandrashekara, M., and Yadav, A. (2017). Water desalination system using solar heat: a review. Renew. Sust. Energ. Rev. 67, 1308–1330.
Deshmukh, H. S., and Thombre, S. B. (2017). Solar distillation with single basin solar still using sensible heat storage materials. Desalination 410, 91–98. doi:10.1016/j.desal.2017.01.030
Dsilva Winfred Rufuss, D., Suganthi, L., Iniyan, S., and Davies, P. A. (2018). Effects of nanoparticle-enhanced phase change material (NPCM) on solar still productivity. J. Clean. Prod. 192, 9–29. doi:10.1016/j.jclepro.2018.04.201
Heidary, B., Hashjin, T. T., Ghobadian, B., and Roshandel, R. (2018). Optimal integration of Small scale hybrid solar wind RO-MSF desalination system. Renew. Energ. Focus 27, 120–134. doi:10.1016/j.ref.2018.05.003
Hong, Z., Pei, J., Wang, Y., Cao, B., Mao, M., Liu, H., et al. (2019). Characteristics of the direct absorption solar collectors based on reduced graphene oxide nanofluids in solar steam evaporation. Energ. Convers. Manag. 199, 112019. doi:10.1016/j.enconman.2019.112019
Huang, L., Jiang, H., Wang, Y., Ouyang, Z., Wang, W., Yang, B., et al. (2020). Enhanced water yield of solar desalination by thermal concentrated multistage distiller. Desalination 477, 114260. doi:10.1016/j.desal.2019.114260
Kabeel, A., Abdelgaied, M., and Mahmoud, G. (2020). Performance evaluation of continuous solar still water desalination system. J. Therm. Anal. Calorim., 1–10. doi:10.1007/s10973-020-09547-5
Kabeel, A. E., El-Said, E. M. S., and Dafea, S. A. (2019a). Design considerations and their effects on the operation and maintenance cost in solar-powered desalination plants. Heat Trans. Asian Res. 48 (5), 1722–1736. doi:10.1002/htj.21454
Kabeel, A. E., Omara, Z. M., Essa, F. A., Abdullah, A. S., Arunkumar, T., and Sathyamurthy, R. (2017). Augmentation of a solar still distillate yield via absorber plate coated with black nanoparticles. Alexandria Eng. J. 56 (4), 433–438. doi:10.1016/j.aej.2017.08.014
Kabeel, A. E., Sathyamurthy, R., Sharshir, S. W., Muthumanokar, A., Panchal, H., Prakash, N., et al. (2019b). Effect of water depth on a novel absorber plate of pyramid solar still coated with TiO2 nano black paint. J. Clean. Prod. 213, 185–191. doi:10.1016/j.jclepro.2018.12.185
Khanafer, K., and Vafai, K. (2018). A review on the applications of nanofluids in solar energy field. Renew. Energ. 123, 398–406. doi:10.1016/j.renene.2018.01.097
Naveenkumar, R., Gurumoorthy, G., Kunjithapatham, G., Bharath, A., and Ravichandran, M. (2020). Impact of adding various nano materials in the efficiency of single slope solar still: A review. Mater. Today Proc 33, 3942–3946. doi:10.1016/j.matpr.2020.06.275
Sathyamurthy, R., Kabeel, A. E., Balasubramanian, M., Devarajan, M., Sharshir, S. W., and Manokar, A. M. (2020). Experimental study on enhancing the yield from stepped solar still coated using fumed silica nanoparticle in black paint. Mater. Lett. 272, 127873. doi:10.1016/j.matlet.2020.127873
Sathyamurthy, R., Kabeel, A. E., El‐Agouz, E. S., Rufus, D., Panchal, H., Arunkumar, T., et al. (2019). Experimental investigation on the effect of MgO and TiO 2 nanoparticles in stepped solar still. Int. J. Energ. Res 43 (8), 3295–3305. doi:10.1002/er.4460
Shanmugan, S., Essa, F. A., Gorjian, S., Kabeel, A. E., Sathyamurthy, R., and Muthu Manokar, A. (2020). Experimental study on single slope single basin solar still using TiO2 nano layer for natural clean water invention. J. Energ. Storage 30, 101522. doi:10.1016/j.est.2020.101522
Shareef, A. S., Rashid, F. L., and Alwan, H. F. (2019). Enhancement of Fresh Water Production in Solar Still Using New Phase Change Materials. J. Adv. Res. Fluid Mech. Therm. Sci. 61 (1), 63–72.
Sharshir, S. W., Peng, G., Wu, L., Yang, N., Essa, F. A., Elsheikh, A. H., et al. (2017). Enhancing the solar still performance using nanofluids and glass cover cooling: experimental study. Appl. Therm. Eng. 113, 684–693. doi:10.1016/j.applthermaleng.2016.11.085
Srithar, K., and Rajaseenivasan, T. (2018). Recent fresh water augmentation techniques in solar still and HDH desalination - A review. Renew. Sust. Energ. Rev. 82, 629–644. doi:10.1016/j.rser.2017.09.056
Keywords: solar still, titanium dioxide, thermal absorber, weight concentration, coating
Citation: Samneang H, Kumar L, Zafar A, Ali MU, Zahid T, Bibi S, Ahmad MS, Ghafoor U and Selvaraj J (2021) A Systematic Indoor and Outdoor Study of the Effect of Particle Size and Concentration of TiO2 in Improving Solar Absorption for Solar Still Application. Front. Mater. 8:683490. doi: 10.3389/fmats.2021.683490
Received: 21 March 2021; Accepted: 05 August 2021;
Published: 21 September 2021.
Edited by:
Peter Reiss, Commissariat à l'Energie Atomique et aux Energies Alternatives, FranceReviewed by:
Ahmed Mourtada Elseman, Central Metallurgical Research and Development Institute, EgyptAdnan Khan, University of Engineering and Technology, Peshawar, Pakistan
Copyright © 2021 Samneang, Kumar, Zafar, Ali, Zahid, Bibi, Ahmad, Ghafoor and Selvaraj. This is an open-access article distributed under the terms of the Creative Commons Attribution License (CC BY). The use, distribution or reproduction in other forums is permitted, provided the original author(s) and the copyright owner(s) are credited and that the original publication in this journal is cited, in accordance with accepted academic practice. No use, distribution or reproduction is permitted which does not comply with these terms.
*Correspondence: Saira Bibi, c2FpcmEuYmliaUBmY20zLnBhZi1pYXN0LmVkdS5waw==; Muhammad Shakeel Ahmad, c2hha2VlbGFscGhhQGdtYWlsLmNvbQ==; Usman Ghafoor, dXNtYW5naGFmb29yOTlAZ21haWwuY29t
†These authors have contributed equally to this work