- 1School of Materials Science and Engineering, Shaanxi University of Technology (SNUT), Hanzhong, China
- 2School of Environmental and Chemistry Engineering, Kunming Metallurgy College, Kunming, China
A new class of near-infrared (NIR) reflectance pigments based on Co1−xMgxCr2−yAlyO4 (x = 0–1 and y = 0–2) was synthesised using the Pechini-type sol-gel process. The developed powders were characterised by thermogravimetry and differential scanning calorimetry, X-ray diffraction (XRD), ultraviolet–visible near-infrared diffuse reflectance spectroscopy, and colour Commission Internationale del'Eclairage (CIE) 1976 (L*a*b*) colour scales. The XRD patterns revealed characteristic peaks of the spinel structure with good crystallinity. Substituting Mg2+ for Co2+ ions in the tetrahedral positions and Al3+ for Cr3+ ions in the octahedral positions of CoCr2O4 reduces the cost and changes the colour of the pigment (green to yellow and then blue). Moreover, the synthesised pigments exhibited high NIR solar reflectance in the 780–2,500 nm wavelength range. The thermal and chemical stability of the pigments was also studied. Our findings demonstrate the potential for applying these pigments in cool colourants.
Highlights
(1) A new class of near-infrared reflectance pigments based on Co1-xMgxCr2-yAlyO4 (x=0-1 and y=0-2) were synthesised via Pechini-type sol-gel method.
(2) The colours of the pigment samples can be tuned from green to yellow and then blue.
(3) Decreasing the Co and Cr contents reduces the cost and environmental damages.
(4) Pigment properties make it a potential candidate for use as cool pigments.
Introduction
In recent years, near-infrared (NIR) reflective pigments have received considerable attention in construction, plastic, ink, and military industries. NIR reflective pigments are complex metal oxides with high reflectance for NIR radiation (Song et al., 2019; Ali et al., 2018; Rossi et al., 2020; Jing et al., 2018; Zhou et al., 2020). The everyday use of solar reflective roofing materials has been proven to save energy, alleviate urban heat islands, and curb global warming (Ferrari et al., 2015; Zou and Zhang, 2020; Yang et al., 2017). Most of the literature on NIR reflective pigments are available as patents (Swiler et al., 2003; Blonski et al., 2001), demonstrating their vast applications. Many of these inorganic pigments consist of hazardous heavy metals such as cobalt (Co), lead (Pb), cadmium (Cd), and chromium (Cr), which are restricted under present environmental regulations. Several of these methods are expensive (Hedayati et al., 2015; Schildhammer et al., 2017; Yuan et al., 2018). Recently, many rare-earth-based NIR reflective pigments have been suggested as substitutes for traditional pigments, owing to their low toxicity (Raj et al., 2019; Han et al., 2014). Aju Thara et al. (2017); Zhao et al. (2015) prepared a series of NIR pigments based on yttrium molybdate (Y2Mo3O12) and yttrium cerate, and illustrated the NIR reflectance and colouring mechanism. However, rare-earth compounds are expensive because they are difficult to mine.
Numerous methods have been employed to synthesise simple oxide or oxide-based systems. Most conventional methods involve solid-state reactions (Zhang et al., 2018), co-precipitation (Frolova et al., 2017), hydrothermal (McMahon et al., 2019; Elakkiya and Sumathi, 2020), evaporative drying (Droguet et al., 2021), and sol-gel (polymeric precursor method) (Lima et al., 2020). The sol-gel method should be emphasised as it has numerous desirable characteristics, such as using affordable reagents and reducing costs, and requiring low temperature for oxide synthesis. In addition, the method has excellent chemical homogeneity at the molecular level of all components and has easy stoichiometric control in complex systems (Gonzaga et al., 2020).
Spinel pigments, which are represented chemically as AB2O4, have generated an increasing interest. These pigments exhibit a myriad of colours and tonalities owing to their ability to accommodate different cations (Bao et al., 2016). For example, cobalt chromite (CoCr2O4) is an essential commercial material and is extensively used as a catalyst, magnetic compound, and pigment owing to its superior properties. Because cobalt and chromium are generally rare and harmful, incorporating less toxic and expensive metals into the CoCr2O4 minimises the cost of production and impact on the environment. Therefore, in this study, Mg2+ substitution for Co2+ and Al3+ substitution for Cr3+ in CoCr2O4 was facilitated using the Pechini-type sol-gel process. The effects of these enrichments on the crystal structures, optical properties, and thermal and chemical stabilities of as-synthesised pigments were thoroughly investigated.
Experimental
Materials and Methods
Magnesium nitrate (Mg(NO3)2·6H2O), cobalt nitrate (Co(NO3)2·6H2O), citric acid (CA, C6H8O7), chromium nitrate (Cr(NO3)3·9H2O), aluminium nitrate (Al(NO3)3·9H2O), and ethylene glycol (EG, C2H6O2) were the starting materials. All chemicals utilised were analytical grade, acquired from Beijing Chemical Reagent Corporation, and used as received without further purification. All pigment samples of Co1−xMgxCr2−yAlyO4 (x = 0–1 and y = 0–2) were synthesised using the Pechini-type sol-gel method.
First, CA was dissolved in distilled water and stirred continuously at 80°C. Subsequently, nitrates of cobalt, chromium, magnesium, and aluminium were added stoichiometrically into the acid solution. The molar ratio of the CA/metal was 3:1. After dissolution, EG was added at a CA/EG ratio of 3:2. All solutions were mixed using a magnetic stirrer. The resultant solution was then heated on a hot plate at approximately 80°C for approximately 1 h to produce polymerisation gel through a polymerisation reaction. Subsequently, the beaker was kept in a thermostat drier at 120°C for 12 h. The preliminary heat treatment (350°C) of the gel was performed in air for 2 h. Gas liberation by combustion during heat treatment partially degraded the organic structure and its expansion, forming a brittle black powder precursor. Finally, the obtained powder of the precursor was ground into a fine powder and then calcined at 900°C at a heating rate of 10°C/min for 6 h to remove residual organic materials, and powder samples were synthesised.
Characterisation Techniques
Thermogravimetry and differential scanning calorimetry (TG-DSC) were performed using a STAR E TGA/DSC1 instrument to analyse the powder precursor and synthesised pigments. The powdered samples were heated under nitrogen in the temperature range 50–900°C at a rate of 20°C/min. Approximately 10 mg of the sample was loaded into aluminium crucibles for testing. Tabular α-Al2O3 was used as the reference for weight loss.
A Rigaku Ultima Ⅳ X-ray diffractometer equipped with Ni-filtered Cu Kα radiation (λ = 0.15406 nm, 40 kV, and 40 mA) was used to examine the crystal structures of the synthesised samples at 25°C. Step scanning over an angular range of 2θ (10°–80°) with a step size of 0.02°and a counting time of 5 s at each step were used for data collection.
The diffuse reflectance of the samples was measured with a 5-nm step size in the range 380–2,500 nm using a Lambda 950 UV–vis NIR spectrophotometer with an integrating sphere attachment, calibrated by a white standard (D65 illumination such as daylight).
The colour Commission Internationale del'Eclairage chromaticity analysis software (Perkin Elmer) was used to estimate the pigment colour parameters from the reflection data of visible light. The CIE 1976 L*a*b*colourimetry method was employed following the recommendations of the CIE. In this system, the L* value signifies the lightness or darkness of the colour (L* is equal to 0 and 100 for black and white, respectively). The values of a* (green (−) → red (+) axis) and b* (blue (−) → yellow (+) axis) indicate the colour hue. The parameter C* (chroma) represents the colour saturation and is defined in Eq. (1) (Sukmarani et al., 2020; Gong et al., 2021).
The measurement standard JG/T 235-2014 was used to guide the calculations for the NIR solar reflectance (R*) in the wavelength range 780–2,500 nm. The function R* was determined using Eq. (2), as follows:
where i(λ) is the solar special irradiance (W m−2 nm−1), and r(λ) corresponds to the spectral reflectance achieved experimentally (W m−2). The NIR solar reflectance value quantifies the unabsorbed solar radiation on the surface of a substance and signifies the build-up of heat in structures upon exposure to the Sun (Hedayati et al., 2015).
Results and Discussion
Thermal Analysis
Figure 1A shows the thermal analysis (TG-DSC) results for the synthesised pigment. The TG curve depicts three different stages with a total weight loss of 43%, which can be observed from the TG curve. For instance, increasing the temperature (50°C–300°C), decreased the weight of the CoCr2O4 precursor by 13%. Weight loss is associated with the removal of residual water, unpolymerised CA, and EG from the precursor. The weight loss increased by more than 25% at 300°C–600°C.
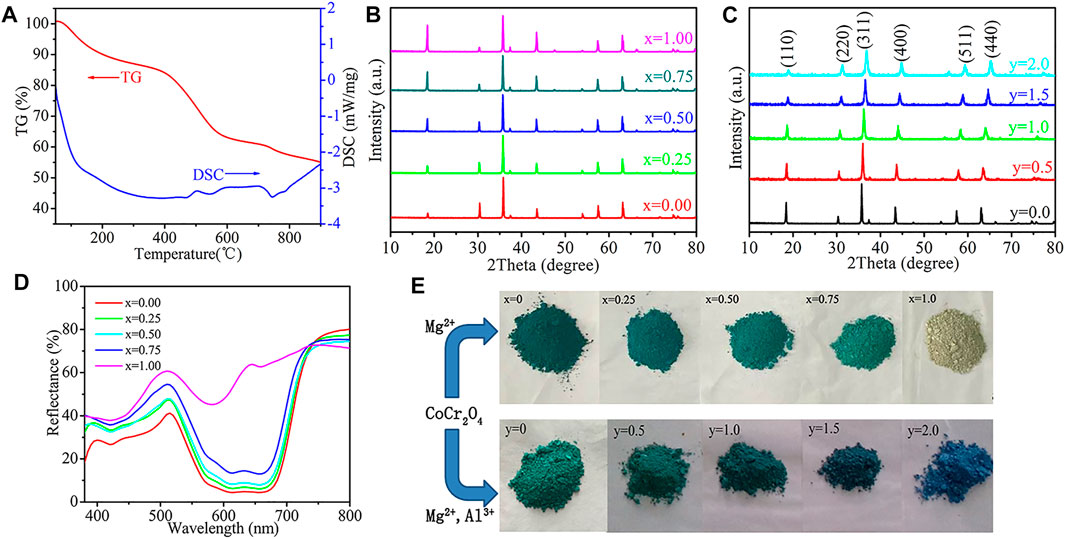
FIGURE 1. (A) TG-DSC curves of CoCr2O4 pigment precursor (B) XRD diffraction patterns of Co1−xMgxCr2O4 (x = 0.00, 0.25, 0.50, 0.75, 1.00) pigments (C) XRD diffraction patterns of Co0.25Mg0.75Cr2−yAlyO4 (y = 0.0, 0.5, 1.0, 1.5, 2.0) pigments (D) UV–vis absorption spectra of Co1−xMgxCr2O4 (x = 0.00, 0.25, 0.50, 0.75, 1.00) powdered pigments (E)photographs of Co1−xMgxCr2O4 (x = 0.00–1.00) and Co0.25Mg0.75Cr2−yAlyO4 (y = 0.0–2.0) pigments.
An exothermic peak in the DSC curve was observed at approximately 500°C–600°C, indicating the evolution of H2O, COx, and NOx via the combustion of the nitrates and organic impurities (Liu et al., 2015). Furthermore, a highly exothermic peak in the DSC curve was observed at 700°C–800°C, which was attributed to the precursor decomposition into CoCr2O4 particles. The mass loss was less than 5% at temperatures higher than 600°C, implying the realisation of final CoCr2O4 pigments.
Powder X-Ray Diffraction Analysis
Figure 1B shows the XRD patterns of the as-synthesised Co1−xMgxCr2O4 (x = 0.00, 0.25, 0.50, 0.75, and 1.00) precursors after grinding and calcining at 900°C for 6 h. The analysis revealed peaks of the cubic spinel structure, which were observed for all samples. The space groups, Fd3m, of CoCr2O4 and MgCr2O4 were distinguished based on JCPDS 22-1084 and 65-3106, respectively. In addition, the XRD patterns did not exhibit non-spinel impurity phases.
Figure 1C illustrates the XRD patterns of Co0.25Mg0.75Cr2−yAlyO4 (y = 0.0, 0.5, 1.0, 1.5, and 2.0). All the diffraction peaks were associated with the cubic spinel crystal structure of CoCr2O4, and the structure was not altered by co-doping with Mg2+ and Al3+. The addition of Al3+ ions slightly shifted the XRD peaks towards higher diffraction angles than the parent oxide. A variation in the cell volume may have caused the observed 2θ shift. Typically, an increase in the Al3+ concentration decreases the cell volume because a smaller Al3+ (0.051 nm) displaces the larger Cr3+ (0.061 nm) ions (Liu et al., 2015; Wu et al., 2018). Supplementary Tables S1, S2 show that there is no obvious change in cell volume with the increase in Mg concentration in CoCr2O4, which is mainly due to the similar ionic radii of Mg2+ and Co2+. With the increase in Al concentration, the cell volume decreases regularly, indicating that the replacement of Cr by Al is successful. The pigment morphology was characterised by SEM (Supplementary Figure S1), and the average grain size was less than 5 μm. Elemental mapping analysis (Supplementary Figure S2) further revealed that Cr, Co, O, Mg, and Al elements exhibited a uniform distribution.
Optical Properties of Magnesium Doping
Figure 1D demonstrates the UV–vis absorption spectra of the powdered Co1−xMgxCr2O4 (x = 0.00, 0.25, 0.50, 0.75, and 1.00) samples. An intense triple band at approximately 570, 610, and 660 nm was observed for the CoCr2O4 sample spectrum. The absorption peaks steadily weakened with increasing and decreasing concentrations of Mg and Co, respectively. According to the Tanabe–Sugano energy level diagrams, the 4F ground-state term separates into three terms, 4A2, 4T2, and 4T1(F) in the tetrahedral coordination of Co2+ ions with the d7 configuration, while the 4P excited-state term transforms into 4T1(P). Thus, the three possible spin transitions are ν1: 4A2(F)→ 4T2(F), ν2: 4A2(F)→ 4T1(F), and ν3: 4A2(F)→ 4T1(P). The first and second bands lie within the IR region, whereas the third band lies in the visible region. The broadband can be ascribed to the ν3 transition (4A2(F)→ 4T1(P) transition) of Co2+ ions, which decomposes into three bands because of L-S Russell–Saunders coupling (Hedayati et al., 2015; Lourenco et al., 2016). Therefore, this broad triple band within 500 and 700 nm results in blue pigmentation of compounds containing Co2+.
The chromatic properties of the synthesised Co1−xMgxCr2O4 pigments were assessed using their CIE 1976 L*a*b*colour coordinates (Supplementary Table S3). The addition of Mg2+ lightened the colour of the samples, as evidenced by the increasing L* from 52.92 to 77.56. In contrast, increasing Mg2+ substitution results in the loss of the green pigment hue, as is apparent from the change in the colour coordinate a* from −38.80 to −7.32. Moreover, the b* values increased from −15.43 to 9.14, indicating intensification of the yellow colour in the pigments. The C* values, representing the richness of the colour hue, decreased significantly from 41.76 to 11.71. The observed hue angles of the pigments are found to be in the green region of the cylindrical colour space. Figure 1E shows the photographs of the synthesised powdered pigment samples.
Figure 2A shows the NIR reflectance spectra of the Co1−xMgxCr2O4 pigments. The base compound, CoCr2O4, has an NIR solar reflectance (R*) of 55.1%. Simultaneously, substituting Co2+ ions with Mg2+ ions decreased the NIR reflectance of the pigments from 55.1 (x = 0.00) to 52.8% (x = 0.50). Further doping of Mg2+ with Co2+ increased the NIR reflectance to 60.3% (x = 1.00). Supplementary Table S3 presents detailed information on the NIR solar reflectance of the powdered pigments. The NIR reflectance of a typical pigment sample Co0.25Mg0.75Cr2O4 (R* = 56.3%) was significantly higher than that of the Y2BaCuO5 green pigment reported elsewhere (R* = 50%) (Shankland et al., 1974; Jose et al., 2014). The designed class of pigments displayed high reflectance in the NIR region, suggesting that these formulations could be considered cooling pigments. In addition, with the increase in Mg doping amount, the as-prepared pigments showed a red shift in the band gap transition.
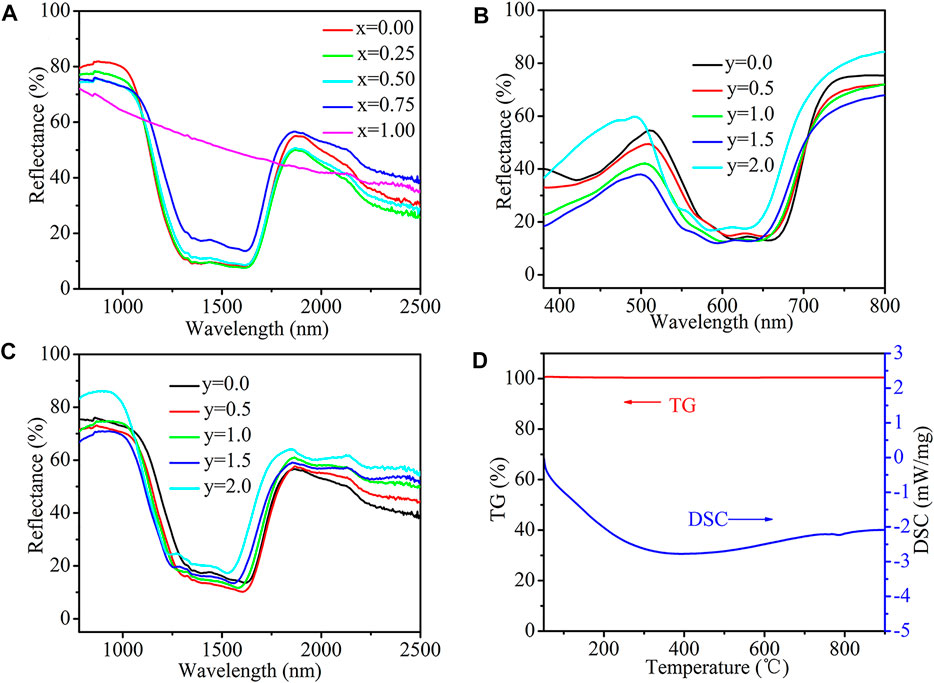
FIGURE 2. (A) NIR reflectance of Co1−xMgxCr2O4 (x = 0.00, 0.25, 0.50, 0.75, 1.00) powdered samples (B) UV–vis absorption spectra of Co0.25Mg0.75Cr2−yAlyO4 (y = 0.0, 0.5, 1.0, 1.5, 2.0) powdered pigments (C) NIR reflectance of Co0.25Mg0.75Cr2−yAlyO4 (y = 0.0, 0.5, 1.0, 1.5, 2.0) powdered samples (D)TG-DSC analysis of powdered Co0.25Mg0.75Al2O4 pigment sample.
Optical Properties of Aluminium Doping
Figure 2B illustrates the UV–vis absorption spectra of the developed Co0.25Mg0.75Cr2−yAlyO4 (y = 0.0, 0.5, 1.0, 1.5, and 2.0) samples. A prominent blue shift of the triple band occurred when the Al3+ content was increased from 0 to 2. The energy level of the excitation state for Co2+ and the position of the absorption band are dependent on the Co2+ ion surroundings. The gradual substitution of Al3+ for Cr3+ indicates a substantial change in the volume of the octahedral site. Subsequently, oxygen ligands experience less attraction from octahedrally coordinated ions because of the lower atomic mass of aluminium than that of chromium. Therefore, the exposure of the oxygen ligands is more to Mg2+and Co2+, enhancing the splitting of the d-orbital energy levels and the blue shift of the absorption band (Figure 2B) (Hedayati et al., 2015; Shankland et al., 1974).
The chromatic properties of the synthesised Co0.25Mg0.75Cr2−yAlyO4 pigments were obtained from their CIE 1976 L*a*b* colour coordinates (Supplementary Table S4). Substituting Al3+ for Cr3+ in Co0.25Mg0.75Cr2O4 spinel increased a* and decreased b*, weakening the intensity of the green colour in the pigments while enhancing the blue pigmentation. Substituting Al3+ with Cr3+ ions in the spinel structure decreased L* from 64.82 to 53.87, darkening the samples. Consequently, C*, which represents the richness of the colour hue, decreased from 34.93 to 24.33. However, the L* and C* values changed slightly when Al3+ was completely substituted with Cr3+ ions in the spinel structure. With the increasement of Al doping, the hue angles of pigment move from green to blue region of the cylindrical colour space. Figure 1E shows photographs of the synthesised powdered pigment samples.
Figure 2C shows the NIR reflectance spectra of the powdered samples. The NIR solar reflectance of the samples decreased from 56.3 (y = 0.0) to 51.9 (y = 1.5) with the substitution of Al3+ for Cr3+. Supplementary Table S4 lists the NIR solar reflectance of the powdered pigments. The reflectance reached a maximum (R* = 61.7%) for y = 2.0, which was higher than that of aluminium-doped cobalt chromite pigments of similar colour, as reported elsewhere (R* = 52%) (Hedayati et al., 2015). Furthermore, with the increase in Al doping amount, the as-obtained pigments also exhibit a red shift in the band gap transition. These observations demonstrate that Mg2+ and Al3+ co-doped CoCr2O4 systems are appropriate cooling pigments.
Studies on Thermal and Chemical Stabilities of the Pigments
The Co0.25Mg0.75Al2O4 pigments were investigated from 50 to 900 °C for their thermal stability. The results indicated that there was a negligible weight loss and phase transition of the pigment (Figure 2D). The chemical resistance of the pigment was investigated using 5% HCl/H2SO4/HNO3 and NaOH (Bao et al., 2016; Jose and Reddy, 2013). A pre-weighed pigment powder was soaked in an acid/alkali solution for 30 min and stirred using a magnetic stirrer. The pigment powder slurry was filtered, washed with water, dried, and weighed again. Negligible weight loss of the pigment was observed for all the tested acids and alkalis. The colour coordinates of the pigments were measured and had negligible total colour difference (△E*) (Supplementary Table S5). These results demonstrate that the pigments were chemically and thermally stable.
Conclusion
In this study, a series of NIR reflective pigments with the general formula Co1−xMgxCr2−yAlyO4 (x = 0–1 and y = 0–2) were synthesised using the Pechini-type sol-gel method. The effect of Mg2+ and Al3+ enrichment on the structure and optical and chromatic properties of the products was investigated. XRD analyses revealed that the pigments were well crystallised in spinel cubic structures. The pigments exhibited a wide range of colours from green to yellow and blue and showed clear NIR reflective performance (greater than 51%). The pigments also showed good thermal and chemical stability.
Data Availability Statement
The original contributions presented in the study are included in the article/Supplementary Material, and further inquiries can be directed to the corresponding authors.
Author Contributions
XW and XZ: Synthesis of pigments and corresponding characterisations and analysis; writing of original drafts. DZ and TA: helped in data analysis; WB revised the manuscript.
Funding
This research was supported by the National Natural Science Foundation of China (Grant Nos. 51504147) and the National & Local Joint Engineering Laboratory for Slag Comprehensive Utilization and Environmental Technology Open Fund (Grant Nos. SLGPT2019KF01-03).
Conflict of Interest
The authors declare that the research was conducted in the absence of any commercial or financial relationships that could be construed as a potential conflict of interest.
Publisher’s Note
All claims expressed in this article are solely those of the authors and do not necessarily represent those of their affiliated organizations, or those of the publisher, the editors and the reviewers. Any product that may be evaluated in this article, or claim that may be made by its manufacturer, is not guaranteed or endorsed by the publisher.
Supplementary Material
The Supplementary Material for this article can be found online at: https://www.frontiersin.org/articles/10.3389/fmats.2022.850115/full#supplementary-material
References
Aju Thara, T. R., Rao, P. P., Divya, S., Raj, A. K. V., and Sreena, T. S. (2017). Enhanced Near Infrared Reflectance with Brilliant Yellow Hues in Scheelite Type Solid Solutions, (LiLaZn)1/3MoO4-BiVO4 for Energy Saving Products. ACS Sustain. Chem. Eng. 5, 5118–5126. doi:10.1021/acssuschemeng.7b00485
Ali, A. A., El Fadaly, E., and Ahmed, I. S. (2018). Near-infrared Reflecting Blue Inorganic Nano-Pigment Based on Cobalt Aluminate Spinel via Combustion Synthesis Method. Dyes Pigments 158, 451–462. doi:10.1016/j.dyepig.2018.05.058
Bao, W., Ma, F., Zhang, Y., Hao, X., Deng, Z., Zou, X., et al. (2016). Synthesis and Characterization of Fe3+ Doped Co0.5Mg0.5Al2O4 Inorganic Pigments with High Near-Infrared Reflectance. Powder Technol. 292, 7–13. doi:10.1016/j.powtec.2016.01.013
Blonski, R. P., Pipoly, R. A., and Sliwinski, T. R. (2001). Infrared Reflective Color Pigment. EP1141105 A1.
Droguet, B., Liang, H., Frka-Petesic, B., Parker, R., De Volder, M., Baumberg, J., et al. (2021). Large-scale Fabrication of Structurally Coloured Cellulose Nanocrystal Films and Effect Pigments. Nat. Mater. 21, 352–358.doi:10.1038/s41563-021-01135-8
Elakkiya, V., and Sumathi, S. (2020). Low-temperature Synthesis of Environment-Friendly Cool Yellow Pigment: Ce Substituted SrMoO4. Mater. Lett. 263, 127246. doi:10.1016/j.matlet.2019.1272460167
Ferrari, C., Muscio, A., Siligardi, C., and Manfredini, T. (2015). Design of a Cool Color Glaze for Solar Reflective Tile Application. Ceram. Int. 41, 11106–11116. doi:10.1016/j.ceramint.2015.05.058
Frolova, L., Pivovarov, A., and Butyrina, T. (2017). Synthesis of Pigments in Fe2O3-Al2O3-CoO by Co-precipitation Method. Prt 46, 356–361. doi:10.1108/PRT-07-2016-0073
Gong, L., Liang, J., Kong, L., Chen, B., Li, Y., and Tian, G. (2021). Synthesis of High-Performance Copper Barium Silicate Composite Pigment from Waste Iron Ore Tailings. Ceram. Int. 47, 27987–27997. doi:10.1016/j.ceramint.2021.06.230
Gonzaga, L. A., Santana, V. T., Bernardi, M. I. B., Hrubý, J., Neugebauer, P., and Mesquita, A. (2020). CeO2 and CeO2 :Pr Nanocrystalline Powders Prepared by the Polymeric Precursor Method: Yellow and Red Pigments with Tunable Color. J. Am. Ceram. Soc. 103, 6280–6288. doi:10.1111/jace.17339
Han, A., Ye, M., Liu, L., Feng, W., and Zhao, M. (2014). Estimating Thermal Performance of Cool Coatings Colored with High Near-Infrared Reflective Inorganic Pigments: Iron Doped La2Mo2O7 Compounds. Energy Build. 84, 698–703. doi:10.1016/j.enbuild.2014.08.024
Hedayati, H. R., Sabbagh Alvani, A. A., Sameie, H., Salimi, R., Moosakhani, S., Tabatabaee, F., et al. (2015). Synthesis and Characterization of Co1−xZnxCr2−yAlyO4 as a Near-Infrared Reflective Color Tunable Nano-Pigment. Dyes Pigments 113, 588–595. doi:10.1016/j.dyepig.2014.09.030
Jing, J., Zhang, Y., Sun, J., Zhao, X., Gao, D., and Zhang, Y. (2018). A Comparative Study on Different RE-doped (RE=Pr, Nd, Sm) SrCuSi4O10 Blue Pigments with High Near-Infrared Reflectance. Dyes Pigments 150, 9–15. doi:10.1016/j.dyepig.2017.10.045
Jose, S., Prakash, A., Laha, S., and Natarajan, S. (2014). Green Colored Nano-Pigments Derived from Y2BaCuO5: NIR Reflective Coatings. Dyes Pigments 107, 118–126. doi:10.1016/j.dyepig.2014.03.025
Jose, S., and Reddy, M. L. (2013). Lanthanum-strontium Copper Silicates as Intense Blue Inorganic Pigments with High Near-Infrared Reflectance. Dyes Pigments 98, 540–546. doi:10.1016/j.dyepig.2013.04.013
Lima, N. A., Alencar, L. D. S., Siu-Li, M., Feitosa, C. A. C., Mesquita, A., M’peko, J.-C., et al. (2020). NiWO4 Powders Prepared via Polymeric Precursor Method for Application as Ceramic Luminescent Pigments. J. Adv. Ceram. 9, 55–63. doi:10.1007/s40145-019-0347-z
Liu, L., Han, A., Ye, M., and Zhao, M. (2015). Synthesis and Characterization of Al3+ Doped LaFeO3 Compounds: A Novel Inorganic Pigments with High Near-Infrared Reflectance. Sol. Energy Mater. Sol. Cells 132, 377–384. doi:10.1016/j.solmat.2014.08.048
Lourenço, S. A., Silva, R. S., and Dantas, N. O. (2016). Tunable Dual Emission in Visible and Near-Infrared Spectra Using Co2+-Doped PbSe Nanocrystals Embedded in a Chalcogenide Glass Matrix. Phys. Chem. Chem. Phys. 18, 23036–23043. doi:10.1039/c6cp04419k
McMahon, M. E., Santucci, R. J., Glover, C. F., Kannan, B., Walsh, Z. R., and Scully, J. R. (2019). A Review of Modern Assessment Methods for Metal and Metal-Oxide Based Primers for Substrate Corrosion Protection. Front. Mat. 6, 190–213. doi:10.3389/fmats.2019.00190
Raj, A. K. V., Rao, P. P., Sreena, T. S., and Thara, T. R. A. (2019). Pigmentary Colors from Yellow to Red in Bi2Ce2O7 by Rare Earth Ion Substitutions as Possible High NIR Reflecting Pigments. Dyes Pigments 160, 177–187. doi:10.1016/j.dyepig.2018.08.010
Rossi, S., Lindmark, H., and Fedel, M. (2020). Colored Paints Containing NIR-Reflective Pigments Exposed to Accelerated Ultraviolet Radiation Aging with Possible Application as Roof Coatings. Coatings 10, 1135–1152. doi:10.3390/coatings10111135
Schildhammer, D., Fuhrmann, G., Petschnig, L., Schottenberger, H., and Huppertz, H. (2017). Synthesis and Optical Properties of New Highly NIR Reflective Inorganic Pigments RE6Mo2O15 (RE = Tb, Dy, Ho, Er). Dyes Pigments 140, 22–28. doi:10.1016/j.dyepig.2017.01.021
Shankland, T. J., Duba, A. G., and Woronow, A. (1974). Pressure Shifts of Optical Absorption Bands in Iron-Bearing Garnet, Spinel, Olivine, Pyroxene, and Periclase. J. Geophys. Res. 79, 3273–3282. doi:10.1029/jb079i023p03273
Song, Y., Chen, W., Lim, X. M., Hu, X., Liu, M., and Zhang, Q. (2019). Electronic Configuration in Outset Orbitals of Doping Elements Plays as a Key Factor in Tuning Near Infrared Reflection of YMn0.9M0.1O3 (M = Cr, Mn, Fe, Co, Al, Ga and In). J. Solid State Chem. 273, 81–84. doi:10.1016/j.jssc.2019.02.040
Sukmarani, G., Kusumaningrum, R., Noviyanto, A., Fauzi, F., Habieb, A. M., Amal, M. I., et al. (2020). Synthesis of Manganese Ferrite from Manganese Ore Prepared by Mechanical Milling and its Application as an Inorganic Heat-Resistant Pigment. J. Mater. Res. Technol. 9, 8497–8506. doi:10.1016/j.jmrt.2020.05.122
Swiler, D. R., Detrie, T. J., and Axtell, E. A. (2003). Rare Earth Transition Metal Oxide Pigments. US, US6582814 B2.
Wu, Y., Qiu, K., Tang, Q., Zhang, W., and Wang, J. (2018). Luminescence Enhancement of Al3+ Co-doped Ca3Sr3(VO4)4:Eu3+ Red-Emitting Phosphors for White LEDs. Ceram. Int. 44, 8190–8195. doi:10.1016/j.ceramint.2018.01.267
Yang, R., Han, A., Ye, M., Chen, X., and Yuan, L. (2017). Synthesis, Characterization and Thermal Performance of Fe/N Co-doped MgTiO3 as a Novel High Near-Infrared Reflective Pigment. Sol. Energy Mater. Sol. Cells 160, 307–318. doi:10.1016/j.solmat.2016.10.045
Yuan, L., Han, A., Ye, M., Chen, X., Yao, L., and Ding, C. (2018). Synthesis and Characterization of Environmentally Benign Inorganic Pigments with High NIR Reflectance: Lanthanum-Doped BiFeO3. Dyes Pigments 148, 137–146. doi:10.1016/j.dyepig.2017.09.008
Zhang, S., Pan, Z., and Wang, Y. (2018). Synthesis and Characterization of (Ni, Sb)-Co-Doped Rutile Ceramic Pigment via Mechanical Activation-Assisted Solid-State Reaction. Particuology 41, 20–29. doi:10.1016/j.partic.2017.12.016
Zhao, X., Zhang, Y., Huang, Y., Gong, H., and Zhao, J. (2015). Synthesis and Characterization of Neodymium Doped Yttrium Molybdate High NIR Reflective Nano Pigments. Dyes Pigments 116, 119–123. doi:10.1016/j.dyepig.2015.01.018
Zhou, N., Sha, S., Zhang, Y., Li, S., Xu, S., and Luan, J. (2020). Coprecipitation Synthesis of a Green Co-doped Wurtzite Structure High Near-Infrared Reflective Pigments Using Ammonia as Precipitant. J. Alloys Compd. 820, 153183–483191. doi:10.1016/j.jallcom.2019.153183
Keywords: near-infrared reflectance, sol-gel method, Mg2+ and Al 3+ co-doped, cool pigments, solar reflectance
Citation: Wei X, Zou X, Deng Z, Bao W, Ai T and Zhang Q (2022) Synthesis and Characterisation of Mg2+ and Al3+ Co-Doped CoCr2O4 Inorganic Pigments With High Near-Infrared Reflectance. Front. Mater. 9:850115. doi: 10.3389/fmats.2022.850115
Received: 07 January 2022; Accepted: 24 May 2022;
Published: 01 July 2022.
Edited by:
Weihua Li, University of Wollongong, AustraliaReviewed by:
Ayman Awad Ali, Benha University, EgyptCopyright © 2022 Wei, Zou, Deng, Bao, Ai and Zhang. This is an open-access article distributed under the terms of the Creative Commons Attribution License (CC BY). The use, distribution or reproduction in other forums is permitted, provided the original author(s) and the copyright owner(s) are credited and that the original publication in this journal is cited, in accordance with accepted academic practice. No use, distribution or reproduction is permitted which does not comply with these terms.
*Correspondence: Weiwei Bao, YmFvd2VpMTgzNEAxNjMuY29t; Qi Zhang, NDgwODA3NzY3QHFxLmNvbQ==