Polyglycerol/Polydopamine-Coated Nanoparticles for Biomedical Applications
- Institute for Protein Research, Osaka University, Osaka, Japan
Nanoparticles play an active role in biomedical science due to their unique properties, which cannot be obtained from bulk materials. Therefore, understanding and controlling the physicochemical properties of nanoparticles are gaining increasing importance for their practical applications. Surface coating is an important technique that controls the physical properties of nanoparticles since the coating is the first part of the nanoparticle that is in contact with the environment. Additionally, the coating creates robust targeting, therapy, imaging, and sensing opportunities. This review first introduced two recently developed outstanding coatings, namely, hyperbranched polyglycerol and polydopamine, and the research achieved by the polyglycerol/polydopamine-coated nanoparticles was then highlighted.
Introduction
Nanoparticles (NPs) are gaining popularity in biomedical applications. They are defined as particles smaller than 100 nm in size, with numerous types reported to date comprising organic, inorganic, and metal NPs (Mcnamara and Tofail, 2016; Maiti et al., 2018; Aflori, 2021). Additionally, NPs show unique chemical, physical, and/or optical properties compared with bulk materials attributed to their nanometer size and large surface-to-volume ratio. These properties vary significantly in size, shape, structure, and composition. For example, the energy level of electrons becomes discrete when they are confined to a nanometric region, revealing peculiar electrical, and optical properties. Also, in the medical field, NPs are useful carriers of biomolecules and/or drugs in certain parts of the body for diagnosis and therapy (Brigger et al., 2012). They also passively accumulate in tumors through their enhanced permeability and retention (EPR) effect (Shi et al., 2020). Recently, crystal defects in inorganic NPs, such as diamond NPs, have attracted new imaging, and sensing applications (Basso et al., 2020). Additionally, small molecules, polymers, and biomolecules modify the surface of NPs. These special properties allow NPs in advanced biomedical applications, such as targeting, therapy, imaging, and sensing (Figure 1A) (Davis et al., 2008; Holzinger et al., 2014; Yang et al., 2019; Mitchell et al., 2021).
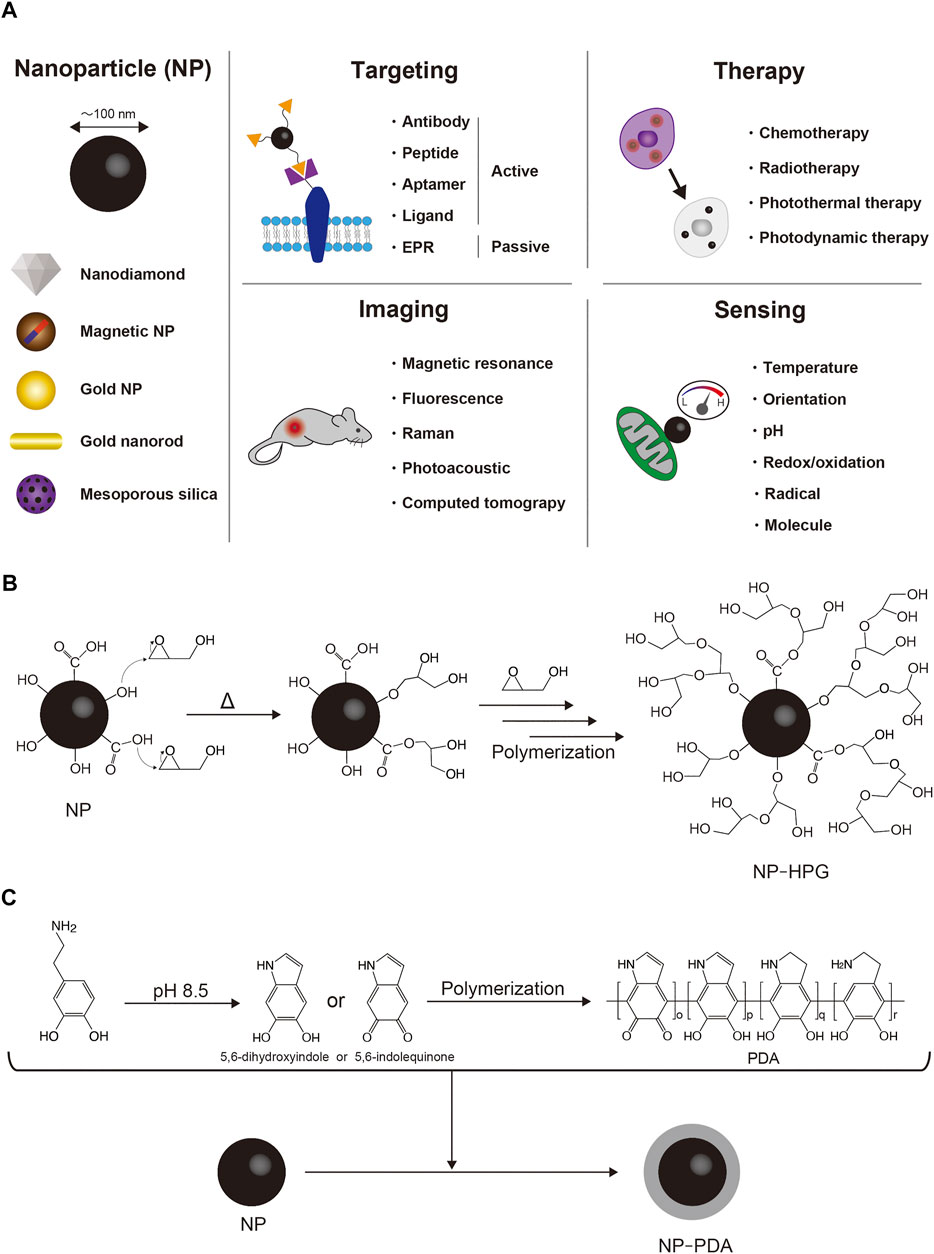
FIGURE 1. Schematic illustration of (A) nanoparticles focused in this review and their biomedical application and synthesis of (B) NP-HPG and (C) NP-PDA.
Surface coating with organic polymers is crucial to controlling the properties of NPs since it determines the interaction between NPs and the environment. For example, the colloidal stability of NPs at different pH values or in the presence of salt greatly depends on the coatings, including an adequate coating that contributes to the aggregation inhibition. Additionally, the coating provides prospects for further functionalization, e.g., imaging probes, targeting agents, biomolecules, and drugs. There are numerous combinations of NPs and surface coatings; thus, the potential applications of hybrids of NPs and polymers are vastly robust. Common approaches applied for surface coating include the atom transfer radical polymerization or reversible addition-fragmentation chain transfer polymerization (Zoppe et al., 2017). However, these methods involve multiple organic synthesis steps, are technically difficult and require transition metal catalysts, such as Cu+ ions, and for polymerization. However, their use in synthesis is best avoided due to the toxicity of these transition metal ions to living organisms. Recently, two surface coatings have been developed with simple, versatile, robust, and metal-free methods: hyperbranched polyglycerol (HPG; Figure 1B) and polydopamine (PDA; Figure 1C) coatings. This article reviewed the fundamental properties of HPG and PDA and recent advances in the bioapplications of NPs with HPG and PDA coatings. Supplementary Table S1 summarizes the size, surface functionalization, and applications of NPs coated with HPG and PDA.
Surface Coating
Polyglycerol
HPG is obtained through a ring-opening reaction of glycidol, with a structure similar to the branched polyethylene glycol and OH groups as a terminal (Khan and Huck, 2003; Wang et al., 2008). As a result, HPG exhibits excellent hydrophilicity reduces nonspecific absorption of biomolecules (Zhao et al., 2011; Zhao et al., 2012; Sotoma et al., 2015; Jafari et al., 2020; Zou et al., 2020). Additionally, HPG can be modified with various functional groups from the terminal OH groups. Notably, Sandler and Berg (Sandler and Berg, 1966) first attempted the demonstration of the polymerization of glycidol, and later, researchers devoted efforts to polymerizing glycidol using various approaches.
In 2011, Komatsu and colleagues reported a simple method of HPG coating on nanodiamond (ND) surfaces, where the ND and glycidol mixture was heated at 140°C in argon atmosphere for 20 h without a catalyst, producing ND-HPG (Zhao et al., 2011). The ring-opening reaction mechanism is the polymerization reaction between an OH group and a carbon atom in the epoxy group initiated from the nucleophilic groups on the surface of NPs. Hence, nanoparticles must be designed to have nucleophilic groups on their surface. The thickness of the HPG layer is controlled by changing the reaction time and temperature (Zou et al., 2020). The HPG modification altered the hydrophobic nature of the ND material such that ND-HPG showed extremely high solubility not only in pure water but also in buffer solutions. Furthermore, the HPG layer blocks nonspecific absorption, with further functionalization with targeting moieties (antibody, ligand, and among others), specifically targeting NPs to the biomolecules of interest (Hsieh et al., 2019). Besides, Sotoma and coworkers reported one-pot functionalization of COOH, amine, and alkyne (Sotoma et al., 2018; Terada et al., 2018; Hsieh et al., 2019). Zhou et al. also revealed that HPG-coated metal quantum dots (QDs) are less toxic than pristine QDs due to the biocompatible envelope of HPG on the QDs (Zhou et al., 2009).
Polydopamine
PDA is a bioinspired polymer similar in capabilities with a mussel’s adhesive foot protein that firmly attaches the mussel to a surface, polymerizing on the NP surfaces (Lee et al., 2007). Although the mechanism remains controversial (Liebscher et al., 2013), dopamine self-polymerizes under basic conditions (pH 8.5), creating a layer of PDA adhering strongly to the surface of NPs without pretreatment. The thickness of the PDA layer is easily controlled by changing the dopamine concentration and reaction time. Also, PDA possesses universal adhesion to any material or surface and provides active platforms for further functionalization through catechol/quinone groups (Liu et al., 2014). The notable feature of PDA is its photothermal effect; PDA nanospheres achieved 40% photothermal conversion efficiency, much higher than that of gold nanorods (GNRs) (22%) (Liu Y. et al., 2013; Jung et al., 2018; Harvey et al., 2019). Hence, it is applied in hyperthermia therapy against cancer (Zelasko-Leon et al., 2015; Li D. et al., 2016; Ding et al., 2016; Cheng Y. et al., 2017). Furthermore, PDA can be easily functionalized with metal nanomaterials by reducing metal ions. Therefore, various metal types can be deposited on the surface of PDA, including Au, Ag, Pt, and Cu (Zeng et al., 2018; Lu et al., 2020).
Nanoparticles
Diamond
The diamond NP is a carbon-based nanomaterial with a broad prospect for bioapplications. This review classified ND into three types: detonation nanodiamond (DND), nanodiamond without a nitrogen-vacancy center (ND), and ND with nitrogen-vacancy centers (FND). The DND is a synthetic diamond obtained from explosives with a 4–6 nm uniform size (Mochalin et al., 2011; Dolmatov et al., 2020). Additionally, since DNDs exhibit tunable surfaces, excellent biocompatibility, and large areas, they are attracted as drug and gene carriers (Zhang et al., 2009; Huang et al., 2010; Mochalin et al., 2011). However, the NDs produced by chemical vapor deposition or high-pressure high-temperature methods with a size of 30–100 nm are used for bioimaging applications (Hui et al., 2010). Therefore, particular attention is given to NDs containing nitrogen-vacancy centers (NVCs), e.g., FNDs (Yu et al., 2005). The FND fluorescence shows no photobleaching or photoblinking, permitting single-particle tracking and long-term fluorescence imaging (Yu et al., 2005). Also, the magneto-optical property is another notable feature of FND. The quantum states of the electron spins in NVCs are optically read at room temperature via optically detected magnetic resonance (ODMR) (Gruber et al., 1997; Degen et al., 2017). The ODMR signal allows nanoscale sensing of an electric field, magnetic field, temperature, and angle with high precision (Wu et al., 2016; Zhang et al., 2021).
Magnet
Magnetic NPs (MNPs), such as Fe3O4, are conventional nanomaterials that offer controlled size, size-dependent magnetic property, manipulation externally, paramagnetic properties, and heat generation in an alternating magnetic field associated with hysteresis loss (Holzinger et al., 2014; Ali et al., 2021; Materón et al., 2021). It is possible to synthesize MNP with uniform size distribution using the bottom-up approach and controlled particle size. Therefore, due to these properties, MNPs are used for drug/gene delivery, protein separation, contrast enhancement in magnetic resonance imaging (MRI), and hyperthermia (Materón et al., 2021). It is also necessary to employ a synthesis method that exposes OH groups on the surface of MNPs for HPG coating.
Gold
GNPs are among the most studied nanomaterials, with their unique optoelectronic properties, indispensable for DDS probes, catalysts, and sensors (Yeh et al., 2012; Elahi et al., 2018). Since the physical, chemical, optical, and electrical properties change with size and shape, various GNPs have been developed, including sphere, rod, hollow, star, and cubic (De Berardis et al., 2021). Principally, GNRs have two surface bands, a short-wavelength band (TSPR: transverse surface plasmon resonance) and a long-wavelength band in the near-infrared region (LSPR: longitudinal SPR). The TSPR is generally observed around 525–586 nm, whereas the LSPR is highly dependent on the aspect ratio of the GNR (Nikoobakht and El-Sayed, 2003; Runowski et al., 2019). Additionally, GNRs generate heat used mainly for photothermal therapy of cancer cells after absorbing light in the region of their TSPR or LSPR (Huang et al., 2008; Zelasko-Leon et al., 2015; Du et al., 2017; Riley and Day, 2017). The surfaces of the synthesized GNPs are stabilized with citric acid or cetyltrimethylammonium bromide. Also, the PDA coating applies to GNP even in the presence of these stabilizers. However, HPG coating is not applicable because GNPs do not have nucleophilic groups on the surface. Sotoma et al. reported the possibility of growing HPG on NP-PDA since PDA acts as a scaffold for the ring-opening polymerization of glycidol (Sotoma and Harada, 2019). Therefore, subsequent HPG coating overcomes the relatively low dispersity of NP-PDA.
Quantum Dot
QDs are 2–10 nm NPs with a fluorescence property because of the quantum confinement effect. They have numerous potential applications, including bioimaging, displays, solar cells, and quantum communication. Various QDs have been developed, including heavy metals, silicon, and carbon. Among them, QDs made of heavy metals show cytotoxicity, requiring surface coating to reduce the toxicity. Additionally, the wavelength of fluorescence emission of QDs can be tuned by controlling their sizes. These properties often contribute to bioimaging and biodiagnosis (Kairdolf et al., 2013). Also, a recent study reported that QDs serve as a nanometric temperature sensor, with monitoring fluorescence intensity or fluorescence lifetime (Medintz et al., 2005; Kalytchuk et al., 2017). However, since PDA coatings absorb light and considerably reduce fluorescence, limited research has been conducted on PDA QD coating.
Mesoporous Silica
Mesoporous silica NPs (MSNPs) are amorphous silica NPs with numerous pores with sizes ranging from 2 to 50 nm (Narayan et al., 2018) that have gained increasing attention due to their catalytic and biomedical applications. Several types of mesoporous silica depends on the morphology, such as SBA, LMU, FSM, MCM, among others, and including varying particle properties (Moritz and Geszke-Moritz, 2015). Molecules can be loaded into the pores and slowly released, with their properties often used for DDS (Narayan et al., 2018; Niculescu, 2020). Although surface modification of MSNPs is relatively easy through silane reagents, HPG-coated MSNPs have not been reported to date.
Application
HPG-Coated NPs
HPG coating is used to increase dispersity and actively target DND (Zhao et al., 2014c; Zhao L. et al., 2015; Li et al., 2018; Li et al., 2019; Yuan et al., 2019; Chen et al., 2020), ND (Zhao et al., 2011; Zhao et al., 2014a; Yoshino et al., 2019; Zou et al., 2020; Zou et al., 2021), FND (Boudou et al., 2013; Sotoma et al., 2015; Sotoma et al., 2016; Torelli et al., 2019; Barton et al., 2020; Suarez-Kelly et al., 2021), or MNP (Wang et al., 2008; Wang et al., 2009; Wang et al., 2011; He et al., 2015). Reports have shown that the aggregation property of DND, which is stronger than that of NDs, is overcome by HPG coating (Sotoma and Shirakawa, 2016). Previous MNP experiment with HPG originates from Zhao et al., who revealed that the HPG layer suppresses nonspecific adsorption of proteins on the MNP surface to a level comparable or superior to commonly used polyethylene glycol (Zhao et al., 2012). In the latter study, Zou et al. reported that the 30 wt% HPG layer on the surface of NPs prevented protein corona formation, thus indicating its stealth effect (Zou et al., 2020). These properties enable the HPG-coated NPs for targeted therapy, imaging, and sensing.
NP-HPGs are used for selective delivery of dox or cisplatin to A549, U937, U87MG, glioblastoma, and breast cancer cells (Zhao et al., 2014b; Zhao et al., 2014c; Li et al., 2019), indicating the enhanced therapy efficacy. For example, Zhao et al. developed ND-HPG further functionalized with RGD peptide as the targeting moiety and platinum as a drug, i.e., ND-HPG-RGD-Pt (Zhao et al., 2014c). Furthermore, the group showed that ND-HPG-RGD-Pt was preferentially assimilated by specific U87MG cells expressing RGD peptide receptors and revealed cytotoxicity, illustrating its potential as a chemotherapy agent.
Additionally, Hsieh et al. used FND-HPG for single-particle tracking. The group selectively targeted FND-HPG to membrane glycoprotein through click chemistry and continuously monitored the protein movements on/in live cells for over 2 h (Hsieh et al., 2019). These results affirm the potential for biomedical applications at the single-molecule level. Finally, Arsalani demonstrated the application of the MNP-HPG for MRI of the liver and kidney in vivo. They found that the particles produced a strong negative contrast, which persisted in the liver and kidney for 80 and 110 min, respectively (Arsalani et al., 2012).
Igarashi et al. reported a unique sensing application by tracking the three-dimensional rotational motion of F1-ATPase using FND-HPG with angular uncertainty of ±3 with a time resolution of 1.7 s (Igarashi et al., 2020).
Additionally, regarding other NPs, HPG coating has been reported for TiO2 (Qin et al., 2016), CdTe (Zhou et al., 2009), CdSe-ZmS (Panja et al., 2017), Mn-ZnSeS (Panja et al., 2017), silicon NPs (Das and Jana, 2014), and carbon dot (Li et al., 2017). Also, Panja et al. used silica coating on CdSe-ZnS, Mn-ZnSeS, γ-Fe2O3, and gold particles as a scaffold for HPG coating. These studies reported that HPG modification does not interfere with QD fluorescence, has high dispersibility, reduces QD cytotoxicity, and improves hemocompatibility. Furthermore, Das et al. succeeded specifically targeting glioblastoma and cervical cancer cells with overexpressing αvβ3 integrin by modifying the HPG surface on silicon QDs with (cRGDfK) peptide (Das and Jana, 2014).
PDA-Coated NPs
The dispersibility and stealth effect of PDA were not high. However, its surface modification was easy, with its dispersibility, and stealth effect enhanced by modifying it with PEG or other chemicals. Additionally, tumor accumulation due to the EPR effect was observed. The high scalability permits nanoparticles to be conjugated and expands the multifunctional application in therapy, imaging, and sensing.
MNP-PDA has absorption properties used for eliminating metals and dyes (Liu R. et al., 2013; Xie et al., 2014; Zhao Y. et al., 2015; Li J. et al., 2016). Zhang et al. reported a protein-imprinted MNP-PDA that improved binding ability of the respective targets using the absorption property, demonstrating high potential for proteomics and drug delivery (Zhang et al., 2012). Moreover, MNP-PDA serves as an imaging/therapy platform (Lin et al., 2014; Zheng et al., 2015; Xue et al., 2017). Lin et al. revealed that PDA exhibited strong NIR absorbance, high fluorescence quenching, and high functionality. Additionally, the group illustrated that MNP-PDA act as a multifunctional agent for intracellular mRNA detection and synergistic MRI and photoacoustic dual-modal imaging-guided photothermal therapy (Lin et al., 2014).
PDA coating is reported for GNP (Choi et al., 2015; Wang C. et al., 2016; Xu et al., 2019), GNR (Black et al., 2013; Zelasko-Leon et al., 2015; Wang S. et al., 2016), hollow (Ju et al., 2015), and nanostar (Li D. et al., 2016; You et al., 2019). Liu et al. assessed GNP-PDA’s cellular uptake and biodegradability in vivo and in vitro (Liu X. et al., 2013). TEM observation revealed that no significant change was affected by the GNP-PDA structure in the lysosomes or cytosol within cells over a 24 h incubation period and in the liver or the spleen from 1 day to 6 weeks after injection. Zhang et al. synthesized GNR-PDA-loaded RGD peptide, cisplatin, and iodine-125 for an image-guided combination chemo-thermal therapy platform (Zhang et al., 2016). The hybrid particles target the tumors, and upon internalization into cells, the particles release cisplatin into endosomes. The particles can be visualized via CT imaging and photoacoustic imaging.
Additionally, MSNPs with PDA shells realize unique DDS applications (Cheng et al., 2017b; Wei et al., 2017; Lei et al., 2019; Shi et al., 2019). Zheng et al. developed a system for drug release control through coating drug-loaded MSNPs with PDA (Zheng et al., 2014). Notably, the system uses PDA as a gatekeeper mechanism to inhibit drug release. Once the particles are placed under acidic conditions such as endosomes, the PDA disassembles, gradually release the drugs into the cell. Cheng et al. developed a nanocarrier system of PDA-coated MSNPs functionalized with d-a-tocopheryl polyethylene glycol 1,000 succinate (TPGS) (i.e., MNSs-DOX@PDA-TPGS). The groups demonstrated that MSNs-DOX@PDA-TPGS displays exceptional overcomes multidrug resistance with better therapeutic efficacy in vivo than free DOX and DOX-loaded NPs without TPGS ligand modification (Cheng et al., 2017a).
FND-PDA functions double-sided since PDA exhibits photothermal properties in nanoheater/thermometer and is also used for intracellular thermal conductivity measurements (Sotoma et al., 2021). Therefore, the measurement of intracellular thermal conductivities of HeLa and MCF-7 cells was examined, revealing a mean conductivity of the two separate cell lines of 0.11 ± 0.04 Wm−1 K−1 (95% confidence) with similar accuracies, significantly lower than that of water (0.6 Wm−1 K−1). Therefore, the latest study group fabricated a composite quantum sensor containing FND, PDA, GNP, and HPG as a dual-purpose, enhanced nanoheater/thermometer (Sotoma and Harada, 2021).
Other interesting bioapplications of NP-PDA have been reported and summarized in excellent papers such as protein sensing (Xia et al., 2013), stimuli-responsive molecule release (Yang et al., 2021), biocatalyst (Liu R. et al., 2013; Xie et al., 2014; Landarani-Isfahani et al., 2015; Deng et al., 2016; Liu et al., 2017; Lu et al., 2020), molecule removal from a solution (Xie et al., 2014; Li J. et al., 2016; Baghban et al., 2018), immune assay (Lai et al., 2013), antibacterial (Fu et al., 2021), wound healing (Zheng et al., 2021), and tissue engineering (Tang et al., 2021).
Conclusions and Perspectives
This review described how HPG and PDA coatings affect the properties of the NPs, including the current applications of HPG/PDA-coated NPs. The ease of coating and functionalization and excellent biocompatibility have greatly improved and broadened the application of the numerous NPs in biomedical science, including targeting, therapy, imaging, sensing, and technologies that combine them.
Nevertheless, HPG and PDA have several hurdles to overcome for future study. For HPG coating, nucleophilic groups on the surface of NPs are necessary for the coating. To overcome this limitation, NPs without nucleophilic groups on their surface must introduce appropriate scaffolds. Also, care must be taken to ensure that this scaffold does not affect the intrinsic properties of the NPs. Despite increasing investigations, PDA’s polymerization mechanism remains controversial. Notably, the structure is vital in understanding its physical properties; however, a deeper understanding will better control the strategy for later functionalization.
HPG and PDA are reported to be less toxic and reduce the inherent toxicity of NPs in biological systems. However, there are limited reports on the interaction of HPG and PDA coatings, which affects cellular functions. Additionally, their long-term stability, and biodegradability in vivo are not fully understood. The systematic investigation on the HPG-NPs and PDA-NPs in animal models accumulate safety knowledge and perspectives, thus increasing potential clinical applications.
Once the abovementioned issues can be addressed, HPG and PDA coatings will greatly impact the biomedical field. Furthermore, the trend of future research will be the combination of NP functions with those of polymers to create nanorobots with multifunctional properties that modify their functions according to external signals and/or the local environment, subsequently establishing a new diagnostic system, and tailor-made medicine. Therefore, I believe the interest in these coatings is expected to increase substantially in the next decades.
Author Contributions
The author confirms being the sole contributor of this work and has approved it for publication.
Funding
Grant-in-Aid for JSPS Research Fellow 18J002870, Early-Career Scientists 19K16089, and 21K15053, by ATI Research Grant RG3004, and Kyoto Technoscience Center Research Grant supported this work.
Conflict of Interest
The author declares that the research was conducted in the absence of any commercial or financial relationships that could be construed as a potential conflict of interest.
Publisher’s Note
All claims expressed in this article are solely those of the authors and do not necessarily represent those of their affiliated organizations, or those of the publisher, the editors and the reviewers. Any product that may be evaluated in this article, or claim that may be made by its manufacturer, is not guaranteed or endorsed by the publisher.
Acknowledgments
The author acknowledges Ms. Mayo Sotoma for her insightful discussion.
Supplementary Material
The Supplementary Material for this article can be found online at: https://www.frontiersin.org/articles/10.3389/fmats.2022.878455/full#supplementary-material
References
Aflori, M. (2021). Smart Nanomaterials for Biomedical Applications-A Review, Nanomaterials (Basel) 11, 20396. doi:10.3390/nano11020396
Ali, A., Shah, T., Ullah, R., Zhou, P., Guo, M., Ovais, M., et al. (2021). Review on Recent Progress in Magnetic Nanoparticles: Synthesis, Characterization, and Diverse Applications. Front. Chem. 9, 629054. doi:10.3389/fchem.2021.629054
Arsalani, N., Fattahi, H., Laurent, S., Burtea, C., Elst, L. V., and Muller, R. N. (2012). Polyglycerol-grafted Superparamagnetic Iron Oxide Nanoparticles: Highly Efficient MRI Contrast Agent for Liver and Kidney Imaging and Potential Scaffold for Cellular and Molecular Imaging. Contrast Media Mol. Imaging 7, 185–194. doi:10.1002/cmmi.479
Baghban, A., Jabbari, M., and Rahimpour, E. (2018). Fe3O4@Polydopamine Core-Shell Nanocomposite as a Sorbent for Efficient Removal of Rhodamine B from Aqueous Solutions: Kinetic and Equilibrium Studies. Iranian J. Chem. Chem. Engineering-International English Edition 37, 17–28. doi:10.30492/IJCCE.2018.26374
Barton, J., Gulka, M., Tarabek, J., Mindarava, Y., Wang, Z., Schimer, J., et al. (2020). Nanoscale Dynamic Readout of a Chemical Redox Process Using Radicals Coupled with Nitrogen-Vacancy Centers in Nanodiamonds. ACS Nano 14, 12938–12950. doi:10.1021/acsnano.0c04010
Basso, L., Cazzanelli, M., Orlandi, M., and Miotello, A. (2020). Nanodiamonds: Synthesis and Application in Sensing, Catalysis, and the Possible Connection with Some Processes Occurring in Space. Appl. Sci. 10, 10124094. doi:10.3390/app10124094
Black, K. C., Yi, J., Rivera, J. G., Zelasko-Leon, D. C., and Messersmith, P. B. (2013). Polydopamine-enabled Surface Functionalization of Gold Nanorods for Cancer Cell-Targeted Imaging and Photothermal Therapy. Nanomedicine 8, 17–28. doi:10.2217/nnm.12.82
Boudou, J.-P., David, M.-O., Joshi, V., Eidi, H., and Curmi, P. A. (2013). Hyperbranched Polyglycerol Modified Fluorescent Nanodiamond for Biomedical Research. Diamond Relat. Mater. 38, 131–138. doi:10.1016/j.diamond.2013.06.019
Brigger, I., Dubernet, C., and Couvreur, P. (2012). Nanoparticles in Cancer Therapy and Diagnosis. Adv. Drug Deliv. Rev. 64, 24–36. doi:10.1016/j.addr.2012.09.006
Chen, Z., Yuan, S.-J., Li, K., Zhang, Q., Li, T.-F., An, H.-C., et al. (2020). Doxorubicin-polyglycerol-nanodiamond Conjugates Disrupt STAT3/IL-6-mediated Reciprocal Activation Loop between Glioblastoma Cells and Astrocytes. J. Controlled Release 320, 469–483. doi:10.1016/j.jconrel.2020.01.044
Cheng, W., Liang, C., Xu, L., Liu, G., Gao, N., Tao, W., et al. (2017a). TPGS-functionalized Polydopamine-Modified Mesoporous Silica as Drug Nanocarriers for Enhanced Lung Cancer Chemotherapy against Multidrug Resistance. Small 13. doi:10.1002/smll.201700623
Cheng, W., Nie, J., Xu, L., Liang, C., Peng, Y., Liu, G., et al. (2017b). pH-Sensitive Delivery Vehicle Based on Folic Acid-Conjugated Polydopamine-Modified Mesoporous Silica Nanoparticles for Targeted Cancer Therapy. ACS Appl. Mater. Inter. 9, 18462–18473. doi:10.1021/acsami.7b02457
Cheng, Y., Zhang, S., Kang, N., Huang, J., Lv, X., Wen, K., et al. (2017c). Polydopamine-Coated Manganese Carbonate Nanoparticles for Amplified Magnetic Resonance Imaging-Guided Photothermal Therapy. ACS Appl. Mater. Inter. 9, 19296–19306. doi:10.1021/acsami.7b03087
Choi, C. K. K., Li, J., Wei, K., Xu, Y. J., Ho, L. W. C., Zhu, M., et al. (2015). A Gold@polydopamine Core-Shell Nanoprobe for Long-Term Intracellular Detection of microRNAs in Differentiating Stem Cells. J. Am. Chem. Soc. 137, 7337–7346. doi:10.1021/jacs.5b01457
Das, P., and Jana, N. R. (2014). Highly Colloidally Stable Hyperbranched Polyglycerol Grafted Red Fluorescent Silicon Nanoparticle as Bioimaging Probe. ACS Appl. Mater. Inter. 6, 4301–4309. doi:10.1021/am406061x
Davis, M. E., Chen, Z., and Shin, D. M. (2008). Nanoparticle Therapeutics: an Emerging Treatment Modality for Cancer. Nat. Rev. Drug Discov. 7, 771–782. doi:10.1038/nrd2614
De Berardis, B., Marchetti, M., Risuglia, A., Ietto, F., Fanizza, C., and Superti, F. (2021). Exposure to Airborne Gold Nanoparticles: a Review of Current Toxicological Data on the Respiratory Tract. J. Nanoparticle Res. 22, 9. doi:10.1007/s11051-020-04966-9
Degen, C. L., Reinhard, F., and Cappellaro, P. (2017). Quantum Sensing. Rev. Mod. Phys. 89. doi:10.1103/revmodphys.89.035002
Deng, X., Cao, S., Li, N., Wu, H., Smith, T. J., Zong, M., et al. (2016). A Magnetic Biocatalyst Based on Mussel-Inspired Polydopamine and its Acylation of Dihydromyricetin. Chin. J. Catal. 37, 584–595. doi:10.1016/s1872-2067(15)61045-2
Ding, Y. H., Floren, M., and Tan, W. (2016). Mussel-inspired Polydopamine for Bio-Surface Functionalization. Biosurface and Biotribology 2, 121–136. doi:10.1016/j.bsbt.2016.11.001
Dolmatov, V. Y., Ozerin, A. N., Kulakova, I. I., Bochechka, O. O., Lapchuk, N. M., Myllymäki, V., et al. (2020). Detonation Nanodiamonds: New Aspects in the Theory and Practice of Synthesis, Properties and Applications. Russ. Chem. Rev. 89, 1428–1462. doi:10.1070/rcr4924
Du, L., Qin, H., Ma, T., Zhang, T., and Xing, D. (2017). In Vivo Imaging-Guided Photothermal/Photoacoustic Synergistic Therapy with Bioorthogonal Metabolic Glycoengineering-Activated Tumor Targeting Nanoparticles. ACS Nano 11, 8930–8943. doi:10.1021/acsnano.7b03226
Elahi, N., Kamali, M., and Baghersad, M. H. (2018). Recent Biomedical Applications of Gold Nanoparticles: A Review. Talanta 184, 537–556. doi:10.1016/j.talanta.2018.02.088
Fu, Y., Yang, L., Zhang, J., Hu, J., Duan, G., Liu, X., et al. (2021). Polydopamine Antibacterial Materials. Mater. Horiz. 8, 1618–1633. doi:10.1039/d0mh01985b
Gruber, A., Dräbenstedt, A., Tietz, C., Fleury, L., Wrachtrup, J., and Borczyskowski, C. v. (1997). Scanning Confocal Optical Microscopy and Magnetic Resonance on Single Defect Centers. Science 276, 2012–2014. doi:10.1126/science.276.5321.2012
Harvey, S., Raabe, M., Ermakova, A., Wu, Y., Zapata, T., Chen, C., et al. (2019). Transferrin‐Coated Nanodiamond–Drug Conjugates for Milliwatt Photothermal Applications. Adv. Ther. 2. doi:10.1002/adtp.201900067
He, Y., Cheng, Z., Qin, Y., Xu, B., Ning, L., and Zhou, L. (2015). Facile Synthesis and Functionalization of Hyperbranched Polyglycerol Capped Magnetic Fe3O4 Nanoparticles for Efficient Dye Removal. Mater. Lett. 151, 100–103. doi:10.1016/j.matlet.2015.03.044
Holzinger, M., Le Goff, A., and Cosnier, S. (2014). Nanomaterials for Biosensing Applications: a Review. Front. Chem. 2, 63. doi:10.3389/fchem.2014.00063
Hsieh, F.-J., Sotoma, S., Lin, H.-H., Cheng, C.-Y., Yu, T.-Y., Hsieh, C.-L., et al. (2019). Bioorthogonal Fluorescent Nanodiamonds for Continuous Long-Term Imaging and Tracking of Membrane Proteins. ACS Appl. Mater. Inter. 11, 19774–19781. doi:10.1021/acsami.9b03640
Huang, H., Pierstorff, E., Liu, K., Ōsawa, E., and Ho, D. (2010). “Nanodiamond-Mediated Delivery of Therapeutics via Particle and Thin Film Architectures,” in Nanodiamonds., 151–174. doi:10.1007/978-1-4419-0531-4_7
Huang, X., Jain, P. K., El-Sayed, I. H., and El-Sayed, M. A. (2008). Plasmonic Photothermal Therapy (PPTT) Using Gold Nanoparticles. Lasers Med. Sci. 23, 217–228. doi:10.1007/s10103-007-0470-x
Hui, Y. Y., Cheng, C.-L., and Chang, H.-C. (2010). Nanodiamonds for Optical Bioimaging. J. Phys. D: Appl. Phys. 43. doi:10.1088/0022-3727/43/37/374021
Igarashi, R., Sugi, T., Sotoma, S., Genjo, T., Kumiya, Y., Walinda, E., et al. (2020). Tracking the 3D Rotational Dynamics in Nanoscopic Biological Systems. J. Am. Chem. Soc. 142, 7542–7554. doi:10.1021/jacs.0c01191
Jafari, M., Abolmaali, S. S., Najafi, H., and Tamaddon, A. M. (2020). Hyperbranched Polyglycerol Nanostructures for Anti-biofouling, Multifunctional Drug Delivery, Bioimaging and Theranostic Applications. Int. J. Pharmaceutics 576, 118959. doi:10.1016/j.ijpharm.2019.118959
Ju, K.-Y., Lee, S., Pyo, J., Choo, J., and Lee, J.-K. (2015). Bio-inspired Development of a Dual-Mode Nanoprobe for MRI and Raman Imaging. Small 11, 84–89. doi:10.1002/smll.201401611
Jung, H.-S., Cho, K.-J., Seol, Y., Takagi, Y., Dittmore, A., Roche, P. A., et al. (2018). Polydopamine Encapsulation of Fluorescent Nanodiamonds for Biomedical Applications. Advanced Functional Materials 28, 1801252. doi:10.1002/adfm.201801252
Kairdolf, B. A., Smith, A. M., Stokes, T. H., Wang, M. D., Young, A. N., and Nie, S. (2013). Semiconductor Quantum Dots for Bioimaging and Biodiagnostic Applications. Annu. Rev. Anal. Chem. 6, 143–162. doi:10.1146/annurev-anchem-060908-155136
Kalytchuk, S., Poláková, K., Wang, Y., Froning, J. P., Cepe, K., Rogach, A. L., et al. (2017). Carbon Dot Nanothermometry: Intracellular Photoluminescence Lifetime Thermal Sensing. ACS Nano 11, 1432–1442. doi:10.1021/acsnano.6b06670
Khan, M., and Huck, W. T. S. (2003). Hyperbranched Polyglycidol on Si/SiO2 Surfaces via Surface-Initiated Polymerization. Macromolecules 36, 5088–5093. doi:10.1021/ma0340762
Lai, G., Zhang, H., Yong, J., and Yu, A. (2013). In Situ deposition of Gold Nanoparticles on Polydopamine Functionalized Silica Nanosphere for Ultrasensitive Nonenzymatic Electrochemical Immunoassay. Biosens. Bioelectron. 47, 178–183. doi:10.1016/j.bios.2013.03.029
Landarani-Isfahani, A., Taheri-Kafrani, A., Amini, M., Mirkhani, V., Moghadam, M., Soozanipour, A., et al. (2015). Xylanase Immobilized on Novel Multifunctional Hyperbranched Polyglycerol-Grafted Magnetic Nanoparticles: An Efficient and Robust Biocatalyst. Langmuir 31, 9219–9227. doi:10.1021/acs.langmuir.5b02004
Lee, H., Dellatore, S. M., Miller, W. M., and Messersmith, P. B. (2007). Mussel-inspired Surface Chemistry for Multifunctional Coatings. Science 318, 426–430. doi:10.1126/science.1147241
Lei, W., Sun, C., Jiang, T., Gao, Y., Yang, Y., Zhao, Q., et al. (2019). Polydopamine-coated Mesoporous Silica Nanoparticles for Multi-Responsive Drug Delivery and Combined Chemo-Photothermal Therapy. Mater. Sci. Eng. C 105, 110103. doi:10.1016/j.msec.2019.110103
Li, D., Zhang, Y., Wen, S., Song, Y., Tang, Y., Zhu, X., et al. (2016a). Construction of Polydopamine-Coated Gold Nanostars for CT Imaging and Enhanced Photothermal Therapy of Tumors: an Innovative Theranostic Strategy. J. Mater. Chem. B 4, 4216–4226. doi:10.1039/c6tb00773b
Li, J., Fan, Q., Wu, Y., Wang, X., Chen, C., Tang, Z., et al. (2016b). Magnetic Polydopamine Decorated with Mg-Al LDH Nanoflakes as a Novel Bio-Based Adsorbent for Simultaneous Removal of Potentially Toxic Metals and Anionic Dyes. J. Mater. Chem. A. 4, 1737–1746. doi:10.1039/c5ta09132b
Li, S., Guo, Z., Feng, R., Zhang, Y., Xue, W., and Liu, Z. (2017). Hyperbranched Polyglycerol Conjugated Fluorescent Carbon Dots with Improved In Vitro Toxicity and Red Blood Cell Compatibility for Bioimaging. RSC Adv. 7, 4975–4982. doi:10.1039/c6ra27159f
Li, T.-F., Li, K., Zhang, Q., Wang, C., Yue, Y., Chen, Z., et al. (2018). Dendritic Cell-Mediated Delivery of Doxorubicin-Polyglycerol-Nanodiamond Composites Elicits Enhanced Anti-cancer Immune Response in Glioblastoma. Biomaterials 181, 35–52. doi:10.1016/j.biomaterials.2018.07.035
Li, T.-F., Xu, Y.-H., Li, K., Wang, C., Liu, X., Yue, Y., et al. (2019). Doxorubicin-polyglycerol-nanodiamond Composites Stimulate Glioblastoma Cell Immunogenicity through Activation of Autophagy. Acta Biomater. 86, 381–394. doi:10.1016/j.actbio.2019.01.020
Liebscher, J., Mrówczyński, R., Scheidt, H. A., Filip, C., Hădade, N. D., Turcu, R., et al. (2013). Structure of Polydopamine: a Never-Ending story? Langmuir 29, 10539–10548. doi:10.1021/la4020288
Lin, L.-S., Cong, Z.-X., Cao, J.-B., Ke, K.-M., Peng, Q.-L., Gao, J., et al. (2014). Multifunctional Fe3O4@Polydopamine Core-Shell Nanocomposites for Intracellular mRNA Detection and Imaging-Guided Photothermal Therapy. ACS Nano 8, 3876–3883. doi:10.1021/nn500722y
Liu, R., Guo, Y., Odusote, G., Qu, F., and Priestley, R. D. (2013a). Core-Shell Fe3O4 Polydopamine Nanoparticles Serve Multipurpose as Drug Carrier, Catalyst Support and Carbon Adsorbent. ACS Appl. Mater. Inter. 5, 9167–9171. doi:10.1021/am402585y
Liu, S., Qileng, A., Huang, J., Gao, Q., and Liu, Y. (2017). Polydopamine as a Bridge to Decorate Monodisperse Gold Nanoparticles on Fe3O4 Nanoclusters for the Catalytic Reduction of 4-nitrophenol. RSC Adv. 7, 45545–45551. doi:10.1039/c7ra09373j
Liu, X., Cao, J., Li, H., Li, J., Jin, Q., Ren, K., et al. (2013b). Mussel-inspired Polydopamine: a Biocompatible and Ultrastable Coating for Nanoparticles In Vivo. ACS Nano 7, 9384–9395. doi:10.1021/nn404117j
Liu, Y., Ai, K., Liu, J., Deng, M., He, Y., and Lu, L. (2013c). Dopamine-melanin Colloidal Nanospheres: an Efficient Near-Infrared Photothermal Therapeutic Agent for In Vivo Cancer Therapy. Adv. Mater. 25, 1353–1359. doi:10.1002/adma.201204683
Liu, Y., Ai, K., and Lu, L. (2014). Polydopamine and its Derivative Materials: Synthesis and Promising Applications in Energy, Environmental, and Biomedical fields. Chem. Rev. 114, 5057–5115. doi:10.1021/cr400407a
Lu, J., Fang, J., Li, J., Wang, C., He, Z., Zhu, L., et al. (2020). Polydopamine Nanotubes Decorated with Ag Nanoparticles as Catalyst for the Reduction of Methylene Blue. ACS Appl. Nano Mater. 3, 156–164. doi:10.1021/acsanm.9b01861
Maiti, D., Tong, X., Mou, X., and Yang, K. (2018). Carbon-Based Nanomaterials for Biomedical Applications: A Recent Study. Front. Pharmacol. 9, 1401. doi:10.3389/fphar.2018.01401
Materón, E. M., Miyazaki, C. M., Carr, O., Joshi, N., Picciani, P. H. S., Dalmaschio, C. J., et al. (2021). Magnetic Nanoparticles in Biomedical Applications: A Review. Appl. Surf. Sci. Adv. 6, 100163. doi:10.1016/j.apsadv.2021.100163
Mcnamara, K., and Tofail, S. A. M. (2016). Nanoparticles in Biomedical Applications. Adv. Phys. X 2, 54–88. doi:10.1080/23746149.2016.1254570
Medintz, I. L., Uyeda, H. T., Goldman, E. R., and Mattoussi, H. (2005). Quantum Dot Bioconjugates for Imaging, Labelling and Sensing. Nat. Mater 4, 435–446. doi:10.1038/nmat1390
Mitchell, M. J., Billingsley, M. M., Haley, R. M., Wechsler, M. E., Peppas, N. A., and Langer, R. (2021). Engineering Precision Nanoparticles for Drug Delivery. Nat. Rev. Drug Discov. 20, 101–124. doi:10.1038/s41573-020-0090-8
Mochalin, V. N., Shenderova, O., Ho, D., and Gogotsi, Y. (2011). The Properties and Applications of Nanodiamonds. Nat. Nanotech 7, 11–23. doi:10.1038/nnano.2011.209
Moritz, M., and Geszke-Moritz, M. (2015). Mesoporous Materials as Multifunctional Tools in Biosciences: Principles and Applications. Mater. Sci. Eng. C 49, 114–151. doi:10.1016/j.msec.2014.12.079
Narayan, R., Nayak, U. Y., Raichur, A. M., and Garg, S. (2018). Mesoporous Silica Nanoparticles: A Comprehensive Review on Synthesis and Recent Advances. Pharmaceutics 10. doi:10.3390/pharmaceutics10030118
Niculescu, V.-C. (2020). Mesoporous Silica Nanoparticles for Bio-Applications. Front. Mater. 7, 36. doi:10.3389/fmats.2020.00036
Nikoobakht, B., and El-Sayed, M. A. (2003). Preparation and Growth Mechanism of Gold Nanorods (NRs) Using Seed-Mediated Growth Method. Chem. Mater. 15, 1957–1962. doi:10.1021/cm020732l
Panja, P., Das, P., Mandal, K., and Jana, N. R. (2017). Hyperbranched Polyglycerol Grafting on the Surface of Silica-Coated Nanoparticles for High Colloidal Stability and Low Nonspecific Interaction. ACS Sustain. Chem. Eng. 5, 4879–4889. doi:10.1021/acssuschemeng.7b00292
Qin, H., Maruyama, K., Amano, T., Murakami, T., and Komatsu, N. (2016). Hyperbranched Polyglycerol-Grafted Titanium Oxide Nanoparticles: Synthesis, Derivatization, Characterization, Size Separation, and Toxicology. Mater. Res. Express 3, 5049. doi:10.1088/2053-1591/3/10/105049
Riley, R. S., and Day, E. S. (2017). Gold Nanoparticle-Mediated Photothermal Therapy: Applications and Opportunities for Multimodal Cancer Treatment. Wiley Interdiscip. Rev. Nanomed Nanobiotechnol 9, 1449. doi:10.1002/wnan.1449
Runowski, M., Sobczak, S., Marciniak, J., Bukalska, I., Lis, S., and Katrusiak, A. (2019). Gold Nanorods as a High-Pressure Sensor of Phase Transitions and Refractive-index Gauge. Nanoscale 11, 8718–8726. doi:10.1039/c9nr02792k
Sandler, S. R., and Berg, F. R. (1966). Room Temperature Polymerization of Glycidol. J. Polym. Sci. A-1 Polym. Chem. 4, 1253–1259. doi:10.1002/pol.1966.150040523
Shi, M., Zhang, J., Li, J., Fan, Y., Wang, J., Sun, W., et al. (2019). Polydopamine-coated Magnetic Mesoporous Silica Nanoparticles for Multimodal Cancer Theranostics. J. Mater. Chem. B 7, 368–372. doi:10.1039/c8tb03021a
Shi, Y., Van Der Meel, R., Chen, X., and Lammers, T. (2020). The EPR Effect and beyond: Strategies to Improve Tumor Targeting and Cancer Nanomedicine Treatment Efficacy. Theranostics 10, 7921–7924. doi:10.7150/thno.49577
Sotoma, S., and Harada, Y. (2019). Polydopamine Coating as a Scaffold for Ring-Opening Chemistry to Functionalize Gold Nanoparticles. Langmuir 35, 8357–8362. doi:10.1021/acs.langmuir.9b00762
Sotoma, S., Hsieh, F. J., and Chang, H. C. (2018). Single-Step Metal-free Grafting of Cationic Polymer Brushes on Fluorescent Nanodiamonds. Materials (Basel) 11, 1479. doi:10.3390/ma11081479
Sotoma, S., Iimura, J., Igarashi, R., Hirosawa, K. M., Ohnishi, H., Mizukami, S., et al. (2016). Selective Labeling of Proteins on Living Cell Membranes Using Fluorescent Nanodiamond Probes. Nanomaterials (Basel) 6, 56. doi:10.3390/nano6040056
Sotoma, S., Zhong, C., Kah, J. C. Y., Yamashita, H., Plakhotnik, T., Harada, Y., et al. (2021). In Situ measurements of Intracellular thermal Conductivity Using Heater-Thermometer Hybrid diamond Nanosensors. Sci. Adv. 7, eabd7888. doi:10.1126/sciadv.abd7888
Sotoma, S., and Harada, Y. (2021). Composite Quantum Sensors Based on Fluorescent Nanodiamonds for Intracellular Controlled Heating in Living Cells. ACS Appl. Nano Mater. 4, 3969–3976. doi:10.1021/acsanm.1c00334
Sotoma, S., Igarashi, R., Iimura, J., Kumiya, Y., Tochio, H., Harada, Y., et al. (2015). Suppression of Nonspecific Protein-Nanodiamond Adsorption Enabling Specific Targeting of Nanodiamonds to Biomolecules of Interest. Chem. Lett. 44, 354–356. doi:10.1246/cl.141036
Sotoma, S., and Shirakawa, M. (2016). Monodispersed Colloidal Solutions of Surface-Modified Detonation-Synthesized Nanodiamonds and Their Aggregation Resistance. Chem. Lett. 45, 697–699. doi:10.1246/cl.160250
Suarez-Kelly, L. P., Sun, S. H., Ren, C., Rampersaud, I. V., Albertson, D., Duggan, M. C., et al. (2021). Antibody Conjugation of Fluorescent Nanodiamonds for Targeted Innate Immune Cell Activation. ACS Appl. Nano Mater. 4, 3122–3139. doi:10.1021/acsanm.1c00256
Tang, Y., Tan, Y., Lin, K., and Zhu, M. (2021). Research Progress on Polydopamine Nanoparticles for Tissue Engineering. Front. Chem. 9, 727123. doi:10.3389/fchem.2021.727123
Terada, D., Sotoma, S., Harada, Y., Igarashi, R., and Shirakawa, M. (2018). One-Pot Synthesis of Highly Dispersible Fluorescent Nanodiamonds for Bioconjugation. Bioconjug. Chem. 29, 2786–2792. doi:10.1021/acs.bioconjchem.8b00412
Torelli, M. D., Rickard, A. G., Backer, M. V., Filonov, D. S., Nunn, N. A., Kinev, A. V., et al. (2019). Targeting Fluorescent Nanodiamonds to Vascular Endothelial Growth Factor Receptors in Tumor. Bioconjug. Chem. 30, 604. doi:10.1021/acs.bioconjchem.8b00803
Wang, C., Zhou, J., Wang, P., He, W., and Duan, H. (2016a). Robust Nanoparticle-DNA Conjugates Based on Mussel-Inspired Polydopamine Coating for Cell Imaging and Tailored Self-Assembly. Bioconjug. Chem. 27, 815–823. doi:10.1021/acs.bioconjchem.6b00021
Wang, L., Neoh, K. G., Kang, E.-T., and Shuter, B. (2011). Multifunctional Polyglycerol-Grafted Fe3O4@SiO2 Nanoparticles for Targeting Ovarian Cancer Cells. Biomaterials 32, 2166–2173. doi:10.1016/j.biomaterials.2010.11.042
Wang, L., Neoh, K. G., Kang, E. T., Shuter, B., and Wang, S.-C. (2009). Superparamagnetic Hyperbranched Polyglycerol-Grafted Fe3O4Nanoparticles as a Novel Magnetic Resonance Imaging Contrast Agent: An In Vitro Assessment. Adv. Funct. Mater. 19, 2615–2622. doi:10.1002/adfm.200801689
Wang, S., Zhao, X., Wang, S., Qian, J., and He, S. (2016b). Biologically Inspired Polydopamine Capped Gold Nanorods for Drug Delivery and Light-Mediated Cancer Therapy. ACS Appl. Mater. Inter. 8, 24368–24384. doi:10.1021/acsami.6b05907
Wang, S., Zhou, Y., Yang, S., and Ding, B. (2008). Growing Hyperbranched Polyglycerols on Magnetic Nanoparticles to Resist Nonspecific Adsorption of Proteins. Colloids Surf. B: Biointerfaces 67, 122–126. doi:10.1016/j.colsurfb.2008.08.009
Wei, Y., Gao, L., Wang, L., Shi, L., Wei, E., Zhou, B., et al. (2017). Polydopamine and Peptide Decorated Doxorubicin-Loaded Mesoporous Silica Nanoparticles as a Targeted Drug Delivery System for Bladder Cancer Therapy. Drug Deliv. 24, 681–691. doi:10.1080/10717544.2017.1309475
Wu, Y., Jelezko, F., Plenio, M. B., and Weil, T. (2016). Diamond Quantum Devices in Biology. Angew. Chem. Int. Ed. 55, 6586–6598. doi:10.1002/anie.201506556
Xia, Z., Lin, Z., Xiao, Y., Wang, L., Zheng, J., Yang, H., et al. (2013). Facile Synthesis of Polydopamine-Coated Molecularly Imprinted Silica Nanoparticles for Protein Recognition and Separation. Biosens. Bioelectron. 47, 120–126. doi:10.1016/j.bios.2013.03.024
Xie, Y., Yan, B., Xu, H., Chen, J., Liu, Q., Deng, Y., et al. (2014). Highly Regenerable Mussel-Inspired Fe3O4@Polydopamine-Ag Core-Shell Microspheres as Catalyst and Adsorbent for Methylene Blue Removal. ACS Appl. Mater. Inter. 6, 8845–8852. doi:10.1021/am501632f
Xu, S., Zhang, G., Fang, B., Xiong, Q., Duan, H., and Lai, W. (2019). Lateral Flow Immunoassay Based on Polydopamine-Coated Gold Nanoparticles for the Sensitive Detection of Zearalenone in Maize. ACS Appl. Mater. Inter. 11, 31283–31290. doi:10.1021/acsami.9b08789
Xue, P., Sun, L., Li, Q., Zhang, L., Guo, J., Xu, Z., et al. (2017). PEGylated Polydopamine-Coated Magnetic Nanoparticles for Combined Targeted Chemotherapy and Photothermal Ablation of Tumour Cells. Colloids Surf. B: Biointerfaces 160, 11–21. doi:10.1016/j.colsurfb.2017.09.012
Yang, P., Zhu, F., Zhang, Z., Cheng, Y., Wang, Z., and Li, Y. (2021). Stimuli-responsive Polydopamine-Based Smart Materials. Chem. Soc. Rev. 50, 8319–8343. doi:10.1039/d1cs00374g
Yang, Y., Wang, L., Wan, B., Gu, Y., and Li, X. (2019). Optically Active Nanomaterials for Bioimaging and Targeted Therapy. Front. Bioeng. Biotechnol. 7, 320. doi:10.3389/fbioe.2019.00320
Yeh, Y.-C., Creran, B., and Rotello, V. M. (2012). Gold Nanoparticles: Preparation, Properties, and Applications in Bionanotechnology. Nanoscale 4, 1871–1880. doi:10.1039/c1nr11188d
Yoshino, F., Amano, T., Zou, Y., Xu, J., Kimura, F., Furusho, Y., et al. (2019). Preferential Tumor Accumulation of Polyglycerol Functionalized Nanodiamond Conjugated with Cyanine Dye Leading to Near-Infrared Fluorescence In Vivo Tumor Imaging. Small 15, e1901930. doi:10.1002/smll.201901930
You, Y.-H., Lin, Y.-F., Nirosha, B., Chang, H.-T., and Huang, Y.-F. (2019). Polydopamine-coated Gold Nanostar for Combined Antitumor and Antiangiogenic Therapy in Multidrug-Resistant Breast Cancer. Nanotheranostics 3, 266–283. doi:10.7150/ntno.36842
Yu, S.-J., Kang, M.-W., Chang, H.-C., Chen, K.-M., and Yu, Y.-C. (2005). Bright Fluorescent Nanodiamonds: No Photobleaching and Low Cytotoxicity. J. Am. Chem. Soc. 127, 17604–17605. doi:10.1021/ja0567081
Yuan, S.-J., Xu, Y.-H., Wang, C., An, H.-C., Xu, H.-Z., Li, K., et al. (2019). Doxorubicin-polyglycerol-nanodiamond Conjugate Is a Cytostatic Agent that Evades Chemoresistance and Reverses Cancer-Induced Immunosuppression in Triple-Negative Breast Cancer. J. Nanobiotechnol 17, 110. doi:10.1186/s12951-019-0541-8
Zelasko-Leon, D. C., Fuentes, C. M., and Messersmith, P. B. (2015). MUC1-Targeted Cancer Cell Photothermal Ablation Using Bioinspired Gold Nanorods. PLoS One 10, e0128756. doi:10.1371/journal.pone.0128756
Zeng, Y., Liu, W., Wang, Z., Singamaneni, S., and Wang, R. (2018). Multifunctional Surface Modification of Nanodiamonds Based on Dopamine Polymerization. Langmuir 34, 4036–4042. doi:10.1021/acs.langmuir.8b00509
Zhang, L., Su, H., Cai, J., Cheng, D., Ma, Y., Zhang, J., et al. (2016). A Multifunctional Platform for Tumor Angiogenesis-Targeted Chemo-Thermal Therapy Using Polydopamine-Coated Gold Nanorods. ACS Nano 10, 10404–10417. doi:10.1021/acsnano.6b06267
Zhang, M., Zhang, X., He, X., Chen, L., and Zhang, Y. (2012). A Self-Assembled Polydopamine Film on the Surface of Magnetic Nanoparticles for Specific Capture of Protein. Nanoscale 4, 3141–3147. doi:10.1039/c2nr30316g
Zhang, T., Pramanik, G., Zhang, K., Gulka, M., Wang, L., Jing, J., et al. (2021). Toward Quantitative Bio-Sensing with Nitrogen–Vacancy Center in Diamond. ACS Sensors.
Zhang, X.-Q., Chen, M., Lam, R., Xu, X., Osawa, E., and Ho, D. (2009). Polymer-functionalized Nanodiamond Platforms as Vehicles for Gene Delivery. ACS Nano 3, 2609–2616. doi:10.1021/nn900865g
Zhao, L., Chano, T., Morikawa, S., Saito, Y., Shiino, A., Shimizu, S., et al. (2012). Hyperbranched Polyglycerol-Grafted Superparamagnetic Iron Oxide Nanoparticles: Synthesis, Characterization, Functionalization, Size Separation, Magnetic Properties, and Biological Applications. Adv. Funct. Mater. 22, 5107–5117. doi:10.1002/adfm.201201060
Zhao, L., Nakae, Y., Qin, H., Ito, T., Kimura, T., Kojima, H., et al. (2014a). Polyglycerol-functionalized Nanodiamond as a Platform for Gene Delivery: Derivatization, Characterization, and Hybridization with DNA. Beilstein J. Org. Chem. 10, 707–713. doi:10.3762/bjoc.10.64
Zhao, L., Shiino, A., Qin, H., Kimura, T., and Komatsu, N. (2015a). Synthesis, Characterization, and Magnetic Resonance Evaluation of Polyglycerol-Functionalized Detonation Nanodiamond Conjugated with Gadolinium(III) Complex. j nanosci nanotechnol 15, 1076–1082. doi:10.1166/jnn.2015.9738
Zhao, L., Takimoto, T., Ito, M., Kitagawa, N., Kimura, T., and Komatsu, N. (2011). Chromatographic Separation of Highly Soluble diamond Nanoparticles Prepared by Polyglycerol Grafting. Angew. Chem. Int. Ed. 50, 1388–1392. doi:10.1002/anie.201006310
Zhao, L., Xu, Y.-H., Akasaka, T., Abe, S., Komatsu, N., Watari, F., et al. (2014c). Polyglycerol-coated Nanodiamond as a Macrophage-Evading Platform for Selective Drug Delivery in Cancer Cells. Biomaterials 35, 5393–5406. doi:10.1016/j.biomaterials.2014.03.041
Zhao, L., Xu, Y.-H., Qin, H., Abe, S., Akasaka, T., Chano, T., et al. (2014b). Platinum on Nanodiamond: A Promising Prodrug Conjugated with Stealth Polyglycerol, Targeting Peptide and Acid-Responsive Antitumor Drug. Adv. Funct. Mater. 24, 5348–5357. doi:10.1002/adfm.201304298
Zhao, Y., Yeh, Y., Liu, R., You, J., and Qu, F. (2015b). Facile Deposition of Gold Nanoparticles on Core-Shell Fe3O4@polydopamine as Recyclable Nanocatalyst. Solid State. Sci. 45, 9–14. doi:10.1016/j.solidstatesciences.2015.04.010
Zheng, D., Huang, C., Zhu, X., Huang, H., and Xu, C. (2021). Performance of Polydopamine Complex and Mechanisms in Wound Healing. Int. J. Mol. Sci. 22. doi:10.3390/ijms221910563
Zheng, Q., Lin, T., Wu, H., Guo, L., Ye, P., Hao, Y., et al. (2014). Mussel-inspired Polydopamine Coated Mesoporous Silica Nanoparticles as pH-Sensitive Nanocarriers for Controlled Release. Int. J. Pharmaceutics 463, 22–26. doi:10.1016/j.ijpharm.2013.12.045
Zheng, R., Wang, S., Tian, Y., Jiang, X., Fu, D., Shen, S., et al. (2015). Polydopamine-Coated Magnetic Composite Particles with an Enhanced Photothermal Effect. ACS Appl. Mater. Inter. 7, 15876–15884. doi:10.1021/acsami.5b03201
Zhou, L., Gao, C., Xu, W., Wang, X., and Xu, Y. (2009). Enhanced Biocompatibility and Biostability of CdTe Quantum Dots by Facile Surface-Initiated Dendritic Polymerization. Biomacromolecules 10, 1865–1874. doi:10.1021/bm9002877
Zoppe, J. O., Ataman, N. C., Mocny, P., Wang, J., Moraes, J., and Klok, H.-A. (2017). Surface-Initiated Controlled Radical Polymerization: State-Of-The-Art, Opportunities, and Challenges in Surface and Interface Engineering with Polymer Brushes. Chem. Rev. 117, 1105–1318. doi:10.1021/acs.chemrev.6b00314
Zou, Y., Ito, S., Yoshino, F., Suzuki, Y., Zhao, L., and Komatsu, N. (2020). Polyglycerol Grafting Shields Nanoparticles from Protein Corona Formation to Avoid Macrophage Uptake. ACS Nano 14, 7216–7226. doi:10.1021/acsnano.0c02289
Zou, Y., Nishikawa, M., Kang, H. G., Cheng, G., Wang, W., Wang, Y., et al. (2021). Effect of Protein Corona on Mitochondrial Targeting Ability and Cytotoxicity of Triphenylphosphonium Conjugated with Polyglycerol-Functionalized Nanodiamond. Mol. Pharmaceutics 18, 2823–2832. doi:10.1021/acs.molpharmaceut.1c00188
Keywords: imaging, nanoparticle, polydopamine, polyglycerol, sensing, targeting, therapy
Citation: Sotoma S (2022) Polyglycerol/Polydopamine-Coated Nanoparticles for Biomedical Applications. Front. Mater. 9:878455. doi: 10.3389/fmats.2022.878455
Received: 18 February 2022; Accepted: 17 March 2022;
Published: 11 April 2022.
Edited by:
Guilherme Mariz de Oliveira Barra, Federal University of Santa Catarina, BrazilCopyright © 2022 Sotoma. This is an open-access article distributed under the terms of the Creative Commons Attribution License (CC BY). The use, distribution or reproduction in other forums is permitted, provided the original author(s) and the copyright owner(s) are credited and that the original publication in this journal is cited, in accordance with accepted academic practice. No use, distribution or reproduction is permitted which does not comply with these terms.
*Correspondence: Shingo Sotoma, ssotoma@protein.osaka-u.ac.jp, shingo.sotoma@gmail.com