Antimicrobial effect of fungal melanin in combination with plant oils for the treatment of wood
- 1Cellulose & Wood Materials Laboratory, Empa, Dübendorf, Switzerland
- 2ETH, Institute for Building Materials, Wood Material Science, Zurich, Switzerland
Microbial deterioration of wood has gradually destroyed precious historic musical instruments. The Serpentino (English: serpent/little snake) is a wind instrument used for over 400 years and is considered the godfather of modern instruments such as the saxophone and the tuba. Many original instruments have been totally destroyed due to colonization and degradation by bacteria and fungi. Reconstructing the serpent in particular and other ancient instruments in general requires the preservation of wood against bio-deterioration. Conventional chemical preservatives based on copper and boron compounds have been used in the wood processing industry; however, they are not ideal for protecting musical instruments and may pose a negative impact on the health and safety of musicians with direct mouth contact. Non-biocidal preservatives, such as plant oils, have attracted a lot of attention. Herein, we report the antimicrobial efficacy of plant oils, alone and in combination with fungal melanin, for protecting walnut wood that is used to make the serpent, against oral bacteria and wood-decay fungi. Linseed and tea tree oils were found to have a strong antibacterial effect, reducing the survival rate of Streptococcus mutans, a bacterium commonly found in human saliva, to below 1%. Impregnation of wood with plant oils and melanin significantly increased the antibacterial effect when compared to that of the oil(s) alone. A satisfactory antifungal effect was also achieved after 2 weeks as indicated by a reduction (<10%) in the survival rate of Chaetomium globosum on the radial longitudinal wood surface. In addition, the treated wood samples absorbed less water than the untreated wood, and maintained their dimensions at different humidity conditions, highlighting the potential of this approach for wood protection.
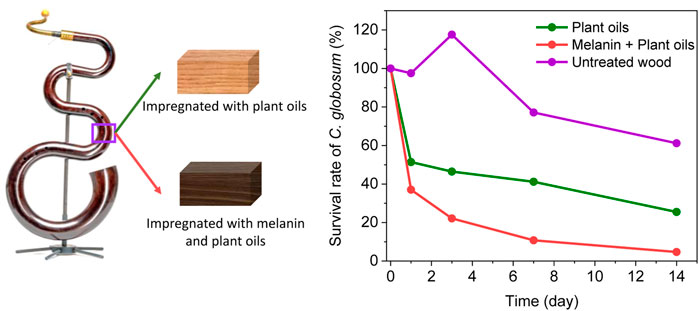
GRAPHICAL ABSTRACT | SYNOPSIS. Melanin boosts the antimicrobial effect of plant oils for wood preservation.
Introduction
Wood used in historical buildings, ancient artworks, or antique furniture is of great importance to human cultural heritage, as it provides valuable information about the past. However, wood is susceptible to various bio-deteriorating agents, which makes restoration and preservation of historical objects and features a significant and complicated process. In this work, the reconstruction of the Serpentino (in English: serpent/little snake), an ancient musical instrument used from the end of the 16th century to the middle of the 19th century, was studied (Holman, 2017). This wind instrument was played in churches to support singing, as it covers the vocal registers of the human voice and can “carry” a choir (Six, 2021).
In the 19th century, the serpent was replaced by less fragile, easier to manufacture, and more sophisticated to play brass instruments such as the ophicleide and the tuba (Palmer, 1990). However, the trend toward historically based performances has led to the renaissance of the serpent, along with other historical instruments.
In 2008, the Swiss serpent maker, Stephan Berger, developed a new processing method for the production of the serpent, in order to counteract the technical inadequacies of the traditional instruments and to produce sound-perfect and robust instruments. However, the snake shape of the instrument (Figure 1) made of walnut (Juglans regia L.) wood creates not only an incomparable sound but also ideal conditions for wood deterioration. Water condensation from the musician’s breath creates a humid microclimate within the tube, promoting the growth of wood decay fungi and bacteria. This led to the decomposition of century-old instruments, with the last original specimens gradually being destroyed (Figure 1). To the best of our knowledge, no studies have investigated the microorganisms that colonize the serpent and cause wood decay.
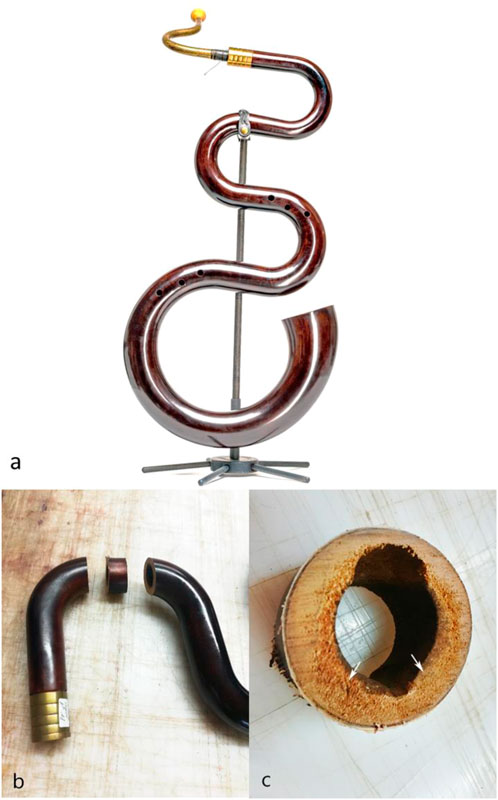
FIGURE 1. (A) Snake-like shape of the serpent helps to position the finger holes in reach. (B) European walnut wood samples showing distinctive interior decay were sawn (circle) from the wooden conical tube. (C) Isolations were made from wood chips extracted from the decayed wood (arrows) and placed onto petri dishes with different growth media. Microorganisms were incubated at 25°C, 50% RH (fungus) and at 23°C, 95% RH (bacteria).
Water-borne or oil-borne chemical preservatives are used to inhibit wood decay. However, the preservation of wood instruments such as the serpent with wood preservatives is challenging since active substances, for example, metal salts such as copper sulfate or zinc borate, although normally considered to have low mammalian toxicity, may pose a negative impact on health and safety of musicians with direct mouth contact (Jorge et al., 2004; Vallero et al., 2010). The interior tube of serpents is traditionally treated with linseed/flaxseed (Linum usitatissimum L.) oil. Linseed oil is an inexpensive, biodegradable, and nontoxic vegetable oil with antimicrobial properties, which has recently attained interest in the manufacturing of coatings (Echard et al., 2010; Lu and Chang, 2020; Vidholdová et al., 2021). Humar and Lesar (2013) demonstrated that linseed oil was an effective substitute for the biocidal treatment of wood in less hazardous applications. Not only did linseed oil prove effective against wood decay fungi but it also reduced short-, medium-, and long-term water uptake. Panov and Terziev (2015) showed that epoxidized linseed oil was an efficient hydrophobic agent in combination with biocides, forming a suitable protective formulation for wood in both above- and in-soil exposure. Nevertheless, the use of linseed oil alone for the treatment of serpents will not prevent the wood from colonization and biodeterioration by wood-decay fungi and bacteria.
We investigated the efficacy of environmentally friendly plant oils in combination with melanin, a bio-derived polymer, for the protection of serpent wood. Melanin can be produced using Armillaria species, which are long-lived and are among the largest living organisms in the world. In Oregon, the upper ground layer was colonized over an area of about 9 km2 by only one mycelial clone of A. ostoyae with a supposed age of 2,400 years (Smith et al., 1992). These widespread wood-decomposing fungi spread in the soil by melanized rhizomorphs. High amounts of incorporated melanin in the rhizomorphs can bind heavy metals (Rigling et al., 2006) and protect them from environmental stresses, such as UV radiation, elevated temperatures, antimicrobial agents, and competition by antagonistic fungi and bacteria (Tran-Ly et al., 2020a). We recently developed a simple and inexpensive method to synthesize and utilize melanin produced by A. cepistipes (Ribera et al., 2019) for a range of industrial applications (Tran-Ly et al., 2020b; Tran-Ly et al., 2021).
Herein, we show that the combination of plant oils and melanin is an effective method to protect the wood of serpents against deterioration. For this purpose, bacteria and fungi from naturally infected serpents were first isolated and used to infect walnut wood artificially. From practical experience, wood of the serpent is infected by soft rot fungi. Traditional studies such as ENV 807 or other laboratory methods to measure mass loss of wood by soft rot fungi are extremely time-consuming. Moreover, as the wood of the serpent is initially colonized via spores of soft rot fungi, we decided to use a short-term survival test and expose the oil–melanin-treated and oil–melanin-untreated wood surfaces to the high concentration of spores or bacterial cells.
We conducted a number of bioassays on the efficacy of melanin-based coatings against the casual wood decay agents. This work highlights the potential use of the combination of melanin and plant oils as a simple and environmentally benign method for wood preservation.
Materials and methods
Isolation and identification of the casual decay microorganisms from wood
The microorganisms were isolated from a serpent no.18 made in January–February 2013 by Stephan Berger from walnut. In 2019, initial signs of internal decay were detected (Figure 1B). A wood sample containing decayed internal areas, approximately 5 cm × 2 cm (diameter × thickness), was excised from the serpent tube as indicated in Figure 1C. The extracted wood sample was lightly sprayed with ethanol to disinfect the exposed surface. Then, under sterile conditions, the sample was carefully broken across the decayed area, to expose a surface free of any potential surface contaminants. Using a sterile scalpel, wood samples approximately 5 × 5 mm in dimension were excised from the freshly exposed wood surface. The samples were then placed with sterile forceps on 2% MEA (Malt Extract Agar, OXOID) for the isolation of deuteromycetes and ascomycetes, or in addition with 20 ml of 2% MEA with 2 ml of thiabendazole dissolved in lactic acid for basidiomycetes (Merck, Darmstadt, Germany) (Sieber, 1995). This procedure was repeated ten times per growth medium. The petri dishes were sealed with parafilm and maintained in the dark at 25°C and 65% relative humidity (RH), and growth was monitored daily. The successful isolations were then maintained on a cellulose–mineral–agar medium containing NaNO3 (3.0 g), K2HPO4 (1.0 g), MSO4 × 7 H2O (0.25 g), KCl (0.25 g), bacteriological agar (10.0 g), and distilled H2O (1,000.0 g). As cellulose source, sterile, transparent, cellophane pieces about 30 µm thick with dimensions of 1 × 1 cm were placed onto the growth media. After colonization by the fungus, the cellophane square was lifted off the growth medium and placed on a glass slide with lactophenol cotton blue, which is commonly used for the microscopic examination and identification of fungi. All cultures were identified microscopically using the keys of Stalpers (1978) for basidiomycetes (Stalpers, 1978) and that of Barnett and Hunter (1998) for deuteromycetes (Barnett and Hunter, 1998).
Bacterial strains used
For the antibacterial assays, three bacterial strains were used: Staphylococcus arlettae Rosenbach (Gram positive), Pseudomonas fluorescens Migula (Gram negative), and a common oral bacterium, Streptococcus mutans Clarke ATCC 25175 (Gram positive). Pure cultures of S. arlettae and P. fluorescens were provided by Swissatest AG, St. Gallen, Switzerland. S. mutans was isolated from the surface of walnut wood samples that were exposed to human saliva. All selected bacterial species were cultivated on Tryptic Soy Agar (TSA, OXOID) and subsequently sub-cultured in Tryptic Soy Broth (TSB, OXOID) at 30°C or 35°C.
Light microscopic observation of naturally infected walnut wood from a serpent
Naturally, decayed walnut wood samples from a serpent (Figure 1) approx. 20 × 5 × 5 mm were excised. For light microscopy, the infected wood was cut into smaller wood blocks (10 × 5 × 5 mm).
The specimens, with transverse, radial, and tangential faces exposed for examination, were fixed in 2% glutaraldehyde buffered at pH 7.2–7.4, dehydrated with acetone, embedded in a methacrylate medium, and subsequently polymerized at 50 °C. The embedded specimens were sectioned at approximately 2 and 3 µm using a rotary microtome (Leica® 2040 Supercut) (Reichert-Jung/Leica 2040, Jung/Leica Instruments GmbH, near Nussloch bei Heidelberg, Germany) fitted with a diamond knife. For general observation of wood anatomy, sections were stained for 12 h in safranine and then counter-stained for 3 min in methylene blue and 30 min in auramine (Schwarze, 2007). Color micrographs (Kodak EPY 64T) were taken with a Leica DM4000 B LED microscope using LAS software version 4.13.0.
Antimicrobial substances
Linseed (Linum usitatissimum L.) oil, tea tree (Melaleuca alternifolia (Maiden & Betche) Cheel) oil, and eucalyptus (Eucalyptus citriodora (Hook.) (K.D.Hill and L.A.S.Johnson)) oil were used for wood impregnation, obtained from Drogerie Spillmann AG, Dübendorf, Switzerland. All oils were used with their original concentration (purity ≥ 99%) for the antimicrobial tests.
Fungal melanin was biosynthesized via the cultivation of Armillaria cepistipes Velen (Empa 665) according to Ribera et al. (2019). Melanin was prepared with a concentration of 0.25 g/L, by diluting the originally collected melanin solution from a culture with Mili-Q water. Optical density measurements were performed using a Genesys 10S UV-Vis spectrophotometer with standard poly(styrene) cuvettes (optical path of 1 cm), scanning a wavelength range from 300 to 800 nm (Ribera et al., 2019). Different test concentrations and combinations of melanin, plant oils, copper sulfate (250, 500, and 1,000 ppm), and chitosan (Sigma Aldrich, product number 448877, MW = 190,000–310,000) were selected for these experiments. All combinations that were used in preliminary studies are summarized in Supplementary Table S1, whereas Table 1 only includes the four most promising combinations of plant oils and melanin. Finally, a commercial antimicrobial solution (Sanitized® Preservation, Switzerland) was used as a benchmark.
Wood treatment
The wood samples used for the tests were excised from the heartwood of European walnut trees. For the antimicrobial tests, the samples were cut into small blocks with dimensions of 25 mm × 15 mm × 10 mm. For every test, wood samples were prepared with two different fiber orientations, which are radial longitudinal (RL = 25R × 15T × 10 L mm) and tangential longitudinal (TL = 10R × 15T × 25 L mm) directions. The average ring width in the wood specimens was >1.5-6 annual rings/cm. Wood specimens were oven-dried at 103 °C and the dry weight was measured. Then the wood specimens were conditioned for 2 weeks at 25°C and 65%RH and placed into 2L glass beakers filled with antimicrobial substances. The glass beakers were sealed in a vacuum chamber. Impregnation was performed for 20–30 min under vacuum (100 hPa), followed by 60 min under ambient pressure for substance uptake. For the linseed and tea tree oil treatment, the oil mixture was prepared with a ratio of 9:1 (linseed:tea tree). For the combination of the other oils and melanin, the impregnation was performed in a two-step process, as the substances are immiscible liquids. First, samples were impregnated with melanin, dried overnight at 45 °C, and finally impregnated with the plant oil(s). After treatment, the samples were stored for at least 2 weeks in a climate chamber at 20 °C/65%RH. The treated wood samples were sterilized with ethylene oxide for 5 h.
Preservative uptake was determined by weighing wood samples immediately after impregnation. Excess oil on the wood surface was removed using clean filter papers. Preservative uptake by walnut wood samples was calculated as follows:
where Wi and We are the weights of 10 wood samples before and after impregnation, respectively, in kg, and V is the volume of wood sample in m3.
Antimicrobial efficacy test
The antimicrobial efficacy test for the potential wood preservatives was conducted according to the antimicrobial efficacy test conditions, GMP, and investigations (Sutton, 2013) with slight modifications to suit the requirements of wood, namely, the size and preconditioning of samples (Figure 2). In the antifungal assay, Chaetomium globosum Kunze spores collected from two Petri dishes of a 4-week-old culture were suspended in a sterile Antarox A-400 solution (0.5 ml per liter of distilled water). Treated and untreated wood samples were placed in the spore suspension for 1–2 min and then air-dried at 25°C and 75%RH. After 1 day, 3, 7, and 14 days, the tested samples were placed again for 10–15 min in a sterile Antarox solution. Then the solutions were diluted several times and placed into five petri dishes with 2% MEA at 25°C to count the number of surviving colonies.
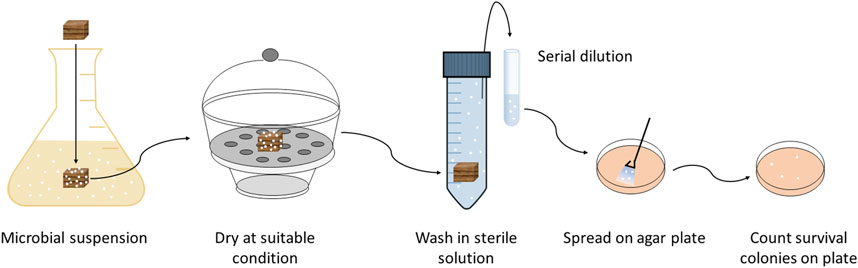
FIGURE 2. Scheme of antimicrobial efficacy test. Microorganisms were incubated at 25°C, 50% RH (fungus) and 23°C, 95% RH (bacteria).
In a similar manner, approximately 106 colony-forming unit (CFU)/mL suspensions of S. arlettae, P. fluorescens, or S. mutans were prepared with quarter-strength Ringer’s solution (Oxoid Thermo Fischer Scientific, Germany). Treated and control wood samples were placed in the microbial suspension for 1–2 min, then immediately transferred to a pre-conditioned desiccator at 23°C, 95%RH. After 1 and 3h, all samples were rinsed again for 10–15 min in a sterile Ringer solution. Two extra time points of 7 and 24 h were applied for S. mutans-exposed samples. Two serial, 1/10 dilutions were made, and 0.1 ml was plated in TSA. Petri dishes with S. mutans were incubated at 30 °C, whereas S. arlettae and P. fluorescens were incubated at 35°C.
The antimicrobial efficacy of melanin and natural oils was determined by measuring the survival rate of bacteria as follows:
where N0 is the total viable count on the surface of control wood samples at the time of incubation (CFUs/cm2) and N is the total viable count of survival after determined time periods.
Water absorption and dimensional stability test
For the dynamic determination of wood moisture and dimensions under different climate conditions, wood specimens with dimensions 40 mm × 40 mm × 10 mm were prepared according to DIN 52182, 52183, and 52184 (Nopens et al., 2019). After vacuum-pressure impregnation, wood samples were conditioned for 4 weeks in different climate chambers starting with 35%RH, subsequently followed by 65% and 85%RH. Manual measurements of mass and dimensional changes of samples were performed with a caliper directly within the climate chambers. Water absorption (WA) and dimensional stability (DS) were calculated according to Equations 3, 4:
where Mi and Me are the mass of 10 wood samples before and after the test, respectively, Te is the dimension of the samples at a conditioned climate (35%RH or 85%RH), and Ti is the dimension of samples at 65%RH.
Statistical analysis
All measurements were performed in triplicates and results were presented as mean value ±SD. Statistical differences were analyzed by an independent sample t-test. The difference was considered significant when p < 0.05.
Results
Isolation and identification of the casual decay fungus
Isolations from wood samples extracted from the damaged serpent did not show any fungal growth on MEA containing 0.2% thiabendazole. The fungal colonies of the aerial mycelium on 2% MEA were pale or olivaceous, often with yellow, gray-green, green, or red exudates. A daily growth rate of (6–) 7–8 mm was measured. Olive, gray-green, or brown ovate ascomata (Figure 3A) up to 500 μm long, with numerous coiled, unbranched setae developed within 7–9 days (Figure 3). Flat lemon-shaped to globose ascospores, brownish when matured, with dimensions of 9–12 × 8–10 × 6–8 μm were produced within the asci of the ascomata (Figure 3B).
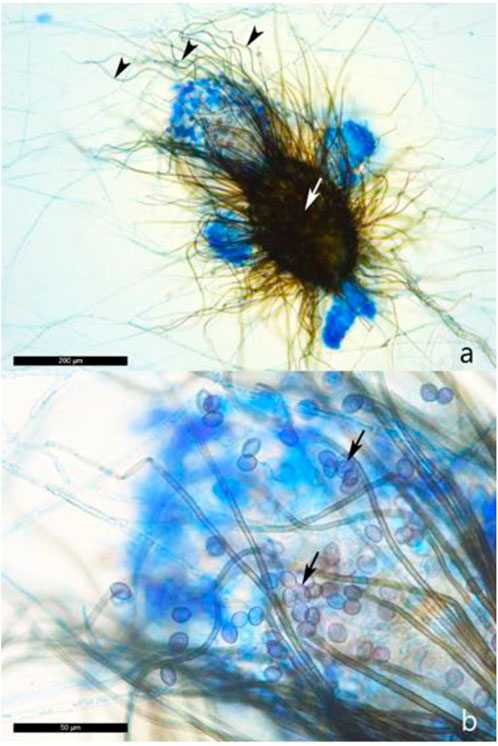
FIGURE 3. (A) Ascomata (white arrow) of Chaetomium globosum with a large number of apical hairs which are spirally coiled (black pointer). (B) Lemon-shaped, olive-brown colored ascospores (black arrows) of Chaetomium globosum, entrapped in the apical hairs.
Light microscopic observation of naturally infected walnut wood from a serpent
The presence of soft rot in the walnut wood caused by C. globosum was revealed by high-resolution light microscopy. The cell walls showed hyphal tunneling along the cellulose microfibrils of the S2 layer, resulting in the appearance of minute cavities in transverse sections (Figure 4A). This mode of attack is typical of Type 1 soft rot. Even at advanced stages, the persistence of a “lignin-rich” skeleton (Figures 4B,C), representing the most lignified components of the wood (Schwarze, 2007), remained mostly intact, preserving stiffness so that the wood becomes brittle.
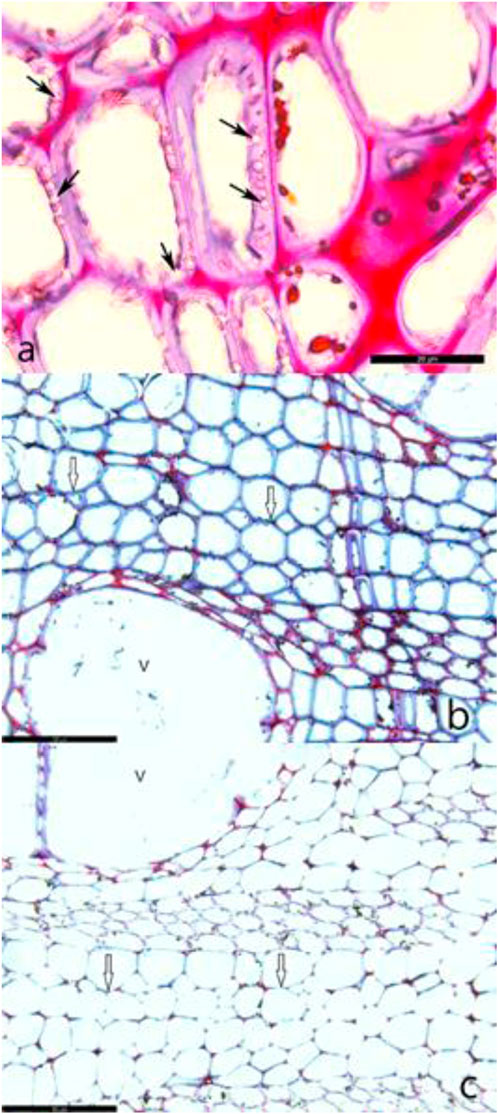
FIGURE 4. (A) Transverse sections of walnut wood naturally infected by Chaetomium globosum showing cavities (arrows) within the secondary walls of fiber-tracheids (bar, 20 μm). (B) During the early stages of decay, abundant hyphae are apparent within the cell lumina, whereas the secondary walls (arrows) remain intact (bar, 100 μm). (C) Progressive cell wall thinning of the fiber-tracheids (arrows) occurs from the lumen outwards (bar, 100 µm).
Uptake of melanin and natural oils in treated wood
The uptakes (in kg/m3) of melanin (Mel) and natural oils after impregnation are shown in Table 2. For both RL and TL wood samples, the oil uptake was similar for both linseed oil (LO) and mixed oil (MO). A slight change in oil absorbed was evident after the impregnation of wood with melanin; however, these changes were not statistically significant.
Antibacterial activity
In preliminary tests (see Supplementary Table S2), the survival of S. arlettae, P. fluorescens, and S. mutans on the untreated walnut wood samples at 23°C and 55% RH was investigated over 1-, 2-, and 3-h periods. A significant reduction in CFUs was recorded after 1h, and with the exception of S. mutans, nearly all bacterial species died within 3 h.
At higher moisture conditions (>90% RH, 23°C) in a closed chamber, CFUs of P. fluorescens (Gram negative) decreased significantly after 1 h (Figure 5), from 850 to 120 CFU/cm2, and almost no CFUs were evident after 3 h. The number of CFUs of S. arlettae decreased rapidly by 85–90% from 1,050 to 1,300 CFU/cm2 for both untreated and treated samples after 3 h. In fact, CFUs of S. mutans increased significantly after 1 day in both untreated RL and TL wood samples, with the RL samples retaining a greater number of CFUs (985 CFU/cm2) than the TL samples (778 CFU/cm2) after 1 h, and this pattern continued over time (Figure 5).
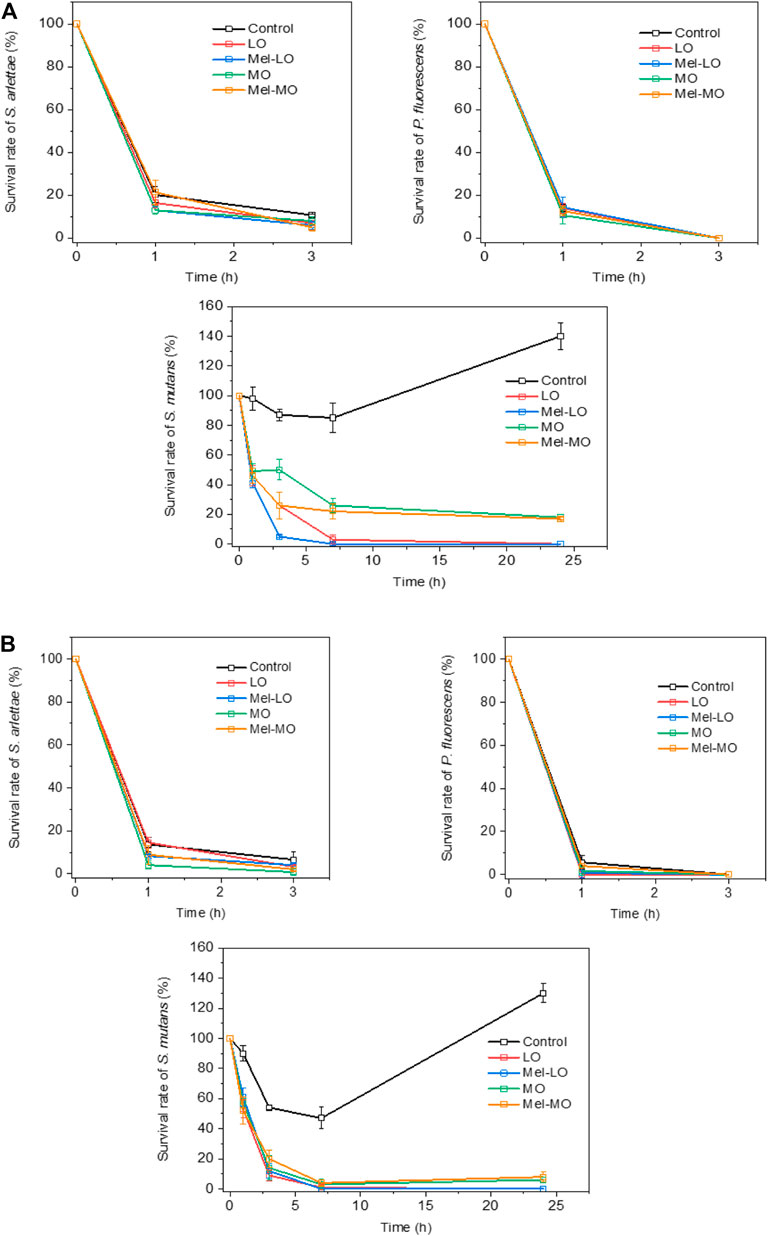
FIGURE 5. (A,B). Survival rate of tested bacteria over time on (A) radial-longitudinal and (B) tangential-longitudinal wood samples.
Antifungal activity
The initial C. globosum inoculum of approx. 950–1,100 CFU/cm2 survived on the wood for a long period and the survival rate remained above 50% on the untreated control wood samples after 14 days (Figure 6). When the wood samples were treated with plant oils, a positive antifungal effect was recorded in all cases. The benchmark preservative (Sanitized®) was more effective after a short period (1 day); however, inhibition of C. globosum rapidly decreased over time. A strong antifungal effect against C. globosum was observed on RL wood samples. The best performance was recorded from wood treated with Mel-MO, which was closely followed by Mel-LO. Both of these combinations provided 25% better efficacy than the benchmark product, and an approximately 5–10% enhanced efficacy after 14 days. At this time, less than 10% of the fungal spores from the initial inoculum survived.
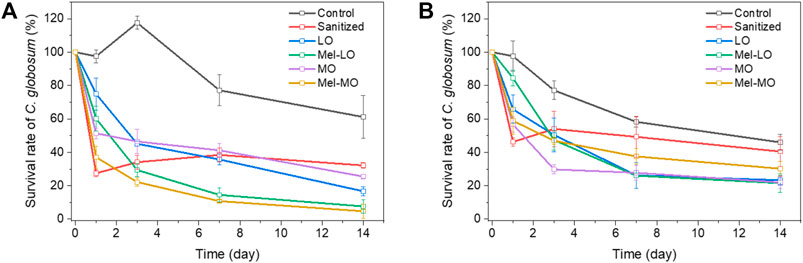
FIGURE 6. Survival rate (%) of C. globosum over time on (A) radial-longitudinal and (B) tangential-longitudinal wood samples.
The antifungal effect of all the plant oil-type combinations was also stronger than that of the benchmark product for TL samples; that is, the survival rate of C. globosum conidia was reduced by 20–25%. As with bacteria, more colonies were found on the RL than on the TL untreated wood samples. Interestingly, the opposite situation was observed on the treated wood samples, as more colonies were present on the TL than on the RL wood samples.
Sorption dynamic
The dimensions of the wood samples are provided in SI. For all walnut wood samples, the length and mass changes at a low RH (35%) and a high RH (85%) were calculated in comparison to wood samples stored at 65% RH (Table 3).
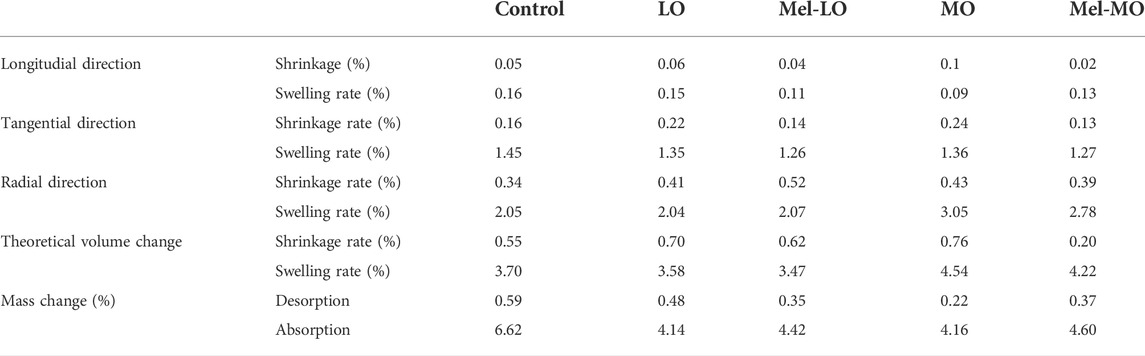
TABLE 3. Shrinkage and swelling rate of walnut wood after different treatments with plant oils and melanin.
As indicated in Table 3, wood samples treated with plant oils show similar shrinkage and swelling rates of dimensions and volume to that of the controls. The shrinkage rates were not significant in the tangential and radial directions, whereas the swelling rates were slightly higher, but less than 3% for all wood samples. It is worth noting that the impregnation of the wood samples with oil reduced moisture uptake (Bahmani and Schmidt, 2018).
Discussion
Identification of the casual decay fungus from infected wood
No fungi were isolated from wood samples from the damaged serpent on MEA containing thiabendazole. The latter growth medium is commonly used as a selective medium for isolating basidiomycetes (Sieber, 1995). The micromorphological features of fungal colonies obtained from infested wood (ascoma, conidia) were typical for Chaetomium globosum. High-resolution microscopy revealed a typical soft rot pattern (Corbett, 1965) in the walnut, which was in good agreement with that proposed by Popescu et al. (2011) who described similar C. globosum in lime wood using SEM.
The persistence of lignin-rich regions of the wood matrix in trees decayed by C. globosum is not only due to their high lignin content per se but also due to their high percentage of guaiacyl lignin (Schwarze, 2007). Most ascomycetes have relatively poor ligninolytic ability, and this is mainly confined to the decomposition of syringyl lignin (Nilsson et al., 1989).
Uptake rate comparison between single and double impregnation
The linseed oil uptake in treated walnut wood was comparable to that in beech wood but lower than that of pine or spruce wood (Humar and Lesar, 2013). This is due to the higher density and lower porosity of hardwoods, for example, beech and walnut, compared to the softwoods. It is worth noting that there was a slight change in oil uptake after melanin impregnation. However, these changes were not statistically significant. This also indicates that the initial impregnation of melanin does not hinder wood permeability. Studies showed that preservatives diffuse more easily in wood through the simple pits of the xylem rays (Schwarze et al., 2006).
The RL wood samples, with more exposed parenchyma cells in multiseriate xylem rays, retained a greater amount of melanin and natural oils than the TL wood samples. The uptake of melanin was significantly higher in the RL samples than in the TL samples. Colonization of the parenchyma cells by fungi and bacteria is also enhanced by the presence of readily degradable carbohydrates (Schwarze, 2007).
Efficacy of preservatives against bacteria
Preliminary tests (SI) suggest that walnut wood has intrinsic antibacterial properties, for example, a naturally occurring high tannin content that suppresses the growth of S. arlettae and P. fluorescens. Hon and Shiraishi (2000) studied the discoloration of hardwoods and found that black walnut heartwood contained 2% tannin (Hon and Nobuo., 2000). Previous studies indicate that some wood species like oak and walnut occlude their early wood vessels by suberized tyloses that have hydrophobic properties. This results in reduced water uptake of the wood, inhibiting the bacteria colonization of wood surfaces (Munir et al., 2019). Moreover, some naturally existing polyphenols in wood have been proven to have antibacterial effects (Plumed-Ferrer et al., 2013).
The studies indicated that P. fluorescens and S. arlettae could not readily colonize walnut. Since the growth of both bacterial species was inhibited on walnut wood, the addition of different plant oils and melanin did not reveal an enhanced antibacterial effect. S. mutans, the bacterium commonly found in human saliva, however, survived for a longer time.
Impregnation of walnut wood with plant oils clearly had an antibacterial effect. CFUs of S. mutans decreased by more than 50% after only 1 h compared to that of the untreated controls. However, the addition of tea tree oil did not enhance the efficacy of linseed oil against bacteria, whereas melanin showed an improved antibacterial effect, especially at the early stages of exposure. For instance, the addition of melanin in combination with oil(s) increased the antibacterial effect by 20–25% after 3 h. This suggests that the two-step impregnation of melanin and linseed oil treatment enhanced the efficacy against S. mutans. Although linseed oil is toxic to most bacteria after 24h, the addition of melanin significantly increased the antibacterial effect of treated wood.
Efficacy of natural oils and melanin against Chaetomium globosum
The antifungal effect of the benchmark product decreased over time, probably due to the water-based formulation of Sanitized® leaching out of the suspension during the dipping step. Unlike the benchmark, the plant oils alone or in combination with melanin (LO, MO, Mel-LO, and Mel-MO) are highly hydrophobic and counteract leaching when in water contact. Although the initial antimicrobial efficacy of these substances was lower than the benchmark, they showed superior results over time. Therefore, the tested wood samples were impregnated with melanin, which is a water-based preservative, prior to the treatment with plant oils to minimize leaching. The plant oils may have formed a water-repelling layer on the wood surface of the samples protecting the melanin within the wood.
The addition of melanin significantly enhanced the antifungal effects of plant oils on walnut. Moreover, the wood surface also impacts preservative efficiency. More colonies were found on the RL than on the TL samples on untreated wood. This may be due to the higher number of exposed parenchyma cells on the RL than on the TL surfaces, which allowed more microbial cells to colonize the wood. However, in the case of the double-impregnated samples, the higher porosity of the RL wood samples can also increase melanin uptake through the simple pits in the xylem ray parenchyma, thereby enhancing the antifungal effects against C. globosum. This is in good agreement with the recorded amounts of melanin uptake for treated wood samples (Table 2).
Effect of impregnation on the dimensional stability of wood
Dimensional changes resulting in a shrinking or swelling of wood are an important factor that may affect the visual and structural properties of a musical instrument. Dimensional changes of untreated wood occur when the moisture content in the wood decreases or increases in response to changes in the relative humidity (RH) of the atmosphere; that is, at a high RH, wood absorbs moisture and swells, while at a low RH, wood loses moisture and shrinks. Dimensional changes for treated wood can also be induced by the adsorption of natural oils or melanin. It is ideal if shrinking and swelling rates of the treated wood are lower or at least not significantly higher than those of the untreated wood. The wood samples treated with plant oils showed similar shrinkage and swelling rates as controls. Shrinkage and swelling rates were minor along the longitudinal direction (below 0.2%) as the cellulose microfibrils are stiff and strong. Thus, negligible dimensional changes are measured in the direction parallel to cellulose microfibrils (Yamashita et al., 2009).
Looking back to the development of woodpreservatives in general (Schultz et al., 2007), the high toxicity of the first-generation preservatives such as creosote and chromated copper arsenate has raised significant concerns over health issues; therefore, their usage is no longer permitted in most European countries. The second-generation wood preservatives are mainly water-borne copper-rich compounds such as alkaline copper quat, copper azole, copper xyligen, and copper betaine, which have good efficacy. Water-borne borates also belong to the second generation and together with the copper compounds, they are suited for residential applications. However, environmental concerns about the leaching of copper and borate, especially in aquatic systems, have led to the preference for non-metallic organic preservatives of the third generation.
Environmentally friendly and nature-derived substances such as essential oils have been investigated and used for many wood-preserving products (Broda, 2020). However, effective preservation requires a match among the oil types, the wood species, and the targeted wood deteriorating microorganisms. There are limited reports on preservatives used for walnut wood, especially walnut wood used in musical instruments. Nevertheless, the efficacy of linseed oil and tea tree oil against wood-decay fungi has been proven for other European wood species such as spruce and beech wood (Humar and Lesar, 2013; Mantil et al., 2015; Broda, 2020). It is worth noting that the conventional methods used to evaluate the antimicrobial efficacy in those studies are agar disk diffusion, broth dilution, or wood–surface contact (Munir et al., 2020). In the latter studies (Humar and Lesar, 2013; Broda, 2020), the results are quantitative and were achieved within 24–72 h, but they lack practical results on real wood samples. The second study (Munir et al., 2020) provides results that were obtained directly on wood samples but required a relatively long time (weeks to months) due to the slow growth of fungi. The convenience of our testing method is that we use the advantages combined from both methodologies, where the results are quantitative and can be obtained in a shorter time than those in traditional methods. Moreover, this is among the first research that investigates the effect of combining plant oils and melanin for wood preservation. The synergic antimicrobial efficacy of plant oils and melanin as reported in this work significantly contributes to the third generation of preservatives, as they are safe for use without health and environmental concerns.
Combinations of melanin and plant oils provided an efficient method for protecting walnut from bio-deterioration, without negatively affecting dimensional stability. Melanin and natural plant oils increased the resistance of walnut wood to colonization by bacteria and C. globosum. This method might be applicable for protecting the walnut used for making serpents and other precious wood species.
Data availability statement
The raw data supporting the conclusion of this article will be made available by the authors, without undue reservation.
Author contributions
AT-L: substantial contributions to the conception or design of the work; or the acquisition, analysis, or interpretation of data for the work; agree to be accountable for all aspects of the work in ensuring that questions related to the accuracy or integrity of any part of the work are appropriately investigated and resolved. FS: drafting the work or revising it critically for important intellectual content, and agree to be accountable for all aspects of the work in ensuring that questions related to the accuracy or integrity of any part of the work are appropriately investigated and resolved. TK: analysis or interpretation of data for the work and approval for publication of the content. MH: agree to be accountable for all aspects of the work in ensuring that questions related to the accuracy or integrity of any part of the work are appropriately investigated and resolved, and approval for the publication of the content.
Funding
This study was funded by Innosuisse (Project No. 36301.1 IP-ENG). Open access funding was provided by Empa—Swiss Federal Laboratories For Materials Science And Technology.
Acknowledgments
The authors are grateful to acknowledge financial support by the Innosuisse (Project No. 36301.1 IP-ENG). The authors thank Daniel Heer for the preparation of walnut wood samples for conducting the bioassays.
Conflict of interest
The authors declare that the research was conducted in the absence of any commercial or financial relationships that could be construed as a potential conflict of interest.
Publisher’s note
All claims expressed in this article are solely those of the authors and do not necessarily represent those of their affiliated organizations, or those of the publisher, the editors, and the reviewers. Any product that may be evaluated in this article, or claim that may be made by its manufacturer, is not guaranteed or endorsed by the publisher.
Supplementary material
The Supplementary Material for this article can be found online at: https://www.frontiersin.org/articles/10.3389/fmats.2022.915607/full#supplementary-material
References
Bahmani, M., and Schmidt, O. (2018). Plant essential oils for environment-friendly protection of wood objects against fungi. Maderas. Cienc. Tecnol. 20, 325–332.
Barnett, H. L., and Hunter, B. B. (1998). Illustrated genera of imperfect fungi. 4th ed. St. Paul, MN, USA: APS Press.
Broda, M. (2020). Natural compounds for wood protection against fungi—a review. Molecules 25 (15), 3538. doi:10.3390/molecules25153538
Corbett, N. H. (1965). Micro-morphological studies on the degradation of lignified cell walls by ascomycetes and fungi imperfecti. J. Inst. Wood Sci. 14, 18–29.
Echard, J.-P., Bertrand, L., von Bohlen, A., Le Hô, A.-S., Paris, C., Bellot-Gurlet, L., et al. (2010). The nature of the extraordinary finish of stradivari’s instruments. Angew. Chem. Int. Ed. 49 (1), 197–201. doi:10.1002/anie.200905131
Holman, C. (2017). Rhythm and metre in French classical plainchant. Early music. 45 (4), 657–664. doi:10.1093/em/cax087
Hon, D. N.-S., and Nobuo., Shiraishi (2000). Wood and cellulosic chemistry, revised, and expanded. Boca Raton: CRC Press.
Humar, M., and Lesar, B. (2013). Efficacy of linseed- and tung-oil-treated wood against wood-decay fungi and water uptake. Int. Biodeterior. Biodegrad. 85, 223–227. doi:10.1016/j.ibiod.2013.07.011
Jorge, F. C., Nunes, L., and Botelho, C. (2004). Boron in wood preservation: Problems, challenges and proposed solutions. An overview on recent research. J. Fac. Sci. Technol. 1, 3–15.
Lu, K.-T., and Chang, J.-P. (2020). Synthesis and antimicrobial activity of metal-containing linseed oil-based waterborne urethane oil wood coatings. Polym. (Basel) 12 (3), 663. doi:10.3390/polym12030663
Mantil, E., Daly, G., and Avis, T. J. (2015). Effect of tea tree (melaleuca alternifolia) oil as a natural antimicrobial agent in lipophilic formulations. Can. J. Microbiol. 61 (1), 82–88. doi:10.1139/cjm-2014-0667
Munir, M. T., Pailhories, H., Eveillard, M., Aviat, F., Lepelletier, D., Christophe, B., et al. (2019). Antimicrobial characteristics of untreated wood: Towards a hygienic environment. Health 11, 152–170. doi:10.4236/health.2019.112014
Munir, M. T., Pailhories, H., Eveillard, M., Irle, M., Aviat, F., Dubreil, L., et al. (2020). Testing the antimicrobial characteristics of wood materials: A review of methods. Antibiotics 9 (5), 225. doi:10.3390/antibiotics9050225
Nilsson, T., Daniel, G., Kent, K., and Obst, J. R. (1989). Chemistry and microscopy of wood decay by some higher ascomycetes. Holzforschung 43 (1), 11–18. doi:10.1515/hfsg.1989.43.1.11
Nopens, M., Riegler, M., Hansmann, C., and Krause, A. (2019). Simultaneous change of wood mass and dimension caused by moisture dynamics. Sci. Rep. 9 (1), 10309. doi:10.1038/s41598-019-46381-8
Palmer, P. R. (1990). In defense of the serpent. Hist. Brass. Soc. J. 2, 132–186. doi:10.2153/0119900011008
Panov, D., and Terziev, N. (2015). Durability of epoxi-oil modified and alkoxysilane treated wood in field testing. BioResources 10, 2479–2491. doi:10.15376/biores.10.2.2479-2491
Plumed-Ferrer, C., Väkeväinen, K., Komulainen, H., Rautiainen, M., Smeds, A., Raitanen, J.-E., et al. (2013). The antimicrobial effects of wood-associated polyphenols on food pathogens and spoilage organisms. Int. J. Food Microbiol. 164 (1), 99–107. doi:10.1016/j.ijfoodmicro.2013.04.001
Popescu, C.-M., Tibirna, C., Manoliu, A. L., Petronela, G., and Vasile, C. (2011). Microscopic study of lime wood decayed by Chaetomium globosum. Cellul. Chem. Technol. 45, 565–569.
Ribera, J., Panzarasa, G., Stobbe, A., Osypova, A., Rupper, P., Klose, D., et al. (2019). Scalable biosynthesis of melanin by the basidiomycete Armillaria cepistipes. J. Agric. Food Chem. 67 (1), 132–139. doi:10.1021/acs.jafc.8b05071
Rigling, D., Günthardt-Goerg, M. S., Blauenstein, H., and Frey, B. (2006). Accumulation of heavy metals into Armillaria rhizomorphs from contaminated soils. For. Snow Landsc. Res. 80 (2), 213–220.
Schultz, T. P., Nicholas, D. D., and Preston, A. F. (2007). A brief review of the past, present and future of wood preservation. Pest Manag. Sci. 63 (8), 784–788. doi:10.1002/ps.1386
Schwarze, F. W. M. R., Landmesser, H., Zgraggen, B., and Heeb, M. (2006). Permeability changes in heartwood of picea abies and abies alba induced by incubation with physisporinus vitreus. Holzforschung 60 (4), 450–454. doi:10.1515/HF.2006.071
Schwarze, F. W. M. R. (2007). Wood decay under the microscope. Fungal Biol. Rev. 21 (4), 133–170. doi:10.1016/j.fbr.2007.09.001
Sieber, T. N. (1995). Pyrenochaeta ligni-putridi sp. Nov., a new coelomycete associated with butt rot of picea abies in Switzerland. Mycol. Res. 99 (3), 274–276. doi:10.1016/S0953-7562(09)80897-6
Six, A. (2021) The black gold of mushrooms. Available at: https://www.empa.ch/web/s604/melanin-aus-pilzen.
Smith, M. L., Bruhn, J. N., and Anderson, J. B. (1992). The fungus Armillaria bulbosa is among the largest and oldest living organisms. Nature 356 (6368), 428–431. doi:10.1038/356428a0
Stalpers, J. A. (1978). Identification of wood-inhabiting aphyllophorales in pure culture studies in mycology. Baarn Netherlands: CABI.
Tran-Ly, A. N., De France, K. J., Rupper, P., Schwarze, F. W. M. R., Reyes, C., Nyström, G., et al. (2021). Melanized-cationic cellulose nanofiber foams for bioinspired removal of cationic dyes. Biomacromolecules 22 (11), 4681–4690. doi:10.1021/acs.biomac.1c00942
Tran-Ly, A. N., Reyes, C., Schwarze, F. W. M. R., and Ribera, J. (2020). Microbial production of melanin and its various applications. World J. Microbiol. Biotechnol. 36 (11), 170. doi:10.1007/s11274-020-02941-z
Tran-Ly, A. N., Ribera, J., Schwarze, F. W. M. R., Brunelli, M., and Fortunato, G. (2020). Fungal melanin-based electrospun membranes for heavy metal detoxification of water. Sustain. Mater. Technol. 23, e00146. doi:10.1016/j.susmat.2019.e00146
Vallero, D., Isukapalli, S., Zartarian, V., McCurdy, T., McKone, T., Georgopoulos, P., et al. (2010). “Chapter 44 - modeling and predicting pesticide exposures,” in Hayes’ handbook of pesticide toxicology. Editor R. Krieger. Third Edition (New York: Academic Press), 995–1020. doi:10.1016/B978-0-12-374367-1.00044-6
Vidholdová, Z., Slabejová, G., and Šmidriaková, M. (2021). Quality of oil- and wax-based surface finishes on thermally modified oak wood. Coatings 11 (2), 143. doi:10.3390/coatings11020143
Keywords: natural wood preservative, plant oil, melanin, antimicrobial property, wood decay
Citation: Tran-Ly AN, Heeb M, Kalac T and Schwarze FWMR (2022) Antimicrobial effect of fungal melanin in combination with plant oils for the treatment of wood. Front. Mater. 9:915607. doi: 10.3389/fmats.2022.915607
Received: 08 April 2022; Accepted: 08 August 2022;
Published: 13 September 2022.
Edited by:
Emil Engelund Thybring, University of Copenhagen, DenmarkReviewed by:
Olaf Schmidt, University of Hamburg, GermanyLisbeth Garbrecht Thygesen, University of Copenhagen, Denmark
Copyright © 2022 Tran-Ly, Heeb, Kalac and Schwarze. This is an open-access article distributed under the terms of the Creative Commons Attribution License (CC BY). The use, distribution or reproduction in other forums is permitted, provided the original author(s) and the copyright owner(s) are credited and that the original publication in this journal is cited, in accordance with accepted academic practice. No use, distribution or reproduction is permitted which does not comply with these terms.
*Correspondence: Francis W. M. R. Schwarze, francis.schwarze@empa.ch