- 1College of Life Science, Northeast Forestry University, Harbin, China
- 2State Key Laboratory of Crop Biology, College of Life Sciences, Shandong Agricultural University, Tai’an, China
- 3Division of Plant Science, Research School of Biology, The Australian National University, Canberra, ACT, Australia
Pseudomonas syringae pv. tabaci (Pst) is a hemibiotrophic bacterial pathogen responsible for tobacco wildfire disease. Although considerable research has been conducted on the tobacco plant’s tolerance to Pst, the role of light in the responses of the photosystems to Pst infection is poorly understood. This study aimed to elucidate the underlying mechanisms of the reduced photosystem damage in tobacco leaves due to Pst infection under light conditions. Compared to dark conditions, Pst infection under light conditions resulted in less chlorophyll degradation and a smaller decline in photosynthetic function. Although the maximal quantum yield of photosystem II (PSII) and the activity of the photosystem I (PSI) complex decreased as Pst infection progressed, damage to PSI and PSII after infection was reduced under light conditions compared to dark conditions. Pst was 17-fold more abundant in tobacco leaves under dark compared to light conditions at 3 days post inoculation (dpi). Additionally, H2O2 accumulated to a high level in tobacco leaves after Pst infection under light conditions; although to a lesser extent, H2O2 accumulation was also significant under dark conditions. Pretreatment with H2O2 alleviated chlorotic lesions and decreased Pst abundance in tobacco leaves at 3 dpi under dark conditions. MV pretreatment had the same effects under light conditions, whereas 3-(3,4-dichlorophenyl)-1,1-dimethylurea pretreatment aggravated chlorotic lesions and increased the Pst population. These results indicate that chlorotic symptoms and the size of the bacterial population are each negatively correlated with H2O2 accumulation. In other words, light appears to suppress the Pst population in tobacco leaves through the accumulation of H2O2 during infection.
Introduction
Plants are simultaneously exposed to abiotic and biotic stresses, and their responses to pathogens (bacteria, fungi, and viruses) at various stages of development are largely dependent on environmental factors such as light, temperature, and water (Bostock et al., 2014; Suzuki et al., 2014). Light is the major external factor influencing plant growth, development and physiology, and it also has an important impact on defense responses as follows: (i) by providing signals for the deployment of defensive barriers; (ii) by influencing the general energy supply and, thus, the ‘fuel’ available to launch and sustain responses against invaders; and (iii) by inducing the production of ROS in chloroplasts and peroxisomes (Kangasjärvi et al., 2012). Light availability is particularly important during the first hours after infection, as the absence of light during the early plant–pathogen interaction stages negatively affects development of the hypersensitive response (HR) at later stages (Griebel and Zeier, 2008).
It is well established that light is required for regulating resistance responses in several plant–pathogen interactions (Roberts and Paul, 2006; Delprato et al., 2015). For example, Cerrudo et al. (2012) revealed that a low red/far-red light ratio reduces resistance to the necrotrophic fungus Botrytis cinerea in Arabidopsis. Mühlenbock et al. (2008) showed that plants acclimate to excessive excitation energy (a condition in which the amount of light absorbed by the photosystems exceeds that required for photosynthetic metabolism) and display increased resistance to a virulent strain of Pseudomonas syringae. In addition, Zeier et al. (2004) reported light-dependent resistance of Arabidopsis to P. syringae pv. tomato DC3000 (Pto) conferred through a different resistance–avirulence gene interaction; they also observed that pathogenesis-related protein expression was light dependent in Arabidopsis plants inoculated with P. syringae pv. maculicola (Zeier et al., 2004). Genoud et al. (2002) demonstrated that light plays important roles in SA-mediated pathways and that it is required for the HR, and Arabidopsis plants grown in the dark exhibit compromised local and systemic resistance responses to Pto.
Pseudomonas syringae pv. tabaci (Pst) is a hemibiotrophic bacterial pathogen responsible for tobacco wildfire disease (Ramegowda et al., 2013). Previous research has shown that a circadian rhythm has a large impact on plant immunity during plant–pathogen interaction (Hua, 2013). For example, the susceptibility of tomato plants to Pto is influenced by a diurnal rhythm, with the greatest susceptibility in the evening (Yang et al., 2015). Light regulation of defense responses is relevant not only during artificial darkening but also during natural light/dark cycles (Kangasjärvi et al., 2012). Considerable research has been conducted on the tobacco plant’s tolerance to Pst (Hann and Rathjen, 2007; Taguchi and Ichinose, 2011; Lee et al., 2013) as well as on the photosynthetic performance of plants infected by other P. syringae pathovars (Bonfig et al., 2006; Rodríguez-Moreno et al., 2008). For example, Berger et al. (2007) found decreased maximum PSII quantum yield (Fv/Fm) in Pto-infected Arabidopsis leaves, and Pérez-Bueno et al. (2015) found decreased photochemical efficiency of PSII in Pto-infected Phaseolus vulgaris leaves. The effects of light on plants under various abiotic stresses have also been well studied. For example, Crous et al. (2012) reported light-induced inhibition of leaf respiration in field-grown Eucalyptus saligna under elevated atmospheric CO2 and drought, and De Frenne et al. (2015) revealed that light accelerates plant responses to warming. However, the underlying mechanisms by which light affects the tobacco–Pst interaction, especially when explored from a physiological perspective, remain poorly understood.
Reactive oxygen species are unavoidable by-products of photosynthesis in plants, and photosynthetic electron transfer reactions in light-exposed green tissues are a significant source of ROS due to the formation of highly reactive singlet oxygen (1O2) and the less reactive superoxide (O2-) and H2O2 which can potentially yield highly reactive hydroxyl free radicals (Aro et al., 2005; Li et al., 2009). The overall level of ROS in cells is the result of both ROS production and ROS scavenging. The main scavenging systems in plant cells include antioxidative enzymes and antioxidants (Mittler et al., 2011); among the former, CAT is thought to play a very important role (Zavaleta-Mancera et al., 2007).
A marked decline in photosynthesis will lead to excessive excitation energy which, if not dissipated safely, will increase the production of ROS in plant leaves (Jia et al., 2010). Despite their potential toxicity, ROS actually have a dual role in vivo, depending on their concentration, site and duration of action, as well as the plant’s previous exposure to stress (Miller et al., 2010). At low concentrations, ROS act as messenger molecules involved in signaling related to acclimation and trigger defense mechanisms against stress; at high concentrations, ROS promote programmed cell death, membrane lipid peroxidation and oxidative damage to the photosynthetic apparatus (Foyer and Noctor, 2009; Kangasjärvi et al., 2009; Galvez-Valdivieso and Mullineaux, 2010; Suzuki et al., 2012).
In this study, we aimed to investigate if the photosynthetic apparatus of tobacco leaves responds to Pst infection differently under light and dark conditions. What is the role of light during tobacco responses to Pst infection, and are ROS involved in this progress? To address these questions, we (1) evaluated changes in the activities of PSI and PSII, (2) monitored the content of H2O2 and the bacterial population, and (3) evaluated the relationship between light, H2O2 and the Pst population after Pst inoculation of tobacco leaves.
Materials and Methods
Plant Material and Pst Infiltration
Seeds of Nicotiana tabacum cv. Longjiang 911, a susceptible cultivar (Sun et al., 2011), were germinated in vermiculite; after 45 days, the seedlings were transplanted into pots containing a compost-soil substrate and grown in a greenhouse (25°C, 70% relative humidity) under long-day conditions (14 h light/10 h darkness). The two upper fully expanded attached leaves of 6- to 8- week-old plants were used for experiments.
Pseudomonas syringae pv. tabaci was grown overnight on solid King’s B agar plates (King et al., 1954) and diluted with distilled water to a concentration 105 cfu ml-1. Distilled water (mock) or bacterial suspensions were hand-infiltrated using a needleless syringe into mesophyll tissue on the abaxial side of the leaves at 8 am. The infiltrated area was approximately 1 cm ×1 cm, and in situ measurements were performed at a distance of 0.5 cm from the infiltration point. Following inoculation, the leaves were kept under a 14 h light (200 μmol m-2 s-1)/10 h dark cycle or continuous darkness at 25°C in a chamber with 70% relative humidity.
To induce or inhibit the generation of H2O2 under light conditions, a solution of MV (1 μM) or DCMU (70 μM) was uniformly sprayed onto the tobacco leaves at 1 h prior to Pst infiltration. At the concentrations used, neither of the chemicals had a detectable effect on Pst growth. To increase the H2O2 content in tobacco leaves under dark conditions, exogenous H2O2 (4 mM) was uniformly sprayed onto tobacco leaves prior to Pst infiltration.
Measurement of Spectral Reflectance in Pst-Infiltrated Zone of Tobacco Leaves
Spectral reflectance was measured by a Unispec SC field portable spectrometer (PP Systems, USA). Leaf reflectance was measured with a bifurcated fiber optic cable and a leaf clip (UNI410, PP Systems, USA). The leaf clip held the fiber optic cable at a 60° angle to the adaxial leaf surface (30° from the normal). Leaf illumination from a tungsten halogen lamp in the spectrometer was provided through one branch of the bifurcated fiber.
Measurements of Total Chlorophyll Content in Tobacco Leaves after Pst Infection
Leaf disks (diameter 1 cm) within the region of infiltration were excised, and total chlorophyll was extracted with 80% acetone in the dark for 72 h at 4°C. The extracts were analyzed using a UV-visible spectrophotometer (UV-1601, Shimadzu, Japan) according to the method of Porra (2002).
Measurements of the Chlorophyll a Fluorescence Transient (OJIP) and PSI Complex Activity in Tobacco Leaves after Pst Infection
The induction kinetics of prompt fluorescence and the MR820nm were simultaneously recorded using a Multifunctional Plant Efficiency Analyzer, M-PEA (Hansatech, UK), as described (Strasser et al., 2010). At 3 dpi under light or dark conditions, all leaves were dark-adapted for 20 min before the measurements. Chlorophyll a fluorescence transients were analyzed using the JIP-test, as follows: Fv/Fm = 1 - (Fo/Fm), where Fo and Fm are the initial and maximum fluorescence values of the induction kinetics curves, respectively.
Detection of H2O2 Generation in Tobacco Leaves after Pst Infection
In situ H2O2 was detected by DAB staining, as described previously (Ueno et al., 2003). Leaf disks (diameter 1 cm) within the region of infiltration were excised and incubated in 1 mg ml-1 DAB-HCl (pH 5.5) for 6 h at room temperature; chlorophyll was removed with 95% ethanol. H2O2 was extracted and quantified according to the method of Patterson et al. (1984). Leaf segments (0.5 g) were ground in liquid nitrogen and extracted with 5 ml of 5% (w/v) trichloroacetic acid; the mixture was centrifuged at 16 000 × g for 10 min and the supernatant was collected. A titanium reagent (0.4 ml of 20 % titanic tetrachloride in concentrated HCl, v/v) was added to 3 ml of the extract followed by the addition of 0.6 ml of NH4OH to precipitate the peroxide-titanium complex. After 5 min of centrifugation at 12 000 × g, the supernatant was discarded, and the precipitate was solubilized in 2 ml of 2 M H2SO4. The absorbance of the final solution was measured at 415 nm. A standard curve was used to determine the concentration of H2O2 in the extract.
Enzyme Assays
Leaf segments (0.5 g) of infiltrated areas at 3 dpi were ground to a fine powder with liquid nitrogen and then homogenized in 5 ml of 50 mM of potassium phosphate buffer (pH 7.8) containing 1 mM EDTA, 0.3 % Triton X-100 and 1% (w/v) polyvinylpyrrolidone. The homogenate was centrifuged at 13000 × g for 20 min at 4°C, and the supernatant was used for the following enzyme assays and protein measurement. SOD (EC 1.15.1.1) activity was assayed by monitoring inhibition of the photochemical reduction of nitro blue tetrazolium according to the method of Giannopolitis and Ries (1977). CAT (EC 1.11.1.6) activity was determined according to the method of Aebi (1984) by monitoring the decrease in absorbance at 240 nm as a result of decomposition of H2O2. The total soluble protein concentration was determined using the method of Bradford (1976) with bovine serum albumin as the standard.
Enumeration of Bacteria in Tobacco Leaf Tissue after Pst Infection
To monitor bacterial propagation in the leaves, two leaf disks (diameter 1 cm) within the region of infiltration were excised from each plant at 8 am and homogenized in 1 ml of 10 mM MgCl2 (Katagiri et al., 2002). After appropriate dilution, the bacterial cfu was assessed by plating an appropriate dilution on solid King’s B agar plates followed by incubation at 25°C.
P. syringae pv. tabaci Growth under Light or Dark Conditions
The direct effect of light on Pst growth was evaluated by plating the same amount of Pst sample on solid King’s B agar plates. The plates were then divided into two groups for cultivation for 3 days at 25°C under light (200 μmol m-2 s-1) or dark conditions. All bacteria from each group were collected, and the total number of Pst cells was counted to reflect the Pst multiplication rate.
Chemicals Used in the Study
All the compounds used in this study were sourced from Sigma–Aldrich (USA).
Statistical Analysis
Means (at least three independent measurements) were compared by analysis of variance (ANOVA) and LSD range test at a 5% level of significance.
Results
The Effect of Light on Spectral Reflectance in Tobacco Leaves after Pst Infection
Spectral reflectance has been widely used to study the physiological status of plants under different environmental conditions. It has been shown that chlorophyll content influences the spectral reflectance in the visual waveband (500 - 700 nm) and that the inner structure of the leaf influences the spectral reflectance in the near-infrared waveband (700-1,300 nm) (Sims and Gamon, 2002). An established vegetation index, i.e., PRI, was calculated as PRI = (R531 - R570)/(R531 + R570). Although the spectral curves of the infiltrated zone revealed an increase in the visual waveband, there were insignificant changes in the near-infrared waveband at 3 dpi under light and dark conditions (Figure 1A). These results indicated that the chlorophyll content in the infiltrated zone of the tobacco leaves decreased markedly but that the inner structure was not severely damaged at 3 dpi under light and dark conditions. The total chlorophyll content in the infiltrated zone was then measured, showing decreases to 68.2 and 53.4% of the untreated values at 3 dpi under light and dark conditions, respectively (Figure 1B). PRI can serve as an estimate of the photosynthetic light use efficiency (Evain et al., 2004) and an indicator of photosynthetic function (Weng et al., 2009). PRI was found to have decreased to 55.2 and 32.1% at 3 dpi under light and dark conditions, respectively (Figure 1B). These results indicated that Pst infection in tobacco leaves led to more severe chlorophyll degradation and photosynthetic function decline under dark conditions than under light conditions.
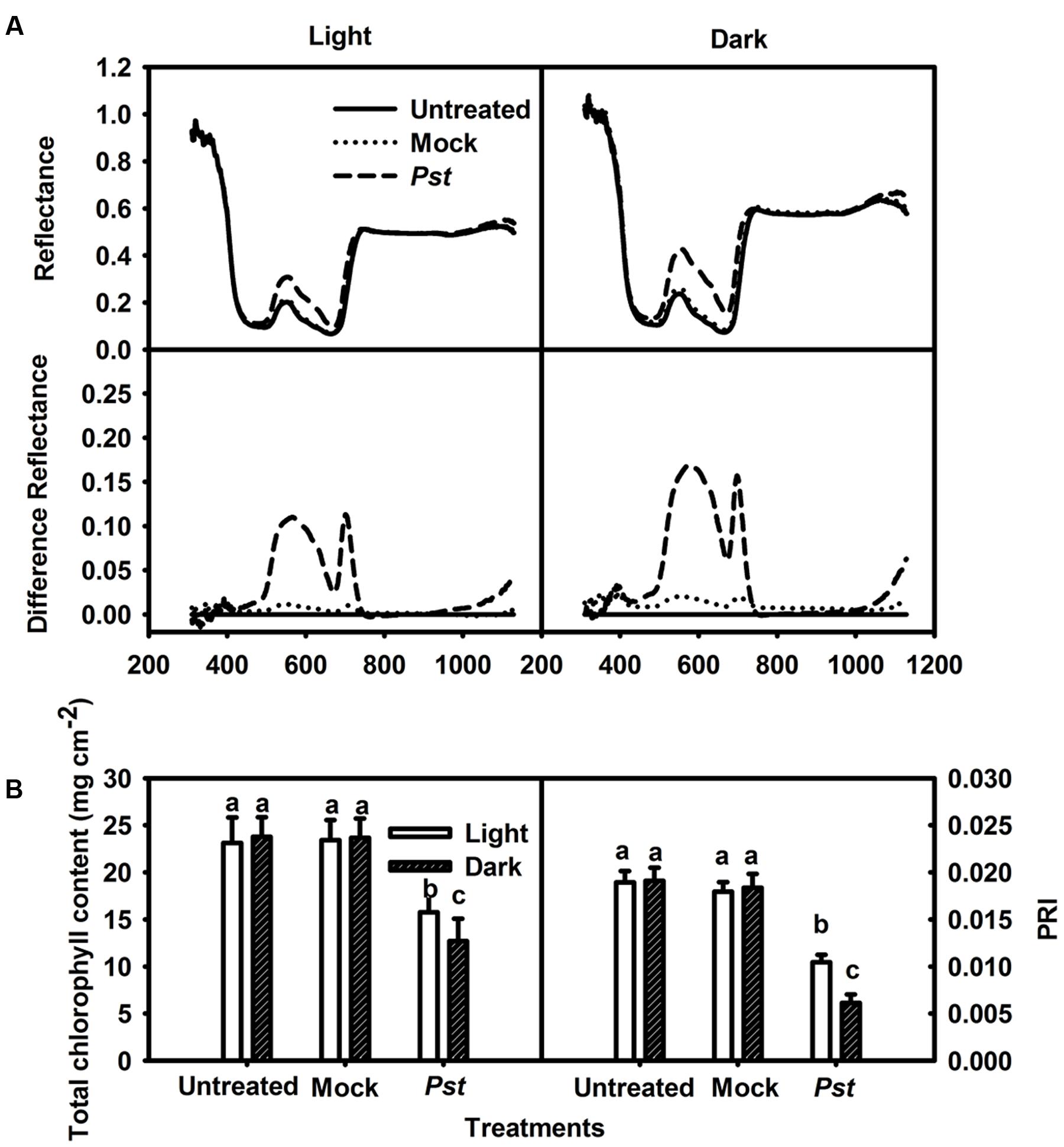
FIGURE 1. Relative changes in spectral reflectance, total chlorophyll content and PRI after Pst infection of tobacco leaves. (A) Relative changes in spectral reflectance in tobacco leaves at 3 dpi under light (200 μmol m-2 s-1) or dark conditions. The difference in reflectance was obtained by subtracting the kinetics of untreated leaves from the kinetics of tobacco leaves treated with distilled water (Mock - untreated) or Pst (Pst- untreated). Each curve represents the average of 50 independent measurements. (B) Relative changes in total chlorophyll content at 3 dpi in tobacco leaves. PRI was calculated from the spectral reflectance. Means ± SE of 50 replicates are shown. Different letters above the columns indicate significant differences between treatments at P < 0.05.
The Effect of Light on the PSI and PSII Activities in Tobacco Leaves after Pst Infection
The effect of Pst infection on the photosynthetic apparatus was evaluated at 3 dpi. The maximum quantum yield of PSII (Fv/Fm) decreased to 93.4 and 89.2% of the values of untreated leaves at 3 dpi under light and dark conditions, respectively (Figure 2). The MR820nm signal provides information about the oxidation status of P700 in PSI, including the oxidation state of plastocyanin that donates electrons to PSI. The induction curve of MR820nm for dark-treated leaves obtained by a saturating red light showed a fast oxidation phase and a subsequent reduction phase. The initial slope of the oxidation phase of MR820nm indicates the capability of P700 to get oxidized, which is then used to reflect the activity of PSI (Oukarroum et al., 2013; Gao et al., 2014), and the PSI complex activities of the treated leaves were 83.5 and 72.4% of those of untreated leaves at 3 dpi under light and dark conditions, respectively (Figure 2). These results indicated that the extent of the decrease in Fv/Fm and PSI complex activity in tobacco leaves was greater in darkness than under light conditions after Pst infection.
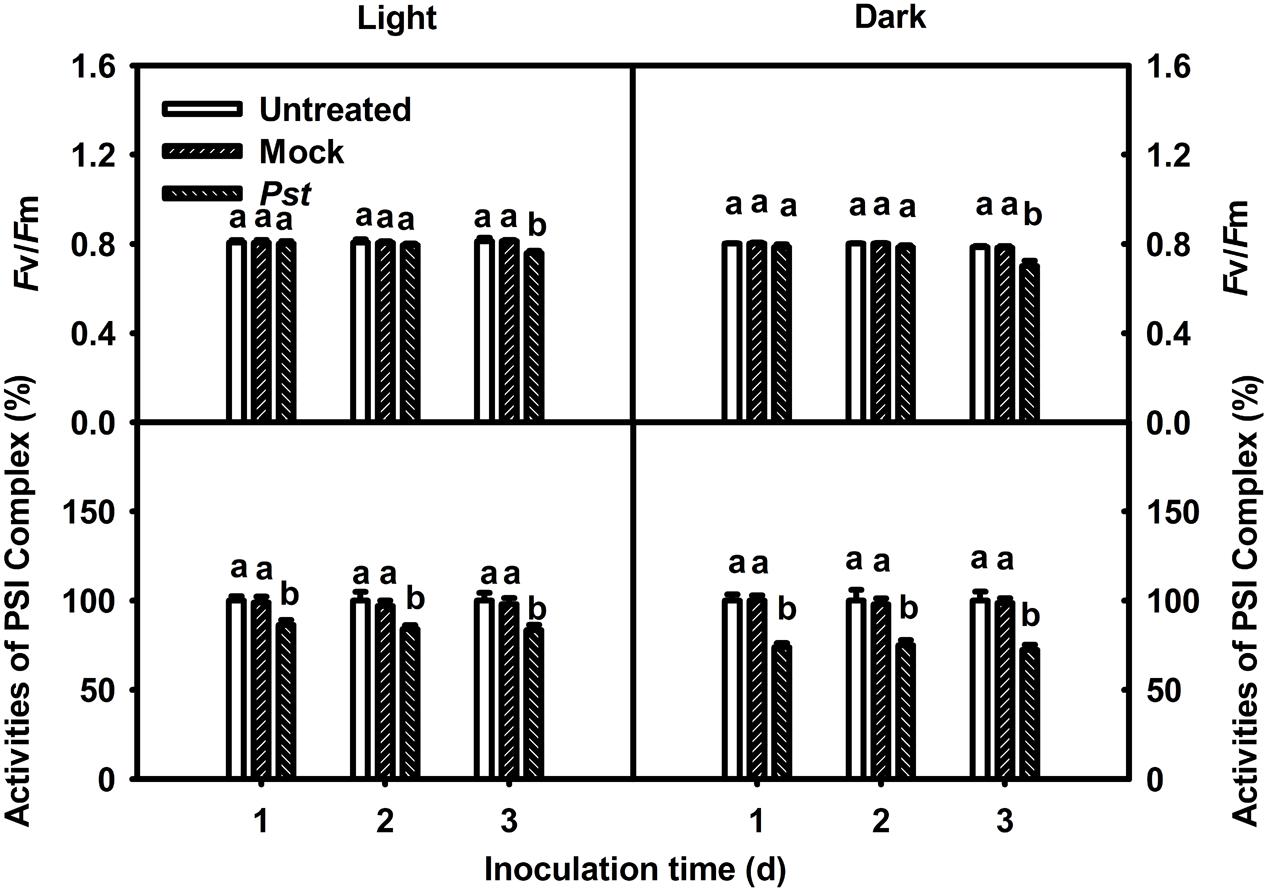
FIGURE 2. Relative changes in Fv/Fm and PSI complex activity after Pst infection of tobacco leaves. Chlorophyll a fluorescence transients were analyzed with the JIP-test. The maximum quantum yield of PSII (Fv/Fm) and PSI complex activity values were calculated after tobacco leaves were inoculated with distilled water (Mock) or Pst for different durations under light (200 μmol m-2 s-1) or dark conditions. The initial PSI complex activity of untreated tobacco leaves was taken as 100%; the activities of mock- and Pst-treated leaves were calculated as the percentage of activity in untreated leaves. Means ± SE of 10 replicates are presented. Different letters above the columns indicate significant differences between treatments at P < 0.05.
The Effect of Light on the Bacterial Population in Tobacco Leaves at 3 dpi after Pst Infection
The population of Pst in leaves increased by several orders of magnitude at 3 dpi compared with that of untreated leaves. Furthermore, the bacterial population was 17-fold greater in darkness than under light conditions at 3 dpi (Figure 3). To exclude a direct effect of light on the multiplication rate of Pst during infection, this effect was evaluated on plate-grown bacteria (without leaves) at a constant temperature. No difference in the Pst population was observed between cultivation in light and dark conditions, indicating that light per se does not have a direct effect on Pst growth (Figure 4).
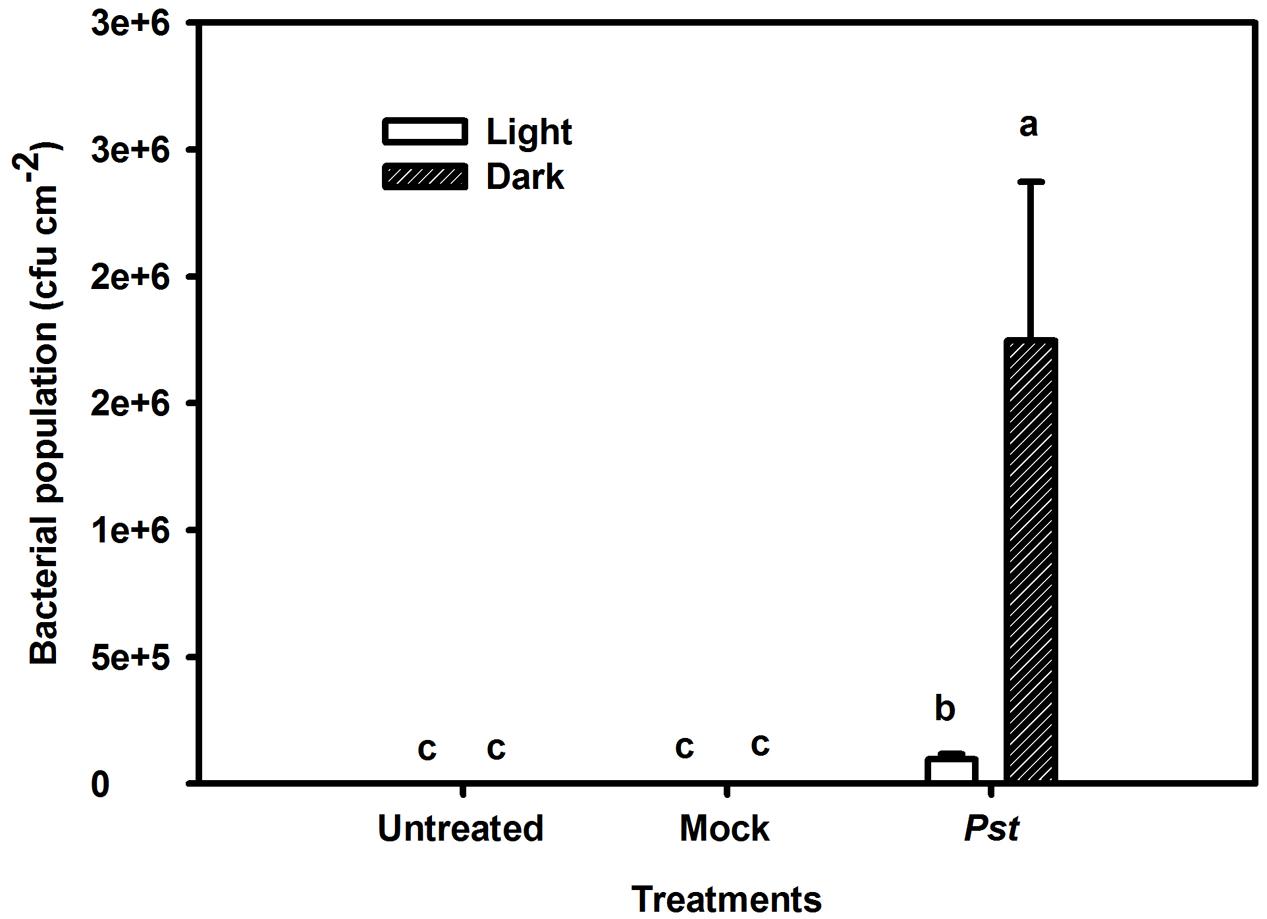
FIGURE 3. Relative changes in the bacterial population after Pst infection in tobacco leaves. Bacterial population was evaluated after leaves were inoculated with distilled water (Mock) or Pst at 3 dpi under light or dark conditions. Means ± SE of six replicates are presented. Different letters above the columns indicate significant differences between treatments at P < 0.05.
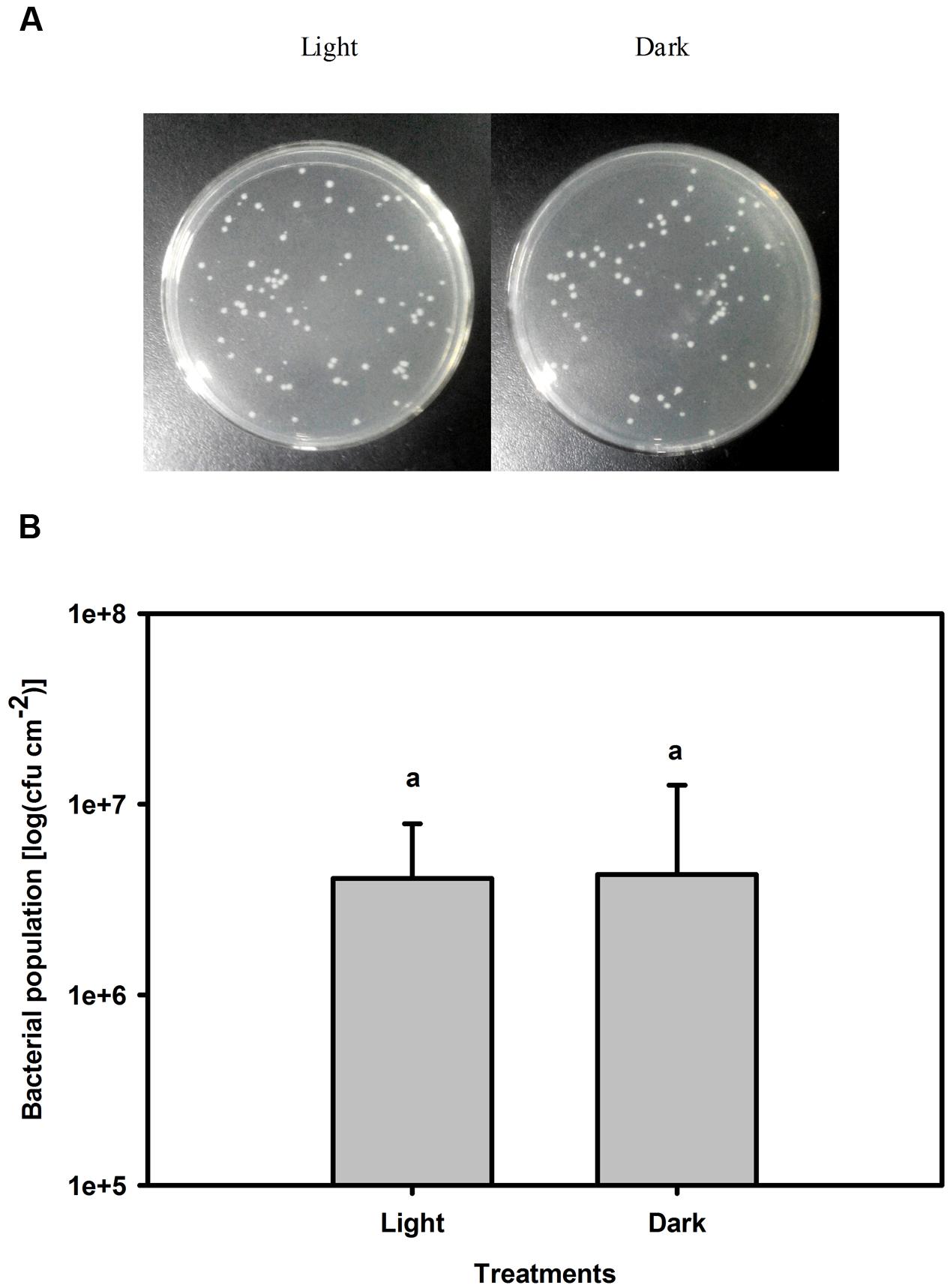
FIGURE 4. Changes in the Pst population after 3 days of cultivation under light or dark conditions. (A) Pst population was counted on solid King’s B agar plates after appropriate dilution. (B) Pst population was calculated after 3 days cultivation under light (200 μmol m-2 s-1) or dark conditions. Means ± SE of four replicates are presented. Different letters above the columns indicate significant differences between treatments at P < 0.05.
Changes in H2O2 Levels and Antioxidative Enzyme Activities in Tobacco Leaves after Pst Infection under Light or Dark Conditions
Although light had no direct effect on Pst multiplication (Figure 4), light may influence ROS production in tobacco leaves after Pst infection. Because H2O2 is the most stable ROS that can be readily measured (Fleury et al., 2002), H2O2 production was evaluated in the Pst-infiltrated zone at 3 dpi. As shown in Figure 5A, Pst infection clearly enhanced H2O2 accumulation in tobacco leaves under light or dark conditions, with the content being increased by 258 and 107% at 3 dpi compared to untreated leaves under light and dark conditions, respectively (Figure 5B). The results indicated that a significant amount of H2O2 accumulated in tobacco leaves due to Pst infection under light and, to a lesser extent, dark conditions. As shown in Figure 6A, the activities of SOD increased in tobacco leaves at 3 dpi. In contrast, the CAT activity was greatly reduced after Pst infection (Figure 6B).
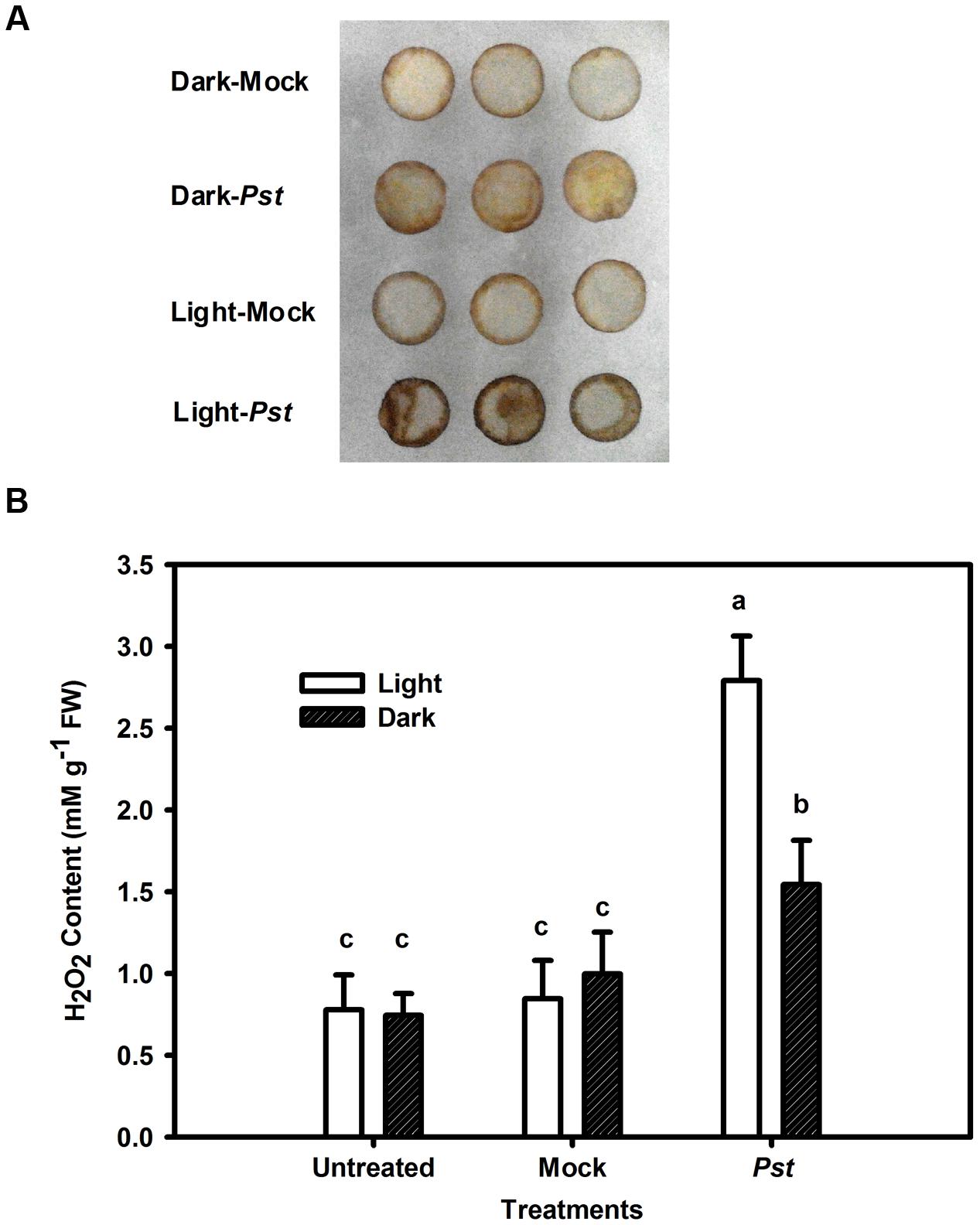
FIGURE 5. Changes in H2O2 content in tobacco leaves after Pst infection. (A) Histochemical detection of H2O2 accumulation using DAB staining in tobacco leaves after infiltration with distilled water (mock) or Pst under light (200 μmol m-2 s-1) or dark conditions. (B) The H2O2 content in tobacco leaves at 3 dpi of Pst under light or dark conditions. Means ± SE of six replicates are presented. Different letters above the columns indicate significant differences between treatments at P < 0.05.
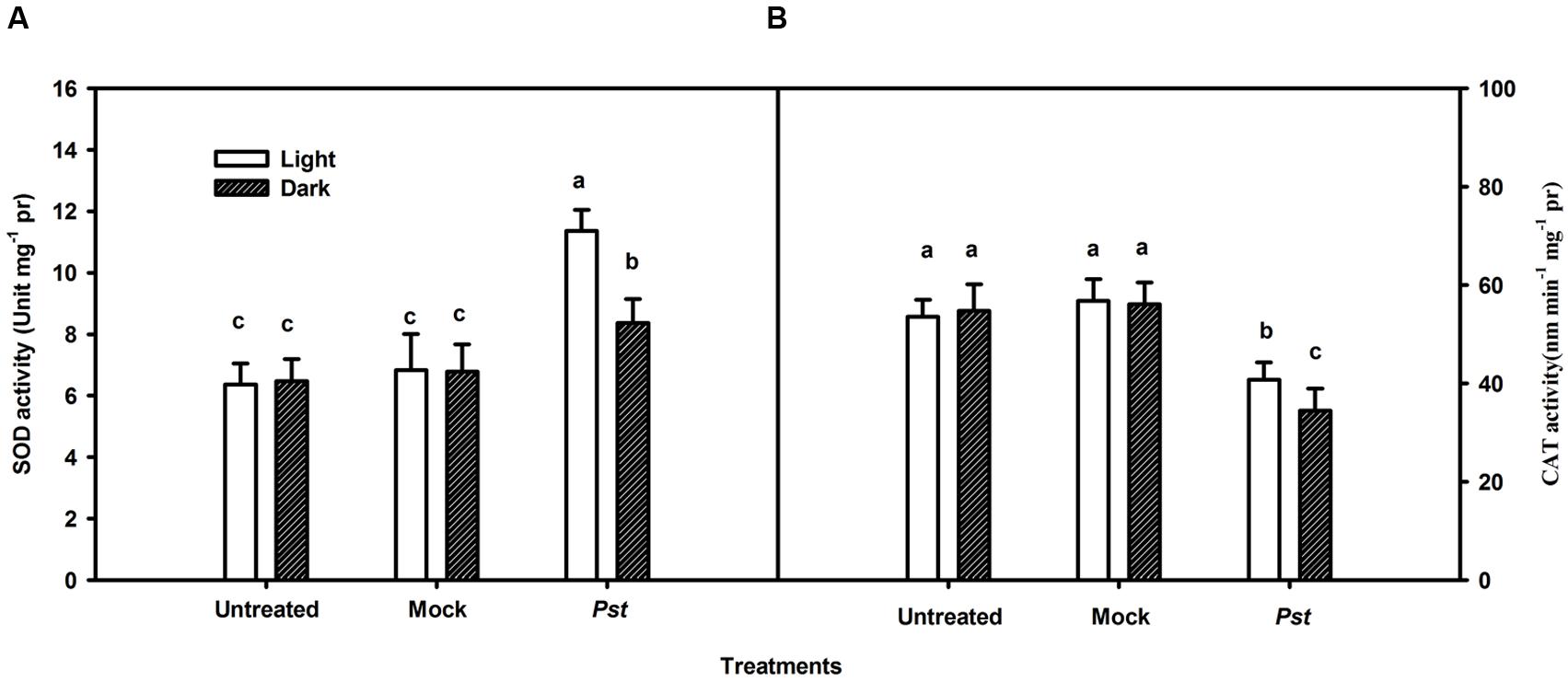
FIGURE 6. Total cellular superoxide dismutase (A) and catalase (B) activities in tobacco leaves after Pst infection. Means ± SE of three replicates are presented. Different letters above the columns indicate significant differences between treatments at P < 0.05.
The Effect of Exogenous H2O2 Pretreatment on the Bacterial Population in Tobacco Leaves after Pst Infection under Dark Conditions
To successfully induce further H2O2 accumulation after Pst infection under dark conditions, exogenous H2O2 was uniformly sprayed onto tobacco leaves immediately prior to Pst infiltration. This H2O2 pretreatment alleviated chlorotic lesions and decreased the Pst population at 3 dpi under dark conditions (Figure 7).
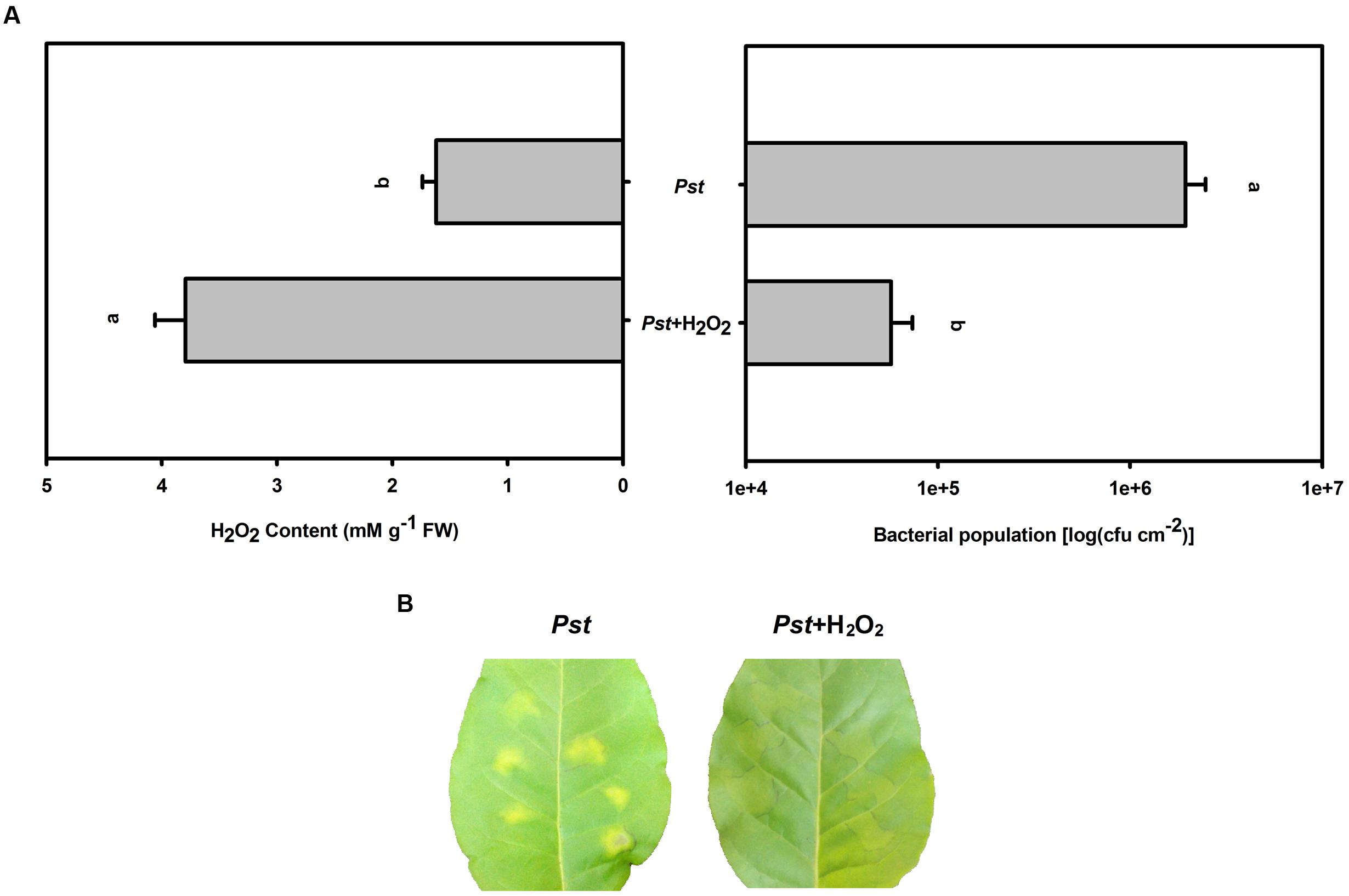
FIGURE 7. The effect of H2O2 pretreatment on the bacterial population after Pst infection in tobacco leaves under dark conditions. (A) H2O2 accumulation and the Pst population in tobacco leaves after pretreatment with exogenous H2O2 prior to Pst infiltration under dark conditions. Means ± SE of six replicates are presented. Different letters above the columns indicate significant differences between treatments at P < 0.05. (B) Representative images of changes in tobacco leaves pretreated with exogenous H2O2 at 3 dpi under dark conditions.
The Effect of MV or DCMU Pretreatment on the Bacterial Population in Tobacco Leaves after Pst Infection under Light Conditions
During illumination, MV preferentially accepts electrons from PSI and donates them to molecular oxygen, generating superoxide, which rapidly dismutates to H2O2 and ultimately results in H2O2 accumulation in chloroplasts (Halliwell, 1981). The herbicide DCMU is an inhibitor of electron transport from the primary quinone electron acceptor to the secondary quinone electron acceptor in PSII, which can decrease H2O2 production in chloroplasts (Marshall et al., 2002). To assess whether the size of the Pst population depends on the degree of H2O2 accumulation under light conditions, MV or DCMU pretreatment was used to increase or decrease H2O2 accumulation in tobacco leaves after Pst infection, respectively. MV pretreatment alleviated chlorotic lesions and decreased the Pst population at 3 dpi (Figure 8), whereas DCMU pretreatment aggravated chlorotic lesions and increased the Pst population (Figure 8).
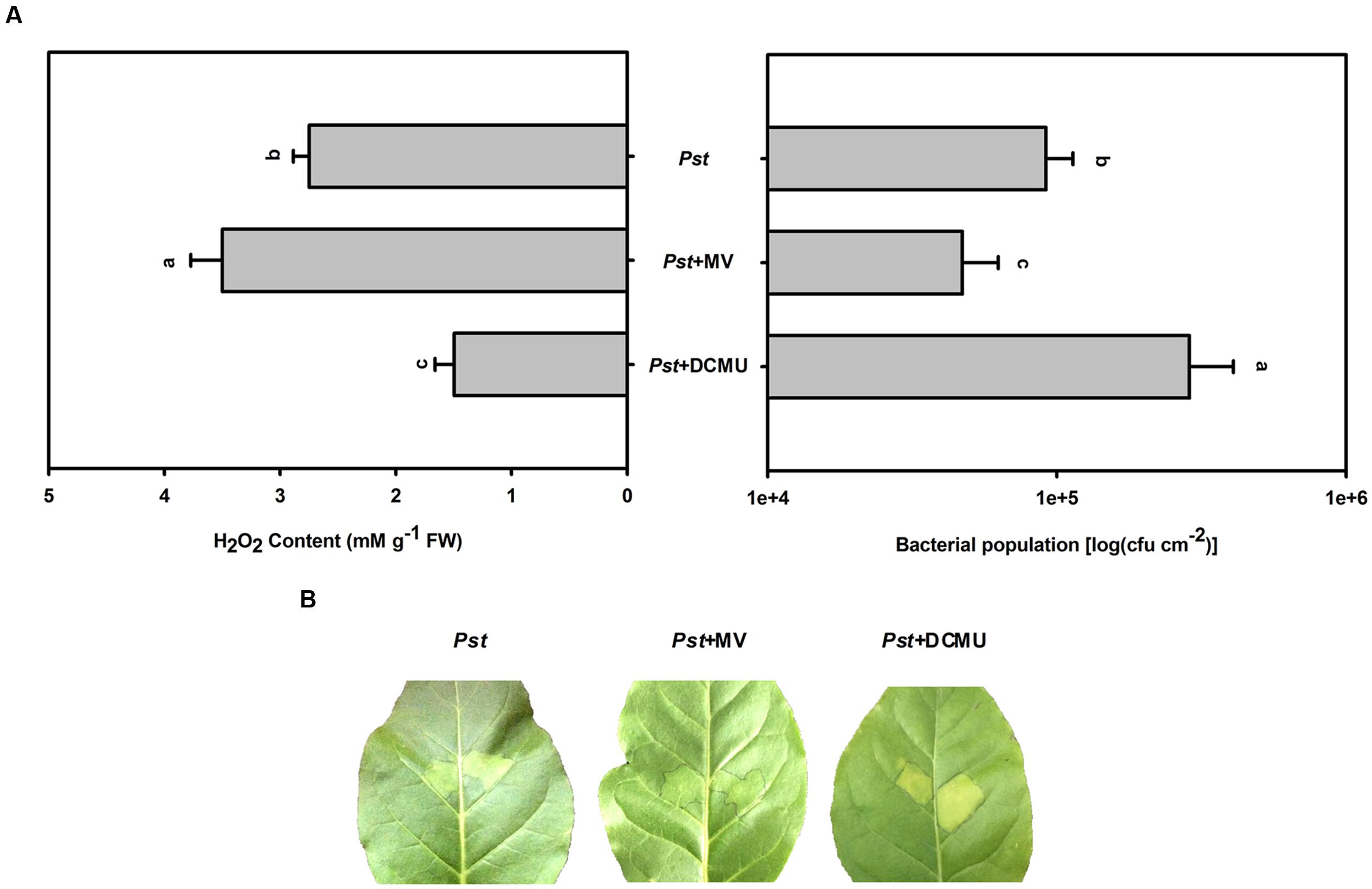
FIGURE 8. The effect of MV or DCMU pretreatment on H2O2 accumulation and the Pst population after Pst infection in tobacco leaves under light conditions. (A) The H2O2 accumulation and the Pst population in tobacco leaves after pretreatment with MV or DCMU prior to Pst infiltration under light conditions (200 μmol m-2 s-1). Means ± SE of six replicates are presented. Different letters above the columns indicate significant differences between treatments at P < 0.05. (B) Representative images of changes in tobacco leaves pretreated with MV or DCMU at 3 dpi under light conditions.
Discussion
In this study, we evaluated the role of light in Pst multiplication during the tobacco–Pst interaction in terms of its impact on ROS production. Less damage to the photosynthetic apparatus and reduced bacterial abundance were observed at 3 dpi under light conditions compared to darkness (Figures 2 and 3). Therefore, it is reasonable to speculate that the attenuation of damage to the photosynthetic apparatus resulted from the smaller bacterial population size observed at 3 dpi under light conditions. There are reports that light-mediated or light-facilitated signaling may play a key role in the defense response of plants to pathogens (Kangasjärvi et al., 2012). For example, Chandra-Shekara et al. (2006) revealed that resistance to turnip crinkle virus and tobacco mosaic virus in Arabidopsis and tobacco, respectively, is influenced by light. Pennypacker (2000) showed that reduced light led to increases in Sclerotinia sclerotiorum infection in soybean (Glycine max) and Verticillium alboatrum. Leone et al. (2014) reported that light plays an important role in regulating the trade-off between growth and defense that occurs in the shadow avoidance syndrome. The findings of host–pathogen interaction studies using Arabidopsis or rice (Oryza sativa) and bacteria also support the view that light-mediated or light-facilitated signaling is required for resistance (Guo et al., 1993; Genoud et al., 2002; Zeier et al., 2004). Moreover, light is required for SA biosynthesis and controls SA perception (Griebel and Zeier, 2008), and SA-associated defenses are effective at restricting invasion by biotrophic and hemibiotrophic pathogens (Glazebrook, 2005). Thus, light-controlled activation of the responses mentioned above is maybe one of the reasons of the reduced damage induced by Pst infection under light conditions.
As it is for plants, light is also a dominant environmental component of certain acclimatory and developmental processes in many pathogens. P. syringae pv. tabaci has three photosensory proteins and two bacteriophytochromes that serve as photoreceptors for visible light (Mandalari et al., 2013; Wu et al., 2013). Therefore, light may have a direct effect on Pst multiplication which ultimately led to the smaller bacterial population at 3 dpi in tobacco leaves under light conditions (Figure 3). However, no difference in the Pst population was observed between light and dark conditions after 3 days of cultivation in the absence of leaf tissue (Figure 4), indicating that the smaller bacterial population observed in leaves under light conditions was not caused by a direct effect of light on Pst growth.
Several reports have suggested that ROS accumulation is involved in plant defense responses (Murata et al., 2007). The direct reduction of oxygen to superoxide by reduced donors associated with PSI occurs during the Mehler reaction (Asada, 1999), and the photosystems of plant leaves are likely to be affected by ROS accumulation during stress (Takahashi and Murata, 2008; Mur et al., 2010). The observed reduced photosystem damage and the greater degree of H2O2 accumulation under light conditions compared to darkness (Figures 2 and 5) indicated that H2O2 accumulation was not the main reason for the photosynthetic apparatus damage induced by Pst infection in tobacco leaves. Besides, PSI attack by ROS occurs only if iron-sulfur centers can be maintained in a reduced state, which requires visible light (Sonoike et al., 1997). However, greater damage to PSI occurred in the dark, further supporting the viewpoint mentioned above. During plant–pathogen interactions, both the pathogen and the plant photosynthetic apparatus can be affected by ROS accumulation. Indeed, it has been reported that a greater abundance of H2O2 may lead to increased production of highly reactive hydroxyl free radicals via the Fenton reaction, with a toxic effect on the pathogen (de Torres Zabala et al., 2015). A smaller Pst population and a greater H2O2 accumulation were observed at 3 dpi in tobacco leaves under light conditions (Figures 3 and 5). Overall, The results indicated that greater H2O2 accumulation in the light after Pst infection in tobacco leaves led to a reduced impact of Pst on the photosynthetic apparatus, the mechanism of which may be associated with the increased generation of OH⋅ due to abundant H2O2.
To verify whether light suppresses the Pst population in tobacco leaves through H2O2 accumulation or a direct effect of the light itself, exogenous H2O2 was used to further increase the H2O2 content after Pst infection under dark conditions. The results showed that exogenous H2O2 pretreatment alleviated chlorotic lesions and decreased the Pst population at 3 dpi (Figure 7). In addition, MV or DCMU pretreatment was used to further increase or partially inhibit H2O2 accumulation in tobacco leaves after Pst infection under light conditions. We found that MV pretreatment alleviated chlorotic lesions and decreased the Pst population in the infiltrated zone at 3 dpi, whereas DCMU pretreatment aggravated chlorotic lesions and increased the Pst population (Figure 8). The results indicated that the chlorotic symptoms and the Pst population are negatively correlated with H2O2 accumulation; light itself had no effect, yet light did suppress the Pst population in tobacco leaves via H2O2 accumulation during infection.
In this study, light was observed to play an important role in the tobacco defense system by suppressing the Pst population through H2O2 accumulation. However, Zurbriggen et al. (2009) reported that transgenic tobacco plants are unable to generate high levels of ROS in chloroplasts and display attenuated cell death upon infection by the non-host pathogen Xanthomonas campestris. Thus, further studies are needed to thoroughly understand the mechanism of the effect of light on tobacco–Pst interaction with regard to H2O2.
Author Contributions
D-DC, Z-SZ, G-YS, X-BS, and MZ designed the study. D-DC and M-JL carried out most of the experiments and data analysis. D-DC, Z-SZ, WC, and Y-BH wrote the paper. All authors read and approved the final manuscript.
Funding
This work was supported by the Fundamental Research Funds for the Central Universities (No. 2572014AA18), National Nature Science Foundation of China (No. 31070307) and the Outstanding Academic Leaders for Innovation Talents of Science and Technology of Harbin City in Heilongjiang Province (No. 2013RFXXJ063).
Conflict of Interest Statement
The authors declare that the research was conducted in the absence of any commercial or financial relationships that could be construed as a potential conflict of interest.
The reviewer JN and handling Editor declared their shared affiliation, and the handling Editor states that the process nevertheless met the standards of a fair and objective review
Acknowledgment
We are very grateful to Prof. Hui-Yuan Gao and Dr. Cheng Yang for technical assistance.
Abbreviations
CAT, catalase; cfu, colony forming units; DAB, 3,3′-diaminobenzidine; DCMU, 3-(3,4-dichlorophenyl)-1,1-dimethylurea; dpi, days post inoculation; Fo and Fm, initial and maximum fluorescence; Fv/Fm, maximal quantum yield of PSII; HR, hypersensitive reaction; H2O2, hydrogen peroxide; MR820 nm, modulated reflected signal of 820 nm; MV, methyl viologen; PRI, the photochemical reflectance index; PSI, photosystem I; PSII, photosystem II; Pst, Pseudomonas syringae pv. tabaci; Pto, Pseudomonas syringae pv. tomato DC3000; ROS, reactive oxygen species; SA, salicylic acid; SOD, superoxide dismutase.
References
Aebi, H. (1984). Catalase in vitro. Methods Enzymol. 105, 121–126. doi: 10.1016/S0076-6879(84)05016-3
Aro, E. M., Suorsa, M., Rokka, A., Allahverdiyeva, Y., Paakkarinen, V., Saleem, A., et al. (2005). Dynamics of photosystem II: a proteomic approach to thylakoid protein complexes. J. Exp. Bot. 56, 347–356. doi: 10.1093/jxb/eri041
Asada, K. (1999). The water-water cycle in chloroplasts: scavenging of active oxygens and dissipation of excess photons. Annu. Rev. Plant Biol. 50, 601–639. doi: 10.1146/annurev.arplant.50.1.601
Berger, S., Benediktyová, Z., Matouš, K., Bonfig, K., Mueller, M. J., Nedbal, L., et al. (2007). Visualization of dynamics of plant–pathogen interaction by novel combination of chlorophyll fluorescence imaging and statistical analysis: differential effects of virulent and avirulent strains of P. syringae and of oxylipins on A. thaliana. J. Exp. Bot. 58, 797–806. doi: 10.1093/jxb/erl208
Bonfig, K. B., Schreiber, U., Gabler, A., Roitsch, T., and Berger, S. (2006). Infection with virulent and avirulent P. syringae strains differentially affects photosynthesis and sink metabolism in Arabidopsis leaves. Planta 225, 1–12. doi: 10.1007/s00425-006-0303-3
Bostock, R. M., Pye, M. F., and Roubtsova, T. V. (2014). Predisposition in plant disease: exploiting the nexus in abiotic and biotic stress perception and response. Annu. Rev. Phytopathol. 52, 517–549. doi: 10.1146/annurev-phyto-081211-172902
Bradford, M. M. (1976). A rapid and sensitive method for the quantitation of microgram quantities of protein utilizing the principle of protein-dye binding. Anal. Biochem. 72, 248–254. doi: 10.1016/0003-2697(76)90527-3
Cerrudo, I., Keller, M. M., Cargnel, M. D., Demkura, P. V., de Wit, M., Patitucci, M. S., et al. (2012). Low red/far-red ratios reduce Arabidopsis resistance to Botrytis cinerea and jasmonate responses via a COI1-JAZ10-dependent, salicylic acid-independent mechanism. Plant Physiol. 158, 2042–2052. doi: 10.1104/pp.112.193359
Chandra-Shekara, A. C., Gupte, M., Navarre, D., Raina, S., Raina, R., Klessig, D., et al. (2006). Light-dependent hypersensitive response and resistance signaling against Turnip Crinkle Virus in Arabidopsis. Plant J. 45, 320–334. doi: 10.1111/j.1365-313X.2005.02618.x
Crous, K. Y., Zaragoza-Castells, J., Ellsworth, D. S., Duursma, R. A., Loew, M., Tissue, D. T., et al. (2012). Light inhibition of leaf respiration in field-grown Eucalyptus saligna in whole-tree chambers under elevated atmospheric CO2 and summer drought. Plant Cell Environ. 35, 966–981. doi: 10.1111/j.1365-3040.2011.02465.x
De Frenne, P., Rodríguez-Sánchez, F., De Schrijver, A., Coomes, D. A., Hermy, M., Vangansbeke, P., et al. (2015). Light accelerates plant responses to warming. Nature Plants 1:15110. doi: 10.1038/nplants.2015.110
de Torres Zabala, M., Littlejohn, G., Jayaraman, S., Studholme, D., Bailey, T., Lawson, T., et al. (2015). Chloroplasts play a central role in plant defence and are targeted by pathogen effectors. Nature Plants 1, 15074. doi: 10.1038/nplants.2015.74
Delprato, M. L., Krapp, A. R., and Carrillo, N. (2015). Green light to plant responses to pathogens: the role of chloroplast light-dependent signaling in biotic stress. Photochem. Photobiol. 91, 1004–1011. doi: 10.1111/php.12466
Evain, S., Flexas, J., and Moya, I. (2004). A new instrument for passive remote sensing:2. Measurement of leaf and canopy reflectance changes at 531 nm and their relationship with photosynthesis and chlorophyll fluorescence. Remote Sens. Environ. 91, 175–185. doi: 10.1016/j.rse.2004.03.012
Fleury, C., Mignotte, B., and Vayssière, J. L. (2002). Mitochondrial reactive oxygen species in cell death signaling. Biochimie 84, 131–141. doi: 10.1016/S0300-9084(02)01369-X
Foyer, C. H., and Noctor, G. (2009). Redox regulation in photosynthetic organisms: signaling, acclimation, and practical implications. Antioxid. Redox Signal. 11, 861–905. doi: 10.1089/ars.2008.2177
Galvez-Valdivieso, G., and Mullineaux, P. M. (2010). The role of reactive oxygen species in signalling from chloroplasts to the nucleus. Physiol. Plant 138, 430–439. doi: 10.1111/j.1399-3054.2009.01331.x
Gao, J., Li, P., Ma, F., and Goltsev, V. (2014). Photosynthetic performance during leaf expansion in Malus micromalus probed by chlorophyll a fluorescence and modulated 820 nm reflection. J. Photochem. Photobiol. B Biol. 137, 144–150. doi: 10.1016/j.jphotobiol.2013.12.005
Genoud, T., Buchala, A. J., Chua, N. H., and Métraux, J. P. (2002). Phytochrome signalling modulates the SA-perceptive pathway in Arabidopsis. Plant J. 31, 87–95. doi: 10.1046/j.1365-313X.2002.01338.x
Giannopolitis, C. N., and Ries, S. K. (1977). Superoxide dismutases I. Occurrence in higher plants. Plant Physiol. 59, 309–314. doi: 10.1104/pp.59.2.309
Glazebrook, J. (2005). Contrasting mechanisms of defense against biotrophic and necrotrophic pathogens. Annu. Rev. Phytopathol. 43, 205–227. doi: 10.1146/annurev.phyto.43.040204.135923
Griebel, T., and Zeier, J. (2008). Light regulation and daytime dependency of inducible plant defenses in Arabidopsis: phytochrome signaling controls systemic acquired resistance rather than local defense. Plant Physiol. 147, 790–801. doi: 10.1104/pp.104.900263
Guo, A., Reimers, P. J., and Leach, J. E. (1993). Effect of light on incompatible interactions between Xanthomonas oryzae pv oryzae and rice. Physiol. Mol. Plant Pathol. 42, 413–425. doi: 10.1006/pmpp.1993.1031
Halliwell, B. (1981). Chloroplast Metabolism. The Structure and Function of Chloroplasts in Green Leaf Cells. Oxford: Clarendon Press.
Hann, D. R., and Rathjen, J. P. (2007). Early events in the pathogenicity of Pseudomonas syringae on Nicotiana benthamiana. Plant J. 49, 607–618. doi: 10.1111/j.1365-313X.2006.02981.x
Hua, J. (2013). Modulation of plant immunity by light, circadian rhythm, and temperature. Curr. Opin. Plant Biol. 16, 406–413. doi: 10.1016/j.pbi.2013.06.017
Jia, Y. J., Cheng, D. D., Wang, W. B., Gao, H. Y., Liu, A. X., Li, X. M., et al. (2010). Different enhancement of senescence induced by metabolic products of Alternaria alternata in tobacco leaves of different ages. Physiol. Plant. 138, 164–175. doi: 10.1111/j.1399-3054.2009.01300.x
Kangasjärvi, S., Neukermans, J., Li, S., Aro, E. M., and Noctor, G. (2012). Photosynthesis, photorespiration, and light signalling in defence responses. J. Exp. Bot. 63, 1619–1636. doi: 10.1093/jxb/err402
Kangasjärvi, S., Nurmi, M., Tikkanen, M., and Aro, E. M. (2009). Cell-specific mechanisms and systemic signalling as emerging themes in light acclimation of C3 plants. Plant Cell Environ. 32, 1230–1240. doi: 10.1111/j.1365-3040.2009.01982.x
Katagiri, F., Thilmony, R., and He, S. Y. (2002). The Arabidopsis thaliana-Pseudomonas syringae interaction. Arabidopsis book 1:e0039. doi: 10.1199/tab.0039
King, E. O., Ward, M. K., and Raney, D. E. (1954). Two simple media for the demonstration of pyocyanin and fluorescin. J. Lab. Clin. Med. 44, 301–307.
Lee, S., Yang, D. S., Uppalapati, S. R., Sumner, L. W., and Mysore, K. S. (2013). Suppression of plant defense responses by extracellular metabolites from Pseudomonas syringae pv. tabaci in Nicotiana benthamiana. BMC Plant Biol. 13:65. doi: 10.1186/1471-2229-13-65
Leone, M., Keller, M. M., Cerrudo, I., and Ballarë, C. L. (2014). To grow or defend? Low red: far-red ratios reduce jasmonate sensitivity in Arabidopsis seedlings bypromoting DELLA degradation and increasing JAZ10 stability. New Phytol. 204, 355–367. doi: 10.1111/nph.12971
Li, Z., Wakao, S., Fischer, B. B., and Niyogi, K. K. (2009). Sensing and responding to excess light. Annu. Rev. Plant Biol 60, 239–260. doi: 10.1146/annurev.arplant.58.032806.103844
Mandalari, C., Losi, A., and Gärtner, W. (2013). Distance-tree analysis, distribution and co-presence of bilin-and flavin-binding prokaryotic photoreceptors for visible light. Photochem. Photobiol. Sci. 12, 1144–1157. doi: 10.1039/C3PP25404F
Marshall, J. A., Hovenden, M., Oda, T., and Hallegraeff, G. M. (2002). Photosynthesis does influence superoxide production in the ichthyotoxic alga Chattonella marina (Raphidophyceae). J. Plankton Res. 24, 1231–1236. doi: 10.1093/plankt/24.11.1231
Miller, G., Suzuki, N., Ciftci-Yilmaz, S., and Mittler, R. (2010). Reactive oxygen species homeostasis and signalling during drought and salinity stresses. Plant Cell Environ. 33, 453–467. doi: 10.1111/j.1365-3040.2009.02041.x
Mittler, R., Vanderauwera, S., Suzuki, N., Miller, G., Tognetti, V. B., Vandepoele, K., et al. (2011). ROS signaling: the new wave? Trends Plant Sci. 16, 300–309. doi: 10.1016/j.tplants.2011.03.007
Mühlenbock, P., Szechyńska-Hebda, M., Płaszczyca, M., Baudo, M., Mateo, A., Mullineaux, P. M., et al. (2008). Chloroplast signaling and LESION SIMULATING DISEASE1 regulate crosstalk between light acclimation and immunity in Arabidopsis. Plant Cell 20, 2339–2356. doi: 10.1105/tpc.108.059618
Mur, L. A., Aubry, S., Mondhe, M., Kingston-Smith, A., Gallagher, J., Timms-Taravella, E., et al. (2010). Accumulation of chlorophyll catabolites photosensitizes the hypersensitive response elicited by Pseudomonas syringae in Arabidopsis. New Phytol. 188, 161–174. doi: 10.1111/j.1469-8137.2010.03377.x
Murata, N., Takahashi, S., Nishiyama, Y., and Allakhverdiev, S. I. (2007). Photoinhibition of photosystem II under environmental stress. Biochim. Biophys. Acta 1767, 414–421. doi: 10.1016/j.bbabio.2006.11.019
Oukarroum, A., Goltsev, V., and Strasser, R. J. (2013). Temperature effects on pea plants probed by simultaneous measurements of the kinetics of prompt fluorescence, delayed fluorescence and modulated 820 nm reflection. PLoS ONE 8:e59433. doi: 10.1371/journal.pone.0059433
Patterson, B. D., MacRae, E. A., and Ferguson, I. B. (1984). Estimation of hydrogen peroxide in plant extracts using titanium (IV). Anal. Biochem. 139, 487–492. doi: 10.1016/0003-2697(84)90039-3
Pennypacker, B. W. (2000). Differential impact of carbon assimilation on the expression of quantitative and qualitative resistance in alfalfa (Medicago sativa). Physiol. Mol. Plant Pathol. 57, 87–93. doi: 10.1006/pmpp.2000.0284
Pérez-Bueno, M. L., Pineda, M., Díaz-Casado, E., and Barón, M. (2015). Spatial and temporal dynamics of primary and secondary metabolism in Phaseolus vulgaris challenged by Pseudomonas syringae. Physiol. Plant 153, 161–174. doi: 10.1111/ppl.12237
Porra, R. J. (2002). The chequered history of the development and use of simultaneous equations for the accurate determination of chlorophylls a and b. Photosynth. Res. 73, 149–156. doi: 10.1007/1-4020-3324-9_56
Ramegowda, V., Senthil-Kumar, M., Ishiga, Y., Kaundal, A., Udayakumar, M., and Mysore, K. S. (2013). Drought stress acclimation imparts tolerance to Sclerotinia sclerotiorum and Pseudomonas syringae in Nicotiana benthamiana. Int. J. Mol. Sci. 14, 9497–9513. doi: 10.3390/ijms14059497
Roberts, M. R., and Paul, N. D. (2006). Seduced by the dark side: integrating molecular and ecological perspectives on the influence of light on plant defence against pests and pathogens. New Phytol. 170, 677–699. doi: 10.1111/j.1469-8137.2006.01707.x
Rodríguez-Moreno, L., Pineda, M., Soukupová, J., Macho, A. P., Beuzón, C. R., Barón, M., et al. (2008). Early detection of bean infection by Pseudomonas syringae in asymptomatic leaf areas using chlorophyll fluorescence imaging. Photosynth. Res. 96, 27–35. doi: 10.1007/s11120-007-9278-6
Sims, D. A., and Gamon, J. A. (2002). Relationships between leaf pigment content and spectral reflectance across a wide range of species, leaf structures and developmental stages. Remote Sens. Environ. 81, 337–354. doi: 10.1016/S0034-4257(02)00010-X
Sonoike, K., Kamo, M., Hihara, Y., Hiyama, T., and Enami, I. (1997). The mechanism of the degradation of psaB gene product, one of the photosynthetic reaction center subunits of photosystem I, upon photoinhibition. Photosynth. Res. 53, 55–63. doi: 10.1023/A:1005852330671
Strasser, R. J., Tsimilli-Michael, M., Qiang, S., and Goltsev, V. (2010). Simultaneous in vivo recording of prompt and delayed fluorescence and 820-nm reflection changes during drying and after rehydration of the resurrection plant Haberlea rhodopensis. Biochim. Biophys. Acta 1797, 1313–1326. doi: 10.1016/j.bbabio.2010.03.008
Sun, J. P., Sun, H. W., and Yu, Y. F. (2011). Differential host screen for physiologic races of Pseudomonas syringae pv. tabaci in Heilongjiang. Tobacco Sci. Technol. 10, 75–77.
Suzuki, N., Koussevitzky, S., Mittler, R., and Miller, G. (2012). ROS and redox signalling in the response of plants to abiotic stress. Plant Cell Environ. 35, 259–270. doi: 10.1111/j.1365-3040.2011.02336.x
Suzuki, N., Rivero, R. M., Shulaev, V., Blumwald, E., and Mittler, R. (2014). Abiotic and biotic stress combinations. New Phytol. 203, 32–43. doi: 10.1111/nph.12797
Taguchi, F., and Ichinose, Y. (2011). Role of type IV pili in virulence of Pseudomonas syringae pv. tabaci 6605: correlation of motility, multidrug resistance, and HR-inducing activity on a nonhost plant. Mol. Plant-Microbe Interact. 24, 1001–1011. doi: 10.1094/MPMI-02-11-0026
Takahashi, S., and Murata, N. (2008). How do environmental stresses accelerate photoinhibition? Trends Plant Sci. 13, 178–182. doi: 10.1016/j.tplants.2008.01.005
Ueno, M., Shibata, H., Kihara, J., Honda, Y., and Arase, S. (2003). Increased tryptophan decarboxylase and monoamine oxidase activities induce Sekiguchi lesion formation in rice infected with Magnaporthe grisea. Plant J. 36, 215–228. doi: 10.1046/j.1365-313X.2003.01875.x
Weng, J. H., Lai, K. M., Liao, T. S., Hwang, M. Y., and Chen, Y. N. (2009). Relationships of photosynthetic capacity to PSII efficiency and to photochemical reflectance index of Pinus taiwanensis through different seasons at high and low elevations of sub-tropical Taiwan. Trees 23, 347–356. doi: 10.1007/s00468-008-0283-y
Wu, L., McGrane, R. S., and Beattie, G. A. (2013). Light regulation of swarming motility in Pseudomonas syringae integrates signaling pathways mediated by a bacteriophytochrome and a LOV protein. MBio 4:e00334-13. doi: 10.1128/mBio.00334-13
Yang, Y. X., Wang, M. M., Yin, Y. L., Onac, E., Zhou, G. F., Peng, S., et al. (2015). RNA-seq analysis reveals the role of red light in resistance against Pseudomonas syringae pv. tomato DC3000 in tomato plants. BMC Genomics 16:120. doi: 10.1186/s12864-015-1228-7
Zavaleta-Mancera, H. A., López-Delgado, H., Loza-Tavera, H., Mora-Herrera, M., Trevilla-García, C., Vargas-Suárez, M., et al. (2007). Cytokinin promotes catalase and ascorbate peroxidase activities and preserves the chloroplast integrity during dark-senescence. J. Plant Physiol. 164, 1572–1582. doi: 10.1016/j.jplph.2007.02.003
Zeier, J., Pink, B., Mueller, M. J., and Berger, S. (2004). Light conditions influence specific defence responses in incompatible plant–pathogen interactions: uncoupling systemic resistance from salicylic acid and PR-1 accumulation. Planta 219, 673–683. doi: 10.1007/s00425-004-1272-z
Zurbriggen, M. D., Carrillo, N., Tognetti, V. B., Melzer, M., Peisker, M., Hause, B., et al. (2009). Chloroplast-generated reactive oxygen species play a major role in localized cell death during the non-host interaction between tobacco and Xanthomonas campestris pv. vesicatoria. Plant J. 60, 962–973. doi: 10.1111/j.1365-313X.2009.04010.x
Keywords: light, Nicotiana tabacum, photosystems, Pseudomonas syringae pv. tabaci, reactive oxygen species
Citation: Cheng D-D, Liu M-J, Sun X-B, Zhao M, Chow WS, Sun G-Y, Zhang Z-S and Hu Y-B (2016) Light Suppresses Bacterial Population through the Accumulation of Hydrogen Peroxide in Tobacco Leaves Infected with Pseudomonas syringae pv. tabaci. Front. Plant Sci. 7:512. doi: 10.3389/fpls.2016.00512
Received: 28 December 2015; Accepted: 31 March 2016;
Published: 21 April 2016.
Edited by:
Wim Van den Ende, KU Leuven, BelgiumReviewed by:
Łukasz Paweł Tarkowski, KU Leuven, BelgiumIsolde Francis, California State University, Bakersfield, USA
Jenny Neukermans, Vlaams Instituut voor Biotechnologie – KU Leuven, Belgium
Copyright © 2016 Cheng, Liu, Sun, Zhao, Chow, Sun, Zhang and Hu. This is an open-access article distributed under the terms of the Creative Commons Attribution License (CC BY). The use, distribution or reproduction in other forums is permitted, provided the original author(s) or licensor are credited and that the original publication in this journal is cited, in accordance with accepted academic practice. No use, distribution or reproduction is permitted which does not comply with these terms.
*Correspondence: Guang-Yu Sun, sungy@vip.sina.com; Zi-Shan Zhang, zhangzishantaian@163.com
†These authors have contributed equally to this work.