- 1School of Life Sciences, Institute of Ecology and Biodiversity, Shandong University, Qingdao, China
- 2Key Laboratory of Agro-Forestry Environmental Processes and Ecological Regulation of Hainan Province, Hainan University, Haikou, China
- 3Hainan Ecological Environment Monitoring Center, Haikou, China
- 4Department of Pharmaceutical Science, Changzhi Medical College, Changzhi, China
- 5Shandong Provincial Engineering and Technology Research Center for Vegetation Ecology, Shandong University, Qingdao, China
- 6Qingdao Forest Ecology Research Station of National Forestry and Grassland Administration, Shandong University, Qingdao, China
It has widely been documented that nitrogen (N) enrichment stimulates plant growth and modifies plant functional traits in the terrestrial ecosystem. However, it remains unclear whether there are critical transitions or tipping points for the response of plant growth or traits to N enrichment, and how these responses differ to different N forms. We chose the native, perennial clonal grass, Leymus chinensis in Inner Mongolia steppe, and conducted a field experiment, in which six N addition rates (0, 2, 5, 10, 20, and 50 g N m–2 year–1) and five N compound types [NH4NO3, (NH4)2SO4, NH4HCO3, CO(NH2)2, slow-release CO(NH2)2] are considered. Here, we found that the different N compound types had no significant effect on the growth of L. chinensis. N addition rate significantly increased plant aboveground biomass and leaf nitrogen concentration, whereas decreased leaf dry matter content. The tipping point for N-induced aboveground biomass increase was at 10 g N m–2 year–1, and the changes in functional traits were at N addition rates of 20 g N m–2 year–1. Our findings suggested that the responses of aboveground biomass and functional traits to N addition were asymmetric, in which responses in aboveground biomass were more sensitive than that in functional traits. The differential sensitivity of aboveground biomass and functional traits of L. chinensis occurred to N deposition highlights the importance of functional traits in mediating ecosystem functioning in the face of N deposition, regardless of which chemical forms dominate in the deposited N.
Introduction
Nitrogen (N) is a major limiting resource for plant growth in diverse natural ecosystems (Vitousek and Howarth, 1991; Elser et al., 2007) and plays a crucial role in photosynthesis and all enzymatic activities (Reich et al., 2004). Human activities such as fossil fuel combustion and fertilizer application have dramatically increased reactive N being deposited into many terrestrial ecosystems (Galloway et al., 2008). There likely exist ecosystem-specific thresholds for N enrichment, beyond which ecosystem start to transit substantially, such as the critical threshold for N-induced species loss to mature Eurasian grasslands is 1.75 g N m–2 year–1 (Bai et al., 2010). An ecological threshold or tipping point is the point at which there is an abrupt change in an ecosystem quality, property, or phenomenon (Groffman et al., 2006). Identifying ecological thresholds in response to climate change such as N deposition is important to guide ecosystem management and maintain ecological services (Rockström et al., 2009).
Plant functional traits reflect plants’ capacities for resource capture and adaptations to environmental changes (Wright et al., 2004). As plant economic spectrum predicts, species with the traits capable of acquiring resource rapidly (e.g., high N concentration, specific leaf area, and photosynthetic rate) would compete for resource more strongly under N enrichment (Wright et al., 2004; La Pierre and Smith, 2015). Recent studies have revealed inconsistent responses of plant functional traits to nutrient addition, while some studies showed that N addition enhanced leaf N concentration, chlorophyll concentration and specific leaf area, thus leading to a marked increase in photosynthetic rates (Tatarko and Knops, 2018; Zheng et al., 2018; Sun et al., 2022). Others found no effect of N addition on leaf mass area, area-based concentrations of foliar N and chlorophyll concentrations (Hu and Wan, 2019). One possible explanation for the inconsistency among these studies is that the response of plant functional traits to N enrichment may be non-linear (Zhang et al., 2019; Song et al., 2022), such that plant functional traits may not necessarily show a significant change until a threshold N level is reached. Nevertheless, it remains unclear whether there is tipping point for the effect of N inputs on plant traits. Moreover, plasticity in plant traits is predicted to play a key role in affecting plant production. For example, the study conducted in the central French alpine grassland found that the productivity was positively associated with fertilization-induced increases in plant height and leaf area (Lavorel and Grigulis, 2012). Another study conducted in an Chinese alpine steppe found that increasing leaf N content and leaf area under N addition significantly promoted plant community productivity (Peng et al., 2017; Zhang et al., 2021). However, to date, very few studies have considered whether plant traits can be associated with the response of plant production to increased N inputs.
In addition to plant functional traits, N enrichment could influence plant growth and productivity by changing soil environmental conditions such as soil N availability and acidification (Bobbink et al., 1998). Increasing soil N availability was the key direct factor driving the plant growth under N addition (Tao et al., 2022). In addition, N deposition can result in soil acidification both directly as a result of acid deposition (nitric acid) and indirectly through processes and reactions in soil and water (Stevens et al., 2010), which results in toxicity through mobilization of metals, leaching of base cations and changing the balance between nitrogenous compounds, and hence causes cascading effects on plant growth (Roem et al., 2002; Stevens et al., 2010).
In this study, we investigated the plant functional traits in a multi-level (0, 2, 5, 10, 20, and 50 g N m–2 year–1) N addition experiment in a temperate grassland in Inner Mongolia, China, to test whether there will be a N threshold for plant functional traits in response to N deposition. The temperate grassland in our study site is dominated by a native perennial rhizomatous grass Leymus chinensis, which is palatable for grazing animals and has high forage value and drought tolerance (Bai et al., 2004; Huang et al., 2015). Here, L. chinensis was chosen as a model plant, we hypothesized that (a) when the N addition level reaches a certain level, aboveground biomass and plant functional traits will cross the critical threshold and show dramatic response, and the threshold will be at similar N addition rate for aboveground biomass and traits, (b) changes in traits and soil conditions will together drive the response of plant production to increasing N addition.
Materials and methods
Study site
The experiment was conducted at the Erguna Forest-Steppe Ecotone Research Station (N50°10’46.1′′, E119°22’56.4′′), Institute of Applied Ecology, the Chinese Academy of Sciences. The grassland had been used for forage harvest before 2013. The long-term mean annual precipitation of the site is 363 mm and the mean annual temperature is -2.45°C (1957–2016). The soil is classified as chernozem according to the Food and Agricultural Organization of the United Nations classification. The dominant species in this ecosystem is Leymus chinensis, which makes up > 45.6% of the total aboveground biomass.
Experimental design
The N addition experiment was started in 2014, with a randomized complete block design to study the effects of rates and types [NH4NO3, (NH4)2SO4, NH4HCO3, CO(NH2)2, slow-release CO(NH2)2] of N addition on plant biomass and leaf functional traits. There were six N addition rates (i.e., 0, 2, 5, 10, 20, and 50 g N m–2 year–1), and five types of N compounds. Each of the 30 treatments had eight replications, leading to 240 treatment plots in total. The area of each plot was 10 m × 10 m. Nitrogen fertilizers were added annually since 2014, in late May. Fertilizers were mixed with sand (because of the low amount of added fertilizer at low addition rates) and added uniformly by hand. Sand was sieved through less than 2 mm in size, washed in water, and then heated at nearly 250°C for 60 min in an iron pan. To avoid potentially confounding effects, all plots received the same amount of sand (0.5 kg per plot).
Field sampling and measurement
At each plot, 15 healthy and mature plant individuals were randomly selected from different locations between 1st and 5th August, 2015. These plant individuals were clipped at the ground level and immediately placed in a portable cooler. In the laboratory, all samples were soaked in water for 6 h to ensure full rehydration. Each leaf was then cut from the stem and gently dried with tissue paper before measurement. Water-saturated leaf mass was weighed, scanned the leaf with a scanner (Canon LiDE120), and determined the leaf area by the ImageJ software. All leaves were then dried for 48 h at 65°C and then weighed. The leaf dry matter content (LDMC), specific leaf area (SLA), and stem: leaf ratio were calculated. After being ground in a ball mill (Retsch MM 400; Retsch, Haan, Germany), the leaf total N concentration was analyzed on an elemental analyzer (Vario MACRO cube, Elementar Analysensysteme, Germany). The grinded leaf samples (50 mg) were digested with H2SO4–H2O2 (Bennett et al., 2002), and measured colorimetrically for leaf P concentration at 880 nm after reaction with molybdenum blue. N: P ratio was reported as mass ratios.
Aboveground biomass of L. chinensis was sampled between August 10th and 20th by clipping plants at the soil surface in a 1 m × 1 m quadrat, which was randomly placed in each plot without a spatial overlap of quadrats among different years and at least 50 cm inside the border of each plot to avoid edge effects. All plant materials were oven-dried at 65°C for 48 h and weighed. After clipping above-ground biomass, three soil cores (0–5 cm depth and 50 cm apart) were collected using a 7 cm diameter soil auger adjacent to each aboveground plant sample plot and mixed in situ into one composite sample. Fresh soil samples were sieved through a 2-mm sieve to remove visible roots, plant residues, and stones, and taken to the laboratory for analysis of soil water content, soil inorganic N and pH. Fresh soil was extracted by 2 M KCl solution, 10 g of soil was extracted in 50 mL of 2 M KCl solution, and then inorganic N concentrations were analyzed with a FLAstar 5000 Analyzer (Foss Tecator, Hillerød, Denmark). Subsamples were air−dried and analyzed soil pH using a pH meter (Thermo Fisher Scientific, America).
Statistical analysis
Linear mixed effect model analysis of variance was performed using the lme function from the nlme package with N addition rate and N compounds type as fixed factors and block as a random factor. Given N compounds showed no significant effect on plant biomass and functional traits (Table 1), we pooled data from different N compounds under a certain N addition rate to simplify our analysis and focus on the effect of N addition rate. We used Duncan’s test to evaluate aboveground biomass, SLA, LDMC, stem: leaf ratio, leaf N concentration, P concentration, leaf N: P ratio of Leymus chinensis, and SIN, soil pH and SWC under varying N addition rates. To examine how aboveground biomass of Leymus chinensis was correlated with SIN or soil pH across different N addition rate, a linear regression model was performed. All analyses were conducted using R version 4.0.2 (R Development Core Team, 2020).
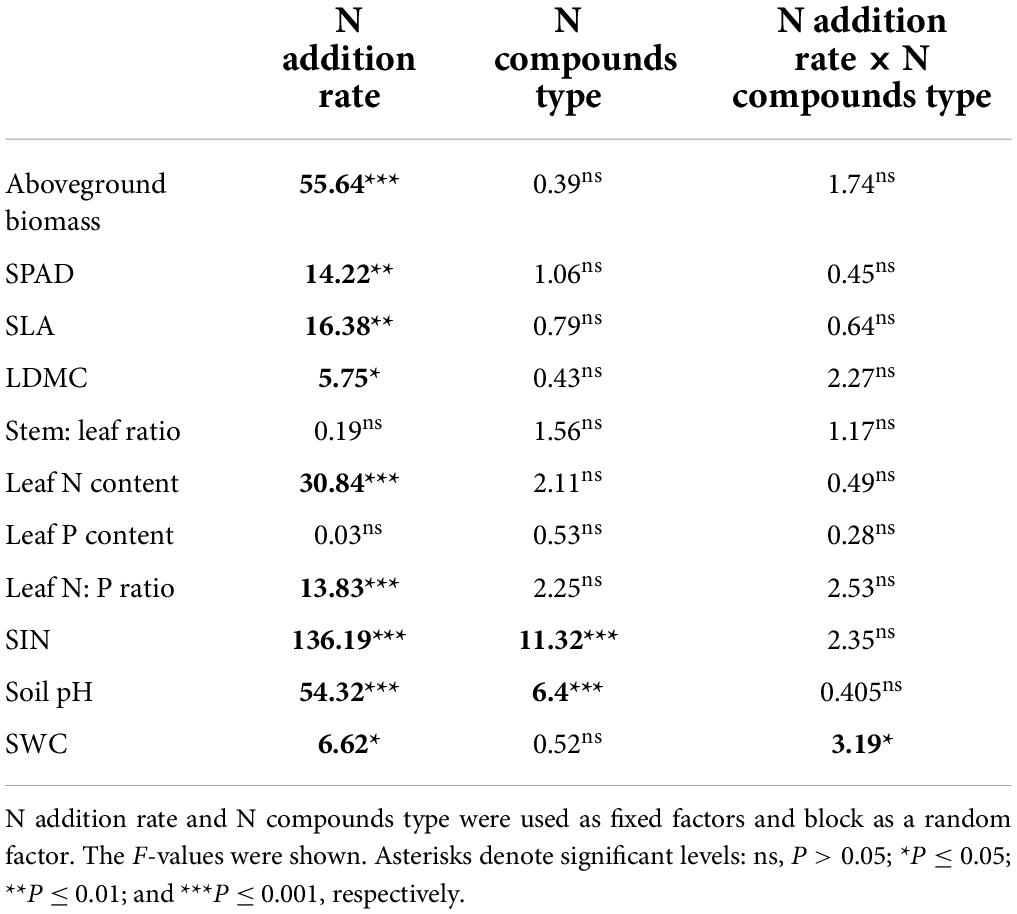
Table 1. Results of mixed model analysis of variance for aboveground biomass, SLA (specific leaf area), LDMC (leaf dry matter content), stem: leaf ratio, leaf N concentration, leaf P concentration, leaf N: P ratio of Leymus chinensis, and soil inorganic nitrogen content (SIN), soil pH, soil water content (SWC).
Results
Effects of N addition on plant functional traits of Leymus chinensis
The compound types of added N had no significant effects on aboveground biomass, SLA, LDMC, stem: leaf ratio, leaf N content, leaf P content, leaf N: P ratio of L. chinensis (Table 1, all P > 0.05). By contrast, N addition rates showed dramatic effects on aboveground biomass, leaf morphology and stoichiometry. Aboveground biomass was not different among the control and N addition treatments up to 5 g N m–2 year–1 but significantly increased at the three highest N addition levels (10, 20, and 50 g N m–2 year–1; Figure 1A). N addition rate increased SLA and leaf N concentration whereas decreased LDMC (Figures 1B,D, 2A), with the tipping point of N-induced changes being at the rate of 20 g N m–2 year–1. The stem: leaf ratio and leaf P concentration were not affected by N addition (Figures 1C, 2B).
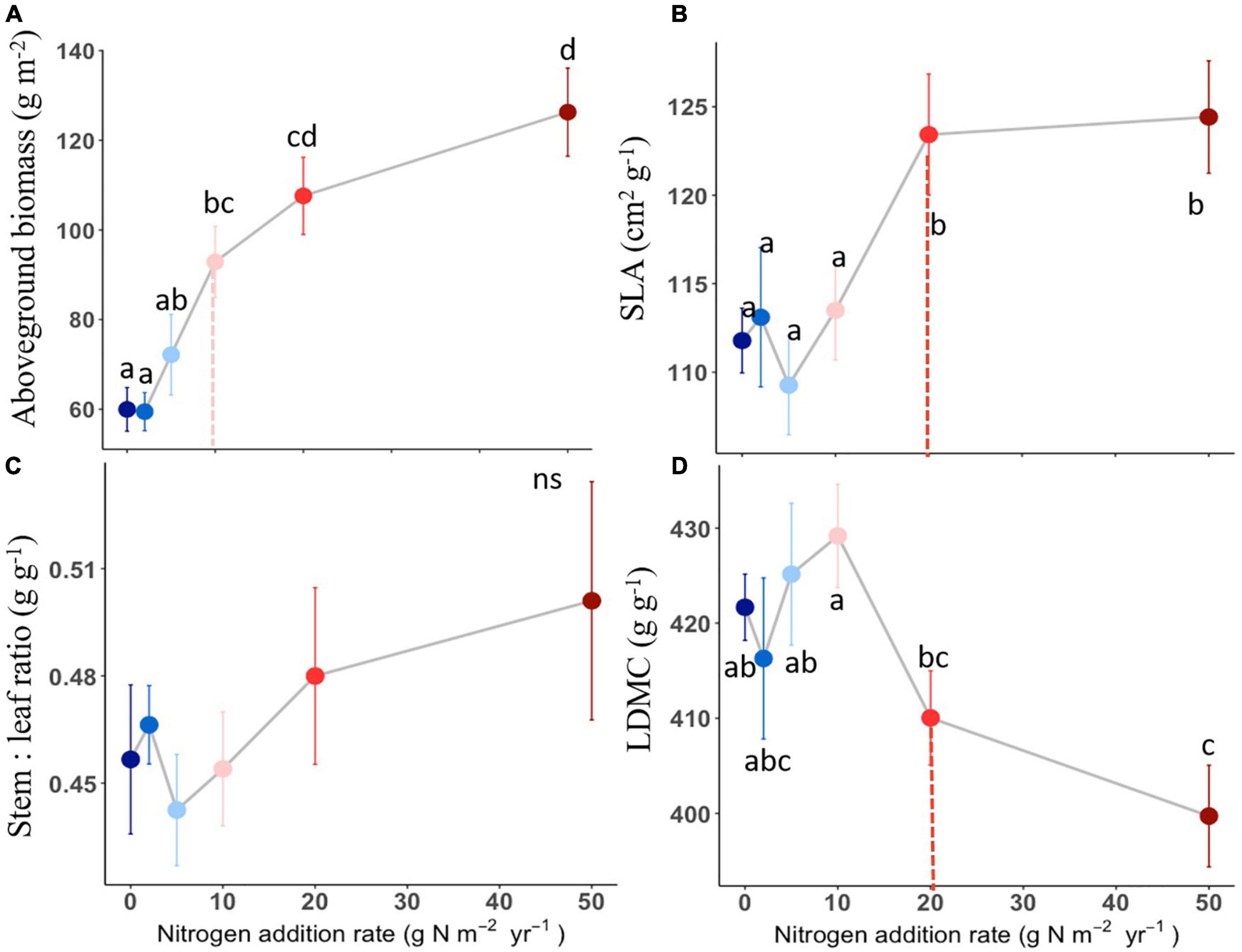
Figure 1. Effects of N addition on plant biomass and leaf traits of L. chinensis. Trait abbreviations are aboveground biomass (A), SLA (specific leaf area) (B), LDMC (leaf dry matter content) (C) and stem: leaf ratio (D). Different lower-case letters indicate significant differences (P < 0.05) among treatments, and ns indicates non-significant (P > 0.05). The dashed line in each panel indicate for tipping points. The data shown are the means with 40 replications ± standard error.
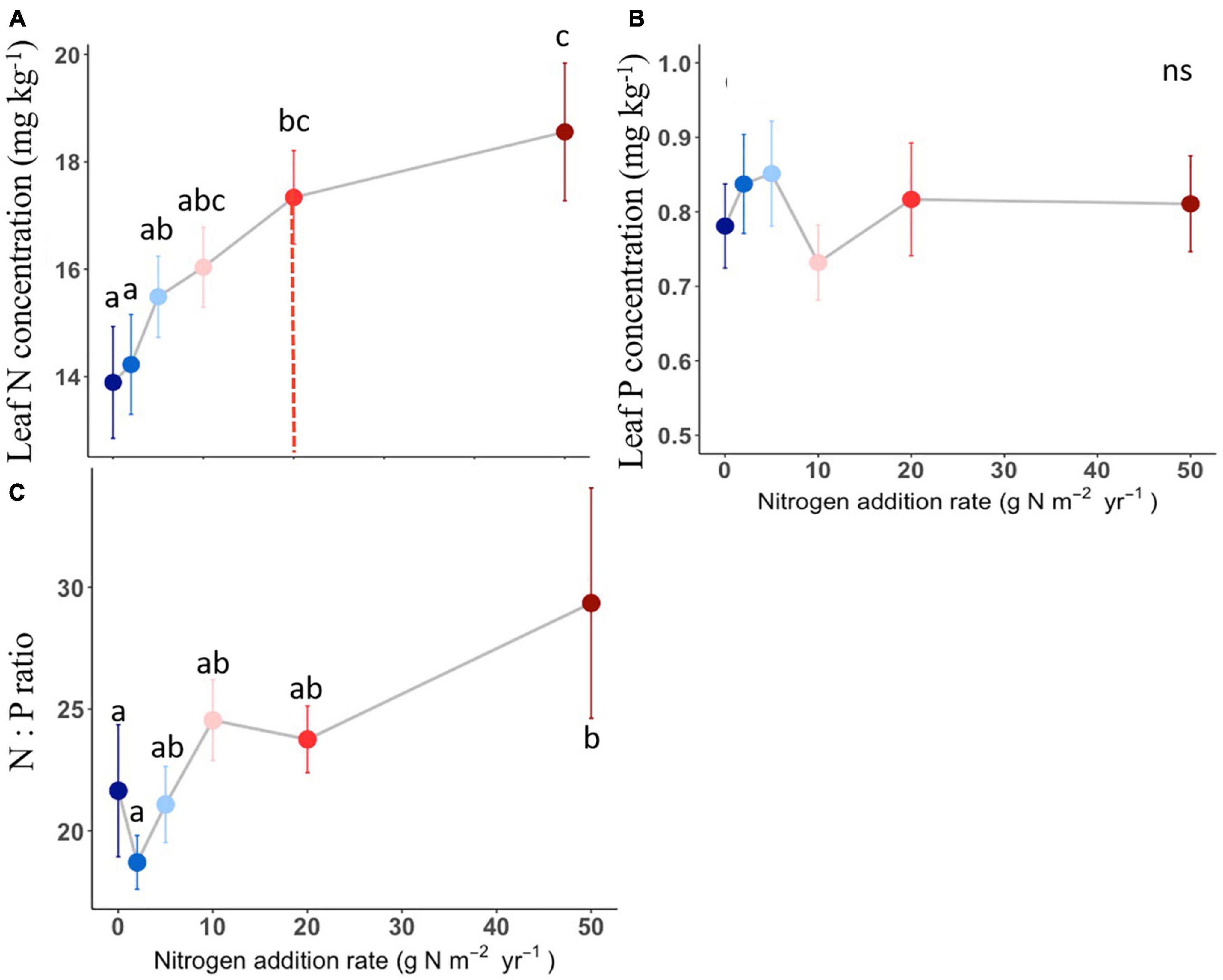
Figure 2. Effects of N addition on leaf stoichiometry of L. chinensis. Trait abbreviations are leaf N concentration (A), leaf P concentration (B), leaf N: P mass ratio (C). Different lower-case letters indicate significant differences (P < 0.05) between treatments, and ns indicates non-significant (P > 0.05). The dashed line in each panel indicate for tipping points. The data shown are the means with 40 replications ± standard error.
Effects of N addition on soil characteristics
Soil inorganic nitrogen increased with N addition once the N addition rate passed the 2 g N m–2 year–1 (Figure 3A). Soil pH in the plots receiving 10, 20, and 50 g N m–2 year–1 was lower than the one receiving N below 10 g N m–2 year–1 level (Figure 3B). However, there was no detectable difference in soil water content among N addition rates (Figure 3C). The effects of N addition on soil inorganic N and soil pH significantly different among N compounds (P < 0.05, Table 1 and Supplementary Figure 1), with the (NH4)2SO4 treatment showing stronger effect than other N compounds.
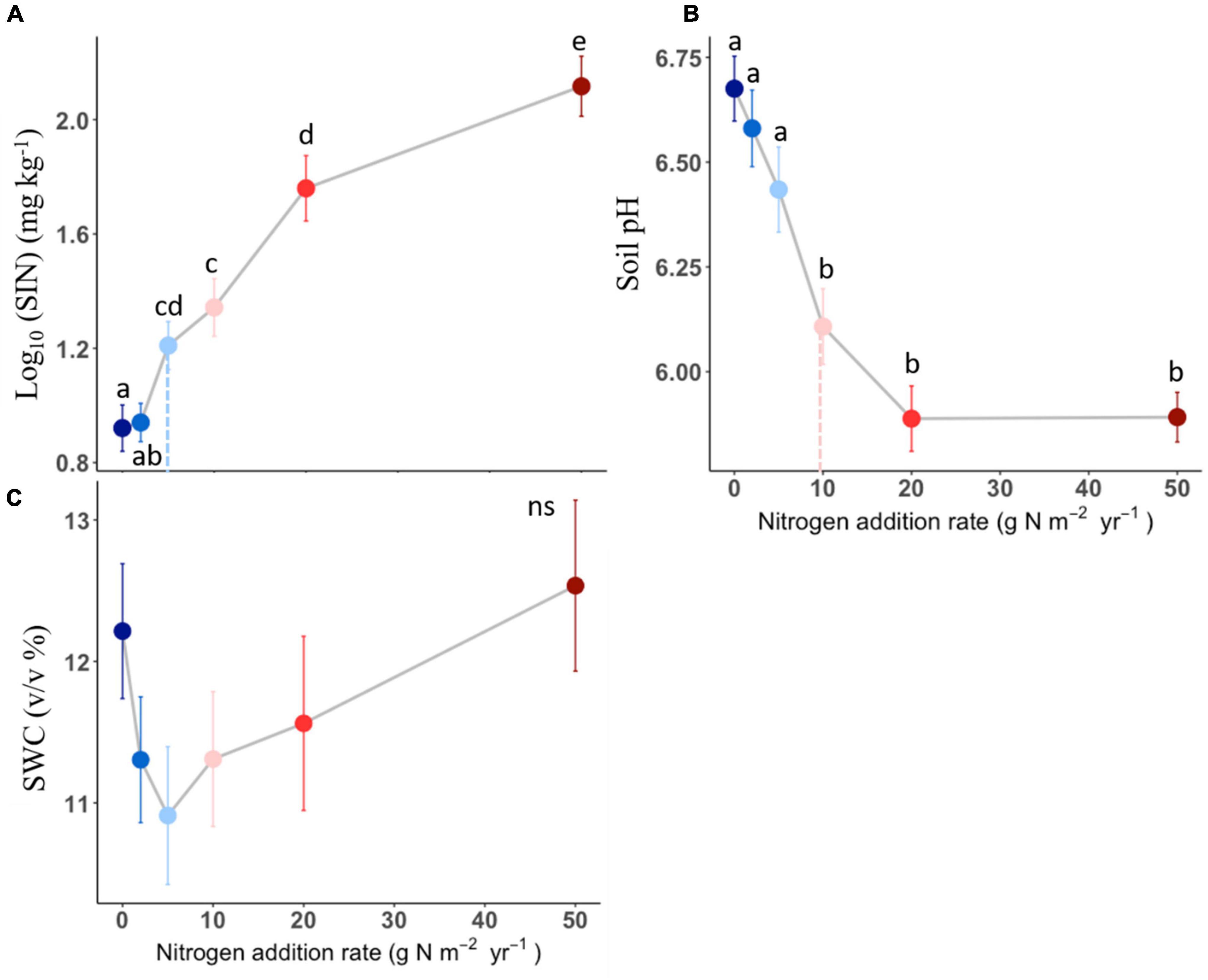
Figure 3. Effects of different N addition on soil inorganic nitrogen (SIN, log10-transformed, A), soil pH (B), soil water content (SWC, C). Different lower-case letters indicate significant differences (P < 0.05) between treatments, and ns indicates non-significant (P > 0.05). The dashed line in each panel indicate for tipping points. The data shown are the means with 40 replications ± standard error.
Correlations between aboveground biomass and soil conditions
The aboveground biomass was positively correlated with soil inorganic N (Figure 4A, R2 = 0.29, P < 0.001), but negatively correlated with soil pH (Figure 4B, R2 = 0.23, P < 0.001). No significant relationship, however, was found between aboveground biomass and functional traits response to N addition (all P > 0.05).
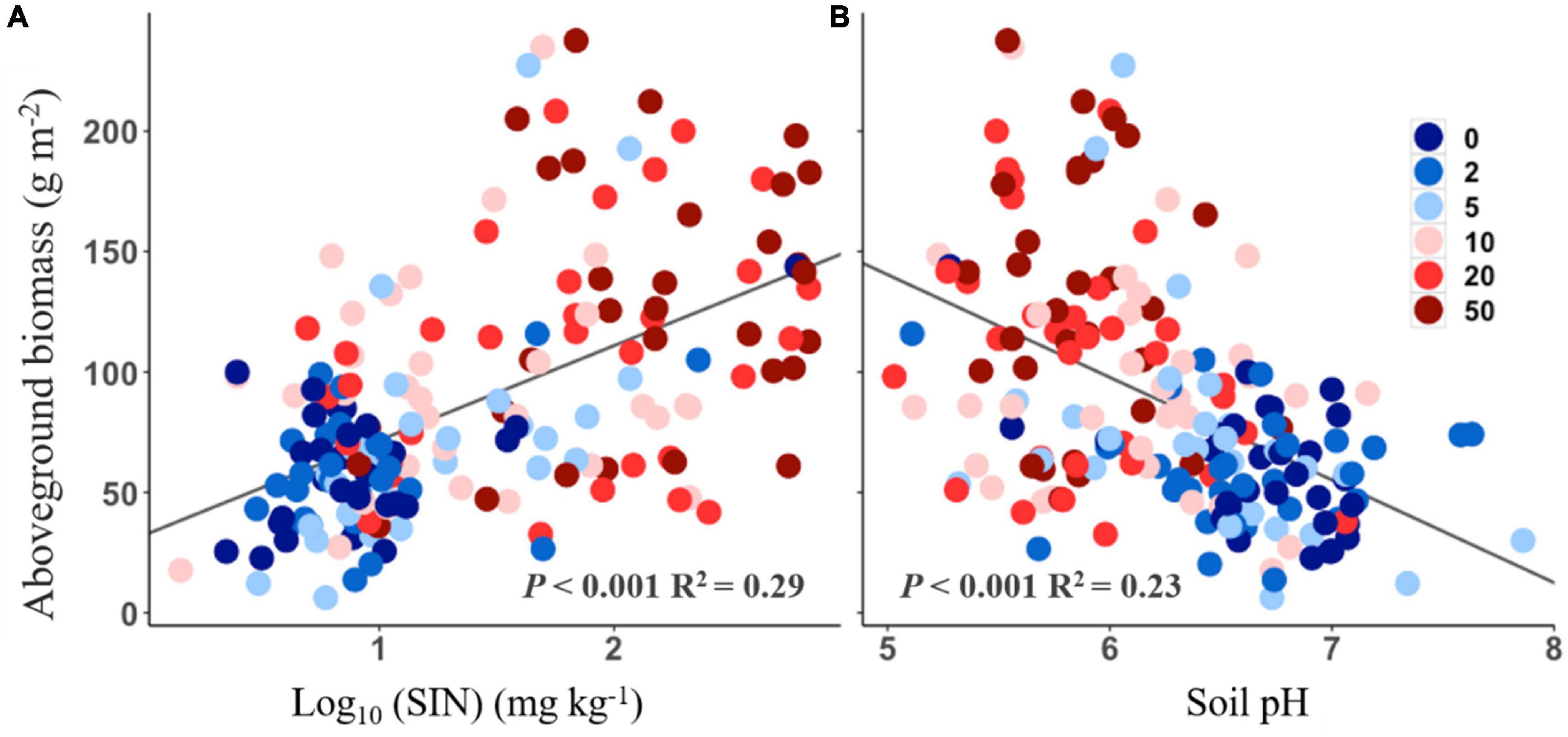
Figure 4. Relationships between aboveground biomass and soil inorganic nitrogen (SIN, log10-transformed, A) and soil pH (B).
Discussion
Non-linear response of aboveground biomass to N addition
Consistent with our hypothesis, we found that aboveground biomass of L. chinensis was not affected by low levels of N addition (i.e., < 5 g N m–2 year–1), but sharply increased between 10 and 50 g N m–2 year–1. These results suggested that plant growth in Inner Mongolia grassland was strongly limited by N and N addition could stimulate plant growth by relieving N limitation, which agreed with observations in Hautier et al. (2009). Moreover, another field experiment in Inner Mongolia at the typical steppe found that increase in N availability also stimulated community aboveground biomass, with the significant positive response occurred at the N addition rate of 1.75 g N m–2 year–1 by the fourth year (Bai et al., 2010). It is lower than that in our study where N threshold was ≥ 10 g N m–2 year–1. This may be mainly due to that our study have been performed just for 2 years, which has lower soil N availability. So the change of L. chinensis under long-term N addition should be investigated in future studies.
Nitrogen-induced changes in soil conditions could mediate aboveground biomass responses to the increasing N addition rate. Correlations between aboveground biomass and soil N availability vs. soil pH indicate that aboveground production is sensitive to changes in soil properties (Figure 4). First, the increase in soil N availability could contribute to plant growth (Gough et al., 2000), especially in our experiment. This because the model species L. chinensis is typical clonal plant. It has been reported that clonal plants have competitive advantages in acquiring soil nutrients and light, which cause their response to N addition to be more positive than non-clonal plants (Gough et al., 2012; Dickson et al., 2014). Second, the soil acidification under N addition could be another reason for the loss of aboveground production. For example, previous study found that decreased soil pH may lead to an increase in mortality of acid−sensitive plants (van den Berg et al., 2005), and induce decreases in plant productivity (Chen et al., 2013). Many studies have documented that soil acidification increases Al3+, which can be directly toxic to plants (van den Berg et al., 2005). However, a previous study showed that L. chinensis was positively correlated with the degree of acidification in a temperate steppe (Lan, 2014), which was in line with our results here. Both results suggested L. chinensis could be tolerant to acid conditions and its relative biomass could continue to increase with increasing N addition even though such a scenario soil acidification became severe (Yang et al., 2019).
Non-linear response of plant functional traits to N addition
Contrary to our hypothesis, we did not find a similar threshold in response to N addition between plant biomass and functional traits. For example, the threshold for N-induced plant traits changes was at N addition rate of 20 g N m–2 year–1, higher than that of aboveground biomass (Figures 1, 2). It reflected that plant traits was relatively less sensitive than the aboveground biomass to N addition rate from the short-term N deposition. The distinct N threshold between the aboveground biomass and plant traits could be explained by the following two aspects. First, N is the limiting nutrient for productivity in the temperate steppe, and N enrichment could increase aboveground production (Bai et al., 2010). The enhanced plant growth induced by N addition could increase the intensity of light competition among plant species (DeMalach et al., 2017). Plants usually develop leaves with high SLA to enhance light capture (Freschet et al., 2015). Therefore, changes of SLA could reflect their adaptive capacities to low light conditions after the enhanced plant growth induced by N addition. Second, the response of traits to one global change driver may also be associated with other drivers. Given that water and N availability can co-limit plant growth in semiarid regions (Hooper and Johnson, 1999; Niu et al., 2010). It is expected that drought would restrain plant N uptake, thus the effects of changing precipitation regimes and global N enrichment will be interdependent (Shen et al., 2020). The long-term mean annual precipitation of the study site is 363 mm, and our study was conducted in a drought year (the mean annual precipitation is 148.2 mm). Previous study found that drought had a stronger effect on leaf traits than short-term N deposition, and the effect of drought on traits is opposite to that of N addition, for example drought had significant negative effects on the leaf N concentrations and net photosynthetic rate (Yu et al., 2019). Therefore, the threshold for N-induced plant traits changes was higher in this study.
High N addition rates increased SLA and leaf N content, decreases LDMC, which is consistent with many previous findings (La Pierre and Smith, 2015; Wang et al., 2016; Valliere et al., 2017; Zheng and Ma, 2018). For those traits, first, SLA indicates captured light resources on LA per unit leaf dry matter investment, which is closely related to plants’ light interception efficiency (Reich, 2014). Previous studies have shown that N addition increased SLA, possibly because it promoted growth and thus increased leaf photosynthesis, or reduced the availability of canopy light to increase the SLA (Zeng et al., 2009; Palmroth et al., 2014). Second, Leaf N is an integral component of the photosynthetic machinery, and changes in leaf N concentration may underpin the greater competitive capability of L. chinensis in the community under enhanced N input (Zheng et al., 2018). In line with our results, a recent work using 2683 observations showed that N addition enhanced leaf N concentration both on mass basis and area basis (Liang et al., 2020). LDMC is an index of conservatism in life history. We found that LDMC decreased under N addition, and low LDMC represent rapid nutrient acquisition, which is conducive to the growth of plants in a nutrient-rich environment (Wilson et al., 1999). Overall, elevated SLA and leaf N content, declined LDMC caused by N enrichment suggests that L. chinensis have a resource acquisitive strategy and such strategy become stronger under exogenous N input.
Here we targeted one single species under short-term N addition by focusing on traits of aboveground tissue, which raised few limitations that need to be addressed in future studies. First, it has been reported that clonal plants response more positively than non-clonal plants to N addition (Dickson et al., 2014). Therefore, further research is needed to explore how other species respond to N addition, whether the responses of aboveground biomass and functional traits to N addition were asymmetric for other species. Second, this study is based on a relatively short-term N-enrichment experiment. The response of functional traits and productivity may differ between short-term and long-term experiments. Therefore, long- term N addition experiments are needed. Thrid, root traits also play pivotal roles in resource acquisition, as plant economic spectrum predicts, species with the traits of high specific root length and low tissue density would more strongly compete for belowground resource (Weemstra et al., 2016; Erktan et al., 2018). Recognition of the different response between above- and below-ground traits could improve our understanding of plant growth to N addition. Thus we need explore further how the root traits would affect plant growth under N deposition scenarios.
Conclusion
By conducting a multi-level of rates and chemical forms of N addition at the field condition in Chinese temperate grassland, here we show evidence to support that there indeed exist tipping points for plant biomass and functional traits in response to N addition. In particular, the tipping point for N-induced increase in L. chinensis aboveground biomass is at N addition rates of 10 g N m–2 year–1, while that critical points in functional traits are at 20 g N m–2 year–1, suggesting that the responses to N addition were more sensitive in aboveground biomass than that in plant functional traits. Our findings can help to understand to what extent and in which rate of N deposition will start to cause the transition of plant functional traits and biomass production. These insights certainly can be useful in forecasting changes in ecosystem service and maintaining ecosystem management under future N deposition scenarios.
Data availability statement
The original contributions presented in the study are included in the article/Supplementary material, further inquiries can be directed to the corresponding authors.
Author contributions
GY, ZZ, and GZ collected the data. GY, ZZ, PZ, and RW developed the research questions. GY and QL analyzed the data. GY wrote the draft with contributions and input from all authors.
Funding
This work was supported by National Natural Science Foundation of China (32001184) and China Postdoctoral Science Foundation (2019M662336).
Acknowledgments
We are grateful to Erguna Forest-Steppe Ecotone Ecosystem Research Station for providing the experimental sites. We thank many undergraduate students from Heilongjiang Bayi Agricultural University for their help in field work.
Conflict of interest
The authors declare that the research was conducted in the absence of any commercial or financial relationships that could be construed as a potential conflict of interest.
Publisher’s note
All claims expressed in this article are solely those of the authors and do not necessarily represent those of their affiliated organizations, or those of the publisher, the editors and the reviewers. Any product that may be evaluated in this article, or claim that may be made by its manufacturer, is not guaranteed or endorsed by the publisher.
Supplementary material
The Supplementary Material for this article can be found online at: https://www.frontiersin.org/articles/10.3389/fpls.2022.982478/full#supplementary-material
References
Bai, Y., Han, X., Wu, J., Chen, Z., and Li, L. (2004). Ecosystem stability and compensatory effects in the Inner Mongolia grassland. Nature 431, 181–184. doi: 10.1038/nature02850
Bai, Y., Wu, J., Clark, C. M., Naeemz, S., Pan, Q., Huang, J., et al. (2010). Tradeoffs and thresholds in the effects of nitrogen addition on biodiversity and ecosystem functioning: Evidence from inner Mongolia Grasslands. Glob. Chang. Biol. 16, 358–372. doi: 10.1111/j.1365-2486.2009.01950.x
Bennett, L. T., Judd, T. S., and Adams, M. A. (2002). Growth and nutrient content of perennial grasslands following burning in semiarid, sub-tropical Australia. Plant Ecol. 164, 185–199. doi: 10.1023/A:1021253600712
Bobbink, R., Hornung, M., and Roelofs, J. G. M. (1998). The effects of air-borne nitrogen pollutants on species diversity in natural and semi-natural European vegetation. J. Ecol. 86, 717–738.
Chen, D., Lan, Z., Bai, X., Grace, J. B., and Bai, Y. (2013). Evidence that acidification-induced declines in plant diversity and productivity are mediated by changes in below-ground communities and soil properties in a semi-arid steppe. J. Ecol. 101, 1322–1334. doi: 10.1111/1365-2745.12119
DeMalach, N., Zaady, E., and Kadmon, R. (2017). Light asymmetry explains the effect of nutrient enrichment on grassland diversity. Ecol. Lett. 20, 60–69. doi: 10.1111/ele.12706
Dickson, T. L., Mittelbach, G. G., Reynolds, H. L., and Gross, K. L. (2014). Height and clonality traits determine plant community responses to fertilization. Ecology 95, 2443–2452. doi: 10.1890/13-1875.1
Elser, J. J., Bracken, M. E. S., Cleland, E. E., Gruner, D. S., Harpole, W. S., Hillebrand, H., et al. (2007). Global analysis of nitrogen and phosphorus limitation of primary producers in freshwater, marine and terrestrial ecosystems. Ecol. Lett. 10, 1135–1142. doi: 10.1111/j.1461-0248.2007.01113.x
Erktan, A., Roumet, C., Bouchet, D., Stokes, A., Pailler, F., and Munoz, F. (2018). Two dimensions define the variation of fine root traits across plant communities under the joint influence of ecological succession and annual mowing. J. Ecol. 106, 2031–2042. doi: 10.1111/1365-2745.12953
Freschet, G. T., Swart, E. M., and Cornelissen, J. H. C. (2015). Integrated plant phenotypic responses to contrasting above- and below-ground resources: Key roles of specific leaf area and root mass fraction. New Phytol. 206, 1247–1260. doi: 10.1111/nph.13352
Galloway, J. N., Townsend, A. R., Erisman, J. W., Bekunda, M., Cai, Z., Freney, J. R., et al. (2008). Transformation of the nitrogen cycle:Recent trends, questions, and potential solutions. Science 320, 889–892. doi: 10.1126/science.1136674
Gough, L., Gross, K. L., Cleland, E. E., Clark, C. M., Collins, S. L., Fargione, J. E., et al. (2012). Incorporating clonal growth form clarifies the role of plant height in response to nitrogen addition. Oecologia 169, 1053–1062. doi: 10.1007/s00442-012-2264-5
Gough, L., Osenberg, C. W., Gross, K. L., Collins, S. L., and Fertilization, S. L. (2000). Fertilization effects on species density and primary productivity in herbaceous plant communities. Oikos 3, 428–439.
Groffman, P. M., Baron, J. S., Blett, T., Gold, A. J., Goodman, I., Gunderson, L. H., et al. (2006). Ecological thresholds: The key to successful environmental management or an important concept with no practical application? Ecosystems 9, 1–13. doi: 10.1007/s10021-003-0142-z
Hautier, Y., Niklaus, P. A., and Hector, A. (2009). Competition for light causes plant biodiversity loss after eutrophication. Science 324, 636–638. doi: 10.1126/science.1169640
Hooper, D. U., and Johnson, L. (1999). Nitrogen limitation in dryland ecosystems: Responses to geographical and temporal variation in precipitation. Biogeochemistry 46, 247–293. doi: 10.1023/A:1006145306009
Hu, M., and Wan, S. (2019). Effects of fire and nitrogen addition on photosynthesis and growth of three dominant understory plant species in a temperate forest. J. Plant Ecol. 12, 759–768. doi: 10.1093/jpe/rtz014
Huang, L., Liang, Z., Suarez, D. L., Wang, Z., Ma, H., Wang, M., et al. (2015). Continuous nitrogen application differentially affects growth, yield, and nitrogen use efficiency of Leymus chinensis in two saline-sodic soils of northeastern China. Agron. J. 107, 314–322. doi: 10.2134/agronj14.0250
Lan, Z. (2014). Effects of nitrogen addition on plant diversity and stability of a typical steppe-mechanism and scale-dependence of a N-induced plant diversity loss. Ph.D. dissertation. Beijing: Institute of Botany, Chinese academy sciences.
La Pierre, K. J., and Smith, M. D. (2015). Functional trait expression of grassland species shift with short- and long-term nutrient additions. Plant Ecol. 216, 307–318. doi: 10.1007/s11258-014-0438-4
Lavorel, S., and Grigulis, K. (2012). How fundamental plant functional trait relationships scale-up to trade-offs and synergies in ecosystem services. J. Ecol. 100, 128–140. doi: 10.1111/j.1365-2745.2011.01914.x
Liang, X., Zhang, T., Lu, X., Ellsworth, D. S., BassiriRad, H., You, C., et al. (2020). Global response patterns of plant photosynthesis to nitrogen addition: A meta-analysis. Glob. Chang. Biol. 26, 3585–3600. doi: 10.1111/gcb.15071
Niu, S., Wu, M., Han, Y., Xia, J., Zhang, Z., Yang, H., et al. (2010). Nitrogen effects on net ecosystem carbon exchange in a temperate steppe. Glob. Chang. Biol. 16, 144–155. doi: 10.1111/j.1365-2486.2009.01894.x
Palmroth, S., Holm Bach, L., Nordin, A., and Palmqvist, K. (2014). Nitrogen-addition effects on leaf traits and photosynthetic carbon gain of boreal forest understory shrubs. Oecologia 175, 457–470. doi: 10.1007/s00442-014-2923-9
Peng, Y., Li, F., Zhou, G., Fang, K., Zhang, D., Li, C., et al. (2017). Linkages of plant stoichiometry to ecosystem production and carbon fluxes with increasing nitrogen inputs in an alpine steppe. Glob. Chang. Biol. 23, 5249–5259. doi: 10.1111/ijlh.12426
R Development Core Team (2020). R: A language and environment for statistical computing. Vienna: R Foundation for Statistical Computing.
Reich, P. B. (2014). The world-wide “fast-slow” plant economics spectrum: A traits manifesto. J. Ecol. 102, 275–301. doi: 10.1111/1365-2745.12211
Reich, P. B., Oleksyn, J., and Tilman, G. D. (2004). Global patterns of plant leaf N and P in relation to temperature and latitude. Proc. Natl. Acad. Sci. U.S.A. 101, 11001–11006. doi: 10.1073/pnas.0403588101
Rockström, J., Steffen, W., Noone, K., Persson, Å., Chapin, F. S. III, Lambin, E. F., et al. (2009). A safe operating space for humanity. Nature 461, 472–475.
Roem, W. J., Klees, H., and Berendse, F. (2002). Effects of nutrient addition and acidification on plant species diversity and seed germination in heathland. J. Appl. Ecol. 39, 937–948.
Shen, H., Dong, S., DiTommaso, A., Li, S., Xiao, J., Yang, M., et al. (2020). Eco-physiological processes are more sensitive to simulated N deposition in leguminous forbs than non-leguminous forbs in an alpine meadow of the Qinghai-Tibetan Plateau. Sci. Total Environ. 744:140612. doi: 10.1016/j.scitotenv.2020.140612
Song, W., Loik, M. E., Cui, H., Fan, M., and Sun, W. (2022). Effect of nitrogen addition on leaf photosynthesis and water use efficiency of the dominant species Leymus chinensis (Trin.) Tzvelev in a semi-arid meadow steppe. Plant Growth Regul. 98, 91–102. doi: 10.1007/s10725-022-00835-8
Stevens, C. J., Thompson, K., Grime, J. P., Long, C. J., and Gowing, D. J. G. (2010). Contribution of acidification and eutrophication to declines in species richness of calcifuge grasslands along a gradient of atmospheric nitrogen deposition. Funct. Ecol. 24, 478–484. doi: 10.1111/j.1365-2435.2009.01663.x
Sun, L., Yang, G., Zhang, Y., Qin, S., Dong, J., and Cui, Y. (2022). Leaf functional traits of two species affected by nitrogen addition rate and period not nitrogen compound type in a meadow grassland. Front. Plant Sci. 13:841464. doi: 10.3389/fpls.2022.841464
Tao, Y., Zhou, X.-B., Li, Y.-G., Liu, H.-L., and Zhang, Y.-M. (2022). Short-term N and P additions differentially alter the multiple functional traits and trait associations of a desert ephemeral plant in China. Environ. Exp. Bot. 200:104932. doi: 10.1016/j.envexpbot.2022.104932
Tatarko, A. R., and Knops, J. M. H. (2018). Nitrogen addition and ecosystem functioning: Both species abundances and traits alter community structure and function. Ecosphere 9:e02087. doi: 10.1002/ecs2.2087
Valliere, J. M., Irvine, I. C., Santiago, L., and Allen, E. B. (2017). High N, dry: Experimental nitrogen deposition exacerbates native shrub loss and nonnative plant invasion during extreme drought. Glob. Chang. Biol. 23, 4333–4345. doi: 10.1111/gcb.13694
van den Berg, L. J. L., Dorland, E., Vergeer, P., Hart, M. A. C., Bobbink, R., and Roelofs, J. G. M. (2005). Decline of acid-sensitive plant species in heathland can be attributed to ammonium toxicity in combination with low pH. New Phytol. 16, 551–564. doi: 10.1111/j.1469-8137.2005.01338.x
Vitousek, P. M., and Howarth, R. W. (1991). Nitrogen limitation on land and in the sea: How can it occur? Biogeochemistry 13, 87–115.
Wang, C., Xiao, H., Liu, J., Zhou, J., and Du, D. (2016). Insights into the effects of simulated nitrogen deposition on leaf functional traits of Rhus Typhina. Polish J. Environ. Stud. 25, 1279–1284. doi: 10.15244/pjoes/61788
Weemstra, M., Mommer, L., Visser, E. J. W., van Ruijven, J., Kuyper, T. W., Mohren, G. M. J., et al. (2016). Towards a multidimensional root trait framework: A tree root review. New Phytol. 211, 1159–1169. doi: 10.1111/nph.14003
Wilson, P. J., Thompson, K., and Hodgson, J. G. (1999). Specific leaf area and leaf dry matter content as alternative predictors of plant strategies. New Phytol. 143, 155–162. doi: 10.1046/j.1469-8137.1999.00427.x
Wright, I. J., Reich, P. B., Westoby, M., Ackerly, D. D., Baruch, Z., Bongers, F., et al. (2004). The worldwide leaf economics spectrum. Nature 428, 821–827. doi: 10.1038/nature02403
Yang, G., Lü, X., Stevens, C. J., Zhang, G., Wang, H., Wang, Z., et al. (2019). Mowing mitigates the negative impacts of N addition on plant species diversity. Oecologia 189, 769–779. doi: 10.1007/s00442-019-04353-9
Yu, H., Cheng, D., Li, B., Xu, C., Zhang, Z., Zhong, Y., et al. (2019). Short-term nitrogen addition does not significantly alter the effects of seasonal drought on leaf functional traits in Machilus pauhoi Kanehira seedlings. Forests 10:78. doi: 10.3390/f10020078
Zeng, D. H., Hu, Y. L., Chang, S. X., and Fan, Z. P. (2009). Land cover change effects on soil chemical and biological properties after planting Mongolian pine (Pinus sylvestris var. mongolica) in sandy lands in Keerqin, northeastern China. Plant Soil 317, 121–133. doi: 10.1007/s11104-008-9793-z
Zhang, D., Peng, Y., Li, F., Yang, G., Wang, J., Yu, J., et al. (2019). Trait identity and functional diversity co-drive response of ecosystem productivity to nitrogen enrichment. J. Ecol. 107, 2402–2414. doi: 10.1111/1365-2745.13184
Zhang, D., Peng, Y., Li, F., Yang, G., Wang, J., Yu, J., et al. (2021). Above- and below-ground resource acquisition strategies determine plant species responses to nitrogen enrichment. Ann. Bot. 128, 31–44. doi: 10.1093/aob/mcab032
Zheng, Z., and Ma, P. (2018). Changes in above and belowground traits of a rhizome clonal plant explain its predominance under nitrogen addition. Plant Soil 432, 415–424. doi: 10.1007/s11104-018-3815-2
Keywords: nitrogen deposition, threshold, functional traits, productivity, steppe
Citation: Yang G, Zhang Z, Zhang G, Liu Q, Zheng P and Wang R (2022) Tipping point of plant functional traits of Leymus chinensis to nitrogen addition in a temperate grassland. Front. Plant Sci. 13:982478. doi: 10.3389/fpls.2022.982478
Received: 30 June 2022; Accepted: 01 August 2022;
Published: 17 August 2022.
Edited by:
Jianping Wu, Yunnan University, ChinaReviewed by:
Dima Chen, China Three Gorges University, ChinaJushan Liu, Northeast Normal University, China
Copyright © 2022 Yang, Zhang, Zhang, Liu, Zheng and Wang. This is an open-access article distributed under the terms of the Creative Commons Attribution License (CC BY). The use, distribution or reproduction in other forums is permitted, provided the original author(s) and the copyright owner(s) are credited and that the original publication in this journal is cited, in accordance with accepted academic practice. No use, distribution or reproduction is permitted which does not comply with these terms.
*Correspondence: Peiming Zheng, zhengpeiming@email.sdu.edu.cn; Zijia Zhang, zhangzijia@gmail.com