- 1Department of Biological Psychology and Neuroergonomics, Technical University of Berlin, Berlin, Germany
- 2Division of Movement and Training Sciences/Biomechanics of Sport, University of Duisburg-Essen, Essen, Germany
- 3Institute of Sports and Sports Sciences, Heidelberg University, Heidelberg, Germany
- 4Clinic for Geriatric Rehabilitation, Robert-Bosch-Krankenhaus Stuttgart, Stuttgart, Germany
- 5Department of Human Movement Science, University of Hamburg, Hamburg, Germany
Introduction: Spatial navigation is a complex cognitive function that declines in older age. Finding one’s way around in familiar and new environments is crucial to live and function independently. However, the current literature illustrates the efficacy of spatial navigation interventions in rehabilitative contexts such as pathological aging and traumatic injury, but an overview of existing training studies for healthy older adults is missing. This scoping review aims to identify current evidence on existing spatial navigation interventions in healthy older adults and analyze their efficacy.
Methods: To identify spatial navigation interventions and assessments and investigate their effectiveness, four electronic databases were searched (Pubmed, Web of Science, CINAHL and EMBASE). Two independent reviewers conducted a screening of title, abstract and full-texts and performed a quality assessment. Studies were eligible if (1) published in English, (2) the full text was accessible, (3) at least one group of healthy older adults was included with (4) mean age of 65 years or older, (5) three or more spatial navigation-related training sessions were conducted and (6) at least one spatial ability outcome was reported.
Results: Ten studies were included (N = 1,003, age-range 20–95 years, 51.5% female), only healthy older adults (n = 368, mean age ≥ 65) were assessed further. Studies differed in sample size (n = 22–401), type of training, total intervention duration (100 min–50 h), and intervention period (1–16 weeks).
Conclusion: The spatial navigation abilities addressed and the measures applied to elicit intervention effects varied in quantity and methodology. Significant improvements were found for at least one spatial ability-related outcome in six of 10 interventions. Two interventions achieved a non-significant positive trend, another revealed no measurable post-training improvement, and one study did not report pre-post-differences. The results indicate that different types of spatial navigation interventions improve components of spatial abilities in healthy older adults. The existing body of research does not allow conclusions on transferability of the trained components on everyday life spatial navigation performance. Future research should focus on reproducing and extending the promising approaches of available evidence. From this, valuable insights on healthy aging could emerge.
Trial Registration: This scoping review was preregistered at Open Science Framework (https://osf.io/m9ab6).
Background
Many older adults perceive an autonomous and meaningful life within their community as a desirable goal and strive to live independently as long as possible (Portegijs et al., 2014). This is challenged by age-related processes leading to motor and cognitive degradation. One complex cognitive ability deteriorating with age is spatial navigation (e.g., planning to reach an intended destination and move through physical environments; Burns, 1999; Moffat et al., 2006; Iaria et al., 2009; Head and Isom, 2010). While the ability to navigate through familiar environments remains relatively stable, coping with unfamiliar environments decreases notably with increasing age (Colombo et al., 2017).
Whether older persons are able to live and function independently on a daily basis is therefore determined by finding their way around familiar and new environments, the ability to move outdoors, as well as adequate physical, cognitive, and mental health (Portegijs et al., 2014; Claessen et al., 2015; Muffato et al., 2020). The steady decline or even loss of any of these capabilities immediately affects the others leading to a vicious circle of fading independence. Decreasing navigational skills are further known to be an early predictor of pathological aging processes like dementia or mild cognitive impairment and are common in trauma-induced contexts (Moffat, 2009; Claessen et al., 2015; Boccia et al., 2019).
This decline can cause fear of not finding a particular destination like the way home, or even getting lost (Phillips et al., 2013; Saajanaho et al., 2015). To reach a destination in the environment, humans primarily use two different reference frames: the egocentric and the allocentric reference frame (Kitchin, 1994; Gramann et al., 2005). The egocentric reference frame refers to the body position from a first-person perspective and integrates landmarks along the way related to the current position and orientation. In comparison, the allocentric reference frame is rather a bird’s eye representation of the environment, possibly including one’s body position and orientation but not taking it as a central reference point (Gramann, 2013; Colombo et al., 2017). In this reference frame, external landmarks are collected to generate a mental representation of the environment, often called a “cognitive map” (Kitchin, 1994; Epstein et al., 2017). Studies in virtual environments showed that spatial abilities, which rely on the egocentric frame of reference, remain stable with age, while allocentric abilities deteriorate (Moffat et al., 2006; Iaria et al., 2009; Fricke and Bock, 2018). This, in turn, can hamper the physical activity and social participation behavior of older adults and might reflect on a person’s life-space mobility (i.e., the social and physical environment a person moves in on a daily basis), safety, and overall well-being (Burns, 1999; Taylor et al., 2019; Muffato et al., 2020).
Next, to the individuals’ cognitive and physical conditions, precursors of smaller life-spaces appear to be disadvantageous neighborhood properties, transportation issues, and lower levels of education and income (Lo et al., 2016). A larger life-space has been associated with a higher quality of life and good physical, cognitive, and psycho-social health (Baker et al., 2003; Sejunaite et al., 2017; Taylor et al., 2019). While the link between reduced life-space mobility and age-related decline of these properties has been well established, decreasing life-space also appears to be a predictor of nursing home admission (Sheppard et al., 2013) and mortality in older persons (Boyle et al., 2010; Mackey et al., 2014; Kennedy et al., 2017). An approach to counter these effects can be found in the evidence that both, the aging body and aging brain remain plastic, and capabilities can be improved through specific cognitive or motor training (Kramer et al., 2004; Erickson and Kramer, 2008; Park and Bischof, 2013; O’Callaghan et al., 2018). To link these three dimensions (environmental conditions, physical and cognitive abilities) and to help older adults maintain adequate resources for mobility, specific training interventions are required.
Numerous studies have investigated the training of spatial navigation abilities within pathologic populations to facilitate autonomy. A variety of different training strategies like augmented or virtual reality training, behavioral techniques, route and landmark learning, and combinations with motor-cognitive training approaches yielded very promising results (McGilton et al., 2003; Provencher et al., 2008; Sejunaite et al., 2017).
Despite these encouraging findings, little research has explored so far whether and how spatial navigation can be trained in a healthy older population. Closing this research gap is crucial, as effective training methods could impact significantly how healthy older adults keep up an independent life for as long as possible. This includes maintaining higher levels of physical and cognitive performance, living autonomously, remaining in familiar surroundings, having the possibility for social support and participation, having lower mental health incidences, and higher satisfaction with life, thereby taking a load off the health care system.
Thus, aiming to summarize previous and to facilitate future research in this field, this scoping review systematically reports the current knowledge about intervention studies addressing spatial navigation in healthy older adults. Furthermore, this scoping review provides the applied intervention characteristics and spatial ability-related outcome measures to address the following questions:
1. Which interventions for healthy older adults can be identified targeting spatial navigation abilities?
2. What spatial ability-related assessments were used in those studies to evaluate the effectiveness of the respective spatial interventions for healthy older adults?
3. Which effects of spatial navigation interventions for healthy older adults were observed on spatial abilities?
Materials and methods
Search strategy
Relevant literature was identified by systematically searching the electronic databases Pubmed, Web of Science, CINAHL, and EMBASE. Systematic searches were conducted from the inception of the respective database up until September 2021. The search strategy was developed and refined according to the PICOS scheme (P = healthy older adults, I = training of spatial navigation, C = control groups, O = motor or cognitive or spatial ability outcomes, S = study design), advised by a librarian. All search results were exported into Mendeley (version 1803). After the removal of duplicates, the remaining citations were uploaded to Rayyan QCRI (Ouzzani et al., 2016), a free-of-charge web application that allows collaboration on systematic reviews. Two reviewers (MF, CM) independently screened the titles and abstracts of all results for eligibility according to the inclusion and exclusion criteria. In case the information provided in the title and abstract was insufficient to decide whether an article should be included or excluded, the full-text version of these articles was retrieved and screened for eligibility. Afterward, full-text versions of all potentially relevant studies were obtained and assessed for inclusion by both reviewers. Disagreements were resolved by discussion and consensus. The PRISMA Flow Diagram (Moher et al., 2011) shows the results of the study selection process and documents the reasons for study exclusion (Figure 1) and an example of the coherent search string for the final search on each database is depicted in Appendix 1. For PubMed and CINAHL the results were limited to humans by using the filter function. Grey literature was searched through Google Scholar. Next to that, reference lists of identified articles and review articles in the associated research field were hand-searched to detect additional relevant publications that were not identified by the databases.
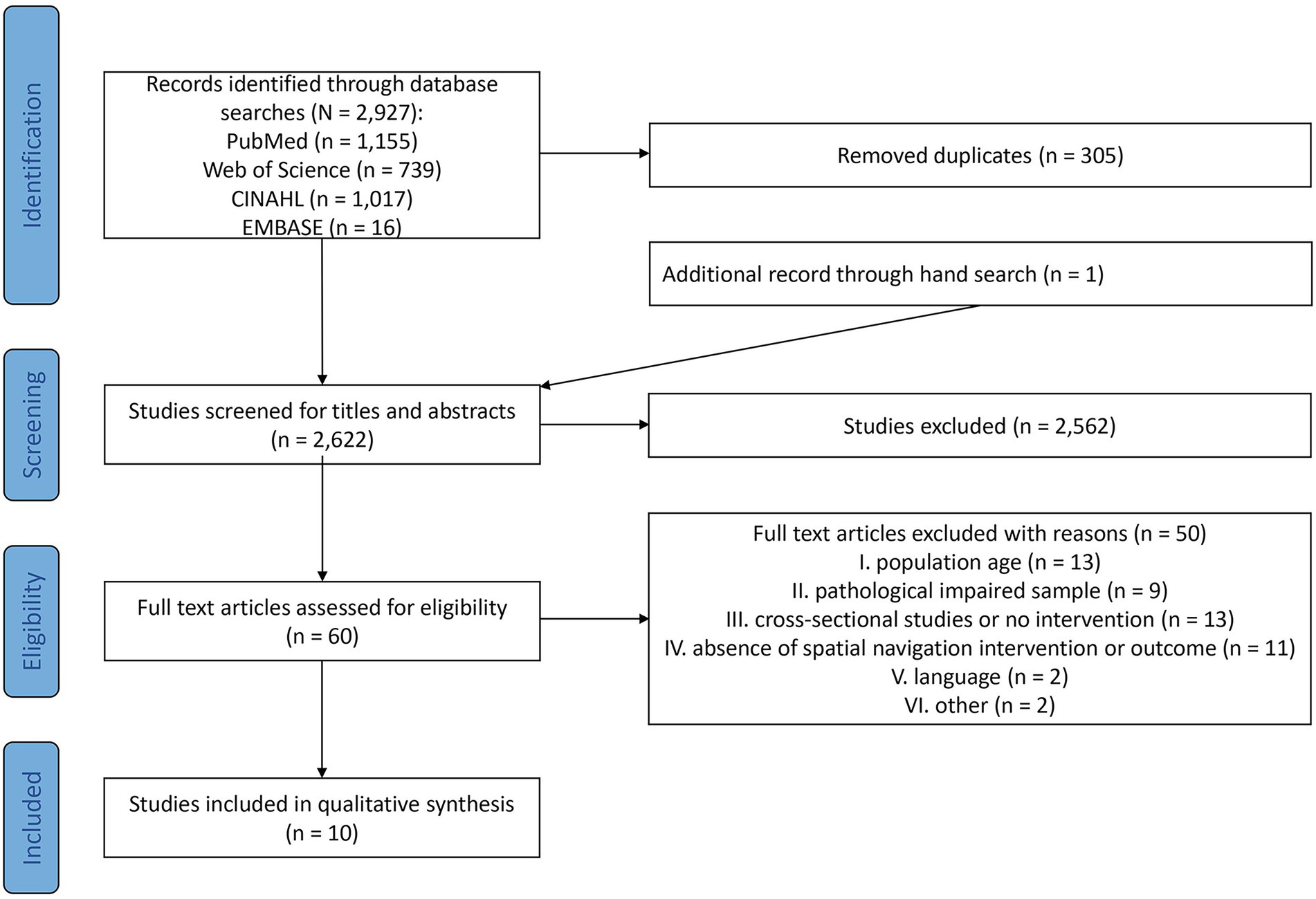
Figure 1. PRISMA flow diagram (according to Moher et al., 2011).
Data charting
Both reviewers documented their data charting process with a modified version of the ‘Data collection form for intervention reviews: RCTs and non-RCTs’. Applied items were key study characteristics like the author(s), year of publication, study design, the aim of the study, population characteristics, intervention characteristics, control interventions, follow-ups, drop-outs, assessed outcomes, and self-reported limitations.
Additionally, information was collected about the content of spatial navigation interventions, training modalities, spatial ability-related outcome measures and outcomes. It should be noted, that only behavioral spatial ability-related outcome measures are reported and discussed, disregarding additional reported data (e.g., imaging data). Data were listed as a mean and standard deviation (SD) if reported. To capture all relevant information, the form was refined in an iterative process and data were updated continuously. Extracted data of both researchers were compared and discrepancies were resolved by discussion. According to the research questions, data about study design, study aim, population and intervention characteristics, spatial ability-related outcome measures and outcomes were used to identify relevant studies and to summarize the applied spatial ability-related assessments and training effects on spatial navigation abilities.
Inclusion and exclusion criteria
Included studies met the following criteria: (I) published in the English language, (II) accessible as a full-text version, (III) at least one group of healthy older adults included with a (IV) mean age of 65 years or older, (V) three or more spatial navigation training sessions conducted and (VI) at least one spatial ability-related outcome reported.
Studies were excluded if they were (I) only cross-sectional or (II) books, reviews, editorials, single case studies, conference abstracts, or interviews. A sample of solely (III) pathologically impaired participants also led to exclusion as well as (IV) the absence of spatial outcomes.
Quality assessment
A quality assessment was performed according to Kmet et al. (2004). This critical appraisal was performed to depict the methodological quality of the included articles and to discriminate the meaningfulness of derived evidence. The original quality assessment contains 14 criteria, of which 11 were applicable to the included studies (cf. criteria 1–11 in Table 1). For each criterion, a maximum of two points was given by two independent reviewers, depending on the degree to which the criterion was met (“yes” = 2, “partial” = 1, “no” = 0, “x” = n/a). Discrepancies were resolved by discussion. For each article, a total score was summed up across relevant items and divided by the total possible score reduced by the number of not applicable criteria times two [i.e., if 10 criteria were fully met but one was not applicable, the score was 20/(22–1*2) = 1]. Due to the limited literature available on this topic, the quality assessment was not used as an inclusion criterion but as a measure of study quality. Referring to Curry et al. (2021) and Lannes et al. (2021) studies with a score of 0.80 and above were considered high quality, whereas scores between 0.70 and 0.79 were defined as good quality. Studies scoring between 0.50 and 0.69 were classified as moderate quality. Studies below the score of 0.50 were classified as poor.
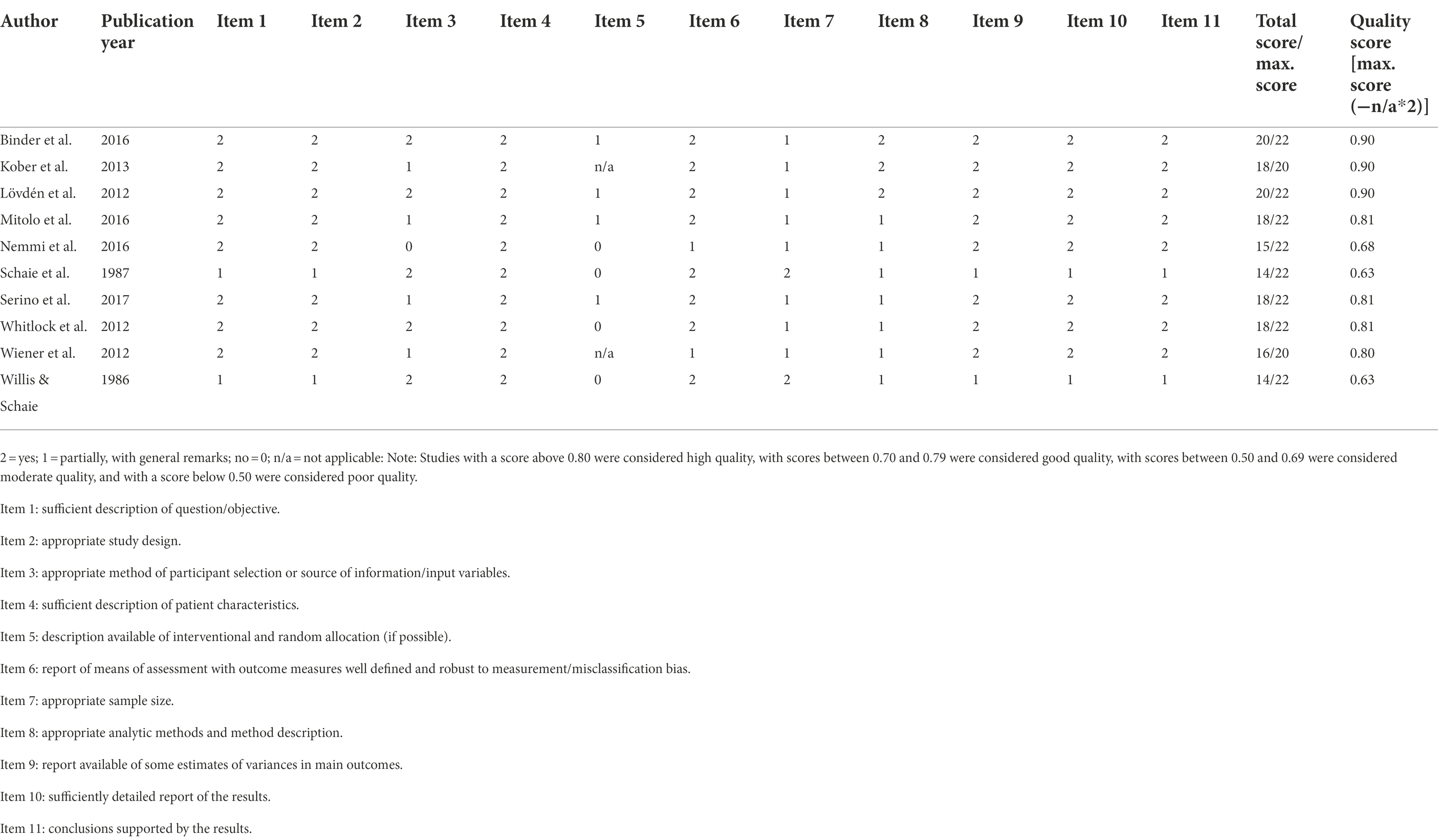
Table 1. Quality assessment (according to Kmet et al. (2004)).
Results
Study selection
As shown in Figure 1, the systematic search within the databases PubMed, Web of Science, CINAHL and EMBASE identified 2,927 articles for consideration. Three hundred-five duplicates were removed. After title and abstract screening, a total of 60 full-text articles were included for further examination. The main reasons for exclusion were for example participants, that did not meet the inclusion criteria for age, pathological impaired samples without a healthy control group, or the absence of spatial navigation interventions or spatial ability-related outcomes. Two of the studies were dropped due to publication in Italian. In the end, 10 articles were incorporated into the qualitative synthesis.
Study characteristics
Table 2 gives a comprehensive overview of the general study characteristics of the included articles. Table 3 provides a deeper insight into the interventions performed, and their training modalities as well as the spatial ability-related assessments used. It further briefly presents the findings concerning the applied spatial ability-related measures.
Participant characteristics
Summarizing all records, a total of 1,003 adults participated in the studies (cf. Table 2), this includes younger or pathologically impaired comparison groups. Participants’ age ranged from 20 to 95 years, however, the older age group that was further assessed had a mean age of 65 years and above. Overall, 516 women and 487 men were included in the research activities. Three hundred-sixty-eight of these participants were healthy older adults receiving a spatial navigation-related intervention, while the others either had pathologic impairments or had been younger, healthy control participants. One study included men only (Lövdén et al., 2012) and two studies included considerably more female participants (Mitolo et al., 2017; Serino et al., 2017), the gender distribution in the remaining studies was approximately even.
Quality assessment
Studies included in this systematic scoping review used heterogeneous designs and differed significantly in methodological quality (Table 1). Six studies were rated as high quality while one study was of good quality. Three studies were classified as moderate quality, none of the studies were considered of poor quality. It should be noted that the studies with the lowest scores omitted to provide sufficient and structured descriptions of their randomization processes, thereby reducing reproducibility. For two of them, this may be a concern of outdated reporting structures since they were published in the 1980s (Willis and Schaie, 1986; Schaie et al., 1987). In summary, the overall quality of the study pool is moderate to high.
Intervention characteristics
The included studies (N = 10) differed in the interventions provided as well as the training methods (cf. Table 3). Whereas one study implemented a group intervention (Mitolo et al., 2017), all remaining studies performed one-on-one trainings. Of those, two studies trained their participants at home, one guided by a trainer (Willis and Schaie, 1986), the other one unaccompanied (Whitlock et al., 2012). All other studies provided training to their participants in a laboratory setting. In terms of training methods, one study implemented iPad-based mini-games (Binder et al., 2016), another study instructed participants to play an original computer game (Whitlock et al., 2012). In four studies participants performed active and passive movements in virtual environments (Lövdén et al., 2012; Wiener et al., 2012; Kober et al., 2013; Serino et al., 2017), one study conducted real-world behavioral training (Mitolo et al., 2017), one trained picture-based (Nemmi et al., 2017), and another study proceeded real-world spatial activities as well as cognitive spatial activities (Willis and Schaie, 1986). Schaie et al. (1987) did not provide detailed information about the type of training implemented. Moreover, training modalities differed across the studies, i.e., training periods ranged from one to 16 weeks with the number of sessions extending from five to 50 sessions in total, while the overall training duration varied from 100 min to 50 h with individual training sessions extending from 20 to 60 min. Two studies did not describe their training modalities in sufficient detail (Wiener et al., 2012; Nemmi et al., 2017). Finally, training frequency varied from one to seven sessions per week.
Spatial ability-related outcome measures
All experimental designs applied at least one form of spatial outcome measure with most studies utilizing more than one spatial ability-related assessment (cf. Table 3). These assessments recorded (visuospatial) short-term memory, variations of the Corsi Block Test (Kober et al., 2013; Binder et al., 2016; Mitolo et al., 2017; Serino et al., 2017), the City Map Path Learning Test (Binder et al., 2016), Pathway Span Task, Jigsaw Puzzle Test (Mitolo et al., 2017), the Spatial 2-back (Lövdén et al., 2012) or the Digit Span Test (Serino et al., 2017). Visual perception and visual memory were rated with the Benton Visual Retention Test and the Visual Pursuit Test (Kober et al., 2013). Moreover, spatial perception and mental rotation abilities were examined utilizing the 3D Spatial Orientation Test (Binder et al., 2016), Primary Mental Abilities Space, Object Rotation (Willis and Schaie, 1986; Schaie et al., 1987), Alphanumeric Rotation and the Mental Rotation Test (Lövdén et al., 2012; Whitlock et al., 2012), the Spatial Imagination Test (Kober et al., 2013) or Cube Comparison (Willis and Schaie, 1986). Further, Lövdén et al. (2012) and Kober et al. (2013) assessed VR route-finding performance or navigation performance tasks to evaluate route learning and route knowledge, while Wiener et al. (2012) and Nemmi et al. (2017) implemented intervention-specific route and direction tasks. Alternatively, the Route Learning Test and the Landmark Sequence Test were used to measure these spatial outcomes. Spatial orientation was assessed by the Object Perspective Tests (Whitlock et al., 2012) and the Guilford-Zimmerman Test (Lövdén et al., 2012). The Route Memory Test, the Location Memory Test and Object Position Memory were applied to test episodic memory (Lövdén et al., 2012). Moreover, executive function and spatial visualization were assessed using the Frontal Assessment Battery (Serino et al., 2017) and the Paper Folding Test (Whitlock et al., 2012), respectively. Mitolo et al. (2017) further applied various spatial questionnaires like the Sense of Direction and Spatial Representations Questionnaire, the Attitude to Environmental Tasks Questionnaire, the Spatial Anxiety Scale, and the Spatial Self-Efficacy Questionnaire. It should be noted that the majority of the reported assessments only partially depict spatial aspects of the complex cognitive process of spatial navigation. Therefore, they might reflect spatial abilities that contribute to spatial navigation to a certain degree, but are not sufficient to map this complex cognitive ability in its entirety.
Effects of spatial navigation training on spatial abilities
Visuospatial short-term/working memory
Five studies evaluated visuospatial short-term or working memory (Lövdén et al., 2012; Kober et al., 2013; Binder et al., 2016; Mitolo et al., 2017; Serino et al., 2017). After a specific route-learning training, significant between-group differences were found only for the backward version of the Corsi Block Test. Improvements were found in favor of the intervention group subsequent to the training phase and the follow-up-testing (Mitolo et al., 2017). A virtual route-finding training (Kober et al., 2013) and a virtual object-relocation training (Serino et al., 2017) did not lead to significant Corsi Block Test improvements in healthy older adults. Furthermore, no significant changes were found in the Digit Span Test (Serino et al., 2017), Jigsaw Puzzle Test (Mitolo et al., 2017) or the Spatial 2-back Test (Lövdén et al., 2012). The Pathway Span Test, however, yielded significant immediate and long-term gains in the intervention group (Mitolo et al., 2017). Binder et al. (2016) reported a composite score of the Corsi Block Forward Test, 3D-spatial orientation Test and the City Map Path Learning Test. They found a statistical trend for improvement in their spatial training group and their multi-domain training group, however, not in the groups performing inhibition or visuomotor function training. The raw score of the Benton Visual Retention Test improved significantly from pre-to post-test in healthy older adults, however, for the Visual Pursuit Test significant improvements emerged in response times, but not for the total score (Kober et al., 2013).
Mental rotation
Seven different tests for mental rotation and spatial perception abilities were performed in five studies. Binder et al. (2016) used the 3D Spatial Orientation Test as part of a composite score regarding spatial abilities. A positive trend was found for healthy older adults within the spatial and the multi-domain training group. The Primary Mental Abilities Space, the Object Rotation and the Alphanumeric Rotation Tests were used by Willis and Schaie (1986) and Schaie et al. (1987). While Schaie et al. (1987) did not perform a statistical evaluation of the intervention effects, Willis and Schaie (1986) added a Cube Comparison Test and found significant improvements in a composite score of these tests for older adults performing spatial navigation training. Whitlock et al. (2012) performed the Mental Rotation Test and found no significant changes for their video gaming intervention group or the passive control group, the same applies for the route and survey learning conducted by Lövdén et al. (2012). On the other hand, Kober et al. (2013) found significant improvements in spatial perception, conducting the Spatial Imagination Test.
Route and survey learning
Route learning was assessed in five studies. Kober et al. (2013) found significant route-finding improvements after their VR-based training. Significant improvements were also found for each of the three route-finding subtests used by Mitolo et al. (2017) after performing a behavioral spatial navigation-related intervention. Another study (Nemmi et al., 2017) used screenshots of crossroads to assess route learning. When participants had to decide on the following direction at each shown crossroad, reaction times improved significantly between training sessions one and two.
In a route direction task, both older and younger adults improved significantly comparing the first and the second half of the experiment (Wiener et al., 2012). In the same study, a route repetition subtask was conducted, the performance of the healthy older adults increased significantly. When participants were asked to indicate the direction required to return to the start location of the same route (route retracing), performance did not improve over time (Wiener et al., 2012). Performance differences were observed for the subsequent landmark sequence task, differentiating between the first half and the second half of the trials. Younger and older healthy adults improved significantly in this task. Survey learning as a spatial ability was addressed only once (Nemmi et al., 2017). Older adults significantly improved their performance in a pointing task, concerning the starting or ending point of a previously learned route. Lövdén et al. (2012) performed a task, which required combined route and survey learning. The old-aged intervention group performed significantly better after the training in a virtual zoo as well as compared to their age-matched walking control group.
Additional assessments
While Serino et al. (2017) found non-significant improvements regarding executive functions, measured by the Frontal Assessment Battery, Whitlock et al. (2012) did not find significant differences in spatial orientation and spatial visualization, measured by the Object Perspective Task and the Paper Folding Test, respectively. However, Lövdén et al. (2012) reported a non-significant trend towards improvement for spatial orientation tested by the Guilford-Zimmerman Spatial Orientation Test. Spatial episodic memory tested by Route Memory Test, Location Memory and Object-Position Memory did not improve after route and survey learning in a virtual zoo (Lövdén et al., 2012).
Questionnaires
None of the questionnaires regarding spatial orientation topics (e.g., sense of direction, spatial representation strategies, spatial anxiety and performance confidence in spatial tasks) showed changes from pre-to posttest.
Discussion
The aim of this scoping review was to outline the current body of evidence on spatial navigation interventions for healthy older adults to increase spatial abilities. In addition, the intervention characteristics, applied spatial ability-related outcome measures, and results were summarized to describe relevant training effects of spatial ability training in healthy older adults. A scoping review of the literature was selected as approach to map out a wide-ranging picture of the available research in this field while still maintaining methodological rigor and diminishing bias (Munn et al., 2018).
Summary of findings
A total number of 10 studies met the applied inclusion criteria and were subjected to a quality assessment. The quality of the included studies was moderate to high. Trials included a total number of 1,003 participants, 368 of those being healthy older adults and therefore target group of this scoping review. Overall, the study outcomes indicate that spatial abilities can be improved in healthy older adults by means of the designed training interventions. Six of nine studies reporting pre-post data found significant improvements for at least one of their spatial ability-related outcomes (Willis and Schaie, 1986; Lövdén et al., 2012; Wiener et al., 2012; Kober et al., 2013; Mitolo et al., 2017; Nemmi et al., 2017), however, applying a methodological variety of training protocols in terms of type of intervention, training periods and training durations. Aiming to identify the most relevant approaches to achieve spatial ability improvements in healthy older adults, several research gaps in the current literature were identified. While the available research is of moderate to high quality, the total number of published studies is low. Nevertheless, there is need for additional methodologically solid research including larger sample sizes, addressing specifically healthy older adults.
Characteristics of observed spatial navigation training interventions
The first aim of this scoping review was to summarize the characteristics of existing spatial navigation interventions to enhance spatial abilities in healthy older adults. A total number of 10 different interventions could be identified. Overall, the implemented training methods varied widely, active and passive movement approaches in virtual environments (Lövdén et al., 2012; Wiener et al., 2012; Kober et al., 2013; Serino et al., 2017) were used most frequently, followed by gamification approaches (Whitlock et al., 2012; Binder et al., 2016), as well as picture-based, cognitive, and real world-approaches (Willis and Schaie, 1986; Mitolo et al., 2017; Nemmi et al., 2017). A recent meta-analysis of combined cognitive-motor interventions revealed positive effects on global cognition in older adults (Wollesen et al., 2020). Despite these promising findings only two of the studies included in this review applied this dual-task type of training protocol (Lövdén et al., 2012; Mitolo et al., 2017) and improved spatial ability-related outcomes successfully. However, the extent of the motor components concurrent to the cognitive training was not stated clearly by Mitolo et al. (2017).
This variety continued for the applied training periods (1–16 weeks), the overall training duration (100 min–50 h) and the duration of training sessions (20–60 min).
From an exercise science point of view, the effect of cognitive-motor interventions is highly dependent on the training control and the conduction of the training load (e.g., progression, intensity; Herold et al., 2019; Wollesen et al., 2020). Improvements in cognitive-motor performance were shown following a minimum overall duration of 330 min of progressive training (Wollesen and Voelcker-Rehage, 2014). This duration was reached in two of the included studies (Lövdén et al., 2012; Mitolo et al., 2017), both showing improvements in spatial ability-related outcomes. In contrast, Kober et al. (2013) found significant improvements in implementing cognitive training in virtual reality with a total training duration of 100 min.
Characteristics of observed spatial ability-related outcome measures
The second aim was to give an overview of spatial ability-related measures to evaluate spatial navigation intervention effects in healthy older adults.
It became evident that the applied measures varied widely from established standardized tests (e.g., variations of the Corsi Block and Mental Rotation Test) addressing cognitive functions (e.g., visual–spatial short-term memory, mental rotation) to task-specific tests (e.g., route learning, survey learning) and spatial ability-related questionnaires. Six of the included studies applied standardized tests (Willis and Schaie, 1986; Schaie et al., 1987; Whitlock et al., 2012; Binder et al., 2016; Mitolo et al., 2017; Serino et al., 2017), while two of them used task-specific assessments (Wiener et al., 2012; Nemmi et al., 2017). It has to be assumed that spatial navigation is a complex multisensory process (Wolbers and Hegarty, 2010). However, a clear definition of spatial navigation and related cognitive processes is missing within the included studies. This hampers the attempt to link spatial cognitive tests with spatial navigation abilities.
Kober et al. (2013) as well as Lövdén et al. (2012) utilized a combination of standardized and task-specific tests. When evaluated by task-specific measurements (Wiener et al., 2012) or a combination of task-specific and standardized measurements (Lövdén et al., 2012; Kober et al., 2013), VR-based interventions led to significant improvements in at least one outcome measure. In contrast, when evaluated by standardized tests only, the VR-intervention failed to show significant improvements regarding spatial navigation abilities (Serino et al., 2017). Two gamified interventions made use of established outcome measures (Whitlock et al., 2012; Binder et al., 2016), both failing to reveal significant improvements. However, Binder and colleagues reported statistical trends of improvement for all their outcome measures. This might be due to the fact that Binder et al. (2016) developed iPad-based mini-games to specifically improve cognitive functions, while Whitlock et al. (2012) used a pre-existing computer game which had been developed without this purpose (World of Warcraft).
The studies performing cognitive training interventions using realistic scenarios (Nemmi et al., 2017: screenshots of existing crossroads; Mitolo et al., 2017: living areas in nursing homes), used either standardized measures (e.g., Forward Corsi Block Test; Mitolo et al., 2017) or task-specific measures (Route Task & Survey Task; Nemmi et al., 2017), both finding significant improvements in at least some of their spatial ability-related outcomes. In the study of Willis and Schaie (1986), a conceptual cognitive training and established assessments were conducted, and significant improvements were observed.
This overview depicts a broad variety of applied spatial ability-related outcome measures in relation to the different intervention approaches. The applied assessments depict partial aspects of the complex cognitive ability called spatial navigation, which needs to be considered when interpreting outcomes in terms of intervention effects. The majority of assessments capture small-scale spatial abilities only, which mainly cover two-and three-dimensional spatial visualization and spatial relations. Few of the used assessments survey large-scale spatial abilities, which refer to the concrete ability to orientate and navigate in complex large-scale environments (Yuan et al., 2019).
This does not allow for drawing conclusions on the eligibility of selected spatial ability-related outcome measures. Overall, it can be assumed that lab-based, standardized assessments of spatial abilities are suitable tools in basic research as they can depict specific cognitive aspects. At the same time, task-specific large-scale assessments might be more suitable to observe intervention effects since they illustrate the complex aspects of spatial navigation performance rather than underlying cognitive mechanisms. Furthermore, these tools could also be considered to reflect the transfer of the measured spatial ability to everyday spatial navigation more accurately.
Effectiveness of observed spatial navigation training interventions
The third study aim was to identify effective spatial navigation training interventions for healthy older adults. Effect sizes generally provide statistical evidence for comparing the range of intervention effects across studies. Six of nine studies reported statistically significant improvements in at least one of the spatial ability-related outcomes post training. Only one of these studies (Mitolo et al., 2017) reported effect sizes reflecting training gains for spatial measures in healthy older adults. Mitolo et al. (2017) calculated effect sizes using Cohen’s d and found a range from 0.1 up to 1.3, reflecting small to large intervention effects for the spatial measures (Cohen, 1992), which significantly improved after training. Some of the other studies also examined effect sizes comparing intervention and control group, but not pre-and posttest data for each group. Since no comparable training effects can be derived from these data, future studies should report the related effect sizes to enable a cross-study evaluation of training effects.
To classify the relevance of specific training approaches, it is also important to evaluate their transfer effects on everyday functioning (Weng et al., 2019). Transfer effects can be divided in near vs. far transfer effects (post-training improvements of the trained tasks vs. improvements in cognitive function and untrained cognitive tasks or performance in everyday situations, respectively, Barnett and Ceci, 2002). Improvements were detected for both forms of interventions addressing cognitive functions like fluid intelligence (Baltes et al., 1989), working memory (Strobach and Huestegge, 2017), or attention (Cassavaugh and Kramer, 2009). Two of the included studies generated near transfer outcomes (Wiener et al., 2012; Nemmi et al., 2017) conducting task-specific assessments (e.g., route learning in a virtual environment), while four of them collected rather far transfer outcomes referring to cognitive functions by standardized measurements (Whitlock et al., 2012; Binder et al., 2016; Mitolo et al., 2017; Serino et al., 2017), but not everyday life spatial navigation performance. Furthermore, Lövdén et al. (2012) and Kober et al. (2013) evaluated both near and far transfer effects, although they also did not cover the everyday life spatial navigation performance. For the studies performed by Schaie et al. (1987) as well as Willis and Schaie (1986) an assignment of transfer effects was inconclusive, as the intervention contents were not clearly described. Overall, none of the included studies applied assessments examining everyday spatial navigation performance. This renders the evaluation of intervention effects in terms of spatial navigation improvements in everyday life impossible.
Nonetheless, the encouraging findings of this scoping review are particularly valuable when assuming an association of efficient spatial abilities and life space-mobility. Since the constriction of life space increases the risk of frailty (Xue et al., 2008), nursing home admission (Sheppard et al., 2013), cognitive decline (Crowe et al., 2008) and social isolation (Taylor et al., 2019), it seems all the more sensible to counteract such life space reduction by promoting and maintaining spatial navigation abilities. Whether these findings are transferable to other population groups needs to be investigated.
Strengths and limitations
The main strength of this review is its structured and thorough methodological approach in line with the PRISMA Extension for Scoping Reviews (PRISMA-ScR; Tricco et al., 2018). As the literature search was performed in a structured, and reproducible manner, it can be assumed that nearly all relevant literature in this underexplored research field has been identified. This allows a comprehensive overview of the available evidence as well as the accentuation of gaps in knowledge. In addition, a quality assessment of the included studies was performed to classify their methodological quality as well as the significance of derived evidence.
Despite conducting a thorough search of the literature, only 10 studies met our inclusion criteria. This may be due to a variety of limiting factors. Even though the search strategy was inclusive, it cannot be ruled out that our choice of keywords and search strings impeded the detection of available literature. Further, only articles published in English were included, which might have led to the exclusion of additional relevant evidence in this emerging field of research. It appears that only little research has investigated this topic for this specific population group applying a structured study design including a pre-and posttest as well as at least three spatial navigation-related intervention sessions. Altogether, sample sizes of healthy older adults involved in the included studies were relatively low as this target group frequently served as control-or comparison group for individuals with pathological impairments or different age groups. Overall, the 10 included studies presented with a strong heterogeneity regarding their study designs, population characteristics, interventions, training modalities and outcome measures. This significantly hampered comparability of their findings. In line with the scope of this review, results were thus presented in a descriptive manner. Moreover, there is a need for diagnostically informative, comparable outcome measures with high reliability and validity that convey a meaningful picture of intervention effects.
Conclusion
The ability to navigate successfully through known and unknown environments determines health-relevant aspects in old age. It ascertains spatial range of motion, which in turn interacts with substantial skills promoting an independent and satisfying lifestyle. Even though the characteristics of spatial navigation interventions appear to be well established in pathological contexts, little is known about how to prevent deterioration of spatial abilities in healthy older adults.
Concerning the research questions, this systematic scoping review reveals a promising contribution of effective but methodologically diverse interventions, implementing training approaches, like cognitive and cognitive-motor trainings in virtual environments, computer games, picture-based and real-world scenarios. This diversity is also reflected in the assessments applied, like established, standardized tests, task-specific assessments, or spatial navigation-related questionnaires. This diversity in the methods limits the comparability of the resultant training effects. Thus, methodologically comparable studies should be conducted to create explicit recommendations for successful spatial navigation training in healthy older adults. Nevertheless, six of nine studies reported statistically significant improvements in at least one of the spatial ability-related outcomes post-training as well as far transfer effects.
Since brain plasticity has been proven for other complex cognitive abilities (e.g., executive functions) in healthy older adults, future research into specific spatial navigation interventions might be a valuable contribution to health prevention and thus not only improve the independence and quality of life in older persons but also take some of the load off the health care systems.
Overall, this scoping review revealed open aspects that should be addressed in future training studies. These are (a) studies have to provide a clear definition of spatial navigation and the involved cognitive processes that are addressed in the training, (b) future studies should report effect sizes for the effect of the training intervention to enable a cross-study evaluation (c) future studies should address the positive benefits of the specific training regimes on near and far transfer of underlying spatial cognitive abilities and (d) future studies should investigate the transfer of positive effects of training to daily activities and real-life navigation.
Future research should focus on reproducing and extending the available approaches. This could for example imply approaches like the comparison of different types of interventions (e.g., VR and real-life approaches) to identify the most promising training approaches. Additionally, the comparison of different training modalities could be beneficial to reveal efficient ways to yield improvements following established training principles. The assessment of life space-mobility may be a valuable addition to depict far transfer achievements of spatial ability training in everyday life.
Author contributions
MF and CM wrote the first draft of the manuscript. MF, CM, AW, C-PJ, TM, KG, and BW have been involved in the drafting and contributed significantly to the revision of this manuscript. All authors contributed to the article and approved the submitted version.
Funding
We acknowledge support by the German Research Foundation and the Open Access Publication Fund of TU Berlin.
Conflict of interest
The authors declare that the research was conducted in the absence of any commercial or financial relationships that could be construed as a potential conflict of interest.
Publisher’s note
All claims expressed in this article are solely those of the authors and do not necessarily represent those of their affiliated organizations, or those of the publisher, the editors and the reviewers. Any product that may be evaluated in this article, or claim that may be made by its manufacturer, is not guaranteed or endorsed by the publisher.
Supplementary material
The Supplementary Material for this article can be found online at: https://www.frontiersin.org/articles/10.3389/fpsyg.2022.867987/full#supplementary-material
Abbreviations
AD, Alzheimer’s disease; CG, control group; CT, controlled trial; IG, intervention group; HOA, healthy older adults; HYA, healthy younger adults; MMSE, Mini Mental State Examination; RCT, randomized controlled trial; RL, Route learning; SL, Survey learning; VR, virtual reality; VE, virtual environment.
References
Appollonio, I., Leone, M., Isella, V., Piamarta, F., Consoli, T., Villa, M. L., et al. (2005). The frontal assessment battery (FAB): normative values in an Italian population sample. Neurol. Sci. 26, 108–116. doi: 10.1007/s10072-005-0443-4
Baker, P., Bodner, E., and Allman, R. (2003). Measuring life-space mobility in community-dwelling older adults. J. Am. Geriatr. Soc. 51, 1610–1614. doi: 10.1046/j.1532-5415.2003.51512.x
Baltes, P. B., Sowarka, D., and Kliegl, R. (1989). Cognitive training research on fluid intelligence in old age: what can older adults achieve by themselves? Psychol. Aging 4, 217–221. doi: 10.1037/0882-7974.4.2.217
Barnett, S., and Ceci, S. (2002). When and where do we apply what we learn?: A taxonomy for far transfer. Psychol. Bull. 128, 612–637. doi: 10.1037/0033-2909.128.4.612
Binder, J., Martin, M., Zöllig, J., Röcke, C., Mérillat, S., Eschen, A., et al. (2016). Multi-domain training enhances attentional control. Psychol. Aging 31, 390–408. doi: 10.1037/pag0000081
Boccia, M., Bonavita, A., Diana, S., Di Vita, A., Ciurli, M., and Guariglia, C. (2019). Topographical disorientation: clinical and theoretical significance of long-lasting improvements following imagery-based training. Front. Hum. Neurosci. 13:0322. doi: 10.3389/fnhum.2019.00322
Borella, E., Carretti, B., and De Beni, R. (2007). Accertamento della Memoria negli Adulti. Firenze: Organizzazioni Speciali.
Boyle, P., Buchman, A., Barnes, L., James, B., and Bennett, D. (2010). Association Between life space and risk of mortality in advanced age. J. Am. Geriatr. Soc. 58, 1925–1930. doi: 10.1111/j.1532-5415.2010.03058.x
Bratfisch, O., and Hagmann, E. (2012). Räumliches Orientierungsvermögen (3D). Wiener Testsystem (WTS). Mödling, Austria: Schuhfried.
Burns, P. (1999). Navigation and the mobility of older drivers. J. Gerontol. Ser. B Psychol. Sci. Soc. Sci. 54B, S49–S55. doi: 10.1093/geronb/54B.1.S49
Cassavaugh, N., and Kramer, A. (2009). Transfer of computer-based training to simulated driving in older adults. Appl. Ergon. 40, 943–952. doi: 10.1016/j.apergo.2009.02.001
Claessen, M., van der Ham, I., Jagersma, E., and Visser-Meily, J. (2015). Navigation strategy training using virtual reality in six chronic stroke patients: A novel and explorative approach to the rehabilitation of navigation impairment. Neuropsychol. Rehabil. 26, 822–846. doi: 10.1080/09602011.2015.1045910
Colombo, D., Serino, S., Tuena, C., Pedroli, E., Dakanalis, A., Cipresso, P., et al. (2017). Egocentric and allocentric spatial reference frames in aging: A systematic review. Neurosci. Biobehav. Rev. 80, 605–621. doi: 10.1016/j.neubiorev.2017.07.012
Corsi, P. M. (1972). Human memory and the medial temporal region of the brain. Diss. Abstr. Int. 34, 891b.
Crowe, M., Andel, R., Wadley, V., Okonkwo, O., Sawyer, P., and Allman, R. (2008). Life-space and cognitive decline in a community-based sample of African American and Caucasian older adults. J. Gerontol. Ser. A Biol. Med. Sci. 63, 1241–1245. doi: 10.1093/gerona/63.11.1241
Curry, J., Patterson, M., Greenley, S., Pearson, M., and Forbes, C. (2021). Feasibility, acceptability, and efficacy of online supportive care for individuals living with and beyond lung cancer: a systematic review. Support Care Cancer 29, 6995–7011. doi: 10.1007/s00520-021-06274-x
Ekstrom, R. B., French, J. W., Harman, H., and Derman, D. (1976). Kit of factor-Referenced Cognitive Tests (revised edition). Educational Testing Service, Princeton, NJ.
Epstein, R., Patai, E., Julian, J., and Spiers, H. (2017). The cognitive map in humans: spatial navigation and beyond. Nat. Neurosci. 20, 1504–1513. doi: 10.1038/nn.4656
Erickson, K., and Kramer, A. (2008). Aerobic exercise effects on cognitive and neural plasticity in older adults. Br. J. Sports Med. 43, 22–24. doi: 10.1136/bjsm.2008.052498
Fricke, M., and Bock, O. (2018). Egocentric navigation is age-resistant: first direct behavioral evidence. Current Neurobio. 9, 69–75.
Gramann, K. (2013). Embodiment of spatial reference frames and individual differences in reference frame proclivity. Spat. Cogn. Comput. 13, 1–25. doi: 10.1080/13875868.2011.589038
Gramann, K., Müller, H. J., Eick, E. M., and Schönebeck, B. (2005). Evidence of separable spatial representations in a virtual navigation task. J. Exp. Psychol. Hum. Percept. Perform. 31, 1199–1223. doi: 10.1037/0096-1523.31.6.1199
Guilford, J. P., and Zimmerman, W. S. (1948). The Guilford-Zimmerman aptitude survey. J. Appl. Psychol. 32, 24–34. doi: 10.1037/h0063610
Härting, C., Markowitsch, H. J., Neufeld, H., Calabrese, P., Deisinger, K., and Kessler, J. (2000). Wechsler Memory Scale-Revised Edition. Bern, Switzerland: Hans Huber Verlag.
Head, D., and Isom, M. (2010). Age effects on wayfinding and route learning skills. Behav. Brain Res. 209, 49–58. doi: 10.1016/j.bbr.2010.01.012
Herold, F., Törpel, A., Schega, L., and Müller, N. G. (2019). Functional and/or structural brain changes in response to resistance exercises and resistance training lead to cognitive improvements–a systematic review. Eur. Rev. Aging Phys. Act. 16, 1–33. doi: 10.1186/s11556-019-0217-2
Iaria, G., Palermo, L., Committeri, G., and Barton, J. J. (2009). Age differences in the formation and use of cognitive maps. Behav. Brain Res. 196, 187–191. doi: 10.1016/j.bbr.2008.08.040
Jäger, A. O., Süss, H.-M., and Beauducel, A. (1997). Test für das Berliner Intelligenzstrukturmodell (BIS). Germany: Hogrefe.
Kennedy, R. E., Sawyer, P., Williams, C. P., Lo, A. X., Ritchie, C. S., Roth, D. L., et al. (2017). Life-space mobility change predicts 6-month mortality. J. Am. Geriatr. Soc. 65, 833–838. doi: 10.1111/jgs.14738
Kitchin, R. M. (1994). Cognitive maps: what are they and why study them? J. Environ. Psychol. 14, 1–19. doi: 10.1016/S0272-4944(05)80194-X
Kmet, L. M., Cook, L. S., and Lee, R. C. (2004). Standard quality assessment criteria for evaluating primary research papers from a variety of fields. Alberta Herit Found Med Res. 13, 1–22.
Kober, S. E., Wood, G., Hofer, D., Kreuzig, W., Kiefer, M., and Neuper, C. (2013). Virtual reality in neurologic rehabilitation of spatial disorientation. J. Neuroeng. Rehabil. 10, 17–13. doi: 10.1186/1743-0003-10-17
Kozhevnikov, M., and Hegarty, M. (2001). A dissociation between object manipulation spatial ability and spatial orientation ability. Mem. Cogn. 29, 745–756. doi: 10.3758/BF03200477
Kramer, A. F., Bherer, L., Colcombe, S. J., Dong, W., and Greenough, W. T. (2004). Environmental influences on cognitive and brain plasticity during aging. J. Gerontol. Ser. A Biol. Med. Sci. 59, M940–M957. doi: 10.1093/gerona/59.9.M940
Lannes, A., Bui, E., Arnaud, C., Raynaud, J. P., and Revet, A. (2021). Preventive interventions in offspring of parents with mental illness: a systematic review and meta-analysis of randomized controlled trials. Psychol. Med. 51, 2321–2336. doi: 10.1017/S0033291721003366
Lawton, C. A. (1994). Gender differences in way-finding strategies: relationship to spatial ability and spatial anxiety. Sex Roles 30, 765–779. doi: 10.1007/BF01544230
Lo, A. X., Rundle, A. G., Buys, D., Kennedy, R. E., Sawyer, P., Allman, R. M., et al. (2016). Neighborhood disadvantage and life-space mobility are associated with incident falls in community-dwelling older adults. J. Am. Geriatr. Soc. 64, 2218–2225. doi: 10.1111/jgs.14353
Lövdén, M., Schaefer, S., Noack, H., Bodammer, N. C., Kühn, S., Heinze, H. J., et al. (2012). Spatial navigation training protects the hippocampus against age-related changes during early and late adulthood. Neurobiol. Aging 33, 620.e9–620.e22. doi: 10.1016/j.neurobiolaging.2011.02.013
Mackey, D. C., Cauley, J. A., Barrett-Connor, E., Schousboe, J. T., Cawthon, P. M., Cummings, S. R., et al. (2014). Life-space mobility and mortality in older men: a prospective cohort study. J. Am. Geriatr. Soc. 62, 1288–1296. doi: 10.1111/jgs.12892
Mammarella, I. C., Toso, C., Pazzaglia, F., and Cornoldi, C.. (2008). BVS-Corsi. Batteria per la valutazione della memoria visiva e spaziale. Edizioni Erickson.
McGilton, K. S., Rivera, T. M., and Dawson, P. (2003). Can we help persons with dementia find their way in a new environment? Aging Ment. Health 7, 363–371. doi: 10.1080/1360786031000150676
Mitolo, M., Borella, E., Meneghetti, C., Carbone, E., and Pazzaglia, F. (2017). How to enhance route learning and visuo-spatial working memory in aging: a training for residential care home residents. Aging Ment. Health 21, 562–570. doi: 10.1080/13607863.2015.1132673
Moffat, S. D. (2009). Aging and spatial navigation: what do we know and where do we go? Neuropsychol. Rev. 19, 478–489. doi: 10.1007/s11065-009-9120-3
Moffat, S. D., Elkins, W., and Resnick, S. M. (2006). Age differences in the neural systems supporting human allocentric spatial navigation. Neurobiol. Aging 27, 965–972. doi: 10.1016/j.neurobiolaging.2005.05.011
Moher, D., Liberati, A., Tetzlaff, J., and Altman, D. G. (2011). Bevorzugte Report Items für systematische Übersichten und Meta-Analysen: Das PRISMA-Statement. DMW-Deutsche Medizinische Wochenschrift 136, e9–e15. doi: 10.1055/s-0031-1272978
Monaco, M., Costa, A., Caltagirone, C., and Carlesimo, G. A. (2013). Forward and backward span for verbal and visuo-spatial data: standardization and normative data from an Italian adult population. Neurol. Sci. 34, 749–754. doi: 10.1007/s10072-012-1130-x
Muffato, V., Meneghetti, C., and De Beni, R. (2020). The role of visuo-spatial abilities in environment learning from maps and navigation over the adult lifespan. Br. J. Psychol. 111, 70–91. doi: 10.1111/bjop.12384
Munn, Z., Peters, M. D., Stern, C., Tufanaru, C., McArthur, A., and Aromataris, E. (2018). Systematic review or scoping review? Guidance for authors when choosing between a systematic or scoping review approach. BMC Med. Res. Methodol. 18, 1–7. doi: 10.1186/s12874-018-0611-x
Nemmi, F., Boccia, M., and Guariglia, C. (2017). Does aging affect the formation of new topographical memories? Evidence from an extensive spatial training. Aging Neuropsychol. Cognit. 24, 29–44. doi: 10.1080/13825585.2016.1167162
O’Callaghan, G., O’Dowd, A., Stapleton, J., Merriman, N. A., Roudaia, E., and Newell, F. N. (2018). Changes in regional brain grey-matter volume following successful completion of a sensori-motor intervention targeted at healthy and fall-prone older adults. Multisens. Res. 31, 317–344. doi: 10.1163/22134808-00002604
Ouzzani, M., Hammady, H., Fedorowicz, Z., and Elmagarmid, A. (2016). Rayyan: A web and mobile app for systematic reviews. Syst. Rev. 5, 1–10. doi: 10.1186/s13643-016-0384-4
Park, D. C., and Bischof, G. N. (2013). The aging mind: neuroplasticity in response to cognitive training. Dialogues Clin. Neurosci. 15, 109–119. doi: 10.31887/DCNS.2013.15.1/dpark
Pazzaglia, F., Cornoldi, C., and De Beni, R. (2000). Differenze individuali nella rappresentazione dello spazio e nell’abilità di orientamento: Presentazione di un questionario autovalutativo. G. Ital. Psicol. 27, 627.
Pazzaglia, F., Poli, M., and De Beni, R. (2004). Orientamento e rappresentazione dello spazio. Attività per migliorare il senso dell’orientamento: leggere le mappe, memorizzare percorsi, organizzare viaggi. Edizioni Erickson.
Peters, M., Laeng, B., Latham, K., Jackson, M., Zaiyouna, R., and Richardson, C. (1995). A redrawn Vandenberg and Kuse mental rotations test-different versions and factors that affect performance. Brain Cogn. 28, 39–58. doi: 10.1006/brcg.1995.1032
Phillips, J., Walford, N., Hockey, A., Foreman, N., and Lewis, M. (2013). Older people and outdoor environments: pedestrian anxieties and barriers in the use of familiar and unfamiliar spaces. Geoforum 47, 113–124. doi: 10.1016/j.geoforum.2013.04.002
Portegijs, E., Rantakokko, M., Mikkola, T. M., Viljanen, A., and Rantanen, T. (2014). Association between physical performance and sense of autonomy in outdoor activities and life-space mobility in community-dwelling older people. J. Am. Geriatr. Soc. 62, 615–621. doi: 10.1111/jgs.12763
Provencher, V., Bier, N., Audet, T., and Gagnon, L. (2008). Errorless-based techniques can improve route finding in early Alzheimer's disease: a case study. Am. J. Alzheimers Dis. Other Dement. 23, 47–56. doi: 10.1177/1533317507307228
Saajanaho, M., Rantakokko, M., Portegijs, E., Törmäkangas, T., Eronen, J., Tsai, L. T., et al. (2015). Personal goals and changes in life-space mobility among older people. Prev. Med. 81, 163–167. doi: 10.1016/j.ypmed.2015.08.015
Schaie, K. W., Willis, S. L., Hertzog, C., and Schulenberg, J. E. (1987). Effects of cognitive training on primary mental ability structure. Psychol. Aging 2, 233–242. doi: 10.1037//0882-7974.2.3.233
Schmiedek, F., Lövdén, M., and Lindenberger, U. (2010). Hundred days of cognitive training enhance broad cognitive abilities in adulthood: findings from the COGITO study. Front. Aging Neurosci. 2, 1–10. doi: 10.3389/fnagi.2010.00027
Sejunaite, K., Lanza, C., Ganders, S., Iljaitsch, A., and Riepe, M. W. (2017). Augmented reality: sustaining autonomous way-finding in the community for older persons with cognitive impairment. J. Frailty Aging 6, 206–211. doi: 10.14283/jfa.2017.25
Serino, S., Pedroli, E., Tuena, C., De Leo, G., Stramba-Badiale, M., Goulene, K., et al. (2017). A novel virtual reality-based training protocol for the enhancement of the “mental frame syncing” in individuals with Alzheimer’s disease: A development-of-concept trial. Front. Aging Neurosci. 9:0240. doi: 10.3389/fnagi.2017.00240
Sheppard, K. D., Sawyer, P., Ritchie, C. S., Allman, R. M., and Brown, C. J. (2013). Life-space mobility predicts nursing home admission over 6 years. J. Aging Health 25, 907–920. doi: 10.1177/0898264313497507
Strobach, T., and Huestegge, L. (2017). Evaluating the effectiveness of commercial brain game training with working-memory tasks. J. Cogn. Enhanc. 1, 539–558. doi: 10.1007/s41465-017-0053-0
Sturm, W., Horn, W., and Willmes, K. (1993). Leistungsprüfsystem für 50-90jährige:(LPS 50+). Handanweisung: Hogrefe, Verlag für Psychologie.
Taylor, J. K., Buchan, I. E., and van der Veer, S. N. (2019). Assessing life-space mobility for a more holistic view on wellbeing in geriatric research and clinical practice. Aging Clin. Exp. Res. 31, 439–445. doi: 10.1007/s40520-018-0999-5
Thurstone, L. L. (1948). Psychological implications of factor analysis. Am. Psychol. 3, 402. doi: 10.1037/h0058069
Tricco, A. C., Lillie, E., Zarin, W., O'Brien, K. K., Colquhoun, H., Levac, D., et al. (2018). PRISMA extension for scoping reviews (PRISMA-ScR): checklist and explanation. Ann. Intern. Med. 169, 467–473. doi: 10.7326/M18-0850
Vandenberg, S. G., and Kuse, A. R. (1978). Mental rotation, a group test of three-dimensional spatial visualization. Percept. Mot. Skills 64, 907–914.
Vienna Test System. Psychological assessment [Internet]. (2022). Schuhfried. Available at: http://psychologischtesten.nl/wp-content/uploads/Catalogus-Vienna-Test-System-SCHUHFRIED_Psychologischtesten.nl_.pdf (Accessed January 13, 2022).
Weng, W., Liang, J., Xue, J., Zhu, T., Jiang, Y., Wang, J., et al. (2019). The transfer effects of cognitive training on working memory among Chinese older adults with mild cognitive impairment: a randomized controlled trial. Front. Aging Neurosci. 11, 212. doi: 10.3389/fnagi.2019.00212
Whitlock, L. A., Collins McLaughlin, A., and Allaire, J. C. (2012). Individual differences in response to cognitive training: using a multi-modal, attentionally demanding game-based intervention for older adults. Comput. Hum. Behav. 28, 1091–1096. doi: 10.1016/j.chb.2012.01.012
Wiener, J. M., Kmecova, H., and de Condappa, O. (2012). Route repetition and route retracing: effects of cognitive aging. Front. Aging Neurosci. 4, 7. doi: 10.3389/fnagi.2012.00007
Willis, S. L., and Schaie, K. W. (1986). Training the elderly on the ability factors of spatial orientation and inductive reasoning. Psychol. Aging 1, 239–247. doi: 10.1037/0882-7974.1.3.239
Wolbers, T., and Hegarty, M. (2010). What determines our navigational abilities? Trends Cogn. Sci. 14, 138–146. doi: 10.1016/j.tics.2010.01.001
Wollesen, B., and Voelcker-Rehage, C. (2014). Training effects on motor–cognitive dual-task performance in older adults. Eur. Rev. Aging Phys. Act. 11, 5–24. doi: 10.1007/s11556-013-0122-z
Wollesen, B., Wildbredt, A., van Schooten, K. S., Lim, M. L., and Delbaere, K. (2020). The effects of cognitive-motor training interventions on executive functions in older people: a systematic review and meta-analysis. Eur. Rev. Aging Phys. Act. 17, 1–22. doi: 10.1186/s11556-020-00240-y
Xue, Q. L., Fried, L. P., Glass, T. A., Laffan, A., and Chaves, P. H. (2008). Life-space constriction, development of frailty, and the competing risk of mortality: the Women's health And aging study I. Am. J. Epidemiol. 167, 240–248. doi: 10.1093/aje/kwm270
Keywords: healthy older adults, cognitive aging, spatial orientation, wayfinding, spatial navigation
Citation: Fricke M, Morawietz C, Wunderlich A, Muehlbauer T, Jansen C-P, Gramann K and Wollesen B (2022) Successful wayfinding in age: A scoping review on spatial navigation training in healthy older adults. Front. Psychol. 13:867987. doi: 10.3389/fpsyg.2022.867987
Edited by:
Ralf Thomas Krampe, KU Leuven, BelgiumReviewed by:
Sabine Schaefer, Saarland University, GermanyIneke Van Der Ham, Leiden University, Netherlands
Copyright © 2022 Fricke, Morawietz, Wunderlich, Muehlbauer, Jansen, Gramann and Wollesen. This is an open-access article distributed under the terms of the Creative Commons Attribution License (CC BY). The use, distribution or reproduction in other forums is permitted, provided the original author(s) and the copyright owner(s) are credited and that the original publication in this journal is cited, in accordance with accepted academic practice. No use, distribution or reproduction is permitted which does not comply with these terms.
*Correspondence: Madeleine Fricke, bS5mcmlja2VAdHUtYmVybGluLmRl
†These authors have contributed equally to this work and share the first authorship