- 1Department of Biological Psychology, Faculty of Behavioural and Movement Sciences, Vrije Universiteit, Amsterdam, Netherlands
- 2School of Exercise and Health, Shanghai University of Sport, Shanghai, China
- 3Amsterdam Public Health Research Institute, Amsterdam University Medical Center, Amsterdam, Netherlands
A much-cited model by Stodden and colleagues has proposed motor competence to be a 17 promising target for intervention to increase childhood physical activity. Motor competence is thought to influence future physical activity through bidirectional causal effects that are partly direct, and partly mediated by perceived motor competence and physical fitness. Here, we argue that the model is incomplete by ignoring potential confounding effects of age-specific and age-invariant factors related to genetics and the shared family environment. We examined 106 systematic reviews and/or meta-analyses on the Stodden model for the mention of familial confounding. These reviews summarized data from 1,344 primary studies on children in the age range 0–18 on the associations in five bidirectional pathways: motor competence—physical activity, motor competence—perceived motor competence, perceived motor competence—physical activity, motor competence—physical fitness, and physical fitness—physical activity. We show that a behavioral genetic perspective has been completely lacking from this vast literature, despite repeated evidence for a substantial contribution of genetic and shared environmental factors to motor competence (h2 = ♂55%—♀58%; c2 = ♂31%—♀29%), physical fitness (h2 = ♂65%—♀67%; c2 = ♂3%—♀2%), and physical activity (h2 = ♂37%—♀29%; c2 = ♂33%—♀49%). Focusing on the alleged causal path from motor competence to physical activity, we find that the systematic reviews provide strong evidence for an association in cross-sectional studies, but weak evidence of prediction of physical activity by motor competence in longitudinal studies, and indeterminate effects of interventions on motor competence. Reviews on interventions on physical activity, in contrast, provide strong evidence for an effect on motor competence. We conclude that reverse causality with familial confounding are the main sources of the observed association between motor competence and physical activity in youth. There is an unabated need studies on the interplay between motor competence, perceived motor competence, physical fitness, and physical activity across early childhood and into adolescence, but such studies need to be done in genetically informative samples.
1 Introduction
1.1 The importance of physical activity in children and adolescents
The paramount importance of regular physical activity (PA) to enhance children’s health has been extensively documented (Elhakeem et al., 2018; Janssen and Leblanc, 2010; Jose et al., 2011; Kaplan et al., 1996; Leskinen et al., 2009; Wendel-Vos et al., 2004). The well-established effects of physical activity have led to the development of physical activity guidelines for youth, widely adopted across the globe (World Health Organization, 2020). Despite this, and the many active policies supporting an increase in physical activity in various settings, the majority of children and adolescents does not meet recommended physical activity levels (Guthold et al., 2020). Furthermore, as children move through childhood and adolescence towards adulthood, physical activity participation rates tend to further decline (Conger et al., 2022).
Of note, these general epidemiological trends describe what happens to the average child but fail to address the large individual differences in physical activity behaviors. These individual differences have been shown to be remarkably stable throughout the lifespan (Breau et al., 2022; Telama et al., 2005; van der Zee et al., 2019) such that children who start out to be more physically active in childhood tend to remain more active later in life. This ‘tracking’ of physical activity suggests that it would pay off to increase the number of active children to arrive at larger numbers of adolescents and adults meeting the recommended physical activity levels. Not surprisingly therefore, much effort has been spent on identifying modifiable determinants of childhood physical activity. One of the more promising traits investigated is motor competence (Øglund et al., 2015; Øglund et al., 2014). Globally, children (3–10 years) demonstrate “below average” to “average” motor competence levels (Bolger et al., 2021), suggesting that there is room for improvement of this trait by targeted intervention. However, such intervention is only meaningful to increase youth physical activity levels to the extent that motor competence has a causal effect on physical activity.
1.2 The role of motor competence in physical activity: the 2008 Stodden model
Motor competence can be defined as the full complement of a person’s motor abilities needed to execute all forms of goal-directed motor acts necessary to manage everyday tasks (Bolger et al., 2021; Henderson and Sugden, 1992). The potential role of motor competence for physical activity received a large boost with the development of the “Stodden model” by Stodden et al. (2008). The Stodden model identifies motor competence as a main determinant of youth and adolescent physical activity, a basic idea foreshadowed by the earlier work of Hands and Larkin (2002). To be physically active as they grow older, children need fundamental motor skills like running, jumping, catching, and throwing. Children that start out with low actual and perceived motor competence may not engage in sufficient physical activity to develop the motor competence and physical fitness needed to engage in the required level of physical activity during middle and late childhood. This will draw them into a negative spiral of disengagement in which the lower levels of physical activity in turn will amplify their motor skill deficits compared to their more active peers. “This will ultimately result in high levels of physical inactivity and will place these individuals at risk for being obese during later childhood, adolescence, and adulthood. “(Stodden et al., 2008, p. 297).
Three characteristics of the Stodden model turn it into a dynamic but complex model that make it difficult to predict the development of stable physical activity habits as well as moments that would be optimal for change by intervention. First, it adds two mediational pathways, acting through physical fitness and through perceived motor competence, to the direct pathways between motor competence and physical activity. Second, it suggests non-recursive, reciprocal effects in the direct and mediated pathways. Third, the model allows changes in the direction of effects in the pathways as a function of age. While being very complete by incorporating age-moderation, bidirectionality, and mediation, the Stodden model at the same time is undercomplete by solely focusing on the possibility that these pathways reflect causal effects.
1.3 The potential confounding by familial factors in pathways of the Stodden model
In its essence, the Stodden model revolves around a set of five associations between motor competence and physical activity, between motor competence and perceived motor competence, between perceived motor competence and physical activity, between motor competence and physical fitness, and between physical fitness and physical activity (MC-PA, MC-PMC, PMC-PA, MC-Fitness, Fitness-PA). These associations can arise through fundamentally different mechanisms governing the development and the ensuing stability of the associations between the traits as well as the changes in these associations over time. Figure 1 depicts potential sources of the association between motor competence and physical activity at each of three different ages, and how these sources can impact on the stability of these associations across developmental time. To maintain intelligibility, the Figure greatly simplifies the continuous nature of development by using discrete ages 2, 7, and 13, rather than a more fine-grained model that uses steps of, e.g., 2 months. It’s aim is merely to provide an illustration of the complexity of interpreting (longitudinal) associations.
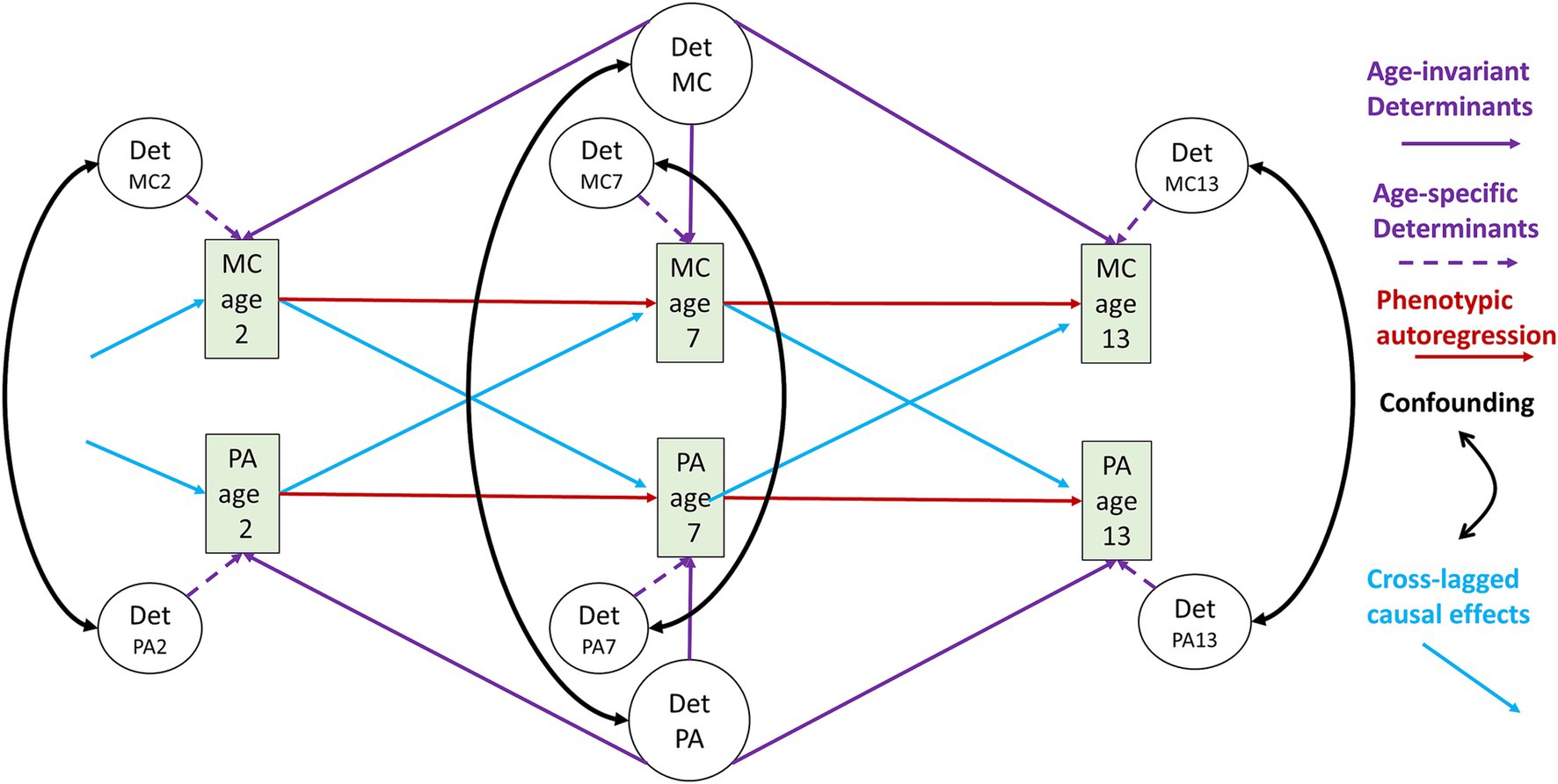
Figure 1. Sources of the association between motor competence and physical activity and its stability over time. Rectangles contain the observed values of the motor competence (MC) and physical activity (PA) traits at the three example ages (2, 7, 13). Ovals contain the set of latent determinants of these traits (DetMC, DetMC2, DetMC7, DetMC13 and DetPA, DetPA2, DetPA7, DetPA13) which may be genetic or environmental determinants. DetMC2, DetMC7, DetMC13 contain the age-specific latent genetic/environmental determinants of motor competence operating on MC at age 2, 7 and 13, respectively. DetMC contains the age-invariant latent genetic/environmental determinants that operate on the traits at all ages. Similar applies to DetPA, DetPA2, DetPA7, DetPA13. Dotted purple arrows from DetMC2, DetMC7, DetMC13 and DetPA2, DetPA7, DetPA13 reflect age-specific effects of these determinants, whereas the solid purple arrows from DetMC and DetPA reflect age-invariant effects of these determinants. Double-headed black arrows indicate correlation of the underlying determinants, which leads to confounding in the MC-PA associations. Red continuous lines indicate the autoregression of the motor competence and physical activity traits across time. Blue arrows indicate true causal effects of motor competence on physical activity, or in reverse, physical activity on motor competence. Blue arrows pointing into age 2 reflect causal effects from earlier ages.
As a major innovation to the Stodden model, Figure 1 adds latent determinants that may act as confounders of the associations between motor competence and physical activity, or its putative mediators, perceived motor competence and physical fitness. Two sets of latent determinants have been repeatedly nominated by the field of behavior genetics to play a role in many developmental traits. The first set of determinants consists of the common or shared environment that contains all factors shared by family members living in the same household, including the physical home environment, family warmth and mutual support, parenting style and example setting, neighborhood characteristics, and socioeconomic status (including education level of the parents). The second set consists of the genetic variance shared by family members which may reflect additive trait effects of the two parental alleles in a gene, or non-additive trait effects due to allelic dominance or allelic interaction (epistasis).
Starting at the top of the model shown in Figure 1, we see that motor competence at age 2 (“MC age 2”) is considered to be influenced by latent determinants (“Det MC2”). These may involve genetic variants that influence sensorimotor brain functioning and neuromuscular control, or differences in motor skill challenges related to the family that children grow up in or other environmental factors such as climate or exposure to structured physical education in school or childcare settings. The influence of these genetic and environmental determinants of motor competence can show substantial stability over time (reflected in the purple arrows emanating from the latent “Det MC” factor that influences motor competence at all ages) because the genetic code does not noticeably change after conception, and influences related to parental rearing styles, and neighborhood or household characteristics can also be stable. However, the influence of some of the latent determinants may be confined to specific ages (reflected in the latent “Det MC2 … Det MC13” factors). For example, parental social support effects may be strong at ages 2 and 7 but become more diluted when children enter secondary school. While some genetic variants may be expressed at all ages, other variants may show age-specific (suppression of) gene-expression as part of maturation. At the bottom of the figure, we see a parallel situation for physical activity, again with both age-invariant and age-specific latent factors influencing physical activity behaviors at the three ages depicted.
At each age, an association between motor competence and physical activity may arise entirely through the correlation of the age-invariant and/or age-specific factors, without the need for a direct causal path between the two traits. For example, part of the many genetic variants that influence motor competence may overlap with those influencing physical activity, creating horizontal genetic pleiotropy when they influence these traits through independent routes (Minica et al., 2020; Minica et al., 2018; Solovieff et al., 2013; Verbanck et al., 2018). Likewise, environmental risk factors like household poverty and parental rearing styles may independently restrict motor competence development and reduce opportunities for regular physical activity. If the effects of genetic or environmental factors change in strength from childhood to adolescence, they may also cause a strengthening or weakening of the associations over time. Such a confounder-induced age-related change in the association would not be discriminable from an age-moderation effect on the putative causal pathway between motor competence and physical activity.
The confounding genetic or environmental effects may work directly on the two traits themselves, but also make use of an intermediate trait that itself exerts a causal effect on both traits. A first example would be that the same genetic variants that influence motor competence also influence physical activity through their effects on the dopaminergic brain systems that influence motor control as well as exercise reward pathways. A second example of such confounding would be that an obesogenic family environment would increase body mass index (BMI), with BMI having effects on both motor competence and physical activity. In short, cross-sectional associations between motor competence and physical activity at each age can reflect confounding by correlated determinants, which may be genetic or environmental in nature.
However, the effect of the latent underlying factors does not rule out the additional existence of causal effects of motor competence at an earlier age on current physical activity. These causal effects are reflected in the cross-lagged paths of Figure 1. For example, physical activity at age 7 may, in part, depend on the ability to perform basic motor actions at a sufficient level to engage in active play with parents, siblings, or peers at school from age 2 to 7. Conversely, the lagged causal effect may also work in the other direction. Daily engagement in physical activity from age 2 to 7, i.e., playing regular ball games in preschool, may actively contribute to building up motor competence, i.e., lead to increased kicking/throwing skills, at age 7. Such bidirectional causal mechanisms are suggested by the Stodden model as the main cause of the association between motor competence and physical activity in middle and late childhood.
Apart from the mechanisms inducing cross-sectional associations at each age, Figure 1 also depicts the mechanisms that lead to stability of the association of motor competence and physical activity over developmental time. A first mechanism causing stability of the associations between motor competence and physical activity is the autoregression of each of the traits separately. Substantial evidence shows that, even if absolute levels show large maturational changes, the individual differences in both motor competence and physical activity are stable across time (Barnett et al., 2010; Branta et al., 1984; Farooq et al., 2020; Malina, 1990; McKenzie et al., 2002; Pereira et al., 2022; Schmutz et al., 2020). This ‘tracking’ of motor competence and physical activity may arise from direct causal influences of the trait level at a starting age on the trait level at a later age. For example, once a neuromotor skill has been mastered (running, balancing on a beam) it will not be easily lost, and habit formation may solidify physical activity behaviors once these have been taken up in an initial period (Rebar et al., 2024).
Autoregression can be a first source of stability of the association of motor competence and physical activity over time. Once an association has come into existence, e.g., is bootstrapped at age 2 by correlated underlying determinants, it will be propagated across time sheerly by the stability in each of the two traits. A second mechanism that leads to stability of the association of motor competence and physical activity over time are the causal effects of motor competence on physical activity and the reverse causal effects of physical activity on motor competence. Finally, a third mechanism causing stability of the association is a correlation of the age-invariant genetic or environmental determinants of motor competence and physical activity (“Det PA” and “Det MC” in Figure 1). These will not just induce cross-sectional association but also longitudinal associations between motor competence and physical activity.
The Stodden model was created when the associations between the traits in the five pathways (MC-PA, MC-PMC, PMC-PA, MC-Fitness, Fitness-PA) were mostly observed in cross-sectional studies. Cross-sectional studies cannot discriminate between the mechanisms outlined in Figure 1 and outlined above. Nonetheless, if one of the hypothesized associations in the Stodden model is found to be absent, it would at once tell us that no causal effect is likely to exist. In that sense, cross-sectional studies are vital in first demonstrating the primary possibility of a causal association. Longitudinal studies are a step up from cross-sectional studies in that they establish the presence of cross-time (lagged) associations between the traits and can rule out reversed causation. If the assumed causal trait (e.g., motor competence at an early age) is seen to predict the assumed caused trait (e.g., physical activity in adolescence) in the future but in parallel, the association is not seen to hold in the opposite direction this would falsify reverse causation of motor competence by physical activity.
The strongest design to show true causality in the pathways of the Stodden model is the intervention design, where either motor competence or physical activity are manipulated, and it is tested whether the induced changes in one trait led to changes in the other trait. Well-conducted RCTs remain the highest level of evidence for a true causal effect. However, large individual differences can be seen in the response to intervention and it is not always clear what is driving these differences (Kennedy et al., 2021; Liu et al., 2024; Ma et al., 2021; Prochaska et al., 2008). Again familial factors are a potential source of the heterogeneity in responding to intervention. Attempts to increase motor competence and physical activity may fall on more fertile ground in some children compared to others, simply based on their genetic abilities and/or more supportive family environment. So, to further add to complexity, the underlying determinants of motor competence and physical activity in Figure 1 may partly act through their moderating effects of (parental or school-based) attempts to change these traits.
If the genetic and shared environmental determinants independently influence motor competence and physical activity behavior, the size of the causal effects hypothesized to underlie the observed association between motor competence and physical activity behavior would be incorrectly estimated from the size of the association when this confounding is not taken into account. Since the publication of the Stodden model, a very large amount of systematic reviews with or without meta-analyses have been published on one or more of the Stodden pathways. A reasonable expectation, therefore, is that this large volume of work has duly taken the potential of familial confounding into account. Cursory inspection of some highly cited reviews (Barnett et al., 2022; De Meester et al., 2020; Engel et al., 2018; Figueroa and An, 2017) suggested that this might not be the case, but more systematic inspection of the large volume of systematic reviews is needed. In addition, for familial confounding to be a potential issue, it is required that the traits in the Stodden model show substantial variance caused by shared environmental or genetic factors. This requires a review of studies on these traits in the behavioral genetics literature.
1.4 The aims of this narrative review
The first aim of this narrative review is to examine whether and how shared environmental or genetic confounding had been considered, and possibly ruled out, in the large body of literature on the five pathways in the Stodden model. To do so, we inspected all systematic reviews and meta-analysis of primary studies published after 2008 and searched for discussions on potential confounding by genetic and shared environmental factors.
As our second aim, we compare the strength of the evidence and effect sizes obtained for the effect of motor competence on physical activity in cross-sectional and longitudinal designs. This path of the Stodden model is important for intervention studies aiming to increase middle and late childhood physical activity. If familial confounding is present in this main Stodden pathway, we expect cross-sectional associations to be stronger than longitudinal associations. In addition, we expect that interventions on motor competence would not increase physical activity to the degree predicted from the cross-sectional effect sizes.
As a third aim, we explicitly test the potential for familial confounding in the five bidirectional pathways of the Stodden model. This requires that the variance in the traits in the Stodden model in childhood and adolescence are caused by genetic and shared environmental factors. This can be tested in a nuclear family design (e.g., parental, spousal and sibling correlations) or in a wider pedigrees (correlations between, e.g., self-aunt/uncle, self-niece/nephew, etc.), but the strongest design focuses on the comparison of MZ and DZ twin correlations (Knopik et al., 2017; Polderman et al., 2015). We, therefore, review the existing twin studies on the contribution of genetic and shared environmental factors to each of the traits in the Stodden model. Furthermore, we review direct tests of familial confounding that estimate the overlap in genetic and shared environmental factors influencing multiple traits, e.g., between motor competence and physical activity.
In short, our research questions are:
1. To what extent have past systematic reviews and meta-analyses on the pathways of the Stodden model considered unmeasured confounding, in by particular genetic and shared environmental factors?
2. In the main pathway between motor competence and physical activity, are the reported cross-sectional associations stronger than longitudinal associations that in turn are stronger than the effects seen in intervention studies?
3. Do twin studies show that, during childhood and adolescence, genetic and shared environmental factors contribute to individual differences in the traits used in the Stodden model?
2 Method
2.1 Search and selection of systematic reviews and meta-analyses on the Stodden model
To address research question 1, a literature search was performed for systematic reviews and/or meta-analyses of the relationships between motor competence, physical activity, perceived motor competence, and physical fitness. Definitions and assessment strategies for these traits can be found in the Supplementary Sections 1, 2. We searched the Pubmed, Web of Science, and EMBASE databases for reviews published after January 1, 2009 (i.e., after the publication of the Stodden model) and before January 15, 2025 (date of final search). The detailed search strategy is shown in Supplementary Methods Section 3’. Of note, for physical activity traits we only extracted results on total physical activity (TPA), moderate-to-vigorous physical activity (MVPA) or leisure time physical activities (LTPA, including exercise and sports) but discarded light physical activity and sedentary behavior.
The extracted titles and abstracts were initially screened by YZ to identify reports fulfilling the inclusion criteria. Articles were stored in the Endnote citation manager. Full-text reading of selected systematic reviews and meta-analyses was performed independently by YZ and EdG. Discrepancies in article selection were discussed and resolved. References were checked to identify additional systematic reviews and meta-analyses on the traits in the Stodden model.
2.1.1 Inclusion and exclusion criteria
We included peer-reviewed systematic reviews or meta-analyses of observational or interventional studies in humans that assessed the association between any two of the four traits. Only reviews published in English with a focus on participants younger than 18 years old were included. We excluded reviews where the traits from the Stodden model were not among the primary outcomes, or were no association statistics or intervention effects were reported. We excluded reviews on special populations such as youth athletes, children with medical problems or psychiatric conditions. Figure 2 provides a flow diagram describing the selection of the reviews included in the data extraction and analysis step.
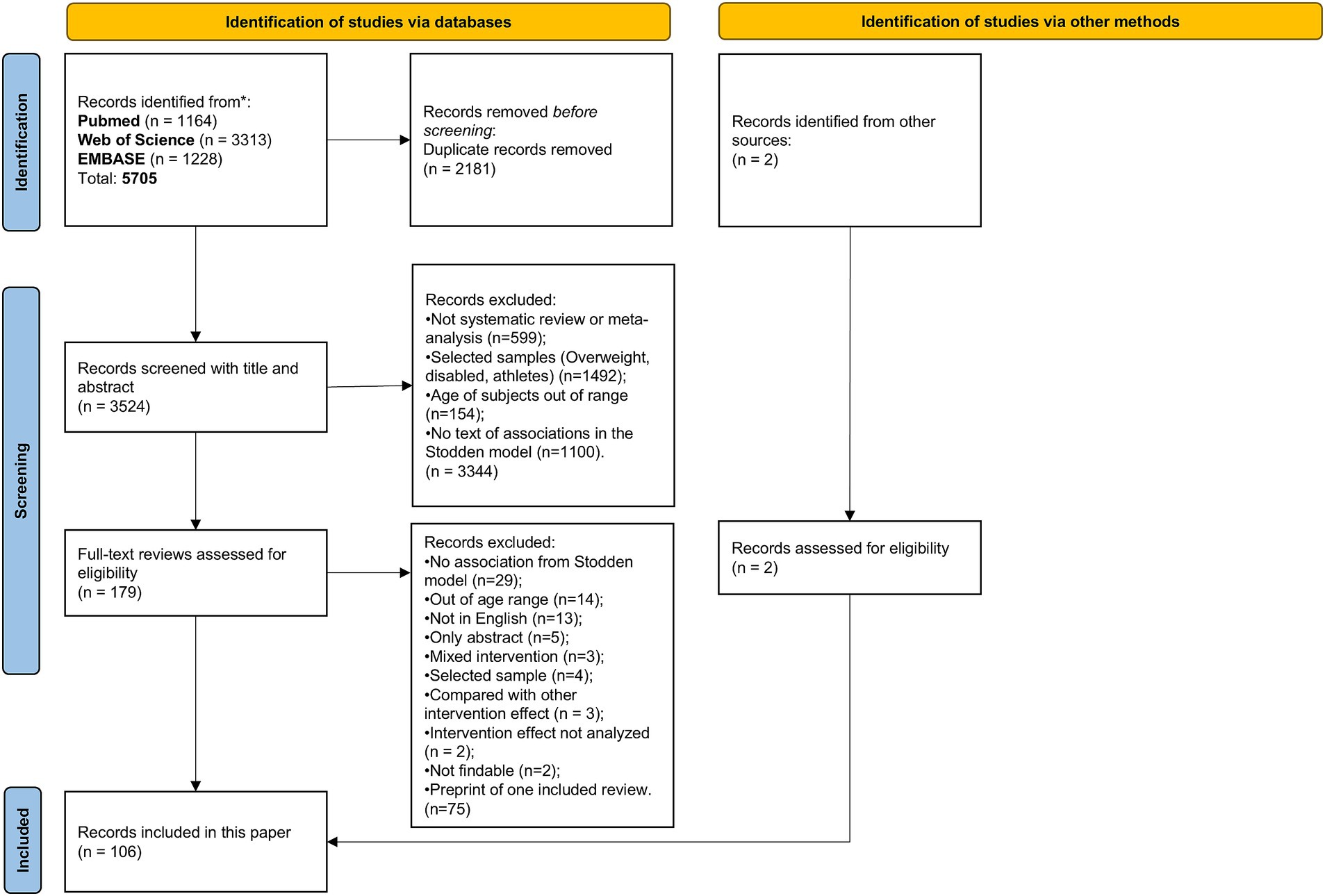
Figure 2. Flow diagram describing the selection of the systematic reviews and meta-analyses on the core pathways of the Stodden model.
2.1.2 Data extraction
We extracted the authors of the systematic reviews, year of publication, presence of a meta-analysis, period covered by the search used, age range of the target population, number of primary studies included in the review, and the study design of the primary studies, i.e., (cluster) randomized controlled trial, non-randomized interventional studies, longitudinal, or cross-sectional studies (see Table 1). We then scrutinized the text of the discussion and conclusion sections of the reviews for mention of concerns about unmeasured confounding in general, and more specifically about genetic and shared environmental confounding. This process was repeated by both authors, and an automated text search for the keywords ‘risk of bias’, ‘confound*’, ‘familial’, ‘environment*’, ‘genetic*’ and ‘heritab*’ was used to verify our manual inspection. All information was extracted separately for all pathways (e.g., motor competence and physical activity, perceived motor competence and actual motor competence, motor competence and fitness, etc.) and ordered by age within each pathway.
2.1.3 Strength of evidence and effect sizes in the motor competence—physical activity pathway
To address research question 2, we extracted additional data on the overall strength of evidence and average effect sizes reported by the included reviews on the main bidirectional pathway of the Sodden model between motor competence and physical activity (see Table 2). The information was separately provided per study design, ordered by age groups (early childhood ~2–5 years of age; middle childhood ~6–12 years of age; and adolescence ~13–18 years of age), and further by the subdomains of the traits (e.g., for motor competence, subdomains like object control skills or balancing skills).

Table 2. Design characteristics and main findings from the reviews on the association between motor competence and physical activity.
The rating for the level of evidence in support of a pathway was based on an adaptation of the methodology developed by Sallis et al. (2000) and later revised by Barnett et al. (2022). Based on the percentage of findings in the primary studies supporting the association according to the systematic review, a pathway was classified as a non-significant (coded as “0”) when only 0 to 33% of studies reported a significant association, or when no significant meta-analytic effects across four or more studies were found. A pathway was classified as an inconsistent or indeterminate (coded as “?”) association when between 34 and 59% of the primary studies reported a significant association or when less than four primary studies in total reported a significant association. Also, a significant meta-analytic effect across less than four primary studies was considered indeterminate. A pathway was classified as strong (coded as “+” or “-”, depending on the direction of the association) when ≥60% of four or more primary studies supporting a significant association, or a significant meta-analytic effect across four or more primary studies was found. The ≥60% criterion to consider evidence “strong” may appear strict but takes into account that there is considerable concern about publication bias towards significant results in sports science in general (Pesce, 2012) and in the specific domain of motor development/physical activity studies (Barnett et al., 2022).
For the effect sizes for the associations/effects reported we used the meta-analytic estimates when a meta-analysis was present. For systematic reviews without meta-analysis, the average value reported in the review was used, or when no average was reported, we computed the median across reported results for the primary studies. The effect sizes were categorized into three types. Following Cohen (1988) and Peterson and Brown (2005) the effect was considered small if the pooled correlation was between 0.10 < r < 0.30, the meta-analytic estimate (Hedges g, Cohen’s d, or standardized mean difference (SMD)) was between 0.2 to 0.5, or the standardized regression β was between 0.05 and 0.25. The effect size was considered moderate if the pooled correlation was between 0.30 < r < 0.50, the meta-analytic estimate (Hedges g, Cohen’s d, or SMD) was between 0.5 to 0.8, or the standardized β was between 0.25–0.45. The effect was large if the pooled correlation was >0.50, the meta-analytic estimate (Hedges’ g, Cohen’s d or SMD) was >0.8, or the standardized β was ≥0.45.
2.2 Twin studies on the traits in the Stodden model
To address research question 3 on the potential for familial confounding, we retrieved all twin studies on the four traits of the Stodden model. The twin design compares the intra-pair resemblance between two types of sibling relationships; genetically identical twins or monozygotic (MZ) twins, a result of division of a single fertilized egg during an early stage in embryonic development, and non-identical twins or dizygotic (DZ) twins, resulting from two separate fertilized eggs (de Geus, 2023). Consequently, MZ twins are genetically identical and the difference between the twins is due to person-specific environmental factors, i.e., experiences that one of the twins has and the co-twin does not. Dizygotic twins shared on average 50% of their genetic make-up. In contrast to familial aggregation studies, that cannot separate genetic and familial environmental sources of covariance, twin studies can decompose all phenotypic variance of the trait of interest into sources of additive (‘A’) and non-additive (‘D’) genetic influences shared environmental influences (influences shared with other family members, e.g., upbringing; referred to as ‘C’) and person-specific environmental influences (influences unique to the individual; referred to as ‘E’).
2.2.1 Search and selection of behavioral genetics studies
We built on the multiple recent reviews conducted by our group (de Geus, 2023; van der Zee and de Geus, 2019; Zi et al., 2023a; Zi et al., 2023b), but additionally added publications from 2023 and 2024. We searched PubMed and Web-of-Science from January 1980 to December 2024 using the keywords (‘Physical Activity’ OR Exercise OR Sports OR Lifestyle OR Fitness OR Endurance OR Strength OR VO2*) AND (Gene* OR Twin OR Family OR Familial OR Heritability) AND “Humans” [MeSH terms]. Reference sections of selected papers were used to identify additional papers missed by these search terms. We included all studies addressing univariate or multivariate genetic and environmental contributions to one or more of the four traits of the Stodden model using a twin design. Studies with less than 50 complete twin pairs were excluded. We also removed studies reporting on twins with a mean age higher than 18 and. In some studies, the same twin sample was re-used for slightly different research questions. For example, two studies (Huppertz et al., 2017; van der Aa et al., 2010) used overlapping samples with three other studies (Aaltonen et al., 2020; Aaltonen et al., 2013; Huppertz et al., 2016). If the exact same PA trait was used, we only extracted data from the study using the largest sample size. For the studies conducted by Maia and colleagues we used data presented in their 2013 summary (Maia et al., 2013). Finally, we discarded results on light physical activity or sedentary behavior and limited inclusion to total PA, moderate-to-vigorous PA, and leisure time PA including structured sports and exercise participation.
2.2.2 Data extraction
We extracted the authors, year of publication, country, mean age and range of the target population, the Stodden trait(s) examined, the number of MZ and DZ twin pairs, the measurement strategy used for the trait examined, and the estimates of genetic and shared environmental contributions to the traits. Non-additivity (D) was generally not found (or modeled) by the twin studies, so we extracted only the A and C parameters. We preferentially used the A and C parameters estimates from full ACE models; when only reduced AE models were reported we followed the authors in their assumption that C was zero, but note that a small effect of C in these studies may have been undetected due to low power. When sex differences in the A or C parameters were tested, the differential male and female results are reported. When they were not tested or explicitly found to be absent, the same estimates are reported for males and females. When multiple models with different covariates were tested, we selected those that only corrected for age and sex.
3 Results
3.1 Reporting of confounding by genetic and shared environmental factors
Our search detected 106 systematic reviews, of which 67 added a meta-analysis, on a total of 1,344 unique primary studies that examined one or more of the associations implied by the Stodden model. Table 1 lists all systematic reviews and meta-analyses and Supplementary Table 1 reports on the primary studies, their study design, sample size, and in which systematic reviews and meta-analyses they had been included.
There were 44 systematic reviews addressing the bidirectional MC-PA pathway, 4 systematic reviews on the bidirectional MC-PMC pathway, 5 systematic reviews on the bidirectional PMC-PA pathway and 10 systematic reviews on the path from MC to Fitness. By far the largest amounts of systematic reviews (n = 54) were done on the path from physical activity to muscular or cardiorespiratory fitness (PA-Fitness) which is a major area of interest in pediatric exercise science. No reviews included primary studies on the path from fitness to MC or fitness to PA, as manipulation of fitness without also manipulating PA is not feasible in children. Many of the systematic reviews on a specific pathway used overlapping sets of the primary studies, and the more recent reviews generally used the largest number of primary studies.
The patterns in the numbers of the primary studies largely followed that of the systematic reviews (see Supplementary Table 1). There were 426 primary studies reporting on the MC-PA pathway, 102 primary studies on the MC-PMC pathway, 74 primary studies reporting on the PMC-PA pathway, and 100 studies reporting on the MC-fitness pathway. Again, the vast majority of primary studies (n = 937) reported on the PA-fitness pathway. As expected, the PA-fitness and MC-fitness studies exclusively tested the effect of MC/PA on fitness traits (not the reverse path). The total number of children and adolescents that have participate in the primary studies testing the Stodden design is over 1.2 million participants.
The very large amount of primary studies described by the systematic reviews should allow us to uncover whether and how past studies on the Stodden model have taken confounding by genetic and shared environmental factors into account. We rely on the authors of the systematic reviews and meta-analyses to have explicitly reported on this. Table 1 reports on our inspection of the discussion sections of the systematic reviews and meta-analyses on text related to potential familial confounding. Our inspection revealed that almost all reviews have taken the potential of unmeasured confounding into account as part of the quality rating the primary studies as prescribed by various guidelines for systematic reviews and meta-analyses, e.g., STROBE, PRISMA, and GRADE (Balshem et al., 2011; Page et al., 2021; von Elm et al., 2007). The quality of the primary studies was often judged to be low to moderate, and it was rarely rated good (see Supplementary Methods Table 4.1). These low scores might in principle have been caused by the majority of primary studies not taking into account potential confounder effects on the associations obtained in the study. We, therefore, considered studies reporting risk of bias as having mentioned ‘general confounding’ (marked by √ in the column of Table 1).
Despite this guideline-enforced attention to the risk of bias, as expressed in study quality scores, explicit mention and discussion of (sources of) familial confounding was very rare. For example, in the main MC-PA pathway, aspects of the family environment were explicitly mentioned as a potential source of confounding in only 5 out of the 44 systematic reviews on this pathway. Most cited shared environmental confounders were parental socioeconomic status and parental social support which may influence both motor competence and physical activity (Barnett et al., 2016; Øglund et al., 2015; Xin et al., 2020). Strikingly, clear mention or discussion of genetic confounding was absent. Only Barnett and colleagues, in their authoritative review of 2022, wrote: “The broad scope of this review meant that we could not assess how other relevant variables (e.g., diet, genetics, cultural settings, growth and maturation, cognition, motivation) related to the core variables in the model.” This indirect allusion is the only reference to genetics in the context of confounding in 106 systematic reviews and meta-analyses.
3.2 Strength of evidence and effect sizes in the association between motor competence and physical activity by study design
Forty-four systematic reviews (n = 22) and meta-analyses (n = 22) specifically investigated the direct or mediated paths between motor competence and physical activity in both directions (see Table 2 for details). Of the reviews, 25% reported on a cross-sectional design, 12% on a longitudinal, 32% on an intervention design and 31% were (cluster) RCTs. Many reviews reported on multiple analyses of the MC-PA association from the same primary studies. Separate results were given for the total score for motor competence, and/or scores for all or one of the specific domains of locomotor, object, and stability skills. Separate results were also given for different types of physical activity, including either TPA or MVPA and cardiorespiratory as well as strength training activities.
3.2.1 Cross-sectional studies
Thirteen systematic reviews (n = 8) or meta-analyses (n = 5) investigated the association between motor competence and physical activity mostly based on primary studies using a cross-sectional design (Barnett et al., 2016; Bingham et al., 2016; Burton et al., 2023; Figueroa and An, 2017; Holfelder and Schott, 2014; Jones et al., 2020; Liu et al., 2023; Logan et al., 2015; Lubans et al., 2010; Poitras et al., 2016; Santos et al., 2023; Xin et al., 2020; Xu et al., 2024). In early childhood, strong evidence for an association between motor competence and TPA or MVPA was found in 12 out of the 22 analyses but indeterminate or no evidence was detected in 10 analyses (Bingham et al., 2016; Figueroa and An, 2017; Jones et al., 2020; Liu et al., 2023; Logan et al., 2015; Santos et al., 2023; Xin et al., 2020; Xu et al., 2024) (see Table 2). The association was much more robust in middle to late childhood, as well as in samples with larger age ranges that included adolescence, with the evidence unanimously strong in all 11 analyses (Barnett et al., 2016; Burton et al., 2023; Holfelder and Schott, 2014; Logan et al., 2015; Lubans et al., 2010; Poitras et al., 2016). Averaged (and meta-analytic) effect sizes were between small and moderate, corresponding to a correlation coefficient of ~0.25. The analyses using the separate fundamental motor skills yielded a very comparable pattern.
3.2.2 Longitudinal studies
Six systematic reviews (n = 2) or meta-analyses (n = 4) evaluated longitudinal studies in which motor competence was used as the predictor of future physical activity (Barnett et al., 2022; Graham et al., 2022; Holfelder and Schott, 2014; Jones et al., 2020; Øglund et al., 2015; Xin et al., 2020) or in which physical activity was used as the predictor of future motor competence (Barnett et al., 2022; Jones et al., 2020; Xin et al., 2020). Strong evidence for predictive effect of motor competence on physical activity was found in only 3 out of the 7 analyses and indeterminate evidence in 4 analyses (see Table 2). The predictive effects of motor competence seemed to hinge mostly on locomotor and stability skills, with no prediction of future physical activity by object skills (Barnett et al., 2022). No different pattern was seen in early versus middle/late childhood, and the effect sizes were typically small.
When a reverse relationship was examined, indeterminate (3 analyses) or no evidence (2 analyses) was found for a predictive effect of physical activity on future motor competence (Barnett et al., 2022; Jones et al., 2020; Xin et al., 2020).
3.3 Intervention studies
Twenty-nine systematic reviews (n = 10) or meta-analyses (n = 19) (Behringer et al., 2011; Carson et al., 2017; Chen et al., 2024; Collins et al., 2019b; Comeras-Chueca et al., 2021; Dudley et al., 2011; Engel et al., 2018; García-Hermoso et al., 2020; Grady et al., 2025; Hassan et al., 2022; Hesketh et al., 2017; Johnstone et al., 2018; Li et al., 2022; Liu et al., 2020; Lorås, 2020; McDonough et al., 2020; Moon et al., 2024; Norris et al., 2016; Oppici et al., 2022; Poitras et al., 2016; Rico-González, 2023; Sinclair and Roscoe, 2023; Sun and Chen, 2024; Timmons et al., 2012; Van Capelle et al., 2017; Veldman et al., 2021; Wang and Zhou, 2024; Zeng et al., 2017; Zhang et al., 2024) directly addressed the causality in the association between motor competence and physical activity by including intervention studies only. Of these, only two reviews focused on the crucial path of the Stodden model where an increase in motor development would lead to an increase in physical activity levels (Engel et al., 2018; Hesketh et al., 2017). Indeterminate evidence at best was found that intervention on motor competence increases physical activity levels, either in early or in middle childhood samples.
All other 27 reviews included primary studies that tested the reverse effects of physical activity interventions on motor competence, the majority using RCTs. Fifteen of these reviews focused on early childhood. Thirteen of these (12 out of 16 analyses) reported strong evidence for a causal effect of physical activity on motor competence, whether expressed in a total score or in separate scores for locomotor and object control skills. Three reviews (4 out of 16 analyses) reported indeterminate evidence (Johnstone et al., 2018; Liu et al., 2020; Oppici et al., 2022). When reported, the average effect sizes of the PA interventions in early childhood varied from small to large, with larger effect sizes seen when PA was mixed with a deliberate motor skills training component (Chen et al., 2024; Wang and Zhou, 2024). Twelve reviews focused on middle-childhood reporting on RCTs using physical activity intervention to improve motor competence in. Strong evidence (13 out of the 17 analyses) was found that increasing physical activity improved a trait in the MC domain, in all but one review. No, or indeterminate, evidence was found in only 4 of the analyses (see Table 2).
3.4 Potential confounding by familial factors
The systematic search for twin studies on the four Stodden traits uncovered only five studies for motor competence (Goetghebuer et al., 2003; Peter et al., 1999; Smith et al., 2017; Zi et al., 2024; Zi et al., 2023b) and none for perceived motor competence. In contrast, very many twin studies were found that reported on cardiorespiratory or muscular fitness and physical activity traits (see Table 3).
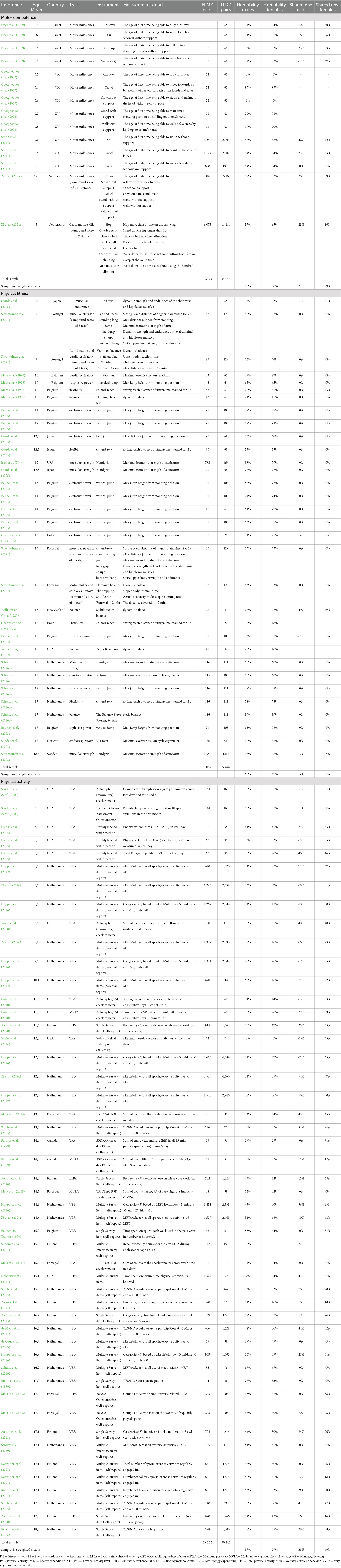
Table 3. Twin studies on the genetic and shared environmental contribution to the variance in motor competence, physical fitness and physical activity.
3.4.1 Genetic and shared environmental effects on motor competence
The top part of Table 3 lists the twin studies on motor competence. High heritability (~55%) and substantial effects of the shared family environment on explained variance (~35%) were found for early motor development in boys and girls as reflected in the timing of early motor milestones development (Goetghebuer et al., 2003; Peter et al., 1999; Smith et al., 2017; Zi et al., 2023b). Shared environmental factors still played a major role in gross motor competence at age 5, but the relative contribution to the total variance was only half to one-third (23% vs. 48% for boys, 16% vs. 48% for girls) of that for motor milestone achievement at age 2 (Zi et al., 2024). At age 5, the individual differences in the mother-reported mastery of seven gross motor skills were even more heritable than age-2 motor milestones attainment in both boys (57% vs. 43%) and girls (65% vs. 44%).
3.4.2 Genetic and shared environmental effects on physical activity
The bottom part of Table 3 lists the twin studies that tested the heritability of physical activity traits. A variety of methods were used, and physical activity was measured either as a total weekly activity score or a score reflecting moderate-to-vigorous activity, often restricted to leisure time activities in particular structured sports and exercise activities. The latter have the advantage of being more reliably assessed by self-report (van der Zee et al., 2020). While the different instruments and traits used induced some heterogeneity in the estimates for heritability and shared environmental influences, this heterogeneity strongly attenuates when only the larger samples are considered. These mostly converge on the sample-size weighted averages across all detected twin studies. On average, 29% of the variance in physical activity in girls was caused by genetic factors and 49% was caused by shared environmental factors. In boys, on average 37% of the variance in physical activity in boys was caused by genetic factors and 33% was caused by shared environmental factors. Heritability estimates from device-based measures of physical activity were very comparable to those from survey-based measures.
3.4.3 Genetic and shared environmental effects on physical fitness
The middle part of Table 3 lists the twin studies that tested the genetic contribution to individual differences in physical fitness in childhood and adolescence, a main mediator in the Stodden model. Three studies reported on the heritability of V.O2max, an index of cardiorespiratory fitness. Heritability estimates varied between 60 and 69% (Maes et al., 1996; Schutte et al., 2019; Schutte et al., 2016b; Sundet et al., 1994). The other twin studies focused on explosive power, muscular strength, muscular endurance, flexibility, and balance. For example, muscular strength measured by the handgrip test was assessed in four large studies with an average heritability of 70% (Isen et al., 2014; Okuda et al., 2005; Schutte et al., 2016b; Silventoinen et al., 2008). A number of studies also measured balancing skills often using tests that strongly overlap with tests used in the motor competence domain. Heritability estimates for balance ability ranged from 27 to 48%.(Maes et al., 1996; Schutte et al., 2019; Schutte et al., 2016b; Vandenberg, 1962; Williams and Gross, 1980).
Averaging across the various fitness measures, the sample-size weighted averages for heritability was 65% for boys and 67% for girls. Of note is that the shared environmental factors were not seen to meaningfully contribute to the variance in physical fitness in youth. Any confounding of pathways in the Stodden model relying on fitness, therefore, would be restricted to genetic confounding.
4 Discussion
The potential role of motor competence for physical activity received a large boost with the development of the “Stodden model” by Stodden and coworkers in 2008. The Stodden model has inspired a large volume of work, with 106 systematic reviews and/or meta-analyses detected by our literature search reporting on 1,344 primary papers. Many of the systematic reviews and meta-analyses end by a plea for interventions on motor competence in order to ensure future mental and physical health. This enigmatic call-to-intervention reflects the laudable desire to provide societally useful knowledge. This desire does not, however, absolve us form the obligation to provide a strong evidence base that intervention on motor competence is directly causal to increases in youth physical activity levels. Such causality is implied by the original Stodden model.
From a behavioral genetic perspective, we suggested that shared environmental and genetic confounders should be added to the model (see Figure 1). We then examined the systematic reviews and meta-analyses on the Stodden model to see if, and to what extent, this potential familial confounding, and in particular genetic confounding had already been taken into account. We find that confounding by shared environmental factors, which include household/neighborhood/parental rearing style characteristics, did receive cursory attention. However, essentially none of the past systematic reviews or meta-analyses of the cross-sectional or longitudinal observational studies on the Stodden model had considered, let alone ruled out, genetic confounding. We identify the lack of attention to familial confounding, specifically genetic confounding, as a huge knowledge gap in the fields of motor development and youth public health.
In contrast to cross-sectional or longitudinal observational studies, studies using experimental interventions on traits of the Stodden model rule out familial confounding by design. Particularly, in the form of RCTs, they remain the preferred method of assessing causal effects. However, not all paths of the Stodden model are amenable to intervention testing. Changing fitness other than by manipulating physical activity levels is near impossible, as it would require giving, e.g., blood doping to young children. This rules out an experimental test of an effect of fitness on physical activity, an effect hypothesized by one of the mediating pathways of the Stodden model. Likewise, manipulating perceived motor competence without changing actual motor competence is hard, if not unfeasible, which complicates experimental testing of the other mediating pathway in the Stodden model.
Manipulating motor competence by specific motor skill training without overly increasing physical activity levels is feasible in principle, although we note that most interventions on motor competence also increase the amount of physical activity as part of the motor skills training. Nonetheless, these intervention on fundamental motor skills are closest to an experimental test of a causal effect of motor competence on future physical activity in the core pathway of the Stodden model. Strikingly, the only two reviews focused such interventions found indeterminate evidence at best that intervention on motor competence increases physical activity levels, either in early or in middle childhood samples (Engel et al., 2018; Hesketh et al., 2017). This suggests that the hypothesized causal path from motor competence to physical activity does not contribute to the observed association between these two traits.
The most feasible intervention to test a number of the hypothesized paths in the Stodden model are those involving a direct increase in physical activity itself. Indeed, the majority of interventions studies on the Stodden model have focused on the effects of extra physical education lessons, and post-school sports and exercise activities. The systematic reviews on intervention studies provide strong evidence for an effect of physical activity on increased motor competence and fitness, even unanimously so in middle and late childhood. This is in keeping with established knowledge in pediatric exercise and suggest that the cross-sectional association between motor competence and physical activity reflects reverse causality. We note, however, that causal effects of physical activity on motor competence do not rule out the potential for additional familial confounding. In addition, the systematic reviews, often observed a large heterogeneity in the intervention effects on motor competence, both across studies but also within primary study samples. We hypothesize that these individual differences in the response to intervention may largely be attributable to genetic or shared environmental confounding. We, therefore, also searched whether the systematic reviews and meta-analyses on intervention studies on the Stodden model had considered familial factors as potential moderators of variation in outcomes across primary studies applying an intervention. Again, we found no single mention of this possibility in the 106 systematic reviews and meta-analyses on interventions on physical activity.
4.1 Potential for familial confounding in the motor development–physical activity pathway
To examine whether the potential for familial confounding was real, we tested the core requirements for such confounding in the motor development - physical activity pathway: (1) the individual differences in motor competence are heritable and/or caused by shared environmental factors, (2) the individual differences in physical activity are heritable and/or caused by shared environmental factors, and (3) there is significant overlap in either the genetic or the shared environmental factors influencing both motor competence and physical activity. We used twin studies on the traits in the Stodden model as our main vehicle.
In our past work, we reviewed family and twin studies on physical activity and fitness traits in childhood and adolescence (de Geus, 2023) but Table 3 provides an update incorporating a number of recent large twin studies on motor competence (Zi et al., 2023a; Zi et al., 2024; Zi et al., 2023b). Across different types of physical activity, across different countries, and across assessment methods (device-based or self-report), we show heritability of physical activity to be around 29% for girls and 37% for boys. Shared environment contributes 49 and 33% to the variance in motor competence in girls and boys, respectively. These findings signal that the first two of the requirements for potential familial confounding are met. The remaining condition for confounding is a substantial overlap in either the genetic or the shared environmental factors influencing both motor competence and physical activity. This question has only been directly addressed in a single paper so far (Zi et al., 2024). Using longitudinal data across a 12-year time span in a large population-based sample of MZ and DZ twins, they investigated the prediction of future exercise behavior by early motor development. Early motor development explained 4.3% of the variance in future exercise behavior in boys but only 1.9% in girls. In boys, there was evidence for a significant overlap in the genetic factors influencing early motor development and future exercise behavior, while the regressions between the shared and unique environmental factors were not significant. In girls, neither genetic nor shared and unique environmental regressions were significant, possibly reflecting low power to detect such effects at this very low amount (1.9%) of explained variance.
4.2 Mediational pathways in the Stodden model
Three systematic reviews on the Stodden model explicitly examined the evidence for mediation of the association between motor competence and physical activity by perceived motor competence or physical fitness (Barnett et al., 2022; Jones et al., 2020; Robinson et al., 2015). Most comprehensive testing was done by Barnett et al. (2022). They conclude that there is indeterminate evidence for mediation by perceived motor competence across the age range 3 to 18 years. In contrast, mediation by physical fitness of the path between physical activity and (future) motor competence, and the reverse path were supported by strong evidence. However, just as is true for the direct associations, associations mediated by fitness could be due to genetic and shared environmental factors independently acting on the traits in the mediating paths, i.e., genetic or environmental confounding of the MC-fitness and fitness-PA pathways.
Past systematic reviews and meta-analyses had shown that, throughout childhood and adolescence, individual differences in cardiorespiratory and muscular fitness are dominated by genetic factors with only a minimal role for shared environmental factors (Miyamoto-Mikami et al., 2018; Schutte et al., 2016b; Zi et al., 2023a). The update on this literature shown in Table 3 confirms this with, on average, 65–67% of the variance in physical fitness traits in both girls and boys being explained by genetic factors, with negligible shared environmental effects. Given the heritability of motor competence and physical activity, this additional heritability of fitness compromises testing of the mediation of the association between motor competence and physical activity by physical fitness. By correcting the direct path for fitness, we correct for the genetic factors influencing fitness. If these genetic factors partly overlap with either those of motor competence or those of physical activity, this correction would attenuate the association between motor competence and physical activity, mimicking ‘mediation by fitness.’
Direct support for a genetic overlap between fitness traits and physical activity comes from bivariate modeling in adolescent and young adult twin studies that assessed cardiorespiratory and muscular fitness phenotypes and daily regular exercise levels, both cross-sectionally and longitudinally (Schutte et al., 2019). This confirmed that physical fitness and physical activity behaviors are genetically overlapping, with genetic correlations between endurance capacity (VO2max) and regular exercise and sports activities in leisure time as high as 0.43. In short, the substantial contributions of genetic factors to motor competence, physical fitness, and physical activity provide a clear potential for genetic confounding in the ‘mediating’ paths using physical fitness.
4.3 How to detect and correct for familial confounding in the Stodden model?
We conclude that to more completely test the pathways in the model by Stodden et al. (2008), studies are needed with designs that can detect and account for potential genetic and shared environmental confounding. In the past we were dependent on the use of behavioral genetics studies in (extended) twin families to achieve this. However, despite the elegance of the twin design, not all researchers will have readily access to twin family data. Fortunately, nowadays we can also rely on DNA sampling and genome-wide genotyping in the participants of any cohort, the added costs of which have gone down to ~50 Euro per participant. From these genome-wide genotype data we can create genetic instruments for Mendelian Randomization and extract polygenetic indexes (PGI’s) using the publicly available current (and upcoming) summary statistics of genome-wide association studies (GWAS) on motor competence, physical fitness, and physical activity.
These two methods can be used to test causal hypotheses while controlling for confounding. Mendelian Randomization (MR) is the experiment of nature that comes most close to an RCT in which participants are allocated to different exposure levels independently of confounding. Like an RCT, MR also rules out reverse causality, but uses nature’s randomization to lifetime exposure to DNA variants that influence the exposure (Davey-Smith and Hemani, 2014; Pingault et al., 2018; Speed et al., 2019). These are determined at birth and an advantage of MR over RCTs is that it captures effects of prolonged exposure to, e.g., a propensity to develop motor skills, rather than the mere weeks or months of motor skill training within an RCT. If a genetic instrument is also available for the outcome, e.g., a set of single nucleotide polymorphisms (SNPs) significantly associated with physical activity, bidirectional MR can explicitly test a possible reciprocal causal relationship between exposure (motor competence) and outcome (physical activity).
When our basic concern is to adjust for genetic confounding of an association, e.g., between motor competence and physical activity, the computation of a PGI is another useful strategy (Choi et al., 2020). If a DNA sample with genome-wide SNP genotyping is available for the study participants, the PGI for motor competence and physical activity can be computed using the summary statistics of GWAS on these traits. The PGI is the sum of all the (tiny) genetic effects on the trait across all hundreds or thousands of SNPs that the GWAS detected as meaningfully contributing to the trait (Kujala et al., 2020). If there is genetic confounding, such that the genetic risk for low motor competence is causing (future) low levels of physical activity, then stratifying observed cross-sectional or longitudinal associations for deciles of the PGI for motor competence should lead to attenuation/disappearance of the motor competence—physical activity relationship within each decile of the PGI. If the relationship does not attenuate in the genetically stratified analysis, the association is shown to not depend on genetic confounding. This means that even individuals with a high genetic vulnerability for low motor competence would see their physical activity levels increased by interventions on their motor competence. Indeed, computing the polygenetic propensity for the traits of the Stodden model can also be used to strongly enrich intervention trials. As explained above, the heterogeneity in responsivity to motor competence or physical activity interventions may be partly due to genetic factors. Intervention trials in youth targeting motor competence or physical activity could start to account for this genetic moderation of intervention effects, simply by adding sampling of DNA in the trial participants to the research protocol and using their PGIs as moderator variables.
To apply the MR and PGI approaches to evaluate the Stodden model, we need publicly available summary statistics of large international consortia performing meta-analyses of genome-wide association studies on hundreds of thousands of children. Such summary statistics based on child samples are currently only available early motor coordination (Mountford et al., 2021) but the sample sizes of this GWAS was relatively modest. Larger GWAS efforts on motor milestones in much large samples are currently underway (Gui et al., 2024). However, for physical activity (Doherty et al., 2018; Klimentidis et al., 2018; Wang et al., 2022) and muscular (Willems et al., 2017) and cardiorespiratory fitness (Klevjer et al., 2022), we only have GWAS results based on adult samples, and the authors have not seen clear initiatives for GWAS on these traits in youth. This is where future work is direly needed because we cannot simply assume that the same genetic variants operate throughout the lifespan.
In summary, our synthesis of reviews leads us to fully support the viewpoint expressed earlier by Barnett et al. (2022) and Burton et al. (2023): To truly test the model authored by Stodden et al. (2008) the field is in need of robust longitudinal studies across early childhood and into adolescence. We agree with their recommendations that such longitudinal assessment should aim to include multiple traits from the model, use a combined motor competence assessment (i.e., process and product), and account for biological maturation. However, we offer an update to these recommendations by a strong plea for doing such studies in genetically informative samples that can quantify and account for potential confounding in all pathways of the Stodden model.
Data availability statement
The original contributions presented in the study are included in the article/Supplementary material, further inquiries can be directed to the corresponding author/s.
Author contributions
YZ: Conceptualization, Data curation, Formal analysis, Funding acquisition, Investigation, Visualization, Writing – original draft, Writing – review & editing. EG: Conceptualization, Data curation, Formal analysis, Supervision, Validation, Visualization, Writing – original draft, Writing – review & editing.
Funding
The author(s) declare that no financial support was received for the research and/or publication of this article.
Acknowledgments
The authors thank all the twin families registered in the Netherlands Twin Registry (NTR) for their support of scientific research. We acknowledge the input from various colleagues participating in the COST Action CA19101 Determinants of Physical Activities in Settings (DE-PASS), supported by COST (European Cooperation in Science and Technology).
Conflict of interest
The authors declare that the research was conducted in the absence of any commercial or financial relationships that could be construed as a potential conflict of interest.
Publisher’s note
All claims expressed in this article are solely those of the authors and do not necessarily represent those of their affiliated organizations, or those of the publisher, the editors and the reviewers. Any product that may be evaluated in this article, or claim that may be made by its manufacturer, is not guaranteed or endorsed by the publisher.
Supplementary material
The Supplementary material for this article can be found online at: https://www.frontiersin.org/articles/10.3389/fpsyg.2025.1480631/full#supplementary-material
References
Aaltonen, S., Latvala, A., Jelenkovic, A., Rose, R. J., Kujala, U. M., Kaprio, J., et al. (2020). Physical activity and academic performance: genetic and environmental associations. Med. Sci. Sports Exerc. 52, 381–390. doi: 10.1249/MSS.0000000000002124
Aaltonen, S., Ortega-Alonso, A., Kujala, U. M., and Kaprio, J. (2013). Genetic and environmental influences on longitudinal changes in leisure-time physical activity from adolescence to young adulthood. Twin Res. Hum. Genet. 16, 535–543. doi: 10.1017/thg.2013.9
Aarnio, M., Winter, T., Kujala, U. M., and Kaprio, J. (1997). Familial aggregation of leisure-time physical activity -- a three generation study. Int. J. Sports Med. 18, 549–556. doi: 10.1055/s-2007-972680
Anico, S., Wilson, L., Eyre, E., and Smith, E. (2022). The effectiveness of school-based run/walk programmes to develop physical literacy and physical activity components in primary school children: a systematic review. J. Sports Sci. 40, 2552–2569. doi: 10.1080/02640414.2023.2174720
Babic, M. J., Morgan, P. J., Plotnikoff, R. C., Lonsdale, C., White, R. L., and Lubans, D. R. (2014). Physical activity and physical self-concept in youth: systematic review and meta-analysis. Sports Med. 44, 1589–1601. doi: 10.1007/s40279-014-0229-z
Balshem, H., Helfand, M., Schunemann, H. J., Oxman, A. D., Kunz, R., Brozek, J., et al. (2011). GRADE guidelines: 3. Rating the quality of evidence. J. Clin. Epidemiol. 64, 401–406. doi: 10.1016/j.jclinepi.2010.07.015
Barnett, L. M., Lai, S. K., Veldman, S. L. C., Hardy, L. L., Cliff, D. P., Morgan, P. J., et al. (2016). Correlates of Gross motor competence in children and adolescents: a systematic review and Meta-analysis. Sports Med. 46, 1663–1688. doi: 10.1007/s40279-016-0495-z
Barnett, L. M., van Beurden, E., Morgan, P. J., Brooks, L. O., and Beard, J. R. (2010). Gender differences in motor skill proficiency from childhood to adolescence: a longitudinal study. Res. Q. Exerc. Sport 81, 162–170. doi: 10.1080/02701367.2010.10599663
Barnett, L. M., Webster, E. K., Hulteen, R. M., De Meester, A., Valentini, N. C., Lenoir, M., et al. (2022). Through the looking glass: a systematic review of longitudinal evidence, providing new insight for motor competence and health. Sports Med. 52, 875–920. doi: 10.1007/s40279-021-01516-8
Bauer, N., Sperlich, B., Holmberg, H. C., and Engel, F. A. (2022). Effects of high-intensity interval training in school on the physical performance and health of children and adolescents: a systematic review with Meta-analysis. Sports Med Open 8:50. doi: 10.1186/s40798-022-00437-8
Beets, M. W., Beighle, A., Erwin, H. E., and Huberty, J. L. (2009). After-school program impact on physical activity and fitness. A Meta-analysis. Am. J. Prev. Med. 36, 527–537. doi: 10.1016/j.amepre.2009.01.033
Behm, D. G., Young, J. D., Whitten, J. H. D., Reid, J. C., Quigley, P. J., Low, J., et al. (2017). Effectiveness of traditional strength vs. power training on muscle strength, power and Speed with youth: a systematic review and Meta-analysis. Front. Physiol. 8, 423. doi: 10.3389/fphys.2017.00423
Behringer, M., Vom Heede, A., Matthews, M., and Mester, J. (2011). Effects of strength training on motor performance skills in children and adolescents: a meta-analysis. Pediatr. Exerc. Sci. 23, 186–206. doi: 10.1123/pes.23.2.186
Behringer, M., vom Heede, A., Yue, Z. Y., and Mester, J. (2010). Effects of resistance training in children and adolescents: a Meta-analysis. Pediatrics 126, E1199–E1210. doi: 10.1542/peds.2010-0445
Beunen, G., and Thomis, M. (1999). Genetic determinants of sports participation and daily physical activity. Int. J. Obes. 23, S55–S63. doi: 10.1038/sj.ijo.0800885
Beunen, G., Thomis, M., Peeters, M., Maes, H. H., Claessens, A. L., and Vlietinck, R. (2003). Genetics of strength and power characteristics in children and adolescents. Pediatr. Exerc. Sci. 15, 128–138. doi: 10.1123/pes.15.2.128
Bingham, D. D., Costa, S., Hinkley, T., Shire, K. A., Clemes, S. A., and Barber, S. E. (2016). Physical activity during the early years: a systematic review of correlates and determinants. Am. J. Prev. Med. 51, 384–402. doi: 10.1016/j.amepre.2016.04.022
Bolger, L. E., Bolger, L. A., O'Neill, C., Coughlan, E., O'Brien, W., Lacey, S., et al. (2021). Global levels of fundamental motor skills in children: a systematic review. J. Sports Sci. 39, 717–753. doi: 10.1080/02640414.2020.1841405
Boomsma, D. I., van den Bree, M. B., Orlebeke, J. F., and Molenaar, P. C. (1989). Resemblances of parents and twins in sports participation and heart rate. Behav. Genet. 19, 123–141. doi: 10.1007/bf01065888
Braaksma, P., Stuive, I., Garst, R. M. E., Wesselink, C. F., van der Sluis, C. K., Dekker, R., et al. (2018). Characteristics of physical activity interventions and effects on cardiorespiratory fitness in children aged 6-12 years-a systematic review. J. Sci. Med. Sport 21, 296–306. doi: 10.1016/j.jsams.2017.07.015
Branta, C., Haubenstricker, J., and Seefeldt, V. (1984). Age changes in motor skills during childhood and adolescence. Exerc. Sport Sci. Rev. 12, 467–520. doi: 10.1249/00003677-198401000-00015
Breau, B., Brandes, M., Veidebaum, T., Tornaritis, M., Moreno, L. A., Molnar, D., et al. (2022). Longitudinal association of childhood physical activity and physical fitness with physical activity in adolescence: insights from the IDEFICS/I. Family study. Int. J. Behav. Nutr. Physical Act. 19:147. doi: 10.1186/s12966-022-01383-0
Breslin, G., Hillyard, M., Brick, N., Shannon, S., McKay-Redmond, B., and McConnell, B. (2023). A systematic review of the effect of the daily mile™ on children's physical activity, physical health, mental health, wellbeing, academic performance and cognitive function. PLoS One 18:e0277375. doi: 10.1371/journal.pone.0277375
Burns, R. D., Brusseau, T. A., and Fu, Y. (2018). Moderators of school-based physical activity interventions on cardiorespiratory endurance in primary school-aged children: a Meta-regression. Int. J. Environ. Res. Public Health 15, 1–17. doi: 10.3390/ijerph15081764
Burton, A. M., Cowburn, I., Thompson, F., Eisenmann, J. C., Nicholson, B., and Till, K. (2023). Associations between motor competence and physical activity, physical fitness and psychosocial characteristics in adolescents: a systematic review and Meta-analysis. Sports Med. 53, 2191–2256. doi: 10.1007/s40279-023-01886-1
Carson, V., Lee, E. Y., Hewitt, L., Jennings, C., Hunter, S., Kuzik, N., et al. (2017). Systematic review of the relationships between physical activity and health indicators in the early years (0-4 years). BMC Public Health 17:854. doi: 10.1186/s12889-017-4860-0
Cattuzzo, M. T., Dos Santos Henrique, R., Re, A. H., de Oliveira, I. S., Melo, B. M., de Sousa Moura, M., et al. (2016). Motor competence and health related physical fitness in youth: a systematic review. J. Sci. Med. Sport 19, 123–129. doi: 10.1016/j.jsams.2014.12.004
Chatterjee, S., and Das, N. (1995). Physical and motor fitness in twins. Jpn. J. Physiol. 45, 519–534. doi: 10.2170/jjphysiol.45.519
Chen, D., Zhao, G., Fu, J., Shun, S., Su, L., He, Z., et al. (2024). Effects of structured and unstructured interventions on fundamental motor skills in preschool children: a meta-analysis. Front. Public Health 12:1345566. doi: 10.3389/fpubh.2024.1345566
Choi, K. W., Zheutlin, A. B., Karlson, R. A., Wang, M. J., Dunn, E. C., Stein, M. B., et al. (2020). Physical activity offsets genetic risk for incident depression assessed via electronic health records in a biobank cohort study. Depress. Anxiety 37, 106–114. doi: 10.1002/da.22967
Cibinello, F. U., de Jesus, C., Neves, J., Janeiro Valenciano, P., Shizuko Fujisawa, D., and Augusto Marcal Camillo, C. (2023). Effects of Pilates in children and adolescents - a systematic review and meta-analysis. J. Bodyw. Mov. Ther. 35, 400–412. doi: 10.1016/j.jbmt.2023.04.028
Clemente, F. M., Moran, J., Ramirez-Campillo, R., Oliveira, R., Brito, J., Silva, A. F., et al. (2022). Recreational soccer training effects on pediatric populations physical fitness and health: a systematic review. Children (Basel) 9, 1–23. doi: 10.3390/children9111776
Collins, H., Booth, J. N., Duncan, A., and Fawkner, S. (2019a). The effect of resistance training interventions on fundamental movement skills in youth: a meta-analysis. Sports Med. Open 5:17. doi: 10.1186/s40798-019-0188-x
Collins, H., Booth, J. N., Duncan, A., Fawkner, S., and Niven, A. (2019b). The effect of resistance training interventions on 'The Self' in youth: a systematic review and Meta-analysis. Sports Med. Open 5:29. doi: 10.1186/s40798-019-0205-0
Comeras-Chueca, C., Marin-Puyalto, J., Matute-Llorente, A., Vicente-Rodriguez, G., Casajus, J. A., and Gonzalez-Aguero, A. (2021). The effects of active video games on health-related physical fitness and motor competence in children and adolescents with healthy weight: a systematic review and Meta-analysis. Int. J. Environ. Res. Public Health 18, 1–23. doi: 10.3390/ijerph18136965
Conger, S. A., Toth, L. P., Cretsinger, C., Raustorp, A., Mitas, J., Inoue, S., et al. (2022). Time trends in physical activity using wearable devices: a systematic review and Meta-analysis of studies from 1995 to 2017. Med. Sci. Sports Exerc. 54, 288–298. doi: 10.1249/MSS.0000000000002794
Costigan, S. A., Eather, N., Plotnikoff, R. C., Taaffe, D. R., and Lubans, D. R. (2015). High-intensity interval training for improving health-related fitness in adolescents: a systematic review and meta-analysis. Br. J. Sports Med. 49, 1253–1261. doi: 10.1136/bjsports-2014-094490
Cox, A., Fairclough, S. J., Kosteli, M. C., and Noonan, R. J. (2020). Efficacy of school-based interventions for improving muscular fitness outcomes in adolescent boys: a systematic review and Meta-analysis. Sports Med. 50, 543–560. doi: 10.1007/s40279-019-01215-5
Craggs, C., Corder, K., van Sluijs, E. M., and Griffin, S. J. (2011). Determinants of change in physical activity in children and adolescents: a systematic review. Am. J. Prev. Med. 40, 645–658. doi: 10.1016/j.amepre.2011.02.025
da Silva Bento, A. F. P., Carrasco Páez, L., and de Mendonça Raimundo, A. M. (2022). School-based high-intensity interval training programs for promoting physical activity and fitness in adolescents: a systematic review. J. Teach. Phys. Educ. 41, 288–300. doi: 10.1123/jtpe.2020-0187
Davey-Smith, G., and Hemani, G. (2014). Mendelian randomization: genetic anchors for causal inference in epidemiological studies. Hum. Mol. Genet. 23, R89–R98. doi: 10.1093/hmg/ddu328
de Andrade Gonçalves, E. C., Augusto Santos Silva, D., and Gimenes Nunes, H. E. (2015). Prevalence and factors associated with Low aerobic performance levels in adolescents: a systematic review. Curr. Pediatr. Rev. 11, 56–70. doi: 10.2174/1573396311666150501003435
de Geus, E. J. C. (2023). Genetic pathways underlying individual differences in regular physical activity. Exerc. Sport Sci. Rev. 51, 2–18. doi: 10.1249/JES.0000000000000305
de Geus, E. J. C., Boomsma, D. I., and Snieder, H. (2003). Genetic correlation of exercise with heart rate and respiratory sinus arrhythmia. Med. Sci. Sports Exerc. 35, 1287–1295. doi: 10.1249/01.MSS.0000079073.20399.11
De Meester, A., Barnett, L. M., Brian, A., Bowe, S. J., Jimenez-Diaz, J., Van Duyse, F., et al. (2020). The relationship between actual and perceived motor competence in children, adolescents and Young adults: a systematic review and Meta-analysis. Sports Med. 50, 2001–2049. doi: 10.1007/s40279-020-01336-2
de Moor, M. H. M., Willemsen, G., Rebollo-Mesa, I., Stubbe, J. H., de Geus, E. J. C., and Boomsma, D. I. (2011). Exercise participation in adolescents and their parents: evidence for genetic and generation specific environmental effects. Behav. Genet. 41, 211–222. doi: 10.1007/s10519-010-9415-4
Doherty, A., Smith-Byrne, K., Ferreira, T., Holmes, M. V., Holmes, C., Pulit, S. L., et al. (2018). GWAS identifies 14 loci for device-measured physical activity and sleep duration. Nat. Commun. 9:5257. doi: 10.1038/s41467-018-07743-4
Dudley, D., Okely, A., Pearson, P., and Cotton, W. (2011). A systematic review of the effectiveness of physical education and school sport interventions targeting physical activity, movement skills and enjoyment of physical activity. Eur. Phys. Educ. Rev. 17, 353–378. doi: 10.1177/1356336x11416734
Duncombe, S. L., Barker, A. R., Bond, B., Earle, R., Varley-Campbell, J., Vlachopoulos, D., et al. (2022). School-based high-intensity interval training programs in children and adolescents: a systematic review and meta-analysis. PLoS One 17:e0266427. doi: 10.1371/journal.pone.0266427
Eather, N., Babic, M., Riley, N., Costigan, S. A., and Lubans, D. R. (2022). Impact of embedding high-intensity interval training in schools and sports training on children and Adolescent's Cardiometabolic health and health-related fitness: systematic review and Meta-analysis. J. Teach. Phys. Educ. 42, 243–255. doi: 10.1123/jtpe.2021-0165
Elhakeem, A., Hardy, R., Bann, D., Kuh, D., and Cooper, R. (2018). Motor performance in early life and participation in leisure-time physical activity up to age 68 years. Paediatr. Perinat. Epidemiol. 32, 327–334. doi: 10.1111/ppe.12467
Engel, A. C., Broderick, C. R., van Doorn, N., Hardy, L. L., and Parmenter, B. J. (2018). Exploring the relationship between fundamental motor skill interventions and physical activity levels in children: a systematic review and Meta-analysis. Sports Med. 48, 1845–1857. doi: 10.1007/s40279-018-0923-3
Errisuriz, V. L., Golaszewski, N. M., Born, K., and Bartholomew, J. B. (2018). Systematic review of physical education-based physical activity interventions among elementary school children. J. Prim. Prev. 39, 303–327. doi: 10.1007/s10935-018-0507-x
Farooq, A., Martin, A., Janssen, X., Wilson, M. G., Gibson, A. M., Hughes, A., et al. (2020). Longitudinal changes in moderate-to-vigorous-intensity physical activity in children and adolescents: a systematic review and meta-analysis. Obes. Rev. 21:e12953. doi: 10.1111/obr.12953
Ferreira, F. A., Santos, C. C., Palmeira, A. L., Fernandes, R. J., and Costa, M. J. (2024). Effects of swimming exercise on early Adolescents' physical conditioning and physical health: a systematic review. J. Funct. Morphol. Kinesiol. 9, 1–18. doi: 10.3390/jfmk9030158
Figueroa, R., and An, R. (2017). Motor skill competence and physical activity in preschoolers: a review. Matern. Child Health J. 21, 136–146. doi: 10.1007/s10995-016-2102-1
Fisher, A., van Jaarsveld, C. H., Llewellyn, C. H., and Wardle, J. (2010). Environmental influences on children's physical activity: quantitative estimates using a twin design. PLoS One 5:e10110. doi: 10.1371/journal.pone.0010110
Franks, P. W., Ravussin, E., Hanson, R. L., Harper, I. T., Allison, D. B., Knowler, W. C., et al. (2005). Habitual physical activity in children: the role of genes and the environment. Am. J. Clin. Nutr. 82, 901–908. doi: 10.1093/ajcn/82.4.901
Gäbler, M., Prieske, O., Hortobágyi, T., and Granacher, U. (2018). The effects of concurrent strength and endurance training on physical fitness and athletic performance in youth: a systematic review and meta-analysis. Front. Physiol. 9:1057. doi: 10.3389/fphys.2018.01057
Garcia-Banos, C., Angel Rubio-Arias, J., Manuel Martinez-Aranda, L., and Jesus Ramos-Campo, D. (2020). Secondary-school-based interventions to improve muscular strength in adolescents: a systematic review. Sustain. For. 12, 1–14. doi: 10.3390/su12176814
Garcia-Hermoso, A., Alonso-Martinez, A. M., Ramirez-Velez, R., and Izquierdo, M. (2020). Effects of exercise intervention on health-related physical fitness and blood pressure in preschool children: a systematic review and Meta-analysis of randomized controlled trials. Sports Med. 50, 187–203. doi: 10.1007/s40279-019-01191-w
García-Hermoso, A., Alonso-Martínez, A. M., Ramírez-Vélez, R., Pérez-Sousa, M., Ramírez-Campillo, R., and Izquierdo, M. (2020). Association of Physical Education with Improvement of health-related physical fitness outcomes and fundamental motor skills among youths: a systematic review and Meta-analysis. JAMA Pediatr. 174:e200223. doi: 10.1001/jamapediatrics.2020.0223
Garcia-Hermoso, A., Ezzatvar, Y., Ramirez-Velez, R., Olloquequi, J., and Izquierdo, M. (2021). Is device-measured vigorous physical activity associated with health-related outcomes in children and adolescents? A systematic review and meta-analysis. J. Sport Health Sci. 10, 296–307. doi: 10.1016/j.jshs.2020.12.001
Goetghebuer, T., Ota, M. O., Kebbeh, B., John, M., Jackson-Sillah, D., Vekemans, J., et al. (2003). Delay in motor development of twins in Africa: a prospective cohort study. Twin Res. 6, 279–284. doi: 10.1375/136905203322296629
Grady, A., Lorch, R., Giles, L., Lamont, H., Anderson, A., Pearson, N., et al. (2025). The impact of early childhood education and care-based interventions on child physical activity, anthropometrics, fundamental movement skills, cognitive functioning, and social–emotional wellbeing: a systematic review and meta-analysis. Obes. Rev. 26:e13852. doi: 10.1111/obr.13852
Graham, M., Azevedo, L., Wright, M., and Innerd, A. L. (2022). The effectiveness of fundamental movement skill interventions on moderate to vigorous physical activity levels in 5-to 11-year-old children: a systematic review and Meta-analysis. Sports Med. 52, 1067–1090. doi: 10.1007/s40279-021-01599-3
Gralla, M. H., McDonald, S. M., Breneman, C., Beets, M. W., and Moore, J. B. (2019). Associations of objectively measured vigorous physical activity with body composition, cardiorespiratory fitness, and Cardiometabolic health in youth: a review. Am. J. Lifestyle Med. 13, 61–97. doi: 10.1177/1559827615624417
Gui, A., Ronald, A., Johnson, M., Hollowell, A., Dudbridge, F., and Arichi, T., the EAGLE consortium. (2024). Motor Milestones. Available online at: https://www.eagle-consortium.org/working-groups/behaviour-and-cognition/motor-milestones/ (accessed January 7, 2025).
Guthold, R., Stevens, G. A., Riley, L. M., and Bull, F. C. (2020). Global trends in insufficient physical activity among adolescents: a pooled analysis of 298 population-based surveys with 1.6 million participants. Lancet Child Adolesc Health 4, 23–35. doi: 10.1016/S2352-4642(19)30323-2
Gutierrez-Garcia, C., Astrain, I., Izquierdo, E., Teresa Gomez-Alonso, M., and Maria Yague, J. (2018). Effects of judo participation in children: a systematic review. J. Martial Arts Anthropol. 18, 63–73. doi: 10.14589/ido.18.4.8
Haberstick, B. C., Zeiger, J. S., and Corley, R. P. (2014). Genetic and environmental influences on the allocation of adolescent leisure time activities. Biomed. Res. Int. 2014:805476. doi: 10.1155/2014/805476
Hands, B., and Larkin, D. (2002). “Physical fitness and developmental coordination disorder” in Developmental coordination disorder. eds. S. Cermak and D. Larkin (Albany (NY): Delmar), 172–184.
Hanna, L., Burns, C., O'Neill, C., and Coughlan, E. (2023). A systematic review of the implementation and effectiveness of 'The daily Mile' on markers of Children's health. Int. J. Environ. Res. Public Health 20, 1–28. doi: 10.3390/ijerph20136203
Hassan, M. A., Liu, W., McDonough, D. J., Su, X., and Gao, Z. (2022). Comparative effectiveness of physical activity intervention programs on motor skills in children and adolescents: a systematic review and network Meta-analysis. Int. J. Environ. Res. Public Health 19, 1–12. doi: 10.3390/ijerph191911914
Henderson, S., and Sugden, D. (1992). The movement assessment battery for children. London: The Psychological Corporation.
Henriques-Neto, D., Peralta, M., Garradas, S., Pelegrini, A., Pinto, A. A., Sanchez-Miguel, P. A., et al. (2020). Active commuting and physical fitness: a systematic review. Int. J. Environ. Res. Public Health 17, 1–15. doi: 10.3390/ijerph17082721
Hesketh, K. R., O'Malley, C., Paes, V. M., Moore, H., Summerbell, C., Ong, K. K., et al. (2017). Determinants of change in physical activity in children 0-6 years of age: a systematic review of quantitative literature. Sports Med. 47, 1349–1374. doi: 10.1007/s40279-016-0656-0
Holfelder, B., and Schott, N. (2014). Relationship of fundamental movement skills and physical activity in children and adolescents: a systematic review. Psychol. Sport Exerc. 15, 382–391. doi: 10.1016/j.psychsport.2014.03.005
Hui, L., Wei, S., Luping, Q., and Nannan, G. (2024). Developing the optimal gross movement interventions to improve the physical fitness of 3-10 year-old children: a systematic review and meta-analysis. Front. Psychol. 15:1355821. doi: 10.3389/fpsyg.2024.1355821
Huppertz, C., Bartels, M., de Geus, E. J. C., van Beijsterveldt, C. E. M., Rose, R. J., Kaprio, J., et al. (2017). The effects of parental education on exercise behavior in childhood and youth: a study in Dutch and Finnish twins. Scand. J. Med. Sci. Sports 27, 1143–1156. doi: 10.1111/sms.12727
Huppertz, C., Bartels, M., de Zeeuw, E. L., van Beijsterveldt, C. E. M., Hudziak, J. J., Willemsen, G., et al. (2016). Individual differences in exercise behavior: stability and change in genetic and environmental determinants from age 7 to 18. Behav. Genet. 46, 665–679. doi: 10.1007/s10519-016-9799-x
Huppertz, C., Bartels, M., Van Beijsterveldt, C. E., Boomsma, D. I., Hudziak, J. J., and de Geus, E. J. (2012). Effect of shared environmental factors on exercise behavior from age 7 to 12 years. Med. Sci. Sports Exerc. 44, 2025–2032. doi: 10.1249/MSS.0b013e31825d358e
Isen, J., McGue, M., and Iacono, W. (2014). Genetic influences on the development of grip strength in adolescence. Am. J. Phys. Anthropol. 154, 189–200. doi: 10.1002/ajpa.22492
Janssen, I., and Leblanc, A. G. (2010). Systematic review of the health benefits of physical activity and fitness in school-aged children and youth. Int. J. Behav. Nutr. Physical Act. 7:40. doi: 10.1186/1479-5868-7-40
Jiang, T., Zhao, G., Fu, J., Sun, S., Chen, R., Chen, D., et al. (2024). Relationship between physical literacy and cardiorespiratory fitness in children and adolescents: A systematic review and Meta-analysis. Sports Med. 55, 473–485. doi: 10.1007/s40279-024-02129-7
Johnstone, A., Hughes, A. R., Martin, A., and Reilly, J. J. (2018). Utilising active play interventions to promote physical activity and improve fundamental movement skills in children: a systematic review and meta-analysis. BMC Public Health 18:789. doi: 10.1186/s12889-018-5687-z
Jones, D., Innerd, A., Giles, E. L., and Azevedo, L. B. (2020). Association between fundamental motor skills and physical activity in the early years: a systematic review and meta-analysis. J. Sport Health Sci. 9, 542–552. doi: 10.1016/j.jshs.2020.03.001
Jose, K. A., Blizzard, L., Dwyer, T., McKercher, C., and Venn, A. J. (2011). Childhood and adolescent predictors of leisure time physical activity during the transition from adolescence to adulthood: a population based cohort study. Int. J. Behav. Nutr. Physical Act. 8:54. doi: 10.1186/1479-5868-8-54
Kaartinen, S., Silventoinen, K., Korhonen, T., Kujala, U. M., Kaprio, J., and Aaltonen, S. (2021). Genetic and environmental effects on the individual variation and continuity of participation in diverse physical activities. Med. Sci. Sports Exerc. 53, 2495–2502. doi: 10.1249/mss.0000000000002744
Kaplan, G. A., Strawbridge, W. J., Cohen, R. D., and Hungerford, L. R. (1996). Natural history of leisure-time physical activity and its correlates: associations with mortality from all causes and cardiovascular disease over 28 years. Am. J. Epidemiol. 144, 793–797. doi: 10.1093/oxfordjournals.aje.a009003
Kennedy, S. G., Sanders, T., Estabrooks, P. A., Smith, J. J., Lonsdale, C., Foster, C., et al. (2021). Implementation at-scale of school-based physical activity interventions: a systematic review utilizing the RE-AIM framework. Obes. Rev. 22:e13184. doi: 10.1111/obr.13184
Klevjer, M., Nordeidet, A. N., Hansen, A. F., Madssen, E., Wisloff, U., Brumpton, B. M., et al. (2022). Genome-wide association study identifies new genetic determinants of cardiorespiratory fitness: the Trondelag health study. Med. Sci. Sports Exerc. 54, 1534–1545. doi: 10.1249/Mss.0000000000002951
Klimentidis, Y. C., Raichlen, D. A., Bea, J., Garcia, D. O., Wineinger, N. E., Mandarino, L. J., et al. (2018). Genome-wide association study of habitual physical activity in over 377, 000 UK biobank participants identifies multiple variants including CADM2 and APOE. Int. J. Obesity 42, 1161–1176. doi: 10.1038/s41366-018-0120-3
Knopik, V. S., Neiderhiser, J. M., DeFries, J. C., and Plomin, R. (2017). Behavioral genetics. New York: Worth publishers, Macmillan Learning.
Koopmans, J. R., van Doornen, L. J. P., and Boomsma, D. I. (1994). Smoking and sports participation. In U. Goldbourt and U. Faire de (Eds.), Genetic factors in coronary heart disease (pp. 217–235). Dordrecht: Kluwer Academic Publisher
Kujala, U. M., Palviainen, T., Pesonen, P., Waller, K., Sillanpaa, E., Niemela, M., et al. (2020). Polygenic risk scores and physical activity. Med. Sci. Sports Exerc. 52, 1518–1524. doi: 10.1249/MSS.0000000000002290
Lang, J. J., Belanger, K., Poitras, V., Janssen, I., Tomkinson, G. R., and Tremblay, M. S. (2018). Systematic review of the relationship between 20m shuttle run performance and health indicators among children and youth. J. Sci. Med. Sport 21, 383–397. doi: 10.1016/j.jsams.2017.08.002
Larouche, R., Saunders, T. J., Faulkner, G. E. J., Colley, R., and Tremblay, M. (2014). Associations between active school transport and physical activity, body composition, and cardiovascular fitness: a systematic review of 68 studies. J. Phys. Act. Health 11, 206–227. doi: 10.1123/jpah.2011-0345
Lei, Y., and Jun, H.-P. (2022). Does taekwondo Poomsae training impact on body composition, physical fitness, and blood composition in children and adolescents? A systematic review. Exercise Sci. 31, 11–25. doi: 10.15857/ksep.2022.00402
Leskinen, T., Waller, K., Mutikainen, S., Aaltonen, S., Ronkainen, P. H., Alen, M., et al. (2009). Effects of 32-year leisure time physical activity discordance in twin pairs on health (TWINACTIVE study): aims, design and results for physical fitness. Twin Res. Hum. Genet. 12, 108–117. doi: 10.1375/twin.12.1.108
Li, B., Liu, J., and Ying, B. (2022). Physical education interventions improve the fundamental movement skills in kindergarten: a systematic review and meta-analysis. Food Sci. Technol. 42, 1–13. doi: 10.1590/fst.46721
Li, Z., Qi, Y., Chen, X., Li, J., Zhang, J., Li, P., et al. (2024). Synergistic effects of concurrent aerobic and strength training on fitness in children and adolescents: a multivariate and network Meta-analysis. Scand. J. Med. Sci. Sports 34:e14764. doi: 10.1111/sms.14764
Lin, J., Zhang, R., Shen, J., and Zhou, A. (2022). Effects of school-based neuromuscular training on fundamental movement skills and physical fitness in children: a systematic review. Peer J. 10:e13726. doi: 10.7717/peerj.13726
Liu, C., Cao, Y., Zhang, Z., Gao, R., and Qu, G. (2023). Correlation of fundamental movement skills with health-related fitness elements in children and adolescents: a systematic review. Front. Public Health 11:1129258. doi: 10.3389/fpubh.2023.1129258
Liu, Y., Li, Z., Yuan, L., and Zhou, Z. (2023). The bidirectional correlation between fundamental motor skill and moderate-to-vigorous physical activities: a systematic review and Meta-analysis. Children (Basel) 10, 1–15. doi: 10.3390/children10091504
Liu, Y., Wadey, C. A., Barker, A. R., and Williams, C. A. (2024). Process evaluation of school-based high-intensity interval training interventions for children and adolescents: a systematic review and meta-analysis of randomized controlled trials. BMC Public Health 24:348. doi: 10.1186/s12889-024-17786-6
Liu, W. X., Zeng, N., McDonough, D. J., and Gao, Z. (2020). Effect of active video games on healthy Children's fundamental motor skills and physical fitness: a systematic review. Int. J. Environ. Res. Public Health 17, 1–17. doi: 10.3390/ijerph17218264
Logan, S. W., Kipling Webster, E., Getchell, N., Pfeizer, K. A., and Robinson, L. E. (2015). Relationship between fundamental motor skill competence and physical activity during childhood and adolescence: a systematic review. Kinesiol. Rev. 4, 416–426. doi: 10.1123/kr.2013-0012
Lorås, H. (2020). The effects of physical education on motor competence in children and adolescents: a systematic review and Meta-analysis. Sports (Basel) 8, 1–14. doi: 10.3390/sports8060088
Lubans, D. R., Boreham, C. A., Kelly, P., and Foster, C. E. (2011). The relationship between active travel to school and health-related fitness in children and adolescents: a systematic review. Int. J. Behav. Nutr. Physical Act. 8:5. doi: 10.1186/1479-5868-8-5
Lubans, D. R., Morgan, P. J., Cliff, D. P., Barnett, L. M., and Okely, A. D. (2010). Fundamental movement skills in children and adolescents review of associated health benefits. Sports Med. 40, 1019–1035. doi: 10.2165/11536850-000000000-00000
Ma, J., Lander, N., Eyre, E. L. J., Barnett, L. M., Essiet, I. A., and Duncan, M. J. (2021). It's not just what you Do but the way you Do it: a systematic review of process evaluation of interventions to improve Gross motor competence. Sports Med. 51, 2547–2569. doi: 10.1007/s40279-021-01519-5
Maes, H. H., Beunen, G. P., Vlietinck, R. F., Neale, M. C., Thomis, M., Vanden Eynde, B., et al. (1996). Inheritance of physical fitness in 10-year-old twins and their parents. Med. Sci. Sports Exerc. 28, 1479–1491. doi: 10.1097/00005768-199612000-00007
Maia, J. A., Santos, D., de Freitas, D. L., and Thomis, M. (2013). Physical activity, physical fitness, gross motor coordination, and metabolic syndrome: focus of twin research in Portugal. Twin Res. Hum. Genet. 16, 296–301. doi: 10.1017/thg.2012.128
Maia, J. A., Thomis, M., and Beunen, G. (2002). Genetic factors in physical activity levels: a twin study. Am. J. Prev. Med. 23, 87–91. doi: 10.1016/s0749-3797(02)00478-6
Malina, R. M. (1990). “Tracking of physical fitness and performance during growth” in Children and exercise. eds. G. Buenen, J. G. Hesquiere, and A. L. Claessens (Stuttgart, Germany: Ferdinand Enke), 1–10.
McDonough, D. J., Liu, W., and Gao, Z. (2020). Effects of physical activity on Children's motor skill development: a systematic review of randomized controlled trials. Biomed. Res. Int. 2020:8160756. doi: 10.1155/2020/8160756
McKenzie, T. L., Sallis, J. F., Broyles, S. L., Zive, M. M., Nader, P. R., Berry, C. C., et al. (2002). Childhood movement skills: predictors of physical activity in Anglo American and Mexican American adolescents? Res. Q. Exerc. Sport 73, 238–244. doi: 10.1080/02701367.2002.10609017
Minatto, G., Barbosa Filho, V. C., Berria, J., and Petroski, E. L. (2016). School-based interventions to improve cardiorespiratory fitness in adolescents: systematic review with Meta-analysis. Sports Med. 46, 1273–1292. doi: 10.1007/s40279-016-0480-6
Minica, C. C., Boomsma, D. I., Dolan, C. V., de Geus, E., and Neale, M. C. (2020). Empirical comparisons of multiple Mendelian randomization approaches in the presence of assortative mating. Int. J. Epidemiol. 49, 1185–1193. doi: 10.1093/ije/dyaa013
Minica, C. C., Dolan, C. V., Boomsma, D. I., de Geus, E., and Neale, M. C. (2018). Extending causality tests with genetic instruments: An integration of Mendelian randomization with the classical twin design. Behav. Genet. 48, 337–349. doi: 10.1007/s10519-018-9904-4
Miyamoto-Mikami, E., Zempo, H., Fuku, N., Kikuchi, N., Miyachi, M., and Murakami, H. (2018). Heritability estimates of endurance-related phenotypes: a systematic review and meta-analysis. Scand. J. Med. Sci. Sports 28, 834–845. doi: 10.1111/sms.12958
Moon, J., Webster, C. A., Stodden, D. F., Brian, A., Mulvey, K. L., Beets, M., et al. (2024). Systematic review and meta-analysis of physical activity interventions to increase elementary children's motor competence: a comprehensive school physical activity program perspective. BMC Public Health 24:826. doi: 10.1186/s12889-024-18145-1
Moran, J., Sandercock, G., Ramirez-Campillo, R., Clark, C. C. T., Fernandes, J. F. T., and Drury, B. (2018). A Meta-analysis of resistance training in female youth: its effect on muscular strength, and shortcomings in the literature. Sports Med. 48, 1661–1671. doi: 10.1007/s40279-018-0914-4
Mountford, H. S., Hill, A., Barnett, A. L., and Newbury, D. F. (2021). Genome-wide association study of motor coordination. Front. Hum. Neurosci. 15:669902. doi: 10.3389/fnhum.2021.669902
Neil-Sztramko, S. E., Caldwell, H., and Dobbins, M. (2021). School-based physical activity programs for promoting physical activity and fitness in children and adolescents aged 6 to 18. Cochrane Database Syst. Rev. 2021:CD007651. doi: 10.1002/14651858.CD007651.pub3
Norris, E., Hamer, M., and Stamatakis, E. (2016). Active video games in schools and effects on physical activity and health: a systematic review. J. Pediatr. 172:40-46e45. doi: 10.1016/j.jpeds.2016.02.001
Øglund, G. P., Hildebrand, M., and Ekelund, U. (2015). Are birth weight, early growth, and motor development determinants of physical activity in children and youth? A systematic review and Meta-analysis. Pediatr. Exerc. Sci. 27, 441–453. doi: 10.1123/pes.2015-0041
Øglund, G. P., Stensrud, T., and Ekelund, U. (2014). Early life determinants of physical activity and sedentary time: current knowledge and future research. Norsk Epidemiol. 24, 1–2. doi: 10.5324/nje.v24i1-2.1820
Okuda, E., Horii, D., and Kano, T. (2005). Genetic and environmental effects on physical fitness and motor performance. Int. J. Sport Health Sci. 3, 1–9. doi: 10.5432/ijshs.3.1
Oppici, L., Stell, F. M., Utesch, T., Woods, C. T., Foweather, L., and Rudd, J. R. (2022). A skill acquisition perspective on the impact of exergaming technology on foundational movement skill development in children 3-12 years: a systematic review and Meta-analysis. Sports Med. Open 8, 1–15. doi: 10.1186/s40798-022-00534-8
Page, M. J., Moher, D., Bossuyt, P. M., Boutron, I., Hoffmann, T. C., Mulrow, C. D., et al. (2021). PRISMA 2020 explanation and elaboration: updated guidance and exemplars for reporting systematic reviews. BMJ 372:n160. doi: 10.1136/bmj.n160
Peeters, M. W., Thomis, M. A., Maes, H. H. M., Loos, R. J. F., Claessens, A. L., Vlietinck, R., et al. (2005). Genetic and environmental causes of tracking in explosive strength during adolescence. Behav. Genet. 35, 551–563. doi: 10.1007/s10519-005-5417-z
Peralta, M., Henriques-Neto, D., Gouveia, É. R., Sardinha, L. B., and Marques, A. (2020). Promoting health-related cardiorespiratory fitness in physical education: a systematic review. PLoS One 15:e0237019. doi: 10.1371/journal.pone.0237019
Pereira, S., Reyes, A. C., Chaves, R., Santos, C., Vasconcelos, O., Tani, G. O., et al. (2022). Correlates of the physical activity decline during childhood. Med. Sci. Sports Exerc. 54, 2129–2137. doi: 10.1249/MSS.0000000000003013
Pérusse, L., Tremblay, A., Leblanc, C., and Bouchard, C. (1989). Genetic and environmental influences on level of habitual physical activity and exercise participation. Am. J. Epidemiol. 129, 1012–1022. doi: 10.1093/oxfordjournals.aje.a115205
Pesce, C. (2012). Shifting the focus from quantitative to qualitative exercise characteristics in exercise and cognition research. J. Sport Exerc. Psychol. 34, 766–786. doi: 10.1123/jsep.34.6.766
Peter, I., Vainder, M., and Livshits, G. (1999). Genetic analysis of motor milestones attainment in early childhood. Twin Res. 2, 1–9. doi: 10.1375/136905299320566040
Peterson, R. A., and Brown, S. P. (2005). On the use of beta coefficients in meta-analysis. J. Appl. Psychol. 90, 175–181. doi: 10.1037/0021-9010.90.1.175
Pingault, J. B., O'Reilly, P. F., Schoeler, T., Ploubidis, G. B., Rijsdijk, F., and Dudbridge, F. (2018). Using genetic data to strengthen causal inference in observational research. Nat. Rev. Genet. 19, 566–580. doi: 10.1038/s41576-018-0020-3
Pinho, C. D. F., Bagatini, N. C., Lisboa, S. D. C., Mello, J. B., and Cunha, G. D. S. (2024). Effects of different supervised and structured physical exercise on the physical fitness trainability of children and adolescents: a meta-analysis and meta-regression: physical fitness trainability in children and adolescents' health. BMC Pediatr. 24, 1–18. doi: 10.1186/s12887-024-04929-2
Poitras, V. J., Gray, C. E., Borghese, M. M., Carson, V., Chaput, J. P., Janssen, I., et al. (2016). Systematic review of the relationships between objectively measured physical activity and health indicators in school-aged children and youth. Appl. Physiol. Nutr. Metab. 41, S197–S239. doi: 10.1139/apnm-2015-0663
Polderman, T. J., Benyamin, B., de Leeuw, C. A., Sullivan, P. F., van Bochoven, A., Visscher, P. M., et al. (2015). Meta-analysis of the heritability of human traits based on fifty years of twin studies. Nat. Genet. 47, 702–709. doi: 10.1038/ng.3285
Pozuelo-Carrascosa, D. P., García-Hermoso, A., Álvarez-Bueno, C., Sánchez-López, M., and Martinez-Vizcaino, V. (2018). Effectiveness of school-based physical activity programmes on cardiorespiratory fitness in children: a meta-analysis of randomised controlled trials. Br. J. Sports Med. 52, 1234–1240. doi: 10.1136/bjsports-2017-097600
Prochaska, J. O., Wright, J. A., and Velicer, W. F. (2008). Evaluating theories of health behavior change: a hierarchy of criteria applied to the transtheoretical model. Appl. Psychol. 57, 561–588. doi: 10.1111/j.1464-0597.2008.00345.x
Ramirez-Campillo, R., Sortwell, A., Moran, J., Afonso, J., Clemente, F. M., Lloyd, R. S., et al. (2023). Plyometric-jump training effects on physical fitness and sport-specific performance according to maturity: a systematic review with Meta-analysis. Sports Med. Open 9:23. doi: 10.1186/s40798-023-00568-6
Rebar, A. L., Lagoa, C. M., Gardner, B., and Conroy, D. E. (2024). The specification of a computational model of physical activity habit. Exerc. Sport Sci. Rev. 52, 102–107. doi: 10.1249/JES.0000000000000340
Reyes-Amigo, T., Gomez, M., Gallardo, M., and Palmeira, A. (2017). Effectiveness of high-intenisty interval training on cardiorespiratory fitness and body composition in preadolescents: a systematic review. Eur. J. Hum. Mov. 39, 32–47.
Rico-González, M. (2023). The effect of primary school-based physical education programs: a systematic review of randomized controlled trials. J. Phys. Act. Health 20, 317–347. doi: 10.1123/jpah.2022-0452
Robinson, L. E., Stodden, D. F., Barnett, L. M., Lopes, V. P., Logan, S. W., Rodrigues, L. P., et al. (2015). Motor competence and its effect on positive developmental trajectories of health. Sports Med. 45, 1273–1284. doi: 10.1007/s40279-015-0351-6
Sallis, J. F., Prochaska, J. J., and Taylor, W. C. (2000). A review of correlates of physical activity of children and adolescents. Med. Sci. Sports Exerc. 32, 963–975. doi: 10.1097/00005768-200005000-00014
Santos, C., Burnay, C., Button, C., and Cordovil, R. (2023). Effects of exposure to formal aquatic activities on babies younger than 36 months: a systematic review. Int. J. Environ. Res. Public Health 20, 1–18. doi: 10.3390/ijerph20085610
Saudino, K. J., and Zapfe, J. A. (2008). Genetic influences on activity level in early childhood: Do situations matter? Child Dev. 79, 930–943. doi: 10.1111/j.1467-8624.2008.01168.x
Schmutz, E. A., Leeger-Aschmann, C. S., Kakebeeke, T. H., Zysset, A. E., Messerli-Burgy, N., Stulb, K., et al. (2020). Motor competence and physical activity in early childhood: stability and relationship. Front. Public Health 8:39. doi: 10.3389/fpubh.2020.00039
Schutte, N. M., Nederend, I., Bartels, M., and de Geus, E. J. C. (2019). A twin study on the correlates of voluntary exercise behavior in adolescence. Psychol. Sport Exerc. 40, 99–109. doi: 10.1016/j.psychsport.2018.10.002
Schutte, N. M., Nederend, I., Hudziak, J. J., Bartels, M., and de Geus, E. J. (2016a). Twin-sibling study and meta-analysis on the heritability of maximal oxygen consumption. Physiol. Genomics 48, 210–219. doi: 10.1152/physiolgenomics.00117.2015
Schutte, N. M., Nederend, I., Hudziak, J. J., de Geus, E. J., and Bartels, M. (2016b). Differences in adolescent physical fitness: a multivariate approach and Meta-analysis. Behav. Genet. 46, 217–227. doi: 10.1007/s10519-015-9754-2
Silventoinen, K., Magnusson, P. K. E., Tynelius, P., Kaprio, J., and Rasmussen, F. (2008). Heritability of body size and muscle strength in young adulthood: a study of one million Swedish men. Genet. Epidemiol. 32, 341–349. doi: 10.1002/gepi.20308
Silventoinen, K., Maia, J., Jelenkovic, A., Pereira, S., Gouveia, É., Antunes, A., et al. (2021). Genetics of somatotype and physical fitness in children and adolescents. Am. J. Hum. Biol. 33:e23470. doi: 10.1002/ajhb.23470
Simonen, R., Levalahti, E., Kaprio, J., Videman, T., and Battie, M. C. (2004). Multivariate genetic analysis of lifetime exercise and environmental factors. Med. Sci. Sports Exerc. 36, 1559–1566.
Sinclair, L., and Roscoe, C. M. P. (2023). The impact of swimming on fundamental movement skill development in children (3-11 years): a systematic literature review. Children (Basel) 10, 1–20. doi: 10.3390/children10081411
Singh, U., Ramachandran, A. K., Ramirez-Campillo, R., Perez-Castilla, A., Afonso, J., Manuel Clemente, F., et al. (2022). Jump rope training effects on health-and sport-related physical fitness in young participants: a systematic review with meta-analysis. J. Sports Sci. 40, 1801–1814. doi: 10.1080/02640414.2022.2099161
Smith, J. J., Eather, N., Weaver, R. G., Riley, N., Beets, M. W., and Lubans, D. R. (2019). Behavioral correlates of muscular fitness in children and adolescents: a systematic review. Sports Med. 49, 887–904. doi: 10.1007/s40279-019-01089-7
Smith, L., van Jaarsveld, C. H. M., Llewellyn, C. H., Fildes, A., Lopez Sanchez, G. F., Wardle, J., et al. (2017). Genetic and environmental influences on developmental milestones and movement: results from the Gemini Cohort study. Res. Q. Exerc. Sport 88, 401–407. doi: 10.1080/02701367.2017.1373268
Solovieff, N., Cotsapas, C., Lee, P. H., Purcell, S. M., and Smoller, J. W. (2013). Pleiotropy in complex traits: challenges and strategies. Nat. Rev. Genet. 14, 483–495. doi: 10.1038/nrg3461
Speed, D., Hemani, G., Speed, M. S., Borglum, A. D., Ostergaard, S. D., and Working, M. D. D. (2019). Investigating the causal relationship between neuroticism and depression via Mendelian randomization. Acta Psychiatr. Scand. 139, 395–397. doi: 10.1111/acps.13009
Stodden, D. F., Goodway, J. D., Langendorfer, S. J., Roberton, M. A., Rudisill, M. E., Garcia, C., et al. (2008). A developmental perspective on the role of motor skill competence in physical activity: An emergent relationship. Quest 60, 290–306. doi: 10.1080/00336297.2008.10483582
Stojanović, S., Andrieieva, O., and Trajković, N. (2024). Associations between number of steps and health outcomes in children and adolescents: a systematic review and meta-analysis. BMC Public Health 24:3310. doi: 10.1186/s12889-024-20835-9
Stubbe, J. H., Boomsma, D. I., and de Geus, E. J. C. (2005). Sports participation during adolescence: a shift from environmental to genetic factors. Med. Sci. Sports Exerc. 37, 563–570. doi: 10.1249/01.MSS.0000158181.75442.8B
Sun, S., and Chen, C. (2024). The effect of sports game intervention on Children's fundamental motor skills: a systematic review and Meta-analysis. Children-Basel 11, 1–12. doi: 10.3390/children11020254
Sun, C., Pezic, A., Tikellis, G., Ponsonby, A. L., Wake, M., Carlin, J. B., et al. (2013). Effects of school-based interventions for direct delivery of physical activity on fitness and cardiometabolic markers in children and adolescents: a systematic review of randomized controlled trials. Obes. Rev. 14, 818–838. doi: 10.1111/obr.12047
Sundet, J. M., Magnus, P., and Tambs, K. (1994). The heritability of maximal aerobic power: a study of Norwegian twins. Scand. J. Med. Sci. Sports 4, 181–185. doi: 10.1111/j.1600-0838.1994.tb00423.x
Szeszulski, J., Lorenzo, E., Shaibi, G. Q., Buman, M. P., Vega-López, S., Hooker, S. P., et al. (2019). Effectiveness of early care and education center-based interventions for improving cardiovascular fitness in early childhood: a systematic review and meta-analysis. Prev. Med. Rep. 15:100915. doi: 10.1016/j.pmedr.2019.100915
Telama, R., Yang, X., Viikari, J., Valimaki, I., Wanne, O., and Raitakari, O. (2005). Physical activity from childhood to adulthood: a 21-year tracking study. Am. J. Prev. Med. 28, 267–273. doi: 10.1016/j.amepre.2004.12.003
Timmons, B. W., LeBlanc, A. G., Carson, V., Gorber, S. C., Dillman, C., Janssen, I., et al. (2012). Systematic review of physical activity and health in the early years (aged 0-4 years). Appl. Physiol. Nutr. Metab. 37, 773–792. doi: 10.1139/H2012-070
Utesch, T., Bardid, F., Busch, D., and Strauss, B. (2019). The relationship between motor competence and physical fitness from early childhood to early adulthood: a Meta-analysis. Sports Med. 49, 541–551. doi: 10.1007/s40279-019-01068-y
Van Capelle, A., Broderick, C. R., van Doorn, N., Ward, R. E., and Parmenter, B. J. (2017). Interventions to improve fundamental motor skills in pre-school aged children: a systematic review and meta-analysis. J. Sci. Med. Sport 20, 658–666. doi: 10.1016/j.jsams.2016.11.008
van der Aa, N., de Geus, E. J., van Beijsterveldt, T. C., Boomsma, D. I., and Bartels, M. (2010). Genetic influences on individual differences in exercise behavior during adolescence. Int. J. Pediatr. 2010:138345, 1–8. doi: 10.1155/2010/138345
van der Zee, M. D., and de Geus, E. (2019). “Is physical activity regulated by genetics? Evidence from studies in humans” in The Routledge handbook of sport and exercise system genetics. eds. J. T. Lightfoot, M. Hubal, and S. R. Roth (Oxford, UK: Taylor & Francis Routledge), 67–79.
van der Zee, M. D., Helmer, Q., Boomsma, D. I., Dolan, C. V., and de Geus, E. J. C. (2020). An extended twin-pedigree study of different classes of voluntary exercise behavior. Behav. Genet. 50, 94–104. doi: 10.1007/s10519-019-09990-7
van der Zee, M. D., van der Mee, D., Bartels, M., and de Geus, E. J. C. (2019). Tracking of voluntary exercise behaviour over the lifespan. Int. J. Behav. Nutr. Physical Act. 16:17. doi: 10.1186/s12966-019-0779-4
Vandenberg, S. G. (1962). The hereditary abilities study: hereditary components in a psychological test battery. Am. J. Hum. Genet. 14, 220–237
Veldman, S. L. C., Chin, A. P. M. J. M., and Altenburg, T. M. (2021). Physical activity and prospective associations with indicators of health and development in children aged <5 years: a systematic review. Int. J. Behav. Nutr. Physical Act. 18:6. doi: 10.1186/s12966-020-01072-w
Verbanck, M., Chen, C. Y., Neale, B., and Do, R. (2018). Publisher correction: detection of widespread horizontal pleiotropy in causal relationships inferred from Mendelian randomization between complex traits and diseases. Nat. Genet. 50:1196. doi: 10.1038/s41588-018-0164-2
Villa-González, E., Barranco-Ruiz, Y., García-Hermoso, A., and Faigenbaum, A. D. (2023). Efficacy of school-based interventions for improving muscular fitness outcomes in children: a systematic review and meta-analysis. Eur. J. Sport Sci. 23, 444–459. doi: 10.1080/17461391.2022.2029578
von Elm, E., Altman, D. G., Egger, M., Pocock, S. J., Gotzsche, P. C., Vandenbroucke, J. P., et al. (2007). The strengthening the reporting of observational studies in epidemiology (STROBE) statement: guidelines for reporting observational studies. Lancet 370, 1453–1457. doi: 10.1016/S0140-6736(07)61602-X
Wang, Z., Emmerich, A., Pillon, N. J., Moore, T., Hemerich, D., Cornelis, M. C., et al. (2022). Genome-wide association analyses of physical activity and sedentary behavior provide insights into underlying mechanisms and roles in disease prevention. Nat. Genet. 54:1332. doi: 10.1038/s41588-022-01165-1
Wang, L., and Zhou, Y. (2023). A systematic review of correlates of the moderate-to-vigorous physical activity of students in elementary school physical education. J. Teach. Phys. Educ. 42, 1–16. doi: 10.1123/jtpe.2020-0197
Wang, X., and Zhou, B. (2024). Motor development-focused exercise training enhances gross motor skills more effectively than ordinary physical activity in healthy preschool children: an updated meta-analysis. Front. Public Health 12:1414152. doi: 10.3389/fpubh.2024.1414152
Wendel-Vos, G. C., Schuit, A. J., Tijhuis, M. A., and Kromhout, D. (2004). Leisure time physical activity and health-related quality of life: cross-sectional and longitudinal associations. Qual. Life Res. 13, 667–677. doi: 10.1023/B:QURE.0000021313.51397.33
White, E., Slane, J. D., Klump, K. L., Burt, S. A., and Pivarnik, J. (2014). Sex differences in genetic and environmental influences on percent body fatness and physical activity. J. Phys. Act. Health 11, 1187–1193. doi: 10.1123/jpah.2012-0221
Willems, S. M., Wright, D. J., Day, F. R., Trajanoska, K., Joshi, P. K., Morris, J. A., et al. (2017). Large-scale GWAS identifies multiple loci for hand grip strength providing biological insights into muscular fitness. Nat. Commun. 8, 1–12. doi: 10.1038/ncomms16015
Williams, L. R., and Gross, J. B. (1980). Heritability of motor skill. Acta Genet. Med. Gemellol. 29, 127–136. doi: 10.1017/s0001566000008606
Wood, A. C., Rijsdijk, F., Saudino, K. J., Asherson, P., and Kuntsi, J. (2008). High heritability for a composite index of children's activity level measures. Behav. Genet. 38, 266–276. doi: 10.1007/s10519-008-9196-1
Woodforde, J., Alsop, T., Salmon, J., Gomersall, S., and Stylianou, M. (2022). Effects of school-based before-school physical activity programmes on children's physical activity levels, health and learning-related outcomes: a systematic review. Br. J. Sports Med. 56, 740–754. doi: 10.1136/bjsports-2021-104470
World Health Organization. (2020). WHO guidelines on physical activity and sedentary behaviour. Geneva: World Health Organization. Licence: CC BY-NC-SA 3.0 IGO.
Wu, C., Xu, Y., Chen, Z., Cao, Y., Yu, K., and Huang, C. (2021). The effect of intensity, frequency, duration and volume of physical activity in children and adolescents on skeletal muscle fitness: a systematic review and Meta-analysis of randomized controlled trials. Int. J. Environ. Res. Public Health 18, 1–18. doi: 10.3390/ijerph18189640
Wu, J., Yang, Y., Yu, H., Li, L., Chen, Y., and Sun, Y. (2023). Comparative effectiveness of school-based exercise interventions on physical fitness in children and adolescents: a systematic review and network meta-analysis. Front. Public Health 11:1194779. doi: 10.3389/fpubh.2023.1194779
Xin, F., Chen, S. T., Clark, C., Hong, J. T., Liu, Y., and Cai, Y. J. (2020). Relationship between fundamental movement skills and physical activity in preschool-aged children: a systematic review. Int. J. Environ. Res. Public Health 17, 1–17. doi: 10.3390/ijerph17103566
Xu, Z., Shen, S.-J., and Wen, Y.-H. (2024). The relationship between fundamental movement skills and physical activity in preschoolers: a systematic review. Early Child Dev. Care 194, 323–334. doi: 10.1080/03004430.2024.2309478
Zamorano-Garcia, D., Infantes-Paniagua, A., Cuevas-Campos, R., and Fernandez-Bustos, J. G. (2023). Impact of physical activity-based interventions on children and Adolescents' physical self-concept: a Meta-analysis. Res. Q. Exerc. Sport 94, 1–14. doi: 10.1080/02701367.2021.1927945
Zeng, N., Ayyub, M., Sun, H., Wen, X., Xiang, P., and Gao, Z. (2017). Effects of physical activity on motor skills and cognitive development in early childhood: a systematic review. Biomed. Res. Int. 2017, 2760716–2760713. doi: 10.1155/2017/2760716
Zhang, D., Soh, K. G., Chan, Y. M., Feng, X., Bashir, M., and Xiao, W. (2024). Effect of functional training on fundamental motor skills among children: a systematic review. Heliyon 10:e39531. doi: 10.1016/j.heliyon.2024.e39531
Zhao, Q., Wang, Y., Niu, Y., and Liu, S. (2023). Jumping rope improves the physical fitness of preadolescents aged 10-12 years: a meta-analysis. J. Sports Sci. Med. 22, 367–380. doi: 10.52082/jssm.2023.367
Zhou, X., Li, J., and Jiang, X. (2024). Effects of different types of exercise intensity on improving health-related physical fitness in children and adolescents: a systematic review. Sci. Rep. 14:14301. doi: 10.1038/s41598-024-64830-x
Zi, Y., Bartels, M., and de Geus, E. (2023a). Genetics of physical activity and physical fitness. In N. Armstrong and W. Mechelen van (Eds.), Oxford textbook of Children’s sport and exercise medicine (4th ed., pp. 239–254). Oxford, UK: Oxford University Press.
Zi, Y., Bartels, M., Dolan, C., and de Geus, E. J. C. (2024). Genetic confounding in the association of early motor development with childhood and adolescent exercise behavior. Int. J. Behav. Nutr. Physical Act. 21:33. doi: 10.1186/s12966-024-01583-w
Keywords: motor development, Stodden model, twin studies, perceived motor competence, cardiorespiratory fitness, muscular fitness
Citation: Zi Y and de Geus EJC (2025) Reviewing the association between motor competence and physical activity from a behavioral genetic perspective. Front. Psychol. 16:1480631. doi: 10.3389/fpsyg.2025.1480631
Edited by:
Matthew A. Stults-Kolehmainen, Yale New Haven Hospital, United StatesCopyright © 2025 Zi and de Geus. This is an open-access article distributed under the terms of the Creative Commons Attribution License (CC BY). The use, distribution or reproduction in other forums is permitted, provided the original author(s) and the copyright owner(s) are credited and that the original publication in this journal is cited, in accordance with accepted academic practice. No use, distribution or reproduction is permitted which does not comply with these terms.
*Correspondence: Eco J. C. de Geus, ai5jLm4uZGUuZ2V1c0B2dS5ubA==