- 1Chemical and Materials Engineering Department, University of Auckland, Auckland, New Zealand
- 2LEAF—Linking Landscape, Environment, Agriculture and Food, Associated Laboratory TERRA, Instituto Superior de Agronomia, Universidade de Lisboa, Lisboa, Portugal
The pasteurization of beer occurs at the end of the industrial production, after fermentation. Generally, a mild thermal process (60°C) is employed aiming to inactivate the fermenting yeast and potential spoilage microorganisms, thus extending the beer shelf-life at room temperature. The heat treatment negatively affects the original beer freshness and flavor. In this study, beer pasteurization using emerging non-thermal technologies, namely, high-pressure processing (HPP), pulsed electric fields (PEF), and ultrasound (US), was reviewed, including the effect on microbial inactivation and beer quality. The combination of non-thermal methods with mild heat for more efficient pasteurization of beer was also reviewed. All technologies caused microbial inactivation in beer. However, room temperature HPP treatment was the most efficient method, delivering the minimum 15 PU (pasteurization units) to beer after seconds (e.g., 300 MPa for 27 s), as opposed to thermal and TS treatments which required several minutes, while causing a negative impact on beer sensory. As expected, PEF + heat caused a higher microbial inactivation than PEF alone, and yeast ascospores were more resistant than vegetative yeast cells. Non-thermal PEF (35–45 kV/cm) caused 3–5.8 log reductions in vegetative bacteria. Studies on thermal assisted PEF and ultrasound combined with low heat (50–55°C) showed processing times in the magnitude of microseconds for PEF pasteurization and 0.5–2 min (depending on the temperature) for TS pasteurization. With respect to impact of these technologies on beer quality, HPP, thermosonication (TS), high pressure homogenization (HPH), and dense phase CO2 (DPCD) treatments revealed less effect on beer sensory properties, better retaining the freshness of original beer, compared to thermally processed beers.
Introduction to Beer Pasteurization
Beer is an alcoholic beverage, which is produced by yeast fermentation of the sugar obtained from malted cereal grains (e.g., barley and wheat). A Sumerian tablet found in Mesopotamia dated 6,000 years ago is the oldest evidence of beer production (Mirsky, 2007; Nelson, 2014). Global beer production amounted to about 1.91 billion of hectolitres in 2018, from 1.30 billion hectolitres in 1998, which demonstrates beer as a high demand beverage in the world (Statista, 2020). The production of beer consists of four stages: malting in which barley (or other cereal) is converted to malt, mashing where hops are added and malt is converted to wort by boiling, fermentation of sugars to ethanol (yeast pitching), and post-fermentation operations. The hops added during production are responsible for the bitter flavor and contribute to its natural preservation. The beer ingredients (water, cereal, hops, and yeast) can be combined in different ways to create different styles of beers such as ale, lager, stout, pilsner, etc. Ale and lager are the two major classes of beers: an ale beer ferments with top-cropping Saccharomyces cerevisiae at temperatures around 15–20°C, and a lager beer is fermented by bottom-cropping yeasts such as Saccharomyces carlsbergensis (pastorianus) or Saccharomyces uvarum at temperatures ranging between 8 and 13°C (Hardwick et al., 1995; Hornsey, 2003). Regions such as the Senne Valley in Belgium still use wild yeasts for spontaneous fermentation.
The fermenting yeast is the dominant microorganism present in beer. Regardless of the pasteurization method, existent microorganisms which survived the process can occasionally grow during storage and negatively affect the quality of beer. As opposed to pitching yeasts, wild yeasts are recognized as redundant yeasts throughout fermentation in beer production. The major groups of wild yeasts that can spoil beer belong to the genus Zygosaccharomyces, Saccharomyces, and Brettanomyces. According to Suzuki (2011), the microbial instability in beers is often caused by lactic acid bacteria of genera Lactobacillus and Pediococcus, and strict anaerobic bacteria belonging to genera Pectinatus and Megasphaera. Other spoilage organisms, particularly found in unpasteurized draft beers, are Micrococcus and Zymophilus bacteria, and moulds (Lawrence, 1988; Esmaeili et al., 2015).
The main post-fermentation operations of bottled or canned beer are clarification/filtration, filling, and pasteurization, which are followed by labeling, box packing, and palletizing. Keg beers might be pasteurized before the keg filling operation. Filtration is one of the oldest non-thermal methods employed by breweries before filling (bottling/canning). The shelf-life of filtered beer is much shorter in comparison to thermal pasteurized beers since some spoilage organisms still remain in the beer after filtration (Curtis, 1968). Then, a process of thermal pasteurization occurs in the industrial production of bottled beer, aiming to extend beer shelf life through the inactivation of undesirable spoilage microorganisms that will otherwise promote unwanted chemical reactions (Priest, 2003; Priest, 2006; Priest and Stewart, 2006; Wray, 2015). As beer also contains carbon dioxide and alcohol, and is made bitter with hops, all of which are natural antimicrobials, a mild thermal pasteurization is effective for its stabilization at room temperature (Silva and Gibbs, 2009) and to ensure instead of ensuring there are no issues with pathogenic microorganisms.
The term pasteurization comes from the scientist Louis Pasteur, who first used thermal pasteurization method, coincidentally to preserve beer. Since then, a variety of beverages and foods (e.g., fruit juices, and milk) have been preserved/stabilized this way to extend shelf-life. The intensity of a thermal pasteurization process is measured in pasteurization units or PU, where 1 PU is defined as 1 min treatment at 60°C (Baselt, 1958; Portno 1968; Tsang and Ingledew, 1982; Reveron et al., 2003; Reveron et al., 2005; Milani et al., 2015b). A 15 PU treatment is the minimum recommended for beer pasteurization, resulting in desired inactivation of spoilage yeasts and bacteria in beer (Del Vecchio et al., 1951; Portno, 1968; European Brewery Convention, 1995). However, the beer industry applies a more severe pasteurization process (e.g., 120–300 PU) to avoid spoilage due to on-going modifications in traditional beer composition such as alcohol-free and less bitter beers (Silva et al., 2014).
Since the conventional thermal process can negatively affect the beer flavor (Milani and Silva, 2016), emerging non-thermal pasteurization technologies like high pressure processing (HPP), pulsed electric fields (PEF), and power ultrasound (US) alone or combined with mild heating have been researched (Walkling-Ribeiro et al., 2011; Evelyn and Silva, 2015, 2016a, 2016b; Milani et al., 2015a; Sulaiman et al., 2015; Milani et al., 2016; Milani and Silva, 2016, 2017). The main objective of this work was to review the application of emerging non-thermal technologies to pasteurize beer, and the effect on beer quality parameters and key beer microorganisms.
Background on Conventional Thermal Pasteurization Effects on Beer Quality
Appearance (e.g., turbidity and haze), aroma and flavor, mouth feel, strength, specific gravity, and alcohol concentration are some of the beer quality parameters controlled in breweries (Eckhardt, 1989; Varnam and Sutherland, 1994; Ogle, 2007). The lightstruck character is a beer off-flavor defect caused by light. This phenomenon can be minimized using amber glass, bottles, or cans (Milani et al., 2015b). Changes in beer chemical composition during beer processing, ageing, and storage alter its sensory attributes (Vanderhaegen et al., 2006).
Craft beers produced in microbreweries are usually not pasteurized so that original properties of the beer produced are retained until consumption. However, this type of artisanal beer needs to be cold stored and distributed. Thermal treatment is the most common pasteurization method used in industrial large-scale breweries. As heat can have a negative impact on beer quality, some studies have reported the effect of thermal process on different quality characteristics of beer (Bernstein and Laufer, 1977; Vanderhaegen et al., 2003; Vanderhaegen et al., 2006; El Gharras, 2009; Lund and Andersen, 2011). Bernstein and Laufer (1977) concluded the formation of 2-furfural during beer thermal pasteurization is one of the most significant parameters causing beer staling and the loss of freshness. The presence of polyphenolic compounds is related to beer sensory characteristics, as these types of compounds are mainly responsible for the degree of bitterness or sweetness and mouthfeel in beer (El Gharras, 2009). Lima et al. (2009) revealed that thermal treatment dramatically affects the phenolic content of different foods which results to significant difference in sensory properties of the beer. Lund and Andersen (2011) concluded that the overall volatile profile of unpasteurized beers contained more fruity character and less staling volatile esters, suggesting that pasteurized beer has lower sensory acceptance than the fresh, non-thermally treated beer. Härnulv and Larsson (1992) studied the influence of thermal pasteurization and membrane filtration on the quality parameters of Swedish lager beer and recorded a staling effect on the thermally pasteurized beers at high PU levels compared to filtered non-heated beer. The thermal pasteurization process can generate off-flavors, as beer is a delicate beverage. With respect to flavor, the possibility of using a method of pasteurization with no heat or less heat would be welcomed by the brewing industry (Folkes, 2004; Milani, 2016). The following sections explore alternative non-thermal methods for beer pasteurization.
High Pressure Processing of Beer
High Pressure Processing Overview
High pressure processing (HPP) is one of the most significant innovations in food processing in recent decades. Pressures in the range of 200–600 MPa can inactivate microorganisms in foods and beverages (Figure 1). This technique retains the food’s natural freshness and quality parameters such as aroma, color, and important food components while destroying the spoilage bacteria, yeasts, and moulds in beverages (e.g., beer, wine, juices) or solid foods. Consequently, HPP technology is a breakthrough in processing solid or liquid foods. Nowadays, HPP food processing is being applied on ever-increasing commercial basis (Balda, 2018). In 2019, a semicontinuous unit appropriate for beverages was designed by Hiperbaric, which was named HPP “in bulk” process, to differentiate from the original HPP “in pack” process (Hiperbaric, 2021). The HPP “in bulk” allows the use of any type of packaging material, as aseptic filling is carried out after the process. Additionally, the combination of HPP with heat allows inactivation of microbial spores which are resistant to HPP at room temperature (Evelyn and Silva, 2015; Evelyn and Silva, 2016a, 2016b, 2017, 2019; Uchida and Silva, 2017; Silva and Evelyn, 2018). The high pressure thermal assisted processing (HPTP) is not applied industrially, although there are several research studies using lab scale units (e.g., 2 L HPP vessel). Although non-linear inactivation of microorganisms by HPP or HPTP has been reported, generally microbial inactivation increases when one instead of any of the three main processing parameters increases: pressure, temperature, or holding time (Farkas and Hoover, 2000; Silva et al., 2012; Milani et al., 2016; Milani and Silva, 2016; Silva and Evelyn, 2018).
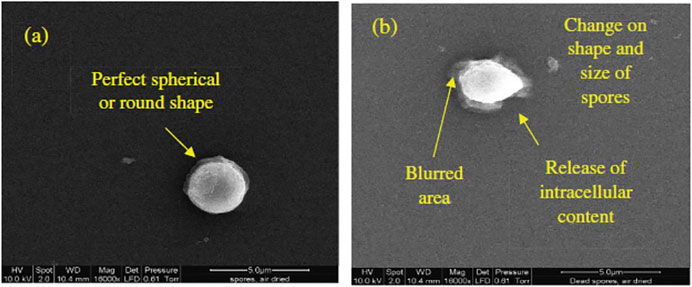
FIGURE 1. Environmental scanning election microscopy (eSEM) images of Saccharomyces cerevisiae free spores (a) untreated live (b) HPP treated dead (16,000
Commercial HPP batch vessel volumes range in size from 30 to 600 L. A common process cycle first loads the vessel with pre-packaged product, then fills the rest of the vessel void space with water, which acts as the pressure-transmitting fluid. Then, the vessel closes, and the expected process pressure is obtained through adding water that is delivered via an intensifier. After keeping the product at the target pressure for the pre-determined time, the vessel is decompressed by releasing the pressure (Balasubramaniam et al., 2008).
There are several studies on the mechanism of microbial inactivation via HPP, which is the result of a combination of factors. The cell membrane is the primary site for pressure-induced microbial inactivation (Figure 1). Microorganisms are known as resistant to selective chemical inhibitors because of their capability to eliminate such agents from the cell, through the cell membrane. However, once the membrane is injured, this capability is lost. Furthermore, HPP leads to some alterations in cell morphology (Rozali et al., 2017) (Figure 1), protein denaturation, and biochemical reactions inside the cell. HPP denature major microbial enzymes and disrupt ribosomes (Linton and Patterson, 2000). Larger injury of the microorganisms results from quick alterations in intracellular-extracellular differences at the membrane interface, leading to higher inactivation rate (Palou et al., 1998).
Clearly, there are opportunities for innovative use and new food products (Ferrentino et al., 2015). According to Balda (2018), more than 300 HPP units were installed and operating in food industries by 2016, with an annual production ratio of ≥250 thousand tones. HPP processed products available in the food market are mainly fruit juices, pre-cooked meals like meat and fish, and other beverages and smoothies (Mújica-Paz et al., 2011; Buzrul, 2012; Balda, 2018; Houška and Pravda). HPP food and beverages have longer shelf life than untreated products, and can meet customer’s quality expectations providing better taste and preservative-free healthier products (Nunes et al., 2017). At present time, one of the main disadvantages of this technology is the high capital investment, limiting HPP application to high value products. Additionally, the fact of being initially designed in batch mode is not advantageous for beverages.
High Pressure Processing Impact on Beer Quality
Table 1 presents a chronological review of past studies of the effect of HPP on beer properties/quality. Although in a few reports information is missing, all treatments are expected to be non-thermal (T < 45°C). For example, Milani and Silva (2016) obtained temperatures ≤36°C for HPP compression times of 15, 26, 45, and 60 s for 200, 300, 400, and 600 MPa, and noted a higher increase of temperature for higher pressures (longer compressions). Fischer et al. (2006) applied HPP at 300 and 700 MPa for 5 min on mash, wort, and pale lager beer. Compared to an untreated mash sample, the content of dissolved protein in HPP treated mash increased as the pressure increased. Moreover, the fermentation degree dropped with the increasing pressure and no changes in pH value were registered. For the HPP treatment of wort the results revealed HPP treatment could increase the bitterness more than thermal treatment. The results on pale lager beer samples showed that 300 MPa HPP treatment for 5 min did not significantly change the color, aromatic compounds, and foam stability, while a 700 MPa treatment for 5 min increased the beer haziness. Pale ale and mild ale beers were submitted to 600 MPa treatment for 5 min by Castellari et al. (2000) and compared to thermally treated beer. Color and permanent haze of pale ale beer were not affected by HPP as opposed to thermally pasteurized beer. Also, there was no effect of HPP on the main constituents of the beer. Permanent haze was more influenced by the stabilizing process in pale ale than in mild ale. Moreover, the results of HPP treatment (500 MPa and 5 min) on lager and pilsner beers that Fischer et al. (2002) carried out revealed no changes on HPP beer turbidity. Buzrul et al. (2005) concluded that HPP treatment of lager beer (300 MPa for 5 min) did not alter the bitterness of the original untreated beer, while thermal pasteurization increased the bitterness. This outcome emphasises the advantage of HPP compared to thermal processing with respect to a pasteurized beer flavor attribute. Recent studies of Milani and Silva (2016) also demonstrated no significant differences in the overall flavor of beers (ale and lager) submitted to 600 MPa for 30 s and untreated beers. In addition, preference testing confirmed no preference between the untreated and HPP treated beers. HPP processing at 600 MPa for 30 s (holding pressure phase) was performed to ensure a minimum treatment of 15 PU (equivalent to 15 min at 60°C) recommended for beer pasteurization (Milani et al., 2015a). Likewise, preference tests confirmed no preference between the untreated and HPP-treated beers.
Yin et al. (2016) sensorial studies on cloudy wheat beer revealed that original beer colloidal haze was retained with HPP treatment (600 MPa, 5 min). Moreover, analysis by dynamic light scattering showed that HPP treatments at 500 MPa/10 min demonstrated more uniform and smaller particle sizes than untreated beer, that leads to a better haze stability during beer storage. Štulíková et al. (2020) analyzed the quality of lager beer after HPP treatment at 250 and 550 MPa for 5 min. Both beers presented higher foam stability for 2 months storage compared to untreated beer. The original beer extract, alcohol content, apparent extract, pH, and colour did not change with the HPP process at 250 MPa. The foaming characteristics and colloidal stability of beer can be enhanced with HPP (Pérez-Lamela et al., 2002).
Overall HPP of beer can better retain the original flavor of the beer than thermal pasteurization, although for certain beers and at very higher pressures (≥700 MPa), the HPP can increase the beer haziness.
High Pressure Processing Microbial Inactivation in Beer
As mentioned previously, different types of microorganisms could contaminate beer during the brewing process. HPP pasteurization treatment often uses pressures up to 600 MPa (87,000 psi) for a particular holding time (Farkas and Hoover, 2000; Anon 2006; van Wyk and Silva, 2017a; van Wyk and Silva, 2017b; van Wyk et al., 2018). A review of the studies of HPP inactivation of yeasts and bacteria in beer is summarized in Table 2. Yin et al. (2016) studied the total yeast count of wheat beer (4.9% alc/vol) after 5 min processing of beer at 600 MPa and recorded 5.6 log reduction. Milani and Silva (2016) studied the non-thermal HPP S. cerevisiae ascospores as the most heat resistant form of spoilage yeasts for inactivation of beer. After 5 min HPP processing at 300 and 400 MPa of 4.8% alc/vol beer, 3.2 and 4.5 log reductions of S. cerevisiae ascospores were recorded, respectively. The minimum pasteurization is achieved in both HPP processes, as 15 PU is equivalent to 1.34 log reductions in the strain used by Milani and Silva (2016). For 7.0% alc/vol beer, 3.0 and 5.2 log reductions were achieved, respectively. Gaunzle et al. (2001) also studied on the effect of HPP on the inactivation of Lactobacillus plantarum in different alcohol level beers. They used a model beer using 5 and 10% alcohol and concluded that ethanol enhanced the effect of pressure on the inactivation rate of L. plantarum to maximum 5 log reduction of L. plantarum at 300 MPa and 5 min of treatment time at room temperature.
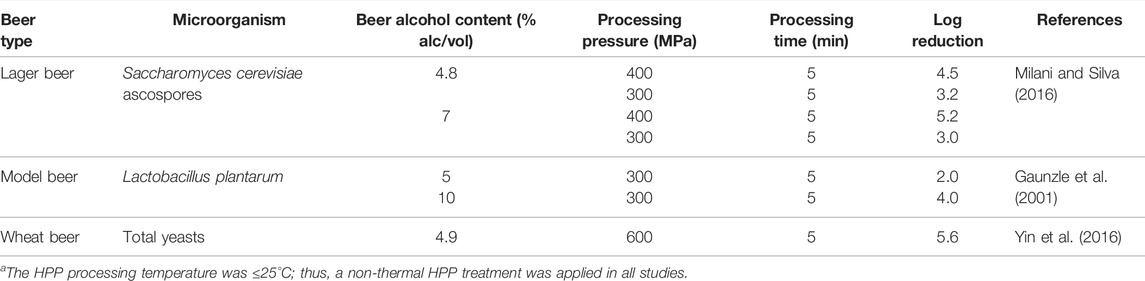
TABLE 2. HPP inactivation of yeasts and bacteria in beer.a
Referring to these results, HPP technology demonstrated an efficient method for beer pasteurization. The environmental scanning electron microscope (eSEM) images of S. cerevisae free spores before and after being submitted to 600 MPa HPP showed the loss of the spore round shape (integrity) with intracellular contents release, with viability loss confirmed by plating (Rozali et al., 2017).
Pulsed Electric Fields Pasteurization of Beer
Pulsed Electric Fields Overview
Sale and Hamilton (1967) initiated research on pulsed electric fields (PEF) in the sixties. From 1990 to date, PEF was investigated broadly in studies that mainly focused on the PEF disinfection of water and pasteurization of liquid food (Toepfl et al., 2006). Having low processing temperature and extremely short residence time, in the magnitude of microseconds, PEF leads to effective microbial inactivation and retention of product quality (Sulaiman et al., 2017). The permeabilization of the cellular tissue by PEF in microseconds could be developed to replace or complement conventional thermal pasteurization techniques. There is a great potential for PEF application to beverages, namely, beer and wine, as PEF can be set in continuous mode (Milani et al., 2015a; van Wyk et al., 2019a) (Figure 2). A typical continuous PEF system is based on a high voltage pulse generator with a treatment chamber and a suitable beverage handling system (pump, tube), as well as controlling devices to monitor inlet and outlet temperatures, electric field intensity, pulse frequency, and duration. Liquid food product is pumped through the treatment chamber, mainly in continuous mode, where two electrodes can be placed with different configurations, according to the chamber design (e.g., parallel, coaxial, and collinear). The high voltage electrical pulses are conveyed to the electrodes, and an electric field is generated between the two electrodes. While flowing within this gap between the 2 electrodes (the treatment chamber), the liquid food is submitted to the pulsed electric fields generated. The electric field intensity is the ratio between peak voltage and the gap between electrodes. Note that the CO2 present in the beer does not conduct the electric energy, possibly causing sparks. This is a process and risk issue to control during treatment. The main process parameters for PEF treatments are the electric field intensity, shape and width of the pulse, frequency of pulses, residence time (calculated from the flow rate and the volume of the treatment chamber), beverage electrical conductivity, and treatment temperature (which is the outlet temperature, Tout). A PEF treatment increases the temperature of the beverage due to Joule effect. A non-thermal PEF process (maximum Tout 40–45°C) can be assured by strict control PEF processing conditions and by lowering the initial temperature of the beverage (van Wyk et al., 2019b). The real treatment time can be calculated from the frequency and width of the PEF pulses, the flow rate of the liquid food product that is pumped into the treatment chamber, and its volume. The selection of conditions for these parameters determines the final lethal effect on the microbial population (Abram et al., 2003; Altunakar and Barbosa-Canovas, 2011; Milani et al., 2015a).
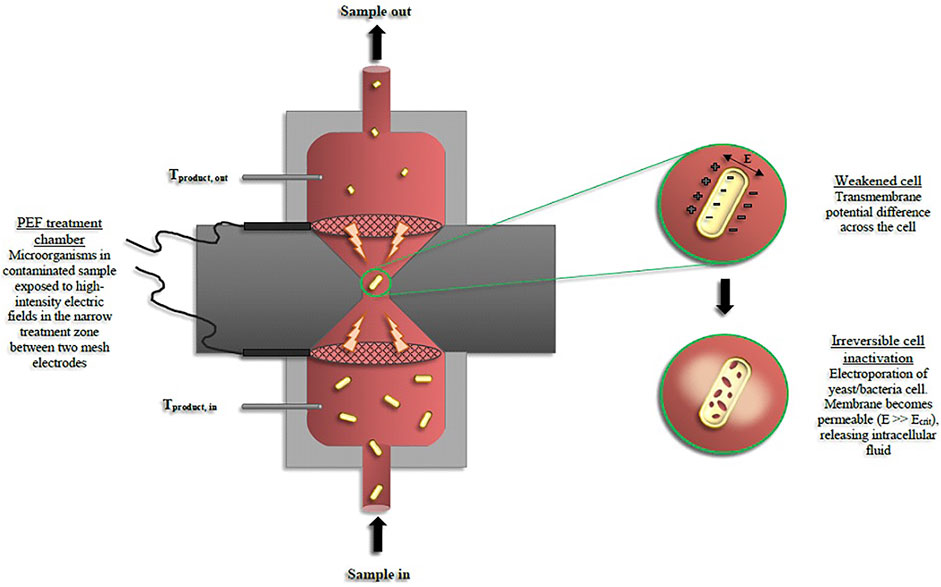
FIGURE 2. Diagram Showing the pulsed electric field (PEF) inactivation of beer spoilage microorganisms (T refers to temperature) in continuous mode (Reprinted from preservatives and preservation Approaches in Beverages, van Wyk, Silva, F. V. M., 2019. Chapter 7-Nonthermal preservation of Wine, PP. 203–235, with permission from Elsevier).
Pulsed Electric Fields Impact on Beer Quality
The main quality concern in the breweries is an off-flavor developed in beer exposed to light, that generates skunky off-notes in the beer, referred as the “lightstruck character” (Marsili et al., 2007). The 3-methyl-2-butene-1-thiol and organic sulphur compounds are responsible for this skunky smell, which limits the beer’s shelf life. The main reason for bottling beers in dark glasses or aluminium cans is to avoid or reduce the development of lightstruck character in beer during storage. Milani et al. (2015a) assessed the lightstruck character in nine different PEF beers (45 kV/cm, 70 µs), by comparing them with a control (untreated beer sample) and a lightstruck beer sample (exposed to sunlight for 8 h). The sensory panel could not detect differences between aroma and flavour of control, PEF, and lightstruck for Pilsner and dark ale beers indicating that these do not have a tendency to develop the lightstruck character with PEF treatment, probably due to the beers dark color. However, the panel could detect the lightstruck flavor/aroma in the rest of light lager beers treated by PEF. Hence, it is important to select the beer and optimize the PEF processing conditions to avoid the development of this and other undesirable flavors. A trained panel of 25 judges detected differences in the beer flavor and mouth feeling, with a significantly lower rating for PEF beer exposed to a treatment of 41 kV/cm for 175 µs treatment time (Evrendilek et al., 2004) compared with control samples (untreated beers). Some panellists detected a metallic mouth feeling, which was probably associated with the migration of compounds from the PEF electrodes to the beverage during the treatment. Oziemblowski et al. (2017) observed lower bitterness and turbidity of PEF processed beer submitted to 200, 300, and 400 pulses in comparison to unpasteurized beer, which could be considered as a beneficial effect of PEF pasteurization of beer.
Overall pulsed electric fields can develop the lightstruck character in certain beers, depending on the processing conditions.
Pulsed Electric Fields Microbial Inactivation in Beer
During the PEF treatment, the electric field inactivates the microorganisms in foods by increasing the transmembrane potential in the cell membrane until electroporation takes place (the permeabilization of the cell and organelles membranes), and subsequent cell death (Heinz et al., 2001). Since PEF pasteurization can be operated in a continuous mode, it has the potential to be implemented in the beverage industry at a commercial scale to preserve drinks. Some studies have investigated the effect of PEF or temperature-assisted PEF on the inactivation of microbial populations in beer. Table 3 shows results of PEF studies carried out with yeasts in beers, while Table 4 shows studies conducted with bacteria in keg and lager beers. Milani et al. (2015a) studied the non-thermal PEF and thermal assisted PEF pasteurization of beer with different alcohol contents to inactivate the heat resistant S. cerevisiae ascospores. PEF treatment of 45 kV/cm electric field for 70 µs(real treatment time) at room temperature for 0.0, 4.0, and 7.0% alc/vol beers and recorded 0.2, 1.1, and 2.2 log reductions of S. cerevisiae ascospores, respectively. Thermally assisted PEF at 52–53°C (45 kV/cm, 70 µs) presented a higher spore inactivation: 0.9, 2.9, and 4.0 log reductions in 0.0, 4.8, and 7.0% alc/vol beers, respectively. The other studies presented in Table 3 were carried out with vegetative forms of yeasts. Walkling-Ribeiro et al. (2011) studied non-thermal and temperature assisted PEF treatment of 3.5% alc/vol beer to inactivate the S. cerevisiae vegetative cells. Non-thermal PEF processes of 35 kV/cm for 574 µs and 45 kV/cm for 402 µs caused 3.8 and ≥6.8 log reductions of S. cerevisae vegetative cells, respectively. Thermal assisted PEF treatment of beer at 55°C, 35 kV/cm for 1145 µs resulted in ≥6.8 log reduction of S. cerevisiae vegetative cells. Evrendilek et al. (2004) studied the effect of PEF on vegetative yeasts and obtained 4.1 log reductions of Saccharomyces uvarum and 4.3 log reductions of Rhodotorula rubra in a keg beer using a 10°C cooling water bath to ensure a non-thermal process (22 kV/cm, 216 µs).
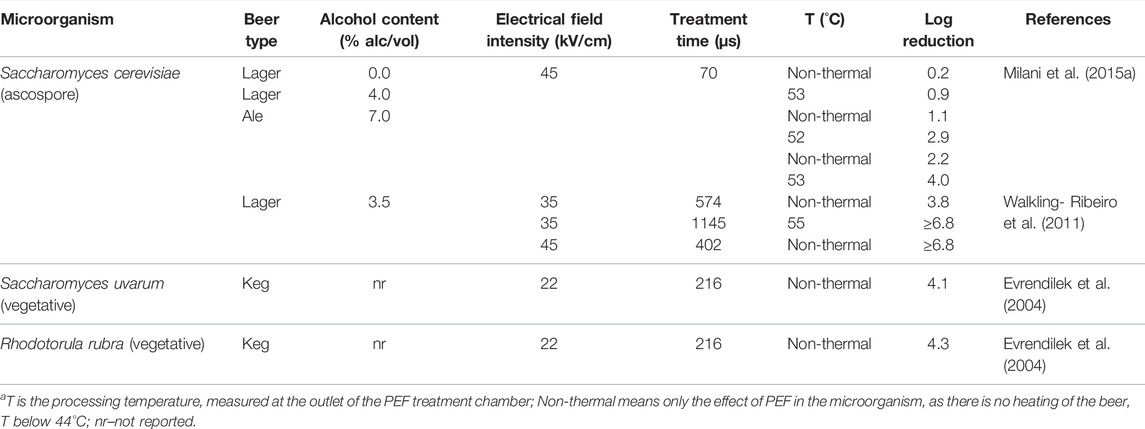
TABLE 3. PEF inactivation of yeasts in beer.a
PEF inactivation of Lactobacillus plantarum vegetative bacteria was studied by Ulmer et al. (2002) in model beer (Table 4). The results showed >3.0 log reduction of bacteria during the non-thermal PEF at 35 kV/cm electric field intensity. Evrendilek et al. (2004) studied the effect of non-thermal PEF treatment (41 kV/cm and 175 µs) on three different bacteria L. plantarum, Pediococus damnosus, and Bacillus subtilis vegetative cells in keg beer and recorded 4.7, 5.8, and 4.8 log reductions, respectively. Walkling-Ribeiro et al. (2011) carried out non-thermal and thermally assisted PEF treatment of L. plantarum, Salmonella enterica, and B. subtilis vegetative bacteria in 3.5% alc/vol lager beer. A non-thermal PEF (35 kV/cm and 574 µs) caused similar inactivation in the three bacteria: 1.8, 2.0, and 1.8 log reductions for L. plantarum, S. enterica, and B. subtilis, respectively. A non-thermal PEF treatment of higher electric field (45 kV/cm, 402 µs) led to a higher inactivation of L. plantarum (3.2 log reductions). Thermally assisted PEF at 52°C, 45 kV/cm, 804 µs caused a higher log reduction of 6.5. Regarding S. enterica, the same authors recorded 4.0 log reduction after a non-thermal PEF process of 45 kV/cm and 536 µs and 5.7 log reduction at 49°C, 45 kV/cm, and 804 µs. With respect to B. subtilis, 3.0 log reductions were achieved at room temperature (45 kV/cm and 536 µs) and 3.4 log reductions at 49°C (45 kV/cm and 402 µs) were registered, respectively. In resume the higher the PEF temperature, the higher the microbial inactivation. Same conclusions were taken by a few PEF-thermal studies carried out with other beverages and foods (Jayaram et al., 1992; Zhang et al., 1995; Vega-Mercado et al., 1997).
The effect of microorganism size on PEF inactivation could be demonstrated by comparing bacteria and yeast inactivation submitted to the same PEF conditions (Walkling-Ribeiro et al., 2011). While the three different bacteria were reduced by a magnitude of approximately 2 logs after a PEF treatment of 35 kV/cm for 574 µs (Table 4), 3.8 decimal reductions were obtained with the yeast S. cerevisiae. Vegetative yeasts are larger (4–6 µm) than bacteria, and thus more susceptible to PEF than bacteria. The review also showed that it is more difficult to inactivate yeast spores by PEF than vegetative yeast cells (Table 3).
Power Ultrasound Treatment of Beer
Ultrasound Overview
Ultrasound are sonic waves of the same physical nature as sound, but with frequencies above the range of human hearing (20–100 kilo Hertz, kHz). Based on frequency difference and sound intensity, ultrasound waves are classified in two categories. High frequency ultrasound (diagnostic ultrasound) operates at frequencies of 2–20 MHz with sound intensities in the range of 0.1–1 W/cm2. Food quality assessment, medical imaging, and non-devastating inspection are examples in which high frequency ultrasound is used. Lower frequencies (20–100 kHz) with a sound intensity of 10–1000 W/cm2 are employed in power ultrasound (also called high intensity ultrasound). Because of high energy level, power ultrasound is considered appropriate to be used in the food industry for microbial destruction and equipment disinfection (Baumann et al., 2005, 2009; Ugarte et al., 2006). Power ultrasound processing, often called sonication, operates through a liquid medium which can be the food or water containing a solid food (Figure 3). Nowadays, ultrasound technology can be used more widely in food pasteurization applications. The main US applications for food processing at a commercial scale are emulsification, size reduction, crystallization, and extraction (Feng and Yang, 2011).
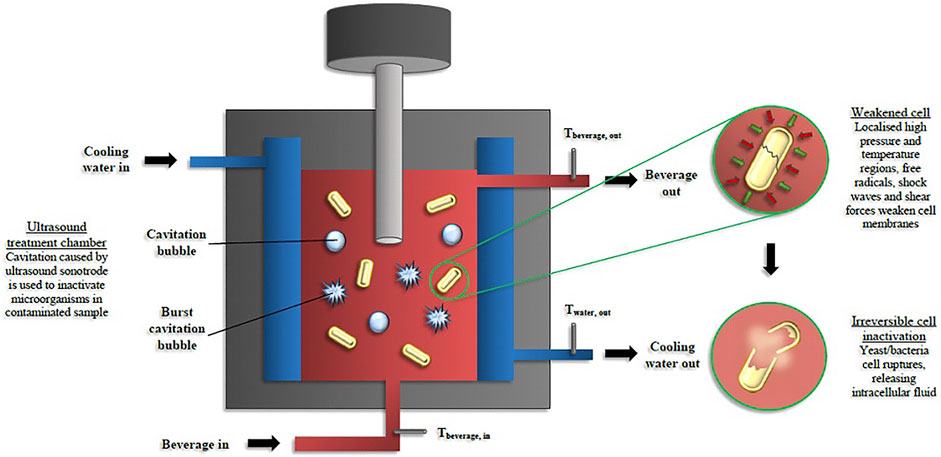
FIGURE 3. Diagram Showing Power ultrasound (US) inactivation of beer spoilage microorganisms (T refer to temperature) in continuous mode (Reprinted from preservatives and preservation Approaches in Beverages, van Wyk, S., Silva, F. V. M., 2019. Chapter 7-Nonthermal preservation of Wine, PP. 203–235, with permission from Elsevier).
Ultrasound Impact on Beer Quality
Ultrasound of 1 MHz has been applied for wines, whiskey, and spirits, and results showed that it has changed the alcohol/ester balance creating an effect similar to ageing (Mason et al., 1996). With respect to applications to beer industry, at the beginning of the mashing process, US increases the beer yield and can reduce the fermentation time. This process increases the oxidation in fermented products, which leads to a better flavor and maturation. It also improves the hygiene of beer defobbing and defoaming operations before bottling (Chemat and Khan, 2011). Deng et al. (2018) did not record significant changes on physicochemical and sensorial properties of Chinese lager beer submitted to the following US and thermosonication (TS, simultaneous exposure to US and heat) conditions: 2.7 W/ml acoustic power density, temperatures of 40, 50, and 60°C, for 2 min treatment time. However, a higher acoustic power density (10.8 W/ml) and temperature (75°C) TS process for 20.5 s, equivalent to a 15 PU process, developed an unacceptable haze in ale and lager beers (Silva et al., 2015). Borsato et al. (2019) concluded that sonication of malted barley increased the size of the starch granules, and a better quality was obtained when malted barley was exposed to TS compared with thermally treated or untreated malted barley. Xiao et al. (2020) showed that the main parameters (ethanol content, original gravity, pH, bitterness, and viscosity) of wheat beer were scarcely affected by TS (at 50°C and 2.7 W/ml for 3 min). Meanwhile, TS-treated beer had higher colloidal haze and foam stability than thermally (60°C-15 min) pasteurized beer. Dynamic light scattering analysis showed that TS treatment resulted in a smaller and more uniform particle size, thus having a positive effect on the desired beer haze stability.
Overall, ultrasound technology seems to have potential for beer pasteurization. An increase in the stability of beer turbidity and haze was observed and the overall beer quality was not much affected by the US process. In addition, this technology can also be applied to improve the yield of other operations (e.g., mashing) and speed up the beer production.
Ultrasound Microbial Inactivation in Beer
Microbial inactivation mechanism of ultrasound can be explained through the effect of cavitation on microbial cell walls (Figure 3). Water jets of liquid, generated by the asymmetric implosion of transient cavitating bubbles, may cause severe cell envelope damage and cleavage of the texture of the polymeric materials of the cell walls (Evelyn and Silva, 2020). In terms of the chemical effects, transient cavitation can create OH- and H radicals and hydrogen peroxide. In addition, stable cavitating bubbles can generate microstreaming alongside the bubble and create high hydrodynamic shear stresses, which cause cell membrane damages. Many studies have been carried out to investigate the application of power ultrasound on the inactivation of different yeasts and bacteria in foods and beverages (Adekunte et al., 2010; Bauman et al., 2005; Char et al., 2010; and; Suslick and Nyborg, 1990), including wine (Luo et al.,2012; Gracin et al., 2016).
Milani and Silva (2017) studied the effect of power ultrasound treatment with and without heat on lager beers pasteurization. A pasteurization of 15 pasteurization units, equivalent to 1.34 log reductions in S. cerevisiae ascospores, was achieved in 4.8% alc/vol beer when batch mode 16.2 W/ml thermosonication at 50°C for 1.9 min or TS at 55°C for 26 s was applied. On the other hand, 16.2 W/ml US without heat at room temperature (23°C) required 20 min for the same level of pasteurization. Deng et al. (2018) demonstrated the inactivation of yeast and spoilage bacteria (including lactic acid bacteria and aerobic bacteria) in lager beer by 2.7 W/ml TS at 50°C and 60°C for 2 min. Xiao et al. (2020) showed that the microbiological stability of TS-treated wheat beer (at 50°C and 2.7 W/ml for 3 min) was comparable with that of the heat-treated sample (at 60°C for 15 min).
The studies demonstrated US and ultimately TS at 50–55°C are efficient methods to pasteurize beer.
Other Non-Thermal Technologies
Dense-Phase CO2
DPCD is known as a non-thermal technique that inactivates spoilage microorganisms and enzymes through carbon dioxide molecular effect at a pressure of below 50 MPa while maintaining the freshness, sensory attributes, and nutritional qualities of food (Damar and Balaban, 2006). Fraser (1951) was the first scientist demonstrating the microbial inactivation by gas pressure of various types of bacteria. The mechanism of microbial inactivation by DPCD is not well known. However, researchers have demonstrated that various processes can be involved in the microbial inactivation (Fraser 1951; Daniels et al., 1985; Lin et al., 1991; Nakamura et al., 1994; Ishikawa et al., 1995): intra- and extracellular pH decrease due to the acidic effect of CO2; proteins and ions precipitation; inhibition of biological activities; and modifications of cell walls that cause cell rupture and extraction of cellular substances, leading to intracellular electrolyte balance disorder (Erkmen and Karaman, 2001).
Some studies with different foods DPCD pasteurization have been carried out (Haas et al., 1989; Arreola et al., 1991; Ishikawa et al., 1997; Shimoda et al., 1998; Erkmen and Karaman, 2001; Folkes, 2004; Kincal et al., 2005; Damar and Balaban, 2006). Apart from fruit juices, DPCD was applied to alcoholic beverages like beer. Folkes (2004) studied the effect of DPCD on microbial inactivation and sensorial parameters of beer and found no significant difference between the untreated beer and DPCD treated beers. Dagan and Balaban (2006) studied the pasteurization of beer by DPCD to inactivate vegetative yeasts and got a maximum 7.38 log reduction of yeasts at 26.5 MPa, 21°C, 9.6% CO2, and 4.77 min residence time. With respect to beer quality, haze was reduced by DPCD pasteurization from 146 nephelometric turbidity units (NTU) to 95. The aroma and flavor of treated beer were not significantly different from fresh beer. Foam capacity and stability were minimally affected by CO2 processing, although changes would most likely be unnoticed by consumers. These results revealed that DPCD technology is an effective treatment for beer pasteurization while retaining the aroma and freshness of beer. This cold pasteurization technique can be used as an alternative method by the industry as it could preserve the nutritional and haze characteristics of beer during storage.
High Pressure Homogenization
High pressure homogenization (HPH) is another non-thermal technology which is widely investigated for food pasteurization (Puig et al., 2008; Franchi et al., 2011; Franchi et al., 2013; Comuzzo et al., 2015). In HPH, the fluid food is exposed to a forced pressure and rapid acceleration of media in a thin gap during a continuous process e.g., 200 m/s at 340 MPa. Then, a sudden drop of pressure causes cavities, shear, and friction of microbial cells that leads to microbiological inactivation (Franchi et al., 2011; Franchi et al., 2013). Franchi et al. (2013) studied the effect of HPH treatment on microbial inactivation and quality parameters of beer. He ran HPH treatment on Pilsen beer inoculated by lactic acid bacteria, acetic bacteria, and yeasts. Lactobacillus delbrueckii was the most resistant microorganism, requiring 250 MPa for a 6 log reduction. Furthermore, Franchi et al. (2011) claims that according to the molecular conformational changes in polysaccharides and proteins of beer, this process promotes a positive effect on beer quality and improves beer color. Also, an addition of a small amount of lysozyme (50 mg/L) would enable a reduction in the level of pressure to 150 MPa, with energy and costs savings. Overall, HPH processing has the potential of cost-effective commercial application in the beer industry.
Ultraviolet Irradiation
Ultraviolet treatment is another promising technology that is widely used for food pasteurization as an alternative for heat treatment (Bintsis et al., 2000). In the food industry, UV irradiation is mainly used for milk, juices, and cider preservation (Basaran et al., 2004; Quintero-Ramos et al., 2004; Matak et al., 2005; Koutchma, 2019). Main parameters in UV-C processing are the time and doses of applied. According to Bintsis et al. (2000), the most efficient wavelengths for pasteurization are between 200 and 280 nm, so called as UV-C. The DNA of virus, bacteria, fungi, yeasts, and molds is destroyed through the exposure to irradiation and hence the reproduction process of microbial cells is stopped (Koutchma, 2019).
Some researchers carried out studies to investigate the effect of UV irradiation on beer pasteurization and its sensory properties. Lu et al. (2010) optimized the optical-fiber distribution density and thin-film thickness for treating beer by UV. The apparatus could reduce more than 4 log of inoculated Lactobacillus brevis in draft beer at UV doses of 9.7 mJ/cm2, while the reduction of S. cerevisiae was not as efficient. They concluded that naturally contaminating lactic acid bacteria and Enterobacteriaceae could be effectively inactivated in draft beer. Moreover, the results of Hosseini et al. (2011) on UV processing of non-alcoholic beer demonstrated that beer spoilage microorganisms were totally inactivated at pulse UV wavelength of 266 nm. Mezui and Swart (2010) studied the sensory parameters of UV treated beer, and reported the formation of lightstruck flavor in beer processed by low ultraviolet light irradiation (UV-C).
While successful microbial inactivation in beer by UV has been reported, more sensory studies are needed to assess the impact of this process on the formation of the lightstruck character in different beers.
Comparison of Technologies and Final Remarks
This review demonstrated the advantages and limitations of novel non-thermal technologies in terms of beer quality and microbial inactivation compared to conventional thermal processing. The CO2 present in beer can cause technological problems, and this issue needs to be addressed (e.g., sparks in PEF treatment). In general PEF, thermosonication, HPP, HPH, and DPCD treatments revealed less effect on beer sensory properties, retaining more of the nutritional and freshness of original beer, compared to thermally processed beers.
Non-thermal HPP inactivation of S. cerevisiae ascospores in beer is much faster (few seconds for 15 PU) than thermosonication (few minutes) and thermal pasteurization (15 min) (Milani et al., 2016; Evelyn et al., 2017). The high pressure (HPP) process is more efficient than thermal treatment for equivalent yeast inactivation, retaining the original beer flavour and requiring less energy (HPP—77 kJ/L vs 60°C thermal—189 kJ/L) (IChemE, 2016; Milani et al., 2016). The most recently developed commercial HPP process “in bulk” followed by aseptic filling allows the use of glass bottles or cans for beer packaging while keeping the freshness of the original non processed beer. Power ultrasound at room temperature presented insufficient microbial inactivation, while thermal assisted sonication (thermosonication) was successful for beer pasteurization (15 PU) and also increased the stability of beer turbidity and haze. For both direct contact or indirect US systems, as the distance from the wave source increases, the efficiency of microbial inactivation decreases. US treatment PEF and UV-C developed the undesirable lightstruck character in beer, with a negative impact in beer sensory quality.
Some of the alternative methods can achieve higher microbial log reductions, with shorter processing times, and less energy requirements compared with the conventional thermal processing, while keeping the freshness of the raw beer.
The research on non-thermal pasteurization of other alcoholic beverages is expanding (van Wyk et al., 2019a). More research is needed to compare capital investment, costs of operation, energy consumption, and sustainability of different technologies. Some of these technologies are not commercially available yet at a larger scale. Therefore, more studies at pilot and industrial scales are needed to elucidate the industrial feasibility of the non-thermal technologies reviewed.
Author Contributions
Both authors have made a substantial, direct, and intellectual contribution to the work and approved it for publication.
Conflict of Interest
The authors declare that the research was conducted in the absence of any commercial or financial relationships that could be construed as a potential conflict of interest.
Publisher’s Note
All claims expressed in this article are solely those of the authors and do not necessarily represent those of their affiliated organizations, or those of the publisher, the editors and the reviewers. Any product that may be evaluated in this article, or claim that may be made by its manufacturer, is not guaranteed or endorsed by the publisher.
References
Abram, F., Smelt, J. P. P. M., Bos, R., and Wouters, P. C. (2003). Modelling and Optimization of Inactivation of Lactobacillus plantarum by Pulsed Electric Field Treatment. J. Appl. Microbiol. 94 (4), 571–579. doi:10.1046/j.1365-2672.2003.01869.x
Adekunte, A., Tiwari, B. K., Scannell, A., Cullen, P. J., and O'Donnell, C. (2010). Modelling of Yeast Inactivation in Sonicated Tomato Juice. Int. J. Food Microbiol. 137 (1), 116–120. doi:10.1016/j.ijfoodmicro.2009.10.006
Altunakar, B., and Barbosa-Cánovas, G. V. (2011). Engineering Aspects of Pulsed Electric fields. Nonthermal Process. Tech. Food 45, 176–189. doi:10.1002/9780470958360.ch12
Anon, A. (2006). Requisite Scientific Parameters for Establishing the Equivalence of Alternative Methods of Pasteurization. J. Food Prot. 69 (5), 1190–1216. doi:10.4315/0362-028x-69.5.1190
Arreola, A. G., Balaban, M. O., Marshall, M. R., Peplow, A. J., Wei, C. I., and Cornell, J. A. (1991). Supercritical Carbon Dioxide Effects on Some Quality Attributes of Single Strength Orange Juice. J. Food Sci. 56 (4), 1030–1033. doi:10.1111/j.1365-2621.1991.tb14634.x
Balasubramaniam, V. M., Farkas, D., and Turek, E. (2008). Preserving Foods through High-Pressure Processing. Food Tech. 62 (11), 32–38.
Balda, F. P. B. (2018). “Current Status of Industrial HPP Equipment for Food Processing,” in High Pressure Processing of Fruit and Vegetable Juices. Editors M. Houška, and F. V. M. Silva, (CRC Press), 73–83.
Basaran, N., Quintero-Ramos, A., Moake, M. M., Churey, J. J., and Worobo, R. W. (2004). Influence of Apple Cultivars on Inactivation of Different Strains of Escherichia coli O157:H7 in Apple Cider by UV Irradiation. Appl. Environ. Microbiol. 70, 6061–6065. doi:10.1128/aem.70.10.6061-6065.2004
Baumann, A. R., Martin, S. E., and Feng, H. (2005). Power Ultrasound Treatment of Listeria monocytogenes in Apple Cider. J. Food Prot. 68 (11), 2333–2340. doi:10.4315/0362-028x-68.11.2333
Baumann, A. R., Martin, S. E., and Feng, H. (2009). Removal of Listeria Monocytogenes Biofilms from Stainless Steel by Use of Ultrasound and Ozone. J. Food Prot. 72, 1306–1309. doi:10.4315/0362-028x-72.6.1306
Bernstein, L., and Laufer, L. (1977). Further Studies on Furfural: The Influence of Raw Materials, Processing Conditions, and Pasteurization Temperatures. J. Am. Soc. Brewing Chemists 35 (1), 21–24. doi:10.1094/asbcj-35-0021
Bintsis, T., Litopoulou-Tzanetaki, E., Davies, R., and Robinson, R. K. (2000). The Antimicrobial Effects of Long-Wave Ultra-violet Light and Furocoumarins on Some Micro-organisms that Occur in Cheese Brines. Food Microbiol. 17 (6), 687–695. doi:10.1006/fmic.2000.0370
Borsato, V. M., Jorge, L. M., Mathias, A. L., and Jorge, R. M. (2019). Ultrasound Assisted Hydration Improves the Quality of the Malt Barley. J. Food Process Eng. 42 (6), e13208. doi:10.1111/jfpe.13208
Buzrul, S., Alpas, H., and Bozoglu, F. (2005). Effect of High Hydrostatic Pressure on Quality Parameters of Lager Beer. J. Sci. Food Agric. 85 (10), 1672–1676. doi:10.1002/jsfa.2166
Buzrul, S. (2012). High Hydrostatic Pressure Treatment of Beer and Wine: A Review. Innovative Food Sci. Emerging Tech. 13, 1–12. doi:10.1016/j.ifset.2011.10.001
Castellari, M., Arfelli, G., Riponi, C., Carpi, G., and Amati, A. (2000). High Hydrostatic Pressure Treatments for Beer Stabilization. J. Food Sci. 65, 974–977. doi:10.1111/j.1365-2621.2000.tb09402.x
Char, C. D., Mitilinaki, E., Guerrero, S. N., and Alzamora, S. M. (2010). Use of High-Intensity Ultrasound and UV-C Light to Inactivate Some Microorganisms in Fruit Juices. Food Bioproc. Technol 3 (6), 797–803. doi:10.1007/s11947-009-0307-7
Chemat, F., Zill-e-Huma, M. K., and Khan, M. K. (2011). Applications of Ultrasound in Food Technology: Processing, Preservation and Extraction. Ultrason. Sonochem. 18 (4), 813–835. doi:10.1016/j.ultsonch.2010.11.023
Comuzzo, P., Calligaris, S., Iacumin, L., Ginaldi, F., Palacios Paz, A. E., and Zironi, R. (2015). Potential of High Pressure Homogenization to Induce Autolysis of Wine Yeasts. Food Chem. 185, 340–348. doi:10.1016/j.foodchem.2015.03.129
Curtis, N. S. (1968). European Brewery Convention. J. Inst. Brewing 74 (4), 330–333. doi:10.1002/j.2050-0416.1968.tb03135.x
Dagan, G. F., and Balaban, M. O. (2006). Pasteurization of Beer by a Continuous Dense‐phase CO2 System. J. Food Sci. 71 (3), 164–169. doi:10.1111/j.1365-2621.2006.tb15630.x
Damar, S., and Balaban, M. O. (2006). Review of Dense Phase CO2 Technology: Microbial and Enzyme Inactivation, and Effects on Food Quality. J. Food Sci. 71 (1), R1–R11. doi:10.1111/j.1365-2621.2006.tb12397.x
Daniels, J. A., Krishnamurthi, R., and Rizvi, S. S. H. (1985). A Review of Effects of Carbon Dioxide on Microbial Growth and Food Quality. J. Food Prot. 48, 532–537. doi:10.4315/0362-028x-48.6.532
Deng, Y., Bi, H., Yin, H., Yu, J., Dong, J., Yang, M., et al. (2018). Influence of Ultrasound Assisted thermal Processing on the Physicochemical and Sensorial Properties of Beer. Ultrason. Sonochem. 40, 166–173. doi:10.1016/j.ultsonch.2017.07.017
El Gharras, H. (2009). Polyphenols: Food Sources, Properties and Applications - a Review. Int. J. Food Sci. Tech. 44 (12), 2512–2518. doi:10.1111/j.1365-2621.2009.02077.x
Erkmen, O., and Karaman, H. (2001). Kinetic Studies on the High Pressure Carbon Dioxide Inactivation of Salmonella typhimurium. J. Food Eng. 50, 25–28. doi:10.1016/s0260-8774(00)00191-6
Esmaeili, S., Mogharrabi, M., Safi, F., Sohrabvandi, S., Mortazavian, A. M., and Bagheripoor-Fallah, N. (2015). The Common Spoilage Microorganisms of Beer: Occurrence, Defects, and Determination-A Review. Carpathian J. Food Sci. Tech. 7 (4).
European Brewery Convention (1995). Beer Pasteurisation (Manual of Good Practice). Nuremberg, Germany: Fachverlag Hans-Carl.
Evelyn and Silva, F. V. M. (2015). High Pressure Processing of Milk: Modeling the Inactivation of Psychrotrophic Bacillus cereus Spores at 38-70°C. J. Food Eng. 165, 141–148. doi:10.1016/j.jfoodeng.2015.06.017
Evelyn and Silva, F. V. M. (2016a). High Pressure thermal Processing for the Inactivation of Clostridium perfringens Spores in Beef Slurry. Inn. Food Sci. Emer. Technol. 33, 26–31. doi:10.1016/j.ifset.2015.12.021
Evelyn and Silva, F. V. M. (2016b). Modeling the Inactivation of Psychrotrophic Bacillus cereus Spores in Beef Slurry by 600 MPa HPP combined with 38–70°C: Comparing with thermal processing and estimating the energy requirements. Food Bioprod. Process. 99, 179–187.
Evelyn and Silva, F. V. M. (2017). “Inactivation of Pathogenic Microorganisms in Foods by High-Pressure Processing,” Chapter 10 in Food Safety and Protection. Editors V. R. Rai, and J. A. Bai (CRC Press), 343–378. doi:10.1201/9781315153414-10
Evelyn, Milani, E., and Silva, F. V. M. (2017). Comparing High Pressure thermal Processing and Thermosonication with thermal Processing for the Inactivation of Bacteria, Moulds, and Yeasts Spores in Foods. J. Food Eng. 214, 90–96. doi:10.1016/j.jfoodeng.2017.06.027
Evelyn and Silva, F. V. M. (2019). Heat Assisted HPP for the Inactivation of Bacteria, Moulds, and Yeast Spores in Foods: Log Reductions and Mathematical Models. Trends Food Sci. Tech. 88, 143–156. doi:10.1016/j.tifs.2019.03.016
Evelyn and Silva, F. V. M. (2020). Ultrasound Assisted thermal Inactivation of Spores in Foods: Pathogenic and Spoilage Bacteria, Molds and Yeasts. Trends Food Sci. Tech. 105, 402–415. doi:10.1016/j.jfoodeng.2017.06.027
Evrendilek, G., Li, S., Dantzer, W., and Zhang, Q. (2004). Pulsed Electric Field Processing of Beer: Microbial, Sensory, and Quality Analyses. J. Food Sci. 69 (8), 228–232. doi:10.1111/j.1365-2621.2004.tb09892.x
Farkas, D. F., and Hoover, D. G. (2000). High Pressure Processing. J. Food Sci. Spec. Suppl. 65, 47–64. doi:10.1111/j.1750-3841.2000.tb00618.x
Feng, H., and Yang, W. (2011). Ultrasonic Processing. Nonthermal Process. Tech. Food 19, 135–154. doi:10.1002/9780470958360.ch10
Ferrentino, G., Spilimbergo, S., and Bertucco, A. (2015). “High-Pressure Processing of Foods toward Their Industrialization and Commercialization: an Up-To-Date Overview,” in Functional Food Ingredients and Nutraceuticals and Processing Technologies. Editor J. Shi (Boca Raton, FL: CRC Press), 427–454. doi:10.1201/b19426-17
Fischer, S., Schöberl, H., Ruß, W., and Meyer-Pittroff, R. (1999). “The Effects of Hydrostatic High Pressure on the Brewing Process and Beer,” in Advances in High Pressure Bioscience and Biotechnology. Editor H. Ludwig (Berlin, Heidelberg: Springer), 419–422. doi:10.1007/978-3-642-60196-5_95
Fischer, S., Russ, W., and Meyer-Pittroff, R. (2002). “High Pressure Advantages for Brewery Processes,” in Trends in High Pressure Bioscience and Biotechnology. Editor R. Hayashi (Elsevier Science), 397–404. doi:10.1016/s0921-0423(02)80130-7
Fischer, S., Russ, W., Buckow, R., Heinz, V., Ulmer, H., and Behr, J. (2006). Effects of Hydrostatic High Pressure on Microbiological and Technological Characteristics of Beer. Monatsschrift für Brauwissenschaft 59, 90–99.
Folkes, G. (2004). Pasteurization of Beer by a Continuous Dense-phase CO2 System. Doctoral dissertation, University of Florida.
Franchi, M. A., Tribst, A. A. L., and Cristianini, M. (2011). Effects of High Pressure Homogenization on Beer Quality Attributes. J. Inst. Brewing 117 (2), 195–198. doi:10.1002/j.2050-0416.2011.tb00460.x
Franchi, M. A., Tribst, A. A. L., and Cristianini, M. (2013). High-pressure Homogenization: a Non-thermal Process Applied for Inactivation of Spoilage Microorganisms in Beer. J. Inst. Brew. 119 (4), 237–241. doi:10.1002/jib.99
Fraser, D. (1951). Bursting Bacteria by Release of Gas Pressure. Nature 167, 33–34. doi:10.1038/167033b0
Gaunzle, M. G., Ulmer, H. M., and Vogel, R. F. (2001). High Pressure Inactivation of Lactobacillus plantarum in a Model Beer System. J. Food Sci. 66 (8), 1174–1181.
Gracin, L., Jambrak, A. R., Juretić, H., Dobrović, S., Barukčić, I., Grozdanović, M., et al. (2016). Influence of High Power Ultrasound on Brettanomyces and Lactic Acid Bacteria in Wine in Continuous Flow Treatment. Appl. Acoust. 103, 143–147. doi:10.1016/j.apacoust.2015.05.005
Haas, G. J., Prescott, H. E., Dudley, E., Dik, R., Hintlian, C., and Keane, L. (1989). Inactivation of Microorganisms by Carbon Dioxide under Pressure. J. Food Saf. 9, 253–265. doi:10.1111/j.1745-4565.1989.tb00525.x
Hardwick, W. A., van Oevelen, D. E. J., Novellie, L., and Yoshizawa, K. (1995). “Kinds of Beer and Beer like Beverages,” in Handbook of Brewing. Editor W. A. Hardwick (New York: Marcel Dekker), 53–86.
Härnulv, B. G., and Larsson, T. (1992). A Study of the Influence of Pasteurization and Membrane Filtration on the Quality of Swedish Lager Beers. USA): Technical quarterly-Master Brewers Association of the Americas.
Heinz, V., Alvarez, I., Angersbach, A., and Knorr, D. (2001). Preservation of Liquid Foods by High Intensity Pulsed Electric fields: Basic Concepts for Process Design. Trends Food Sci. Tech. 12 (3), 103–111. doi:10.1016/s0924-2244(01)00064-4
Hosseini, S., Azar-Daryany, M., Massudi, R., and Elikaei, A. (2011). Pulsed UV Laser Light on Escherichia coli and Saccharomyces cerevisiae Suspended in Non-alcoholic Beer. Iran J. Microbiol. 3 (1), 31–35.
Houška, M., and Pravda, P. (2018). Examples of Commercial Fruit and Vegetable Juices and Smoothies Cold Pasteurized by High Pressure, in High Pressure Process. Fruit Veg. Prod.. Editor M. Houška, and F. V. M. Silva (Cambridge, UK: The Royal Society of Chemistry Paperbacks). Internet site https://www.hiperbaric.com/en/hpp-technology/equipment/hpp-in-bulk/, consulted on 28/9/2021.
IChemE (2016). IChemE Awards 2016 Finalist - ‘Technologies to Pasteurise Beer', University of Auckland and DB Breweries. NZ. Youtube video.
Hiperbaric (20212003). “HPP In-Bulk: Large Productions of Bulk Liquids before Bottling,” in A History of Beer and Brewing. Editor I. Hornsey (Cambridge, UK: The Royal Society of Chemistry Paperbacks). Internet site https://www.hiperbaric.com/en/hpp-technology/equipment/hpp-in-bulk/, consulted on 28/9/2021.
Ishikawa, H., Shimoda, M., Shiratsuchi, H., and Osajima, Y. (1995). Sterilization of Microorganisms by the Supercritical Carbon Dioxide Micro-bubble Method. Biosci. Biotechnol. Biochem. 59 (10), 1949–1950. doi:10.1271/bbb.59.1949
Ishikawa, H., Shimoda, M., Tamaya, K., Yonekura, A., Kawano, T., and Osajima, Y. (1997). Inactivation ofBacillusSpores by the Supercritical Carbon Dioxide Micro-bubble Method. Biosci. Biotechnol. Biochem. 61 (6), 1022–1023. doi:10.1271/bbb.61.1022
Jayaram, S., Castle, G. S. P., and Margaritis, A. (1992). Kinetics of Sterilization ofLactobacillus Brevis Cells by the Application of High Voltage Pulses. Biotechnol. Bioeng. 40 (11), 1412–1420. doi:10.1002/bit.260401116
Kincal, D., Hill, W. S., Balaban, M. O., Portier, K. M., Wei, C. I., and Marshall, M. R. (2005). A Continuous High Pressure Carbon Dioxide System for Microbial Reduction in Orange Juice. J. Food Sci. 70 (5), M249–M254. doi:10.1111/j.1365-2621.2005.tb09979.x
Koutchma, T. (2019). Ultraviolet Light in Food Technology: Principles and Applications, Vol. 2. CRC Press.
Lawrence, D. R. (1988). “Spoilage Organisms in Beer,” in Developments in Food Microbiology. Editor R. K. Robinson (London: Elsevier), 3, 1–48. doi:10.1007/978-1-4613-1085-3_1
Lima, G. P. P., Lopes, T. d. V. C., Rossetto, M. R. M., and Vianello, F. (2009). Nutritional Composition, Phenolic Compounds, Nitrate Content in Eatable Vegetables Obtained by Conventional and Certified Organic Grown Culture Subject to thermal Treatment. Int. J. Food Sci. Tech. 44, 1118–1124. doi:10.1111/j.1365-2621.2009.01928.x
Lin, H. M., Chan, E. C., Chen, C., and Chen, L. F. (1991). Disintegration of Yeast Cells by Pressurized Carbon Dioxide. Biotechnol. Prog. 7, 201–204. doi:10.1021/bp00009a001
Linton, M., and Patterson, M. F. (2000). High Pressure Processing of Foods for Microbiological Safety and Quality (A Short Review). Acta Microbiol. Immunol. Hung 47 (2–3), 175–182. doi:10.1556/AMicr.47.2000.2-3.3
Lu, G., Li, C., Liu, P., Cui, H., Yao, Y., and Zhang, Q. (2010). UV Inactivation of Microorganisms in Beer by a Novel Thin-Film Apparatus. Food Control 21 (10), 1312–1317. doi:10.1016/j.foodcont.2010.03.007
Lund, M. N., and Andersen, M. L. (2011). Detection of Thiol Groups in Beer and Their Correlation with Oxidative Stability. J. Am. Soc. Brewing Chemists 69, 163–169. doi:10.1094/asbcj-2011-0620-01
Luo, H., Schmid, F., Grbin, P. R., and Jiranek, V. (2012). Viability of Common Wine Spoilage Organisms after Exposure to High Power Ultrasonics. Ultrason. Sonochem. 19 (3), 415–420. doi:10.1016/j.ultsonch.2011.06.009
Marsili, R. T., Laskonis, L. C., and Kenaan, C. (2007). Evaluation of PDMS-Based Extraction Techniques and GC-TOFMS for the Analysis of Off-Flavor Chemicals in Beer. J. Am. Soc. Brewing Chemists 65 (3), 129–137. doi:10.1094/asbcj-2007-0617-01
Mason, T. J., Paniwnyk, L., and Lorimer, J. P. (1996). The Use of Ultrasound in Food Technology. Ultrason. Sonochem. 3, 253–260. doi:10.1016/s1350-4177(96)00034-x
Matak, K. E., Churey, J. J., Worobo, R. W., Sumner, S. S., Hovingh, E., Hackney, C. R., et al. (2005). Efficacy of UV Light for the Reduction of Listeria Monocytogenes in Goat's Milk. J. Food Prot. 68, 2212–2216. doi:10.4315/0362-028x-68.10.2212
Mezui, A. M., and Swart, P. (2010). Effect of UV-C Disinfection of Beer - Sensory Analyses and Consumer Ranking. J. Inst. Brewing 116 (4), 348–353. doi:10.1002/j.2050-0416.2010.tb00785.x
Milani, E. A., Alkhafaji, S., and Silva, F. V. M. (2015a). Pulsed Electric Fields Continuous Pasteurization of Beer. Food Control 50, 223–229. doi:10.1007/978-3-319-32886-7_132
Milani, E. A., Gardner, R., and Silva, F. V. M. (2015b). Thermal resistance of Saccharomyces yeast ascospores in beers. Int. J. Food Microbiol. 206, 75–80. doi:10.1016/j.foodcont.2014.08.033
Milani, E. A. (2016). Nonthermal Pasteurization Of Beer. Doctoral Dissertation. Chemical and Materials Engineering Department, University of Auckland.
Milani, E. A., Ramsey, J. G., and Silva, F. V. M. (2016). High Pressure Processing and Thermosonication of Beer: Comparing the Energy Requirements and Saccharomyces cerevisiae Ascospores Inactivation with thermal Processing and Modeling. J. Food Eng. 181, 35–41. doi:10.1016/j.jfoodeng.2016.02.023
Milani, E. A., and Silva, F. V. M. (2016). Nonthermal Pasteurization of Beer by High Pressure Processing: Modelling the Inactivation of Saccharomyces cerevisiae Ascospores in Different Alcohol Beers. High Press. Res. 36 (4), 595–609. doi:10.1080/08957959.2016.1190354
Milani, E. A., and Silva, F. V. M. (2017). Ultrasound Assisted thermal Pasteurization of Beers with Different Alcohol Levels: Inactivation of Saccharomyces cerevisiae Ascospores. J. Food Eng. 198, 45–53. doi:10.1016/j.jfoodeng.2016.11.015
Mirsky, S. (2007). Ale's Well with the World. Sci. Am. 296 (5), 102. doi:10.1038/scientificamerican0507-102
Mújica-Paz, H., Valdez-Fragoso, A., Samson, C. T., Welti-Chanes, J., and Torres, A. (2011). High-pressure Processing Technologies for the Pasteurization and Sterilization of Foods. Food Bioproc. Tech. 4 (6), 969–985.
Nakamura, K., Enomoto, A., Fukushima, H., Nagai, K., and Hakoda, M. (1994). Disruption of Microbial Cells by the Flash Discharge of High-Pressure Carbon Dioxide. Biosci. Biotechnol. Biochem. 58 (7), 1297–1301. doi:10.1271/bbb.58.1297
Nelson, M. (2014). “The Geography of Beer in Europe from 1000 BC to AD 1000,” in The Geography of Beer. Editors M. Patterson, and N. Hoalst-Pullen (Netherlands: Springer), 9–21. doi:10.1007/978-94-007-7787-3_2
Nunes, C., Santos, M. C., Saraiva, J. A., Rocha, S. M., and Coimbra, M. A. (2017). Influence of High Hydrostatic Pressure Technology on Wine Chemical and Sensorial Characteristics. Adv. Food Nutr. Res. 82, 205–235. doi:10.1016/bs.afnr.2017.01.003
Oziembłowski, M., Dróżdż, M., Kiełbasa, P., Dróżdż, T., Gliniak, M., Nawara, P., et al. (20172017). Progress in Applied Electrical Engineering (PAEE). IEEE, 1–4.June). Impact of Pulsed Electric Field on the Quality of Unpasteurized Beer
Palou, E., López-Malo, A., Barbosa-cánovas, G. V., Welti-Chanes, J., and Swanson, B. G. (1998). Oscillatory High Hydrostatic Pressure Inactivation of Zygosaccharomyces bailii. Journal. Food Prot. 61, 1213–1215. doi:10.4315/0362-028x-61.9.1213
Pérez‐Lamela, C., Ledward, D. A., Reed, R. J. R., and Simal‐Gándara, J. (2002). Application of High‐pressure Treatment in the Mashing of white Malt in the Elaboration Process of Beer. J. Sci. Food Agric. 82 (3), 258–262.
Portno, A. D. (1968). Pasteurization and Sterilization of Beer-A Review*. J. Inst. Brewing 74 (3), 291–300. doi:10.1002/j.2050-0416.1968.tb03129.x
Priest, F. G. (2003). “Gram-positive Brewery Bacteria,” in Brewing Microbiology. Editors F. G. Priest, and I. Campbell (USA: Springer), 181–217. doi:10.1007/978-1-4419-9250-5_5
Priest, F. G. (2006). “Microbiology and Microbiological Control in the Brewery,” in Handbook of Brewing (CRC Press), 622–643. doi:10.1201/9781420015171.ch16
Priest, F. G., and Stewart, G. G. (2006). “Handbook of Brewing,” in Food Science and Technology. Editors F. G. Priest, and G. G. Stewart (USA: CRC Press), 157.
Puig, A., Olmos, P., Quevedo, J. M., Guamis, B., and Mínguez, S. (2008). Microbiological and Sensory Effects of Musts Treated by High-Pressure Homogenization. Food Sci. Technol. Int. 14 (5_Suppl. l), 5–11. doi:10.1177/1082013208094579
Quintero-Ramos, A., Churey, J. J., Hartman, P., Barnard, J., and Worobo, R. W. (2004). Modeling of Escherichia coli Inactivation by UV Irradiation at Different pH Values in Apple Cider. J. Food Prot. 67 (6), 1153–1156. doi:10.4315/0362-028x-67.6.1153
Reveron, I. M., Barreiro, J. A., and Sandoval, A. J. (2005). Thermal Death Characteristics of Lactobacillus Paracasei and Aspergillus niger in Pilsen Beer. J. Food Eng. 66 (2), 239–243. doi:10.1016/j.jfoodeng.2004.03.014
Reveron, I. M., Barreiro, J. A., and Sandoval, A. J. (2003). Thermal Resistance of Saccharomyces cerevisiae in Pilsen Beer. J. Inst. Brewing 109 (2), 120–123. doi:10.1002/j.2050-0416.2003.tb00140.x
Rozali, S. N. M., Milani, E. A., Deed, R. C., and Silva, F. V. M. (2017). Bacteria, Mould and Yeast Spore Inactivation Studies by Scanning Electron Microscope. Int. J. Food Microbiol. 263, 17–25. doi:10.1016/j.ijfoodmicro.2017.10.008
Sale, A. J. H., and Hamilton, W. A. (1968). Effects of high electric fields on microorganisms: Killing of bacteria and yeasts. Biochimica et Biophysica Acta (BBA)-General Subjects 148 (3), 781–788.
Silva, F. V. M., and Gibbs, P. A. (2009). “Principles of thermal Processing: Pasteurization,” in Engineering Aspects of Thermal Food Processing. Editor R. Simpson (USA: CRC Press), 13–49.
Silva, F. V. M., Tan, E. K., and Farid, M. (2012). Bacterial Spore Inactivation at 45-65 °C Using High Pressure Processing: Study of Alicyclobacillus acidoterrestris in orange Juice. Food Microbiol. 32, 206–211. doi:10.1016/j.fm.2012.04.019
Silva, F. V. M., Gibbs, P. A., Nuñez, H., Almonacid, S., and Simpson, R. (2014). “THERMAL PROCESSES | Pasteurization,” in Encyclopedia of Food Microbiology. Editors C. A. Batt, and M. L. Tortorello. 2nd. ed. (Amsterdam: Elsevier), 577–595. doi:10.1016/b978-0-12-384730-0.00404-3
Silva, F. V. M., Milani, E. A., and Carr, K. (2015). Beer Pasteurized by Pulsed Electric Fields, High Pressure Processing, and Power Ultrasound: Taste Assessments, Poster Presented at 12th International Congress of Engineering and Food (ICEF). Quebec: Canada.
Silva, F. V. M., and Evelyn, (2018). “High Pressure Processing Effect on Microorganisms in Fruit and Vegetable Products,” in High Pressure Processing of Fruit and Vegetable Juices. Editors M. Houška, and F. V. M. Silva (CRC Press), 3–37. (Chapter 2).
Shimoda, M., Yamamoto, Y., Cocunubo-Castellanos, J., Tonoike, H., Kawano, T., Ishikawa, H., et al. (1998). Antimicrobial Effects of Pressured Carbon Dioxide in a Continuous Flow System. J. Food Sci. 63, 709–712.
Statista (2020). Beer Production Worldwide from 1998 to 2019. Available at: https://www.statista.com/statistics/270275/worldwide-beer-production/#:∼:text=In%202018%2C%20the%20global%20beer,the%20United%20States%20and%20Brazil.
Štulíková, K., Bulíř, T., Nešpor, J., Jelínek, L., Karabín, M., and Dostálek, P. (2020). Application of High Pressure Processing to Assure the Storage Stability of Unfiltered Lager Beer. Molecules 25 (10), 2414.
Sulaiman, A., Soo, M. J., Farid, M., and Silva, F. V. M. (2015). Thermosonication for Polyphenoloxidase Inactivation in Fruits: Modeling the Ultrasound and thermal Kinetics in Pear, Apple and Strawberry Purees at Different Temperatures. J. Food Eng. 165, 133–140. doi:10.1016/j.jfoodeng.2015.06.020
Sulaiman, A., Farid, M., and Silva, F. V. M. (2017). Quality Stability and Sensory Attributes of Apple Juice Processed by Thermosonication, Pulsed Electric Field and thermal Processing. Food Sci. Tech. Int. 23 (3), 265–276. doi:10.1016/j.jfoodeng.2015.06.020
Suslick, K. S., and Nyborg, W. L. (1990). Ultrasound: Its Chemical, Physical and Biological Effects.
Suzuki, K. (2011). 125th Anniversary Review: Microbiological Instability of Beer Caused by Spoilage Bacteria. J. Inst. Brewing 117 (2), 131–155. doi:10.1002/j.2050-0416.2011.tb00454.x
Toepfl, S., Heinz, V., and Knorr, D. (2006). “Application of Pulsed Electric Fields in Liquid Processing,” in Processing Developments for Liquids (Kolonia: EFFoST).
Tsang, E., and Ingledew, W. (1982). Studies on the Heat Resistance of Wild Yeasts and Bacteria in Beer. J. Am. Soc. Brewing Chemists 40. doi:10.1094/asbcj-40-0001
Uchida, R., and Silva, F. V. M. (2017). Alicyclobacillus acidoterrestris Spore Inactivation by High Pressure Combined with Mild Heat: Modeling the Effects of Temperature and Soluble Solids. Food Control 73 (B), 426–432. doi:10.1016/j.foodcont.2016.08.034
Ugarte, E., Feng, H., Martin, S. E., and Cadwallader, K. R. (2006). Inactivation of Escherichia coli with Power Ultrasound in Apple Cider. J. Food Sci. 71 (2), 102–108. doi:10.1111/j.1365-2621.2006.tb08890.x
Ulmer, H. M., Heinz, V., Ganzle, M. G., Knorr, D., and Vogel, R. F. (2002). Effects of Pulsed Electric Fields on Inactivation and Metabolic Activity of Lactobacillus plantarum in Model Beer. J. Appl. Microbiol. 93 (2), 326–335. doi:10.1046/j.1365-2672.2002.01699.x
van Wyk, S., and Silva, F. V. M. (2017a). High Pressure Inactivation of Brettanomyces bruxellensis in Red Wine. Food Microbiol. 63, 199–204. doi:10.1016/j.fm.2016.11.020
van Wyk, S., and Silva, F. V. M. (2017b). High Pressure Processing Inactivation of Brettanomyces bruxellensis in Seven Different Table Wines. Food Control 81, 1–8. doi:10.1016/j.foodcont.2017.05.028
van Wyk, S., Farid, M. M., and Silva, F. V. M. (2018). SO2, High Pressure Processing and Pulsed Electric Field Treatments of Red Wine: Effect on Sensory, Brettanomyces Inactivation and Other Quality Parameters during One Year Storage. Innovative Food Sci. Emerging Tech. 48, 204–211. doi:10.1016/j.ifset.2018.06.016
van Wyk, S., and Silva, F. V. M. (2019a). “Nonthermal Preservation of Wine,”. Chapter 7 in: in Preservatives and Preservation Methods for the Beverage Industry. The Science of Beverages Series (Elsevier), 15, 203–235.
van Wyk, S., Silva, F. V. M., and Farid, M. (2019b). Pulsed Electric Field Treatment of Red Wine: Inactivation of Brettanomyces and Potential hazard Caused by Metal Ion Dissolution. Innovative Food Sci. Emerging Tech. 52, 57–65. doi:10.1016/b978-0-12-816685-7.00007-0
Vanderhaegen, B., Neven, H., Coghe, S., Verstrepen, K. J., Verachtert, H., and Derdelinckx, G. (2003). Evolution of Chemical and Sensory Properties during Aging of Top-Fermented Beer. J. Agric. Food Chem. 51 (23), 6782–6790. doi:10.1021/jf034631z
Vanderhaegen, B., Neven, H., Verachtert, H., and Derdelinckx, G. (2006). The Chemistry of Beer Aging - a Critical Review. Food Chem. 95, 357–381. doi:10.1016/j.foodchem.2005.01.006
Varnam, A., and Sutherland, J. M. (1994). Beverages: Technology, Chemistry and Microbiology, 2. USA: Springer Science & Business Media.
Vecchio, H. W. D., Dayharsh, C. A., and Baselt, F. C. (1951). Thermal Death Time Studies on Beer Spoilage Organisms-I. Proc. Annu. Meet. - Am. Soc. Brewing Chemists 9, 45–50. doi:10.1080/00960845.1951.12006391
Vega-Mercado, H., Martín-Belloso, O., Qin, B.-L., Chang, F. J., Marcela Góngora-Nieto, M., Barbosa-Cánovas, G. V., et al. (1997). Non-thermal Food Preservation: Pulsed Electric fields. Trends Food Sci. Tech. 8 (5), 151–157. doi:10.1016/s0924-2244(97)01016-9
Walkling-Ribeiro, M., Rodríguez-González, O., Jayaram, S. H., and Griffiths, M. W. (2011). Processing Temperature, Alcohol and Carbonation Levels, and Their Impact on Pulsed Electric Fields (PEF) Mitigation of Selected Characteristic Microorganisms in Beer. Food Res. Int. 44 (8), 2524–2533.
Wouters, P. C., and Smelt, J. P. P. M. (1997). Inactivation of Microorganisms with Pulsed Electric fields: Potential for Food Preservation. Food Biotechnol. 11 (3), 193–229. doi:10.1080/08905439709549933
Wray, E. (2015). “Reducing Microbial Spoilage of Beer Using Pasteurisation,” in Brewing Microbiology (Woodhead Publishing), 253–269. doi:10.1016/b978-1-78242-331-7.00012-5
Xiao, Y., Piao, M., and Deng, Y. (2020). Effect of Thermosonication Processing on the Physicochemical and Sensorial Properties of Cloudy Wheat Beer. Shipin Kexue/Food Sci. 41 (11), 77–82.
Yin, H., Dong, J., Yu, J., Chang, Z., Qian, Z., Liu, M., et al. (2016). A Preliminary Study about the Influence of High Hydrostatic Pressure Processing on the Physicochemical and Sensorial Properties of a Cloudy Wheat Beer. J. Inst. Brew. 122 (3), 462–467. doi:10.1002/jib.344
Keywords: HPP, high pressure processing, PEF, pulsed electical field, ultrasound, quality, microbial inactivation, yeast
Citation: Milani EA and Silva FV (2022) Pasteurization of Beer by Non-Thermal Technologies. Front. Food. Sci. Technol. 1:798676. doi: 10.3389/frfst.2021.798676
Received: 20 October 2021; Accepted: 10 December 2021;
Published: 23 March 2022.
Edited by:
Guibing Chen, North Carolina Agricultural and Technical State University, United StatesReviewed by:
Sevcan Unluturk, Izmir Institute of Technology, TurkeyHafiz Shahbaz, University of Veterinary and Animal Sciences, Pakistan
Copyright © 2022 Milani and Silva. This is an open-access article distributed under the terms of the Creative Commons Attribution License (CC BY). The use, distribution or reproduction in other forums is permitted, provided the original author(s) and the copyright owner(s) are credited and that the original publication in this journal is cited, in accordance with accepted academic practice. No use, distribution or reproduction is permitted which does not comply with these terms.
*Correspondence: Filipa V.M. Silva, ZnZzaWx2YUBpc2EudWxpc2JvYS5wdA==, ZmlsaXBhdmluYWdyZXNpbHZhQGdtYWlsLmNvbQ==