- 1Department of Food Science and Human Nutrition, Iowa State University, Ames, IA, United States
- 2Interdepartmental Microbiology Graduate Program, Iowa State University, Ames, IA, United States
- 3School of Agriculture, University of Wisconsin-Platteville, Platteville, WI, United States
- 4Department of Food Science, Nutrition and Health Promotion, Mississippi State University, Starkville, MS, United States
The main objective of the present study was to determine the efficacy of dielectric barrier discharge (DBD) high voltage atmospheric cold plasma (HVACP) at 70 kV for inactivating Salmonella enterica and shiga-toxin-producing Escherichia coli (STEC) on desiccated shredded coconut. Additional objectives were to evaluate sub-lethal injury in pathogen survivors, and selected quality characteristics of the coconut as affected by HVACP. Shredded coconut inoculated with ∼7.74 log10 CFU/g S. enterica or STEC, was exposed to HVACP (70 kV) for 3–15 min (S. enterica) and up to 5 min (E. coli). Non-HVACP-treated, inoculated coconut served as control. Samples were held at 23 ± 1°C for 24 h after HVACP treatment, and analyzed for survivors via the plate count method using thin agar layer (TAL) media and selective (SEL) agar. For each pathogen, differences in numbers of survivors on TAL medium and selective agar were used to evaluate sub-lethal injury. Color and texture of the coconut were determined using a ColorFlex EZ Spectrophotometer and a TA. XT Plus C Texture Analyzer, respectively. HVACP treatments significantly decreased the initial numbers of viable S. enterica and STEC (p < 0.05) with more survivors consistently recovered on TAL media compared to selective agar. Reductions (log10 CFU/g) of STEC and S. enterica after 3 min of HVACP treatment were 1.97 and 1.56, respectively (p < 0.05) based on survivors on TAL medium. A greater extent of sub-lethal injury was observed in STEC survivors compared to S. enterica (p < 0.05). The TAL method allowed detection of sub-lethally injured pathogens in HVACP-treated coconut that would have otherwise remained undetected if only selective agar media were used. There were minimal changes in color and texture of the coconut after HVACP (70 kV) treatment for 12 min. Based on these results, HVACP (70 kV) has good potential for inactivating enteric pathogens in desiccated shredded coconut to improve the microbial safety of this popular food ingredient with minimal effect on product color and texture.
Introduction
Coconut (Cocos nucifera L.) belongs to the family Palmae, and is native to the tropical and sub-tropical regions of the world. It is grown in over 90 countries with the largest coconut producers being Indonesia, Philippines, India, Sri Lanka and Brazil (FAO, 2019; FAOSTAT, 2020). Among the various coconut products, desiccated coconut is the second most commonly traded product accounting for 200 million tons produced globally (FAO, 1999). Desiccated coconut is prepared by drying the shredded or ground coconut flesh, commonly referred to as coconut meat, after removing it from the brown testa (Sangamithra et al., 2013). This dried product is widely used as an ingredient in a vast array of confectionery and bakery products (Lamdande et al., 2018). Desiccated coconut is in the dried fruits and vegetables subcategory of high risk low water activity (aW) food products. That categorization was based on collation and analysis of microbiological hazards of low aW foods that ultimately resulted in the ranking of those foods of greatest concern in terms of microbial food safety (FAO, 2014). In this regard, desiccated coconut is viewed by the World Health Organization (WHO) as a major microbial food safety concern (WHO, 2022).
Currently used methods to control microbial contaminants on coconut meat include heat pasteurization at 80°C–90°C in 2% sodium metabisulphite solution for 20 min, passage through boiling water, or exposure to 80°C for 5 minutes via steaming in stainless steel blanchers. The pieces of coconut meat are then shredded and dehydrated in a two-phase process in hot-air driers (Tetra Pak, 2016) to achieve a moisture content of about 4.0% (Codex Alimentarius Commission, 1994). Dhowlaghar et al. (2021) observed that thermal resistance of Salmonella increased with decreasing aW of the coconut. Therefore, in such situations, additional heating is crucial to eliminate the pathogen. This in turn would likely limit the marketability of the shredded coconut due to reduced quality attributes particularly product color and texture, as this product is largely used as an ingredient of other food products.
The presence of pathogens, most commonly Salmonella, molds, and sulfur dioxide in desiccated shredded coconut are major food safety issues (Manley, 2011; Kumar Mahnot et al., 2019; Dhowlaghar et al., 2021). In low aW foods, Salmonella is a major pathogen of concern. Although Salmonella will not grow at aW <0.94, it can survive for extended periods in low aW foods including dried coconut (Podolack et al., 2010; Nascimento et al., 2018; Liu et al., 2022). In the United States, Salmonella was implicated in multiple outbreaks involving dried and frozen shredded coconut (CDC, 2018a; CDC, 2018b; Luna et al., 2018), and in recalls of shredded coconut and coconut products (CFIA, 2018; FDA, 2019). Although to date there are no reported outbreaks or recalls of desiccated shredded coconut due to shiga toxin-producing Escherichia coli (STEC), fresh coconut can support the growth of enteric bacteria (Escudero and Schaffner, 1981). Moreover, the complexities of handling involved in preparing coconut for shredding and drying, along with the low acidity of that product make it susceptible to microbial contamination.
Over the past few decades, research on developing non-thermal technologies such as ultraviolet radiation, high hydrostatic pressure, and pulsed electrical fields for control of foodborne pathogenic microorganisms has increased (Los et al., 2018; Chacha et al., 2021; Jadhav et al., 2021). For instance, Gabriel et al. (2016) reported on the application of UV-C radiation for microbial decontamination of desiccated shredded coconut. Apart from UV-C radiation, published reports on the application of other non-thermal methods to shredded coconut meat are scarce.
Another emerging non-thermal technology is atmospheric cold plasma (ACP) (Bourke et al., 2017). Plasma is an ionized gas consisting of ions, atoms, free radicals, free electrons, and molecules in their basic or excited states. ACP is a partly ionized gas state maintained at room temperature at atmospheric pressure, with ionization of about 5% or less of the gas (Ekezie et al., 2017). ACP technology provides several advantages in food industry including short processing times (from a few seconds to minutes), effectiveness at room temperature which is desirable for heat-labile food products and ingredients, and low-energy requirement (Kim et al., 2014). ACP has effectively inactivated microorganisms in a variety of food products including fruits and vegetables (Misra et al., 2014; Ziuzina et al., 2014; Won et al., 2016; Molina-Hernandez et al., 2022), whole black pepper (Hertwig et al., 2015), tender coconut water (Kumar Mahnot et al., 2019), wheat grains (Thomas-Popo et al., 2019), nuts (Basaran et al., 2008), meat (Jayasena et al., 2015), rice cereal bars (Suhem et al., 2013) and poultry meat (Noriega et al., 2011; Moutiq et al., 2020).
Microorganisms may be in a sub-lethally injured state in food products that are subjected to thermal or non-thermal preservation methods. If sub-lethally injured pathogens are not detected, they may later repair their lesions, multiply, and regain their pathogenicity in foods (Wesche et al., 2009). The commonly used method for evaluating bacterial sub-lethal injury after application of antimicrobial treatments involve plating treated samples on both non-selective and selective agar media (Ray 1989; Busch and Donnelly, 1992; Wesche et al., 2009; Espina et al., 2016). While selective agents in selective media can suppress growth of non-target organisms, they may inhibit resuscitation and detection of sub-lethally injured pathogens (Wesche et al., 2009). In this respect the difference in colony counts of bacteria on non-selective and selective media is used in calculating the percent sub-lethal injury in the surviving population.
While there is a growing body of information on the application ACP for microbial control in foods, there are no published research reports on the fate of human enteric pathogens on shredded coconut after treatment with high voltage ACP (HVACP). Accordingly, the primary objective of the present study was to determine the efficacy of dielectric barrier discharge (DBD) HVACP at 70 kV for killing S. enterica and STEC on dried shredded coconut. Secondary objectives were to evaluate extent of sub-lethal injury in pathogen survivors, and the effect of HVACP on selected quality characteristics of shredded coconut.
Materials and methods
Bacterial strains and culture conditions
Five serovars of Salmonella enterica (Enteritidis-ATCC13076, Typhimurium ATCC 14028, Gaminara ATCC 8324, Newport ATCC 6962, and Thompson BAA-3141) and shiga-toxin producing Escherichia coli (STEC) [O121, and O157 (ATCC 35150, ATCC 43894, ATCC 43895, FRIK 125)] were obtained from the culture collection of the Microbial Food Safety Laboratory, Iowa State University, Ames, IA. The frozen stock cultures (−80°C) in brain heart infusion (BHI) broth (Difco; Becton Dickinson, Sparks, MD) with 10% (v/v) added glycerol were thawed under cold running water, then activated in tryptic soy broth supplemented with 0.6% yeast extract (TSBYE; pH 7.2; Difco) at 35°C. Working cultures were held at 4°C for use in the experiments. For each experiment, two consecutive 24-h transfers of each working culture in TSBYE (35°C) were performed to prepare bacterial cells for the inoculation of the shredded coconut.
Preparation of inoculum
Six milliliters of each of the five working cultures of S. enterica or STEC were separately combined in a sterile 50 mL centrifuge tubes. The cells were harvested by centrifugation (10,000 x g, 10 min, 4°C) using a Sorvall Super T21 centrifuge (American Laboratory Trading, Inc., East Lyme, CT). The supernatants were discarded and the pelleted cells were suspended and washed once in sterile 0.85% (w/v) NaCl (saline). The washed cells were harvested by centrifugation as previously described and re-suspended in 30 mL of sterile saline to obtain a viable cell concentration of ∼9.0 log10 colony forming units (CFU)/mL. The five-strain cell suspension for each pathogen was aseptically transferred to a separate sterile, appropriately labeled, calibrated spray bottle. The numbers of viable cells in each cell suspension were determined by serial dilution (10-fold) and surface plating on TSAYE, xylose lysine tergitol 4 (XLT 4) agar (for Salmonella) or sorbitol MacConkey agar (SMAC) for STEC, and thin agar layer (TAL) medium (Kang and Fung, 2000). The TAL medium was prepared by overlaying 14 mL of molten TSAYE (48°C) onto solidified selective agar (XLT-4 or SMAC). Bacterial colonies were enumerated after aerobic incubation (35°C) of TSAYE and TAL plates for 24 h and selective agar plates (XLT-4 and SMAC) for 48 h.
Preparation and inoculation of shredded coconut
Packages of desiccated shredded coconut with no added preservatives and from the same production lot (Bob’s Red Mill Shredded Coconut) were purchased from a local store in Ames, IA. Based on results of tests for sulfite using HPLC, that brand of coconut contained no added sulfite, which is commonly used as a processing aid in the production of shredded coconut. A package of shredded coconut was aseptically emptied into a large sterile beaker, and via use of a sterile spatula, the product was manually mixed. Portions (25-g) of shredded coconut were aseptically weighed and placed into separate sterile Petri dishes (150 mm × 15 mm). Each Petri dish was gently shaken to distribute the shreds in a monolayer. Samples (25-g) were each mist-inoculated with the 5-strain cell suspension of S. enterica or STEC using a sterile calibrated spray bottle. The spray bottle dispensed ∼1.40 mL of cell suspension via two sprays applied to shredded coconut to obtain ∼5.6 × 107 CFU of the respective pathogen per gram of sample. The inoculation was performed in a large biohazard bag in a BSL2 laminar flow biosafety cabinet. The Petri dishes containing the inoculated coconut were placed on ethanol (100%) soaked paper towels on sheets of aluminum foil. The outer sides of the Petri dishes were disinfected using a paper towel soaked with ethanol (100%). To facilitate drying of the inoculum and attachment of the pathogens to the coconut shreds, the inoculated samples were held at ambient temperature (22°C ± 1°C) for 1 h in the biosafety cabinet with the blower off.
Water activity of shredded coconut
The water activity (aW) of non-inoculated shredded coconut was measured using an AquaLab Series 3 water activity meter (Decagon Devices, Inc., Pullman, WA). Separate sets of samples were sprayed with sterile water to simulate inoculation, and the aW was measured just after spraying and after air drying at 22°C ± 1°C for 1 h in the laminar flow hood.
Application of high voltage atmospheric cold plasma
A dielectric barrier discharge (DBD) system designed for in-package plasma treatment (Yepez and Keener, 2016) was used in the present study. Figure 1 shows a schematic of that system. The voltage (70 kV) was produced by a step-up transformer (Phenix Technologies, MD, United States) with a voltage output of 0–130 kV at a frequency of 60 Hz. The gap (discharge distance) between the two circular aluminum disk electrodes was 41 mm, representing the height of the polypropylene box. Each aluminum electrode had an outer diameter of 152 mm. Plexiglass (10 mm thick) and 4 mm thick polypropylene sheets served as dielectric barriers. These barriers were used to prevent arc transitions and facilitate the homogeneity of the plasma during HVACP treatment of the coconut samples.
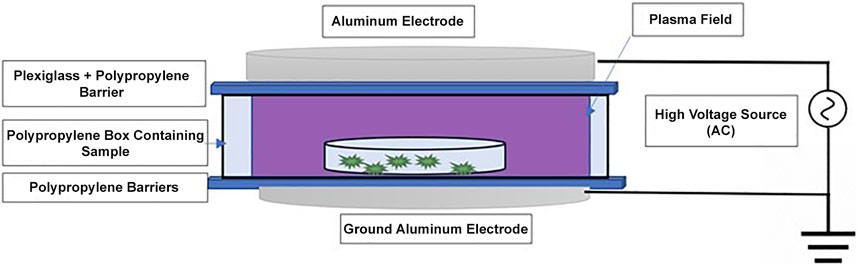
FIGURE 1. Schematic of the experimental set-up for atmospheric cold plasma treatment of desiccated shredded coconut.
The Petri dishes with inoculated shredded coconut were placed in 27.6 cm × 7.25 cm x 4.1 cm polypropylene boxes with lids. The boxes (lids closed) were placed in 29.2 cm × 33.0 cm high-barrier polypropylene Cryovac bags (Cryovac Sealed Air Corp., Duncan, SC, United States) filled with atmospheric air at atmospheric pressure. The temperature and the relative humidity were recorded for each experiment. The bags containing the boxes of shredded coconut were heat sealed and placed between the dielectric barriers separating the aluminum electrodes connected to the transformer. The samples were exposed to direct HVACP (70 kV) in atmospheric air at atmospheric pressure for pre-determined time intervals (minutes). Inoculated control samples were similarly packaged but received no HVACP treatment. Control and HVACP-treated samples were held at ambient temperature (22°C ± 1°C) for 24 h before they were analyzed for survivors.
Microbiological analysis
To analyze non-inoculated shredded coconut for naturally occurring Salmonella and E. coli, 25-g portions of that product were homogenized with 75-mL 0.1% (w/v) peptone. Aliquots (1.0 or 0.1-mL) of the homogenate were surface plated on selective agar (XLT-4 and SMAC) and on non-selective TSAYE. The inoculated agar plates were incubated at 35°C and bacterial colonies were enumerated after 48 h. Universal pre-enrichment broth (225 mL) was added to the remaining homogenate, and the mixture was incubated at 35°C. At 24 h and 48 h after incubation the enriched homogenate was streak plated onto XLT-4 and SMAC agar.
Bags containing control and HVACP-treated shredded coconut were cut open to remove the samples from the polypropylene boxes. The samples were aseptically transferred to sterile Seward™ Stomacher™ Lab Blender bags (Fisher Scientific, Fair Lawn, NJ). Seventy-5 mL of 0.1% (w/v) peptone (diluent) were added to each 25-g sample. Each mixture of coconut and diluent were homogenized for 1 min in a laboratory stomacher blender (Stomacher 400 Circulator, Steward, England) operating at medium speed (230 rpm). Ten-fold serial dilutions of the homogenate were prepared in 0.1% (w/v) peptone. Aliquots of diluted homogenate were surface plated in duplicate on selective agar (XLT 4 or SMAC) and Thin Agar Layer (TAL) recovery media to enumerate non-injured and sub-lethally injured pathogens. Bacterial colonies were enumerated after aerobic incubation (35°C) for 24 h (TAL media) or 48 h (selective agar).
Determination of sub-lethal injury
Based on bacterial colonies on selective agar (SMAC or XLT-4) and TAL medium for each time of exposure to HVACP, survivor curves were prepared. For each type of agar medium, the reduction in viability of each pathogen, termed the reduction factor (RF), was determined. The RF is the ratio of the CFU/g of non-treated (control) sample to the CFU/g of the HVACP-treated sample. For each type of agar medium, the logarithm of the RF was used to determine reduction in pathogen viability after each exposure time via use of the following equation (Wuytack, et al., 2003):
Log RF = log[CFU/g before HVACP treatment ÷ CFU/g after HVACP treatment].
The log of RF for XLT-4 or SMAC was plotted on the y-axis against the log of RF for TAL agar on the x-axis. Using Microsoft Excel 4.0 spreadsheet program (Microsoft Corporation, Redmond, WA), linear regression lines were fitted through the data points and sub-lethal injury was determined when the slope and y-intercept of a regression line was significantly (p < 0.05) greater than 1.0 and 0, respectively (Wuytack, et al., 2003).
Color evaluation of shredded coconut
The effect of HVACP treatment on the color of the shredded coconut was examined. The ColorFlex EZ Spectrophotometer (Hunter Labs, Reston, VA, United States) with a D65/10° illuminant/observer combination was utilized to determine any changes color of HVACP-treated samples compared to control. Ten-gram portions of non-inoculated HVACP-treated and control shredded coconut were aseptically placed in 35 mm × 10 mm petri dishes The petri dish was then placed on the ColorFlex EZ measuring port and covered with an opaque sample cup cover. Each sample was tested in duplicate and CIE L*a*b* was used to determine changes in color.
Texture evaluation of shredded coconut
A TA. XT Plus C Texture Analyzer (Stable Micro Systems, Surrey, UK) was used to evaluate changes in texture of HVACP-treated shredded coconut compared to control. A portion (10-g) of coconut was placed on the analyzer and compressed (70% compression) in two cycles at 2 mm/sec via use of a TA-11 probe with a diameter 25 mm. Each sample was evaluated in duplicate, and the results were subjected to Texture Profile Analysis to evaluate texture changes.
Statistical analysis
Four replicate experiments were completed. Data on the average numbers of pathogen survivors were analyzed using JMP Pro statistical software version 16 (SAS Institute, Inc., Cary NC). For each pathogen, significant differences (p < 0.05) in numbers of survivors as a function of exposure time to HVACP were tested using analysis of variance (ANOVA). Tukey’s means comparison post-hoc test was performed to determine significant differences between treatments (p < 0.05). Tests were carried out at a 5% significance level.
Results and discussion
Water activity (aW) of shredded coconut
The average water activity (aW) of the non-inoculated desiccated coconut samples, which were sprayed with distilled water to simulate inoculation, was 0.52 ± 0.004 (before spraying) and 0.72 ± 0.001 (after spraying). The observed increase in aW of the coconut shreds from 0.52 to 0.72 is not surprising and is caused by absorption of moisture from the spraying with the distilled water. After air drying of the samples in a laminar flow hood at 22 ± 1 °C for 1 h, the average water activity was 0.67 ± 0.001. That lower aW indicated loss of moisture during simulated drying of the inoculum on the coconut shreds. At 24 h following HVACP (70 kV) treatment for 12 min, the average aW of samples of desiccated coconut was 0.53, which was close to the initial aW of 0.52 ± 0.004. This indicates that further loss of moisture from the coconut shreds occurred following HVACP (70 kV) treatment for 12 min, and during in-package holding of the samples for 24 h prior to microbial analysis. Based on these results, spray inoculation followed by drying of the inoculum did not significantly (p < 0.05) alter the aW of the desiccated coconut shreds to exclude this product from the category of low aW foods which have a water activity of <0.70 (Gurtler et al., 2014).
HVACP inactivation of pathogens on shredded coconut
Naturally occurring Salmonella or E. coli was not detected in the non-inoculated desiccated coconut shreds by direct plating on TAL medium or by liquid enrichment. The efficacy of direct HVACP treatment (70 kV) against S. enterica on artificially inoculated desiccated shredded coconut is shown in Figure 2. Based on colony counts on TAL medium, the viable count of S. enterica on control coconut was 6.64 ± 0.16 log10 CFU/g. This was ∼1.10 log10 CFU/g lower than the viable count of the pathogen (7.74 ± 0.02 log10 CFU/g) on the coconut shreds before drying of the inoculum at 22°C ± 1°C for 1 h. Reductions of viable counts of pathogens during drying of inoculated surfaces have been reported. For example, the initial viable count of S. enterica (7.22 log10 CFU) and E. coli O157:H7 (7.21 log10 CFU) on the surface of whole tomatoes was reduced by 1.37 and 1.07 log, respectively, after1 h of air drying at ambient temperature (Lang et al., 2004). These results and those of the present study suggest that substantial numbers of pathogens lose viability during drying of the inoculum. In the present study, it is likely that only cells that were less stressed or sub-lethally injured by desiccation survived the drying process.
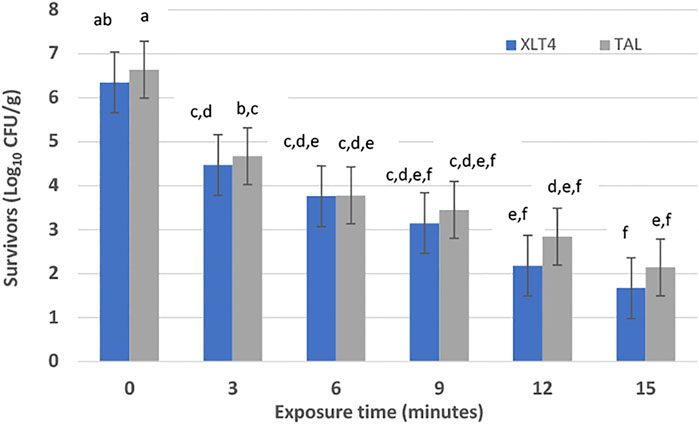
FIGURE 2. Salmonella enterica survivors on desiccated shredded coconut following high voltage atmospheric cold plasma (HVACP) treatment at 70 kV. Numbers of survivors are means from four replicate experiments. Error bars represent standard error of the mean. Bars that do not have the same letter(s) are significantly different (p < 0.05).
Compared to control (0 min) all HVACP treatments significantly (p < 0.05) decreased the initial numbers of viable S. enterica irrespective of agar medium used to recover survivors. Based on colony counts on TAL medium, log10 CFU/g reductions of S. enterica were 1.97, 3.19, and 4.20 after HVACP treatment for 3, 9, and 15 min, respectively. From results of using XLT4 agar, reductions (log10 CFU/g) of the pathogen in HVACP-treated samples were 2.17 (3 min), 3.49 (9 min) and 5.07 (15 min). In general, a higher number of survivors were consistently recovered on TAL medium compared to XLT4 agar; however, the differences were not statistically significant (p > 0.05). The inability of some S. enterica survivors to grow on the selective XLT-4 agar suggests that a portion of the survivors was sub-lethally injured by HVACP.
Figure 3 shows STEC survivors on shredded coconut after direct treatment with HVACP (70 kV). Based on colony counts on TAL medium, the initial viable count (log10 CFU/g) of STEC after 1 h of drying of the inoculum at 22°C ± 1°C, was 5.99. As observed with S. enterica, a portion of the STEC population lost viability after drying the inoculum on the shredded coconut. The initial viable counts of STEC decreased with increased exposure to HVACP irrespective of the recovery medium (Figure 3). Significant inactivation of the pathogen occurred after 2–5 min of HVACP treatment (p < 0.05). Based on colony counts on TAL medium, reductions (log10 CFU/g) of the pathogen were 0.87, 1.56, 1.77, and 2.05 after HVACP treatments for 2, 3, 4, and 5 min respectively. For the selective agar (SMAC), reductions (log10 CFU/g) of the pathogen in HVACP-treated samples were 1.46 (2 min), 1.71 (3 min), 1.99 (4 min), and 2.22 (5 min). Although higher numbers of STEC survivors were consistently recovered on TAL medium compared to SMAC, the differences were not statistically significant (p > 0.05).
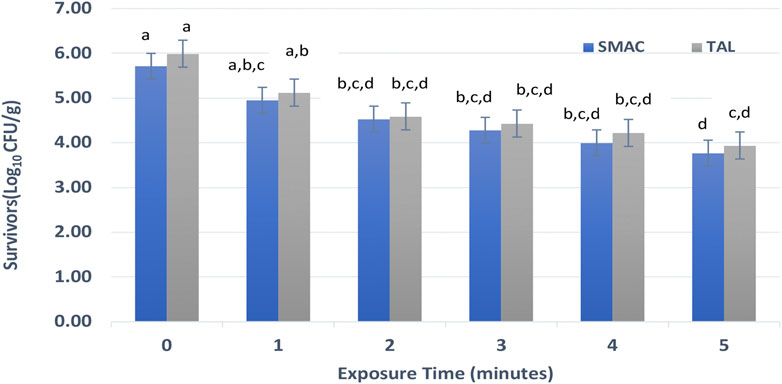
FIGURE 3. Shiga toxin-producing Escherichia coli survivors on desiccated shredded coconut following high voltage cold plasma (HVACP) treatment at 70 kV. Numbers of survivors are means from four replicate experiments. Error bars represent standard error of the mean. Bars that do not have the same letter(s) are significantly different (p < 0.05).
Our results are consistent with those of published reports indicating a decline in viability S. enterica or E. coli with increased exposure to ACP on other low aW products such as peppercorns (Hertwig et al., 2015), black pepper (Mosovska et al., 2018), wheat grains (Thomas-Popo et al., 2019), and almonds (Niemira, 2012; Hertwig et al., 2017). This pattern of inactivation is likely due to longer exposure of the pathogens to reactive oxygen species (ROS) and reactive nitrogen species (RNS) in plasma that inflict more damage to the pathogens (Lu et al., 2014; Olatunde et al., 2019). Structural and metabolic damage by ROS such as oxygen atoms, hydroxyl radicals, metastable oxygen molecules, and ozone, play a major role in microbial inactivation (Deng et al., 2006). Moreover, RNS such as nitric oxide and nitrogen dioxide can cause DNA cleavage, enzyme inactivation, and cellular damage via lipid peroxidation (Deng et al., 2006; Han et al., 2014).
Following exposure of both pathogens to the same HVACP treatment (70 kV, 3 min), reductions (log10 CFU/g) in viable counts of STEC and S. enterica were 1.97 and 1.56, respectively (p < 0.05) based on survivors on TAL medium. Those results suggest that STEC is more tolerant than S. enterica to HVACP under the specific conditions presented by that 3-min treatment. Our results are consistent with those of Ziuzina et al. (2014) who reported that Escherichia coli was more resistant than S. enterica (p < 0.05) to HVACP (70 kV) treatment of cherry tomatoes with air as the carrier gas at atmospheric pressure. However, contrary to our results, Thomas-Popo et al. (2019) reported no significant differences in the death rate between E. coli O157:H7 and S. enterica following direct ACP (44 kV) treatment of tempered wheat grains (p > 0.05). Niemira (2012) reported no clear pattern of pathogen resistance in E. coli and S. enterica on artificially inoculated raw almond kernels exposed to a cold plasma jet for 10 or 20 s. The inconsistencies in those previously mentioned findings may be attributed to various factors such as bacterial strains, type of plasma source, surface properties of the food product, ACP treatment parameters, type of carrier gas, the relative humidity, and the physiological state of pathogens (Bourke et al., 2017). Moreover, differences in cold plasma generating systems and food matrix characteristics pose challenges to meaningful comparisons of reported results on microbial inactivation by ACP.
Sub-lethal injury in pathogen survivors on HVACP-treated coconut
The mean values of sub-lethal injury in populations of S. enterica and STEC survivors in HVACP-treated shredded coconut are shown in Table 1. Those values are expressed by linear regression parameters. HVACP-induced sub-lethal injury in pathogen survivors was determined from the slopes and y intercepts of the regression lines (Wuytack et al., 2003). A slope of 1.0 and y intercept of 0 indicate no sub-lethal injury because the same reduction in pathogen viability occurs on the selective agar and the TAL recovery medium. When a slope and y intercept, respectively, are significantly >1.0 and >0, there is sub-lethal injury because of the higher reduction in viability on selective agar compared to the TAL medium (Wuytack et al., 2003). In the present study, the y-intercept of the regression line for STEC is significantly different (p < 0.05) from 0. This difference indicates significant sub-lethal injury in the population of STEC survivors. For S. enterica, while the y-intercept exhibited a greater difference from 0 compared to STEC, that difference was not statistically significant (p > 0.05).

TABLE 1. Sub-lethal injury (expressed by linear regression parameters*) in surviving populations of Salmonella enterica and shiga toxin-producing Escherichia coli following high voltage atmosphere cold plasma (70 kV) treatment of shredded coconut.
Detecting sub-lethally injured pathogens in foods following a decontamination treatment is critical to microbial food safety because it precludes erroneous overestimation of the effectiveness of that treatment. Moreover, it can provide opportunities for the combined use of additional food preservation technologies in a hurdle format to eliminate sub-lethally injured pathogens (Aaliya et al., 2021; Ucar et al., 2021). The underestimation of pathogen survivors due to sub-lethal injury occurs from use of selective media, which will only allow non-injured survivors to form colonies (Busta, 1976; Wesche et al., 2009). One of the major impediments in determining sub-lethally injured pathogens in food products is the growth of background microflora. Given that selective media can inhibit the resuscitation of sub-lethally injured cells, the use of non-selective media seems logical. However, the use of non-selective media facilitates overgrowth by the natural microflora thus precluding detection of sub-lethally injured cells. To circumvent this problem, Kang and Fung (2000) developed the thin layer agar (TAL) method to enable the resuscitation of sub-lethally injured cells while inhibiting the growth of background microflora. In the present study, the use of the TAL method facilitated detection of sub-lethally injured S. enterica and STEC in HVACP-treated desiccated coconut that would have otherwise remained undetected if only selective agar media were used. This in turn would have underestimated the numbers of viable pathogen survivors in that desiccated food product.
High variability in percent injury data across all replications of the experiment was observed. This could be due to the varying degrees by which the HVACP could have injured the cells since sub-lethal injury can range from mild to severe. The type of injury, structural and/or metabolic, is also an important consideration (Wesche et al., 2009). Moreover, HVACP is a dynamic activated gas with varying amounts and types of reactive species which can cause a spectrum of sub-lethal injuries (from mild to severe) to bacterial cells. Severely injured bacteria may suffer from oxygen toxicity from damage to oxygen detoxifying enzymes, whereby they fail to grow aerobically unless the injury is repaired. In this regard, the use non-selective agar combined with anaerobic incubation could be an effective approach to facilitate detection of such severely injured bacteria (Knabel et al., 1990; Mendonca and Knabel, 1994). Although anaerobic incubation conditions were not used in the present study, the likely oxygen sensitivity that can occur in pathogens with severe sub-lethal injury justifies further research on use of those conditions for recovering survivors of HVACP treatments.
Effect of HVACP on color of shredded coconut
Change in color of shredded coconut was determined using CIE L*a*b, which is based on an Opponent-Color Theory. That theory assumes that humans perceive color as pairs of opposites. These pairs are (i) L—light and dark, (ii) a—red and green, and (iii) b—yellow and blue. Thus, the L* value indicates the level of light (50–100) or dark (0–50), a* value redness (positive) or greenness (negative), and b* value yellowness (positive) or blueness (negative). These values make up the three categories in which all color is organized, namely, lightness, saturation (chroma), and hue. Lightness refers the amount of white or black present in a color. Saturation is the amount of grey in the color indicating brightness. Hue refers to the amount of white, black, and grey in a color and thus theoretically represents what humans perceive to be color (Hunter, 2012; Ly et al., 2020).
Table 2 shows mean values for L*, a* b*, hue, and saturation for control and HVACP (70 kV)-treated (12 min) desiccated shredded coconut. Samples of coconut exposed to HVACP for12 min were selected for instrumental analysis of color and texture because the longer treatment time (16 min) caused slight clumping or stickiness of the coconut shreds. The a* value for HVACP-treated samples was significantly lower (p < 0.05) than that of control samples, indicating a decrease in redness of the treated samples. No significant difference (p > 0.05) between control and HVACP-treated samples was observed for L*, b*, or saturation index; however, the hue of the HVACP-treated samples was significantly higher (p < 0.05) compared to control. This result suggests that the hue of the HVACP-treated samples was closer to yellow, given the increased angle, in comparison to control samples, which were closer to red. Overall, the data indicate that the HVACP-treated samples were less red and slightly darker than the control samples.

TABLE 2. Color evaluation of desiccated shredded coconut after treatment with high voltage atmospheric cold plasma (HVACP) at 70 kV for 12 min.
Despite this significantly higher hue of the HVACP-treated coconut compared to control, a color difference was not perceptible to the naked eye. Gabriel et al. (2016) reported similar changes for shredded coconut treated with ultraviolet-C radiation. In that study, slight and significant differences in the color parameters (via instrumental measurements) suggested a slight darkening of the coconut; however, those changes were indistinguishable to consumers, and the product was deemed acceptable. In a review of the literature on ACP treatment of foods, Pankaj et al. (2018) concluded that, overall, cold plasma processing has minimal effects on the color of food products.
Effect of HVACP on texture of shredded coconut
Average values for texture of control and HVACP-treated (12 min) shredded coconut are shown in Table 3. The HVACP-treated samples exhibited increased firmness, cohesiveness and resilience compared to the control (p < 0.05). There was no significant difference in adhesiveness between control and HVACP-treated samples (p > 0.05). Based on these results we infer that the HVACP-treated shredded coconut might be “tougher” than the control as it better withstood deformation in the texture analyzer. From a practical perspective, this toughness of shredded coconut is likely to be insignificant considering the way in which this food ingredient is used. Most shredded coconut is utilized as an ingredient in baked products (cookies, cakes, biscuits, pie fillings), confectioneries, and blended drinks such as coconut-flavored smoothies (Sangamithra et al., 2013). In such instances, the tough texture of the shredded coconut may not be unfavorable to consumers due to both moistness and the dilution effect of other bulk ingredients that are major components of those food products.

TABLE 3. Average values from texture analysis of desiccated shredded coconut after exposure to high voltage atmospheric cold plasma (HVACP) at 70 kV for 12 min.
Conclusion
Direct HVACP (70 kV) treatment of desiccated shredded coconut significantly inactivated S. enterica and STEC in that product. Higher numbers of S. enterica or STEC survivors in HVACP-treated shredded coconut were detected using the TAL method compared to selective agar media. In this regard, the use of selective agar media to evaluate pathogen survivors in HVACP-treated desiccated coconut shreds can pose a food safety risk by failing to detect sub-lethally injured pathogens. HVACP treatment of shredded coconut for up to 12 min caused minimal changes in color and texture. Based on the results of the present study, HVACP (70 kV) has good potential for improving the microbial safety of shredded coconut without substantially altering color and texture of this popular food ingredient.
Data availability statement
The raw data supporting the conclusion of this article will be made available by the authors, without undue reservation.
Author contributions
AM conceived the research idea, designed the study, and guided the research performed by TD. AM, PF-D-S, and ZW supervised the conduct of all experiments involving HVACP. TB guided TD in the performance of all physiochemical tests. TD conducted the experiments and drafted the manuscript. TD and ET-P performed statistical analysis of the data. AM, BB-S, and SW provided revisions of the manuscript. All authors contributed to the article and approved the submitted version.
Funding
This work was supported by the Iowa Agriculture and Home Economics Experiment Station Project No. IOW04202, sponsored by the Hatch Act and State of Iowa funds.
Acknowledgments
The authors thank Ali El Sadiq for technical assistance.
Conflict of interest
The authors declare that the research was conducted in the absence of any commercial or financial relationships that could be construed as a potential conflict of interest.
The authors AM declared that they were an editorial board member of Frontiers, at the time of submission. This had no impact on the peer review process and the final decision.
Publisher’s note
All claims expressed in this article are solely those of the authors and do not necessarily represent those of their affiliated organizations, or those of the publisher, the editors and the reviewers. Any product that may be evaluated in this article, or claim that may be made by its manufacturer, is not guaranteed or endorsed by the publisher.
References
Aaliya, B., Sunooj, K. V., Navaf, M., Akhila, P. P., Sudheesh, C., Mir, S. A., et al. (2021). Recent trends in bacterial decontamination of food products by hurdle technology A synergistic approach using thermal and non-thermal processing techniques. Food Res. Int. 147, 110514. doi:10.1016/j.foodres.2021.110514
Basaran, P., Basaran-Akgul, N., and Oksuz, L. (2008). Elimination of Aspergillus parasiticus from nut surface with low pressure cold plasma (LPCP) treatment. FOOD Microbiol. 25, 626–632. doi:10.1016/j.fm.2007.12.005
Bourke, P., Ziuzina, D., Boehm, D., Patrick, A., Cullen, J., and Keener, K. (2017). Special issue Plasma biotechnology the potential of cold plasma for safe and sustainable. Food Prod. 36, 615–626. doi:10.1016/j.tibtech.2017.11.001
Busta, F. F. (1976). Practical implications of injured microorganisms in food. J. Milk. Food Techno 39 (2), 138–145. doi:10.4315/0022-2747-39.2.138
CDC (2018a). Multistate outbreak of Salmonella infections linked to coconut tree brand frozen shredded coconut. https://www.cdc.gov/salmonella/coconut-01-18/index.html.
CDC (2018b). Multistate outbreak of Salmonella Typhimurium infections linked to dried coconut. https://www.cdc.gov/salmonella/typhimurium-03-18/index.html.
CFIA (2018). Food Recall Warning - various brands of coconut products recalled due to Salmonella. Available at: https://www.in.spection.gc.ca/about-the-cfia/newsroom/food-recall-warnings/complete-listing/2018-01-24/eng/1516842455613/1516842460165 (Accessed June 2, 2022).
Chacha, J. S., Zhang, L., Ofoedu, C. E., Suleiman, R. A., Dotto, J. M., Roobab, U., et al. (2021). Revisiting non-thermal food processing and preservation methods—action mechanisms, pros and cons A technological update (2016–2021). Foods 10 (6), 1430. doi:10.3390/foods10061430
Codex Alimentarius Commission. (1994). Codex standard for grated desiccated coconut–-CODEXSTAN 177–1991. Codex Alimentarius Commission, Rome.
Dhowlaghar, N., Tang, J., and Zhu, M. J. (2021). Thermal inactivation of Salmonella, Listeria monocytogenes and Enterococcus faecium NRRL B-2354 in desiccated shredded coconut. LWT 149, 111851. doi:10.1016/J.LWT.2021.111851
Escudero, C. A., and Schaffner, C. P. (1981). U.S. Patent No. 4,307,120. Washington, DC, USA U.S. Patent and Trademark Office.Bacterial decontamination using microwaves and sulfur dioxid
FAO (2014). Ranking of low moisture foods in support of microbiological risk assessment. Report of an FAO/WHO consultation process. Available at: http://ucfoodsafety.ucdavis. edu/files/209893.pdf (Accessed June 2, 2022).
Faostat, (2020). "Crops". FAOSTAT. Countries - select All; regions - world + (total); elements - production quantity; items - coconuts; years - 2017 + 2016. Available at: http://www.fao.org/faostat/en/#data/QC/(Accessed June 1, 2022).
Fda, (2019). Natural grocers issues recall on coconut smiles organic due to Salmonella. Available at: https://www.fda.gov/safety/recalls-market-withdrawals-safety-alerts/natural-grocers-issues-recall-coconut-smiles-organic-due-salmonella (Accessed June 2, 2022).
Food Agriculture Organization, (2019). Production of coconuts Top 10 producers. http://www.fao.org/faostat/en/#data/QC/(Accessed June 1, 2022).
Gabriel, A. A., Margarita, A., Tongco, P., and Barnes, A. A. (2016). Utility of UV-C radiation as anti-Salmonella decontamination treatment for desiccated coconut flakes. Food control. 71, 117–123. doi:10.1016/j.foodcont.2016.06.026
Gurtler, J. B., Doyle, M. P., and Kornacki, J. L. (2014). The microbiological safety of low water activity foods and spices. New York, NY, USA Springer, 3–13.
Hertwig, C., Reineke, K., Ehlbeck, J., Knorr, D., and Schlüter, O. (2015). Decontamination of whole black pepper using different cold at-mospheric pressure plasma applications. Food control. 55, 221–229. doi:10.1016/j.foodcont.2015.03.003
Hunter, Lab. (2012). Application note measuring color using hunter L, a, b versus CIE 1976 L*a*b*. Virginia, United States Hunter Lab.
Jadhav, H. B., Annapure, U. S., and Deshmukh, R. R. (2021). Non-thermal technologies for food processing. Front. Nutr., 8.657090, doi:10.3389/fnut.2021.657090
Jayasena, D. D., Kim, H. J., Yong, H. I., Park, S., Kim, K., Choe, W., et al. (2015). Flexible thin-layer dielectric barrier discharge plasma treatment of pork butt and beef loin Effects on pathogen inactivation and meat-quality attributes. Food Microbiol. 46, 51–57. doi:10.1016/J.FM.2014.07.009
Kang, D.-H., and Fung, D. Y. C. (2000). Application of thin agar layer method for recovery of injured Salmonella Typhimurium. Int. J. Food Microbiol. 54, 127–132. doi:10.1016/s0168-1605(99)00174-9
Knabel, S. J., Walker, H. W., Hartman, P. A., and Mendonca, A. F. (1990). Effects of growth temperature and strictly anaerobic recovery on the survival of Listeria monocytogenes during pasteurization. Appl. Environ. Microbiol. 56 (2), 370–376. doi:10.1128/AEM.56.2.370-376.1990
Kumar Mahnot, N., Lata Mahanta, C., Farkas, B. E., Keener, K. M., and Misra, N. N. (2019). Atmospheric cold plasma inactivation of Escherichia coli and Listeria monocytogenes in tender coconut water Inoculation and accelerated shelf-life studies. Food control. 106, 106678. doi:10.1016/j.foodcont.2019.06.004
Lamdande, A. G., Prakash, M., and Ksms, R. (2018). Storage study and quality evaluation of fresh coconut grating. J. Food Process. Preserv. 42 (1), e13350. doi:10.1111/jfpp.13350
Liu, S., Roopesh, M. S., Tang, J., Wu, Q., and Qin, W. (2022). Recent development in low-moisture foods Microbial safety and thermal process. Food Res. Int. 155, 111072. doi:10.1016/j.foodres.2022.111072
Los, A., Ziuzina, D., and Bourke, P. (2018). Current and future technologies for microbiological decontamination of cereal grains. J. Food Sci. 83 (6), 1484–1493. doi:10.1111/1750-3841.14181
Lu, H., Patil, S., Keener, K. M., Cullen, P. J., and Bourke, P. (2014). Bacterial inactivation by high-voltage atmospheric cold plasma Influence of process parameters and effects on cell leakage and DNA. J. Appl. Microbiol. 116 (4), 784–794. doi:10.1111/jam.12426
Luna, S., Taylor, M., Galanis, E., Asplin, R., Huffman, J., Wagner, D., et al. (2018). Outbreak of Salmonella Chailey infections linked to precut coconut pieces - United States and Canada, 2017. Morb. Mortal. Wkly. Rep. 67 (39), 264–266. doi:10.14745/ccdr.v44i10a05
Ly, B. C. K., Dyer, E. B., Feig, J. L., Chien, A. L., and Del Bino, S. (2020). Research techniques made simple Cutaneous colorimetry A reliable technique for objective skin color measurement. J. Investigative Dermatology 140 (1), 3–12.e1. doi:10.1016/j.jid.2019.11.003
Manley, D. (2011). “Dried fruits and nuts as biscuit ingredients,” in Manley’s technology of biscuits, crackers and cookies (Sawston, United Kingdom Woodhead Publishing), 200–208.
Mendonca, A. F., and Knabel, S. J. (1994). A novel strictly anaerobic recovery and enrichment system incorporating lithium for detection of heat-injured Listeria monocytogenes in pasteurized milk containing background microflora. Appl. Environ. Microbiol. 60 (11), 4001–4008. doi:10.1128/aem.60.11.4001-4008.1994
Misra, N. N., Keener, K. M., Bourke, P., Mosnier, J.-P., and Cullen, P. J. (2014). In-package atmospheric pressure cold plasma treatment of cherry tomatoes, 118. doi:10.1016/j.jbiosc.2014.02.005J. Biosci. Bioeng.
Molina-Hernandez, J. B., and Laika, J., (2022). Influence of atmospheric cold plasma exposure on naturally present fungal spores and physicochemical characteristics of sundried tomatoes (Solanum lycopersicum L). Food 11, 210. doi:10.3390/foods11020210
Mošovská, Silvia, Medvecká, Veronika, Halászová, Noémi, Ďurina, Pavol, Valík, Ľubomír, Anna, Mikulajová, et al. (2018). Cold atmospheric pressure ambient air plasma inhibition of pathogenic bacteria on the surface of black pepper. Food Res. Int. 106, 862–869. doi:10.1016/j.foodres.2018.01.066
Moutiq, R., Misra, N. N., Mendonça, A., and Keener, K. (2019). In-package decontamination of chicken breast using cold plasma technology Microbial, quality and storage studies. Meat Sci. 159, 107942. doi:10.1016/j.meatsci.2019.107942
Nascimento, M. S., Carminati, J. A., Morishita, K. N., Amorim Neto, D. P., Pinheiro, H. P., and Maia, R. P. (2018). Long-term kinetics of Salmonella Typhimurium ATCC 14028 survival on peanuts and peanut confectionery products. PloS one 13 (2), e0192457. doi:10.1371/journal.pone.0192457
Niemira, B. A. (2012). Cold plasma reduction of Salmonella and Escherichia coli O157:H7 on almonds using ambient pressure gases. J. Food Sci. 77, M171–M175. doi:10.1111/j.1750-3841.2011.02594.x
Noriega, E., Shama, G., Laca, A., Díaz, M., and Kong, M. G. (2011). Cold atmospheric gas plasma disinfection of chicken meat and chicken skin contaminated with Listeria innocua. Food Microbiol. 28 (7), 1293–1300. doi:10.1016/j.fm.2011.05.007
Olatunde, O. O., Benjakul, S., and Vongkamjan, K. (2019). Dielectric barrier discharge cold atmospheric plasma Bacterial inactivation mechanism. J. Food Saf. 39 (6), e12705. doi:10.1111/jfs.12705
Pankaj, S. K., Wan, Z., and Keener, K. M. (2018). Effects of cold plasma on food quality A review. Foods 7 (4), 4. doi:10.3390/foods7010004
Podolak, R., Enache, E., Stone, W., Black, D. G., and Elliott, P. H. (2010). Sources and risk factors for contamination, survival, persistence, and heat resistance of Salmonella in low-moisture foods. J. food Prot. 73 (10), 1919–1936. doi:10.4315/0362-028x-73.10.1919
FAO (1999). “Coconut post-harvest operations. INPhO-post-harvest compendium,”. Editors P. G. Punchihewa, and R. N. Arancon, Available at: http://www.fao.org/fileadmin/user_upload/inpho/docs/Post_Harvest_Compendium_-_Coconut.pdf (Accessed April 27, 2023).
Sangamithra, A., Swamy Gabriela, J., Sorna Prema, R., Chandrasekar, V., Sasikala, S., and Hasker, E. (2013). Coconut An extensive review on value added products. Indian Food Ind. Mag. 32 (6), 29–36.
Suhem, K., Matan, N., Nisoa, M., and Matan, N. (2013). Inhibition of Aspergillus flavus on agar media and brown rice cereal bars using cold atmospheric plasma treatment. Int. J. Food Microbiol. 161 (2), 107–111. doi:10.1016/J.IJFOODMICRO.2012.12.002
Tetra Pak, (2016). “Coconut food production,” in Coconut handbook (Denton, Texas, United States Tetra Pak).
Thomas-Popo, E., Mendonça, A., Misra, N. N., Little, A., Wan, Z., Moutiq, R., et al. (2019). Inactivation of Shiga-toxin-producing Escherichia coli, Salmonella enterica and natural microflora on tempered wheat grains by atmospheric cold plasma. Food control. 104, 231–239. doi:10.1016/j.foodcont.2019.04.025
Ucar, Y., Ceylan, Z., Durmus, M., Tomar, O., and Cetinkaya, T. (2021). Application of cold plasma technology in the food industry and its combination with other emerging technologies. Trends Food Sci. Technol. 114, 355–371. doi:10.1016/j.tifs.2021.06.004
Wesche, A. M., Gurtler, J. B., Marks, B. P., and Ryser, E. T. (2009). Stress, sublethal injury, resuscitation, and virulence of bacterial foodborne pathogens. J. Food Prot. 72 (5), 1121–1138. doi:10.4315/0362-028x-72.5.1121
Won, M. Y., Lee, J., and Min, S. C. (2016). Mandarin preservation by microwave-powered cold plasma treatment. Innovative Food Sci. Emerg. Technol. 39, 25–32. doi:10.1016/j.ifset.2016.10.021
World Health Organization, (2022). Ranking of low-moisture foods in support of microbiological risk management Meeting report and systematic review. https://apps.who.int/iris/bitstream/handle/10665/363626/9789240044036-eng.pdf?sequence=1 (Accessed April 28, 2023).
Wuytack, E. Y., Phuong, L. D. T., Aertsen, A., Reyns, K. M. F., Marquenie, D., De Ketelaere, B., et al. (2003). Comparison of sublethal injury induced in Salmonella enterica serovar Typhimurium by heat and by different nonthermal treatments. J. Food Prot. 66 (1), 31–37. doi:10.4315/0362-028x-66.1.31
Keywords: Salmonella, Escherichia coli, desiccated shredded coconut, atmospheric cold plasma, sub-lethal injury, thin agar layer medium
Citation: Durand TS, Mendonca A, Fortes-Da-Silva P, Brehm-Stecher B, Boylston T, Thomas-Popo E, Wan Z and White S (2023) Inactivation of Salmonella enterica and shiga toxin-producing Escherichia coli on desiccated shredded coconut by high voltage atmospheric cold plasma. Front. Food. Sci. Technol. 3:1215328. doi: 10.3389/frfst.2023.1215328
Received: 01 May 2023; Accepted: 03 August 2023;
Published: 16 August 2023.
Edited by:
Evgenia Spyrelli, Agricultural University of Athens, GreeceReviewed by:
Daniela Borda, Dunarea de Jos University, RomaniaSandra Guerrero, University of Buenos Aires, Argentina
Copyright © 2023 Durand, Mendonca, Fortes-Da-Silva, Brehm-Stecher, Boylston, Thomas-Popo, Wan and White. This is an open-access article distributed under the terms of the Creative Commons Attribution License (CC BY). The use, distribution or reproduction in other forums is permitted, provided the original author(s) and the copyright owner(s) are credited and that the original publication in this journal is cited, in accordance with accepted academic practice. No use, distribution or reproduction is permitted which does not comply with these terms.
*Correspondence: Aubrey Mendonca, YW1lbmRvbkBpYXN0YXRlLmVkdQ==