- Department of Sport, Food and Natural Sciences, Faculty of Teacher Education, Arts and Sports, Western Norway University of Applied Sciences, Sogndal, Norway
Objectives: This study aims to validate a submaximal treadmill walking test for estimation of maximal oxygen consumption (VO2max) in individuals at high risk of or with chronic health conditions.
Method: Eighteen participants (age 62 ± 16 years; VO2max 31.2 ± 5.9 ml kg−1 min−1) at high risk of getting or with established chronic diseases performed two valid modified Balke treadmill walking protocols, one submaximal protocol, and one maximal protocol. Test duration, heart rate (HR), and rate of perceived exertion (RPE) were measured during both tests. VO2max was measured during the maximal test. VO2max was estimated from the submaximal test by multiple regression using time to RPE ≥ 17, gender, age, and body mass as independent variables. Model fit was reported as explained variance (R2) and standard error of the estimate (SEE).
Results: The model fit for estimation of VO2max from time to RPE ≥ 17 at the submaximal test, body mass, age, and gender was R2 = 0.78 (SEE = 3.1 ml kg−1 min−1, p ≤ 0.001). Including heart rate measurement did not improve the model fit.
Conclusions: The submaximal walking test is feasible and valid for assessing cardiorespiratory fitness in individuals with high risk of or chronic health conditions.
Introduction
Cardiorespiratory fitness is a strong predictor of all-cause mortality and longer life expectancy (Kodama et al., 2009; Barry et al., 2014). The gold standard for assessing an individual's cardiorespiratory fitness is to directly measure their maximal oxygen consumption (VO2max) by the measurement of expiratory gases during maximal exercise (ATS/ACCP, 2003). VO2max was first defined by Hill et al. (1924) as the oxygen consumption at a training intensity where oxygen intake reaches a plateau, despite an increase in workload. Thus, measurement of VO2max is often performed during maximal graded exercise tests (Vehrs et al., 2007), where workload is increased until exhaustion. However, the direct measurement of VO2max requires highly skilled personnel and sophisticated instrumentation and relies on participants' motivation and capability to push themselves to their physical limit.
When treadmill and cycle ergometer testing were first introduced into clinical practice, practitioners often used protocols such as the Balke protocol (Balke and Ware, 1959), from which VO2max can be estimated from time to exhaustion (Pollock et al., 1982). A modified version of the Balke protocol has been used to assess cardiorespiratory fitness in a large sample of adults and elderly in Norway, where it was shown that VO2max could be estimated reasonably well from time to exhaustion [R2 = 0.78, standard error of the estimate (SEE) = 4.6 ml kg−1 min−1] (Aadland et al., 2016). However, such maximal protocols may be less suitable for individuals with a variety of chronic health conditions (Lennon et al., 2012; Sartor et al., 2013), which has stimulated the development of submaximal tests for estimation of VO2max (Jørgensen et al., 2009; Sartor et al., 2013).
In Norway, about half of the municipalities have established healthy life centers (HLCs) as a primary health care service to promote physical activity, smoking cessation, and a healthy diet among individuals at high risk of getting or with established chronic diseases (Ekornrud and Thonstad, 2018; Helsedirektoratet, 2019). The HLCs primarily recruit obese adults and older adults with multiple chronic conditions including musculoskeletal disorders and cardiovascular risk factors and diseases (Blom et al., 2019). The HLCs have applied a modified submaximal Balke walking treadmill protocol to asses cardiorespiratory fitness (Blom et al., 2020a), using Borg scale measured rate of perceived exertion (RPE) ≥ 17 (Borg, 1982) as the criterion for completing the test. The protocol was modified to suit the target group with multiple chronic conditions (i.e., with lower start speed than the original protocol) and for application with various types of treadmills with limited inclination and speed adjustment intervals. However, the validity of the submaximal protocol used in Norwegian HLCs has not been evaluated. Therefore, our aim was to validate this protocol in individuals at high risk of or with chronic health conditions against VO2max.
Materials and Methods
Design
In the present study, we performed two modified Balke protocols (Aadland et al., 2016; Blom et al., 2020a) on a motor-driven treadmill (Woodway PPS 55; WOODWAY GmbH, Weil am Rhein, Germany). The participants first performed a submaximal test that was terminated at RPE ≥ 17 and then a maximal test to exhaustion. The two tests were separated by a minimum of 4 days (12 ± 11) to allow the participants to recover between the tests.
Patient and Public Involvement
During data collection in a previously published study (Blom et al., 2020b), employees at HLCs expressed the need for a valid tool to interpret and communicate HLC participants' cardiorespiratory fitness. Through communication with the HLC employees participating in the study (Blom et al., 2020a), the research question for the present study was developed. The local HLC recruited participants for the present study. The HLCs will be provided with the equation to estimate VO2max for each participant undertaking the submaximal test and hence be able to interpret and communicate the participant's cardiorespiratory fitness by comparison with normal values.
Participants
We recruited 23 adult participants (14 women and 9 men) aged 18–85 years taking part in exercise programs for individuals at high risk of or with established chronic disease(s) (i.e., HLC, union for heart and lung disease, cancer rehabilitation, and rheumatism habitation). The participants self-reported their risk factors for cardiometabolic diseases and known cardiometabolic diseases in a questionnaire previously used in other Norwegian studies testing cardiorespiratory fitness in HLCs (Blom et al., 2020a) and the general population (Aadland et al., 2016). Three participants (13%) had known heart disease, two (9%) occasionally felt chest pain at rest or when performing physical activity, five (22%) had hypertension, six (26%) used medication for hypertension or heart disease, and four (17%) had close relatives with heart infraction of sudden death before the age of 55 and 65 years for men and women, respectively. Three participants (13%) smoked regularly, six (26%) had hypercholesterolemia, and four had diabetes. Eight participants (35%) had none of the conditions mentioned above. Participants were not eligible if they had been advised by their physician to avoid heavy physical work. No potential participants volunteered for the study and were found not eligible. The test laboratory had access to a defibrillator, and an emergency room with a physician on call situated <1 km away provided medical backup. The study was approved by the Norwegian Centre for Research Data (reference number: 663351) and conducted according to the Helsinki declaration. All participants provided written informed consent and completed a health declaration prior to performing any tests. Information about the tests was given to each participant in a standardized manner.
Tests
Submaximal Test Used in Norwegian HLC (Blom et al., 2020a)
Participants performed a self-paced treadmill familiarization prior to testing (speed 2.0–3.5 km h−1 for 2–7 min). The test started with a walking speed of 4.0 km h−1 on a flat treadmill. After 4 min, the treadmill inclination increased by 2% every minute until an inclination of 12%. We then increased the speed by 0.5 km h−1 every minute. The participants were asked to report RPE on the Borg 6–20 scale (Borg, 1982) 10 s before each increase in workload, and the test was terminated the first time an RPE ≥ 17 was reported. A progressive test protocol up to RPE 17 at the Borg scale has previously been shown to predict maximal oxygen uptake with reasonable accuracy compared to lower RPE (Coquart et al., 2014). Performance is reported as the time to RPE ≥ 17.
Maximal Test Previously Used in a Large Norwegian Study in Individuals ≥55 Years Old (Aadland et al., 2016)
A self-paced familiarization was performed prior to testing (speed 2.0–4.0 km h−1 for 2–7 min). The test started with a walking speed of 3.8 km h−1 at 2% inclination. After 4 min, inclination was increased by 2% each minute until it reached 20%. We then increased the speed by 0.5 km h−1 every minute until exhaustion. The test was terminated when participants were unable to keep up with the increasing workload despite verbal encouragement. Oxygen consumption was measured using the Moxus Modular VO2 system with mixing chamber and averaged over 30 s (AEI Technologies, Pittsburgh, Pennsylvania, USA), through a mask connected to a two-way valve (Hans Rudolph Inc., Kansas City, USA). The mask was thoroughly checked for any leaks prior to testing. VO2 was measured every 30th second and VO2max was defined as the mean of the two highest, subsequent measured values. The VO2max was considered valid if two of the three following criteria was achieved: (1) RPE ≥ 19, (2) respiratory exchange ratio (RER) ≥ 1.0, and (3) ≥97% of estimated heart rate maximal values (HRmax). VO2max is reported as milliliters per kilogram per minute. The end criteria was set to secure maximal intensity at test termination and to end up with a reasonable sample size. Besides VO2max, performance was measured using time to exhaustion and time to RPE ≥ 17 (for comparison with the submaximal test).
RPE and heart rate (HR) were measured continuously during both tests. HR was measured using a chest strap (Polar, Kempele, Finland). During the submaximal test, HR was displayed on the treadmill and measured every minute simultaneously with RPE, 10 s before increase in workload. During the maximal test, HR was measured through the Moxus software with a 30-s interval. RPE was assessed using the Borg 6–20 scale (Borg, 1982). HR is presented as the maximal values (HRmax) and as a percent of estimated HRmax (HRmax%) according to a previously published equation (211 – 0.64·age) (Nes et al., 2013). We measured height (without shoes) and body mass (with light clothing and no shoes) prior to the maximal test.
Statistical Analysis
Data are presented as means and standard deviations (SD) unless stated otherwise. Associations between various measures of performance obtained from the two protocols are reported as the explained variance (R2). Differences between the included and excluded participants were tested with unpaired t-tests. We estimated VO2max on the maximal protocol using multiple linear regression with time to RPE ≥ 17 at the submaximal protocol, age, body mass, gender, and HRmax% as independent variables. The model fit is reported as the explained variance (R2) and standard error of the estimate (SEE). Assumption for linear regression was assessed with Kolmogorov–Smirnov and Shapiro–Wilk tests to test for normality in the dependent variable and collinearity between the independent variables; correlation between the independent variable was tested with Pearson's coefficient of correlation; normality of the residuals of the dependent variable was tested with plots (P-P and scatter); and residual statistics were assessed with standard residuals and Cook's distance. All analyses were performed using SPSS v. 25 (IBM Corporation, Software Group, Somers, NY).
Results
Of the 23 participants, one participant only performed the submaximal test and four were excluded for not meeting the present study's criteria for a valid VO2max test (three had too low HR and RER and two had too low HR, RER, and RPE). Thus, the main analysis comprise18 participants aged 35–85 years (11 women and 7 men). Table 1 presents descriptive data and results from the exercise tests for both the included and the excluded participants. In addition to lower HR and RER, the excluded participants had lower performance at the maximal test and lower heart rate at the submaximal test. The association between time to RPE ≥ 17 at the submaximal test and time to exhaustion at the maximal test was R2 = 0.71, the association between time to RPE ≥ 17 at the submaximal test and time to RPE ≥ 17 at the maximal test was R2 = 0.81, and the association between time to exhaustion at the maximal test and time to RPE ≥ 17 at the maximal test was R2 = 0.89 (all p ≤ 0.001) (Figure 1).
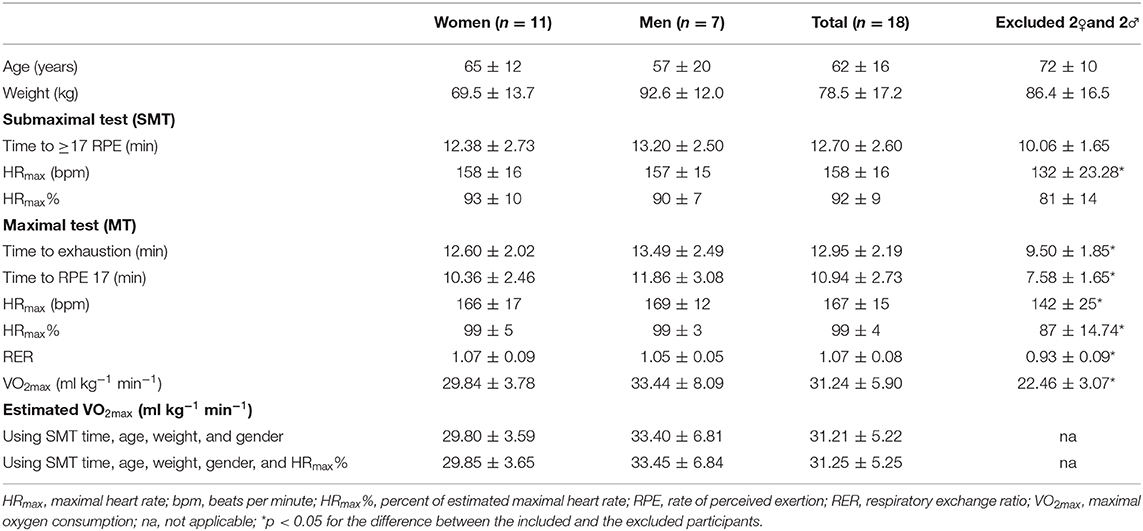
Table 1. Descriptive data, results from cardiorespiratory fitness tests, and estimated VO2max in individuals at high risk of getting or with established chronic diseases.
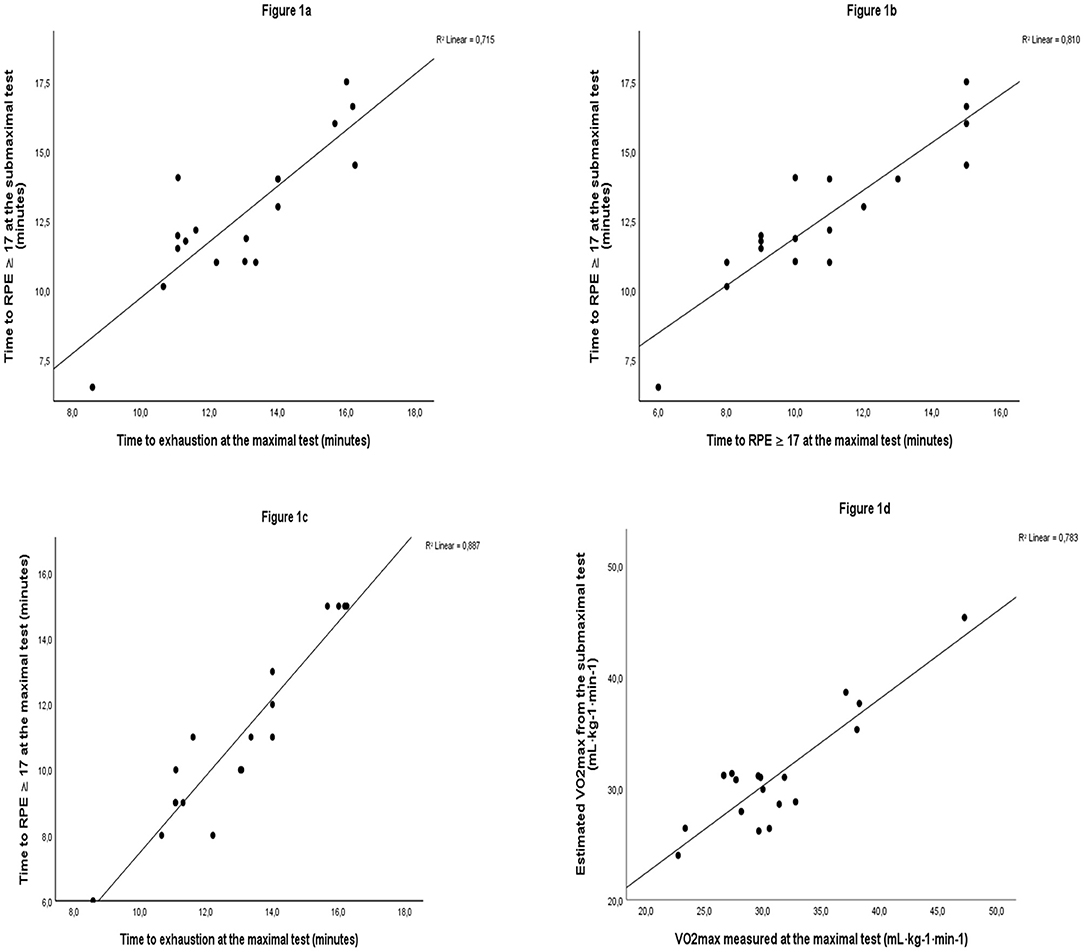
Figure 1. The correlation between time to RPE ≥17 at the submaximal test and time to exhaustion at the maximal test (a), time to RPE ≥17 at the submaximal test and time to RPE ≥17 at the maximal test (b), time to RPE ≥17 at the maximal test and time to exhaustion at the maximal test (c), and estimated VO2max and measured VO2max (d) in individuals at high risk of getting or with established chronic diseases.
The best model for estimation of VO2max from the submaximal test was as follows: VO2max = 45.873 + (1.159 submaximal test time) + (−0.301 body mass) + (−0.264 age) + (7.627 gender). This model showed strong association between estimated VO2max and measured VO2max (R2 = 0.78, SEE = 3.1 ml kg−1 min−1, p ≤ 0.001). Adding HRmax% from the submaximal test to this model did not improve its performance (R2 = 0.79, SEE = 3.2, p ≤ 0.001).
As sensitivity analysis, we performed the analysis including both the 18 included participants and the four participants excluded for not meeting the present study's criteria for a valid VO2max test, and the association between estimated VO2max and measured VO2max was identical (R2 = 0.79, SEE = 2.9, p ≤ 0.001).
VO2max was normally distributed (p = 0.12/0.07), and there was no collinearity between the independent variables (r < 0.7); age (r = −0.5) and RPE ≥ 17 at the submaximal test (r = 0.6) were correlated with VO2max. Weight was not correlated to VO2max (r = 0.1). Standard residuals ranged from −1.5 to 1.3 and Cook's distance ranged from 0.0 to 0.5.
Discussion
This study shows that performance at the submaximal exercise test used to assess cardiorespiratory fitness among individuals at high risk of or with chronic health conditions at HLCs in Norway associated strongly with performance at the maximal test performed according to the gold standard for cardiorespiratory fitness testing. Thus, time to reach RPE 17 is a valid measure of cardiorespiratory fitness in this at-risk population and can be used to estimate VO2max by the equation suggested herein.
The present study showed a strong association between estimated VO2max from performance at the submaximal test and directly measured VO2max in individuals at high risk of getting or with established chronic diseases. Pollock et al. (1982) and Vehrs et al. (2007) reported an even stronger association with lower SEE when estimating VO2max from a graded maximal treadmill walk test in healthy women and for a submaximal treadmill jogging test at a self-selected pace in fit adults, respectively. However, the association found in the present study was similar to the ability of the maximal modified Balke treadmill protocol used to estimate VO2max in a large Norwegian adult population (Aadland et al., 2016) and more accurate than a previously published model estimating VO2max from a submaximal treadmill walking test in overweight children (Nemeth et al., 2009). Thus, we regard the submaximal walking protocol evaluated in the present study well-suited for the assessment of cardiorespiratory fitness in an adult clinical and subclinical population.
The submaximal test used at Norwegian HLCs does not require measurements of HR. However, we investigated if including HRmax% during the submaximal test improved the model fit when estimating VO2max, based on the linear relationship between HR and oxygen consumption (Sartor et al., 2013). Yet, adding HRmax% to the model did not improve the model. Given that the performance of both models was similar, adding measurements of HR for estimation of cardiorespiratory fitness is not necessary.
Potential Bias
The mean number of days between the tests was 12 ± 11 days. This illustrates that some participants had several weeks from the submaximal test to the maximal. We have no information about the lifestyle or change in lifestyle within this period, and fitness may have changed between the two tests. For instance, if participants lost weight, stopped smoking, or became more physically active in the period between the tests, this change may have had an impact on their performance. Indeed, the time to RPE ≥ 17 at the maximal test was more strongly associated with measured VO2max than time to RPE ≥ 17 at the submaximal test, which might indicate some day-to-day variation in fitness and/or change in fitness between the tests.
RPE is a simple, valid, and reliable means to quantify the subjective feeling of exercise tolerance and exertion (Kang et al., 1998; Doherty et al., 2001). Borg's scale relies on verbal anchors connected to the different scores of RPE, and it is possible that individuals at high risk of disease interpret the meanings of the verbal anchors differently than those used to construct the scale (Dawes et al., 2005). The participants in this study had little or no previous experience with exercise, use of Borg scale, and treadmills and lacked experience with strenuous exercise, which may have introduced a bias to the measurements. However, the population in the present study is comparable to participants in HLCs performing the tests as a part of their lifestyle change program. At the maximal test, the participants had already performed a walking test on a treadmill, and the difference in experience with strenuous treadmill walking may have introduced a systematic bias between the two tests. We could have randomized the order of the two tests or performed a familiarization test to reduce this systematic bias. However, we wanted to perform the submaximal tests as close as possible to the HLCs' test setting, as we believe that the bias introduced by familiarization to strenuous treadmill walking ahead of the submaximal test would be larger than the bias introduced by the fixed order of tests.
The duration of the maximal test was 13 ± 2.2 min; thus, it exceeded the traditionally accepted optimal VO2max test duration of 10 ± 2 min (Ross, 2003). However, in their review, Midgley et al. (2008) found that treadmill protocols lasting between 5 and 26 min produced valid VO2max measurements. Given that time to exhaustion from this study slightly exceeded the traditional 8–12-min duration and that Midgley et al. (2008) found that much shorter and longer treadmill protocols also produced valid VO2max measurments indicate that the duration of our maximal test did not compromise the validity of the VO2max measurement.
Participants were allowed to slightly support themselves if feeling pain or to maintain balance. However, supporting oneself during a submaximal test may increase time to “exhaustion” and thus lead to an overestimation of VO2max (Manfre et al., 1994). In our study, participants supporting themselves during the submaximal test also supported themselves during the maximal test to standardize the test condition. Supporting oneself during direct measurement of VO2max will probably not increase VO2max, as support during treadmill walking/running decreases the exercise load, and one must increase exercise load to increase VO2. Thus, support may create a bias in estimation of VO2max, as it might be overestimated in subjects supporting themselves and underestimated in subjects not supporting themselves. However, in a real-life test setting, some subjects with chronic health conditions will need to support themselves, and we chose to include both participants supporting themselves and those not supporting themselves to increase the external validity of our results.
Unfortunately, the questionnaire assessing risk factors for cardiometabolic diseases and known cardiometabolic diseases was used as eligibility screening, and the answers were not linked to the result from the tests; thus, we cannot link the results from the questionnaire to individuals. Six participants used medication for hypertension or heart disease. We do not know the type of medication. If someone took beta-blockers, it would probably blunt the heart rate response to the exercise tests (Wonisch et al., 2003). However, all included participants reached ≥97% of estimated HRmax, and the performance of the model remained the same also when we included the participants not fulfilling our criteria for a valid VO2max test. Thus, the use of beta blockers probably did not affect the results in the present study.
Submaximal tests for estimating VO2max have shown to produce valid and reliable measures in different populations (Oja et al., 1991; Vehrs et al., 2007; Nemeth et al., 2009). Estimation using the UKK walk test (Oja et al., 1991) is a simple self-paced field test and can be applied in large groups of healthy adults, but it requires a large area of flat ground. Our findings suggest the submaximal protocol evaluated herein is a valid and feasible test. Since treadmills are accessible in most fitness facilities and laboratories, the test setting is easily standardized and not limited by climate or weather. Using the best-fit model from this study will allow HLCs to compare their participants with national reference values and ultimately define the health risk of this specific population.
Strengths and Limitations
A strength of this study is the inclusion of a clinical or subclinical population with various health conditions, leaving direct evidence of test performance in this group for which the test is intended for use. Another strength is the strict criteria for verification of a maximal performance, ensuring that all included VO2max measurements were valid. The appropriate criteria for VO2max is highly debated (Edvardsen et al., 2014), and we opted for stricter criteria for VO2max than the criteria used for testing the general population in Norway (Aadland et al., 2016). Our criteria for valid VO2max may have been stricter than necessary as when we included the four subjects not meeting our criteria, the performance of the model remained unchanged. Our sample includes 18 subjects, which is lower than the minimum sample size for regression of 25 recommended by Jenkins and Quintana-Ascencio (Jenkins and Quintana-Ascencio, 2020). Thus, the low sample size reduces the power and generalizability of our findings. The data met all the tested assumptions for linear regression except for a correlation between VO2max and age; however, age was a significant contributor in the model. Due to the small sample size, we could not perform a cross validation of our equation for estimation of VO2max. Thus, despite a relatively large variation in age, cardiorespiratory fitness, and health conditions among our participants, which strengthens the generalizability of our findings, the findings should be interpreted with caution, and future studies should seek to include a larger sample and perform a cross validation in a similar population.
Conclusion
The present study found a strong association between performance on a submaximal treadmill walking protocol and measured VO2max in a clinical or subclinical population. The study demonstrates that the submaximal walking test is valid and feasible as a means for assessing cardiorespiratory fitness in a population and in a setting where direct measurement of VO2max is challenging. However, our findings need verification in a larger study sample.
Data Availability Statement
The datasets presented in this article are not readily available because the subjects signed an informed consent stating that the data should be handled by the researchers conducting the study and deleted after the study was published according to the approval given by Norwegian Centre for Research Data. Requests to access the datasets should be directed to Amund Riiser, YW11bmQucmlpc2VyQGh2bC5ubw==.
Ethics Statement
The study was reviewed and approved by Norwegian Centre for Research Data (Reference No: 663351). The participants provided their written informed consent to participate in this study.
Author Contributions
GE designed the study, collected the data, drafted the manuscript, and approved the final version. EA designed the study, planned the analysis, contributed to the manuscript, and approved the final version. EB designed the study, contributed to the manuscript, and approved the final version. AR planned the study, performed the analysis, and finalized the manuscript.
Conflict of Interest
The authors declare that the research was conducted in the absence of any commercial or financial relationships that could be construed as a potential conflict of interest.
Acknowledgments
Appreciation is extended to Sogn HLC for helping with the recruitment of participants. We also would like to thank all participants included in this study for their participation.
References
Aadland, E., Solbraa, A. K., Resaland, G. K., Steene-Johannessen, J., Edvardsen, E., Hansen, B. H., et al. (2016). Reference values for and cross-validation of time to exhaustion on a modified balke protocol in norwegian men and women. Scand. J. Med. Sci. Sports 27, 1248–1257. doi: 10.1111/sms.12750
ATS/ACCP (2003). ATS/ACCP Statement on cardiopulmonary exercise testing. Am. J. Respir Crit. Care Med. 167, 211–277. doi: 10.1164/rccm.167.2.211
Balke, B., and Ware, R. W. (1959). An experimental study of “physical fitness” of air force personnel. U S Armed Forces Med. J. 10, 675–688. doi: 10.21236/ADA036235
Barry, V. W., Baruth, M., Beets, M. W., Durstine, J. L., Liu, J., and Blair, S. N. (2014). Fitness vs. fatness on all-cause mortality: a meta-analysis. Prog. Cardiovasc. Dis. 56, 382–390. doi: 10.1016/j.pcad.2013.09.002
Blom, E. E., Aadland, E., Skrove, G. K., Solbraa, A. K., and Oldervoll, L. M. (2019). Health-related quality of life and intensity-specific physical activity in high-risk adults attending a behavior change service within primary care. PLoS ONE 14:e0226613. doi: 10.1371/journal.pone.0226613
Blom, E. E., Aadland, E., Solbraa, A. K., and Oldervoll, L. M. (2020b). Healthy Life Centres: a 3-month behaviour change programme's impact on participants' physical activity levels, aerobic fitness and obesity: an observational study. BMJ Open 10:e035888. doi: 10.1136/bmjopen-2019-035888
Blom, E. E., Oldervoll, L., Aadland, E., Solbraa, A. K., and Skrove, G. K. (2020a). Impact and implementation of healthy life centres, a primary-care service intervention for behaviour change in Norway: Study design. Scand. J. Public Health 48, 594–601. doi: 10.1177/1403494819856832
Borg, G. A. V. (1982). Psychophysical bases of perceived exertion. Med. Sci. Sports Exercise 14, 377–381. doi: 10.1249/00005768-198205000-00012
Coquart, J. B., Garcin, M., Parfitt, G., Tourny-Chollet, C., and Eston, R. G. (2014). Prediction of maximal or peak oxygen uptake from ratings of perceived exertion. Sports Med. 44, 563–578. doi: 10.1007/s40279-013-0139-5
Dawes, H. N., Barker, K. L., Cockburn, J., Roach, N., Scott, O., and Wade, D. (2005). Borg's rating of perceived exertion scales: do the verbal anchors mean the same for different clinical groups? Arch. Phys. Med. Rehabilit. 86, 912–916. doi: 10.1016/j.apmr.2004.10.043
Doherty, M., Smith, P. M., Hughes, M. G., and Collins, D. (2001). Rating of percieved exertion during high-intensity treadmill running. Med. Sci. Sports Exercise 33, 1953–1958. doi: 10.1097/00005768-200111000-00023
Edvardsen, E., Hem, E., and Anderssen, S. A. (2014). End criteria for reaching maximal oxygen uptake must be strict and adjusted to sex and age: a cross-sectional study. PLoS ONE 9:85276. doi: 10.1371/journal.pone.0085276
Ekornrud, T., and Thonstad, M. (2018). Frisklivssentralar i kommunane. Ei kartlegging og analyse av førebyggande og helsefremjande arbeid og tilbod i norske kommunar i perioden 2013–2016 [Healthy Life centres in the municipalities. A survey and analysis of health-promoting work and offers in Norwegian municipalities during the period 2013–2016]. Oslo - Kongsvinger: Statistics Norway. Contract No.: 2018/27.
Helsedirektoratet. (2019). Veileder for kommunale frisklivssentraler. Etablering, organisering og tilbud [Recommendations for municipal healthy life centres]. Oslo: Helsedirektoratet (The Norwegian Directorate of Health).
Hill, A. V., Long, C. N. H., and Lupton, H. (1924). Muscular exercise, lactic acid and the supply and utilisation of oxygen. Proc. R. Soc. B 97, 155–176. doi: 10.1098/rspb.1924.0048
Jenkins, D. G., and Quintana-Ascencio, P. F. (2020). A solution to minimum sample size for regressions. PLoS ONE. 15:e0229345. doi: 10.1371/journal.pone.0229345
Jørgensen, T., Andersen, L. B., Froberg, K., Maeder, U., Von Huth Smith, L., and Aadahl, M. (2009). Position statement: testing physical condition in a population – how good are the methods? Eur. J. Sport Sci. 9, 257–267. doi: 10.1080/17461390902862664
Kang, J., Chaloupka, E. C., Mastrangelo, M. A., Donnelly, M. S., Martz, W. P., and Robertson, R. J. (1998). Regulating exercise intensity using ratings of perceived exertion during arm and leg ergometry. Eur. J. Appl. Physiol. 78, 241–246. doi: 10.1007/s004210050414
Kodama, S., Saito, K., Tanaka, S., Maki, M., Yachi, Y., Asumi, M., et al. (2009). Cardiorespiratory fitness as a quantitative predictor of all-cause mortality and cardiovascular events in healthy men and women: a meta-analysis. JAMA 301, 2024–2035. doi: 10.1001/jama.2009.681
Lennon, O. C., Denis, R. S., Grace, N., and Blake, C. (2012). Feasibility, criterion validity and retest reliability of exercise testing using the Astrand-rhyming test protocol with an adaptive ergometer in stroke patients. Disabil. Rehabilitat. 34, 1149–1156. doi: 10.3109/09638288.2011.635748
Manfre, M. J., Yu, G.-H., Varm,á, A. A., Mallis, G. I., Kearney, K., and Karageorgis, M. A. (1994). The effect of limited handrail support on total treadmill time and the prediction of vo2max. Clin. Cardiol. 17, 445–450. doi: 10.1002/clc.4960170808
Midgley, A. W., Bentley, D. J., and Millet, G. P. (2008). Challenging a dogma of exercise physiology. does an incremantal exercise test for valid vo2max determination really need to last between 8 and 12 Minutes? Sports Med. 38, 441–7. doi: 10.2165/00007256-200838060-00001
Nemeth, B. A., Carrel, A. L., Eickhoff, J., Clark, R. R., Peterson, S. E., and Allen, D. B. (2009). Submaximal treadmill test predicts VO2max in overweight children. 154, 677–81.e1. doi: 10.1016/j.jpeds.2008.11.032
Nes, B. M., Janszky, I., Wisløff, U., Støylen, A., and Karlsen, T. (2013). Age-predicted maximal heart rate in healthy subjects: the HUNT fitness study. Scand. J. Med. Scie. Sports 23, 697–704. doi: 10.1111/j.1600-0838.2012.01445.x
Oja, P., Laukkanen, R., Pasanen, M., Tyry, T., and Vuori, I. (1991). A 2-km walking test for assessing the cardiorespiratory fitness of healthy adults. Int. J. Sports Med. 12, 356–362. doi: 10.1055/s-2007-1024694
Pollock, M. L., Foster, C., Schmidt, D., Hellman, C., Linnerud, A. C., and Ward, A. (1982). Comparative analysis of physiologic responses to three different maximal graded exercise test protocols in healthy women. Am. Heart J. 103, 363–373. doi: 10.1016/0002-8703(82)90275-7
Ross, R. M. (2003). ATS/ACCP statement on cardiopulmonary exercise testing. Am. J. Respir. Crit Care Med. 167:1451. doi: 10.1164/ajrccm.167.10.950
Sartor, F., Vernillo, G., de Morree, H. M., Bonomi, A. G., La Torre, A., Kubis, H.-P., et al. (2013). Estimation of maximal oxygen uptake via submaximal exercise testing in sports, clinical, and home settings. Sports Med. 43, 865–873. doi: 10.1007/s40279-013-0068-3
Vehrs, P. R., George, J. D., Fellingham, G. W., Plowman, S. A., and Dustman-Allen, K. (2007). Submaximal treadmill exercise test to predict VO 2 max in fit adults. 11, 61–72. doi: 10.1080/10913670701294047
Keywords: submaximal test, estimation, maximal oxygen consumption, aerobic fitness, healthy life centers
Citation: Eike GSH, Aadland E, Blom EE and Riiser A (2021) Validation of a Modified Submaximal Balke Protocol to Assess Cardiorespiratory Fitness in Individuals at High Risk of or With Chronic Health Conditions—A Pilot Study. Front. Sports Act. Living 3:642538. doi: 10.3389/fspor.2021.642538
Received: 16 December 2020; Accepted: 03 March 2021;
Published: 22 April 2021.
Edited by:
Carol Ewing Garber, Columbia University, United StatesReviewed by:
Cemal Ozemek, University of Illinois at Chicago, United StatesDirk Lund Christensen, University of Copenhagen, Denmark
Copyright © 2021 Eike, Aadland, Blom and Riiser. This is an open-access article distributed under the terms of the Creative Commons Attribution License (CC BY). The use, distribution or reproduction in other forums is permitted, provided the original author(s) and the copyright owner(s) are credited and that the original publication in this journal is cited, in accordance with accepted academic practice. No use, distribution or reproduction is permitted which does not comply with these terms.
*Correspondence: Amund Riiser, YW11bmQucmlpc2VyQGh2bC5ubw==