- 1School of Health and Welfare, Dalarna University, Falun, Sweden
- 2Swedish Unit for Metrology in Sports, Dalarna University, Falun, Sweden
The purpose of the study was to investigate whether there are energy-efficiency differences between the execution of the old-fashioned double-poling technique (DPOLD) and the modern double-poling technique (DPMOD) at a submaximal work intensity among elite male cross-country skiers. Fifteen elite male cross-country skiers completed two 4-min tests at a constant mechanical work rate (MWR) using the DPMOD and DPOLD. During the last minute of each test, the mean oxygen uptake (VO2) and respiratory exchange ratio (RER) were analyzed, from which the metabolic rate (MR) and gross efficiency (GE) were calculated. In addition, the difference between pretest and posttest blood-lactate concentrations (BLadiff) was determined. For each technique, skiers' joint angles (i.e., heel, ankle, knee, hip, shoulder, and elbow) were analyzed at the highest and lowest positions during the double-poling cycle. Paired-samples t-tests were used to investigate differences between DPMOD and DPOLD outcomes. There were no significant differences in either VO2mean, MR, GE, or BLadiff (all P > 0.05) between the DPMOD and DPOLD tests. DPMOD execution was associated with a higher RER (P < 0.05). Significant technique-specific differences were found in either the highest and/or the lowest position for all six analyzed joint angles (all P < 0.001). Hence, despite decades of double-poling technique development, which is reflected in the significant biomechanical differences between DPOLD and DPMOD execution, at submaximal work intensity, the modern technique is not more energy efficient than the old-fashioned technique.
Introduction
From a physiological perspective, endurance performance is suggested to be determined by the sum of the aerobic and anaerobic energy contributions multiplied by gross efficiency (GE) (Joyner and Coyle, 2008). In line with this model, GE has been suggested to be important for performance in elite male cross-country skiing; more specifically, skiers with higher performance levels have been found to have a higher GE than skiers with lower levels of performance (Sandbakk et al., 2010; Ainegren et al., 2013). Cross-country skiers use different sub-techniques during a competition to minimize energy expenditure and/or maximize skiing speed on course sections with different inclinations (Welde et al., 2017; Strøm Solli et al., 2020).
The four main sub-techniques for propulsion in the classical technique are the diagonal-stride technique (DS), double-poling technique (DP), double-poling technique with leg kick (DPK), and herringbone technique (Nilsson et al., 2004). In the DS, the arm's force is transferred through the pole simultaneously with the push off with the leg on the contralateral side of the body (Nilsson et al., 2004). The DP is characterized by a parallel movement of the arms with a synchronous force transfer solely through the poles. However, while using the DPK, the force contribution for propulsion comes from both the synchronous pole-force transfer used in the DP and a simultaneous kick with one of the legs, where the force is transferred to the ground by a kicking motion (Nilsson et al., 2004).
Traditionally, the predominant sub-technique in classical cross-country skiing was the DS, and this rhythmical movement was occasionally broken with the use of the DP or DPK (Saltin, 1996). During recent decades, there have been great developments related to track preparation, functional characteristics and reduction of the mass of ski equipment (Street, 1992). These developments accompanied by improved upper-body strength/power and technique development in elite skiers (Holmberg et al., 2005; Stöggl and Holmberg, 2011, 2016), have led to changes in skiers' sub-technique usage. Recently, it has been shown that elite skiers prefer the DS at an incline of ~7° (Dahl et al., 2017). The DP has been found to be skiers' preferred classical sub-technique on intermediate inclinations (2–4°) (Pellegrini et al., 2013; Andersson et al., 2016; Welde et al., 2017; Strøm Solli et al., 2020); for these inclinations, the DP is associated with a lower oxygen cost and higher GE than the DS (Hoffman et al., 1994; Pellegrini et al., 2013; Andersson et al., 2016; Dahl et al., 2017). Hence, a higher GE is related to reduced metabolic demand for a given skiing speed, which would be advantageous for performance when using the DP compared to using the DS on intermediate terrain.
The technique development in the DP has enabled this sub-technique to be used more extensively on a variety of inclines (Stöggl and Holmberg, 2016), and in the 15-km classical technique race of the 2016 Norwegian championship, the winner used the DP for propulsive force contribution throughout the race (Welde et al., 2017). On intermediate terrain in a 10 or 15 km race, analyses showed that faster skiers used the DP and DPK to a greater extent than slower skiers (Stöggl et al., 2018), and recently, it was found that elite male skiers used the DP 77% of their skiing time on inclines between 2 and 4° during a 15-km race (Strøm Solli et al., 2020). Furthermore, it was reported that elite male skiers improved their skiing performance by ~23 s in a 5-km race when they used the DP exclusively compared with when they skied with a free choice of sub-techniques within the classical technique (Stöggl et al., 2019). Hence, DP is a frequently used sub-technique among elite male skiers, and performance on flat terrain has been suggested to be more important for competitive success in elite male skiers than in elite female skiers (Stöggl et al., 2018).
There are two main phases in the DP: the repositioning phase and the poling phase. During the repositioning phase, the skier extends the joints that are flexed during the previous force transfer (i.e., ankle, knee, and hip joints) to reposition the body into an upright position with a simultaneous raising of the center of body mass (CoM). This increase in gravitational potential energy is thereafter transformed, through the poles, to kinetic energy for forward propulsion during the poling phase. During this phase, the force produced by skeletal muscles also contributes to propulsion.
The “old-fashioned” DP (DPOLD), used in the 1980s and 1990s, was characterized by pronounced trunk flexion and elbow extension at the later stage of the poling phase, where the skier's hands and pole handles pass below knee level with a simultaneous forward inclination of the poles to increase the horizontal propulsive force components (Smith, 2003). It has been suggested that trunk flexion not only lowers the arms and pole handles but also allows the shoulders and elbows to remain in their mid-range positions, where greater joint torque can be generated; this causes more poling force to be exerted in the poling-phase sequence in which the poles are effectively inclined (Smith, 2003). The DP started to evolve in the beginning of the 21st century and today “modern” DP (DPMOD) is the technique predominantly used by elite skiers (Pellegrini et al., 2018a). The DPMOD is characterized by reduced angular joint movements accompanied by higher flexion velocities and greater poling forces (Holmberg et al., 2005), and compared to the characteristics of the DPOLD, this technique development leads to shorter poling times and thereby an improved ability to generate higher skiing speeds in flat terrain (Lindinger et al., 2009). As a result of the reduced joint flexion, compared to that of the DPOLD, the CoM is in a higher position when the repositioning phase is initiated, and reduced work against gravity is necessary to reposition the body before the subsequent poling phase.
Hence, from a biomechanical perspective the DPOLD and DPMOD differ, and it was previously reported that the CoM displacement within the DPMOD explains differences in energy cost between groups with different levels of performance ability (Zoppirolli et al., 2015). Recently, it was shown that a pronounced trunk inclination was related to an increased energy cost during DP (Pellegrini et al., 2018b). They also proposed that, during the last three decades, the DP technique among elite skiers has evolved from a technique characterized by pronounced trunk flexion toward a technique with greater emphasis on shoulder motion during the propulsion phase (Pellegrini et al., 2018b). However, no previous study has investigated whether these biomechanical differences are reflected by physiological differences between techniques. The purpose of the study was to investigate whether there are energy-efficiency differences between the execution of the DPOLD and DPMOD at a submaximal work intensity among elite male cross-country skiers. It was hypothesized that execution of DPOLD would be associated with higher mean oxygen uptake (VO2), respiratory exchange ratio (RER), metabolic rate (MR), and blood lactate concentration (BLa) as well as lower GE compared to the values observed when the DPMOD was used.
Materials and Methods
Participants
Fifteen elite male cross-country skiers (age: 22 ± 4 years; stature: 183 ± 9 cm; body mass: 79.0 ± 9.9 kg) volunteered to participate in the study, and 10 of the skiers had competed in the World Ski Championships and/or the World Cup.
Testing Procedures
Prior to the tests, the height of each participant was measured (Harpenden Stadiometer, Holtain Limited, Crymych, Great Britain). Thereafter, the total mass (m) of each participant and his equipment (i.e., roller skis, poles, ski boots, safety harness, heart-rate receiver, gloves, and clothes) were analyzed (Midrics 2, Sartorius AG, Goettingen, Germany). The participants' own poles were equipped with plastic tips (black plastic tip; LEKI Lenhart GmbH, Kirchheim, Germany) to allow skiers to achieve an adequate grip on the belt of the motor-driven treadmill (Saturn, 450/300 rs, h/p/cosmos sports & medical GmbH, Nussdorf-Traunstein, Germany) during the double-poling tests. The roller skis (Pro-Ski C2, Sterner Specialfabrik AB, Dala-Järna, Sweden) was provided by the laboratory, and the coefficient of rolling resistance of the roller skis (μ) was determined to be 0.022 by using the negative-inclination-equilibrium method previously described (Carlsson et al., 2016).
Before the warm-up, the participants were shown a video clip from the 50 km competition during the Olympics in Sarajevo 1984 (Gullemun, 2009), where the skiers used the DPOLD. In the video clip, the skier had great hip flexion where the trunk in the lower position was almost parallel to the surface. Another technique-specific characteristic for the DPOLD, which was shown in the video, was that the skier's arms were almost fully extended at the pole plant and the skiers' hands passed below the knees at the end of the propulsive phase. Moreover, to be familiar with the execution of the DPOLD, the participants tried the technique during the initial 4 min of the warm-up at a treadmill speed of 2.2 m/s (i.e., 8.0 km/h) and an inclination (α) of 2.5°. Throughout the familiarization period, the individuals conducting the test gave feedback on the skiers' execution based on the technique-specific characteristics to ensure that the participants were able to execute the DPOLD properly. For the remaining 8 min of the warm-up, the treadmill speed was adjusted to find an adequate work intensity for each participant to use during the double-poling tests. The speed adjustments started from a precalculated treadmill speed, which was based on an approximation of an appropriate MR during the double-poling tests. The participants estimated their VO2max, based on previous test results, and 90% of this value was expected to indicate the peak aerobic power during DP (VO2peak) (Holmberg et al., 2007; Björklund et al., 2010; Skattebo et al., 2019). The VO2peak was then multiplied by a factor of 0.82, which was derived from unpublished data collected during a previous study (Carlsson et al., 2014), to establish an MR equivalent to a BLa of ~2.0 mmol/l. Based on the predetermined μ, m, α, and an estimated GE of 16.5% (Dahl et al., 2017), the treadmill speed corresponding to the approximated MR was calculated. Additionally, in the last 4 min of the warm-up, the participants used the diagonal-stride technique at a work intensity (~2.8 m/s, 5.0°) corresponding to the intensity of the subsequent double-poling tests, to minimize differences in BLa values prior to each specific test. Immediately after the warm-up, capillary blood samples were collected to determine the participants' BLa (Biosen 5140, EKF-diagnostic GmbH, Barleben, Germany) prior to the performance of the first double-poling test.
During the first test, the participants were instructed to use their ordinary double-poling technique (i.e., DPMOD), and 1 min after the warm-up, the 4-min test was initiated. The submaximal work intensity during the DPMOD test was constant with an α of 2.5°, and the fixed treadmill speed (v) varied between 3.5 and 4.0 m/s (i.e., 12.6 and 14.3 km/h) depending on the physiological status of the participant. During the 1-min pause between tests, capillary blood samples were collected from the participants. Thereafter, the participants performed the 4-min test using the DPOLD at the same individual submaximal work intensity. Throughout both double-poling tests, the skiers' expired air was continuously analyzed using a metabolic cart in mixing-chamber mode (Jaeger Oxycon Pro, Erich Jaeger Gmbh, Hoechberg, Germany) to continuously determine VO2, RER, ventilation (VE), and breathing frequency (BF).
The calculations of MR and GE were based on the VO2 (l/min) and the RER (l/l) during the last minute of each test. The MR (J/s) was determined using the formula (3.815 + 1.232 · RER) · VO2 · k1 (Lusk, 1928), where k1 was 69.73 and converted kcal/min to J/s. GE is the ratio of the mechanical work rate (MWR) to MR. Based on basic physics, the MWR (J/s) is the sum of the work against gravity and the work related to overcoming the rolling resistance of the roller skis; hence, the MWR was calculated in accordance with the formula m · g · sin α · v + m · g · cos α · μ · v, where g is the acceleration due to gravity.
All double-poling tests were recorded using a video camera (Logitech Rally Camera, Logitech International S.A., Lausanne, Switzerland) positioned perpendicular to the skiing direction with a 3.4-m distance between the camera and the right side of the skiers' body. The video recordings were made to enable subsequent analyses of their sagittal joint angles at the highest and lowest positions during a DP cycle using a video analysis program (Live S, Dartfish SA, Fribourg, Switzerland). The analyzed angles for the two positions were β1, heel; β2, ankle; β3, knee; β4, hip; β5, shoulder; and β6, elbow (Figure 1). For each technique, the joint angles were analyzed for four double-poling cycles at 45 s, 35 s, 25 s, and 15 s before the end of the test. Moreover, the participants' technique-specific cycle rate (CR), and thereby the cycle length (CL), was determined by analyzing the last minute of the video recording.
To estimate the CoM for the two analyzed positions of each double-poling cycle, the length of each body segment, as a fraction of the stature, was determined using a previously published humanoid model (Winter, 2009). Based on the segments' lengths and the six joint angles, the x-coordinate and z-coordinate for each joint center were calculated. Thereafter, the segments' mass and its CoM were determined using a standard model (Robertson et al., 2014). The participants' vertical CoM in each position was calculated as the sum of each segment's mass multiplied by the z-coordinate of the CoM of the segment divided by the body mass. The vertical CoM displacement during each double-poling cycle was calculated as the difference in the vertical CoM between the highest and lowest positions.
Statistics
The results for the biomechanical and physiological variables are presented as the means and standard deviations. The normality of the distributions of test variables was assessed by using the Shapiro–Wilk test. For each test variable, a 95% confidence interval (95% CI) was calculated for the difference between double-poling techniques. Hedges' g, with a correction for small sample size, was used to interpret the magnitude of the effect size (ES) and to enable more informative inferences to be made from the results. Interpretations of the size of the effect were as follows: 0.2 ≤ ES < 0.5 signified a small effect, 0.5 ≤ ES < 0.8 indicated a moderate effect, and ES ≥ 0.8 denoted a large effect (Cohen, 1988). All statistical analyses were assumed to be significant at an alpha level of 0.05. The statistical analyses were conducted using IBM SPSS Statistics software, Version 26 (IBM Corporation, Armonk, USA).
Results
There were technique-specific differences in the joint angles between the DPMOD and DPOLD at the highest position for heel (t = 5.34; P < 0.001; 95% CI [5.48, 12.94]; ES = 1.37), ankle (t = 4.92; P < 0.001; 95% CI [5.09, 13.06]; ES = 1.19), knee (t = −4.73; P < 0.001; 95% CI [−5.10, −1.90]; ES = −0.78), shoulder (t = −11.34; P < 0.001; 95% CI [−36.82, −25.04]; ES = −3.45), and elbow (t = −10.59; P < 0.001; 95% CI [−43.86, −29.00]; ES = −2.84), whereas no difference was found in the measurements of hip (t = −0.57; P = 0.58; 95% CI [−2.74, 1.60]; ES = −0.086) (Table 1). For the lowest position, there were significant differences between the DPMOD and DPOLD in the skiers' joint angles ankle (t = −4.37; P < 0.001; 95% CI [−4.80, −1.63]; ES = −0.73), hip (t = 10.66; P < 0.001; 95% CI [19.88, 29.98]; ES = 3.55), shoulder (t = −8.50; P < 0.001; 95% CI [−33.41, −19.87]; ES = −2.98), and elbow (t = −6.43; P < 0.001; 95% CI [−32.45, −16.12]; ES = −1.82); however, there were no differences in the measurements of the skiers' heel (t = 0.00; P = 1.00; 95% CI [NaN]; ES = NaN) and knee (t = 0.00; P = 1.00; 95% CI [−3.39, 3.39]; ES = 0.00) (Table 1). The presented results related to the video analyses are based on 14 participants because the recording of one participant was corrupted.
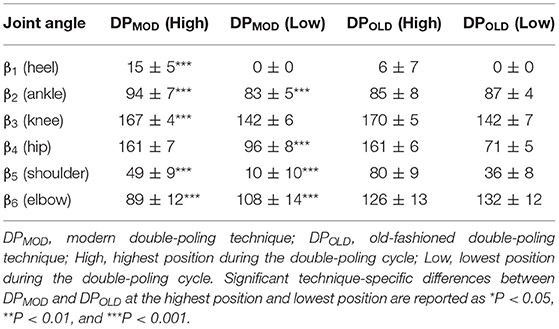
Table 1. Skiers' joint angles in the highest and lowest position for each double-poling technique (mean ± standard deviation).
Skiers' vertical CoM at the highest position when using the DPMOD and DPOLD were 1.12 ± 0.06 m and 1.11 ± 0.06 m, respectively (Figure 2). The skiers' corresponding CoMs for the lowest position were 0.94 ± 0.06 m for the DPMOD and 0.83 ± 0.05 m for the DPOLD (Figure 2). There was a significant difference in skiers' vertical CoM displacement between the DPMOD and DPOLD (0.19 ± 0.02 m vs. 0.28 ± 0.03 m) (t = −10.59; P < 0.001; 95% CI [−11.36, −7.51]; ES = −3.33).
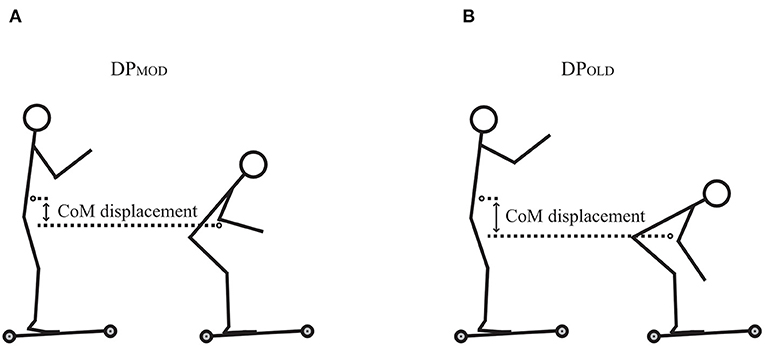
Figure 2. Skiers' mean vertical center of mass (CoM) displacement between the highest and lowest positions in the (A) DPMOD and (B) DPOLD tests.
Skiers' CRs during the DPMOD and DPOLD tests were 0.86 ± 0.06 Hz and 0.69 ± 0.06 Hz, respectively. Skiers' corresponding CLs were 4.36 ± 0.27 m for the DPMOD test and 5.47 ± 0.45 m for the DPOLD test. The CRs were significantly higher during the DPMOD test (t = 9.97; P < 0.001; 95% CI [0.14, 0.21]; ES = 2.77), whereas the CLs were significantly greater during the DPOLD test (t = −9.69; P < 0.001; 95% CI [−1.36, −0.86]; ES = −2.90). Skiers' total CoM displacement per minute from the lowest position to the highest position was 9.82 ± 1.20 m during the DPMOD test and 11.73 ± 1.45 m during the DPOLD test. The corresponding work related to lifting the CoM against gravity was 127 ± 24 J/s for the DPMOD test and 151 ± 28 J/s for the DPOLD test, where skiers performed significantly greater amount of work during the DPOLD test (t = −5.47; P < 0.001; 95% CI [−2.07, −0.90]; ES = −0.91).
The results for the physiological variables collected during the last minute of each test as well as after the tests are presented in Table 2.
There were no significant differences between measurements taken during the DPMOD and DPOLD tests for either VO2 (t = −1.37; P = 0.19; 95% CI [−0.14, 0.03]; ES = −0.14), MR (t = −1.01; P = 0.33; 95% CI [−43.17, 15.57]; ES = −0.09) or GE (t = 0.74; P = 0.47; 95% CI [−0.27, 0.56]; ES = 0.17) (Table 2). The MWR was the same in both test conditions (t = 0.00; P = 1.00; 95% CI [NaN]; ES = NaN), but the RER was higher during the DPMOD test than during the DPOLD test (t = 2.20; P = 0.045; 95% CI [0.0004, 0.03]; ES = 0.43) (Table 2). Skiers' BLapost values were not significantly different (t = −1.70; P = 0.11; 95% CI [−0.57, 0.07]; ES = −0.30), although their BLapre values were higher before the DPOLD test (t = −2.62; P = 0.020; 95% CI [−0.69, −0.07]; ES = −0.51). No significant difference in BLadiff values was found between tests (t = 0.53; P = 0.060; 95% CI [−0.39, 0.64]; ES = 0.22). The VE was significantly lower during the DPMOD than during the DPOLD (t = −3.11; P = 0.0076; 95% CI [−10.08, −1.86]; ES = −0.55), but no difference between techniques was found for BF (t = −1.44; P = 0.17; 95% CI [−0.11, 0.02]; ES = −0.32).
Discussion
The results presented herein show that DPOLD and DPMOD differ substantially from a biomechanical perspective, where many of the analyzed joint angles in the highest and lowest positions during the DP cycle were significantly different between the two techniques. These biomechanical differences resulted in a greater CoM displacement for each DP cycle and a significantly greater amount of work related to lifting the body mass against gravity per minute as well as a lower CR (i.e., greater CL) when skiers performed the DPOLD than when skiers performed the DPMOD. Despite the technique-specific differences, the results of this study demonstrated that there were no substantial energy-efficiency differences between DPOLD and DPMOD at a submaximal work intensity, as indicated by the lack of significant between-test differences for VO2, MR, GE, and BLadiff measurements.
The novel finding that DPOLD is not associated with an increased energy expenditure compared to DPMOD was somewhat unexpected. Based on the theoretically greater CoM displacement using DPOLD and the technique development and refinement during the last decades, we hypothesized that execution of the DPOLD would be more physically demanding than the DPMOD and should therefore be related to a higher oxygen consumption and consequently a higher energy expenditure for the standardized submaximal work intensity. Based on the results, the hypothesis was rejected despite the pronounced biomechanical differences noted between the techniques, where the majority of the measured joint angles in the highest and lowest positions (9 out of 12) differed significantly between techniques.
To explain the non-significant difference in energy expenditure, it is necessary to compare the two techniques from an energetic perspective. During the roller-skiing tests, the energy demand related to work against gravity and the work related to overcoming the rolling resistance of the roller skis was equal for both tests. Moreover, while roller skiing on a treadmill, there is no energy expenditure related to air resistance. Therefore, the MWR was the same for both DP tests. However, it could be assumed that DPOLD and DPMOD differ biomechanically in three factors during the DP cycle: increase in potential energy, translational kinetic energy, and rotational kinetic energy.
There was a significant between-technique difference in the skiers' CoM displacement for each DP cycle (28 cm during DPOLD vs. 19 cm during DPMOD). These results are in line with the previously reported displacements of 25–30 cm for the DPOLD (Smith, 2003), and ~18–19 cm for elite male skiers using the DPMOD while roller skiing at an inclination/speed combination similar to the combination used in the current study (Zoppirolli et al., 2015; Danielsen et al., 2019). The difference between techniques in terms of energy expenditure related to lifting the CoM against gravity is somewhat reduced because of the lower CR of DPOLD. In total, the increase in potential energy associated with CoM displacement is ~19% greater using DPOLD (151 J/s during DPOLD vs. 127 J/s during DPMOD). Consequently, the more pronounced trunk flexion using DPOLD, indicated by the smaller hip angle in the lowest position compared to that of DPMOD, is disadvantageous for DPOLD from an energetic perspective. This is in line with a previous study that showed that the CoM displacement explained differences in energy cost between groups with different levels of performance ability (Zoppirolli et al., 2015).
The translational kinetic energy depends on the difference between the squared highest and lowest velocities during the stride cycle, where a higher CR is assumed to minimize power fluctuations and thereby reduce the energy demand related to this factor (Bergh, 1987). Hence, a large variation in intra-cycle speeds, between minimum speed and maximum speed within the DP cycle, results in a greater acceleration of body mass compared to a situation with lower intra-cycle speed variation. Acceleration of the body is costly from an energetic perspective and in line with this statement a significant correlation was found between an increase in intracycle variation in swimming speed and energy cost (Barbosa et al., 2005). Therefore, a greater CR would be preferable to minimize the energy expenditure associated with the translational kinetic energy. However, it has been shown that skiing with an imposed excessively high CR of 80 cycles/min is associated with a significantly lower GE compared to a CR of 40 cycles/min (Lindinger and Holmberg, 2011). The greater energy demand is to some extent related to the increased rotational kinetic energy due to higher angular velocities for body segments involved during the DP cycle. In the current study, the CR was higher when performing the DPMOD. However, the moment of inertia is probably higher for DPOLD, because of the greater joint angle in the elbow throughout the DP cycle. Therefore, a more comprehensive biomechanical analysis is necessary to determine which technique is more energy demanding from a rotational kinetic energy perspective.
The total energy expenditure linked to these three biomechanically related factors (i.e., increase in potential energy, translational kinetic energy, and rotational kinetic energy during the DP cycle) does not differ significantly between DPMOD and DPOLD, and the advantages and disadvantages for each technique are outbalanced from an energetic perspective. As a consequence of the nonsignificant difference in MR between techniques and the higher CR for DPMOD, it could be concluded that the energy expenditure per DP cycle is higher for DPOLD. Therefore, the ratio between propulsive force impulse and energy expenditure is greater when using the DPOLD than when executing the DPMOD. The greater hip flexion together with the hands passing below the knees results in a relatively large angular displacement of the ski poles at the later stage of the poling phase during DPOLD, which will thereby increase the poling force component in the direction of the track (Hoffman et al., 1990). Through the more effective positioning of the poles during the DPOLD, as much as 90% of the poling force contributes to propulsion (Smith, 2003). Together with the finding that the extensor muscles in the shoulder and elbow joints remain in their mid-range positions during the later stage of the poling phase (Smith, 2003), the more effective force contribution to forward propulsion when executing DPOLD results in longer CL compared to the CL when using DPMOD.
The longer poling time and higher propulsive force impulse when performing the DPOLD did not generate higher values of either BLadiff or BLapost than after the DPMOD test. One possible explanation for the non-significant difference in BLa between techniques is the greater absolute recovery time during the repositioning phase when using DPOLD, which allows a better blood flow with oxygenated blood to the force-producing muscles. Even at moderate intensities during DP execution, force production by the arms is suggested to lead to mechanical hindrance of the oxygen supply, resulting in a lower oxygen extraction in the arms than in the legs (Stöggl et al., 2013). An impaired oxygen supply to the arm musculature implies that there is a higher reliance on glycolytic type II muscle fibers for force production; thus, there is increased lactate production (Ahlborg and Jensen-Urstad, 1991; van Hall, 2000). This reasoning is in line with a recent review in which it was suggested that a rapid force application requires a greater involvement of type II muscle fibers and that a DP strategy with longer poling time could thereby reduce energy expenditure (Zoppirolli et al., 2020b).
The RER was significantly higher during the DPMOD, which to some extent could reflect a more extensive use of type II muscle fibers. Another potential explanation for the reduced RER when performing the DPOLD is related to the higher VE. It has previously been reported that ventilation and saturation are better during DP than during DS, because during execution of the DP technique the skier bends the upper body from an upright position to a nearly horizontal position by contraction of the abdominal muscles which in turn assists exhalation (Holmberg and Calbet, 2007). As indicated by the biomechanical differences between the DPMOD and the DPOLD, the bending motion is more pronounced during DPOLD contributing to higher tidal volume by a reduction of end-expiratory lung volume compared to that when executing the DPMOD. Another important factor for the ventilation difference between the two DP techniques is the better synchronization of respiratory and poling frequencies during DPOLD; the ratio between BF and CR is close to 1:1 when DPOLD is used, whereas the corresponding ratio when using DPMOD is ~1:1.5.
In total, the aerobic energy expenditure was equivalent for both techniques, as indicated by the lack of significant differences in VO2, MR, and GE. The GE of 16.8% in the DPMOD test was in line with a previous study, which reported a mean GE value of ~16.7% for elite male skiers at a similar inclination/speed combination (Dahl et al., 2017). Previously, it has been suggested that individual differences in exercise efficiency are influenced by a weighted sum of physiological and biomechanical factors (Williams and Cavanagh, 1987). In line with this suggestion, exercise efficiency has been suggested to be determined by cardiorespiratory, metabolic, neuromuscular and biomechanical efficiencies (Barnes and Kilding, 2015). Additionally, for skiers of different levels of performance, one or several of these efficiencies could differ between groups, which ultimately results in a higher MR for a given MWR and thereby a lower GE for regional-level skiers.
Performance-level differences in GE have previously been found (Sandbakk et al., 2010; Ainegren et al., 2013), but in the current study, we investigated one group of elite male skiers who performed two different techniques. From the results, it was reasonable to assume that their cardiorespiratory, metabolic, and neuromuscular efficiencies did not differ between DPOLD and DPMOD execution. However, despite significant differences in joint angles and CoM displacement between techniques, GE values did not differ, which suggests that the generally accepted greater biomechanical efficiency for the DPMOD is not correct, at least when DP at a submaximal work intensity. This suggestion is supported by results from computer simulations of skiing efficiency in the double-poling technique using a 3D full-body musculoskeletal simulation model (Holmberg et al., 2013), which found that the traditional technique (i.e., similar to the DPOLD) had a 0.4% higher efficiency than the modern technique.
However, when the DP speed reaches maximum or close to maximum, there is need for a high force impulse to achieve a long CL (Stöggl and Holmberg, 2011, 2016), which requires that the skiers use and master the execution of DPMOD (Stöggl et al., 2011; Zoppirolli et al., 2017a,b). Recently, it was shown that better skiers had shorter duty cycles (% time of the poling phase within the poling cycle), as a result of a shorter poling phase and longer reposition phase compared to skiers with a lower level of performance (Zoppirolli et al., 2020a).
Limitations
There are several limitations in this study. First, the participants did not use the DPOLD in their daily training, which could be considered a limitation of the current study. However, despite their limited experience using DPOLD, there was no difference in energy efficiency between techniques. It could be speculated that a training period, where the skiers used DPOLD in their daily training, would improve the execution of the technique and an improved biomechanical efficiency would thereby lead to a higher GE. This speculation needs to be confirmed or disconfirmed in future studies. Another limitation in the current study is the non-randomized test order in that all skiers started with the DPMOD test; this approach was chosen because we wanted to avoid a potential negative physiological effect of DPOLD on DPMOD. However, in light of the results that DPOLD was not less energy efficient, randomization of the test order would have been possible without significant carry-over effects between tests. In fact, the chosen test procedure might have disfavored DPOLD with a somewhat higher physiological stress at the beginning of the test. Another important issue to further investigate is the effect of prolonged exercise using either DPMOD or DPOLD on muscular fatigue and GE. Moreover, it would be of great importance to analyze the effect of a work intensity closer to the intensity during a race on metabolic stress for the two DP techniques. It would also be of interest to investigate whether the double-poling techniques differs in terms of physiological stress when skiing on snow. Potentially, the load profile on the skis differ between techniques and the grip vax might therefore influence skiing friction differently for DPMOD and DPOLD. All these aspects should also be considered when investigating elite female skiers and skiers with different levels of performance ability.
Data Availability Statement
The raw data supporting the conclusions of this article will be made available by the authors, without undue reservation.
Ethics Statement
The studies involving human participants were reviewed and approved by the Swedish Ethical Review Authority, Lund, Sweden (Dnr 2020-00775). The patients/participants provided their written informed consent to participate in this study.
Author Contributions
TC and MC formulated the first concepts for the study, conducted data analysis and statistical analysis, and wrote the first draft of the manuscript. TC, WF, LW, MS, and MC designed the experimental protocol. LW and WF conducted the experiments. All authors read and approved the final manuscript.
Conflict of Interest
The authors declare that the research was conducted in the absence of any commercial or financial relationships that could be construed as a potential conflict of interest.
Publisher's Note
All claims expressed in this article are solely those of the authors and do not necessarily represent those of their affiliated organizations, or those of the publisher, the editors and the reviewers. Any product that may be evaluated in this article, or claim that may be made by its manufacturer, is not guaranteed or endorsed by the publisher.
Acknowledgments
The authors thank the skiers for their dedicated participation.
References
Ahlborg, G., and Jensen-Urstad, M. (1991). Metabolism in exercising arm vs. leg muscle. Clin. Physiol. 11, 459–468. doi: 10.1111/j.1475-097X.1991.tb00818.x
Ainegren, M., Carlsson, P., Tinnsten, M., and Laaksonen, M. S. (2013). Skiing economy and efficiency in recreational and elite cross-country skiers. J. Strength Cond. Res. 27, 1239–1252. doi: 10.1519/JSC.0b013e31824f206c
Andersson, E., Björklund, G., Holmberg, H. C., and Ørtenblad, N. (2016). Energy system contributions and determinants of performance in sprint cross-country skiing. Scand. J. Med. Sci. Sports 27, 385–398. doi: 10.1111/sms.12666
Barbosa, T. M., Keskinen, K. L., Fernandes, R., Colaco, P., Lima, A. B., and Vilas-Boas, J. P. (2005). Energy cost and intracyclic variation of the velocity of the centre of mass in butterfly stroke. Eur. J. Appl. Physiol. 93, 519–523. doi: 10.1007/s00421-004-1251-x
Barnes, K. R., and Kilding, A. E. (2015). Running economy: measurement, norms, and determining factors. Sports Med. Open 1, 1–15. doi: 10.1186/s40798-015-0007-y
Bergh, U. (1987). The influence of body mass in cross-country skiing. Med. Sci. Sports Exerc. 19, 324–331. doi: 10.1249/00005768-198708000-00002
Björklund, G., Stöggl, T., and Holmberg, H. C. (2010). Biomechanically influenced differences in O2 extraction in diagonal skiing: arm versus leg. Med. Sci. Sports Exerc. 42, 1899–1908. doi: 10.1249/MSS.0b013e3181da4339
Carlsson, M., Carlsson, T., Knutsson, M., Malm, C., and Tonkonogi, M. (2014). Oxygen uptake at different intensities and sub-techniques predicts sprint performance in elite male cross-country skiers. Eur. J. Appl. Physiol. 114, 2587–2595. doi: 10.1007/s00421-014-2980-0
Carlsson, M., Carlsson, T., Wedholm, L., Nilsson, M., Malm, C., and Tonkonogi, M. (2016). Physiological demands of competitive sprint and distance performance in elite female cross-country skiing. J. Strength Cond. Res. 30, 2138–2144. doi: 10.1519/JSC.0000000000001327
Cohen, J. W. (1988). Statistical Power Analysis for the Behavioral Sciences. Hillsdale: Lawrence Erlbaum Associates.
Dahl, C., Sandbakk, Ø., Danielsen, J., and Ettema, G. (2017). The role of power fluctuations in the preference of diagonal vs. double poling sub-technique at different incline-speed combinations in elite cross-country skiers. Front. Physiol. 8, 94. doi: 10.3389/fphys.2017.00094
Danielsen, J., Sandbakk, Ø., McGhie, D., and Ettema, G. (2019). Mechanical energetics and dynamics of uphill double-poling on roller-skis at different incline-speed combinations. PLoS ONE 14, e0212500. doi: 10.1371/journal.pone.0212500
Gullemun (2009). Thomas Wassberg mot Gunde Svan Sarajevo OS 5 mil 1984. Available online at: https://www.youtube.com/watch?v=cuxHS-Tx500&t=287s (accessed August 8, 2021)
Hoffman, M. D., Clifford, P. S., Foley, P. J., and Brice, A. G. (1990). Physiological responses to different roller skiing techniques. Med. Sci. Sports Exerc. 22, 391–396. doi: 10.1249/00005768-199006000-00017
Hoffman, M. D., Clifford, P. S., Watts, P. B., Drobish, K. M., Gibbons, T. P., Newbury, V. S., et al. (1994). Physiological comparison of uphill roller skiing: diagonal stride versus double pole. Med. Sci. Sports Exerc. 26, 1284–1289. doi: 10.1249/00005768-199410000-00017
Holmberg, H. C., and Calbet, J. A. (2007). Insufficient ventilation as a cause of impaired pulmonary gas exchange during submaximal exercise. Respir. Physiol. Neurobiol. 157, 348–359. doi: 10.1016/j.resp.2006.12.013
Holmberg, H. C., Lindinger, S., Stöggl, T., Eitzlmair, E., and Müller, E. (2005). Biomechanical analysis of double poling in elite cross-country skiers. Med. Sci. Sports Exerc. 37, 807–818. doi: 10.1249/01.MSS.0000162615.47763.C8
Holmberg, H. C., Rosdahl, H., and Svedenhag, J. (2007). Lung function, arterial saturation and oxygen uptake in elite cross country skiers: influence of exercise mode. Scand. J. Med. Sci. Sports 17, 437–444. doi: 10.1111/j.1600-0838.2006.00592.x
Holmberg, L. J., Lund Ohlsson, M., Supej, M., and Holmberg, H. C. (2013). Skiing efficiency versus performance in double-poling ergometry. Comput. Methods Biomech. Biomed. Engin. 16, 987–992. doi: 10.1080/10255842.2011.648376
Joyner, M. J., and Coyle, E. F. (2008). Endurance exercise performance: the physiology of champions. J. Physiol. 586, 35–44. doi: 10.1113/jphysiol.2007.143834
Lindinger, S. J., and Holmberg, H. C. (2011). How do elite cross-country skiers adapt to different double poling frequencies at low to high speeds? Eur. J. Appl. Physiol. 111, 1103–1119. doi: 10.1007/s00421-010-1736-8
Lindinger, S. J., Stöggl, T., Muller, E., and Holmberg, H. C. (2009). Control of speed during the double poling technique performed by elite cross-country skiers. Med. Sci. Sports Exerc. 41, 210–220. doi: 10.1249/MSS.0b013e318184f436
Nilsson, J., Tveit, P., and Eikrehagen, O. (2004). Effects of speed on temporal patterns in classical style and freestyle cross-country skiing. Sports Biomech. 3, 85–107. doi: 10.1080/14763140408522832
Pellegrini, B., Stöggl, T. L., and Holmberg, H. C. (2018a). Developments in the biomechanics and equipment of Olympic cross-country skiers. Front. Physiol. 9, 976. doi: 10.3389/fphys.2018.00976
Pellegrini, B., Zoppirolli, C., Boccia, G., Bortolan, L., and Schena, F. (2018b). Cross-country skiing movement factorization to explore relationships between skiing economy and athletes' skills. Scand. J. Med. Sci. Sports 28, 565–574. doi: 10.1111/sms.12938
Pellegrini, B., Zoppirolli, C., Bortolan, L., Holmberg, H. C., Zamparo, P., and Schena, F. (2013). Biomechanical and energetic determinants of technique selection in classical cross-country skiing. Hum. Mov. Sci. 32, 1415–1429. doi: 10.1016/j.humov.2013.07.010
Robertson, D. G. E., Caldwell, G. E., Hamill, J., Kamen, G., and Whittlesey, S. N. (2014). Research Methods in Biomechanics. Champaign: Human Kinetics. doi: 10.5040/9781492595809
Saltin, B. (1996). “The physiology of competitive c.c. skiing across a four decade perspective; with a note on training induced adptations and role of training at medium altitude,” in Sience and Skiing, eds. E. Müller, H. Schwameder, E. Kornexl and C. Raschner (London: E & FN Spon), 435–469.
Sandbakk, Ø., Holmberg, H. C., Leirdal, S., and Ettema, G. (2010). Metabolic rate and gross efficiency at high work rates in world class and national level sprint skiers. Eur. J. Appl. Physiol. 109, 473–481. doi: 10.1007/s00421-010-1372-3
Skattebo, Ø., Losnegard, T., and Stadheim, H. K. (2019). Double-poling physiology and kinematics of elite cross-country skiers: Specialized long-distance versus all-round skiers. Int. J. Sports Physiol. Perform. 1190–1199. doi: 10.1123/ijspp.2018-0471
Smith, G. A. (2003). “Biomechanics of cross country skiing,” in Cross Country Skiing: Handbook of Sports Medicine, ed H. Rusko (Oxford: Blackwell Publishing), 32–61. doi: 10.1002/9780470693834.ch2
Stöggl, T., Björklund, G., and Holmberg, H. C. (2013). Biomechanical determinants of oxygen extraction during cross-country skiing. Scand. J. Med. Sci. Sports 23, e9–e20. doi: 10.1111/sms.12004
Stöggl, T., and Holmberg, H. C. (2011). Force interaction and 3D pole movement in double poling. Scand. J. Med. Sci. Sports 21, e393–e404. doi: 10.1111/j.1600-0838.2011.01324.x
Stöggl, T., Muller, E., Ainegren, M., and Holmberg, H. C. (2011). General strength and kinetics: fundamental to sprinting faster in cross country skiing? Scand. J. Med. Sci. Sports 21, 791–803. doi: 10.1111/j.1600-0838.2009.01078.x
Stöggl, T., Ohtonen, O., Takeda, M., Miyamoto, N., Snyder, C., Lemmettyl,ä, T., et al. (2019). Comparison of exclusive double poling to classic techniques of cross-country skiing. Med. Sci. Sports Exerc. 51, 760–772. doi: 10.1249/MSS.0000000000001840
Stöggl, T., Pellegrini, B., and Holmberg, H. C. (2018). Pacing and predictors of performance during cross-country skiing races: a systematic review. J. Sport Health Sci. 7, 381–393. doi: 10.1016/j.jshs.2018.09.005
Stöggl, T. L., and Holmberg, H. C. (2016). Double-poling biomechanics of elite cross-country skiers: flat versus uphill terrain. Med. Sci. Sports Exerc. 48, 1580–1589. doi: 10.1249/MSS.0000000000000943
Street, G. M. (1992). Technological advances in cross-country ski equipment. Med. Sci. Sports Exerc. 24, 1048–1054. doi: 10.1249/00005768-199209000-00015
Strøm Solli, G., Kocbach, J., Sandbakk, S. B., Haugnes, P., Losnegard, T., and Sandbakk, Ø. (2020). Sex-based differences in sub-technique selection during an international classical cross-country skiing competition. PLoS ONE 15, e0239862. doi: 10.1371/journal.pone.0239862
van Hall, G. (2000). Lactate as a fuel for mitochondrial respiration. Acta Physiol. Scand. 168, 643–656. doi: 10.1046/j.1365-201x.2000.00716.x
Welde, B., Stöggl, T. L., Mathisen, G. E., Supej, M., Zoppirolli, C., Winther, A. K., et al. (2017). The pacing strategy and technique of male cross-country skiers with different levels of performance during a 15-km classical race. PLoS ONE 12, e0187111. doi: 10.1371/journal.pone.0187111
Williams, K. R., and Cavanagh, P. R. (1987). Relationship between distance running mechanics, running economy, and performance. J. Appl. Physiol. 63, 1236–1245. doi: 10.1152/jappl.1987.63.3.1236
Winter, D. A. (2009). Biomechanics and Motor Control of Human Movement. Hoboken: John Wiley & Sons, Inc. doi: 10.1002/9780470549148
Zoppirolli, C., Boccia, G., Bortolan, L., Schena, F., and Pellegrini, B. (2017a). Functional significance of extent and timing of muscle activation during double poling on-snow with increasing speed. Eur. J. Appl. Physiol. 117, 2149–2157. doi: 10.1007/s00421-017-3703-0
Zoppirolli, C., Bortolan, L., Schena, F., and Pellegrini, B. (2020a). Double poling kinematic changes during the course of a long-distance race: effect of performance level. J. Sports Sci. 38, 863–872. doi: 10.1080/02640414.2020.1736246
Zoppirolli, C., Hebert-Losier, K., Holmberg, H. C., and Pellegrini, B. (2020b). Biomechanical determinants of cross-country skiing performance: a systematic review. J. Sports Sci. 38, 2127–2148. doi: 10.1080/02640414.2020.1775375
Zoppirolli, C., Pellegrini, B., Bortolan, L., and Schena, F. (2015). Energetics and biomechanics of double poling in regional and high-level cross-country skiers. Eur. J. Appl. Physiol. 115, 969–979. doi: 10.1007/s00421-014-3078-4
Keywords: cross-country skiing, gross efficiency, oxygen uptake, blood lactate concentration, biomechanical analysis, kinematics, double poling
Citation: Carlsson T, Fjordell W, Wedholm L, Swarén M and Carlsson M (2022) The Modern Double-Poling Technique Is Not More Energy Efficient Than the Old-Fashioned Double-Poling Technique at a Submaximal Work Intensity. Front. Sports Act. Living 4:850541. doi: 10.3389/fspor.2022.850541
Received: 07 January 2022; Accepted: 26 April 2022;
Published: 18 May 2022.
Edited by:
Jaap Van Dieen, VU Amsterdam, NetherlandsReviewed by:
Hugo A. Kerhervé, University of Rennes 2 – Upper Brittany, FranceFrédéric Meyer, University of Oslo, Norway
Copyright © 2022 Carlsson, Fjordell, Wedholm, Swarén and Carlsson. This is an open-access article distributed under the terms of the Creative Commons Attribution License (CC BY). The use, distribution or reproduction in other forums is permitted, provided the original author(s) and the copyright owner(s) are credited and that the original publication in this journal is cited, in accordance with accepted academic practice. No use, distribution or reproduction is permitted which does not comply with these terms.
*Correspondence: Tomas Carlsson, dGNhQGR1LnNl