- 1Research Institute of Physical Fitness, Japan Women’s College of Physical Education, Setagaya City, Japan
- 2Department of Clinical Laboratory, Faculty of Medicine, University of Tsukuba, Tsukuba City, Japan
- 3Research Team for Promoting Independence and Mental Health, Tokyo Metropolitan Institute for Geriatrics and Gerontology, Itabashi City, Japan
- 4Department of Sports Science, Japan Women’s College of Physical Education, Setagaya City, Japan
- 5Master’s Program in Medical Sciences, Graduate School of Comprehensive Human Sciences, University of Tsukuba, Tsukuba City, Japan
- 6Embodied Wisdom Division, Center for Liberal Education and Learning, Sophia University, Chiyoda City, Japan
- 7Department of Sports and Health Science, Japan Women’s College of Physical Education, Setagaya City, Japan
Introduction: We aimed to determine the effects of exercise on cell-free DNA (cfDNA) levels and concentration changes during the menstrual cycle in participants with regular menstrual cycles and no exercise habits.
Methods: Eleven sedentary female students with regular menstrual cycles and ovulation performed bicycle exercises at 60% VO2max for 30 min during the menstrual, ovulatory, and luteal phases. Blood samples were collected before (Pre), immediately after (Post 0), 30 min after (Post 30), and 60 min after (Post 60) exercise. Blood concentrations of ovarian hormones, cfDNA, prostaglandin F2a (PGF2α), interleukin-6 (IL-6), and aromatase were evaluated.
Results: Based on the concentration of ovarian hormones, seven individuals were finally analyzed. No significant phase difference was observed in cfDNA across all time points. cfDNA (menstrual phase: p = 0.028, ovulatory phase: p = 0.018, and luteal phase: p = 0.048) and aromatase concentrations (menstrual phase: p = 0.040, ovulatory phase: p = 0.039, and luteal phase: p = 0.045) significantly increased from Pre to Post 0 in all phases. Serum estradiol (E2) levels were significantly higher in the luteal phase at all time points than in the menstrual phase (Pre: p < 0.001, Post 0: p < 0.001, Post 30: p = 0.005, and Post 60: p = 0.011); however, serum progesterone (P4) levels were significantly higher in the luteal phase at all time points than in the menstrual (Pre: p < 0.001, Post 0: p < 0.001, Post 30: p < 0.001, and Post 60: p < 0.001) and ovulatory phases (Pre: p = 0.005, Post 0: p = 0.005, Post 30: p = 0.003, and Post 60: p = 0.003). E2 levels significantly increased from Pre to Post 0 in the ovulatory and luteal phases, whereas P4 levels increased in the luteal phase. Progesterone to estradiol level ratio (P4/E2) changes from Pre to Post 0 (%baseline) during the luteal phase were significantly negatively correlated (r = −0.82, p = 0.046) with the changes in cfDNA from Pre to Post 0. Furthermore, the repeated measures correlation between P4/E2 and cfDNA level showed a significant negative correlation in ovulatory and luteal phases.
Discussion: The results indicate that while resting cfDNA levels are unlikely to be affected by a woman's menstrual cycle, the increase in cfDNA after exercise is higher in the ovulatory phase (when only E2 increases) and lower in the luteal phase (when E2 and P4 increase with exercise) compared to that in the menstrual phase (when E2 and P4 are in low levels), suggesting the contribution of increased ovarian hormone levels after exercise.
1 Introduction
Recently, people have become more health-and-fitness-conscious, and the number of people engaging in exercise is increasing (1). Thus, monitoring performance, fatigue, and recovery is essential for safe and effective training and exercise therapy. Parameters being monitored include neutrophils and cytokines as indicators of inflammation (2–4), cortisol as an indicator of mental fatigue (5), and creatine kinase and myoglobin as the indicators of muscle damage (6); however, their usefulness has varied. Some responses are specific or delayed, while some parameters are unsuitable as low-intensity indicators (6, 7). Here, we focused on cell-free DNA (cfDNA) as a novel marker with the potential to function as a simple and immediate response indicator.
cfDNA is a mixture of DNA fragments that can be observed not only in diseases, such as cancer and atherosclerosis (8–11), but also after exercise. In recent years, cfDNA has been reported to increase nearly 5-fold in ultramarathons (12), 1.4- to 1.7-fold in eight types of strength training (13), 3-fold in weightlifting training (14), and 2.5- to 5-fold during ergometer loading tests (15–17), including acute exercise. Furthermore, cfDNA may be affected by sex differences. Yuwono et al., reported a cohort study of healthy individuals, and Alghofaili et al., Çayir et al., and Jylhävä et al. found plasma cfDNA levels of 12.7 and 10.9 ng/ml (18), 707 ± 252 and 586 ± 586 ng/ml (19), and 0.729 and 0.699 μg/ml (20) in men and women, respectively, with higher levels observed in males than in females, suggesting that sex differences may be involved (21). Although a precise mechanism has not been elucidated, previous studies have stated that cfDNA concentrations were examined only in men to avoid the effects of menstruation (22) and fluctuations in hormone concentrations (13) that occur in female participants. Therefore, examining the dynamics of cfDNA in women due to exercise while considering fluctuations in female hormone levels and associated phases in the menstrual cycle is crucial. To date, only two studies have examined cfDNA concentrations during the menstrual cycle in women; however, no studies have found significant differences in cfDNA concentrations in the resting state during the menstrual cycle (23, 24). Pölcher et al. did not include the luteal phase when P4 concentration increased (23), and Yuwono et al. (24) included smokers and individuals taking low-dose estrogen-progestin combination (LEP) preparations, making it impossible to conclude the relationships within the menstrual cycle in the resting state. As the performance and conditioning in females are often affected by the menstrual cycle (i.e., changes in the secretion of ovarian hormones) (25–28), it is necessary to establish a method to appropriately manage menstruation. Therefore, the relationship between the menstrual cycle and cfDNA, which can be used as a simple indicator, and the effects of differences in ovarian hormone levels before and after exercise must examined. Hence, it is essential to first examine women with normal menstrual cycles who do not exercise to rule out the effects of regular exercise. Prior research indicates significant increases in cfDNA concentrations at lactate thresholds during moderate-intensity aerobic exercise (29). Consequently, the present study examined changes in cfDNA before and after moderate-intensity exercise loading, considering the health of participants without exercise habits.
In this study, it was important to consider various factors to ensure that moderate-intensity exercise provides an appropriate load. Thus, we evaluated IL-6 levels to investigate whether moderate intensity aerobic exercise, such as that reaching 60% VO2max, effectively induces an inflammatory response. Furthermore, because our study focused on female hormone levels, we evaluated aromatase, which is an enzyme involved in the synthesis of sex hormones. In addition, we specifically evaluated PGF2α levels, which tend to increase considerably during the menstrual cycle, to investigate differences within the menstrual cycle, considering the possible contribution of endometrium-derived cfDNA. Hence, we aimed to determine (i) the cfDNA levels during the menstrual cycle and (ii) changes in cfDNA concentration during the menstrual cycles associated with exercise in individuals with regular menstrual cycles without exercise habits. The results of this study will clarify the effects of exercise on the menstrual cycle and establish the usefulness of biomarkers in exercise for women.
2 Materials and methods
2.1 Participants
Eleven female students (20–32 years old) enrolled at universities and graduate schools in Tokyo and Chiba prefecture were included in this study. The inclusion criteria were (i) no exercise habits within the last 6 months, (ii) regular menstrual cycle with ovulation, (iii) no oral contraceptive use, (iv) no smoking habit, and (v) no medical disease, trauma, disorder, or psychiatric disorder (30). In this study, women without a daily exercise habit were recruited to eliminate the effects of habitual moderate to high-intensity exercise. Individuals who did not meet these criteria and those who had problems with the measurements on the day of the test were excluded.
2.2 Study design
This observational study was conducted between October 2021 and March 2022. VO2max was measured once on the 7th to 14th day of menstruation, and exercise tests were performed once in each of the three phases of the subsequent menstrual cycle. All measurements were performed indoors. Before starting the study, its purpose, content, and the possibility of withdrawing were explained to the participants, and their informed consent to participate was obtained. This study was approved by the Research Ethics Committee of Japan Women's College of Physical Education (2021-7). All procedures were in accordance with the 1964 Helsinki Declaration and its later amendments.
2.3 Study procedure
The measurement procedure is illustrated in Figure 1. The basal body temperature of participants was measured every morning before and after waking from 2 months before the start of the study until the end. Based on the basal body temperature, menstrual cycle length (number of days), and ovulation detection using an ovulation test, we determined the regular menstrual cycle and schedule for the three exercise tests. For all measurements, alcohol and caffeine intake were prohibited on the day before the test. All exercise tests were performed between 7:00 and 9:00 a.m. On the morning of the exercise test, the body composition was measured prior, expiratory gases and RPE were measured during the exercise test, and venous blood samples were collected before and after the exercise test under fasting conditions.
2.4 Phases in the menstrual cycle
The menstrual cycle is divided into three phases: the menstrual phase (a period of menstrual bleeding, low basal body temperature, and low serum E2 and P4 levels), the ovulatory phase (a period of ovulation, high serum E2 levels, and low P4 levels), and the luteal phase (a period after ovulation to the next menstruation, high basal body temperature, and high serum E2 and P4 levels) (31).
In this study, based on the onset of menstruation, basal body temperature, serum hormone levels, and ovulation detected by ovulation testing, the menstrual phase was classified as days 1–4 from menstruation; the ovulatory phase as within 2 days, including the day ovulation was detected; and the luteal phase as 5–10 days before the next expected menstrual onset date.
2.5 Measurements
2.5.1 Physical characteristics
The height, weight, body water volume, and fat mass of the study participants were measured. Height was measured using a digital height meter (AD-6400; A&D Company, Tokyo, Japan), while weight, body water volume, and fat mass were measured using a body composition analyzer (InBody770; InBody Co., LTD, Seoul, Korea).
2.5.2 Basal body temperature
Basal body temperature was measured for 30 s every morning before and after awakening by sublingual thermometry using a gynecological thermometer (Terumo electronic thermometer, ET-W525DZ; Terumo Corporation, Tokyo, Japan). Data transfer was performed on the day the participants visited the laboratory for measurement.
2.5.3 Serum ovarian hormone concentration
Ovarian E2 and P4 levels were also measured. After measuring body composition, blood samples (8 ml) were collected from the median vein of the participants in a sitting position at rest immediately, 30 min, and 60 min after the exercise test was performed. Blood was centrifuged at 3,000 rpm for 15 min, and the serum was frozen at −80 °C for storage. Hormone concentrations were measured by chemiluminescent enzyme immunoassay using a mobile immunoluminescent assay device (PATHFAST; LSI Medience Corporation, Tokyo, Japan).
2.6 Exercise test and oxygen consumption
An aerobic bike (75XL III; Konami Co., Ltd., Tokyo, Japan) was used to determine the maximal oxygen uptake (VO2max) and perform the exercise stress test. The flow meter of the exhaled gas analyzer was calibrated before the start of the experimental day and before each participant's measurement. VO2max was measured at an intensity of 40 W at 60 rpm for 10 min as a warm-up after 3 min of sitting at rest. The exercise was terminated when the pedal speed could no longer be maintained at 50–60 rpm by ramp loading at 15 W/min (the participant was informed when the pedal speed was about to drop below 50 rpm, and after 5 s elapsed after the pedal speed dropped below 50 rpm). Exhaled gases during exercise were measured by the breath-by-breath method using a mass spectrometer for biogas analysis (ARCO-2000; Arcosystem, Chiba, Japan). VO2max was defined as the 1-min average until the maximum oxygen uptake was recorded (VO2peak) and divided by the participant's body weight. VO2max was calculated by dividing the VO2max by the body weight of the participant.
In the exercise test during the three periods of the menstrual cycle, after 3 min of sitting at rest on the ergometer, the participants warmed up for 10 min at an intensity of 40 W and 60 rpm. Subsequently, each participant performed a pedaling exercise for 30 min at 60% VO2max intensity. The exhaled gases were measured at rest for 3 min, 2 min after 8–10 min of exercise at 60% VO2max, 2 min after 18–20 min, and 2 min after 28–30 min. In this study, we targeted individuals with no regular exercise habits and regular menstrual cycles to eliminate the bias of daily physical activity on parameters such as cfDNA concentration and pro-inflammatory markers. Consequently, the exercise intervention was conducted at a moderate-intensity aerobic level, specifically at 60% VO2max, considering the safety of the participants and the intensity at which cfDNA concentrations tend to increase (29).
2.7 Cell-free DNA
In this study, plasma samples were used because serum samples may contain cfDNA (32, 33) derived from leukocytes that are dissolved during blood coagulation. After measuring body composition, blood samples (2 ml) were collected from the participant's median vein in a sitting position at rest immediately, 30 min, and 60 min after the exercise test. The cfDNA was extracted using a Plasma/Serum Cell-Free Circulating DNA Purification Mini Kit (Cat. 55100; Norgen Biotek Corp., Thorold, ON, Canada). In total, 40 μl of cfDNA was extracted from 500 μl of plasma according to the manufacturer's protocol. DNA purity was determined using a NanoDrop One Ultra-trace Spectrophotometer (Thermo Fisher Scientific, Waltham, MA, USA).
A High Sensitivity DNA Kit (Cat. 5067-4626; Agilent Technologies, Santa Clara, CA, USA) and a 2100 Bioanalyzer (Agilent Technologies) were used for cfDNA analysis. The analysis was performed in accordance with the manufacturer's instructions. One microliter of the extracted cfDNA sample was used for quantitative analysis.
2.8 Sex hormone-synthesizing enzyme
Sex hormones are produced from cholesterol by modifying various sex hormone synthase enzymes (34). E2, a female hormone, is converted from the male hormone testosterone by aromatase (P450arom: aromatase). In this study, we measured blood aromatase concentrations to examine the effects of exercise on cfDNA concentrations and other inflammatory parameters at different levels of female hormones. A Human ARO (aromatase) ELISA Kit (Cat. EH2665; Wuhan Fine Biotech Co., Ltd., Wuhan, China) was used to measure aromatase activity. The assay was performed according to the product protocol, and 100 μl of a 2-fold diluted serum sample was used. The absorbance was measured at 450 nm using a Varioskan LUX Multimode Microplate Reader (Thermo Fisher Scientific). A four-parameter logistic (4PL) curve was generated using ImageJ (NIH, Bethesda, MD, USA) from the absorbance of the standard solution, and the concentration of aromatase in each serum sample was calculated.
2.9 Inflammatory response
In parallel with cfDNA, IL-6, and PGF2α levels were measured to confirm the inflammatory response induced by exercise. IL-6 was measured using Human IL-6 Quantikine HS ELISA (Cat.HS600C; R&D Systems, Minneapolis, MN, USA). The assay was performed according to the product protocol, and 100 μl of serum sample was used without dilution. The absorbance was measured at 450 nm using a Varioskan LUX Multimode Microplate Reader (Thermo Fisher Scientific). A 4PL curve was generated from the absorbance of the standard solution using ImageJ, and the level of IL-6 in each serum sample was calculated. PGF2α was measured using a Prostaglandin F2 alpha ELISA Kit (Cat.KA0310; Abnova, Taipei, Taiwan). The manufacturer's instructions were followed, and 100 μl of a 10-fold diluted serum sample was used. The absorbance was measured at 405 nm using a Varioskan LUX Multimode Microplate Reader (Thermo Fisher Scientific). The binding rates of the standard solution and each serum sample were calculated, and a 4PL curve was generated from the binding rates of the standard solution using ImageJ. The PGF2α concentration in each serum sample was calculated.
2.10 Statistical analysis
All results of this study are presented as the mean ± standard deviation. Two-way repeated measures analysis of variance (RM-ANOVA) was performed to compare the three different phases over the four time points (Pre, Post 0 min, Post 30 min, and Post 60 min before and after exercise). The Bonferroni multiple testing correction method was used to adjust p-values.
The Spearman's rank correlation coefficient was used to examine the relationship between Pre-to-Post 0 changes (%baseline) in the progesterone to estradiol level ratio (P4/E2) and cfDNA, IL-6, aromatase, and PGF2α. Associations between cfDNA and each hormone over time and as well as the associations between %changes in cfDNA and in each hormone were assessed based on the basis of the repeated measurement correlation coefficient.
SPSS ver. 27 (SPSS Inc., Chicago, IL, USA) and R version 4.2.2 (https://www.r-project.org/) with the rstatix, rmcorr, and pwr package (35) were used for statistical analysis. The statistical significance level was set at p < 0.05.
3 Results
Eleven sedentary female students were enrolled in the study, and seven were included in the final analysis, except for one who withdrew during the experiment and three who showed serum P4 levels <10 ng/ml in the luteal phase.
3.1 Basic characteristics
Oxygen uptake significantly increased at 8–10, 18–20, and 28–30 min of exercise compared with that observed in Pre, with values averaging approximately 29–31 ml/min/kg over the three phases. No significant differences were observed between phases at all time points. The mean VO2max was 45.3 ± 3.3 (ml/min/kg).
Table 1 presents the baseline characteristics of the seven participants. No significant differences were observed in the body weight, water, or fat mass during the menstrual, ovulatory, and luteal phases.
Serum E2 and P4 concentrations before and after exercise are presented in Figures 2A, B. No statistically significant interactions were observed between both hormones. Significant main effects in the phase (p = 0.008) and time (p = 0.003) were observed for the serum E2 level (Figures 2A). Comparisons made using the pairwise paired t-test showed that exercise had a significant incremental effect on E2 levels at Post 0 (p = 0.005), and significant decreases were observed after exercise (p = 0.002 for Post 60 vs. Post 0, p = 0.04 for Post 60 vs. Post 30). Furthermore, comparisons made using pairwise paired t-test showed significant differences between the menstrual and ovulatory phases (p < 0.001), menstrual and luteal phases (p = 0.029), and ovulatory and luteal phases (p < 0.001), respectively. Furthermore, the main effect of the phase was significant for serum P4 levels (p = 0.004) (Figure 2B). Comparisons made using pairwise paired t-test showed significant differences between the menstrual and ovulatory phases (p < 0.001), menstrual and luteal phases (p < 0.001), and ovulatory and luteal phases (p < 0.001), respectively.
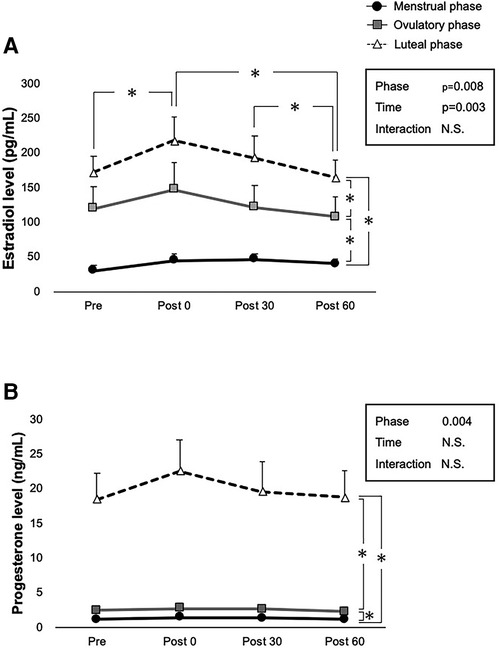
Figure 2. Ovarian hormone levels at each time point over three phases. Interactions of time and phases are evaluated using two-way ANOVA for (A) E2 concentration, and (B) P4 concentration in pre-exercise (Pre), immediately after exercise (Post 0), 30 min after exercise (Post 30), and 60 min after exercise (Post 60). Menstrual, ovulatory, and luteal phases are represented by black circles with black lines, gray squares with gray lines, and white triangles with dashed lines, respectively. B indicates p < 0.05 between time points in the ovulatory phase, and c in the luteal phase. *indicates p < 0.05 between time points and phases according to the Bonferroni post-hoc test.
3.2 cfDNA levels
The cfDNA concentrations before and after exercise are shown in Figure 3. No statistically significant interaction was observed between phase and time on cfDNA levels (p = 0.327); however, the main effect of time was significant (p = 0.010). Comparisons using pairwise paired t-test showed that exercise had a significant incremental effect on cfDNA levels at Post 0 (p = 0.002) but not at Post 30 (p = 0.302) and Post 60 (p = 0.954). Significant decreases in cfDNA were observed between all time points after exercise (p < 0.001 for Post 30 vs. Post 0, Post 60 vs. Post 0, and Post 60 vs. Post 30). The main effect of phase was not significant (p = 0.814).
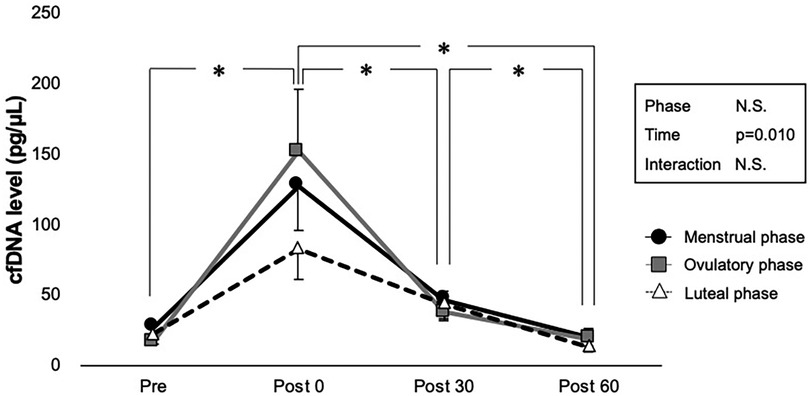
Figure 3. Interaction of time and phases are shown by two-way ANOVA for cfDNA level. Menstrual, ovulatory, and luteal phases are represented in black circles with black lines, gray squares with gray lines, and white triangles with dashed lines, respectively. *Indicates p < 0.05 between time points according to the Bonferroni post hoc test.
3.3 PGF2α, IL-6, and aromatase levels
PGF2α, IL-6, and aromatase levels before and after exercise are shown in Figures 4A–C. No statistically significant interaction was observed between phase and time on these hormone levels (p = 0.277 for PGF2α, p = 0.058 for IL-6, and p = 0.432 for aromatase); however, the main effect of time was significant in each PGF2α (p = 0.001), IL-6 (p = 0.009), and aromatase (p < 0.001). The main effect of phase was not significant (p = 0.071 for PGF2α, p = 0.324 for IL-6, and p = 0.237 for aromatase). Comparisons using pairwise paired t-test showed a significant decrease in PGF2α in Pre to Post 60 (p = 0.026), Post 0 to Post 30 (p = 0.030), and Post 0 to Post 60 (p < 0.001).
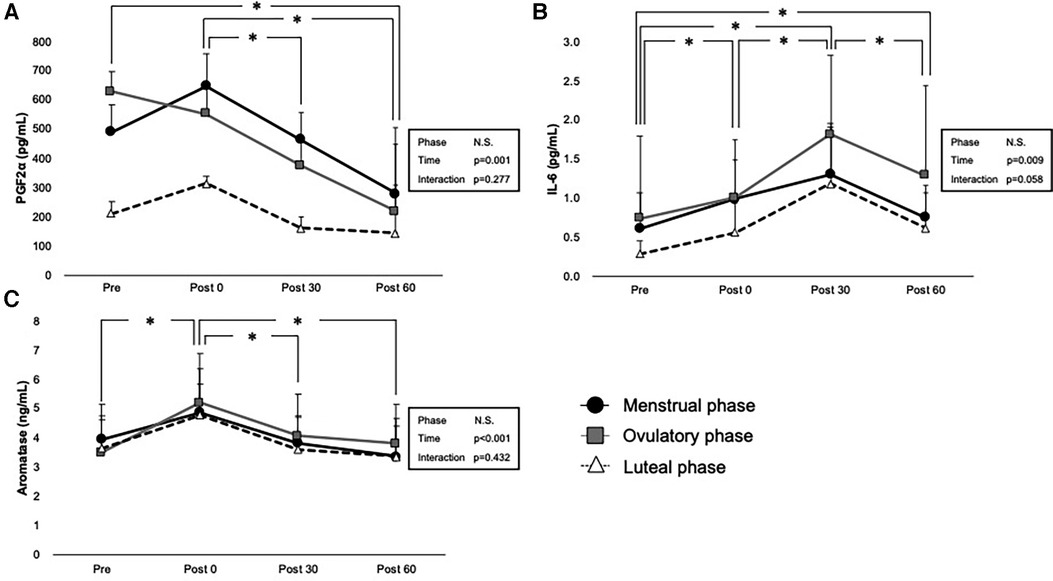
Figure 4. Interaction of time and phases are shown by two-way ANOVA for (A) PGF2a, (B) IL-6, and (C) aromatase levels. Menstrual, ovulatory phase, and luteal phases are represented in black circles with black lines, gray squares with gray lines, and white triangles with dashed lines, respectively. * indicates p < 0.05 between time points according to the Bonferroni post hoc test.
Exercise had a significant incremental effect on IL-6 levels at Post 0 (p = 0.007), Post 30 (p < 0.001), and Post 60 (p = 0.032), showing the peak level on Post 30 (p < 0.001 for Post 0 vs. Post 30, p < 0.001 for Post 30 vs. Post 60).
A significant increase in aromatase was observed immediately after exercise (p < 0.001 for Pre vs. Post 0), and it significantly decreased after exercise (p < 0.001 for Post 0 vs. Post 30) and Post 60 (p < 0.001 for Post 0 vs. Post 30).
3.4 Correlation with cfDNA
Table 2 shows the repeated measures correlation between cfDNA and each hormone over the three phases, and Table 3 shows the repeated measures correlation between changes in cfDNA and each hormone over the three phases. Table 4 shows the correlation between Pre-to-Post 0 changes (%baseline) in P4/E2 and cfDNA levels over 3 phases.
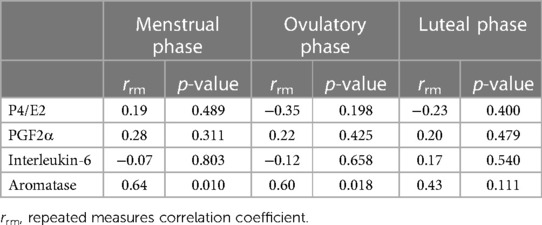
Table 3. Repeated measures correlation between changes (%baseline) in cfDNA and each hormone over three phases.
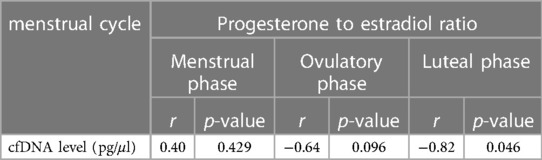
Table 4. Correlation between Pre-to-post 0 changes (%baseline) in P4/E2 and cfDNA over three phases.
According to Table 2 and Figure 5, the results indicate the association between cfDNA levels and each hormone. The association between P4/E2 and cfDNA was observed in the ovulatory and luteal phases (ovulatory phase; rrm = −0.56, p = 0.007, statistical power = 0.80, luteal phase; rrm = −0.45, p = 0.038, statistical power = 0.57); however, no association was found in the menstrual phase (p = 0.757, statistical power = 0.06). Moreover, point estimates of the association between PGF2α and cfDNA were higher in the menstrual phase than in the ovulatory and luteal phases. Aromatase was associated with cfDNA in all three phases, showing a significant positive correlation (menstrual phase: rrm = 0.52, p = 0.013, statistical power = 0.73; ovulatory phase: rrm = 0.61, p = 0.002, statistical power = 0.89; and luteal phase: rrm = 0.63, p = 0.002, statistical power = 0.91).
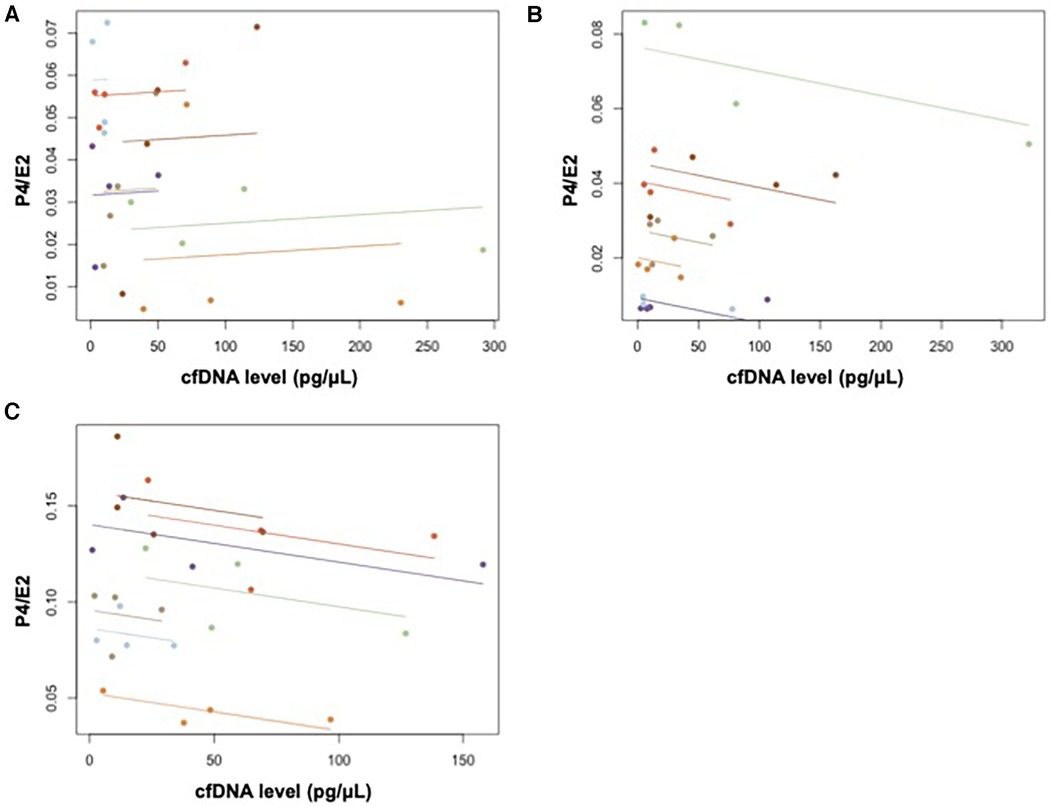
Figure 5. Repeated measures correlation between cfDNA and P4/E2 in (A) menstrual, (B) ovulatory, and (C) luteal phases.
According to Table 3, the results of the association between changes (%baseline) in cfDNA levels and each hormone showed that P4/E2, PGF2a, and IL-6 were not significantly associated with cfDNA over the three phases. However, aromatase was associated with cfDNA in both menstrual and ovulatory phases, showing a significant positive correlation (menstrual phase: rrm = 0.64, p = 0.010, statistical power = 0.92; ovulatory phase: rrm = 0.60, p = 0.018, statistical power = 0.87), but not in the luteal phase.
A significant negative correlation was observed between the Pre- to-Post 0%changes in P4/E2 and cfDNA levels in the luteal phase as shown in Table 4 (r = −0.82, p = 0.046, statistical power = 0.58).
4 Discussion
This study investigated the levels of circulating cfDNA before and after exercise during three distinct phases of the menstrual cycle: menstrual (when both ovarian hormone levels are low), ovulatory (characterized by elevated estrogen levels), and luteal phases (when both hormones are at high levels). In this study, we determined the resting cfDNA levels during the menstrual cycle and changes in cfDNA levels during the cycle in individuals with a regular menstrual cycle. Our results showed that the baseline values did not change significantly during the menstrual cycle, and exercise-induced changes in cfDNA levels and related blood markers may be associated with female hormone levels.
The mean VO2max in this study was 45.3 ± 3.3 (ml/min/kg), 60% of which amounts to 27.2 (ml/min/kg). The oxygen uptake results suggest that the exercise load in this study was equivalent to 70% VO2max in this study. This exercise intensity, at 70% of VO2max, corresponds to a moderate-intensity exercise. Considering that athletes frequently reach this intensity (36), it can be concluded that a practical intensity was used for the purposes of this study.
No significant main effect of the phase was observed for cfDNA in this study, which is consistent with previous studies showing no significant variation in resting cfDNA levels during the menstrual cycle (23, 24). Nevertheless, Pölcher et al. omitted the luteal phase during the documented increase in P4 concentration (23), and Yuwono et al. (24) incorporated individuals who were smokers or who used low-dose estrogen-progestin combination (LEP) preparations, rendering it challenging to infer conclusions regarding relationships within the menstrual cycle in a resting state.
In this study, pairwise paired t-test comparisons showed that exercise had a significant incremental effect on cfDNA levels at Post 0, indicating the influence of exercise load. cfDNA concentrations reportedly increased up to five-fold from resting levels in an exercise stress test using all-out cycling (16, 17), suggesting that a certain level of exercise stress influenced cfDNA concentrations in the current study.
Aromatase level showed a significant positive correlation with the cfDNA level in the repeated measures correlation across the three phases. The expression of aromatase increases immediately after exercise (37), and aromatase is expressed in skeletal muscle, where it synthesizes estrogen via dehydroepiandrosterone (DHEA) (38, 39). Through repeated measures correlation, a significant negative correlation was observed between P4/E2 and cfDNA in the ovulatory and luteal phases, as well as in the %changes between Pre to Post 0 in the luteal phase. The ovulatory phase is characterized by elevated estradiol levels, while the luteal phase has high levels of both estradiol and progesterone. Therefore, compared to the menstrual phase with low hormone levels, the increase in cfDNA concentration due to exercise may be attributed to the individual effect of estradiol and the combined effects of both hormones.
The repeated measures correlation between PGF2α and cfDNA concentration during the menstrual phase had a higher point estimate than those during the other phases (rrm = 0.38, p = 0.078). None of the study participants reported menstrual symptoms severe enough to interfere with measurements. Notably, a significant increase was only observed during the menstrual phase when compared before and immediately after exercise (p = 0.042, data not shown). PGF2α causes menstrual pain, and heightened production of PGF2α has been linked to the heightened sensitivity of uterine muscle fibers, a consequence of impaired blood flow resulting from vigorous contractions of the myometrium (40, 41). Therefore, it can be hypothesized that an increase in cfDNA concentration during menstruation may be observed in women with severe menstrual pain, such as those with dysmenorrhea. Further investigation is necessary to explore this relationship.
The sample size in this study is small (n = 7). While this may be a limitation, the assessment of cfDNA secretion before and after exercise during the menstrual, ovulatory, and luteal phases based on the evaluation of female hormone concentrations provides preliminary insights for future studies targeting athletes. These findings are considered the minimum knowledge base for future investigations in this population.
Based on the findings of this study, we propose that future research should focus on individuals who engage in regular exercise or athletes. Even when similar exercises are performed, differences in the dynamics of cfDNA before and after exercise have been reported depending on the presence or absence of exercise habits (42). This may involve the potential influence of psychological stress (29), and elevated cfDNA concentrations can persist for 2–3 days after prolonged exercise loads (43, 44), suggesting potential differences compared to transient exercise. Additionally, our study revealed that high levels of female hormones associated with exercise, as well as the effect of PGF2α on uterine contractions, can impact cfDNA. Therefore, future research must consider the effects of menstrual symptoms and menstrual status (such as oligomenorrhea and amenorrhea). Menstrual irregularities, particularly rare or absent menstruation, are not uncommon among athletes, and these individuals may have a reduced influence from female hormones. As a future prospect, we aim to include individuals with different menstrual statuses to gain a better understanding of the relationship between female hormones and cfDNA dynamics during exercise.
To our knowledge, this study is the first to investigate the levels of circulating cfDNA before and after exercise during three distinct phases of the menstrual cycle: the menstrual (when both ovarian hormone levels are low), ovulatory (characterized by elevated estrogen levels), and luteal phases (when both hormones are at high levels). This research may offer novel insights into exercise physiology in relation to cfDNA, a biomarker that immediately responds to exercise, and its interaction with ovarian hormone concentrations.
5 Conclusions
When the female menstrual cycle is considered, resting cfDNA levels are unlikely to be affected. However, the dynamics of cfDNA through acute moderate-intensity exercise may be influenced by the ratio of ovarian hormone concentrations.
Data availability statement
The original contributions presented in the study are included in the article, further inquiries can be directed to the corresponding author/s.
Ethics statement
The studies involving humans were approved by the Research Ethics Committee of Japan Women's College of Physical Education (2021-7) and all procedures were in accordance with the 1964 Helsinki Declaration and its later amendments. The studies were conducted in accordance with the local legislation and institutional requirements. The participants provided their written informed consent to participate in this study.
Author contributions
AS: Conceptualization, Data curation, Formal Analysis, Investigation, Methodology, Project administration, Software, Validation, Visualization, Writing – original draft, Writing – review & editing. TS: Conceptualization, Data curation, Formal Analysis, Investigation, Methodology, Software, Validation, Visualization, Writing – original draft, Writing – review & editing. YH: Investigation, Methodology, Writing – review & editing. SH: Formal Analysis, Writing – review & editing. MU: Investigation, Writing – review & editing. YK: Investigation, Writing – review & editing. KT: Formal Analysis, Methodology, Validation, Writing – review & editing. HN: Investigation, Writing – review & editing. YK: Funding acquisition, Resources, Writing – review & editing. KT: Funding acquisition, Project administration, Resources, Supervision, Validation, Visualization, Writing – review & editing.
Funding
The author(s) declare financial support was received for the research, authorship, and/or publication of this article.
This study was supported by the JSPS KAKENHI (grant number: JP 23H03278).
Acknowledgments
We would like to thank the participants for their cooperation and the collaborative faculty members for recruiting the participants.
Conflict of interest
The authors declare that the research was conducted in the absence of any commercial or financial relationships that could be construed as a potential conflict of interest.
Publisher's note
All claims expressed in this article are solely those of the authors and do not necessarily represent those of their affiliated organizations, or those of the publisher, the editors and the reviewers. Any product that may be evaluated in this article, or claim that may be made by its manufacturer, is not guaranteed or endorsed by the publisher.
References
1. Japan Sports Agency. Results of Public Opinion Survey on the Status of Sports in Fiscal 2021 (in Japanese). Available online at: Available at: https://www.mext.go.jp/sports/content/20220222-spt_kensport01-000020451_1.pdf (accessed October 3, 2023).
2. Allen J, Sun Y, Woods JA. Exercise and the regulation of inflammatory responses. Prog Mol Biol Transl Sci. (2015) 135:337–54. doi: 10.1016/bs.pmbts.2015.07.003
3. Hennigar SR, McClung JP, Pasiakos SM. Nutritional interventions and the IL-6 response to exercise. FASEB J. (2017) 31:3719–28. doi: 10.1096/fj.201700080R
4. Shaw DM, Merien F, Braakhuis A, Dulson D. T-cells and their cytokine production: the anti-inflammatory and immunosuppressive effects of strenuous exercise. Cytokine. (2018) 104:136–42. doi: 10.1016/j.cyto.2017.10.001
5. Sterczala AJ, Fry AC, Chiu LZF, Schilling BK, Weiss LW, Nicoll JX. β2-adrenergic receptor maladaptations to high power resistance exercise overreaching. Hum Physiol. (2017) 43:446–54. doi: 10.1134/S0362119717040144
6. Draganidis D, Chatzinikolaou A, Jamurtas AZ, Carlos Barbero J, Tsoukas D, Theodorou AS, et al. The time-frame of acute resistance exercise effects on football skill performance: the impact of exercise intensity. J Sports Sci. (2013) 31:714–22. doi: 10.1080/02640414.2012.746725
7. Fedewa MV, Hathaway ED, Ward-Ritacco CL. Effect of exercise training on C reactive protein: a systematic review and meta-analysis of randomised and non-randomised controlled trials. Br J Sports Med. (2017) 51:670–6. doi: 10.1136/bjsports-2016-095999
8. Atamaniuk J, Kopecky C, Skoupy S, Säemann MD, Weichhart T. Apoptotic cell-free DNA promotes inflammation in haemodialysis patients. Nephrol Dial Transplant. (2012) 27:902–5. doi: 10.1093/ndt/gfr695
9. Borissoff JI, Joosen IA, Versteylen MO, Brill A, Fuchs TA, Savchenko AS, et al. Elevated levels of circulating DNA and chromatin are independently associated with severe coronary atherosclerosis and a prothrombotic state. Arterioscler Thromb Vasc Biol. (2013) 33:2032–40. doi: 10.1161/ATVBAHA.113.301627
10. Jung K, Fleischhacker M, Rabien A. Cell-free DNA in the blood as a solid tumor biomarker—a critical appraisal of the literature. Clin Chim Acta. (2010) 411:1611–24. doi: 10.1016/j.cca.2010.07.032
11. Ziegler A, Zangemeister-Wittke U, Stahel RA. Circulating DNA: a new diagnostic gold mine? Cancer Treat Rev. (2002) 28:255–71. doi: 10.1016/S0305-7372(02)00077-4
12. Atamaniuk J, Stuhlmeier KM, Vidotto C, Tschan H, Dossenbach-Glaninger A, Mueller MM. Effects of ultra-marathon on circulating DNA and mRNA expression of pro- and anti-apoptotic genes in mononuclear cells. Eur J Appl Physiol. (2008) 104:711–7. doi: 10.1007/s00421-008-0827-2
13. Tug S, Tross AK, Hegen P, Neuberger EWI, Helmig S, Schöllhorn W, et al. Acute effects of strength exercises and effects of regular strength training on cell free DNA concentrations in blood plasma. PLoS One. (2017a) 12:e0184668. doi: 10.1371/journal.pone.0184668
14. Atamaniuk J, Vidotto C, Kinzlbauer M, Bachl N, Tiran B, Tschan H. Cell-free plasma DNA and purine nucleotide degradation markers following weightlifting exercise. Eur J Appl Physiol. (2010) 110:695–701. doi: 10.1007/s00421-010-1532-5
15. Mavropalias G, Calapre L, Morici M, Koeda T, Poon WCK, Barley OR, et al. Changes in plasma hydroxyproline and plasma cell-free DNA concentrations after higher- versus lower-intensity eccentric cycling. Eur J Appl Physiol. (2021) 121:1087–97. doi: 10.1007/s00421-020-04593-1
16. Brahmer A, Neuberger E, Esch-Heisser L, Haller N, Jorgensen MM, Baek R, et al. Platelets, endothelial cells and leukocytes contribute to the exercise-triggered release of extracellular vesicles into the circulation. J Extracell Vesicles. (2019) 8:1615820. doi: 10.1080/20013078.2019.1615820
17. Tug S, Mehdorn M, Helmig S, Breitbach S, Ehlert T, Simon P. Exploring the potential of cell-free-DNA measurements after an exhaustive cycle-ergometer test as a marker for performance-related parameters. Int J Sports Physiol Perform. (2017b) 12:597–604. doi: 10.1123/ijspp.2016-0157
18. Alghofaili L, Almubarak H, Gassem K, Islam SS, Coskun S, Kaya N, et al. Cell-free DNA levels of twins and sibling pairs indicate individuality and possible use as a personalized biomarker. PLoS One. (2019) 14:e0223470. doi: 10.1371/journal.pone.0223470
19. Çayir A, Coskun M, Coskun M, Cobanoglu H. DNA Damage and circulating cell free DNA in greenhouse workers exposed to pesticides. Environ Mol Mutagen. (2018) 59:161–9. doi: 10.1002/em.22148
20. Jylhävä J, Jylhä M, Lehtimäki T, Hervonen A, Hurme M. Circulating cell-free DNA is associated with mortality and inflammatory markers in nonagenarians: the vitality 90+ study. Exp Gerontol. (2012) 47:372–8. doi: 10.1016/j.exger.2012.02.011
21. Yuwono NL, Warton K, Ford CE. The influence of biological and lifestyle factors on circulating cell-free DNA in blood plasma. eLife. (2021a) 10:e69679. doi: 10.7554/eLife.69679
22. Stawski R, Walczak K, Kosielski P, Meissner P, Budlewski T, Padula G, et al. Repeated bouts of exhaustive exercise increase circulating cell free nuclear and mitochondrial DNA without development of tolerance in healthy men. PLoS One. (2017) 12:e0178216. doi: 10.1371/journal.pone.0178216
23. Pölcher M, Ellinger J, Willems S, El-Maarri O, Höller T, Amann C, et al. Impact of the menstrual cycle on circulating cell-free DNA. Anticancer Res. (2010) 30:2235–40. PMID: 20651374.
24. Yuwono NL, Henry CE, Ford CE, Warton K. Total and endothelial cell-derived cell-free DNA in blood plasma does not change during menstruation. PLoS One. (2021b) 16:e0250561. doi: 10.1371/journal.pone.0250561
25. Cook CJ, Kilduff LP, Crewther BT. Basal and stress-induced salivary testosterone variation across the menstrual cycle and linkage to motivation and muscle power. Scand J Med Sci Sports. (2018) 28:1345–53. doi: 10.1111/sms.13041
26. Dokumacı B, Hazır T. Effects of the menstrual cycle on running economy: oxygen cost versus caloric cost. Res Q Exerc Sport. (2019) 90:318–26. doi: 10.1080/02701367.2019.1599800
27. Graja A, Kacem M, Hammouda O, Borji R, Bouzid MA, Souissi N, et al. Physical, biochemical, and neuromuscular responses to repeated sprint exercise in eumenorrheic female handball players: effect of menstrual cycle phases. J Strength Cond Res. (2022) 36:2268–76. doi: 10.1519/JSC.0000000000003556
28. Greenhall M, Taipale RS, Ihalainen JK, Hackney AC. Influence of the menstrual cycle phase on marathon performance in recreational runners. Int J Sports Physiol Perform. (2021) 16:601–4. doi: 10.1123/ijspp.2020-0238
29. Haller N, Tug S, Breitbach S, Jörgensen A, Simon P. Increases in circulating cell-free DNA during aerobic running depend on intensity and duration. Int J Sports Physiol Perform. (2017) 12:455–62. doi: 10.1123/ijspp.2015-0540
30. Hummel EM, Hessas E, Müller S, Beiter T, Fisch M, Eibl A, et al. Cell-free DNA release under psychosocial and physical stress conditions. Transl Psychiatry. (2018) 8:236. doi: 10.1038/s41398-018-0264-x
31. Farage MA, Neill S, MacLean AB. Physiological changes associated with the menstrual cycle: a review. Obstet Gynecol Surv. (2009) 64:58–72. doi: 10.1097/OGX.0b013e3181932a37
32. Warton K, Lin V, Navin T, Armstrong NJ, Kaplan W, Ying K, et al. Methylation-capture and next-generation sequencing of free circulating DNA from human plasma. BMC Genomics. (2014) 15:476. doi: 10.1186/1471-2164-15-476
33. Trigg RM, Martinson LJ, Parpart-Li S, Shaw JA. Factors that influence quality and yield of circulating-free DNA: a systematic review of the methodology literature. Heliyon. (2018) 4(7):e00699. doi: 10.1016/j.heliyon.2018.e00699
34. Labrie F. Intracrinology. Mol Cell Endocrinol. (1991) 78:C113–8. doi: 10.1016/0303-7207(91)90116-A
35. Bakdash JZ, Marusich LR. Repeated measures correlation. Front Psychol. (2017) 8:456. doi: 10.3389/fpsyg.2017.00456
36. Mitchell JH, Haskell W, Snell P, Van Camp SP. Task force 8: classification of sports. J Am Coll Cardiol. (2005) 45(8):1364–7. doi: 10.1016/j.jacc.2005.02.015
37. Shi R, Tian X, Feng Y, Cheng Z, Lu J, Brann DW, et al. Expression of aromatase and synthesis of sex steroid hormones in skeletal muscle following exercise training in ovariectomized rats. Steroids. (2019) 143:91–6. doi: 10.1016/j.steroids.2019.01.003
38. Aizawa K, Iemitsu M, Maeda S, Jesmin S, Otsuki T, Mowa CN, et al. Expression of steroidogenic enzymes and synthesis of sex steroid hormones from DHEA in skeletal muscle of rats. Am J Physiol Endocrinol Metab. (2007) 292:E577–84. doi: 10.1152/ajpendo.00367.2006
39. Sato K, Iemitsu M, Aizawa K, Ajisaka R. Testosterone and DHEA activate the glucose metabolism-related signaling pathway in skeletal muscle. Am J Physiol Endocrinol Metab. (2008) 294:E961–8. doi: 10.1152/ajpendo.00678.2007
40. Jabbour HN, Sales KJ, Smith OP, Battersby S, Boddy SC. Prostaglandin receptors are mediators of vascular function in endometrial pathologies. Mol Cell Endocrinol. (2006) 252:191–200. doi: 10.1016/j.mce.2006.03.025
41. Ito S, Okuda-Ashitaka E, Minami T. Central and peripheral roles of prostaglandins in pain and their interactions with novel neuropeptides nociceptin and nocistatin. Neurosci Res. (2001) 41:299–332. doi: 10.1016/s0168-0102(01)00289-9
42. Humińska-Lisowska K, Mieszkowski J, Kochanowicz A, Stankiewicz B, Niespodziński B, Brzezińska P, et al. cfDNA changes in maximal exercises as a sport adaptation predictor. Genes (Basel). (2021) 12:1238. doi: 10.3390/genes12081238
43. Fatouros IG, Destouni A, Margonis K, Jamurtas AZ, Vrettou C, Kouretas D, et al. Cell-free plasma DNA as a novel marker of aseptic inflammation severity related to exercise overtraining. Clin Chem. (2006) 52:1820–4. doi: 10.1373/clinchem.2006.070417
44. Margeli A, Skenderi K, Tsironi M, Hantzi E, Matalas AL, Vrettou C, et al. Dramatic elevations of interleukin-6 and acute-phase reactants in athletes participating in the ultradistance foot race spartathlon: severe systemic inflammation and lipid and lipoprotein changes in protracted exercise. J Clin Endocrinol Metab. (2005) 90:3914–8. doi: 10.1210/jc.2004-2346
Keywords: cfDNA, menstrual cycle, exercise, ovulation, progesterone
Citation: Sawai A, Shida T, Hoshikawa Y, Hatanaka S, Ueda M, Kato Y, Tokinoya K, Natsui H, Kawakami Y and Takekoshi K (2024) Effect of acute moderate-intensity cycling on cfDNA levels considering menstrual cycle phases. Front. Sports Act. Living 6:1322295. doi: 10.3389/fspor.2024.1322295
Received: 16 October 2023; Accepted: 15 January 2024;
Published: 29 January 2024.
Edited by:
David Morales-Alamo, University of Las Palmas de Gran Canaria, SpainReviewed by:
Julia Maria Kröpfl, University of Basel, SwitzerlandChun-Jung Huang, Florida Atlantic University, United States
© 2024 Sawai, Shida, Hoshikawa, Hatanaka, Ueda, Kato, Tokinoya, Natsui, Kawakami and Takekoshi. This is an open-access article distributed under the terms of the Creative Commons Attribution License (CC BY). The use, distribution or reproduction in other forums is permitted, provided the original author(s) and the copyright owner(s) are credited and that the original publication in this journal is cited, in accordance with accepted academic practice. No use, distribution or reproduction is permitted which does not comply with these terms.
*Correspondence: Kazuhiro Takekoshi ay10YWtlbWRAbWQudHN1a3ViYS5hYy5qcA==
†These authors have contributed equally to this work