- Canadian Centre for Rural and Agricultural Health, College of Medicine, University of Saskatchewan, Saskatoon, SK, Canada
Introduction: Inertial measurement units allow for quantitative assessment of body motion in many environments. Determining the ability to measure upper limb motion with inertial measurement units, leveraging procedures traditionally used in the lab such as scapular calibration procedures and humeral axial rotation calculation, would expand the opportunities to assess upper limb function in externally valid environments. This study examined if humeral and scapular motion measured in different field settings is consistent with motion measured in a lab setting in similar tasks.
Methods: Twenty-eight adults participated in the study (14 field setting, 14 lab setting). Three different types of field settings were included: home (n = 5), work (n = 4), and sports (n = 5). Field participants were matched to lab participants based on sex and body height. All participants were equipped with five inertial and magnetic measurement units (Xsens Awinda, Xsens Technlogies, NL, Fs = 100 Hz) on the torso, humeri, and scapulae. Humeral and scapular angles were measured during a functional task protocol consisting of seven tasks. Data from all three field settings were combined. Statistical parametric mapping (α = .05) was used to assess differences in waveforms between the lab and field data.
Results and discussion: Five out of seven tasks displayed no differences for humeral elevation and humeral axial rotation, while scapular upward rotation and tilt were not statistically different for any tasks. Scapular internal rotation variability was very high for the field setting, but not for the lab setting. Task-based differences in humeral elevation and humeral axial rotation may be related to equipment modifications for the field protocol and between subjects' variability in task performance. Data indicate that humeral elevation, humeral axial rotation, and scapular upward rotation can be measured in externally valid field settings, which is promising for the evaluation of upper limb movement in natural environments.
1 Introduction
Quantitative scapular and humeral motion assessments are required to understand shoulder movement and upper limb abilities. Scapular and humeral motion are associated with shoulder injury risk (1–3), and shoulder injuries are prevalent in both the general population (4, 5) and athletic populations (6–8), necessitating further research into how the scapulae and humeri move in daily life. Inertial and magnetic measurement units (IMMUs) present a unique opportunity for quantitative assessment of scapular and humeral motion during functional movements in real-world settings, such as at work or during sport. However, there is a research gap regarding the use of IMMUs to measure humeral and scapular motion outside of the research laboratory. While some in-field work-specific assessments of posture or kinematics have been done for the upper arm (9–12), these assessments generally do not include humeral axial rotation or scapular motion, despite their importance to upper limb function and injury (13). Indeed, even in research lab settings, healthy and pathological scapular kinematics are most frequently measured during planar arm elevation (2, 14–20). To our knowledge, there exists no in-field measurement of the scapula during functional tasks, and humeral assessments are almost solely focused on arm elevation exposures (9–12, 21). Improved measurement of upper limb postures, including externally valid in-field assessments, could better inform interventions to prevent or address upper limb musculoskeletal disorders.
Best practices for IMMU use for measuring the humeri and scapulae during lab-based planar arm elevation have been previously defined (22–24). Recent research from our research group has validated the use of IMMUs for measuring both scapular movement (25) and humeral axial rotation (26) during a range of functional and work-related tasks. However, these studies have been conducted exclusively in lab settings, despite the portability of the IMMUs and their potential for in-field applications. These procedures differ from typical in-field motion capture set-up or analysis (27) due to external calibration tools for scapular tracking (23, 25) and calculations of rotation about the long axis of the arm (25, 28), which may be difficult to implement with field work. IMMUs can also be prone to drift or magnetic disturbance (29, 30); therefore, identifying if IMMUs and the necessary measurement and analysis protocol for measuring humeral and scapular motion can be applied to different field settings is necessary to confirm the utility of these methods or to identify potential sources of error that need to be addressed.
The primary objective of this study was to determine how humeral and scapular motion measured with IMMUs in different field settings (home, work, sport) compares to motion measured in a lab setting (26, 31). It was anticipated that the IMMUs will be able to successfully and accurately measure humeral and scapular kinematics in all in-field settings, as demonstrated by no difference in kinematic outcomes, as well as similar shape and magnitude of waveforms, between the field settings and the lab setting.
2 Methods
2.1 Settings
Three settings were used to test the in-field application of IMMUs for humeral and scapular motion capture: work, home, and sport. These settings were chosen to represent different areas of in-field motion capture application. For the work setting, data were collected in workshops or garages at farms or acreages. For the home setting, data were collected in participants' homes. For the sport setting, data were collected at a local outdoor baseball diamond. It is possible that different settings could have different environmental disturbances or practical data collection challenges, necessitating the testing in more than one field setting. For example, the work setting may cause more sensor disturbance from the surrounding equipment and tools, while the sport setting could present practical challenges regarding consistent sensor placement and computer operation due to the elements (i.e., wind, sun, etc). For the lab setting, participants were required to travel to the research lab on the University campus for data collection. Further detail about the lab sample can be found in previous research (26, 31). Participants from the lab dataset were selected and matched to participants in field settings based on sex and body height (±5 cm).
2.2 Participants
To be eligible for participation, prospective participants needed to be between the age of 18 and 65 years and free from any self-reported upper limb impairments such as shoulder pain. As this study was part of a larger protocol, additional inclusion criteria were required for the work and sport settings. Participants for the work setting were required to live or work on a farm or acreage in the area surrounding the University community. Participants for the sports setting needed to be actively participating in a throwing sport (e.g., baseball). The study protocol received approval from the institutional research ethics board. All participants provided written informed consent prior to participation.
2.3 Protocol
Participant demographic information, including biological sex, age, body mass, body height, and handedness, were recorded. Participants completed the Quick Disability of the Arm, Hands, and Shoulder (QuickDASH) questionnaire to assess upper limb abilities. Participants were equipped with inertial and magnetic measurement units (Xsens Awinda, Xsens Technlogies, NL, Fs = 100 Hz) on the flat part of the acromion of the scapulae, the posterior and distal aspects of the humeri halfway between the epicondyles, and the manubrium of the sternum based on previous research (22, 25). Bilateral scapular calibrations for the double calibration method (23, 25, 32) were completed by aligning a locator (23) (Figure 1) with the anatomical points of the scapulae to define the scapular orientation in a neutral position and at maximum humeral elevation. This calibration process is described in detail elsewhere (23, 25, 31). IMMU placements and calibrations were conducted by the same researcher across all field participants. For the lab setting, a different researcher from the field settings conducted all of the IMMU placements and calibrations across lab participants.
All participants completed the same functional task protocol called the Work-Related Activities and Functional Task (WRAFT) protocol to allow for direct comparisons between settings. The WRAFT protocol is an established lab protocol, which consists of several functional tasks that require participants to move their arms in many different planes and directions, encompassing many of the possible movements required during daily life (Table 1) (26). Specific start and end positions varied with task. Start positions were either arms by sides (Tie Apron, Floor Lift), hand resting on lap (Comb Hair, Wash Axilla), or hand resting on shelf or table (Overhead Reach, Forward Transfer, Overhead Lift). End positions are displayed in Table 1. Data for a Side Reach task typically included in the WRAFT protocol was not collected as previous work has found this task to have poor repeatability and high measurement error (26). We attempted to replicate the WRAFT protocol tasks in the field settings with the resources available and a custom-made shelf that was transportable and easy to set up. However, the shelf set ups were slightly different between the lab and field settings. In the laboratory, the distance between the data collection platform and the shelves prevented participants from sitting or standing directly in front of the bottom shelf. In contrast, participants in the field settings were able to sit or stand closer to the shelves, resulting in slightly different starting positions for the Overhead Reach, Forward Transfer, and Overhead Lift (Figure 2). Additionally, the Forward Transfer task was completed using available tables in field settings or by reaching forward to touch an imaginary tabletop if a table was not present. For all participants, three trials of each WRAFT task (three trials per arm for the unilateral tasks, three trials for bilateral tasks) were completed. For both the lab and field settings, each data collection session was less than 45 min in duration.
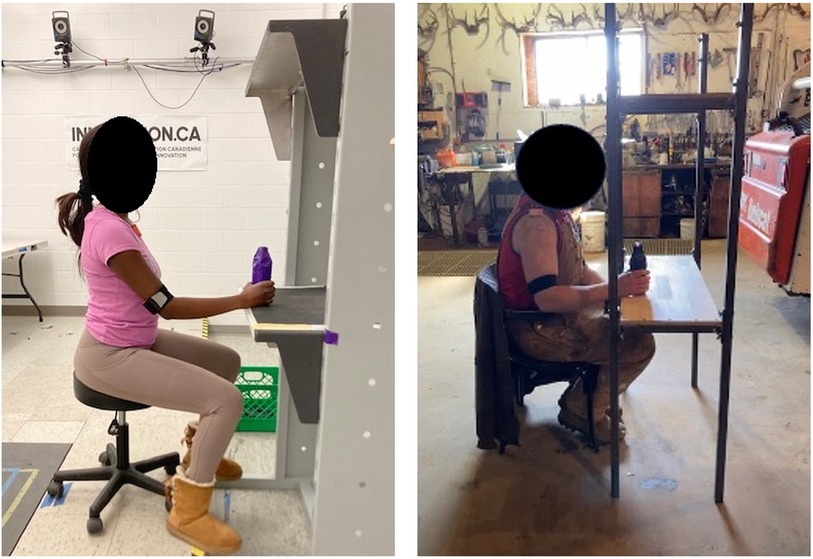
Figure 2. Lab setting (left) and field setting (right) shelf set up and start positions for overhead reach. For the lab setting, shelf heights for the lower and upper shelf were 75 cm and 143 cm, respectively. For the field settings, shelf heights were 75 cm and 150 cm. Participants were further away from the bottom shelf in the lab setting due to equipment positioning.
2.4 Data analysis
Rotation matrices were exported from XSENS MT ManagerTM, and scapular and humeral angles were calculated using custom Matlab® scripts. Humeral elevation was calculated as the angle between the long axes of the torso and humerus as this is the most consistent method to define humeral elevation. Humeral axial rotation was calculated using the True axial rotation method (26, 28, 33). The True axial rotation method is calculated by numerically integrating the humeral angular velocity vector projection onto the humeral longitudinal axis (28) and has been determined to be the most reliable method for calculated axial rotation using different motion capture methods (33). Scapular angles (internal rotation, upward rotation, and tilt) were calculated as Euler angles using the YXZ sequence (26, 34). Trials within each task and participant were averaged and used for the analysis. Scapular and humeral angle waveforms were plotted over defined movement cycles starting at 5 degrees of humeral movement to peak humeral position (33).
2.5 Statistical analysis
Data from all three field settings were averaged to improve statistical power, as the preliminary analysis indicated that the data collected in the different field settings were not statistically different. Differences in demographic characteristics between the field and lab groups were tested using an independent samples t-test (α = .05) for height and Mann–Whitney U tests (α = .05) for mass, age, and QuickDASH scores. For each WRAFT protocol task, humeral and scapular angle waveforms from the field settings were compared to waveforms in the lab descriptively and with statistical parametric mapping (SPM) (35, 36) (α = .05) in Matlab®. SPM was chosen to compare these two different groups, as it can compare full waveforms to assess magnitude and temporal factors. Additionally, because variability between different datasets can influence results, standard deviation for each angle waveform was compared between settings using Mann–Whitney U tests (α = .05). Traditional agreement or repeatability measures are not reported as this was not a test-retest study design.
3 Results
Twenty-eight adults (14 in-field, 14 in-lab) participated in the study (Table 2): five in the home setting, four in the work setting, five in the sport setting, and fourteen in the lab. There were no significant differences in age, mass, height, and QuickDASH scores between field participants and lab participants (p > .05) (Table 2). Additional data from two participants collected in the field settings (one home, one work) (not included in the above group of 14 participants) were not incorporated into the analysis due to calibration and collection errors, respectively.
3.1 Humeral elevation
Humeral elevation waveforms for five of seven WRAFT protocol tasks were not statistically different between the field and lab (p > .05) (Figure 3). Statistical differences in humeral elevation magnitudes (max mean difference = 20°) were present for Overhead Reach and Overhead Lift only at the very beginning of the tasks (0%–6%), with greater humeral elevation in the lab setting (p < .05) (Figure 3). Average standard deviations were similar between settings (14.1° in lab vs. 13.7° in field, p = .70).
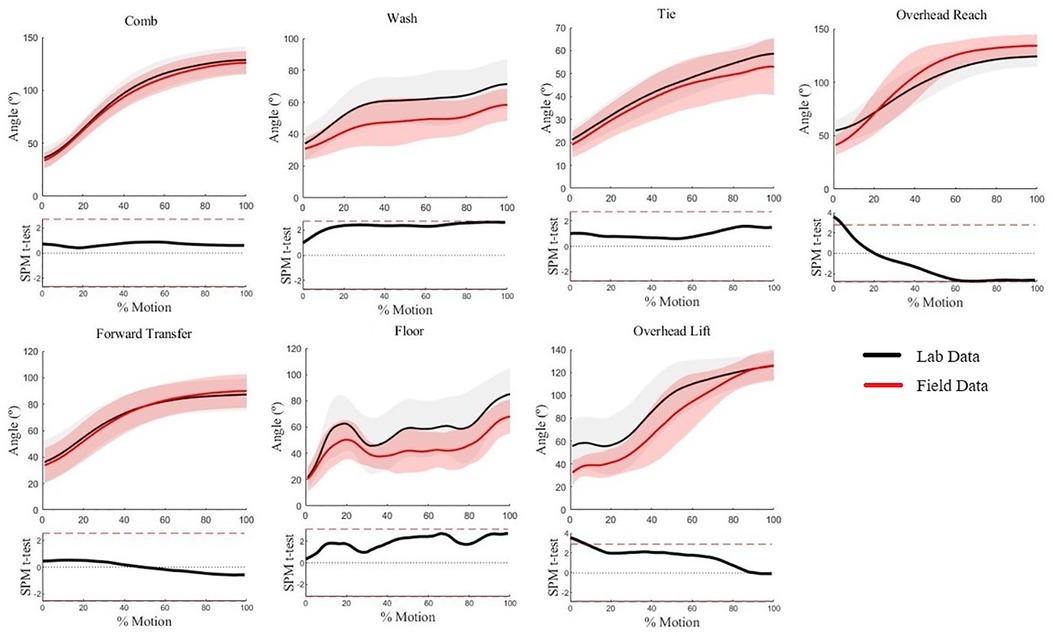
Figure 3. Humeral elevation waveforms for each WRAFT protocol task performed in the field settings (red) and the lab setting (black). Bold lines represent mean waveforms, shaded areas represent one standard deviation. The corresponding statistical parametric mapping test comparing waveforms between the field and the lab setting are provided below the waveform plot for each task.
3.2 Humeral axial rotation
Humeral axial rotation waveforms for five of seven WRAFT protocol tasks were not statistically different between settings (p > .05) (Figure 4). There was greater humeral external rotation in the lab setting when compared to the field settings between 60%–100% of the Forward Transfer task (max mean difference = 11°, p < .05) (Figure 4). There was greater humeral external rotation in the field settings compared to the lab setting at the beginning of the Overhead Lift task (max mean difference = 9°, p < .05) (Figure 4). Average standard deviations were similar between settings (9.1° vs. 10.7°, p = .33).
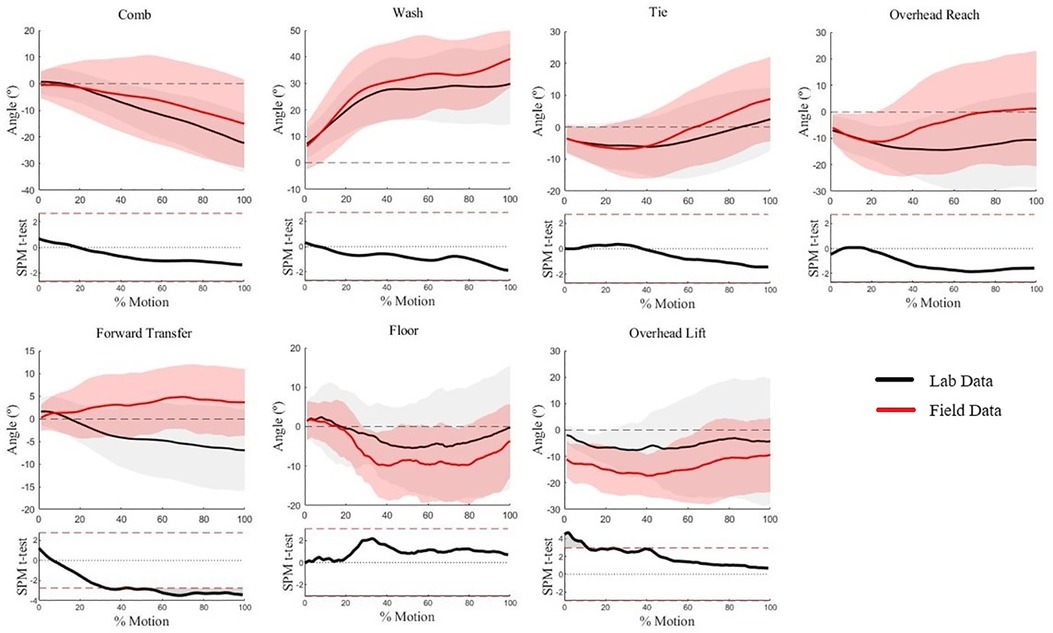
Figure 4. Humeral axial rotation waveforms for each WRAFT protocol task performed in the field settings (red) and the lab setting (black). Bold lines represent mean waveforms, shaded areas represent one standard deviation. The corresponding statistical parametric mapping test comparing waveforms between the field and the lab setting are provided below the waveform plot for each task.
3.3 Scapular upward rotation
Scapular upward rotation waveforms for all WRAFT protocol tasks were not statistically different between settings (p > .05) (Figure 5), and average standard deviations were similar across tasks and settings (10.3° vs. 12.9°, p = .17). Small magnitude differences were evident from visual analysis for Wash Axilla and Overhead Lift, but these differences did not reach statistical significance (Figure 5).
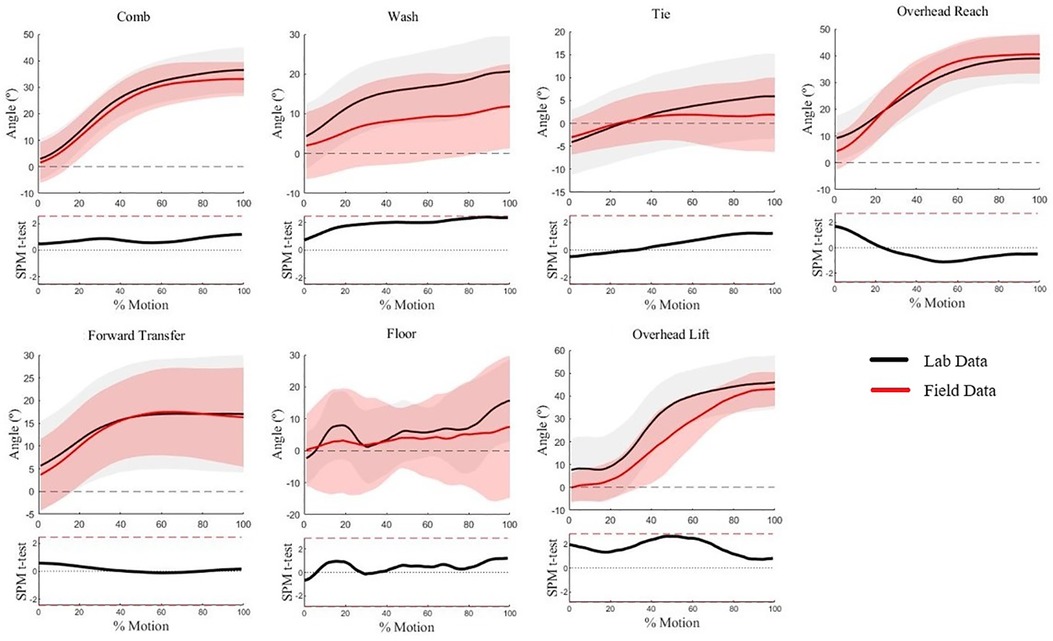
Figure 5. Scapular upward rotation waveforms for each WRAFT protocol task performed in the field settings (red) and the lab setting (black). Bold lines represent mean waveforms, shaded areas represent one standard deviation. The corresponding statistical parametric mapping test comparing waveforms between the field and the lab setting are provided below the waveform plot for each task.
3.4 Scapular tilt
Scapular tilt waveforms were not statistically different between settings (p > .05) (Figure 6). A consistent magnitude offset of approximately 5° is visually present for many tasks, but waveform trajectory is similar for all tasks expect for the overhead lift. However, average standard deviations were significantly higher for the field settings (8.0° vs. 16.3°, p = 0.017).
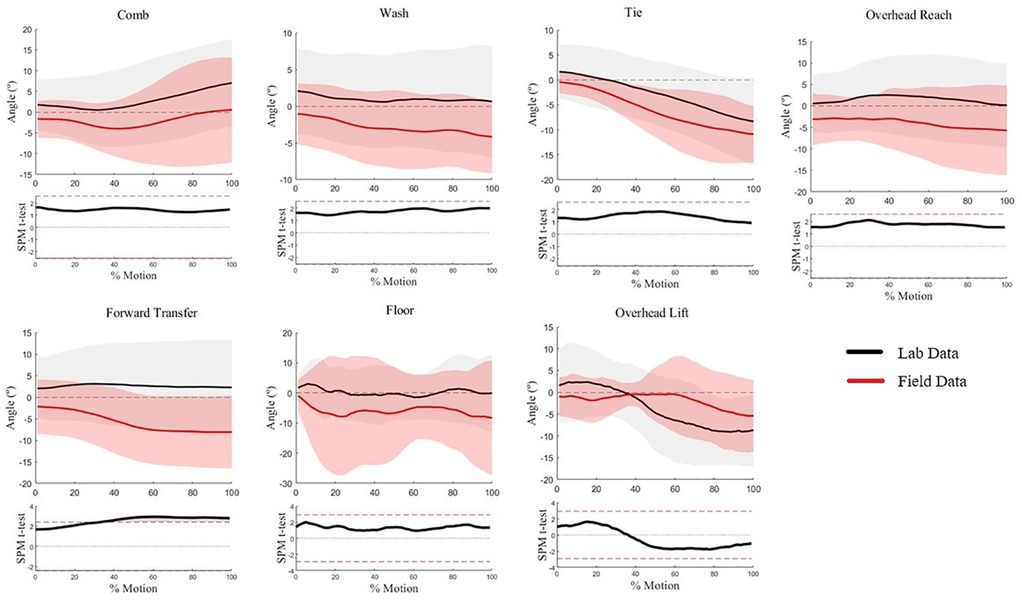
Figure 6. Scapular tilt waveforms for each WRAFT protocol task performed in the field settings (red) and the lab setting (black). Bold lines represent mean waveforms, shaded areas represent one standard deviation. The corresponding statistical parametric mapping test comparing waveforms between the field and the lab setting are provided below the waveform plot for each task.
3.5 Scapular internal rotation
While scapular internal rotation waveforms displayed no significant differences between settings (p > .05) (Figure 7), the standard deviation of the field setting (the shaded light pink area on Figure 7) is substantially and significantly larger than the lab setting (22° vs. 76°, p = .002).
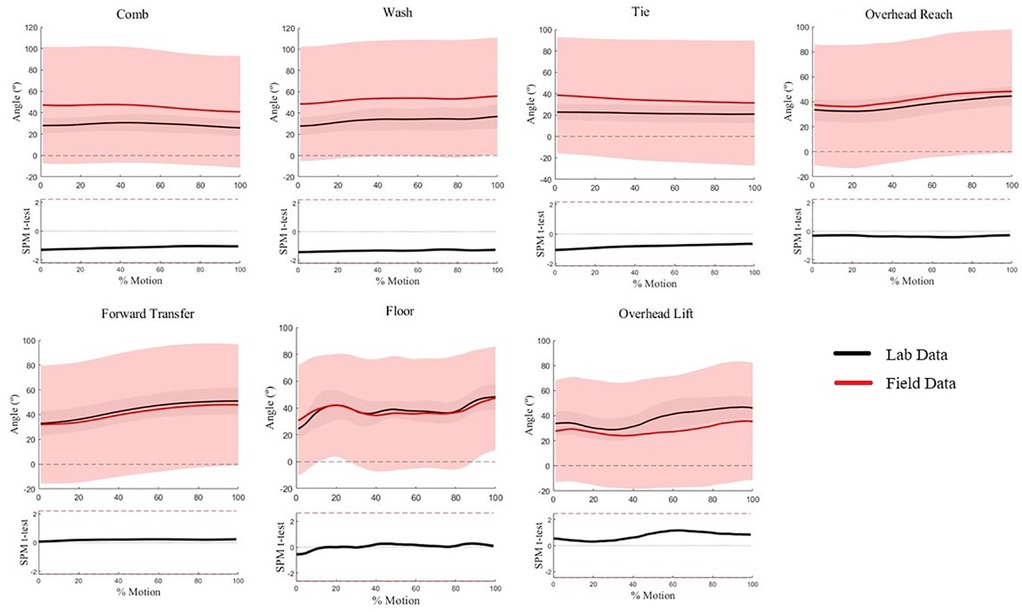
Figure 7. Scapular internal rotation waveforms for each WRAFT protocol task performed in the field settings (red) and the lab setting (black). Bold lines represent mean waveforms, shaded areas represent one standard deviation. The corresponding statistical parametric mapping test comparing waveforms between the field and the lab setting are provided below the waveform plot for each task.
4 Discussion
The current study examined whether humeral and scapular kinematic data collected using IMMUs in various field settings (home, work, sport) is comparable to IMMU data collected in a lab setting. Findings indicate that humeral elevation, humeral axial rotation, scapular upward rotation, and, to some extent, scapular tilt measured in-field were not different from data collected in a controlled lab setting. Field data were collected in different home, work, and sport settings suggesting that these methods can be applied for in-field research and may be robust and generalizable across settings. Researchers can begin to use these methods to assess upper limb motion in natural environments, such as sporting arenas or workshops, which may better inform understanding of upper limb musculoskeletal disorders. The ability to capture natural movements, whether in sport, work, or daily life activities, will have positive implications for detailed investigations of how biomechanics influences performance and injury risk.
Given the similarity of humeral waveforms for most WRAFT protocol tasks, some of the observed differences in upper limb motion may be explained by equipment modifications required to implement the protocol in the field settings (37–39). Different set ups between the lab and field settings (Figure 2) may explain greater humeral elevation and external rotation in the lab at the beginning of the Overhead Reach and Lift tasks. Greater humeral elevation magnitudes at the beginning of the Overhead Reach and Overhead Lift tasks may be due to different start positions in the lab and field settings. In the lab setting, participants were not able to sit or stand as close to the shelf in the lab setting as compared to the field settings. Greater humeral external rotation for the Forward Transfer task in the lab setting compared to the field settings may also be due to differences in task set up: in the lab, all participants sat at the same table, while in the field setting, participants either sat at a table that was available in that setting or sat in front of the set of shelves and reached beyond to an “imaginary” table top. The observed differences in upper limb motion are explainable, suggesting that the observed differences may be largely due to equipment or set up modifications and not the measurement system.
The between-subjects' design may have contributed to visual differences in waveform magnitude for scapular upward rotation and tilt outcomes and increased variability in tilt. Between-subjects' variability was considered by matching field participants to lab participants by sex and body height, but because the comparisons are of two different samples, individual variation likely affected the results (40, 41). For scapular tilt, the larger standard deviations for the field data could mask significant differences; therefore, caution should be used when interpreting those results.
The upper limb movements during WRAFT protocol tasks were relatively unconstrained, allowing for self-selected movement patterns following standardized verbal instruction and visual demonstration of each task. Increasing task constraints, such as movement speed (42, 43), could have further minimized the differences between settings. Despite this variation, humeral elevation, humeral rotation, and scapular upward rotation demonstrate minimal differences, similar visual movement trajectories, and similar variability between settings and to previously reported data (i.e., 10°–24°) (16, 31, 36, 44–47). As between-groups comparisons are prevalent in biomechanics, the similarity of the mean and standard deviation of angle waveforms between the lab and field datasets is promising. However, a test-retest study design would increase the robustness of the findings and interpretation.
Scapular internal rotation measurement was not successful in this study. Significant differences are not present due to the very wide standard deviations for the in-field measures, demonstrating the inconsistency of the scapular internal rotation measurements. There may be two primary explanations for this issue: (1) IMMU movement on the skin, and (2) distortion of the IMMUs. First, in the initial lab-based validation of the scapular IMMU measurement methods (25), the tracking IMMU was placed directly on, and likely braced by, the rigid, L-shaped motion capture acromial marker cluster. In the field, the IMMU was placed directly on the skin above the flat part of the acromion (25, 48, 49). Without the support of the motion capture cluster, the tracking IMMU could have moved on the skin around the Y axis, affecting internal rotation outcomes. Second, it is documented that magnetic distortion of IMMUs largely affects rotation about the Y axis (29, 50), which corresponds with scapular internal rotation in this set-up. The error levels in this previous research are considerably lower than the variability in the current study; it is possible that any distortion affects could be compounded as the scapular angle calculations rely on three separate IMMUs (locator, sternum, scapular tracking IMMU). These same issues are not present in the humeral axial rotation, which is traditionally calculated as rotation about the humeral Y axis (34, 51), because the current study leverages the newer “True axial rotation” method, which does not rely on Euler angles. Overall, it may not be possible to accurately analyze scapular internal rotation with the current set-up. Future research will further investigate possible distortion affects and mitigation strategies.
There are some limitations to address with this study. Although we attempted to make the lab and field protocols identical, there were differences in equipment and protocol between lab and field groups. While our study demonstrates that good quality data can be collected over periods of approximately 45 min, it is unclear whether results can be extended to data recording sessions spanning over several hours. Additionally, data may not generalize across all outdoor and indoor settings, as data could differ from what is reported in settings with local magnetic field disturbances. Future research should consider the specific environment when measuring movement with IMMUs. Importantly, due to the between subjects' design, we were not able to use traditional agreement, repeatability, or reliability tests such as Bland-Altman plots, intraclass correlation coefficients, or standard error of measurement. However, as the purpose was to assess if the setting affected IMMU kinematic outputs, the lack of differences for many angles, and the similarity of many waveforms, even for these two separate (matched) datasets, we believe our approach and conclusions are valid. Future research will explicitly explore agreement between settings using a test-retest design with the same participants. Finally, as mentioned scapular internal rotation did not demonstrate acceptability similarity to the lab data. Scapular angles were calculated from Euler angles, suggesting angle calculation crosstalk could also affect upward rotation and tilt, although findings suggest these degrees of freedom were minimally affected. Further work is needed to understand and address this issue.
These findings provide a foundation for future research with IMMUs. Beyond the methodological work to refine these methods, as described above, the next steps should focus on applying these methods to measure real, daily life movements in each environment. Application of IMMUs in different field settings presents an opportunity for interdisciplinary research exploring how biomechanics, sport sciences, wearable technology, and clinical science can work together to address research gaps with modern solutions. Specifically, upcoming research will build on this work to assess sport-specific movements with IMMUs, such as overhead throws and different pitch types on baseball diamonds, or work-specific tasks, such as machinery maintenance and animal care on grain and large animal farms.
5 Conclusion
Humeral elevation and axial rotation and scapular upward rotation show promise for biomechanical assessments in different field settings using IMMUs with the methods used in this study. However, due to increased variability in field data, scapular tilt outcomes should be interpreted with caution and scapular internal rotation should not be assessed with IMMUs outside of the laboratory with these procedures. These results are encouraging for the evaluation of upper limb motion in natural environments.
Data availability statement
The raw data supporting the conclusions of this article will be made available by the authors, without undue reservation.
Ethics statement
The studies involving humans were approved by University of Saskatchewan Biomedical Research Ethics Board. The studies were conducted in accordance with the local legislation and institutional requirements. The participants provided their written informed consent to participate in this study.
Author contributions
JL: Data curation, Formal Analysis, Investigation, Methodology, Project administration, Writing – original draft, Writing – review & editing. OA: Formal Analysis, Investigation, Methodology, Writing – review & editing. AL: Conceptualization, Data curation, Formal Analysis, Funding acquisition, Investigation, Methodology, Resources, Software, Supervision, Visualization, Writing – original draft, Writing – review & editing, Project administration.
Funding
The author(s) declare financial support was received for the research, authorship, and/or publication of this article.
This research was funded by the University of Saskatchewan’s College of Medicine Research Awards.
Acknowledgments
We thank the study participants for participation and University of Saskatchewan College of Medicine for funding this work.
Conflict of interest
The authors declare that the research was conducted in the absence of any commercial or financial relationships that could be construed as a potential conflict of interest.
Publisher's note
All claims expressed in this article are solely those of the authors and do not necessarily represent those of their affiliated organizations, or those of the publisher, the editors and the reviewers. Any product that may be evaluated in this article, or claim that may be made by its manufacturer, is not guaranteed or endorsed by the publisher.
References
1. Kibler BW, Sciascia A, Wilkes T. Scapular dyskinesis and its relation to shoulder injury. J Am Acad Orthop Surg. (2012) 20(6):364–72. doi: 10.5435/JAAOS-20-06-364
2. Ludewig PM, Reynolds JF. The association of scapular kinematics and glenohumeral joint pathologies. J Orthop Sports Phys Ther. (2009) 39(2):90–104. doi: 10.2519/jospt.2009.2808
3. Hickey D, Solvig V, Cavalheri V, Harrold M, Mckenna L. Scapular dyskinesis increases the risk of future shoulder pain by 43% in asymptomatic athletes: a systematic review and meta-analysis. Br J Sports Med. (2017) 52:102–10. doi: 10.1136/bjsports-2017-097559
4. Lucas J, van Doorn P, Hegedus E, Lewis J, van der Windt D. A systematic review of the global prevalence and incidence of shoulder pain. BMC Musculoskelet Disord. (2022) 23(1):1. doi: 10.1186/s12891-022-05973-8
5. Hodgetts CJ, Leboeuf-Yde C, Beynon A, Walker BF. Shoulder pain prevalence by age and within occupational groups: a systematic review. Arch Physiother. (2021) 11:1–2. doi: 10.1186/s40945-021-00119-w
6. de Oliveira VM, Pitangui AC, Gomes MR, da Silva HA, Dos Passos MH, de Araújo RC. Shoulder pain in adolescent athletes: prevalence, associated factors and its influence on upper limb function. Braz J Phys Ther. (2017) 21(2):107–13. doi: 10.1016/j.bjpt.2017.03.005
7. Kraan RB, de Nobel D, Eygendaal D, Daams JG, Kuijer PP, Maas M. Incidence, prevalence, and risk factors for elbow and shoulder overuse injuries in youth athletes: a systematic review. Transl Sports Med. (2019) 2(4):186–95. doi: 10.1002/tsm2.82
8. Laudner K, Sipes R. The incidence of shoulder injury among collegiate overhead athletes. J Intercollegiate Sport. (2009) 2(2):260–8. doi: 10.1123/jis.2.2.260
9. Fethke NB, Schall MC Jr, Chen H, Branch CA, Merlino LA. Biomechanical factors during common agricultural activities: results of on-farm exposure assessments using direct measurement methods. J Occup Environ Hyg. (2020) 17(2–3):85–96. doi: 10.1080/15459624.2020.1717502
10. Wahlström J, Bergsten E, Trask C, Mathiassen SE, Jackson J, Forsman M. Full-shift trunk and upper arm postures and movements among aircraft baggage handlers. Ann Occup Hyg. (2016) 60(8):977–90. doi: 10.1093/annhyg/mew043
11. Lin MY, Barbir A, Dennerlein JT. Evaluating biomechanics of user-selected sitting and standing computer workstation. Appl Ergon. (2017) 65:382–8. doi: 10.1016/j.apergo.2017.04.006
12. McDonald AC, Mulla DM, Keir PJ. Muscular and kinematic adaptations to fatiguing repetitive upper extremity work. Appl Ergon. (2019) 75:250–6. doi: 10.1016/j.apergo.2018.11.001
13. Keshavarz R, Tajali SB, Mir SM, Ashrafi H. The role of scapular kinematics in patients with different shoulder musculoskeletal disorders: a systematic review approach. J Bodyw Mov Ther. (2017) 21(2):386–400. doi: 10.1016/j.jbmt.2016.09.002
14. McClure PW, Michener LA, Sennett BJ, Karduna AR. Direct 3-dimensional measurement of scapular kinematics during dynamic movements in vivo. J Shoulder Elbow Surg. (2001) 10(3):269–77. doi: 10.1067/mse.2001.112954
15. Ludewig PM, Phadke V, Braman JP, Hassett DR, Cieminski CJ, LaPrade RF. Motion of the shoulder complex during multiplanar humeral elevation. J Bone Joint Surg Am. (2009) 91(2):378. doi: 10.2106/JBJS.G.01483
16. van Andel CJ, Wolterbeek N, Doorenbosch CA, Veeger DH, Harlaar J. Complete 3D kinematics of upper extremity functional tasks. Gait Posture. (2008) 27(1):120–7. doi: 10.1016/j.gaitpost.2007.03.002
17. Lukasiewicz AC, McClure P, Michener L, Pratt N, Sennett B. Comparison of 3-dimensional scapular position and orientation between subjects with and without shoulder impingement. J Orthop Sports Phys Ther. (1999) 29(10):574–86. doi: 10.2519/jospt.1999.29.10.574
18. Borstad JD, Ludewig PM. Comparison of scapular kinematics between elevation and lowering of the arm in the scapular plane. Clin Biomech. (2002) 17(9–10):650–9. doi: 10.1016/S0268-0033(02)00136-5
19. McClure PW, Michener LA, Karduna AR. Shoulder function and 3-dimensional scapular kinematics in people with and without shoulder impingement syndrome. Phys Ther. (2006) 86(8):1075–90. doi: 10.1093/ptj/86.8.1075
20. Fayad F, Roby-Brami A, Yazbeck C, Hanneton S, Lefevre-Colau MM, Gautheron V, et al. Three-dimensional scapular kinematics and scapulohumeral rhythm in patients with glenohumeral osteoarthritis or frozen shoulder. J Biomech. (2008) 41(2):326–32. doi: 10.1016/j.jbiomech.2007.09.004
21. Lang AE, Maciukiewicz JM, Vidt ME, Grenier SG, Dickerson CR. Workstation configuration and container type influence upper limb posture in grocery bagging. Appl Ergon. (2018) 73:206–13. doi: 10.1016/j.apergo.2018.07.012
22. Cutti AG, Giovanardi A, Rocchi L, Davalli A, Sacchetti R. Ambulatory measurement of shoulder and elbow kinematics through inertial and magnetic sensors. Med Biol Eng Comput. (2008) 46:169–78. doi: 10.1007/s11517-007-0296-5
23. van den Noort JC, Wiertsema SH, Hekman KM, Schönhuth CP, Dekker J, Harlaar J. Measurement of scapular dyskinesis using wireless inertial and magnetic sensors: importance of scapula calibration. J Biomech. (2015) 48(12):3460–8. doi: 10.1016/j.jbiomech.2015.05.036
24. Höglund G, Grip H, Öhberg F. The importance of inertial measurement unit placement in assessing upper limb motion. Med Eng Phys. (2021) 92:1–9. doi: 10.1016/j.medengphy.2021.03.010
25. Friesen KB, Sigurdson A, Lang AE. Comparison of scapular kinematics from optical motion capture and inertial measurement units during a work-related and functional task protocol. Med Biol Eng Comput. (2023) 61:1–1. doi: 10.1007/s11517-023-02794-2
26. Friesen KB, Wu LZ, Waslen A, Lang AE. Defining repeatability for scapulothoracic and thoracohumeral motion during the novel work-related activities and functional task (WRAFT) protocol. J Biomech. (2023) 153:111596. doi: 10.1016/j.jbiomech.2023.111596
27. Myn UG, Link MV, Awinda MV. Xsens MVN User Manual (2015). Available online at: https://fccid.io/QILMTW2-3A7G6/User-Manual/Users-Manual-2695756.pdf (accessed November, 2023).
28. Aliaj K, Foreman KB, Chalmers PN, Henninger HB. Beyond Euler/Cardan analysis: true glenohumeral axial rotation during arm elevation and rotation. Gait Posture. (2021) 88:28–36. doi: 10.1016/j.gaitpost.2021.05.004
29. De Vries WH, Veeger HE, Baten CT, Van Der Helm FC. Magnetic distortion in motion labs, implications for validating inertial magnetic sensors. Gait Posture. (2009) 29(4):535–41. doi: 10.1016/j.gaitpost.2008.12.004
30. Roetenberg D, Baten CT, Veltink PH. Estimating body segment orientation by applying inertial and magnetic sensing near ferromagnetic materials. IEEE Trans Neural Syst Rehabil Eng. (2007) 15(3):469–71. doi: 10.1109/TNSRE.2007.903946
31. Waslen A, Friesen KB, Lang AE. Do sex and age influence scapular and thoracohumeral kinematics during a functional task protocol? J Appl Biomech. (2023) 1(aop):1. doi: 10.1123/jab.2023-0085
32. Brochard S, Lempereur M, Rémy-Néris O. Double calibration: an accurate, reliable and easy-to-use method for 3D scapular motion analysis. J Biomech. (2011) 44(4):751–4. doi: 10.1016/j.jbiomech.2010.11.017
33. Lang AE, Friesen KB. Defining humeral axial rotation with optical motion capture and inertial measurement units during functional task assessment. Med Biol Eng Comput. (2023) 61:1–8. doi: 10.1007/s11517-023-02894-z
34. Wu G, Van der Helm FC, Veeger HD, Makhsous M, Van Roy P, Anglin C, et al. ISB recommendation on definitions of joint coordinate systems of various joints for the reporting of human joint motion—part II: shoulder, elbow, wrist and hand. J Biomech. (2005) 38(5):981–92. doi: 10.1016/j.jbiomech.2004.05.042
35. Pataky TC. One-dimensional statistical parametric mapping in python. Comput Methods Biomech Biomed Engin. (2012) 15(3):295–301. doi: 10.1080/10255842.2010.527837
36. Kobayashi K, Umehara J, Pataky TC, Yagi M, Hirono T, Ueda Y, et al. Application of statistical parametric mapping for comparison of scapular kinematics and EMG. J Biomech. (2022) 145:111357. doi: 10.1016/j.jbiomech.2022.111357
37. Feingold-Polak R, Yelkin A, Edelman S, Shapiro A, Levy-Tzedek S. The effects of an object’s height and weight on force calibration and kinematics when post-stroke and healthy individuals reach and grasp. Sci Rep. (2021) 11(1):20559. doi: 10.1038/s41598-021-00036-9
38. Valdés BA, Glegg SM, Van der Loos HM. Trunk compensation during bimanual reaching at different heights by healthy and hemiparetic adults. J Mot Behav. (2017) 49(5):580–92. doi: 10.1080/00222895.2016.1241748
39. Vandenberghe A, Levin O, De Schutter J, Swinnen S, Jonkers I. Three-dimensional reaching tasks: effect of reaching height and width on upper limb kinematics and muscle activity. Gait Posture. (2010) 32(4):500–7. doi: 10.1016/j.gaitpost.2010.07.009
40. Keren G. Between-or within-subjects design: a methodological dilemma. In: A Handbook for Data Analysis in the Behaviorial Sciences (Volume 1). Hillsdale, New Jersey: Lawrence Erlbaum Associates (2014). p. 257–72.
41. Charness G, Gneezy U, Kuhn MA. Experimental methods: between-subject and within-subject design. J Econ Behav Organ. (2012) 81(1):1–8. doi: 10.1016/j.jebo.2011.08.009
42. Asgari M, Sanjari MA, Mokhtarinia HR, Sedeh SM, Khalaf K, Parnianpour M. The effects of movement speed on kinematic variability and dynamic stability of the trunk in healthy individuals and low back pain patients. Clin Biomech. (2015) 30(7):682–8. doi: 10.1016/j.clinbiomech.2015.05.005
43. Li L, Haddad JM, Hamill J. Stability and variability may respond differently to changes in walking speed. Hum Mov Sci. (2005) 24(2):257–67. doi: 10.1016/j.humov.2005.03.003
44. Rundquist PJ, Obrecht C, Woodruff L. Three-dimensional shoulder kinematics to complete activities of daily living. Am J Phys Med Rehabil. (2009) 88(8):623–9. doi: 10.1097/PHM.0b013e3181ae0733
45. Yildiz TI, Eraslan L, Demirci S, Kara D, Ulusoy B, Turgut E, et al. The repeatability of 3-dimensional scapular kinematic analysis during bilateral upper extremity movements. J Bodyw Mov Ther. (2020) 24(4):37–42. doi: 10.1016/j.jbmt.2020.06.038
46. Haik MN, Alburquerque-Sendín F, Camargo PR. Reliability and minimal detectable change of 3-dimensional scapular orientation in individuals with and without shoulder impingement. J Orthop Sports Phys Ther. (2014) 44(5):341–9. doi: 10.2519/jospt.2014.4705
47. van den Noort JC, Wiertsema SH, Hekman KM, Schönhuth CP, Dekker J, Harlaar J. Reliability and precision of 3D wireless measurement of scapular kinematics. Med Biol Eng Comput. (2014) 52:921–31. doi: 10.1007/s11517-014-1186-2
48. Karduna AR, McClure PW, Michener LA, Sennett B. Dynamic measurements of three-dimensional scapular kinematics: a validation study. J Biomech Eng. (2001) 123(2):184–90. doi: 10.1115/1.1351892
49. MacLean KF, Chopp JN, Grewal TJ, Picco BR, Dickerson CR. Three-dimensional comparison of static and dynamic scapular motion tracking techniques. J Electromyogr Kinesiol. (2014) 24(1):65–71. doi: 10.1016/j.jelekin.2013.09.011
50. Robert-Lachaine X, Mecheri H, Larue C, Plamondon A. Effect of local magnetic field disturbances on inertial measurement units accuracy. Appl Ergon. (2017) 63:123–32. doi: 10.1016/j.apergo.2017.04.011
Keywords: wearable sensors, shoulder, home, work, sport, humeral elevation, humeral axial rotation, scapular upward rotation
Citation: Lordall J, Akinluyi OV and Lang AE (2024) Towards in-field assessment of humeral and scapular kinematics: a comparison between laboratory and field settings using inertial sensors. Front. Sports Act. Living 6:1349570. doi: 10.3389/fspor.2024.1349570
Received: 4 December 2023; Accepted: 16 February 2024;
Published: 28 February 2024.
Edited by:
Pietro Picerno, University of Sassari, ItalyReviewed by:
Dana Badau, George Emil Palade University of Medicine, Pharmacy, Sciences and Technology of Târgu Mureş, RomaniaBrice Bouvier, Independent Researcher, Paris, France
© 2024 Lordall, Akinluyi and Lang. This is an open-access article distributed under the terms of the Creative Commons Attribution License (CC BY). The use, distribution or reproduction in other forums is permitted, provided the original author(s) and the copyright owner(s) are credited and that the original publication in this journal is cited, in accordance with accepted academic practice. No use, distribution or reproduction is permitted which does not comply with these terms.
*Correspondence: Angelica E. Lang YW5nZWxpY2EubGFuZ0B1c2Fzay5jYQ==