- 1Institute of Chemistry, The Islamia University of Bahawalpur, Bahawalpur, Pakistan
- 2Department of Biology, College of Science, University of Hafr Al Batin, Hafar Al Batin, Saudi Arabia
- 3Department of Chemistry, COMSATS University Islamabad, Abbottabad Campus, Abbottabad, Pakistan
- 4Magan Centre of Applied Mycology (MCAM), Faculty of Engineering and Applied Sciences, Cranfield University, Cranfield, United Kingdom
- 5Biomolecular Innovation Group, Laboratorio de Ciencias Naturales, Facultad de Agronomía, Universidad Autónoma de Nuevo León, General Escobedo, Nuevo Leon, Mexico
Environmental pollution has become an inexorable problem for the planet Earth. The precise detection and degradation of heavy metals, pesticides, industrial-, pharmaceutical- and personal care- products is needed. Nanotechnology holds great promise in addressing global issues. Over the past decades, nanozymic nanomaterials have exceptionally overcome the intrinsic limitations of natural enzymes. Carbon dots (CDs) exhibit unique structures, surface properties, high catalytic activities, and low toxicity. Different techniques, such as doping or surface passivation, can enhance these exceptional properties. Doping modifies CDs’ electronic, magnetic, optical, and catalytic properties considerably. Metal doping, a more significant strategy, involves the introduction of metallic impurities, which offer insight into enhancing the physicochemical properties of CDs. Metal-doped CDs exhibit higher optical absorbance and catalytic performance than pristine CDs. The literature shows that researchers have utilized various synthetic approaches to fabricate CDs-Metal nanozymes. Researchers have reported the metal-doped and hybrid CDs’ peroxidase, catalase, laccase, and superoxide dismutase-like activities. These metal-doped nanozymes put forward substantial environmental remediations and applications such as sensing, photocatalytic degradation, adsorption, and removal of environmental contaminants. This review thoroughly discussed the metal-based functionalization of CDs, the enzyme-like properties, and the ecological applications of metal-doped and hybrid enzymes. The review also presents the current novelties, remaining challenges, and future directions with key examples.
1 Introduction
The environment is essential for sustaining life, but environmental pollution causes alarming obstacles to the viability of Earth. Several anthropogenic practices, such as industrialization, agriculture, and transportation, have been the leading cause of environmental contamination (Awewomom et al., 2024; Ukaogo et al., 2020). Such contamination eventually leads to ecological, health, and economic consequences. Regarding health issues, cancer, respiratory problems, and numerous chronic diseases are caused by chemical pollutants (Kim et al., 2018). However, global efforts are steadily being made to alleviate environmental pollution and protect human health. Nanotechnology is an advanced field unveiling significant ecological protection (Khin et al., 2012). Owing to their ultra-compact size, high surface area, non/less toxicity, and easy biodegradation, carbon nanomaterials are considered valuable (Liu Y. et al., 2020; Choudhary et al., 2014; Cao et al., 2013). The advancement of carbon dots has attracted attention in the crossover field of carbon materials and pristine quantum dots.
Carbon dots (CDs), the quasi-spherical, zero-dimensional competitive members (less than 10 nm in size), belong to the family of carbon nanomaterials (Sagbas and Sahiner, 2019; Zhao Q. et al., 2020; Cui et al., 2021; Langer et al., 2021). In 2004, CDs were accidentally discovered during the purification of single-walled nanotubes (SWNTs) using an arc discharge method. Thereafter, the research on CDs rapidly increased. CDs exhibit small size, larger surface-to-volume area, active edges, fluorescent properties, strong absorption, resistance to photo-decomposition, low toxicity, biocompatibility, and enhanced catalytic activity (Figure 1; Zhang et al., 2021; Innocenzi and Stagi, 2023; Ge et al., 2018; Chen et al., 2021). Carbon, hydrogen, and oxygen are the main constituents of CDs. The carbon core consisting of crystalline sp2 carbon comprises amino (-NH2), hydroxyl (-OH) and carboxyl (-COOH) groups.
CDs are diverse, and are further classified. Each class’s size, properties, structure, and formation mechanism may vary. Graphene Quantum Dots (GQDs), Polymer Dots (PDs), and Carbon Nanodots (CNDs) are the main categories. The CNDs exhibit amorphous spherical morphology (Pathak et al., 2023). Their core contains a high degree of carbonization (Langer et al., 2021). CNDs bifurcates into Carbon Quantum Dots (CQDs) and Carbon Nanoparticles (CNPs). The amorphous CNPs do not exhibit Quantum Confinement Effect (QCE), while the CQDs having surface chemical groups and graphene-layer show QCE (Ozyurt et al., 2023; Bacon et al., 2014). GQDs have significant properties and are excellent fluorophores and electro-catalysts (Mansuriya and Altintas, 2020; Ghaffarkhah et al., 2022). GQDs may show the QCE. The last type, polymer dots (PDs), comprise low carbonization; they have either linear polymers or monomers grouping (Ozyurt et al., 2023; Song et al., 2017). Different methods have been employed for their synthesis, such as chemical oxidation, microwave-assisted synthesis, laser ablation, atmospheric plasma-based synthesis, thermolysis, hydrothermal carbonization, solvothermal, electrochemical, and ultrasonic methods. Among all these synthetic techniques, the solvothermal method (particularly hydrothermal) is commonly used to synthesize doped CDs.
Despite the unique physicochemical properties of CDs, pristine CDs show some limitations, such as, low quantum yield and ease of contamination. Hence, extensive research has been conducted in improving and tailoring their functionality over the years. Doping has been widely utilized in optimizing CDs’ intrinsic chemical, electrical and optical properties. Top-down and bottom-up approaches are the two main synthetic methods for reporting hetero-atom-doped CDs (Miao et al., 2020). The former approach majorly involves tailoring pre-existing carbonaceous material. In contrast, the bottom-up approach involves synthesizing material from the ground up. Compared to the top-down, the bottom-up approach allows precise manipulation of synthesis conditions and, hence, offers more control over CD material structure and properties (Alafeef et al., 2024).
Researchers have reported peroxidase, oxidase, superoxide dismutase, and catalase-like activity in functionalized CDs (Zhu et al., 2014; Wang H. et al., 2021; Wu et al., 2019; Zhang et al., 2023). These CDs exhibit excellent stability than natural enzymes and are utilized as enzyme alternatives for environmental applications (Gao et al., 2007). Like natural enzymes, their catalytic activity is sensitive to pH, temperature, and concentration. The peroxidase-like catalytic activity of CDs was first reported by Shi et al. (2011). Later, many other researchers synthesized doped and/or hybrid CDs (especially metal-doped CDs) to enhance the enzyme-mimicking activity of CDs (Lopez-Cantu et al., 2022). A comparative analysis between different metal-doped (including Ce-doped, Cr-doped, Cu-doped, Fe-doped, and Mn-doped CDs) and non-metal-doped CDs as peroxidase-mimics has also been reported (Yuxin et al., 2022). The results confirmed that both non-metal and metal-doped CDs exhibit peroxidase-like catalytic activity; however, the metal-doped CDs, such as Fe-doped CDs, show the highest catalytic activity and act as excellent artificial enzymes. In other words, the metal-doped CDs exhibit more catalytic active sites and improved binding selectivity (Gallareta-Olivares et al., 2023).
The fluorometric/colorimetric property of metal-doped and hybrid CDs for detecting chemical pollutants facilitates a sensitive monitoring system (Nejad et al., 2020). The common insecticide and high-risk water contaminant, p-nitrophenol (p-NP), has been detected through fluorometric Cr-doped CDs sensors (Li C. et al., 2019). Similarly, the hybrid nanozymic-nanoreactors based on Fe-doped CDs (Fe-CDs) and MOF-808 were reported to detect and degrade organophosphorus pesticides. The author also noted a synergistic effect of Fe-CDs on MOF-808-comprised complex to sense paraoxon and parathion (Ma et al., 2023). Similarly, Cu-doped Tragacanth/Chitosan CDs and Fe-doped N@CDs detect isoniazid (INH) and doxycycline (Shekarbeygi et al., 2020; Kaur et al., 2022a).
2 The structural/functional rationale CDs
Carbon Dots (CDs) are a class of zero-dimensional, quasi-spherical shaped nanomaterial having a usual size of less than 10 nm (Cui et al., 2021; Kurian and Paul, 2021). Researchers report that the surface area of CDs varies from 16.4 m2 g−1 to 1,690 m2 g−1 (Wang Q. et al., 2013; Ren et al., 2019). The carbon present in their structure is sp2 and sp3 hybridized. The sp2/sp3 carbons comprised of CDs attach many functional groups and polymer chains. Depending on the degree of carbonization, the sp2/sp3 carbon-skeleton shows an amorphous-form or a graphite-lattice (Mansuriya and Altintas, 2021). Relative to Quantum Dots and other nanomaterials, CDs are functionally less toxic, highly luminescent, biocompatible, inexpensive, and thermally stable. Regarding Photoluminescence (PL) studies, they show dispersion, UV-vis absorption, quantum yield, and phosphorescence. They also exhibit core-state emission, molecular fluorescence, and surface-state emission (Li Z. et al., 2019). Despite the advanced applications of CDs, few of the fundamental properties of CDs are still uncertain. Such uncertainties hinder scientists from enhancing CDs’ properties and applications for future visions (Mintz et al., 2021). Figure 2 shows the characteristic description of CDs’ morphological and photoluminescent features.
CDs offer several beneficial properties due to functional groups on the surface. These functional groups also impart catalytic activity to CDs. Both reducing and oxidizing species can be used to synthesize CDs. As a result, CDs can lead the redox transformation reactions (Mokoloko et al., 2023). These redox reactions give rise to many applications in pollution control, industrial catalysis, waste treatment, and degradation of pharmaceuticals (Tarumi et al., 2019; Toral-Sánchez et al., 2018). Moreover, the functional groups are highly significant in enhancing the properties of CDs in biosensing and molecule detection. Yan et al. reported that CDs’ properties could be improved by modification procedures in which the covalent or non-covalent bonding is built between the reactants and the CD surface. Studies show that two different CDs can react to a new CD, exhibiting the surface properties of both parent CDs. Similarly, three other types of CDs were produced by Zhou. et al. The reaction was kept at room-temperature conditions, and reactant CDs formed a link between their functional groups. CDs formed in products were utilized as nanocarriers in drug delivery systems (Mokoloko et al., 2023).
Hydrophilic and hydrophobic groups on CDs enhance their solubility and stability in water and organic solvents (Li Z. et al., 2019; Mokoloko et al., 2023). The hydrophobicity and hydrophilicity of CDs can be altered by using precursors and passivators (Rui et al., 2023). For Bioimaging and NIR-Anticounterfeiting the hydrophobic CDs have been utilized (Shi et al., 2023). Hydrophilic CDs act as metal-ion detectors and fluorescent pH sensors (Liu X. et al., 2018). The hydrophilic properties of CD surface also offer several applications in medicinal chemistry (Jana and Dev, 2022). Moreover, CDs can also be used to modify several reactions (Mokoloko et al., 2023).
CDs carry photocatalytic processes in environmental applications. CDs-based photocatalysis works in the degradation of pollutants and energy conversion as well. Carbon allotropes have been used in titania-supported photocatalysis in the past few years (Nguyen et al., 2015). The researchers have demonstrated the degradation of Tetracycline through titania nanoparticles-based photocatalysis (Zhu et al., 2013). Moreover, the metal-doped nanoparticles (NPs) increase light absorption properties by interacting incident photons with metal NPs electrons (Yao et al., 2022; Jana et al., 2016). This interaction is referred to as the Surface Plasmon Resonance Effect. CDs comprise ease in their functionalization (Gallareta-Olivares et al., 2023). The chemical and physical properties of CDs are enhanced and adjusted through Doping. Moreover, the doped-CDs have different energy gaps and electron densities than the un-doped ones (Li X. et al., 2022). The choice of dopant element is significant in obtaining desired features. Compared to non-metallic ions, metal ions have large atomic radii, more electrons, and unoccupied orbitals (Li X. et al., 2022). For these reasons, metal-doped CDs show excellent performance in bioimaging, biocatalysis, photocatalysis, and phototherapy (Boakye-Yiadom et al., 2019).
3 The physicochemical properties and functional elements
CDs show unique physical properties, i.e., high reactivity, good chemical reactivity, surface functionality, biocompatibility, hydrophilicity, electroconductivity, and low toxicity (Miao et al., 2020; Ranjan et al., 2022; Fawaz et al., 2023). The high reactivity of CDs can be justified by their smaller size, well-defined shapes, greater surface area, and integrated functional groups. Preference to reactive precursors and particular synthetic approaches can also significantly improve the reactivity (Papaioannou et al., 2019). The existence of different functional groups attached to the surface of CDs is referred to as surface and structural functionality (Figure 3). Such functional groups greatly influence other physicochemical properties. Surface functionality can originate from surface functionalization or precursors utilized in the synthesis (Yan et al., 2018). Hydroxy (-OH), amino (-NH2), and carboxy (-COOH) groups are the most familiar functional groups found on CDs. The oxygenated functional groups, more specifically, affect the photoluminescence properties. Characteristic CDs, designed through surface modification, can further aid in developing multifunctional fluorescent probes for different purposes (Liu et al., 2016).
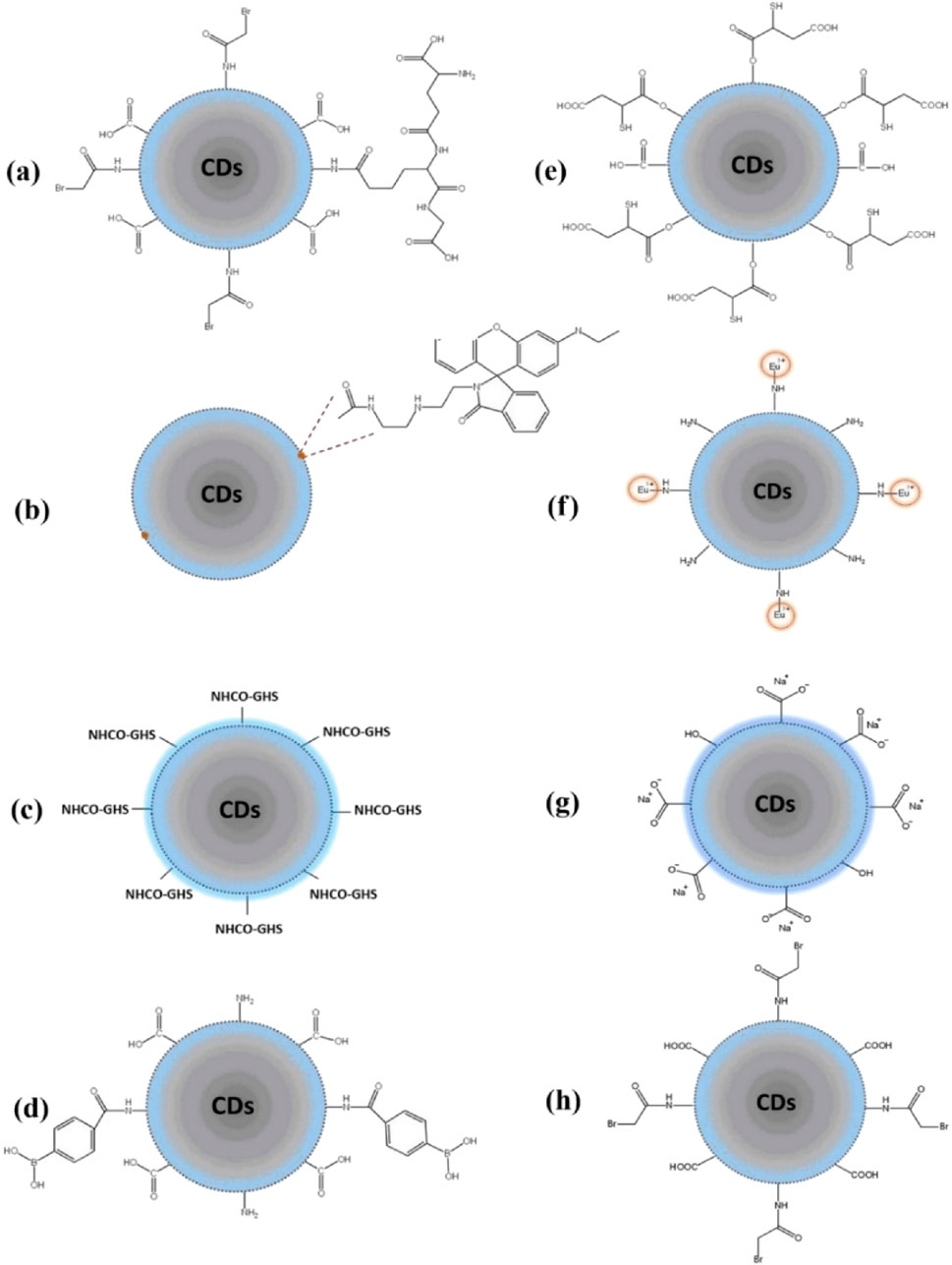
Figure 3. Illustration of Structural Functionalities Present on Carbon Dots developed via Amide Coupling Reaction in (a–d, h); Esterification Reaction in (e); Sulfonylation Reaction in (f) and Complexation in (g).
CDs’ tailorable hydrophilic and hydrophobic properties are also promising in developing more advanced biological and environmental applications (Ozyurt et al., 2023; Zhang et al., 2024; Zhao and Zhu, 2018; Liu J. et al., 2020). Hydrophilicity provides stability to CDs in aqueous media, enhancing dispersion. Utilization of ionic liquids (ILs), surface functionalization, and control of terminal alkyl chain length are a few of the significant methods involved in tuning the hydrophilicity of CDs (Ozyurt et al., 2023; Guo et al., 2019; Varisco et al., 2017; Guo et al., 2021; Zheng et al., 2019). In surface functionalization, hydrophilic functional groups such as -OH, -COOH, and -HN3 are introduced on the CDs surface (Zhang et al., 2024; Zhao and Zhu, 2018). ILs (such as 1-alkyl-3-methylimidazolium dicyanamide) have been reported to synthesize both hydrophilic and hydrophobic CDs (Guo et al., 2019). Moreover, the amphiphilic carbon dots (ACDs) exhibit hydrophilic and lipophilic properties (Nandi et al., 2014; Pandit et al., 2021). They show interaction with both polar and non-polar media. Compared to standard CDs, ACDs offer a broader range of environmental applications. For instance, the amphiphilic fluorescent carbon nanodots detect tetracycline and nitrite in both aqueous and organic media (Omer et al., 2020). Despite the difference in structures, synthetic approaches, precursors, dopants, and chemical functionalities, CDs show similar optical properties (Ozyurt et al., 2023; Das et al., 2021a). They exhibit UV-visible absorbance, which is a convincing parameter in examining the chemical structure of CDs (Das et al., 2021a). CDs show single or more distinguished absorption peaks in the UV region and an extended tail in the visible region (Baker and Baker, 2010; Li D. et al., 2018; Liu, 2020). The 200–280 nm peak is attributed to the π-π* transition of doubly-bonded carbon atoms, i.e., C=C. The 280–400 nm absorption peak corresponds to the n-π* transition of C=O. Moreover, the overlapped bands above 400 nm regions correspond to the n-π* transition, originating from the absorption of surface-states with electron lone-pairs (Liu, 2020; Wang Y. et al., 2014).
However, CDs’ photoluminescence (PL) properties are not fully understood (Langer et al., 2021; Zhang et al., 2021; Barman and Patra, 2018; Sk et al., 2014). The two major causes for the uncertain PL mechanism are the (i) complex structure of CDs and (ii) variation in the PL centers (Langer et al., 2021). PL of GQDs and CQDs depends on surface states (such as heteroatom dopants, surface functionalities, surface configurations, surface defects, etc.) and quantum confinement effects (QCE) (Cui et al., 2021; Sk et al., 2014; Ai et al., 2021; Zhu Z. et al., 2019). The QCE can be governed by various factors such as particle size, shape, and solvent involved in the synthesis (Sk et al., 2014; Kim et al., 2012; Qu et al., 2015). All these factors affect the sp2 conjugation length. More significant conjugation length of sp2 carbon domains results in a reduced band gap, leading to red emission shift (Pedrueza et al., 2013). Likewise, the large, aromatic ring containing GQDs structures shows redshifts (Sk et al., 2014; Kim et al., 2012; Eda et al., 2010). The utilization of certain solvents (for example, DFM) in the synthesis process of CDs also affects the band gap and PL properties (Qu et al., 2016; Tian et al., 2017; Zhu et al., 2011; Bai et al., 2019). On the other hand, the surface states arise synergistic hybridization between the attached functional groups and carbon backbone (Ding H. et al., 2020). Hence, the hetero-atom doping and surface modifications tune the PL properties of CDs (Yuan et al., 2018; Fan et al., 2014). Integrating functionalities (hydroxyl, carbonyl, carboxylic acid, epoxy and epoxy ether, alcohol, amine, and thiol) forms surface states and defects. These surface states shift emission to longer wavelengths. For instance, using tartaric acid in the reaction mixture of CDs synthesis enhances the degree of oxidation and creates redshift. Figure 4 represents the factors affecting the optical properties of CDs.
Heteroatom dopants (B, F, N, and S) also tune the electronic structure and enhance fluorescence quantum yield (Qu et al., 2015; Yang et al., 2018; Peng et al., 2016; Zuo et al., 2017; Hao et al., 2015; Qin Z. et al., 2019; Feng et al., 2021; Gao D. et al., 2020). This property has notably acquired great attention in monitoring and sensing applications of CDs. Furthermore, the pH (Feng T. et al., 2018; Lei et al., 2019; Chao et al., 2020), reaction temperature (Sun et al., 2020; Chen et al., 2017; Miao et al., 2018), concentration (Chen et al., 2018; Meng et al., 2017; Wang et al., 2018a; Ba et al., 2020; Zhang et al., 2019; Su et al., 2020), and aggregations (Wang et al., 2017; Lv et al., 2019; Chen et al., 2012; Yang et al., 2019) dependent PL properties of CDs have also been reported. Moreover, CDs exhibit good chemical stability. Chiral CDs show more excellent chemical stability than the simple CDs (Li S. et al., 2021; Ru et al., 2020). Compared to metal complexes, metal-doped CDs exhibit a low degree of cytotoxicity. It is because of the presence of carbon, which hinders the free release of metal ions (Li X. et al., 2022).
Furthermore, the study of chemical properties involves the consideration of CDs structure. Several characterization techniques are employed (Ozyurt et al., 2023; Song et al., 2015; Bhaisare et al., 2015; Singh et al., 2018; Siddique et al., 2018). These techniques include scrutinizing size, shape, crystal structure, chemical functionality, and particle-size distribution (Ozyurt et al., 2023). Regarding the size, shape, and average-size investigation, TEM (Transmission Electron Microscopy) is preferably a significant analytical technique (Song et al., 2015; Bhaisare et al., 2015; Singh et al., 2018). TEM images also reveal the functional groups present on GQDs. Whereas, determining particle-thickness involves using AFM (Atomic Force Microscopy). It also gives information about topological profile and graphene-layer count in GQDs (Ozyurt et al., 2023). However, the crystal structure can be investigated by HRTEM (High-Resolution Transmission Electron Microscopy and XRD (X-ray Diffraction) (Mintz et al., 2021; Nie et al., 2014). HRTEM images represent the crystallographic planes, i.e., lattice fringes in CD-aggregates (Nie et al., 2014; Kurdekar et al., 2016). Raman Spectroscopy is used to investigate CDs’ crystallinity and functional groups (Dager et al., 2019). In Raman spectra, CDs show two bands, i.e., G and D bands (Ozyurt et al., 2023). The D and G band correspond to the vibration originating from graphitic sp3-carbon and graphitic sp2-carbon defects (edges) (Shi et al., 2015). The surface functional group on CDs can be recognized through Elementary Analysis and Infrared Spectroscopy (El-Shafey, 2021). The chemical functionalities can be identified by X-ray Photoelectron Spectroscopy, Nuclear Resonance Spectroscopy (NMR), and Fourier transform infrared (FTIR) technique (Ozyurt et al., 2023).
4 Metal-based functionalization of CDs
Due to the easy preparation and potent applications, CDs’ functionalization has attracted researchers’ attention (Ðorđević et al., 2019; Stepanidenko et al., 2021; Park et al., 2016). Functionalization controls and enhances CDs’ optical, electrical, chemical, and biological properties (Li X. et al., 2022; Park et al., 2016; Chen et al., 2019; Dhenadhayalan et al., 2020). Surface modification and doping are the two major approaches for CDs functionalization. In surface modification, functional ligands such as ions, polymers, proteins, DNA, or other molecules modify CDs. The CD-surface and functional ligand interact through electrostatic interaction, covalent bond, non-covalent bond, or coordination (Chen et al., 2019). Along with the improvement in properties, surface modification provides new reaction sites for more surface reactions to occur as well (Wang et al., 2023). Fe3+, Mn2+, Cu2+, Tb3+, and Eu3+ have been utilized in the surface modification of CDs (Bourlinos et al., 2017; Wang Y. et al., 2015).
Doping also optimizes the physicochemical properties of CDs. It causes variation in the intrinsic structure of CDs, and eventually, the electronic distribution changes through the generation of n-type or p-type carriers (Li X. et al., 2022; Chen et al., 2019). The introduction of copper-metal causes an enhancement in the electronic properties, which improves the catalytic properties of CDs (Duan et al., 2019). Doping can be further classified into metal doping or non-metal doping. Boron, nitrogen, phosphorus, sulfur, and fluorine (either solely or in combination) are non-metallic dopants. Calcium, magnesium, scandium, manganese, iron, copper, nickel, cobalt, zinc, gallium, bismuth, molybdenum, silver, gold, gadolinium, etc., act as metallic dopants. Both metallic and non-metallic dopants design HOMO-LUMO energy band gaps. This energy band gap decides doped CDs’ (photo-optical) properties (Kumar et al., 2022; Feng J. et al., 2018).
Due to more electrons, unoccupied orbitals, and large atomic radii of metal ions, metal-based CDs offer a broad range of intrinsic properties. Research shows that Cu-based doping can increase the electron-donating and accepting abilities, resulting in copper-to-graphite charge transfer, and eventually, the electrical conductivity is enhanced (Wu et al., 2015). Another research shows that the Cu-doped CQDs can form Cu-N bonding, which produces the copper-to-graphite charge transfer. Ultimately, the absorption intensity increases (Eda et al., 2010; Wu et al., 2015). Owing to the low energy level, when UV light of 365 nm strikes the Cu-doped CQDs, the photo-excited holes intensely compete with Cu ions, which results in the production of Cu ions, enabling their utilization for Husigen 1,3 dipolar cyclo-addition reaction (Li et al., 2015). Figure 5b represents the Cu2+-based synthesis of CDs (Jin et al., 2017). These functionalized CDs are synthesized by using carrots as a carbon source. The CDs were modified using positively charged polyethylene (PEI) and Nile Blue. The significance of these functionalized CDs lies in their action as a two-photon fluorescence probe. Mn-based functionalization of CDs can adjust the fluorescent emission, resulting in emission at longer wavelength (Chen et al., 2019). In addition, zinc doping has been proposed to give higher photocatalytic activity and QY (Chen et al., 2019; Li et al., 2013; Xu et al., 2016). Moreover, Zn-doped CDs pose stabilized excited states, increasing the fluorescence lifetime. The defects present in Zn-CDs enhance solubility and are helpful for the photodegradation of Cr(VI) (Chen et al., 2019). It has been reported that CDs with transition metal ions exhibit higher efficiency than non-transition ones (Bandi et al., 2022).
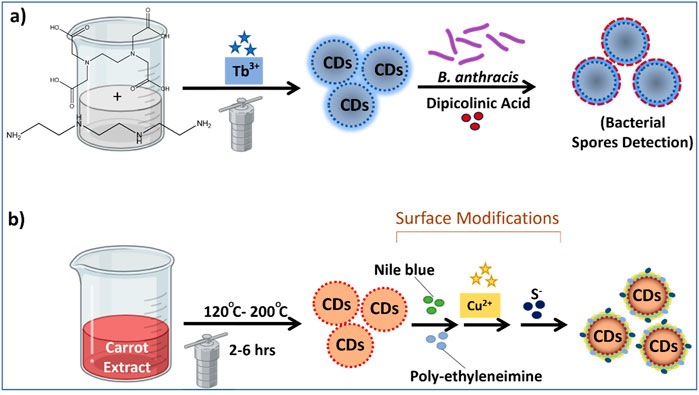
Figure 5. Surface functionalization of carbon dots (CDs), (a) Terbium-based CDs, and (b) Copper-based CDs.
Lanthanides-based functionalized CDs have gained much importance due to their unique fluorescent properties (Chen et al., 2019). Gd, Y, Nd, Tb, Tm, and Eu have been utilized to functionalize metal-doped CDs (Chen B. B. et al., 2016; Chen Y. et al., 2016). However, Y and Nd are highly significant for their long fluorescence lifetime NIR emission. Nd 3+ and Yb3+ have been utilized as dopants to synthesize Ln-based CQDs exhibiting characteristic NIR emission (Wu et al., 2016). In addition, Eu-based CDs have also been prepared via a microwave-assisted method. Eu functionalized CDs offer exceptionally enhanced quantum yield (QY) (Chen Y. et al., 2016). Figure 5a represents the Tb3+-based functionalization of CDs (Chen et al., 2015). The Tb3+-based CDs were synthesized by complexing Terbium with amino and carboxyl groups on the CD surface. These functionalized CDs can detect dipicolinic acid. Regarding their application, they can rapidly detect biomolecules and bacterial spores.
Another common approach for the metal-based functionalization of CDs involves using noble metals (Ru, Rh, Os, Ir, Pt, Au, and Ag) as dopants (Zaheer et al., 2023). Noble metal-based CDs contain a synergistic effect of both components, i.e., noble metal and CDs (Fu et al., 2020). Functionalization usually occurs through ion exchange, hydrothermal, or galvanic replacement reactions (GRR). These functionalized CDs pose advanced properties, such as a specific large surface area, extensive catalytic activity, and sufficient electron transfer pathways (Zaheer et al., 2023).
Metal-based functionalization of CDs pursues vast applications in the degradation and adsorption of pollutants from the environment (Gallareta-Olivares et al., 2023). Cu, Fe, Ga, Zn, Au, and Ag are excellent metal dopants in catalysis. Due to copper atoms (which act as both electron donor and acceptor), Co-based CDs are considered a strong catalyst (Ma et al., 2017). Fe, Zn, and Cr can be doped into CDs for metal detection in water (Han et al., 2018; Xu et al., 2018; Zhang H.-Y. et al., 2017). Fe2+ doped CDs were synthesized for enhanced fluorescence and detection specificity (Han et al., 2018). Cu-CDs catalyze the ozonation reaction of textile dyes, i.e., Methyl Orange (MO), Reactive Black 5 (RB-5), Orange II sodium salt (Orange (II)), and Remazol Brilliant Blue R (RBB-R). In the absence of Cu-CDs, the rate of discoloration is low. The presence of Cu-CDs results in increases in the rate of reaction (%Inc = 24%) with 90% efficiency in 60 min for azo dyes (i.e., MO, O (II) and RB-R) and 30 min for anthraquinone dye (i.e., RBB-5). However, the presence of carbonate in water inhibits the ozone decomposition. Hence, the high concentration of carbonates in textile sewers limits the application of Cu-CDs for wastewater treatment.
Moreover, studies show that Zn2+ improves the fluorescence of silver nanoclusters (Xu et al., 2018). Zn-CDs and Co-CDs can be utilized to detect Hg2+ and Cr (IV) ions in water, respectively (Xu et al., 2018; Zhang H.-Y. et al., 2017). Cu and Ni can be used for CDs’ doping in NIR-mediated phototherapy. Thiophanate-methyl (TM) is a potent fungicide that can be detected and treated in Cu-based CDs (Han et al., 2019). This sensor overcomes the single response drawback of ratiometric fluorescent sensors. Cr (III)-based CDs were effectively demonstrated to detect p-nitrophenol, which acts as a pesticide (Li C. et al., 2019). Moreover, Mg-based N-CDs (formed via co-doping) act as a paraoxon-insecticide detector (Peng et al., 2019). Zn/ZnO-based nanocomposites, BiOCl hybrid nanocomposites, and Cu, N co-doped CDs were also reported to perform photodegradation of pollutants (Guo et al., 2017; Phophayu et al., 2020; Mou et al., 2019; Mu et al., 2019). Subsequently, Bi-based CDs can remove dyes and metal contaminants from water. Several other environmentally related applications of CDs/functionalized CDs and metal doped and Hybrid CDs are summarized in Table 1.
5 Solvothermal vs. hydrothermal synthesis route
The development of solvothermal and hydrothermal synthesis routes has more than 100 years of history. Both methods are inexpensive, rapid, effective, and environmentally friendly (Li X. et al., 2022; Feng and Li, 2017; Tejwan et al., 2021; Ndlwana et al., 2021). These routes are significant for the current and future fabrication and tuning of CDs for several applications in catalysis, water treatment, pollutant degradation, metal detection, and the biomedical field (Wang et al., 2018b; Manohara et al., 2019; Zhou et al., 2021; Ge et al., 2021). The synthesis involves heating precursor solutions at a high temperature for many hours (Li X. et al., 2022). Usually, metallic salts and organic molecules are utilized as precursors. The prepared particles exhibit a diameter of less than 10 nm. Solvothermal and hydrothermal methods are similar to each other. However, in the solvothermal method, the organic solvent is used instead of water (Li X. et al., 2022; Tejwan et al., 2021). Research reports that Mn, Cu, Zn, Mg, Gd, Co, Ag, and Cr ions have been doped on CDs through solvothermal synthesis (Li X. et al., 2022).
Wang et al. prepared porous carbon-protected magnetite (Fe3O4@C) nanoparticles from ferrocene (Fe(C5H5)2) and hydrogen peroxide (H2O2) using acetone (Wang H. et al., 2013). This solvothermal synthesis was accomplished at 200°C in 24 h. Later, Fe3O4@C nanoparticles were loaded with silver ions (Ag+), followed by the in situ Ag+ reduction in the carbon shell at room temperature. Dumbbell-shaped Fe3O4@C-Ag hybrid nanoparticles were synthesized, having 110 nm size. These catalytic hybrid nanoparticles enhanced the reduction reaction of organic dyes in water. Due to the carbon shell protection, these nanoparticles also resisted photo-bleaching.
Using solvothermal treatment, Zhang et al. synthesized Co-doped CDs (CCDs) from 1-(2-Pyridylazo)-2-naphthol, CoCl2, and ethanol as solvent. Based on photoluminescence quenching, these CCDs were successfully utilized to sense Cr(VI) in water. The author proposed that the fluorescence of CCDs could be quenched in the presence of Cr(VI) ions. The detection limit calculated was 0.12 ppm for Cr(VI). These CCDs help control chromium contaminant intake from water and reservoirs. Wang et al. synthesized spherical, 100 nm-sized hybrid-nanoparticles of iron oxide-fluorescent CDs from a solvothermal route in which acetone solvent was used (Wang H. et al., 2014). The reaction was held in an autoclave for 48 h at 200°C, followed by 15 min of sonication at room temperature. These hybrid nanoparticles exhibited NIR light-absorbing properties, upconversion fluorescent activity, and high magnetic response.
Zhou et al. used citric acid, urea, Mn(OAc)2, and toluene as solvents to synthesize Mn-based CDs (2.95 nm diameter) (Liu Y. et al., 2018). In these CDs, the release of Mn2+ enhanced the degree of conjugated sp2 domain, surface states, and FLQY up to 68.6%. A synergistic strategy was observed to develop these efficient CDs, in which solvent worked as a tuning agent and Mn2+ as an enhancing agent. The author demonstrated that the solvent is a primary factor in tailoring the absorption band. These Mn-based CDs exhibit versatile properties to peruse several applications.
Wang et al. fabricated magnetite-porous carbon-protected Au-based nanoparticles (AuNPs) through a solvothermal technique (Wang H. et al., 2015). They utilized Fe3O4@PC-CDs and Fe3O4@PC-CDs-Ag and then proceeded with a galvanic cell reaction to replace Ag with Au3+ in the presence of HAuCl4. The product achieved was Fe3O4@PC-CDs-Au nanoparticles. These Au-doped hybrid nanoparticles show high photothermal conversion and enhance photo-stability. Liu et al. prepared NiFe2O4 integrated EDTA-derived CDs from a solvothermal route, in which NiCl2, FeCl3.6H2O, and NaAc were dissolved in ethylene glycol (Liu et al., 2017). After the sonication, the reaction mixture was autoclaved for 10 h at 200°C. These Ni/Fe-based CDs exhibit high water dispersibility and excellent adsorption of tetracycline from water. Using a hydrothermal approach, Sachdev et al. prepared multifunctional CeO2-based spherical CDs (Sachdev and Gopinath, 2016). The reaction of ammonium cerium branched poly (ethyleneimine), and aqueous solution form of chitosan proceeded at 220°C for 12 h. The author demonstrated that, due to the catalytic effect of CeO2, these nanocomposites can interact and neutralize hydrogen peroxide. Thus, they prevent the fluorescent activity of CDs.
Yang et al. fabricated Mg/N co-doped CDs by hydrothermal method at 200°C and 5 h (Yang et al., 2016). They utilized acrylic acid, magnesium hydroxide, and ethylenediamine, followed by the conjugation of CDs with Cholrin e6. These CDs show a QY of 84.6%, along with high fluorescence resonance energy transfer (FRET). The CDs-Ce6 system offers high hydrophobicity and changeable FRET efficiency as well. Zhang et al. synthesized La-doped CQDs (La-CQDs) from hydrothermal treatment at 160°C for 8 h (Zhang et al., 2017b). They used ATP and LaCl3.2H2O to form La-CQDs (4.3 ± 0.3 nm diameter). These CQDs show high thermal stability and intense fluorescence. Due to numerous -COOH, -NH2, PO43− and -OH groups, La-LQDs have excellent photoluminescence and high-water solubility. These CQDs have significantly detected Hg2+ (detection limit observed = 0.1 μM).
Zhang et al. synthesized intrinsic photoluminescent and magneto-fluorescent Fe/N co-doped spherical CDs (FeN@CDs) by facile hydrothermal method at 200°C for 10 h (Zhang et al., 2017c). Initially, they utilized FeCl3.6H2O and chitosan to prepare FeN@CDs. These FeN@CDs were further conjugated with riboflavin and folic acid. The T2 relaxation time increased with the increase in iron concentration. Later, their cross-linked polymer nanostructures were utilized to achieve NIR-triggered photothermal and photodynamic therapy. Das et al. (2018) synthesized spherical N/Zn co-doped CDs (4.5 nm diameter) via hydrothermal treatment at 200°C for 4 h using citric acid, zinc acetate, and tris(hydroxymethyl)aminomethane (Das et al., 2018). These CDs exhibited high fluorescence, QY, monodispersity, and thermal stability. Due to their excellent luminescence, N/Zn co-doped CDs also act as an intriguing FL-probe for detecting H2O2 in the Fenton reaction mechanism.
Moradlou et al. synthesized CQDs of hematite nanostructure deposited on titanium (CQDs@α -Fe2O3) via hydrothermal treatment of Fe3+, CQDs, NaNO3 and Ti sheet for 6 h at 100°C temperature (Moradlou et al., 2019). Qing et al. fabricated low-cost Cu2+−based CDs using a one-step hydrothermal carbonization treatment of waste tea extract, copper acetate, and ethylenediamine at 150°C temperature for 6 h (Qing et al., 2020). Malmir et al. opted for a hydrothermal technique to synthesize TiO2-based CQs nanocomposites (Malmir et al., 2020). They used chitosan, ascorbic acid, and TiO2 at 140 oC for 24 h.
Water is safe, non-toxic, cheap, green, and readily available solvent. In contrast, most organic solvents are expensive, toxic, and hazardous to living and non-living systems. Organic solvents can also change the concentration of reactive species in the reaction mixture, which ultimately changes the product composition (Feng and Li, 2017). Thus, hydrothermal is a more significant and popular technique for preparing metal-based CDs. Table 2 summarizes various hydrothermally synthesized metal doped and hybrid CDs involved in environmental remediation.
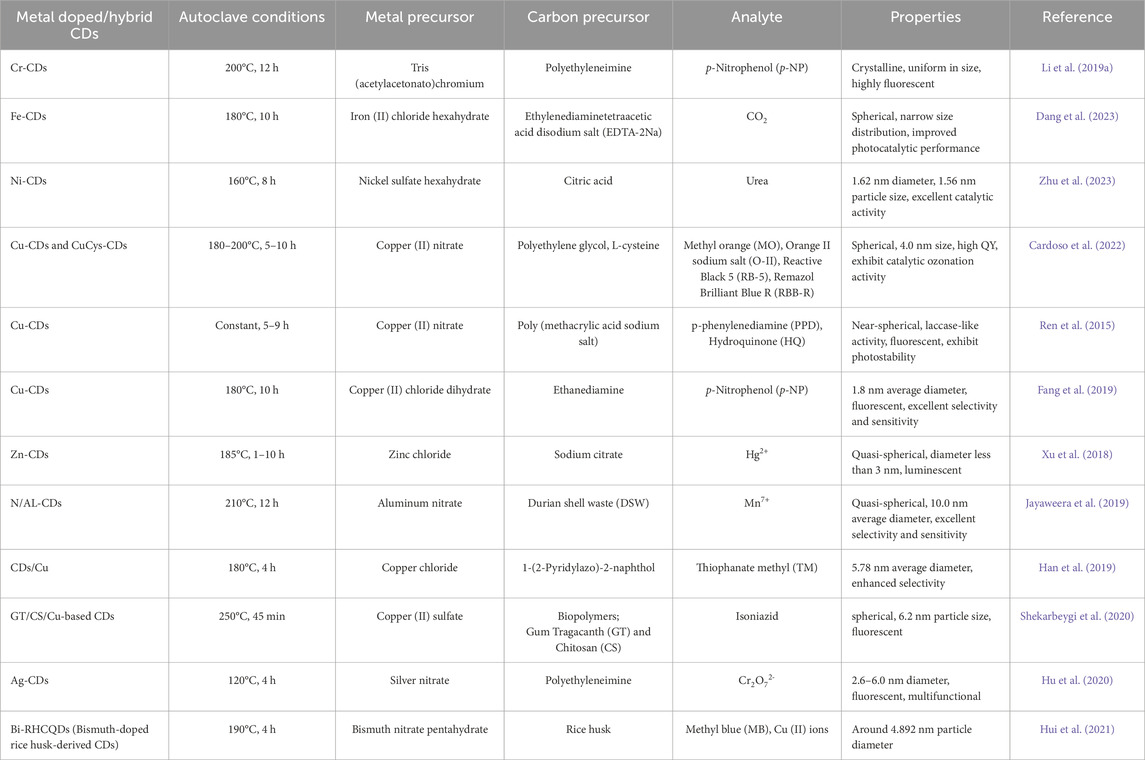
Table 2. Hydrothermally synthesized Metal Doped and Hybrid Carbon Dots involved in Environmental Remediation.
6 CDs as promising enzyme mimics
It is practically significant to synthesize enzyme-mimicking nanomaterial to tackle the intrinsic limitations of natural enzymes (Dhiman et al., 2022; Jin et al., 2021). Nanozymes effectively mimic the catalytic sites of natural enzymes (Li Y. et al., 2020). Representative examples of enzyme mimetic metal-doped and hybrid CDs are summarized in Table 3. They show numerous properties, such as facile synthesis, high catalytic stability, easy modification, and long storage time (Jin et al., 2021; Sun et al., 2018; Huang et al., 2019). They can be remotely controlled with stimuli such as heat, light, ultrasound, and magnetic field (Jiang et al., 2019). The three different possibilities in which CDs exhibit enzyme-like properties are heteroatom-doped CDs, CD-based nanozyme hybrids, and pristine CDs with innate enzyme-mimic properties (Lopez-Cantu et al., 2022). Researchers have studied the enzyme-mimicking properties in pristine CDs (Zhu et al., 2014; Das et al., 2021b). In pristine CDs, oxidase-like activity is based on the adsorption of dissolved oxygen. This adsorption is followed by the transfer of delocalized electrons (of CDs (Li S. et al., 2020)) to oxygen. Ultimately, the oxygen reduces into O2− (Li S. et al., 2020; Li F. et al., 2020). However, nanozymes based on pristine CDs give decreased catalytic activity in neutral pH conditions. Hence, the researchers proposed the CD-based hybrid nanozymes. CuZn-based CDs show catalase-like activity (Xue et al., 2021). The CDs were covalently doped with copper and zinc. These CDs exhibit high stability and excellent electron transfer capability. Zhuo et al. synthesized oxidase-like Mn-doped CDs (Mn-CDs) via facile hydrothermal method. These CDs catalyzed the oxidation of 3,3′,5,5,-tetramethylbenzidine. Su et al. fabricated Co/N-doped CDs using Co(NH3)2.6H2O and Nitrogen-CDs. These CDs exhibited peroxidase-lie catalytic activity to o-phenyldiamine (Su et al., 2022). Yang et al. synthesized Iron and nitrogen co-doped CDs via a facile solvothermal method (Figure 6a; Yang et al., 2017). These CDs exhibit high water solubility and dimensional homogeneity. The intrinsic peroxidase-like (POD-like) activity of N, Fe-CDs is attributed to iron-doping. In the presence of H2O2 N, Fe-CDs catalyze the oxidation of TMB and form blue-colored TMBox as an oxidation product. Hence, they act as highly efficient peroxidase mimetic and promising probes in chemical and biological reactions. Likewise, the Copper and Chlorine (Cu, Cl-CDs co-doped CDs were synthesized via a hydrothermal method (Zhao et al., 2022). N, Cu-CDs exhibit both oxidase- and peroxidase-like activity. Figure 6b represents the synthesis, oxidase-like (OD-like), and peroxidase-like (POD-like) activity of N, Cu-CDs. The limit of detection for TMB and hydroquinone (HQ) is 0.35 µM and 0.08 µM, respectively.
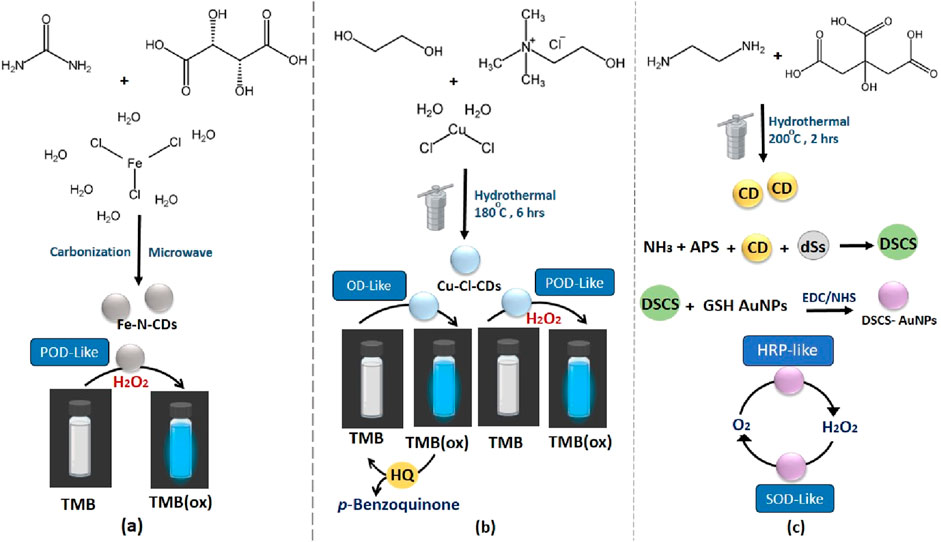
Figure 6. Functionalized CDs illustrating enzyme-like capacities, (a) peroxidase-like (POD-like) activity, (b) oxidase-like (OD-like) activity and peroxidase-like (POD-like) activity, and (c) superoxide dismutase (SOD-like) activity and horseradish peroxidase-like (HRP-like) activity.
Duan et al. synthesized Cu-doped CDs using a solid-phase synthesis technique using Cu(NO3)2.3H2O dopant (Duan et al., 2019). These CDs exhibited catalytic properties and specifically showed peroxidase-like activity. Moreover, Cu-CDs show good stability and enzyme-like activity in a broad range of temperature and pH values. Several oxidase-like enzymes have been synthesized with a relevant interest. These metal-doped CDs can catalyze oxidation reactions in the presence of dioxygen (Lopez-Cantu et al., 2022). Dioxygen accepts electrons and gives the formation of water and hydrogen peroxide. Li et al. synthesized cerium-mediated CD-based nanozyme, exhibiting oxidase-like activity (Li S. et al., 2020). Its catalytic activity could be tailored by changing the concentration ratio of CDs and Cerium ions. Researchers have also synthesized CD-based nanozyme hybrids with other materials. This approach develops a synergistic effect to increase the enzymatic properties of CDs (Ngo et al., 2021). Metal-doped carbon-based nanozymes work as electron transport mediators; thus, the reaction rate increases (Feng et al., 2022). The synergism between metal and carbon structures increases the electron flow in enzymatic processes. Subsequently, these nanozymes provide a large surface area and hinder metal agglomeration (Sun Y. et al., 2023).
Zhuo et al. synthesized dSCSAu-NCDs hybrid nanostructures (Zhao L. et al., 2020) (Figure 6c). The CDs were immobilized into amino-based dendritic silica spheres (dSs) with linked gold nanoclusters (Au-NCs). This hybrid nanostructure based on dual-nanozymes shows superoxide dismutase-like activity. The single-atom strategy is another significant approach to tuning the electronic and catalytic properties (Wu et al., 2020). This strategy overcomes the conventional doping limitations and provides excellent dispersibility and utilization of functionalized CDs (Sun Y. et al., 2023). Researchers have reported several single-atom nanozymes. For instance, Qin et al. synthesized PEGylated silica-based Fe single-atom CDs (Fe/CDs@PPSNs). These nanostructures exhibited higher catalytic activity than simple iron oxide-based CDs (Qin et al., 2022). Single-atom Ru-based CDs have been synthesized to achieve catalytic activities (Wang W. et al., 2021). These CDs exhibit peroxidase, oxidase, and glutathione oxidase-like activity. Theoretical results show that these CDs more specifically mimic peroxidase-like activity. The Ru-4d orbital electrons are transferred to the oxygen atom of hydrogen peroxide. It results in the production of hydro-oxide radicals.
7 CDs as nanozymes with peroxidase-like catalytic activities
Natural peroxidases belong to a large group of oxidoreductases, which functionally catalyze the reduction of peroxides and oxidation of inorganic and organic compounds (Hamid and Khalil-ur-Rehman, 2009; de Oliveira et al., 2021). Chemically, these comprise heam proteins containing Fe (III) with protoporphyrin IX as a prosthetic group. NADH, glutathione, and iodine peroxidase are specific enzymes belonging to the peroxidase group (Li M. et al., 2023; Shigeto and Tsutsumi, 2016; Riyazuddin et al., 2023). However, the non-specific enzymes are referred to as peroxidases. Peroxidase catalytic activity is essential in the chemical, environmental, biomedical, and industrial fields (Jiang et al., 2019; Passardi et al., 2005; Neill et al., 2002). The immobilized oxidative enzymes are widely significant and suitable to tackle the current challenges of environmental pollution (Wei et al., 2020; Aitken et al., 1994; Dasappa and Loehr, 1991).
Regarding the peroxidase-like catalytic phenomenon of CDs, many authors reported the occurrence of a “Ping-Pong catalytic mechanism” (Shi et al., 2011; Wang B. et al., 2016; Shamsipur et al., 2014; Mohammadpour et al., 2014; Long et al., 2016; Zhu et al., 2015). However, depending on the nanozyme composition, the action mechanism may vary. On the other hand, two substrates, i.e., a hydrogen acceptor and a hydrogen donor, are generally involved in the catalytic reaction. The nanozymes reduce the H2O2 and generate reactive intermediates such as ·OH. ·OH further reacts with the second substrate to proceed with the reaction (Gao L. et al., 2020). In another research, a systematic study reports that the catalytic activity can be induced by the presence of hydrophilic groups (such as -COOH, -C=O, and -NH2) on the CD surface (Das et al., 2021b). These groups generate an affinity with peroxides, ultimately acting as a catalytic site for catalyzed reactions. The author reported that the carboxylic group induces maximum peroxidase-like activity in CDs. Nitrogen and copper co-doped CDs (N, Cu-CDs) were synthesized via a facile hydrothermal method (Lin et al., 2019). These CDs exhibit peroxidase-like (POD) catalytic activity even at a broader range of pH and temperature. The POD-like activity of N, Cu-CDs catalyzes the oxidation of para-, ortho-, and m-phenylenediamine by peroxide. The oxidation products of o-phenylenediamine (OPD) and p-phenylenediamine are yellow and brown, respectively. Hence, the POD-like N, Cu-CDs result in the colorimetric discrimination of OPD and PPD.
The extent of peroxidase-like activity of metal-doped CDs depends on different factors, such as the electron transferring capacity and variable valance states of dopant-metal (Li S. et al., 2018; Berglund et al., 2002). The significance of the electron transfer process in enhancing the peroxidase-like activity of CDs has been reported (Shamsipur et al., 2014). For instance, CDs with greater charge density show enhanced electron transfer from substrate to CDs. The substrate oxidation reaction rate increases due to enhanced electron transfer and radical formation. Moreover, CDs with smaller sizes and larger specific surfaces exhibit more excellent peroxidase-like catalytic activity (Zhou et al., 2019; Tang et al., 2019). Figure 7a represents the hydrothermal synthesis of peroxidase-like Fe-CDs (Zhu D. et al., 2019). Methylthymol blue sodium and ferric chloride hexahydrate were used as carbon and metal precursor/sources, respectively. The catalytic activity of Fe-CDs corresponds to the oxidation of the colorless o-phenylenediamine (OPD) solution. Under the peroxidase-like activity of Fe-CDs, the oxidation product OPD forms 2,3-diaminophenazine (DAP). The sensing of H2O2 (detection limit 0.47 µM) is based on the visible yellow color formed in Fe-CDs/H2O2/OPD (Figure 7b).
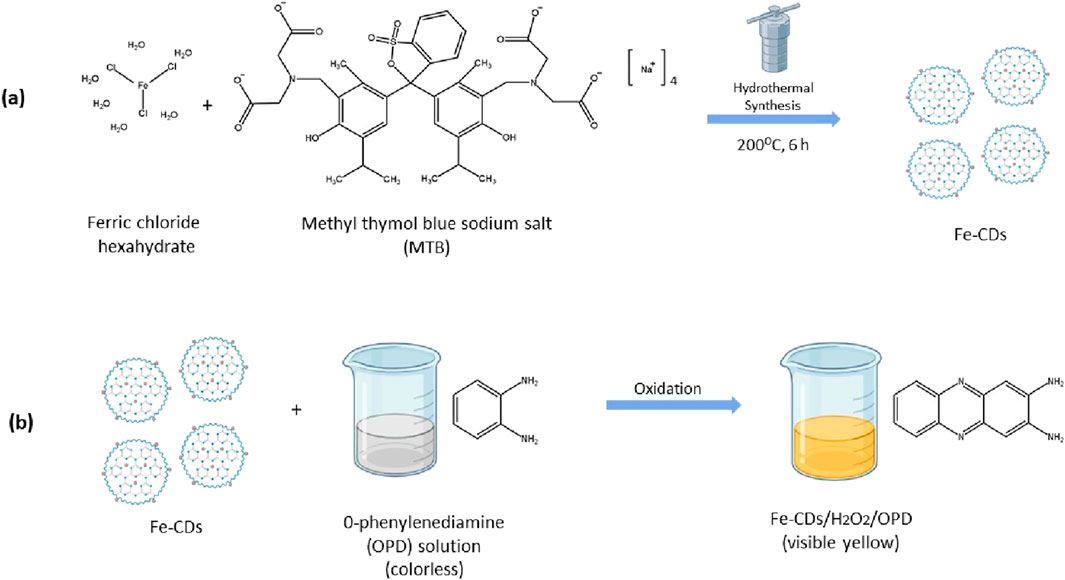
Figure 7. (a) Synthesis of peroxidase-like Fe-CDs (b) Fluorescent sensing of H2O2 in the presence of Fe-CDs.
Pristine, doped, and/or hybrid CD nanozymes have been reported to exhibit peroxidase-like activity (Figure 8; Zhu et al., 2014; Duan et al., 2019; Li Y. et al., 2020; Shamsipur et al., 2014; Tang et al., 2019; Zhang L. et al., 2017; Wu et al., 2014; Zheng et al., 2016; Dong et al., 2015; Gan et al., 2021; Yang et al., 2021; Habibi and Heidari, 2016; Roushani et al., 2018). In this manner, Figure 8 represents the CDs, Co@CDs, Gd-CDs, and N, Cu-CDs as pristine, hybrid, doped, and co-doped CDs with peroxidase-like activities.
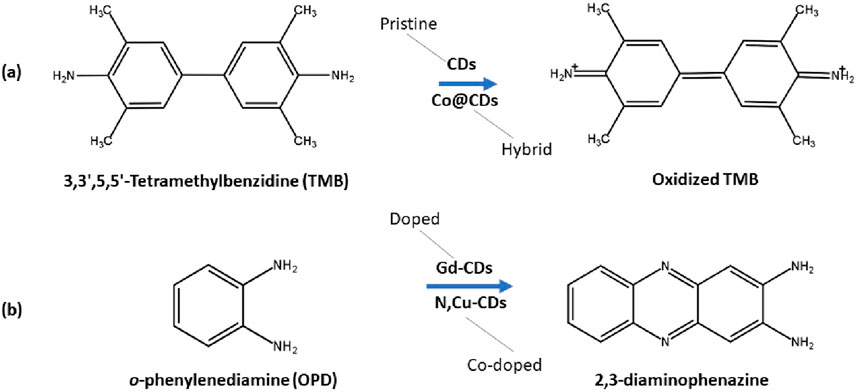
Figure 8. (a) Catalytic Oxidation of 3,3′5,5′-Tetramethylbenzidine (TMB) in the presence of CDs and Co@CDs, and (b) o-Phenylenediamine in the presence of Gd-CDs and N,Cu-CDs.
Along with catalytic activity, few nanozymes show highly unique properties. Wang et al. synthesized multicolor photoluminescent CNPs (C1H0.677O0.586N0.015Na0.069) with high catalytic activity (Wang et al., 2011). The EDX spectrum of these CNPs demonstrated that no metal catalyst was involved in the system. Na+ only acts as a counter ion and exhibits no catalytic activity. Similarly, CNPs and peroxide could not oxidize the substrate. However, there existed an interaction between CNPs, peroxide and substrate. This interaction induces catalytic activity in CNPs. Several Hybrid nanozymes with excellent temperature and pH tolerability (such as thermally stable Fe3O4/GQD-based nanozymes, thermally stable and reusable NCDs/Fe3O4-based nanozymes and acid pH-independent Co, N-doped CD-based hybrid nanozymes) have also been reported (Su et al., 2022; Huang et al., 2023; Shen et al., 2019). Likewise, the Cu-doped CDs were synthesized through a facile solid phase treatment of citric acid as a carbon source and Cu(NO3)2.3H2O as a metal source (Duan et al., 2019). These CDs show high stability and excellent peroxidase-like activity within a broad range of pH and temperature. In another research, N, Fe-codoped CDs were synthesized using FeCl3.6H2O, B-cyclodextrin, and ethylenediamine via hydrothermal treatment at 180°C for half an hour (Li Y. et al., 2020). In the presence of peroxide, these CDs were reported to exhibit color-changing properties along with more excellent catalytic activity than that of horseradish peroxidase (HRP). However, synthesizing peroxidase-like complex CD-based hybrid nanozymes is a relatively complicated but potent approach (Shi et al., 2019). For example, Li et al. synthesized Au@HgNPs nanozymes using gold nanoparticles (Au-NPs), nor-adrenaline-based CDs (NA-CDs), and methyl mercury ions (MeHg+) (Li Q. et al., 2023). The synthesis phenomenon was observed to be quite complex. However, the radical formation and enhanced electron transfer from metals (Au and Hg) to CDs induce high peroxidase-like catalytic activity in the nanozymes.
8 Environmental application
CD-based nanozymes can be noticeably used to monitor, degrade and adsorb the environmental pollutants (Gallareta-Olivares et al., 2023; Jelinek, 2017; Lin et al., 2018; Liang et al., 2020). Environmental applications of enzyme mimetic metal doped/hybrid CDs are summarized in Table 4. CD-based sensors either directly interact with the analyte and show a change in fluorescence or are functionalized with recognition molecule(s), which detect(s) the target species (Long et al., 2021). In these sensing systems, primarily nanozymes oxidize the peroxide and form OH•. This OH• proceeds the conversion of substrate into product. These products exhibit different fluorescence, absorbance, and electric properties from those of the substrate. CD-based nanozymes exhibit higher colorimetric and fluorescent detection properties (Long et al., 2021).
Relative to all other analytical methods for sensing environmental pollutants, CD-based fluorescence detection is more efficient, rapid, and straightforward (Sun et al., 2017; Molaei, 2020; Devi et al., 2019). The colorimetric CD-based nanozymes are even more practical, cost-effective, and simpler than fluorescent sensors (Song et al., 2011). Several metal-doped CDs-based nanoparticles, such as CDs@polymoxometallate have been utilized in photocatalytic degradation of organic pollutants (Di et al., 2015). Hydrothermally synthesized, CD-stabilized Cu4O3 nanozymes have also been reported to be used in environmental treatments (Li F. et al., 2020). These hybrid nanozymes show a synergistic effect between peroxidase and oxidase-like catalytic activity, leading to the vigorous oxidation of organic dyes. Moreover, CD-stabilized Cu4O3 nanocomposites are highly stable, cost-effective, and versatile in photocatalytic applications. Sari et al. also fabricated Fe3O4/CDs photocatalysts via hydrothermal technique. These photocatalysts have been demonstrated for dye degradation. Moreover, Fe-N-CDs have been shown to exhibit peroxidase-like activity. Research reveals that the nitrogen doping in CDs (N-CDs) offers pollutant degradation activity. Similarly, Au-nanoparticle loaded graphitic carbon nanosheets offer degradation of organic pollutants. Li et al. synthesized CDs-stabilized Cu4O3 (CDs@Cu4O3) multi-enzyme mimetics by a hydrothermal method (Li F. et al., 2020). These nanozymes exhibit peroxidase (PD) and oxidase-like (OD) catalytic behavior. Interestingly, the synergistic enhancement effect of PD and OD results in fast oxidation of organic substrates such as methylene blue. Hence, CDs@Cu4O3 nanozymes offer wide-ranging uses in environmental treatments.
8.1 Degradation of antibiotics using peroxidase-like CDs
Antibiotics enter the environment in several ways, i.e., synthesis of therapeutic agents, excretion, and discharging of vacant medicines (Larsson, 2014). However, the released concentration of antibiotics through direct disposal from manufacturing sites is higher than that of industrial and excretion effluents. Compared to tetracyclines and fluoroquinolones, penicillin degrades quickly from the environment. The former groups of antibiotics amplify in the surroundings too. Antibiotic residues in water reservoirs eventually cause environmental and organismic health issues. Consequently, deleting antibiotic residues from the environment is necessary (Liu et al., 2024). The competence of CDs to induce peroxidase-like activity significantly enhances the degradation of antibiotics, leading to effective and advanced removal of such polluting agents from various environmental matrices. However, assessing the environmental and safety impacts of metal-doped CDs-based nanozymes is also significant. The presence of heme-consisting peroxidase in organisms utilizes hydrogen peroxide to catalyze the oxidation of various antibiotics. Likewise, the metalloenzymes play a significant role in the degradation of antibiotics. Hence, it is evident that peroxidase-like metal-doped CDs exhibit a promising role in the degradation of antibiotics from the environment. Similarly, CDs/g-C3N4/ZnO nanocomposites exhibit highly enhanced photocatalytic activities for the degradation of tetracycline (Guo et al., 2017). The presence of CDs in these nanocomposites significantly proceeds with the catalytic degradation mechanism of tetracycline.
Liu et al. fabricated magnetic NiFe2O4-carbon dots (NiFeC) with excellent renderability and enhanced TC-removing ability (Liu et al., 2017). The one-pot solvothermal method synthesized these nanocomposites using FeCl3 and EDTA-2Na as precursors. The high surface functionalities and saturation magnetization make NiFeC a promising candidate for TC adsorption from wastewater. Correspondingly, CDs-MgAl-LDHs@MnO2/Fe3O4 micromotors exhibited dual functionality, i.e., the detection and degradation of an oxytetracycline antibiotic from water (Liu et al., 2024). This dual functionality is due to enzymic activity and a self-propelled motion for diffusion in an aqueous solution. In the presence of hydrogen peroxide, these metal-containing CDs-based micromotors promote the degradation of oxytetracycline from water.
8.2 Degradation of pesticides using peroxidase-like CDs
Pesticides have many benefits, yet they give rise to severe environmental issues (Mahmood et al., 2016). They contaminate the environment differently, including excessive use, unchecked disposal, and run-off from crops and storage containers, etc., (Zacharia, 2011). However, their fate in environmental compartments may vary. Organophosphorus pesticides, triazine herbicides, and chlorinated insecticides are extensively utilized and seriously threaten the environment and biodiversity (Ma et al., 2023; Zacharia, 2011). In this regard, CDs’ peroxidase-like activity facilitates pesticide degradation through oxidative processes (Almaqdi et al., 2019). Utilizing metal-organic frameworks (MOFs) and CDs enhances the degradation potential, biocompatibility, specificity, and selectivity of nanozymes (Guan et al., 2012; Li P. et al., 2021). Fe-CDs@MOF-808 and Fe-CDs/MOF-808 nanoreactors were synthesized to detect and degrade parathion and paraoxon, respectively (Ma et al., 2023). Fe-CDs detect organophosphorus pesticides and cause the photocatalytic degradation of parathion (Figure 9).
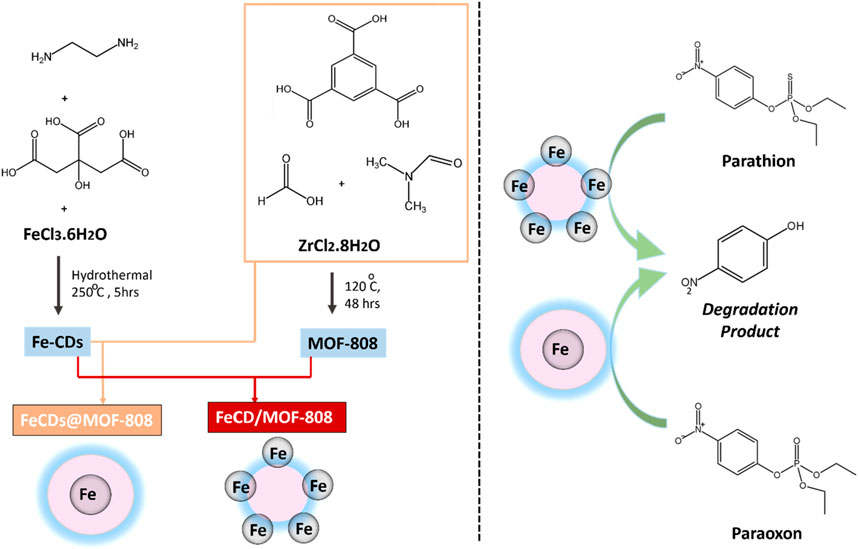
Figure 9. Schematic illustration of the synthesis of Fe-CDs@MOF-808 and Fe-CDs/MOF-808 nanoreactors and their deployment for the degradation of pesticides.
Peroxidase-like nanozymes based on metal-organic frameworks (MOFs) and CDs degrade atrazine (Zhang et al., 2022). Integrating MOFs and CDs results in a highly synergistic system for detecting and degrading atrazine. Depending on the composition of MOF-CD hybrid materials, the peroxidase-like activity could be controllable. Additionally, these nanozymes proceeded with the visual detection and photocatalytic degradation of atrazine in a single process. Furthermore, the heterometallic nanocomposites exhibit higher peroxidase-like activity than single metal or metal oxide-based nanocomposites. For instance, Fe3O4-TiO2/rGO nanocomposites exhibit excellent peroxidase-like activity toward the atrazine degradation (Duarah et al., 2022). In the future, this approach could be suitable for treating triazine herbicides.
8.3 Degradation of phenolics using peroxidase-like CDs
Phenolic compounds are diverse, ranging from simpler phenolic to polymerized compounds (Al Mamari, 2021). Agricultural, industrial, domestic, and natural discharge of these compounds majorly cause environmental pollution (Anku et al., 2017; Fernández et al., 2010). Due to their toxic, carcinogenic, and harmful effects on human health, it is essential to remove phenolic compounds from water (Anku et al., 2017; Rahman et al., 2021; Mu’azu et al., 2017). Carbon-based nanomaterial’s significant use and potential as peroxidase-mimetics has been demonstrated for the degradation (removal) of phenolic compounds from water (Zeng et al., 2017). Likewise, metal doping enhances the photocatalytic degradation of phenolic pollutants, too. For example, TiO2-P25 impregnated with Cr, Cu, and V metals enhances the Vis-light harvesting (Belekbir et al., 2020). Compared to TiO2-P25, the metal-impregnation in photocatalysts offers more effective removal. However, the mechanisms for enhanced photoactivation may vary for different metals. Correspondingly, the peroxidase-like conjugated system of deuterohemin-peptide and metal-organic framework (DhHP-6-c-ZrMOF) can also degrade phenol. DhHP-6-c-ZrMOF, which acts as a Fenton catalyst, can effectively degrade phenolic pollutants (Ding Y. et al., 2020).
Graphitic carbon nitride/copper-doped CDs nanocomposites (g-C3N4/Cu-CDs) offer the potential for the degradation of phenolics from water (Li Q. et al., 2022). They exhibit both oxidase and peroxidase-like catalytic activity. And the graphitic carbon nitride (g-C3N4) synergies the nanozymic-activity of Cu-CDs. These nanocomposites also detect phenolic compounds. Introducing H2O2 in the g-C3N4/Cu-CDs system enhances the oxidation of o-phenylenediamine (OPD) into a fluorescent compound 2,3-diaminophenazine (DAP). This oxidation process gives yellow fluorescence. However, phenolic substances with OPD + H2O2+g-C3N4/Cu-CDs (combined system) diminish the yellow fluorescence. This phenomenon helps to detect phenolic compounds in water. Moreover, the oxidase-like activity of g-C3N4/Cu-CDs converts the phenol and 4-aminoantipyrin into quinoeimine chromogen.
9 Novelties and challenges
Metal-doped and hybrid CDs as enzyme mimics are cutting-edge innovations, offering novelties in environmental remediations and research techniques. For instance, the nucleation of silver-doped and palladium-doped CDs (Ag@CDs and Pd@CDs) is a novel approach to discoloring methylene blue (MB) in daylight. Moreover, the incorporation of metals in CQDs corresponds to synergistic effects in the sensing and catalytic performance. Metal-doped CQDs have also shown the property of boosting electron transfer and photooxidation reactions. Owing to such properties, metal-doped CDs emerge as novel candidates in biomedical and environmental applications.
However, the research is still in its early stages. CDs lack systematic and ascendable synthesis methodology for developing cost-effective and reproducible applications compared to carbon nanomaterials. The effect of CD structures (such as size, morphology, presence of functional groups, and surface states), precursors, impurities, and reaction conditions (such as pH, time, and temperature) on the final product’s performance must be examined systematically. Owing to the non-standard synthetic routes, the exact formation process and nucleation mechanisms are also undefined. Moreover, the intrinsic mechanism of PL emission in CDs is unclear (Ai et al., 2021). It is also unclear how metal dopants enhance the fluorescence properties of carbon dots. To determine the PL mechanism to a greater extent, more research work (based on advanced structural characterization techniques such as spherical-aberration correction electron microscopy, synchronous X-ray radiation, and matrix-assisted laser desorption ionization time-of-flight mass spectroscopy, and theoretical examination) is required (Liu J. et al., 2020).
Similarly, metal doping on the CD’s center or edge remains challenging. Metal-doped CDs also lack proper characterization techniques to identify whether the dopants are integrated into the carbonaceous core’s lattice or CD’s surface. Metal ions exhibit redox properties, adversely affecting metal-doped CDs’ synthesis process. These ions may form metal oxides required to isolate from M-CDs. Incorporating metal ions in the carbon dots also introduces negative concerns, such as metal toxicity, synthesis complexity, limited stability, and increased cost considerations. Each system of nanozyme possesses a different performance, which can only be explained by a specific mechanism. Hence, further research is needed to understand the structure-activity relation thoroughly. Moreover, the environmental applications involve the direct contact of nanozymes with natural systems. Some CD-based nanozymes do not exhibit biosafety when exposed to the environment. This is because the degree of carbonization affects cytotoxicity. Therefore, it is necessary to have an in-depth understanding of safety protocols for using metal-doped CD-based nanozymes for practical use. Furthermore, metal-doped CD-based nanozymes are less diverse. It is also challenging to achieve high selectivity and specificity like natural enzymes. Multifunctional hybrid nanozymes are complex, too. They require intricate optimization strategies to reach multiple functionalities while maintaining stability and efficiency.
10 Authors’ viewpoint about notable drawbacks and suggestions to mitigate them
10.1 Toxicity and environmental impact due to metal leaching, biodegradability, and cytotoxicity concerns
Over time, the leaching of metal ions from metal-doped/metal-hybrid CDs is a must, considering that this limitation can cause significant environmental contamination and toxicity to aquatic organisms. For example, cadmium-doped CDs can potentially release toxic cadmium ions into water bodies to a certain extent. Over time, those leached cadmium ions can significantly bioaccumulate and transport through the food chain and pose serious health concerns to living beings. Likewise, the biodegradability of these metal-based CDs is a crucial concern that leads to long-term persistence in the environment. Such long-term persistence can lead to chronic exposure and adverse effects on the living environment. Moreover, some other metals used in doping, e.g., lead and mercury, are considered cytotoxic and can potentially cause cellular damage and/or DNA mutations.
10.2 Complex synthesis and scalability concerns
Multiple intricate steps, such as high-temperature treatments and the use of chemicals, complicate the production process. For example, fabricating gold-doped CDs requires specific control over reaction conditions, including reaction temperature, pH environment, and concentration of reactants to accomplish the anticipated size and functional attributes. Such complexity confines the scalability of production, as maintaining consistent quality within large batches can be challenging. Furthermore, reaction solvents and reagents can sometimes be toxic, pose safety risks, and require stringent handling and disposal protocols. This additionally poses another scalability concern to be considered effectively. Moreover, the need for specialized equipment and expertise further adds to large-scale production’s overall cost-effective ratio and complexity.
10.3 Catalytic efficiency limitations
Compared to natural enzymes, metal-doped or metal-based hybrid CDs, as enzyme mimics, may demonstrate lower catalytic efficiency, which can restrain their effectiveness and deployment in specific applications. For example, CDs are doped with iron to mimic CDs with peroxidase-like activity. However, iron-doped CDs often display decreased peroxidase-like activity owing to the lower density of active sites on their surface as compared to natural horseradish peroxidase. Such catalytic limitations hold back their implementation in pollutant degradation and/or biosensing applications. Though the addition of functional groups or co-doping with multiple metals can help improve catalytic performance. However, they cause other notable limitations, such as complex synthesis and scalability concerns. To avoid such limitations, understanding the reaction mechanisms at the molecular level can provide deeper insights into designing highly efficient catalysts.
10.4 Stability issues
Metal-doped or metal-based hybrid CDs can experience stability challenges over time due to metal agglomeration and surface deactivation, which may lessen their catalytic efficiency. A notable example is silver-doped CDs that may aggregate owing to the presence of strong van der Waals forces between silver, reducing surface area and loss of catalytic activity. Moreover, impurities or oxidation products cause reactive site hindrance that leads to the surface deactivation phenomenon, further diminishing both catalytic performance and stability. Therefore, ensuring long-term stability needs strategies, e.g., encapsulation techniques, to counteract agglomeration and maintain active surface availability.
10.5 Environmental and regulatory hurdles
Deploying metal-doped CDs in real-time environmental applications encounters substantial regulatory challenges due to potential environmental hazards. Therefore, strict safety assessments are needed to guarantee that these materials do not harm human health or ecosystems. For example, using copper-doped CDs in wastewater treatment must comply with environmental regulations that limit copper concentration to prevent toxicity. Regulatory agencies have been provided with comprehensive toxicity guidelines, environmental impact assessments, and monitoring plans that must be followed to maintain adequate and sustainable processing materials. In this context, collaborative efforts are equally needed by researchers, industry stakeholders, and regulatory bodies to develop standardized protocols and guidelines to limit such environmental and regulatory hurdles.
10.6 Suggestions to mitigate the drawbacks
Several strategies can be adopted to mitigate the drawbacks of metal-doped and hybrid carbon dots (CDs) as enzyme mimics in environmental applications. Firstly, researchers should explore safer doping elements and develop biodegradable CDs to address toxicity and environmental impact to reduce long-term persistence and ecological contamination. For complex synthesis and scalability issues, adopting green chemistry approaches and innovative synthesis methods, such as microwave-assisted synthesis, can simplify production and enhance eco-friendliness. Enhancing catalytic efficiency can be achieved through surface modification techniques, co-doping with multiple metals, and optimizing the doping process to increase the number of active sites. Stability issues can be mitigated by employing encapsulation techniques, such as coating them with protective polymers or silica shells and using metals that form stable complexes. Finally, addressing environmental and regulatory hurdles requires close alliance amongst researchers, industry stakeholders, and regulatory bodies to develop standardized testing protocols and ensure comprehensive safety assessments, enabling smoother regulatory approval and safer deployment of metal-doped CDs in environmental applications.
11 Conclusion and future directions
Carbon dots reveal distinctive properties such as strong fluorescence, high photostability, good biocompatibility, low toxicity, chemical stability, environment friendliness, derivatization ability, easy surface functionalization and enzyme-like activities. Owing to all these properties, the worth of CDs cannot be denied. The physicochemical properties of CDs can be tailored by hetero-atom doping. In this manner, metal doping has been demonstrated to enhance the chemical, electrical, optical, magnetic, and catalytic properties. Its significance involves high quantum yield, sensitive and precise analyte detection, and enhanced catalysis. Among numerous synthetic approaches, the hydrothermal synthesis of metal-doped CDs is more facile. Subsequently, the enzyme mimicking properties of metal-doped and hybrid carbon dots has increased the emphasis on environmental applications. The metal-doped and hybrid CDs nanozymes are stable, reusable, inexpensive, and potent substitutes for natural enzymes even under a wide range of pH, temperature, and concentrations. Among the oxidoreductase enzymes, the peroxidase and oxidase (laccase) mimetic metal-doped and hybrid carbon dots offer more excellent environmental applications such as fluorescent/colorimetric detection and degradation/photodegradation of heavy metals, pesticides, phenolic compounds, organic dyes, and pharmaceuticals (antibiotic-residues). However, the applications for the ‘degradation’ of pesticides using peroxidase-like metal-doped and hybrid CDs are limited.
Based on the recent reports’ studies, the following future directions are suggested: (1) In-depth research is required to enhance the emission wavelength of metal-doped CDs. (2) the construction of fluorescent ratio and signal response is needed for colorimetric and more sensitive analyte detection. (3) precise control over the synthetic process is required to ensure the synergistic interaction of different components within hybrid nanozymes. (4) Research should focus on optimizing the synthesis of metal-doped CDs to further control and improve catalytic activity and stability. (5) Research should underscore the detailed mechanisms of peroxidase-like activity of CDs, identifying the active sites and substrate binding sites. (6) It is essential to explore the diversity, cost-effectiveness, and scalability of producing metal-doped CDs for large-scale environmental remediations. (7) Bioinspiration will be helpful to explore more potentials of metal-doped CDs as enzyme mimics for the degradation of pollutants. In future studies, we expect metal-doped and hybrid carbon dots to be more extensively utilized in environmental applications.
Author contributions
AY: Writing – original draft, Writing – review and editing. MI: Conceptualization, Supervision, Writing – original draft, Writing – review and editing. MF: Writing – original draft, Writing – review and editing. IA: Writing – original draft, Writing – review and editing. FK: Writing – original draft, Writing – review and editing. RP-S: Writing – original draft, Writing – review and editing. GG-S: Writing – original draft, Writing – review and editing. HMNI: Conceptualization, Supervision, Writing – original draft, Writing – review and editing.
Funding
The author(s) declare that no financial support was received for the research and/or publication of this article.
Acknowledgments
The listed co-authors of this comprehensive review extend their genuine gratefulness to their corresponding associated institutes/universities for their valuable support in providing access to the required literature sources. This literature assistance was instrumental in the productive finishing point of this scholarly review work.
Conflict of interest
The authors declare that the research was conducted in the absence of any commercial or financial relationships that could be construed as a potential conflict of interest.
The author(s) declared that they were an editorial board member of Frontiers, at the time of submission. This had no impact on the peer review process and the final decision.
Generative AI statement
The author(s) declare that no Generative AI was used in the creation of this manuscript.
Publisher’s note
All claims expressed in this article are solely those of the authors and do not necessarily represent those of their affiliated organizations, or those of the publisher, the editors and the reviewers. Any product that may be evaluated in this article, or claim that may be made by its manufacturer, is not guaranteed or endorsed by the publisher.
References
Ai, L., Yang, Y., Wang, B., Chang, J., Tang, Z., Yang, B., et al. (2021). Insights into photoluminescence mechanisms of carbon dots: advances and perspectives. Sci. Bull. 66 (8), 839–856. doi:10.1016/j.scib.2020.12.015
Aitken, M. D., Massey, I., Chen, T., and Heck, P. E. (1994). Characterization of reaction products from the enzyme catalyzed oxidation of phenolic pollutants. Water Res. 28 (9), 1879–1889. doi:10.1016/0043-1354(94)90163-5
Alafeef, M., Srivastava, I., Aditya, T., and Pan, D. (2024). Carbon dots: from synthesis to unraveling the fluorescence mechanism. Small 20 (4), 2303937. doi:10.1002/smll.202303937
Al Mamari, H. H. (2021). “Phenolic compounds: Classification, chemistry, and updated techniques of analysis and synthesis,” in Phenolic compounds-chemistry, synthesis, diversity, non-conventional industrial, pharmaceutical and therapeutic applications. IntechOpen. doi:10.5772/intechopen.98958
Almaqdi, K. A., Morsi, R., Alhayuti, B., Alharthi, F., and Ashraf, S. S. (2019). LC-MSMS based screening of emerging pollutant degradation by different peroxidases. BMC Biotechnol. 19, 83–16. doi:10.1186/s12896-019-0574-y
Alqahtani, Y. S., Mahmoud, A. M., and El-Wekil, M. M. (2023). Bifunctional nanoprobe for dual-mode detection based on blue emissive iron and nitrogen co-doped carbon dots as a peroxidase-mimic platform. Talanta 253, 124024. doi:10.1016/j.talanta.2022.124024
Anku, W. W., Mamo, M. A., and Govender, P. P. (2017). “Phenolic compounds in water: sources, reactivity, toxicity and treatment methods,” in Phenolic compounds-natural sources, importance and applications. IntechOpen. doi:10.5772/66927
Ashrafi Tafreshi, F., Fatahi, Z., Ghasemi, S. F., Taherian, A., and Esfandiari, N. (2020). Ultrasensitive fluorescent detection of pesticides in real sample by using green carbon dots. Plos one 15 (3), e0230646. doi:10.1371/journal.pone.0230646
Awewomom, J., Dzeble, F., Takyi, Y. D., Ashie, W. B., Ettey, E. N. Y. O., Afua, P. E., et al. (2024). Addressing global environmental pollution using environmental control techniques: a focus on environmental policy and preventive environmental management. Discov. Environ. 2 (1), 8. doi:10.1007/s44274-024-00033-5
Ba, X.-X., Zhang, L., Yin, Y. L., Jiang, F. L., Jiang, P., and Liu, Y. (2020). Luminescent carbon dots with concentration-dependent emission in solution and yellow emission in solid state. J. colloid interface Sci. 565, 77–85. doi:10.1016/j.jcis.2020.01.007
Bacon, M., Bradley, S. J., and Nann, T. (2014). Graphene quantum dots. Part. and Part. Syst. Charact. 31 (4), 415–428. doi:10.1002/ppsc.201300252
Bai, J., Ma, Y., Yuan, G., Chen, X., Mei, J., Zhang, L., et al. (2019). Solvent-controlled and solvent-dependent strategies for the synthesis of multicolor carbon dots for pH sensing and cell imaging. J. Mater. Chem. C 7 (31), 9709–9718. doi:10.1039/c9tc02422k
Baker, S. N., and Baker, G. A. (2010). Luminescent carbon nanodots: emergent nanolights. Angew. Chem. Int. Ed. 49 (38), 6726–6744. doi:10.1002/anie.200906623
Bandi, R., Tummala, S., Dadigala, R., Alle, M., and Lee, S. H. (2022). “Role of metal-doped carbon dots in bioimaging and cancer therapy,” in Smart nanomaterials in biomedical applications (Springer, Cham: Springer International Publishing), 101–123. doi:10.1007/978-3-030-84262-8_4
Barman, M. K., and Patra, A. (2018). Current status and prospects on chemical structure driven photoluminescence behaviour of carbon dots. J. Photochem. Photobiol. C Photochem. Rev. 37, 1–22. doi:10.1016/j.jphotochemrev.2018.08.001
Belekbir, S., El Azzouzi, M., El Hamidi, A., Rodríguez-Lorenzo, L., Santaballa, J. A., and Canle, M. (2020). Improved photocatalyzed degradation of phenol, as a model pollutant, over metal-impregnated nanosized TiO2. Nanomaterials 10 (5), 996. doi:10.3390/nano10050996
Berglund, G. I., Carlsson, G. H., Smith, A. T., Szöke, H., Henriksen, A., and Hajdu, J. (2002). The catalytic pathway of horseradish peroxidase at high resolution. Nature 417 (6887), 463–468. doi:10.1038/417463a
Bhaisare, M. L., Talib, A., Khan, M. S., Pandey, S., and Wu, H. F. (2015). Synthesis of fluorescent carbon dots via microwave carbonization of citric acid in presence of tetraoctylammonium ion, and their application to cellular bioimaging. Microchim. Acta 182, 2173–2181. doi:10.1007/s00604-015-1541-5
Boakye-Yiadom, K. O., Kesse, S., Opoku-Damoah, Y., Filli, M. S., Aquib, M., Joelle, M. M. B., et al. (2019). Carbon dots: applications in bioimaging and theranostics. Int. J. Pharm. X. 564, 308–317. doi:10.1016/j.ijpharm.2019.04.055
Bourlinos, A. B., Rathi, A. K., Gawande, M. B., Hola, K., Goswami, A., Kalytchuk, S., et al. (2017). Fe (III)-functionalized carbon dots—highly efficient photoluminescence redox catalyst for hydrogenations of olefins and decomposition of hydrogen peroxide. Appl. Mater. Today 7, 179–184. doi:10.1016/j.apmt.2017.03.002
Cao, L., Meziani, M. J., Sahu, S., and Sun, Y. P. (2013). Photoluminescence properties of graphene versus other carbon nanomaterials. Accounts Chem. Res. 46 (1), 171–180. doi:10.1021/ar300128j
Cardoso, R. M., Cardoso, I. M. F., da Silva, L. P., and Esteves da Silva, J. C. G. (2022). Copper (II)-Doped carbon dots as catalyst for ozone degradation of textile dyes. Nanomaterials 12 (7), 1211. doi:10.3390/nano12071211
Chao, D., Chen, J., Dong, Q., Wu, W., Qi, D., and Dong, S. (2020). Ultrastable and ultrasensitive pH-switchable carbon dots with high quantum yield for water quality identification, glucose detection, and two starch-based solid-state fluorescence materials. Nano Res. 13, 3012–3018. doi:10.1007/s12274-020-2965-8
Chen, B. B., Liu, M. L., Li, C. M., and Huang, C. Z. (2019). Fluorescent carbon dots functionalization. Adv. Colloid Interface Sci. 270, 165–190. doi:10.1016/j.cis.2019.06.008
Chen, B. B., Liu, Z. X., Zou, H. Y., and Huang, C. Z. (2016a). Highly selective detection of 2, 4, 6-trinitrophenol by using newly developed terbium-doped blue carbon dots. Analyst 141 (9), 2676–2681. doi:10.1039/c5an02569a
Chen, D., Gao, H., Chen, X., Fang, G., Yuan, S., and Yuan, Y. (2017). Excitation-independent dual-color carbon dots: surface-state controlling and solid-state lighting. Acs Photonics 4 (9), 2352–2358. doi:10.1021/acsphotonics.7b00675
Chen, H., Xie, Y., Kirillov, A. M., Liu, L., Yu, M., Liu, W., et al. (2015). A ratiometric fluorescent nanoprobe based on terbium functionalized carbon dots for highly sensitive detection of an anthrax biomarker. Chem. Commun. 51 (24), 5036–5039. doi:10.1039/c5cc00757g
Chen, J., Xiao, G., Duan, G., Wu, Y., Zhao, X., and Gong, X. (2021). Structural design of carbon dots/porous materials composites and their applications. Chem. Eng. J. 421, 127743. doi:10.1016/j.cej.2020.127743
Chen, S., Liu, J. W., Chen, M. L., Chen, X. W., and Wang, J. H. (2012). Unusual emission transformation of graphene quantum dots induced by self-assembled aggregation. Chem. Commun. 48 (61), 7637–7639. doi:10.1039/c2cc32984k
Chen, Y., Lian, H., Wei, Y., He, X., Chen, Y., Wang, B., et al. (2018). Concentration-induced multi-colored emissions in carbon dots: origination from triple fluorescent centers. Nanoscale 10 (14), 6734–6743. doi:10.1039/c8nr00204e
Chen, Y., Qin, X., Yuan, C., Shi, R., and Wang, Y. (2020). Double responsive analysis of carbaryl pesticide based on carbon quantum dots and Au nanoparticles. Dyes Pigments 181, 108529. doi:10.1016/j.dyepig.2020.108529
Chen, Y., Xu, J., Liu, B., Li, J., Fang, X., Xiong, L., et al. (2016b). Enhanced photoluminescence properties of carbon dots by doping with europium. J. Nanosci. Nanotechnol. 16 (4), 3735–3738. doi:10.1166/jnn.2016.11800
Choudhary, N., Hwang, S., and Choi, W. (2014). “Carbon nanomaterials: a review,” in Handbook of nanomaterials properties, 709–769.
Cui, L., Ren, X., Sun, M., Liu, H., and Xia, L. (2021). Carbon dots: synthesis, properties and applications. Nanomaterials 11 (12), 3419. doi:10.3390/nano11123419
Dadkhah, S., Mehdinia, A., Jabbari, A., and Manbohi, A. (2022). Catalytic nanozyme Zn/Cl-doped carbon quantum dots as ratiometric fluorescent probe for sequential on-off-on detection of riboflavin, Cu2+ and thiamine. Sci. Rep. 12 (1), 18276. doi:10.1038/s41598-022-23055-6
Dager, A., Uchida, T., Maekawa, T., and Tachibana, M. (2019). Synthesis and characterization of mono-disperse carbon quantum dots from fennel seeds: photoluminescence analysis using machine learning. Sci. Rep. 9 (1), 14004. doi:10.1038/s41598-019-50397-5
Dang, Y. Q., Li, B., Feng, X., Jia, J., Li, K., and Zhang, Y. (2023). Preparation of iron-doped carbon dots and their application in photocatalytic reduction of carbon dioxide. ChemPhotoChem 7 (2), e202200156. doi:10.1002/cptc.202200156
Das, P., Ganguly, S., Bose, M., Mondal, S., Choudhary, S., Gangopadhyay, S., et al. (2018). Zinc and nitrogen ornamented bluish white luminescent carbon dots for engrossing bacteriostatic activity and Fenton based bio-sensor. Mater. Sci. Eng. C 88, 115–129. doi:10.1016/j.msec.2018.03.010
Das, S., Ngashangva, L., and Goswami, P. (2021a). Carbon dots: an emerging smart material for analytical applications. Micromachines 12 (1), 84. doi:10.3390/mi12010084
Das, S., Ngashangva, L., Mog, H., Gogoi, S., and Goswami, P. (2021b). An insight into the mechanism of peroxidase-like activity of carbon dots. Opt. Mater. (Amst). 115, 111017. doi:10.1016/j.optmat.2021.111017
Dasappa, S. M., and Loehr, R. C. (1991). Toxicity reduction in contaminated soil bioremediation processes. Water Res. 25 (9), 1121–1130. doi:10.1016/0043-1354(91)90205-5
Deka, M. J., Dutta, P., Sarma, S., Medhi, O. K., Talukdar, N., and Chowdhury, D. (2019). Carbon dots derived from water hyacinth and their application as a sensor for pretilachlor. Heliyon 5 (6), e01985. doi:10.1016/j.heliyon.2019.e01985
de Oliveira, F. K., Santos, L. O., and Buffon, J. G. J. F. R. I. (2021). Mechanism of action, sources, and application of peroxidases. Food Res. Int. 143, 110266. doi:10.1016/j.foodres.2021.110266
Devi, P., Rajput, P., Thakur, A., Kim, K. H., and Kumar, P. (2019). Recent advances in carbon quantum dot-based sensing of heavy metals in water. TrAC Trends Anal. Chem. 114, 171–195. doi:10.1016/j.trac.2019.03.003
Dhenadhayalan, N., Lin, K. C., and Saleh, T. A. J. S. (2020). Recent advances in functionalized carbon dots toward the design of efficient materials for sensing and catalysis applications. Small 16 (1), 1905767. doi:10.1002/smll.201905767
Dhiman, N., Ghosh, S., Mishra, Y. K., and Tripathi, K. M. (2022). Prospects of nano-carbons as emerging catalysts for enzyme-mimetic applications. Mater. Adv. 3 (7), 3101–3122. doi:10.1039/d2ma00034b
Di, J., Xia, J., Ge, Y., Li, H., Ji, H., Xu, H., et al. (2015). Novel visible-light-driven CQDs/Bi2WO6 hybrid materials with enhanced photocatalytic activity toward organic pollutants degradation and mechanism insight. Appl. Catal. B Environ. 168, 51–61. doi:10.1016/j.apcatb.2014.11.057
Ding, H., Li, X. H., Chen, X. B., Wei, J. S., Li, X. B., and Xiong, H. M. (2020a). Surface states of carbon dots and their influences on luminescence. J. Appl. Phys. 127 (23). doi:10.1063/1.5143819
Ding, Y., Li, Z., Jiang, W., Yuan, B., Huang, T., Wang, L., et al. (2020b). Degradation of phenol using a peroxidase mimetic catalyst through conjugating deuterohemin-peptide onto metal-organic framework with enhanced catalytic activity. Catal. Commun. 134, 105859. doi:10.1016/j.catcom.2019.105859
Dong, Y., Zhang, J., Jiang, P., Wang, G., Wu, X., Zhao, H., et al. (2015). Superior peroxidase mimetic activity of carbon dots–Pt nanocomposites relies on synergistic effects. New J. Chem. 39 (5), 4141–4146. doi:10.1039/c5nj00012b
Ðorđević, L., Arcudi, F., and Prato, M. J. N. P. (2019). Preparation, functionalization and characterization of engineered carbon nanodots. Nat. Protoc. 14 (10), 2931–2953. doi:10.1038/s41596-019-0207-x
Duan, Y., Huang, Y., Chen, S., Zuo, W., and Shi, B. (2019). Cu-doped carbon dots as catalysts for the chemiluminescence detection of glucose. Acs Omega 4 (6), 9911–9917. doi:10.1021/acsomega.9b00738
Duarah, R., Das, P., Boruah, P. K., and Das, M. R. (2022). Current perspective on the development of 2D nanosheets and their composites for photocatalytic degradation of organic pollutants. Heterogeneous Nanocatalysis Energy Environ. Sustain. 2, 95–115. doi:10.1002/9781119772057.ch18
Eda, G., Lin, Y., Mattevi, C., Yamaguchi, H., Chen, H., Chen, I., et al. (2010). Blue photoluminescence from chemically derived graphene oxide. Adv. Mater. 22 (4), 505–509. doi:10.1002/adma.200901996
El-Shafey, A. M. (2021). Carbon dots: discovery, structure, fluorescent properties, and applications. Green Process. Synthesis 10 (1), 134–156. doi:10.1515/gps-2021-0006
Fan, R.-J., Sun, Q., Zhang, L., Zhang, Y., and Lu, A. H. (2014). Photoluminescent carbon dots directly derived from polyethylene glycol and their application for cellular imaging. Carbon 71, 87–93. doi:10.1016/j.carbon.2014.01.016
Fang, J., Zhuo, S., and Zhu, C. (2019). Fluorescent sensing platform for the detection of p-nitrophenol based on Cu-doped carbon dots. Opt. Mater. 97, 109396. doi:10.1016/j.optmat.2019.109396
Fawaz, W., Hasian, J., and Alghoraibi, I. (2023). Synthesis and physicochemical characterization of carbon quantum dots produced from folic acid. Sci. Rep. 13 (1), 18641. doi:10.1038/s41598-023-46084-1
Feng, J., Dong, H., Pang, B., Shao, F., Zhang, C., Yu, L., et al. (2018b). Theoretical study on the optical and electronic properties of graphene quantum dots doped with heteroatoms. Phys. Chem. Chem. Phys. 20 (22), 15244–15252. doi:10.1039/c8cp01403e
Feng, L., Zhang, L., Chu, S., Zhang, S., Chen, X., Du, Z., et al. (2022). Controllable doping of Fe atoms into MoS2 nanosheets towards peroxidase-like nanozyme with enhanced catalysis for colorimetric analysis of glucose. Appl. Surf. Sci. 583, 152496. doi:10.1016/j.apsusc.2022.152496
Feng, Q., Xie, Z., and Zheng, M. (2021). Colour-tunable ultralong-lifetime room temperature phosphorescence with external heavy-atom effect in boron-doped carbon dots. Chem. Eng. J. 420, 127647. doi:10.1016/j.cej.2020.127647
Feng, S.-H., and Li, G.-H. (2017). “Hydrothermal and solvothermal syntheses,” in Modern inorganic synthetic chemistry (Elsevier), 73–104.
Feng, T., Zeng, Q., Lu, S., Yan, X., Liu, J., Tao, S., et al. (2018a). Color-tunable carbon dots possessing solid-state emission for full-color light-emitting diodes applications. Acs Photonics 5 (2), 502–510. doi:10.1021/acsphotonics.7b01010
Fernández, C., Larrechi, M. S., and Callao, M. P. (2010). An analytical overview of processes for removing organic dyes from wastewater effluents. TrAC Trends Anal. Chem. 29 (10), 1202–1211. doi:10.1016/j.trac.2010.07.011
Fu, Y., Zeng, G., Lai, C., Huang, D., Qin, L., Yi, H., et al. (2020). Hybrid architectures based on noble metals and carbon-based dots nanomaterials: a review of recent progress in synthesis and applications. Chem. Eng. J. 399, 125743. doi:10.1016/j.cej.2020.125743
Gallareta-Olivares, G., Rivas-Sanchez, A., Cruz-Cruz, A., Hussain, S. M., González-González, R. B., Cárdenas-Alcaide, M. F., et al. (2023). Metal-doped carbon dots as robust nanomaterials for the monitoring and degradation of water pollutants. Chemosphere 312, 137190. doi:10.1016/j.chemosphere.2022.137190
Gan, H., Han, W., Fu, Z., and Wang, L. (2021). The chain-like Au/carbon dots nanocomposites with peroxidase-like activity and their application for glucose detection. Colloids Surfaces B Biointerfaces 199, 111553. doi:10.1016/j.colsurfb.2020.111553
Gao, D., Zhang, Y., Liu, A., Zhu, Y., Chen, S., Wei, D., et al. (2020a). Photoluminescence-tunable carbon dots from synergy effect of sulfur doping and water engineering. Chem. Eng. J. 388, 124199. doi:10.1016/j.cej.2020.124199
Gao, L., Gao, X., and Yan, X. (2020b). “Kinetics and mechanisms for nanozymes,” in Nanozymology. Editors X. Yan (Springer, Singapore: Nanostructure Science and Technology), 17–39. doi:10.1007/978-981-15-1490-6_2
Gao, L., Zhuang, J., Nie, L., Zhang, J., Zhang, Y., Gu, N., et al. (2007). Intrinsic peroxidase-like activity of ferromagnetic nanoparticles. Nat. Nanotechnol. 2 (9), 577–583. doi:10.1038/nnano.2007.260
Ge, G., Li, L., Wang, D., Chen, M., Zeng, Z., Xiong, W., et al. (2021). Carbon dots: synthesis, properties and biomedical applications. J. Mater. Chem. B 9 (33), 6553–6575. doi:10.1039/d1tb01077h
Ge, L., Pan, N., Jin, J., Wang, P., LeCroy, G. E., Liang, W., et al. (2018). Systematic comparison of carbon dots from different preparations—consistent optical properties and photoinduced redox characteristics in visible spectrum and structural and mechanistic implications. J. Phys. Chem. C 122 (37), 21667–21676. doi:10.1021/acs.jpcc.8b06998
Ghaffarkhah, A., Hosseini, E., Kamkar, M., Sehat, A. A., Dordanihaghighi, S., Allahbakhsh, A., et al. (2022). Synthesis, applications, and prospects of graphene quantum dots: a comprehensive review. Small 18 (2), 2102683. doi:10.1002/smll.202102683
Guan, G., Yang, L., Mei, Q., Zhang, K., Zhang, Z., and Han, M. Y. (2012). Chemiluminescence switching on peroxidase-like Fe3O4 nanoparticles for selective detection and simultaneous determination of various pesticides. Anal. Chem. 84 (21), 9492–9497. doi:10.1021/ac302341b
Guo, F., Shi, W., Guan, W., Huang, H., and Liu, Y. (2017). Carbon dots/g-C3N4/ZnO nanocomposite as efficient visible-light driven photocatalyst for tetracycline total degradation. Sep. Purif. Technol. 173, 295–303. doi:10.1016/j.seppur.2016.09.040
Guo, F., Zhu, Z., Zheng, Z., Jin, Y., Di, X., Xu, Z., et al. (2020). Facile synthesis of highly efficient fluorescent carbon dots for tetracycline detection. Environ. Sci. Pollut. Res. 27, 4520–4527. doi:10.1007/s11356-019-06779-3
Guo, T., Wang, X., Shu, Y., and Wang, J. (2021). Effects of alkyl side-chain length on binding with bovine serum albumin, cytotoxicity, and antibacterial properties of 1-alkyl-3-methylimidazolium dicyanamide ionic liquids. J. Mol. Liq. 339, 116835. doi:10.1016/j.molliq.2021.116835
Guo, T., Zheng, A., Chen, X., Shu, Y., and Wang, J. (2019). The structure-activity relationship of hydrophilic carbon dots regulated by the nature of precursor ionic liquids. J. colloid interface Sci. 554, 722–730. doi:10.1016/j.jcis.2019.07.058
Guo, Y., Liu, X., Yang, C., Wang, X., Wang, D., Iqbal, A., et al. (2015). Synthesis and peroxidase-like activity of cobalt@ carbon-dots hybrid material. ChemCatChem 7 (16), 2467–2474. doi:10.1002/cctc.201500263
Habibi, E., and Heidari, H. (2016). Renewable surface carbon-composite electrode bulk modified with GQD-RuCl3 nano-composite for high sensitive detection of l-tyrosine. Electroanalysis 28 (10), 2559–2564. doi:10.1002/elan.201600010
Hamid, M. J. F. C., and Khalil-ur-Rehman, (2009). Potential applications of peroxidases. Food Chem. x. 115 (4), 1177–1186. doi:10.1016/j.foodchem.2009.02.035
Han, B., Peng, T., Yu, M., Chi, C., Li, Y., Hu, X., et al. (2018). One-pot synthesis of highly fluorescent Fe 2+-doped carbon dots for a dual-emissive nanohybrid for the detection of zinc ions and histidine. New J. Chem. 42 (16), 13651–13659. doi:10.1039/c8nj01858h
Han, Y., Yang, W., Luo, X., He, X., Yu, Y., Li, C., et al. (2019). Cu2+-triggered carbon dots with synchronous response of dual emission for ultrasensitive ratiometric fluorescence determination of thiophanate-methyl residues. J. Agric. food Chem. 67 (45), 12576–12583. doi:10.1021/acs.jafc.9b04720
Hao, Y.-N., Guo, H. L., Tian, L., and Kang, X. (2015). Enhanced photoluminescence of pyrrolic-nitrogen enriched graphene quantum dots. RSC Adv. 5 (54), 43750–43755. doi:10.1039/c5ra07745a
Hiremath, S. D., Priyadarshi, B., Banerjee, M., and Chatterjee, A. (2020). Carbon dots-MnO2 based turn-on fluorescent probe for rapid and sensitive detection of hydrazine in water. J. Photochem. Photobiol. A Chem. 389, 112258. doi:10.1016/j.jphotochem.2019.112258
Hu, B., Ma, Y., and Wang, N. (2020). A novel water pollution monitoring and treatment agent: Ag doped carbon nanoparticles for sensing dichromate, morphological analysis of Cr and sterilization. Microchem. J. 157, 104855. doi:10.1016/j.microc.2020.104855
Hu, C., Yin, Y., Huang, C., Dong, Y., Liu, J., Xiao, F., et al. (2024). Cobalt-nitrogen co-doped carbon dots for the colorimetric and fluorometric dual-mode detection of gallic acid. Microchem. J. 197, 109735. doi:10.1016/j.microc.2023.109735
Huang, Y., Ding, Z., Li, Y., Xi, F., and Liu, J. (2023). Magnetic nanozyme based on loading nitrogen-doped carbon dots on mesoporous Fe3O4 nanoparticles for the colorimetric detection of glucose. Molecules 28 (12), 4573. doi:10.3390/molecules28124573
Huang, Y., Ren, J., and Qu, X. J. C. R. (2019). Nanozymes: classification, catalytic mechanisms, activity regulation, and applications. Chem. Rev. 119 (6), 4357–4412. doi:10.1021/acs.chemrev.8b00672
Hui, K. C., Ang, W. L., and Sambudi, N. S. (2021). Nitrogen and bismuth-doped rice husk-derived carbon quantum dots for dye degradation and heavy metal removal. J. Photochem. Photobiol. A Chem. 418, 113411. doi:10.1016/j.jphotochem.2021.113411
Innocenzi, P., and Stagi, L. (2023). Carbon dots as oxidant-antioxidant nanomaterials, understanding the structure-properties relationship. A critical review. A Crit. Rev. Nano Today 50, 101837. doi:10.1016/j.nantod.2023.101837
Jana, J., Ganguly, M., and Pal, T. J. R. A. (2016). Enlightening surface plasmon resonance effect of metal nanoparticles for practical spectroscopic application. RSC Adv. 6 (89), 86174–86211. doi:10.1039/c6ra14173k
Jana, P., and Dev, A. (2022). Carbon quantum dots: a promising nanocarrier for bioimaging and drug delivery in cancer. Mater. Today Commun. 32, 104068. doi:10.1016/j.mtcomm.2022.104068
Jayaweera, S., Yin, K., Hu, X., and Ng, W. J. (2019). Fluorescent N/Al Co-doped carbon dots from cellulose biomass for sensitive detection of manganese (VII). J. Fluoresc. 29, 1291–1300. doi:10.1007/s10895-019-02452-7
Jiang, D., Ni, D., Rosenkrans, Z. T., Huang, P., Yan, X., and Cai, W. (2019). Nanozyme: new horizons for responsive biomedical applications. Chem. Soc. Rev. 48 (14), 3683–3704. doi:10.1039/c8cs00718g
Jin, H., Gui, R., Wang, Y., and Sun, J. (2017). Carrot-derived carbon dots modified with polyethyleneimine and nile blue for ratiometric two-photon fluorescence turn-on sensing of sulfide anion in biological fluids. Talanta 169, 141–148. doi:10.1016/j.talanta.2017.03.083
Jin, J., Li, L., Zhang, L., Luan, Z., Xin, S., and Song, K. (2021). Progress in the application of carbon dots-based nanozymes. Front. Chem. 9, 748044. doi:10.3389/fchem.2021.748044
Kaur, N., Tiwari, P., Abbas, Z., and Mobin, S. M. (2022a). Doxycycline detection and degradation in aqueous media via simultaneous synthesis of Fe-N@ carbon dots and Fe3O4-carbon dot hybrid nanoparticles: a one arrow two hawk approach. J. Mater. Chem. B 10 (27), 5251–5262. doi:10.1039/d2tb00475e
Kaur, N., Tiwari, P., Abbas, Z., and Mobin, S. M. (2022b). Doxycycline detection and degradation in aqueous media via simultaneous synthesis of Fe-N@ carbon dots and Fe 3 O 4-carbon dot hybrid nanoparticles: a one arrow two hawk approach. J. Mater. Chem. B 10 (27), 5251–5262. doi:10.1039/d2tb00475e
Khaledian, S., Noroozi-Aghideh, A., Kahrizi, D., Moradi, S., Abdoli, M., Ghasemalian, A. H., et al. (2021). Rapid detection of diazinon as an organophosphorus poison in real samples using fluorescence carbon dots. Inorg. Chem. Commun. 130, 108676. doi:10.1016/j.inoche.2021.108676
Khin, M. M., Nair, A. S., Babu, V. J., Murugan, R., and Ramakrishna, S. (2012). A review on nanomaterials for environmental remediation. Energy and Environ. Sci. 5 (8), 8075–8109. doi:10.1039/c2ee21818f
Kim, D., Chen, Z., Zhou, L., and Huang, S. (2018). Air pollutants and early origins of respiratory diseases. Chronic Dis. Transl. Med. 4 (2), 75–94. doi:10.1016/j.cdtm.2018.03.003
Kim, S., Hwang, S. W., Kim, M. K., Shin, D. Y., Shin, D. H., Kim, C. O., et al. (2012). Anomalous behaviors of visible luminescence from graphene quantum dots: interplay between size and shape. ACS nano 6 (9), 8203–8208. doi:10.1021/nn302878r
Korram, J., Dewangan, L., Nagwanshi, R., Karbhal, I., Ghosh, K. K., and Satnami, M. L. (2019). A carbon quantum dot–gold nanoparticle system as a probe for the inhibition and reactivation of acetylcholinesterase: detection of pesticides. New J. Chem. 43 (18), 6874–6882. doi:10.1039/c9nj00555b
Kumar, V. B., Porat, Z. E., and Gedanken, A. J. N. (2022). Synthesis of doped/hybrid carbon dots and their biomedical application. Nanomater. (Basel). 12 (6), 898. doi:10.3390/nano12060898
Kurdekar, A., Chunduri, L. A. A., Bulagonda, E. P., Haleyurgirisetty, M. K., Kamisetti, V., and Hewlett, I. K. (2016). Comparative performance evaluation of carbon dot-based paper immunoassay on Whatman filter paper and nitrocellulose paper in the detection of HIV infection. Microfluid. Nanofluidics 20, 99–13. doi:10.1007/s10404-016-1763-9
Kurian, M., and Paul, A. (2021). Recent trends in the use of green sources for carbon dot synthesis–A short review. Carbon Trends 3, 100032. doi:10.1016/j.cartre.2021.100032
Langer, M., Paloncýová, M., Medveď, M., Pykal, M., Nachtigallová, D., Shi, B., et al. (2021). Progress and challenges in understanding of photoluminescence properties of carbon dots based on theoretical computations. Appl. Mater. Today 22, 100924. doi:10.1016/j.apmt.2020.100924
Larsson, D. J. (2014). Antibiotics in the environment. Upsala J. Med. Sci. 119 (2), 108–112. doi:10.3109/03009734.2014.896438
Lei, C.-W., Hsieh, M.-L., and Liu, W.-R. (2019). A facile approach to synthesize carbon quantum dots with pH-dependent properties. Dyes Pigments 169, 73–80. doi:10.1016/j.dyepig.2019.05.014
Li, C., Zheng, Y., Ding, H., Jiang, H., and Wang, X. (2019a). Chromium (III)-doped carbon dots: fluorometric detection of p-nitrophenol via inner filter effect quenching. Microchim. Acta 186, 384–388. doi:10.1007/s00604-019-3444-3
Li, D., Jing, P., Sun, L., An, Y., Shan, X., Lu, X., et al. (2018a). Near-infrared excitation/emission and multiphoton-induced fluorescence of carbon dots. Adv. Mater. 30 (13), 1705913. doi:10.1002/adma.201705913
Li, F., Chang, Q., Li, N., Xue, C., Liu, H., Yang, J., et al. (2020b). Carbon dots-stabilized Cu4O3 for a multi-responsive nanozyme with exceptionally high activity. Chem. Eng. J. 394, 125045. doi:10.1016/j.cej.2020.125045
Li, M., Yang, Z., Chen, S., Liu, Z., Tong, L., Zheng, S., et al. (2023a). Sphaerotilus natans hemoglobins have an NADH oxidation activity and promote the yield of limonene in an engineered E. coli strain. Int. J. Biol. Macromol. 254, 128112. doi:10.1016/j.ijbiomac.2023.128112
Li, P., Sun, X.-Y., and Shen, J.-S. (2021c). A Multi-catalytic sensing for hydrogen peroxide, glucose, and organophosphorus pesticides based on carbon dots. Front. Chem. 9, 713104. doi:10.3389/fchem.2021.713104
Li, Q., Li, H., Li, K., Gu, Y., Wang, Y., Yang, D., et al. (2023b). Specific colorimetric detection of methylmercury based on peroxidase-like activity regulation of carbon dots/Au NPs nanozyme. J. Hazard. Mater. 441, 129919. doi:10.1016/j.jhazmat.2022.129919
Li, Q., Yang, D., Yin, Q., Li, W., and Yang, Y. (2022b). Graphitic carbon nitride nanosheets decorated with Cu-doped carbon dots for the detection and degradation of phenolic pollutants. ACS Appl. Nano Mater. 5 (2), 1925–1934. doi:10.1021/acsanm.1c03551
Li, S., Li, L., Tu, H., Zhang, H., Silvester, D. S., Banks, C. E., et al. (2021a). The development of carbon dots: from the perspective of materials chemistry. Mater. Today 51, 188–207. doi:10.1016/j.mattod.2021.07.028
Li, S., Liu, X., Chai, H., and Huang, Y. (2018b). Recent advances in the construction and analytical applications of metal-organic frameworks-based nanozymes. TrAC Trends Anal. Chem. 105, 391–403. doi:10.1016/j.trac.2018.06.001
Li, S., Pang, E., Gao, C., Chang, Q., and Hu, S. (2020a). Cerium-mediated photooxidation for tuning pH-dependent oxidase-like activity. Chem. Eng. J. 397, 125471. doi:10.1016/j.cej.2020.125471
Li, X., Fu, Y., Zhao, S., Xiao, J., Lan, M., Wang, B., et al. (2022a). Metal ions-doped carbon dots: synthesis, properties, and applications. Chem. Eng. J. 430, 133101. doi:10.1016/j.cej.2021.133101
Li, Y., Weng, Y., Lu, S., Xue, M., Yao, B., Weng, W., et al. (2020c). One-step hydrothermal synthesis of N, Fe-codoped carbon dots as mimic peroxidase and application on hydrogen peroxide and glucose detection. J. Nanomater. 2020, 1–11. doi:10.1155/2020/1363212
Li, Y., Zhang, B. P., Zhao, J. X., Ge, Z. H., Zhao, X. K., and Zou, L. (2013). ZnO/carbon quantum dots heterostructure with enhanced photocatalytic properties. Appl. Surf. Sci. 279, 367–373. doi:10.1016/j.apsusc.2013.04.114
Li, Y.-F., Lin, Z. Z., Hong, C. Y., and Huang, Z. Y. (2021b). Colorimetric detection of putrescine and cadaverine in aquatic products based on the mimic enzyme of (Fe, Co) codoped carbon dots. J. Food Meas. Charact. 15, 1747–1753. doi:10.1007/s11694-020-00782-w
Li, Z., Ye, R., Feng, R., Kang, Y., Zhu, X., Tour, J. M., et al. (2015). Graphene quantum dots doping of MoS2 monolayers. Adv. Mater. 27 (35), 5235–5240. doi:10.1002/adma.201501888
Li, Z., Wang, L., Li, Y., Feng, Y., and Feng, W. (2019b). Frontiers in carbon dots: design, properties and applications. Mater. Chem. Front. 3 (12), 2571–2601. doi:10.1039/c9qm00415g
Liang, C., Lan, Y., Sun, Z., Zhou, L., Li, Y., Liang, X., et al. (2020). Synthesis of carbon quantum dots with iron and nitrogen from Passiflora edulis and their peroxidase-mimicking activity for colorimetric determination of uric acid. Microchim. Acta 187, 405–410. doi:10.1007/s00604-020-04391-8
Lin, L., Luo, Y., Tsai, P., Wang, J., and Chen, X. (2018). Metal ions doped carbon quantum dots: synthesis, physicochemical properties, and their applications. Chem. Eng. J. 103, 87–101. doi:10.1016/j.trac.2018.03.015
Lin, L., Xiao, Y., Wang, Y., Zeng, Y., Lin, Z., and Chen, X. (2019). Hydrothermal synthesis of nitrogen and copper co-doped carbon dots with intrinsic peroxidase-like activity for colorimetric discrimination of phenylenediamine isomers. Microchim. Acta 186, 288–8. doi:10.1007/s00604-019-3404-y
Liu, C., Li, J., Zuo, M., Ng, D. H., Yang, X., Gao, S., et al. (2024). Synthesis of carbon dot LDHs@ MnO2 tubular magnetic micromotors for detection and degradation of oxytetracycline. Sep. Purif. Technol. 340, 126650. doi:10.1016/j.seppur.2024.126650
Liu, F., Zhang, W., Chen, W., Wang, J., Yang, Q., Zhu, W., et al. (2017). One-pot synthesis of NiFe2O4 integrated with EDTA-derived carbon dots for enhanced removal of tetracycline. Chem. Eng. J. 310, 187–196. doi:10.1016/j.cej.2016.10.116
Liu, J., Li, R., and Yang, B. (2020b). Carbon dots: a new type of carbon-based nanomaterial with wide applications. ACS Central Sci. 6 (12), 2179–2195. doi:10.1021/acscentsci.0c01306
Liu, M. (2020). Optical properties of carbon dots: a review. Nanoarchitectonics 1, 1–12. doi:10.37256/nat.112020124.1-12
Liu, W., Li, C., Ren, Y., Sun, X., Pan, W., Li, Y., et al. (2016). Carbon dots: surface engineering and applications. J. Mater. Chem. B 4 (35), 5772–5788. doi:10.1039/c6tb00976j
Liu, X., Yang, C., Zheng, B., Dai, J., Yan, L., Zhuang, Z., et al. (2018a). Green anhydrous synthesis of hydrophilic carbon dots on large-scale and their application for broad fluorescent pH sensing. Microchem. J. 255, 572–579. doi:10.1016/j.snb.2017.08.101
Liu, Y., Chao, D., Zhou, L., Li, Y., Deng, R., and Zhang, H. (2018b). Yellow emissive carbon dots with quantum yield up to 68.6% from manganese ions. Carbon N. Y. 135, 253–259. doi:10.1016/j.carbon.2018.02.004
Liu, Y., Huang, H., Cao, W., Mao, B., and Kang, Z. (2020a). Advances in carbon dots: from the perspective of traditional quantum dots. Mater. Chem. Front. 4 (6), 1586–1613. doi:10.1039/d0qm00090f
Long, C., Jiang, Z., Shangguan, J., Qing, T., Zhang, P., and Feng, B. (2021). Applications of carbon dots in environmental pollution control: a review. Chem. Eng. J. 406, 126848. doi:10.1016/j.cej.2020.126848
Long, Y., Wang, X., Shen, D., and Zheng, H. (2016). Detection of glucose based on the peroxidase-like activity of reduced state carbon dots. Talanta 159, 122–126. doi:10.1016/j.talanta.2016.06.012
Lopez-Cantu, D. O., González-González, R. B., Melchor-Martínez, E. M., Martínez, S. A. H., Araújo, R. G., Parra-Arroyo, L., et al. (2022). Enzyme-mimicking capacities of carbon-dots nanozymes: properties, catalytic mechanism, and applications–A review. Int. J. Biol. Macromol. 194, 676–687. doi:10.1016/j.ijbiomac.2021.11.112
Lv, W., Lin, M., Li, R., Zhang, Q., Liu, H., Wang, J., et al. (2019). Aggregation-induced emission enhancement of yellow photoluminescent carbon dots for highly selective detection of environmental and intracellular copper (II) ions. Chin. Chem. Lett. 30 (7), 1410–1414. doi:10.1016/j.cclet.2019.04.011
Ma, X., Ou, Q., Yuan, J., Yang, J., Xu, S., and Zhang, X. (2023). Multifunctional Fe-doped carbon dots and metal-organic frameworks nanoreactor for cascade degradation and detection of organophosphorus pesticides. Chem. Eng. J. 464, 142480. doi:10.1016/j.cej.2023.142480
Ma, Y., Cen, Y., Sohail, M., Xu, G., Wei, F., Shi, M., et al. (2017). A ratiometric fluorescence universal platform based on N, Cu codoped carbon dots to detect metabolites participating in H2O2-generation reactions. ACS Appl. Mater. Interfaces 9 (38), 33011–33019. doi:10.1021/acsami.7b10548
Mahmood, I., Imadi, S. R., Shazadi, K., Gul, A., and Hakeem, K. R. (2016). Effects of pesticides on environment. Plant, soil microbes volume 1 Implic. crop Sci., 253–269. doi:10.1007/978-3-319-27455-3_13
Malmir, S., Karbalaei, A., Pourmadadi, M., Hamedi, J., Yazdian, F., and Navaee, M. (2020). Antibacterial properties of a bacterial cellulose CQD-TiO2 nanocomposite. Carbohydr. Polym. 234, 115835. doi:10.1016/j.carbpol.2020.115835
Manohara, H. M., Aruchamy, K., Chakraborty, S., Radha, N., Nidhi, M. R., Ghosh, D., et al. (2019). Sustainable water purification using an engineered solvothermal carbon based membrane derived from a eutectic system. ACS Sustain. Chem. Eng. 7 (11), 10143–10153. doi:10.1021/acssuschemeng.9b01983
Mansuriya, B. D., and Altintas, Z. J. N. (2021). Carbon Dots: classification, properties, synthesis, characterization, and applications in health care—an updated review (2018–2021). Nanomaterials 11 (10), 2525. doi:10.3390/nano11102525
Mansuriya, B. D., and Altintas, Z. J. S. (2020). Applications of graphene quantum dots in biomedical sensors. Sensors 20 (4), 1072. doi:10.3390/s20041072
Meng, X., Chang, Q., Xue, C., Yang, J., and Hu, S. (2017). Full-colour carbon dots: from energy-efficient synthesis to concentration-dependent photoluminescence properties. Chem. Commun. 53 (21), 3074–3077. doi:10.1039/c7cc00461c
Miao, S., Liang, K., Zhu, J., Yang, B., Zhao, D., and Kong, B. (2020). Hetero-atom-doped carbon dots: doping strategies, properties and applications. Nano Today 33, 100879. doi:10.1016/j.nantod.2020.100879
Miao, X., Qu, D., Yang, D., Nie, B., Zhao, Y., Fan, H., et al. (2018). Synthesis of carbon dots with multiple color emission by controlled graphitization and surface functionalization. Adv. Mater. 30 (1), 1704740. doi:10.1002/adma.201704740
Mintz, K. J., Bartoli, M., Rovere, M., Zhou, Y., Hettiarachchi, S. D., Paudyal, S., et al. (2021). A deep investigation into the structure of carbon dots. Carbon 173, 433–447. doi:10.1016/j.carbon.2020.11.017
Mohammadpour, Z., Safavi, A., and Shamsipur, M. (2014). A new label free colorimetric chemosensor for detection of mercury ion with tunable dynamic range using carbon nanodots as enzyme mimics. Chem. Eng. J. 255, 1–7. doi:10.1016/j.cej.2014.06.012
Mokoloko, L. L., Forbes, R. P., and Coville, N. J. J. C. (2023). The behavior of carbon dots in catalytic reactions. Catalysts 13 (8), 1201. doi:10.3390/catal13081201
Molaei, M. (2020). Principles, mechanisms, and application of carbon quantum dots in sensors: a review. Anal. Methods 12 (10), 1266–1287. doi:10.1039/c9ay02696g
Moradlou, O., Rabiei, Z., and Delavari, N. (2019). Antibacterial effects of carbon quantum dots@ hematite nanostructures deposited on titanium against Gram-positive and Gram-negative bacteria. J. Photochem. Photobiol. A Chem. 379, 144–149. doi:10.1016/j.jphotochem.2019.04.047
Mou, Z., Zhang, H., Liu, Z., Sun, J., and Zhu, M. (2019). Ultrathin BiOCl/nitrogen-doped graphene quantum dots composites with strong adsorption and effective photocatalytic activity for the degradation of antibiotic ciprofloxacin. Appl. Surf. Sci. 496, 143655. doi:10.1016/j.apsusc.2019.143655
Mu, Z., Hua, J., Kumar Tammina, S., and Yang, Y. (2019). Visible light photocatalytic activity of Cu, N co-doped carbon dots/Ag3PO4 nanocomposites for neutral red under green LED radiation. Colloids Surfaces A Physicochem. Eng. Aspects 578, 123643. doi:10.1016/j.colsurfa.2019.123643
Mu’azu, N. D., Jarrah, N., Zubair, M., and Alagha, O. (2017). Removal of phenolic compounds from water using sewage sludge-based activated carbon adsorption: a review. Int. J. Environ. Res. public health 14 (10), 1094. doi:10.3390/ijerph14101094
Nandi, S., Malishev, R., Parambath Kootery, K., Mirsky, Y., Kolusheva, S., and Jelinek, R. (2014). Membrane analysis with amphiphilic carbon dots. Chem. Commun. 50 (71), 10299–10302. doi:10.1039/c4cc03504f
Ndlwana, L., Raleie, N., Dimpe, K. M., Ogutu, H. F., Oseghe, E. O., Motsa, M. M., et al. (2021). Sustainable hydrothermal and solvothermal synthesis of advanced carbon materials in multidimensional applications: a review. Materials 14 (17), 5094. doi:10.3390/ma14175094
Neill, S., Desikan, R., and Hancock, J. (2002). Hydrogen peroxide signalling. Curr. Opin. Plant Biol. 5 (5), 388–395. doi:10.1016/s1369-5266(02)00282-0
Nejad, M. A. F., Bigdeli, A., and Hormozi-Nezhad, M. R. (2020). Wide color-varying visualization of sulfide with a dual emissive ratiometric fluorescence assay using carbon dots and gold nanoclusters. Microchem. J. 157, 104960. doi:10.1016/j.microc.2020.104960
Ngo, Y.-L. T., Nguyen, P. L., Jana, J., Choi, W. M., Chung, J. S., and Hur, S. H. (2021). Simple paper-based colorimetric and fluorescent glucose sensor using N-doped carbon dots and metal oxide hybrid structures. Anal. Chim. Acta X. 1147, 187–198. doi:10.1016/j.aca.2020.11.023
Nguyen, B. H., Nguyen, V. H., and Vu, D. L. (2015). Photocatalytic composites based on titania nanoparticles and carbon nanomaterials. Adv. Nat. Sci. Nanosci. Nanotechnol. 6 (3), 033001. doi:10.1088/2043-6262/6/3/033001
Nie, H., Li, M., Li, Q., Liang, S., Tan, Y., Sheng, L., et al. (2014). Carbon dots with continuously tunable full-color emission and their application in ratiometric pH sensing. Chem. Mater. 26 (10), 3104–3112. doi:10.1021/cm5003669
Omer, K. M., Idrees, S. A., Hassan, A. Q., and Jamil, L. A. (2020). Amphiphilic fluorescent carbon nanodots as a selective nanoprobe for nitrite and tetracycline both in aqueous and organic solutions. New J. Chem. 44 (13), 5120–5126. doi:10.1039/d0nj00435a
Ozyurt, D., Kobaisi, M. A., Hocking, R. K., and Fox, B. (2023). Properties, synthesis, and applications of carbon dots: a review. Carbon Trends 12, 100276. doi:10.1016/j.cartre.2023.100276
Pandit, S., Mondal, S., and De, M. (2021). Surface engineered amphiphilic carbon dots: solvatochromic behavior and applicability as a molecular probe. J. Mater. Chem. B 9 (5), 1432–1440. doi:10.1039/d0tb02007a
Papaioannou, N., Titirici, M.-M., and Sapelkin, A. (2019). Investigating the effect of reaction time on carbon dot formation, structure, and optical properties. ACS omega 4 (26), 21658–21665. doi:10.1021/acsomega.9b01798
Park, Y., Yoo, J., Lim, B., Kwon, W., and Rhee, S. W. (2016). Improving the functionality of carbon nanodots: doping and surface functionalization. J. Mater. Chem. A Mater. 4 (30), 11582–11603. doi:10.1039/c6ta04813g
Passardi, F., Cosio, C., Penel, C., and Dunand, C. (2005). Peroxidases have more functions than a Swiss army knife. Plant Cell Rep. 24, 255–265. doi:10.1007/s00299-005-0972-6
Pathak, R., Punetha, V. D., Bhatt, S., and Punetha, M. (2023). Multifunctional role of carbon dot-based polymer nanocomposites in biomedical applications: a review. J. Mater. Sci. 58 (15), 6419–6443. doi:10.1007/s10853-023-08408-4
Pedrueza, E., Segura, A., Abargues, R., Bailach, J. B., Chervin, J. C., and Martínez-Pastor, J. P. (2013). The effect of quantum size confinement on the optical properties of PbSe nanocrystals as a function of temperature and hydrostatic pressure. Nanotechnology 24 (20), 205701. doi:10.1088/0957-4484/24/20/205701
Peng, H., Li, Y., Jiang, C., Luo, C., Qi, R., Huang, R., et al. (2016). Tuning the properties of luminescent nitrogen-doped carbon dots by reaction precursors. Carbon 100, 386–394. doi:10.1016/j.carbon.2016.01.029
Peng, J., Yin, W., Shi, J., Jin, X., and Ni, G. (2019). Magnesium and nitrogen co-doped carbon dots as fluorescent probes for quenchometric determination of paraoxon using pralidoxime as a linker. Microchim. Acta 186, 24–29. doi:10.1007/s00604-018-3147-1
Peng, P., Chen., L., Yan, X., Bai, R., Adyari, B., Zhou, X., et al. (2024). N-doped carbon dots/g-C3N4 photoexcitation simultaneously enhanced the microbial degradation of nitrate and oxytetracycline in a low C/N ratio aquaculture wastewater. Chem. Eng. J. 491, 151763. doi:10.1016/j.cej.2024.151763
Phophayu, S., Pimpang, P., Wongrerkdee, S., Sujinnapram, S., and Wongrerkdee, S. (2020). Modified graphene quantum dots-zinc oxide nanocomposites for photocatalytic degradation of organic dyes and commercial herbicide. J. Reinf. Plast. Compos. 39 (3-4), 81–94. doi:10.1177/0731684419891245
Qin, L., Gan, J., Niu, D., Cao, Y., Duan, X., Qin, X., et al. (2022). Interfacial-confined coordination to single-atom nanotherapeutics. Nat. Commun. 13 (1), 91. doi:10.1038/s41467-021-27640-7
Qin, X., Lu, Y., Bian, M., Xiao, Z., Zhang, Y., and Yuan, Y. (2019b). Influence of gold nanoparticles in different aggregation states on the fluorescence of carbon dots and its application. Anal. Chim. Acta 1091, 119–126. doi:10.1016/j.aca.2019.09.031
Qin, Z., Wang, W., Zhan, X., Du, X., Zhang, Q., Zhang, R., et al. (2019a). One-pot synthesis of dual carbon dots using only an N and S co-existed dopant for fluorescence detection of Ag+. Spectrochimica Acta Part A Mol. Biomol. Spectrosc. 208, 162–171. doi:10.1016/j.saa.2018.10.004
Qing, W., Chen, K., Yang, Y., Wang, Y., and Liu, X. (2020). Cu2+-doped carbon dots as fluorescence probe for specific recognition of Cr (VI) and its antimicrobial activity. Microchem. J. 152, 104262. doi:10.1016/j.microc.2019.104262
Qiu, M., Guo, P., Lei, X., Li, Y., Li, S., Meng, R., et al. (2024). Core-shell spherical cerium oxide, N-doped carbon dots and copper oxide nanocomposites with oxidase-like activity for colorimetric detection of hexavalent chromiums. J. Environ. Chem. Eng. 12, 112334. doi:10.1016/j.jece.2024.112334
Qu, D., Zheng, M., Li, J., Xie, Z., and Sun, Z. (2015). Tailoring color emissions from N-doped graphene quantum dots for bioimaging applications. Light Sci. and Appl. 4 (12), e364. doi:10.1038/lsa.2015.137
Qu, S., Zhou, D., Li, D., Ji, W., Jing, P., Han, D., et al. (2016). Toward efficient orange emissive carbon nanodots through conjugated sp2-domain controlling and surface charges engineering. Adv. Mater. 28 (18), 3516–3521. doi:10.1002/adma.201504891
Radhakrishnan, K., and Panneerselvam, P. (2018). Green synthesis of surface-passivated carbon dots from the prickly pear cactus as a fluorescent probe for the dual detection of arsenic (iii) and hypochlorite ions from drinking water. RSC Adv. 8 (53), 30455–30467. doi:10.1039/c8ra05861j
Rahman, M. M., Rahaman, M. S., Islam, M. R., Rahman, F., Mithi, F. M., Alqahtani, T., et al. (2021). Role of phenolic compounds in human disease: current knowledge and future prospects. Molecules 27 (1), 233. doi:10.3390/molecules27010233
Ranjan, P., Khan, R., Yadav, S., Sadique, M. A., Murali, S., Ban, M. K., et al. (2022). “Physical and chemical properties of carbon dots,” in Carbon dots in agricultural systems (Academic Press), 117–133. doi:10.1016/B978-0-323-90260-1.00005-X
Ren, X., Liu, J., Ren, J., Tang, F., and Meng, X. (2015). One-pot synthesis of active copper-containing carbon dots with laccase-like activities. Nanoscale 7 (46), 19641–19646. doi:10.1039/c5nr04685h
Ren, X., Zhang, F., Guo, B., Gao, N., and Zhang, X. (2019). Synthesis of N-doped micropore carbon quantum dots with high quantum yield and dual-wavelength photoluminescence emission from biomass for cellular imaging. Nanomaterials 9 (4), 495. doi:10.3390/nano9040495
Riyazuddin, R., Singh, K., Iqbal, N., Nisha, N., Rani, A., Kumar, M., et al. (2023). Iodine: an emerging biostimulant of growth and stress responses in plants. Plant Soil 486 (1-2), 119–133. doi:10.1007/s11104-022-05750-5
Roushani, M., Ghanbari, K., and Hoseini, S. (2018). Designing an electrochemical aptasensor based on immobilization of the aptamer onto nanocomposite for detection of the streptomycin antibiotic. Micro. J. 141, 96–103.
Ru, Y., Ai, L., Jia, T., Liu, X., Lu, S., Tang, Z., et al. (2020). Recent advances in chiral carbonized polymer dots: from synthesis and properties to applications. Nano Today 34, 100953. doi:10.1016/j.nantod.2020.100953
Rui, S., Song, L., Lan, J., Wang, D., Feng, S., Lu, J., et al. (2023). Recent advances in carbon dots-based nanoplatforms: physicochemical properties and biomedical applications. Chem. Eng. J. 476, 146593. doi:10.1016/j.cej.2023.146593
Sachdev, A., and Gopinath, P. J. C. (2016). Monitoring the intracellular distribution and ROS scavenging potential of carbon dot–cerium oxide nanocomposites in fibroblast cells. ChemNanoMat 2 (3), 226–235. doi:10.1002/cnma.201500224
Sagbas, S., and Sahiner, N. (2019). “Carbon dots: preparation, properties, and application,” in Nanocarbon and its composites (Elsevier), 651–676.
Sanni, S. O., Moundzounga, T. H. G., Oseghe, E. O., Haneklaus, N. H., Viljoen, E. L., and Brink, H. G. (2022). One-step green synthesis of water-soluble fluorescent carbon dots and its application in the detection of Cu2+. Nanomaterials 12 (6), 958. doi:10.3390/nano12060958
Shamsipur, M., Safavi, A., and Mohammadpour, Z. (2014). Indirect colorimetric detection of glutathione based on its radical restoration ability using carbon nanodots as nanozymes. Sensors Actuators B Chem. 199, 463–469. doi:10.1016/j.snb.2014.04.006
Shekarbeygi, Z., Farhadian, N., Ansari, M., Shahlaei, M., and Moradi, S. (2020). An innovative green sensing strategy based on Cu-doped Tragacanth/Chitosan nano carbon dots for Isoniazid detection. Spectrochimica Acta Part A Mol. Biomol. Spectrosc. 228, 117848. doi:10.1016/j.saa.2019.117848
Shen, H., Song, J., Yang, Y., Su, P., and Yang, Y. (2019). DNA-directed enzyme immobilization on Fe3O4 modified with nitrogen-doped graphene quantum dots as a highly efficient and stable multi-catalyst system. J. Mater. Sci. 54 (3), 2535–2551. doi:10.1007/s10853-018-2992-y
Shen, Y., Zhang, R., and Wang, Y. (2023). One-pot hydrothermal synthesis of metal-doped carbon dot nanozymes using protein cages as precursors. RSC Adv. 13 (10), 6760–6767. doi:10.1039/d2ra07222j
Shi, B., Su, Y., Duan, Y., Chen, S., and Zuo, W. (2019). A nanocomposite prepared from copper (II) and nitrogen-doped graphene quantum dots with peroxidase mimicking properties for chemiluminescent determination of uric acid. Microchim. Acta 186, 397–410. doi:10.1007/s00604-019-3491-9
Shi, W., Fan, H., Ai, S., and Zhu, L. (2015). Preparation of fluorescent graphene quantum dots from humic acid for bioimaging application. New J. Chem. 39 (9), 7054–7059. doi:10.1039/c5nj00760g
Shi, W., Gao, J., Sun, H., Liu, Z., Guo, F., and Wang, L. (2022). Highly efficient visible/near-infrared light photocatalytic degradation of antibiotic wastewater over 3D yolk-shell ZnFe2O4 supported 0D carbon dots with up-conversion property. Chin. J. Chem. Eng. 49, 213–223. doi:10.1016/j.cjche.2021.11.026
Shi, W., Guo, F., Wang, H., Liu, C., Fu, Y., Yuan, S., et al. (2018). Carbon dots decorated magnetic ZnFe2O4 nanoparticles with enhanced adsorption capacity for the removal of dye from aqueous solution. Appl. Surf. Sci. 433, 790–797. doi:10.1016/j.apsusc.2017.10.099
Shi, W., Wang, Q., Long, Y., Cheng, Z., Chen, S., Zheng, H., et al. (2011). Carbon nanodots as peroxidase mimetics and their applications to glucose detection. Chem. Commun. 47 (23), 6695–6697. doi:10.1039/c1cc11943e
Shi, X., Wang, X., Zhang, S., Zhang, Z., Meng, X., Liu, H., et al. (2023). Hydrophobic carbon dots derived from organic pollutants and applications in NIR anticounterfeiting and bioimaging. Langmuir 39 (14), 5056–5064. doi:10.1021/acs.langmuir.3c00075
Shigeto, J., and Tsutsumi, Y. (2016). Diverse functions and reactions of class III peroxidases. New Phytol. 209 (4), 1395–1402. doi:10.1111/nph.13738
Siddique, A. B., Pramanick, A. K., Chatterjee, S., and Ray, M. (2018). Amorphous carbon dots and their remarkable ability to detect 2, 4, 6-trinitrophenol. Sci. Rep. 8 (1), 9770. doi:10.1038/s41598-018-28021-9
Singh, I., Arora, R., Dhiman, H., and Pahwa, R. (2018). Carbon quantum dots: synthesis, characterization and biomedical applications. Turk. J. Pharm. Sci. 15 (2), 219–230. doi:10.4274/tjps.63497
Sk, M. A., Ananthanarayanan, A., Huang, L., Lim, K. H., and Chen, P. (2014). Revealing the tunable photoluminescence properties of graphene quantum dots. J. Mater. Chem. C 2 (34), 6954–6960. doi:10.1039/c4tc01191k
Song, L., Shi, J., Lu, J., and Lu, C. (2015). Structure observation of graphene quantum dots by single-layered formation in layered confinement space. Chem. Sci. 6 (8), 4846–4850. doi:10.1039/c5sc01416f
Song, S., Wu, K., Wu, H., Guo, J., and Zhang, L. (2019). Multi-shelled ZnO decorated with nitrogen and phosphorus co-doped carbon quantum dots: synthesis and enhanced photodegradation activity of methylene blue in aqueous solutions. RSC Adv. 9 (13), 7362–7374. doi:10.1039/c9ra00168a
Song, Y., Wei, W., and Qu, X. (2011). Colorimetric biosensing using smart materials. Adv. Mater. 23 (37), 4215–4236. doi:10.1002/adma.201101853
Song, Y., Zhu, S., Shao, J., and Yang, B. (2017). Polymer carbon dots—a highlight reviewing their unique structure, bright emission and probable photoluminescence mechanism. J. Polym. Sci. Part A Polym. Chem. 55 (4), 610–615. doi:10.1002/pola.28416
Stepanidenko, E. A., Ushakova, E. V., Fedorov, A. V., and Rogach, A. L. (2021). Applications of carbon dots in optoelectronics. Nanomater. (Basel). 11 (2), 364. doi:10.3390/nano11020364
Su, L., Qin, S., Cai, Y., Wang, L., Dong, W., Mao, G., et al. (2022). Co, N-doped carbon dot nanozymes with acid pH-independence and substrate selectivity for biosensing and bioimaging. Sensors Actuators B Chem. 353, 131150. doi:10.1016/j.snb.2021.131150
Su, Y., Xie, Z., and Zheng, M. (2020). Carbon dots with concentration-modulated fluorescence: aggregation-induced multicolor emission. J. colloid interface Sci. 573, 241–249. doi:10.1016/j.jcis.2020.04.004
Sun, D., Liu, T., Li, S., Wang, C., and Zhuo, K. (2020). Preparation and application of carbon dots with tunable luminescence by controlling surface functionalization. Opt. Mater. 108, 110450. doi:10.1016/j.optmat.2020.110450
Sun, H., Zhou, Y., Ren, J., and Qu, X. (2018). Carbon nanozymes: enzymatic properties, catalytic mechanism, and applications. Angew. Chem. Int. Ed. 57 (30), 9224–9237. doi:10.1002/anie.201712469
Sun, S., Sun, Y., Yang, F., Che, S., Zhang, X., Zhang, G., et al. (2023a). Electrochemical synthesis of Ni doped carbon quantum dots for simultaneous fluorometric determination of Fe3+ and Cu2+ ion facilely. Green Chem. Eng. 4 (1), 115–122. doi:10.1016/j.gce.2022.05.004
Sun, X., and Lei, Y. (2017). Fluorescent carbon dots and their sensing applications. TrAC Trends Anal. Chem. 89, 163–180. doi:10.1016/j.trac.2017.02.001
Sun, Y., Xu, B., Pan, X., Wang, H., Wu, Q., Li, S., et al. (2023b). Carbon-based nanozymes: design, catalytic mechanism, and bioapplication. Coord. Chem. Rev. 475, 214896. doi:10.1016/j.ccr.2022.214896
Tang, M., Zhu, B., Wang, Y., Wu, H., Chai, F., Qu, F., et al. (2019). Nitrogen-and sulfur-doped carbon dots as peroxidase mimetics: colorimetric determination of hydrogen peroxide and glutathione, and fluorimetric determination of lead (II). Microchim. Acta 186, 604–614. doi:10.1007/s00604-019-3710-4
Tao, H., Liao, X., Sun, C., Xie, X., Zhong, F., Yi, Z., et al. (2015). A carbon dots-CdTe quantum dots fluorescence resonance energy transfer system for the analysis of ultra-trace chlortoluron in water. Spectrochimica Acta Part A Mol. Biomol. Spectrosc. 136, 1328–1334. doi:10.1016/j.saa.2014.10.020
Tarumi, M., Matsuzaki, Y., and Suzuki, K. (2019). Theoretical study on the redox reaction mechanism of quinone compounds in industrial processes. Chem. Eng. Sci. 199, 381–387. doi:10.1016/j.ces.2019.01.006
Tejwan, N., Saini, A. K., Sharma, A., Singh, T. A., Kumar, N., and Das, J. (2021). Metal-doped and hybrid carbon dots: a comprehensive review on their synthesis and biomedical applications. J. Control. Release 330, 132–150. doi:10.1016/j.jconrel.2020.12.023
Tian, Z., Zhang, X., Li, D., Zhou, D., Jing, P., Shen, D., et al. (2017). Full-color inorganic carbon dot phosphors for white-light-emitting diodes. Adv. Opt. Mater. 5 (19), 1700416. doi:10.1002/adom.201700416
Toral-Sánchez, E., Rangel-Mendez, J. R., Hurt, R. H., Ascacio Valdés, J. A., Aguilar, C. N., and Cervantes, F. J. (2018). Novel application of magnetic nano-carbon composite as redox mediator in the reductive biodegradation of iopromide in anaerobic continuous systems. Appl. Microbiol. Biotechnol. 102, 8951–8961. doi:10.1007/s00253-018-9250-8
Ukaogo, P. O., Ewuzie, U., and Onwuka, C. V. (2020). “Environmental pollution: causes, effects, and the remedies,” in Microorganisms for sustainable environment and health (Elsevier), 419–429.
Varisco, M., Zufferey, D., Ruggi, A., Zhang, Y., Erni, R., and Mamula, O. (2017). Synthesis of hydrophilic and hydrophobic carbon quantum dots from waste of wine fermentation. R. Soc. open Sci. 4 (12), 170900. doi:10.1098/rsos.170900
Venkatesan, S., Mariadoss, A. J., Arunkumar, K., and Muthupandian, A. (2019). Fuel waste to fluorescent carbon dots and its multifarious applications. Sensors Actuators B Chem. 282, 972–983. doi:10.1016/j.snb.2018.11.144
Wang, B., Chen, Y., Wu, Y., Weng, B., and Liu, Y. (2016b). Synthesis of nitrogen-and iron-containing carbon dots, and their application to colorimetric and fluorometric determination of dopamine. Microchim. Acta 183, 2491–2500. doi:10.1007/s00604-016-1885-5
Wang, C., Hu, T., Wen, Z., Zhou, J., Wang, X., Wu, Q., et al. (2018a). Concentration-dependent color tunability of nitrogen-doped carbon dots and their application for iron (III) detection and multicolor bioimaging. J. colloid interface Sci. 521, 33–41. doi:10.1016/j.jcis.2018.03.021
Wang, C., Yang, K., Wei, X., Ding, S., Tian, F., and Li, F. (2018b). One-pot solvothermal synthesis of carbon dots/Ag nanoparticles/TiO2 nanocomposites with enhanced photocatalytic performance. Ceram. Int. 44 (18), 22481–22488. doi:10.1016/j.ceramint.2018.09.017
Wang, H., Cao, G., Gai, Z., Hong, K., Banerjee, P., and Zhou, S. (2015b). Magnetic/NIR-responsive drug carrier, multicolor cell imaging, and enhanced photothermal therapy of gold capped magnetite-fluorescent carbon hybrid nanoparticles. Nanoscale 7 (17), 7885–7895. doi:10.1039/c4nr07335e
Wang, H., Ai, L., Song, Z., Nie, M., Xiao, J., Li, G., et al. (2023). Surface modification functionalized carbon dots. Chemis. Euro. J. 29 (65), e202302383. doi:10.1002/chem.202302383
Wang, H., Shen, J., Li, Y., Wei, Z., Cao, G., Gai, Z., et al. (2013b). Porous carbon protected magnetite and silver hybrid nanoparticles: morphological control, recyclable catalysts, and multicolor cell imaging. ACS Appl. Mater. Interfaces 5 (19), 9446–9453. doi:10.1021/am4032532
Wang, H., Shen, J., Li, Y., Wei, Z., Cao, G., Gai, Z., et al. (2014b). Magnetic iron oxide–fluorescent carbon dots integrated nanoparticles for dual-modal imaging, near-infrared light-responsive drug carrier and photothermal therapy. Biomater. Sci. 2 (6), 915–923. doi:10.1039/c3bm60297d
Wang, H., Zhang, M., Wei, K., Zhao, Y., Nie, H., Ma, Y., et al. (2021a). Pyrrolic nitrogen dominated the carbon dot mimic oxidase activity. Carbon 179, 692–700. doi:10.1016/j.carbon.2021.04.061
Wang, N., Liu, Z. X., Li, R. S., Zhang, H. Z., Huang, C. Z., and Wang, J. (2017). The aggregation induced emission quenching of graphene quantum dots for visualizing the dynamic invasions of cobalt (ii) into living cells. J. Mater. Chem. B 5 (31), 6394–6399. doi:10.1039/c7tb01316g
Wang, Q., Huang, X., Long, Y., Wang, X., Zhang, H., Zhu, R., et al. (2013a). Hollow luminescent carbon dots for drug delivery. Carbon 59, 192–199. doi:10.1016/j.carbon.2013.03.009
Wang, W., Zhu, Y., Zhu, X., Zhao, Y., Xue, Z., Xiong, C., et al. (2021b). Biocompatible ruthenium single-atom catalyst for cascade enzyme-mimicking therapy. ACS Appl. Mater. Interfaces 13 (38), 45269–45278. doi:10.1021/acsami.1c12706
Wang, X., Cheng, Z., Zhou, Y., Tammina, S. K., and Yang, Y. (2020). A double carbon dot system composed of N, Cl-doped carbon dots and N, Cu-doped carbon dots as peroxidase mimics and as fluorescent probes for the determination of hydroquinone by fluorescence. Microchim. Acta 187, 350–358. doi:10.1007/s00604-020-04322-7
Wang, X., Qu, K., Xu, B., Ren, J., and Qu, X. (2011). Multicolor luminescent carbon nanoparticles: synthesis, supramolecular assembly with porphyrin, intrinsic peroxidase-like catalytic activity and applications. Nano Res. 4, 908–920. doi:10.1007/s12274-011-0147-4
Wang, Y., Kalytchuk, S., Zhang, Y., Shi, H., Kershaw, S. V., and Rogach, A. L. (2014a). Thickness-dependent full-color emission tunability in a flexible carbon dot ionogel. J. Phys. Chem. Lett. 5 (8), 1412–1420. doi:10.1021/jz5005335
Wang, Y., Zhang, Y., Jia, M., Meng, H., Li, H., Guan, Y., et al. (2015a). Functionalization of carbonaceous nanodots from MnII-coordinating functional knots. Chem. A Eur. J. 21 (42), 14843–14850. doi:10.1002/chem.201502463
Wei, S.-C., Lin, Y. W., and Chang, H. T. (2020). Carbon dots as artificial peroxidases for analytical applications. J. Food Drug Anal. 28 (4), 559–575. doi:10.38212/2224-6614.1090
Wu, F., Su, H., Zhu, X., Wang, K., Zhang, Z., and Wong, W. K. (2016). Near-infrared emissive lanthanide hybridized carbon quantum dots for bioimaging applications. J. Mater. Chem. B 4 (38), 6366–6372. doi:10.1039/c6tb01646d
Wu, G., Berka, V., Derry, P. J., Mendoza, K., Kakadiaris, E., Roy, T., et al. (2019). Critical comparison of the superoxide dismutase-like activity of carbon antioxidant nanozymes by direct superoxide consumption kinetic measurements. ACS Nano 13 (10), 11203–11213. doi:10.1021/acsnano.9b04229
Wu, W., Huang, L., Wang, E., and Dong, S. (2020). Atomic engineering of single-atom nanozymes for enzyme-like catalysis. Chem. Sci. 11 (36), 9741–9756. doi:10.1039/d0sc03522j
Wu, W., Zhan, L., Fan, W., Song, J., Li, X., Li, Z., et al. (2015). Cu–N dopants boost electron transfer and photooxidation reactions of carbon dots. Angew. Chem. Int. Ed. 54 (22), 6540–6544. doi:10.1002/anie.201501912
Wu, X., Zhang, Y., Han, T., Wu, H., Guo, S., and Zhang, J. (2014). Composite of graphene quantum dots and Fe 3 O 4 nanoparticles: peroxidase activity and application in phenolic compound removal. RSC Adv. 4 (7), 3299–3305. doi:10.1039/c3ra44709j
Xu, Q., Cai, W., Zhang, M., Su, R., Ye, Y., Li, Y., et al. (2018). Photoluminescence mechanism and applications of Zn-doped carbon dots. RSC Adv. 8 (31), 17254–17262. doi:10.1039/c8ra02756k
Xu, Q., Liu, Y., Su, R., Cai, L., Li, B., Zhang, Y., et al. (2016). Highly fluorescent Zn-doped carbon dots as Fenton reaction-based bio-sensors: an integrative experimental–theoretical consideration. Nanoscale 8 (41), 17919–17927. doi:10.1039/c6nr05434j
Xu, S., Zhang, S., Li, Y., and Liu, J. (2023). Facile synthesis of iron and nitrogen co-doped carbon dot nanozyme as highly efficient peroxidase mimics for visualized detection of metabolites. Molecules 28 (16), 6064. doi:10.3390/molecules28166064
Xue, S., Zhang, T., Wang, X., Zhang, Q., Huang, S., Zhang, L., et al. (2021). Cu, Zn dopants boost electron transfer of carbon dots for antioxidation. Small 17 (31), 2102178. doi:10.1002/smll.202102178
Yan, F., Jiang, Y., Sun, X., Bai, Z., Zhang, Y., and Zhou, X. (2018). Surface modification and chemical functionalization of carbon dots: a review. Microchim. Acta 185, 424–434. doi:10.1007/s00604-018-2953-9
Yang, H., Liu, Y., Guo, Z., Lei, B., Zhuang, J., Zhang, X., et al. (2019). Hydrophobic carbon dots with blue dispersed emission and red aggregation-induced emission. Nat. Commun. 10 (1), 1789. doi:10.1038/s41467-019-09830-6
Yang, K., Li, F., Che, W., Hu, X., Liu, C., and Tian, F. (2016). Increment of the FRET efficiency between carbon dots and photosensitizers for enhanced photodynamic therapy. RSC Adv. 6 (103), 101447–101451. doi:10.1039/c6ra20412k
Yang, W., Huang, T., Zhao, M., Luo, F., Weng, W., Wei, Q., et al. (2017). High peroxidase-like activity of iron and nitrogen co-doped carbon dots and its application in immunosorbent assay. Talanta 164, 1–6. doi:10.1016/j.talanta.2016.10.099
Yang, W., Zhang, H., Lai, J., Peng, X., Hu, Y., Gu, W., et al. (2018). Carbon dots with red-shifted photoluminescence by fluorine doping for optical bio-imaging. Carbon 128, 78–85. doi:10.1016/j.carbon.2017.11.069
Yang, Z., Liu, Y., Yue, G., Wang, Y., Rao, H., Zhang, W., et al. (2021). One-pot synthesis of CeO2-carbon dots with enhanced peroxidase-like activity and carbon dots for ratiometric fluorescence detection of H2O2 and cholesterol. J. Alloys Compd. 862, 158323. doi:10.1016/j.jallcom.2020.158323
Yao, Y., Zhang, H., Hu, K., Nie, G., Yang, Y., Wang, Y., et al. (2022). Carbon dots based photocatalysis for environmental applications. J. Environ. Chem. Eng. 10 (2), 107336. doi:10.1016/j.jece.2022.107336
Yuan, K., Zhang, X., Qin, R., Ji, X., Cheng, Y., Li, L., et al. (2018). Surface state modulation of red emitting carbon dots for white light-emitting diodes. J. Mater. Chem. C 6 (46), 12631–12637. doi:10.1039/c8tc04468f
Yuxin, X., Laipeng, S., Kang, L., Haipeng, S., Zonghua, W., and Wenjing, W. (2022). Metal-doped carbon dots as peroxidase mimic for hydrogen peroxide and glucose detection. Anal. Bioanal. Chem. 414 (19), 5857–5867. doi:10.1007/s00216-022-04149-6
Zacharia, J. T. (2011). “Ecological effects of pesticides,” in Pesticides in the modern world-Risks and Benefits Editors M. Stoytcheva (IntechPublisher). Available from: https://www.intechopen.com/chapters/21176 (Accessed April 12, 2025)
Zaheer, T., Zia, S., Pal, K., Aqib, A. I., Fatima, M., Muneer, A., et al. (2024). Recent trends in nobel metals and carbon dots in the costume of hybrid nano architecture. Topics. Cataly. 67 (1), 280–299. doi:10.1007/s11244-023-01869
Zeng, Y., Miao, F., Zhao, Z., Zhu, Y., Liu, T., Chen, R., et al. (2017). Low-cost nanocarbon-based peroxidases from graphite and carbon fibers. Appl. Sci. 7 (9), 924. doi:10.3390/app7090924
Zhang, C., Ying, Z., Jiang, Y., Wang, H., Zhou, X., Xuan, W., et al. (2024). Solvent-controlled synthesis of hydrophilic and hydrophobic carbon dots. Phys. Chem. Chem. Phys. 26 (1), 314–322. doi:10.1039/d3cp04273a
Zhang, H.-Y., Wang, Y., Xiao, S., Wang, H., Wang, J. H., and Feng, L. (2017a). Rapid detection of Cr (VI) ions based on cobalt (II)-doped carbon dots. Biosens. Bioelectron. 87, 46–52. doi:10.1016/j.bios.2016.08.010
Zhang, L., Hai, X., Xia, C., Chen, X. W., and Wang, J. H. (2017d). Growth of CuO nanoneedles on graphene quantum dots as peroxidase mimics for sensitive colorimetric detection of hydrogen peroxide and glucose. Sensors Actuators B Chem. 248, 374–384. doi:10.1016/j.snb.2017.04.011
Zhang, M., Wang, W., Yuan, P., Chi, C., Zhang, J., and Zhou, N. (2017b). Synthesis of lanthanum doped carbon dots for detection of mercury ion, multi-color imaging of cells and tissue, and bacteriostasis. Chem. Eng. J. 330, 1137–1147. doi:10.1016/j.cej.2017.07.166
Zhang, M., Wang, W., Zhou, N., Yuan, P., Su, Y., Shao, M., et al. (2017c). Near-infrared light triggered photo-therapy, in combination with chemotherapy using magnetofluorescent carbon quantum dots for effective cancer treating. Carbon N. Y. 118, 752–764. doi:10.1016/j.carbon.2017.03.085
Zhang, Q., Wang, R., Feng, B., Zhong, X., and Ostrikov, K. (2021). Photoluminescence mechanism of carbon dots: triggering high-color-purity red fluorescence emission through edge amino protonation. Nat. Commun. 12 (1), 6856. doi:10.1038/s41467-021-27071-4
Zhang, S., Jin, L., Liu, J., Wang, Q., and Jiao, L. (2020b). A label-free yellow-emissive carbon dot-based nanosensor for sensitive and selective ratiometric detection of chromium (VI) in environmental water samples. Mater. Chem. Phys. 248, 122912. doi:10.1016/j.matchemphys.2020.122912
Zhang, S., Wang, H., Li, Y., Data, F. Y., Wang, Q., and Jiao, L. (2020a). Bright-yellow-emissive carbon dots with a large Stokes shift for selective fluorescent detection of 2, 4, 6-trinitrophenol in environmental water samples. Mater. Lett. 263, 127208. doi:10.1016/j.matlet.2019.127208
Zhang, X., Liao, X., Hou, Y., Jia, B., Fu, L., Jia, M., et al. (2022). Recent advances in synthesis and modification of carbon dots for optical sensing of pesticides. J. Hazard. Mater. 422, 126881. doi:10.1016/j.jhazmat.2021.126881
Zhang, Y., Gao, W., Ma, Y., Cheng, L., Zhang, L., Liu, Q., et al. (2023). Integrating Pt nanoparticles with carbon nanodots to achieve robust cascade superoxide dismutase-catalase nanozyme for antioxidant therapy. Nano Today 49, 101768. doi:10.1016/j.nantod.2023.101768
Zhang, Y., Xiao, J., Zhuo, P., Yin, H., Fan, Y., Liu, X., et al. (2019). Carbon dots exhibiting concentration-dependent full-visible-spectrum emission for light-emitting diode applications. ACS Appl. Mater. and interfaces 11 (49), 46054–46061. doi:10.1021/acsami.9b14472
Zhao, D., Zhang, C., Liu, H., Jiao, Y., and Xiao, X. (2024). Gadolinium-doped carbon dots with dual enzyme activity for visual detection of Cu2+ and antibacterial applications. ACS Appl. Nano Mater. 7, 4228–4238. doi:10.1021/acsanm.3c05763
Zhao, L., Ren, X., Zhang, J., Zhang, W., Chen, X., and Meng, X. (2020b). Dendritic silica with carbon dots and gold nanoclusters for dual nanozymes. New J. Chem. 44 (5), 1988–1992. doi:10.1039/c9nj05655f
Zhao, N., Song, J., and Zhao, L. (2022). Metallic deep eutectic solvents-assisted synthesis of Cu, Cl-doped carbon dots as oxidase-like and peroxidase-like nanozyme for colorimetric assay of hydroquinone and H2O2. Colloids Surfaces A Physicochem. Eng. Aspects 648, 129390. doi:10.1016/j.colsurfa.2022.129390
Zhao, P., and Zhu, L. (2018). Dispersibility of carbon dots in aqueous and/or organic solvents. Chem. Commun. 54 (43), 5401–5406. doi:10.1039/c8cc02279h
Zhao, Q., Song, W., Zhao, B., and Yang, B. (2020a). Spectroscopic studies of the optical properties of carbon dots: recent advances and future prospects. Mater. Chem. Front. 4 (2), 472–488. doi:10.1039/c9qm00592g
Zheng, A., Guo, T., Guan, F., Chen, X., Shu, Y., and Wang, J. (2019). Ionic liquid mediated carbon dots: preparations, properties and applications. TrAC Trends Anal. Chem. 119, 115638. doi:10.1016/j.trac.2019.115638
Zheng, C., Ke, W., Yin, T., and An, X. (2016). Intrinsic peroxidase-like activity and the catalytic mechanism of gold@ carbon dots nanocomposites. RSC Adv. 6 (42), 35280–35286. doi:10.1039/c6ra01917j
Zheng, M., Wang, C., Wang, Y., Wei, W., Ma, S., Sun, X., et al. (2018). Green synthesis of carbon dots functionalized silver nanoparticles for the colorimetric detection of phoxim. Talanta 185, 309–315. doi:10.1016/j.talanta.2018.03.066
Zhou, X.-L., Zhang, H., Shao, L. M., Lü, F., and He, P. J. (2021). Preparation and application of hierarchical porous carbon materials from waste and biomass: a review. Waste Biomass Valorization 12, 1699–1724. doi:10.1007/s12649-020-01109-y
Zhou, Y., Zahran, E. M., Quiroga, B. A., Perez, J., Mintz, K. J., Peng, Z., et al. (2019). Size-dependent photocatalytic activity of carbon dots with surface-state determined photoluminescence. Appl. Catal. B Environ. 248, 157–166. doi:10.1016/j.apcatb.2019.02.019
Zhu, D., Zhuo, S., Zhu, C., Zhang, P., and Shen, W. (2019b). Synthesis of catalytically active peroxidase-like Fe-doped carbon dots and application in ratiometric fluorescence detection of hydrogen peroxide and glucose. Anal. methods 11 (20), 2663–2668. doi:10.1039/c9ay00342h
Zhu, S., Zhang, J., Qiao, C., Tang, S., Li, Y., Yuan, W., et al. (2011). Strongly green-photoluminescent graphene quantum dots for bioimaging applications. Chem. Commun. 47 (24), 6858–6860. doi:10.1039/c1cc11122a
Zhu, S., Zhao, X. E., You, J., Xu, G., and Wang, H. (2015). Carboxylic-group-functionalized single-walled carbon nanohorns as peroxidase mimetics and their application to glucose detection. Analyst 140 (18), 6398–6403. doi:10.1039/c5an01104c
Zhu, W., Zhang, J., Jiang, Z., Wang, W., and Liu, X. (2014). High-quality carbon dots: synthesis, peroxidase-like activity and their application in the detection of H 2 O 2, Ag+ and Fe 3+. RSC Adv. 4 (33), 17387–17392. doi:10.1039/c3ra47593j
Zhu, X.-D., Wang, Y. J., Sun, R. J., and Zhou, D. M. (2013). Photocatalytic degradation of tetracycline in aqueous solution by nanosized TiO2. Chemosphere 92 (8), 925–932. doi:10.1016/j.chemosphere.2013.02.066
Zhu, Z., Ge, K., Li, Z., Hu, J., Chen, P., and Bi, H. (2023). Nickel-doped carbon dots as an efficient and stable electrocatalyst for urea oxidation. Small 19 (31), 2205234. doi:10.1002/smll.202205234
Zhu, Z., Zhai, Y., Li, Z., Zhu, P., Mao, S., Zhu, C., et al. (2019a). Red carbon dots: optical property regulations and applications. Mater. Today 30, 52–79. doi:10.1016/j.mattod.2019.05.003
Zhuo, S., Fang, J., Li, M., Wang, J., Zhu, C., and Du, J. (2019). Manganese (II)-doped carbon dots as effective oxidase mimics for sensitive colorimetric determination of ascorbic acid. Microchim. Acta 186, 745–748. doi:10.1007/s00604-019-3887-6
Keywords: carbon dots, robust materials, functional attributes, efficient catalysis, enzyme mimics, remediation
Citation: Yousaf A, Imran M, Farooq Warsi M, Alsafari IA, Khan FA, Parra-Saldívar R, Gutiérrez-Soto G and Iqbal HMN (2025) Nanomaterials as a new frontier platform: metal-doped and hybrid carbon dots as enzyme mimics for environmental applications. Front. Mater. 12:1553214. doi: 10.3389/fmats.2025.1553214
Received: 30 December 2024; Accepted: 31 March 2025;
Published: 25 April 2025.
Edited by:
Nicola Maria Pugno, University of Trento, ItalyReviewed by:
Madhuri Sharon, Walchand Centre for Research in Naynotechnology and Bionanotechnolog, IndiaMarimuthu Karunakaran, Alagappa Government Arts College, India
Copyright © 2025 Yousaf, Imran, Farooq Warsi, Alsafari, Khan, Parra-Saldívar, Gutiérrez-Soto and Iqbal. This is an open-access article distributed under the terms of the Creative Commons Attribution License (CC BY). The use, distribution or reproduction in other forums is permitted, provided the original author(s) and the copyright owner(s) are credited and that the original publication in this journal is cited, in accordance with accepted academic practice. No use, distribution or reproduction is permitted which does not comply with these terms.
*Correspondence: Muhammad Imran, bXVoYW1tYWQuaW1yYW5AaXViLmVkdS5waw==; Hafiz M. N. Iqbal, aGFmaXouaXFiYWx4QHVhbmwuZWR1Lm14