- 1China State Shipbuilding Corporation (CSSC) Systems Engineering Research Institute, Beijing, China
- 2School of Mechanics, Civil Engineering and Architecture, Northwestern Polytechnical University, Xi’an, China
- 3QianYuan National Laboratory, Hangzhou, China
Marine environments pose significant challenges to the reliability of electronic packaging materials. This review summarizes the main degradation mechanisms and reliability impacts of electronic packaging materials under marine conditions, including salt spray corrosion, high humidity, thermal cycling, and mechanical shock. Salt spray corrosion initiates localized galvanic corrosion through chloride ion (Cl−) diffusion, creating corrosion pits and stress concentration, thereby accelerating electrochemical-mechanical coupled failures. High humidity promotes moisture ingress into polymer packaging materials, resulting in hygroscopic plasticization, weakened interfacial adhesion, and delamination failure. Thermal cycling, due to mismatched coefficients of thermal expansion (CTE), induces growth of interfacial intermetallic compound (IMC) layers at solder joints and creep-fatigue interactions, further promoting interfacial crack propagation. Mechanical shock generates transient, high-strain-rate loading, rapidly initiating and propagating cracks within brittle packaging structures, ultimately leading to structural failure. Additionally, this paper discusses the current status and limitations of Physics of Failure (PoF)-based reliability models such as the Coffin-Manson and Arrhenius models for evaluating electronic packaging reliability in marine environments. Finally, it suggests that future studies should further develop multiphysics coupling models to more accurately predict long-term material performance under extreme marine conditions.
1 Introduction
Marine environments pose severe challenges to the reliability of electronic packaging materials. Electronic systems employed in ships, ocean exploration, offshore communication, and underwater equipment frequently encounter extreme environmental factors, such as high salinity, high humidity, large temperature variations, and complex mechanical loading (Yi et al., 2015b; Jia, 2021). Generally, the internal environment of a ship remains relatively stable but not entirely uniform. During navigation, the internal temperature and humidity conditions vary depending on ship type, location, external climate, and operational status. For instance, large cruise ships typically maintain comfortable temperatures and humidity levels within passenger cabins and main public areas to ensure comfort for passengers and crew. However, conditions can differ significantly in electronics-rich areas near the exterior or specialized compartments, such as engine rooms, control rooms, and cargo holds. These regions experience greater humidity and pronounced temperature fluctuations, placing electronic equipment under higher stress and risks. In contrast, electronic equipment on military and cargo vessels commonly faces extreme humidity levels of 85%–98% and temperature fluctuations from −40°C to 85°C. These harsh conditions represent critical challenges to the reliability of electronic packaging materials. Therefore, it is especially crucial to investigate the performance of electronic packaging materials used in these specific shipboard areas under extreme marine environmental conditions (Wang et al., 2022). Extreme environmental factors not only cause the degradation of material performance but also induce various failure modes (Comizzoli et al., 1986; Jia et al., 2020), such as metallic corrosion, solder joint fatigue, polymer insulation deterioration, and structural brittle fracture, severely affecting the stability and lifespan of electronic equipment (Zhu et al., 2008; Istrate and Mureșan, 2021). Thus, a thorough understanding of failure mechanisms of electronic materials in marine environments, and the development of reliability evaluation methodologies based on the Physics of Failure (PoF), is crucial to enhancing the long-term serviceability of electronic systems (Li et al., 2019; He et al., 2024). Specifically, salt spray corrosion is a major factor affecting the reliability of metal interconnections and solder joints in marine environments. The highly corrosive chloride ions (Cl−) cause electrochemical corrosion in metal conductors, leading to bridging by corrosion products, short circuits, and deterioration of electrical performance. Moreover, high humidity environments accelerate moisture absorption by polymeric materials, reducing interfacial adhesion strength and exacerbating mismatches in the coefficients of thermal expansion (CTE), which promote delamination of packaging layers (Abdel-Samad et al., 2014; Deflorian et al., 2015). Under thermal cycling conditions, periodic thermal stresses arise due to CTE mismatch, inducing creep-fatigue interactions and promoting fatigue failure in solder joints. Mechanical shock generates transient, high-strain-rate loads on brittle materials and soldered structures, causing structural damage. Although numerous studies have proposed mitigation strategies for these failure mechanisms, including protective coatings, self-healing materials, and metal coatings, their durability and interfacial stability remain challenging under the combined action of salt spray, thermal cycling, and mechanical shock. Therefore, investigating the effects of marine environments on electronic packaging reliability is essential for understanding degradation mechanisms (Ding et al., 2019; Rong et al., 2021). As systematically illustrated in Figure 1, typical degradation and damage characteristics of electronic packaging materials caused by high salinity, humidity, temperature variations, and mechanical loads in marine environments are summarized.
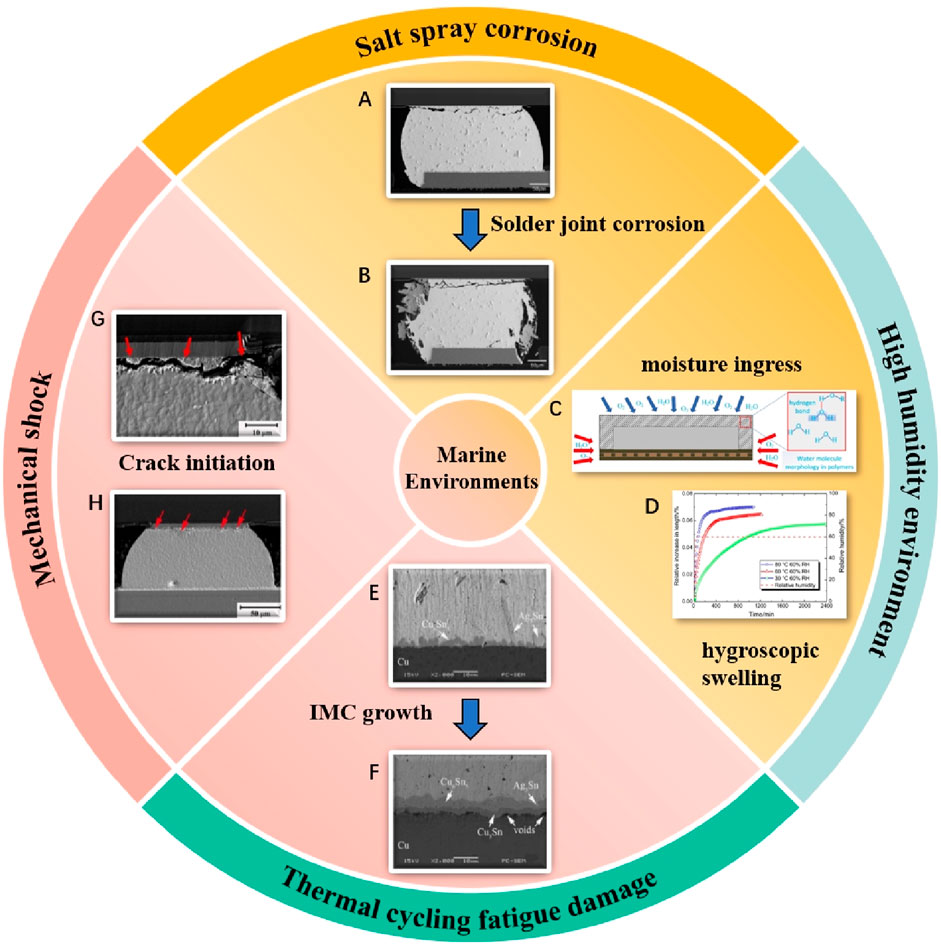
Figure 1. Typical degradation and damage characteristics of electronic packaging materials induced by salt spray corrosion, high humidity, thermal cycling, and mechanical shock in marine environments. (A) Cross-sectional SEM image of SAC305 solder joint after thermal cycling without salt spray pretreatment; (B) Severe corrosion of SAC305 solder joint after thermal cycling with 5% NaCl salt spray pretreatment. [Adapted from Ref (Liu et al., 2011)]; (C) Moisture ingress into packaging materials, exhibiting three states of water molecules: chemically bound water, physically adsorbed water, and free water. [Adapted from Ref (Tian et al., 2024)]; (D) Hygroscopic swelling behavior of packaging materials under different temperature and humidity conditions. [Adapted from Ref (He and Kabiri, 2022)]; (E, F) Growth of intermetallic compound (IMC) layers at solder joint interfaces and the formation of interfacial voids after aging at 150°C. [Adapted from Ref (Liu et al., 2014)]; (G, H) Crack initiation and propagation along IMC interfaces in SAC305 solder joints subjected to mechanical vibration loading, showing typical brittle interfacial fracture features. [Adapted from Ref (Pongvittayapanu et al., 2022)].
In this review, we focus on four primary failure mechanisms impacting electronic packaging reliability in marine environments: electrochemical-mechanical coupling failures in salt spray corrosion, hygroscopic plasticization and interfacial failures under high humidity, thermomechanical fatigue damage induced by thermal cycling, and brittle fracture caused by mechanical shocks. Finally, reliability assessment approaches based on PoF models are summarized to provide theoretical support and engineering guidance for long-term stability of electronic materials used in marine environments (Neville, 1995).
2 Influence of various factors on shipboard electronic packaging materials in marine environments
2.1 Electrochemical-mechanical coupling failures induced by salt spray corrosion
The core mechanism of salt spray corrosion in marine environments involves the diffusion dynamics of chloride ions (Cl−) within thin liquid films, which determine both the corrosion rate and morphological evolution of materials (Yi et al., 2015a). Specifically, Cl− ions diffuse through the thin liquid film to vulnerable or defective sites on metallic surfaces, resulting in localized areas of high chloride concentration and accelerating localized pitting or galvanic corrosion. The formation of localized corrosion pits or cavities subsequently reduces the cross-sectional area of the material, causing local stress concentration and significant degradation in mechanical properties. Stress concentration induced by localized corrosion further promotes pit growth and crack initiation, resulting in a coupling effect between electrochemical corrosion and mechanical damage, ultimately leading to comprehensive material failure (Guedon-Gracia et al., 2016; Yan et al., 2016). Galvanic corrosion of Al-Cu alloys and Sn-Pb solder systems primarily manifests as accelerated anodic dissolution, whereas magnesium alloys exhibit prominent pitting corrosion behaviors influenced by alloying elements and coating structures (Grassini et al., 2011; Xiao et al., 2020). Such corrosion not only leads to yield strength degradation in Cu conductors (Zhang et al., 2014), but also causes internal delamination in magnesium alloys (Shi et al., 2013) and reduces the volume resistivity of epoxy resins, triggering insulation failure in printed circuit boards (PCBs) (Liu et al., 2022). In electronic systems, electrochemical-mechanical coupling effects often lead to multi-stage failures, including circuit shorting due to bridging by metal corrosion products (Xu et al., 2011), and corrosion channels in Ni-P coatings arising from conflicting thickness and porosity requirements (García-Rodríguez et al., 2019), all of which significantly affect the reliability of electronic packaging materials (Qin et al., 2024). Therefore, a deeper understanding of electrochemical-mechanical coupling failure mechanisms associated with salt spray corrosion in marine environments is crucial for accurately predicting the long-term performance degradation of electronic packaging materials and effectively mitigating risks to material reliability.
2.2 Hygroscopic plasticization and interfacial failure under high humidity
Under high-humidity conditions, the impact of moisture diffusion on material reliability is not confined merely to the diffusion behavior within the material but extends critically to subsequent changes in material properties and interfacial failure mechanisms. Specifically, after moisture diffuses into polymeric materials, water molecules infiltrate into the molecular chain gaps and interact via hydrogen bonds with polymer chains. This interaction increases the mobility of polymer chain segments, causing a hygroscopic plasticization effect that significantly reduces the elastic modulus and yield strength of the polymer. The plasticization effect further reduces interfacial adhesion strength, weakening the interface’s ability to resist stress (Wang et al., 2020; Zheng et al., 2022). Concurrently, moisture ingress at the interface decreases the interfacial surface free energy, impairing interfacial adhesion, and eventually causing interface delamination and layer separation (Jiang et al., 2008; Zhendong et al., 2008).
In non-hermetic environments, moisture diffusion into polymeric materials can be modeled by Fick’s law, and its rate is significantly accelerated by increasing humidity and temperature. For example, moisture diffusion rates can increase by up to threefold when the ambient relative humidity exceeds 80% (Mei and Yao, 2011). Materials with higher moisture absorption capability become particularly susceptible to moisture interference in humid environments, undergoing substantial changes in material properties. Furthermore, the interactions between moisture molecules and polymeric chains alter internal stress equilibrium, introducing certain transient and complicated effects. Extensive experimental evidence has demonstrated that moisture absorption significantly weakens the internal stress structure of epoxy resins, resulting in pronounced plasticization effects and reducing their elastic modulus by over 20% (Su et al., 2006; Nguyen et al., 2017). Moisture-induced hygroscopic expansion in printed circuit board (PCB) substrates results in mismatched coefficients of thermal expansion (CTE), increasing solder joint delamination rates under thermal cycling conditions. Experimental results indicate that delamination areas exceeding 5% in ball grid array (BGA) packages represent a failure criterion (Fan et al., 2008). It is important to note that moisture diffusion itself is not the direct cause of material failure; rather, the associated polymer plasticization and reduction in interfacial adhesion strength fundamentally drive plasticization and interfacial failures. Hygroscopic plasticization reduces structural strength, while CTE mismatch concentrates stress at interfaces, thereby accelerating delamination propagation under combined heat and humidity conditions (Tong and Ng, 2002; Shi et al., 2008).
Therefore, high humidity conditions—by inducing hygroscopic plasticization and weakening interfacial adhesion—significantly degrade the mechanical integrity and interfacial stability of electronic packaging materials, accelerating their damage progression and ultimately severely compromising their long-term reliability in marine environments.
2.3 Damage degradation induced by thermal cycling
In electronic packaging, thermo-mechanical coupling effects primarily arise from mismatches in the coefficient of thermal expansion (CTE) among constituent materials. Particularly at metal-ceramic interfaces and solder joints, these CTE mismatches cause accumulation of thermal stresses at interfaces, progressively evolving into fatigue damage during thermal cycling (Xiao et al., 2013). For SnAgCu solder joints, thermal cycling induces creep deformation at elevated temperatures, while at lower temperatures, brittle cracks tend to initiate, reflecting a creep-fatigue interaction (Nousiainen et al., 2007). Experimental studies indicate that, under cyclic conditions from −40°C to 125°C, the interfacial intermetallic compound (IMC) layer, primarily Cu6Sn5, progressively thickens at the solder joint interfaces, causing localized stress concentrations that subsequently reduce fatigue life (Teo, 2007). Research has demonstrated that, under typical marine environmental thermal cycling conditions (−40°C–125°C at approximately 12 cycles/hour), IMC layers initially grow rapidly, then gradually stabilize, following a characteristic parabolic growth pattern involving a rapid initial growth phase, a stable growth phase, and eventually a coarsening phase. With increasing numbers of thermal cycles, the interfacial IMC layers transition from dense structures to porous and coarsened morphologies, accompanied by void formation and micro-crack initiation. These changes significantly degrade the mechanical performance of the solder joint interface and dramatically increase the probability of interfacial failure beyond a critical number of cycles (Xu et al., 2021; Zhou et al., 2022). Furthermore, the recrystallization of solder joints is identified as a key mechanism for crack propagation (Schmitz et al., 2014). Under thermal cycling, significant degradation in mechanical properties and reliability of packaging materials occurs. For instance, aluminum alloy heat sinks experience a reduction in yield strength with increasing cycles, while plastic encapsulation materials undergo glass-transition processes at elevated temperatures, becoming more brittle at lower temperatures (Khatibi et al., 2018). In solder joint failures, cracks commonly propagate along Cu6Sn5/SnAgCu interfaces in ball grid array (BGA) packages, with failure rates significantly elevated under high cyclic loading conditions (Chen et al., 2013). Additionally, substrate warpage may trigger component debonding, particularly in packaging structures with substantial CTE mismatches (Xie et al., 2009). Thus, fatigue damage driven by thermo-mechanical coupling effects under thermal cycling conditions significantly accelerates performance degradation and interfacial failure risks of electronic packaging materials, critically affecting their long-term reliability and service life in marine environments.
2.4 Dynamic loading and brittle fracture caused by mechanical shock
In marine environments, dynamic loading induced by mechanical shock typically manifests as transient shock waves characterized by high strain rates. When shock waves impact electronic packaging structures, initial transmission and reflection occur at the outer structural interfaces. The superposition of reflected and incident waves significantly amplifies localized stresses within the packaging (Gharaibeh, 2022). Specifically, as shock waves propagate through multilayered structures such as chips, solder joints, substrates, and encapsulants, partial reflections and transmissions arise due to differences in mechanical impedance between distinct materials. The resulting wave interference markedly increases transient stress amplitudes, particularly at structurally vulnerable regions including chip-solder interfaces, package corners, and pin roots, where stresses can be several times higher than elsewhere. Consequently, this effect rapidly initiates and propagates micro-cracks within solder interfaces or brittle materials, ultimately culminating in evident brittle failure (Liu et al., 2021; Wu and Chang, 2021).
Experimental studies (Zhou et al., 2023) indicate that stress concentrations caused by propagating shock waves, especially at metal-ceramic or metal-polymer interfaces, readily induce localized failures, resulting in solder joint detachment or chip-substrate interface cracking. Numerical simulation results (Jiang et al., 2024) further reveal that increasing shock loads accelerate interfacial micro-crack propagation, causing fatigue-induced solder joint fractures and compromising overall system reliability. In practical marine applications, such rapid failures, particularly under frequent shock loading, may lead to immediate or cumulative equipment failure, severely impacting the operational stability of marine electronic systems. Moreover, material responses to shock loading vary significantly, with brittle materials showing pronounced reductions in strength and toughness under high-strain-rate conditions (Zheng et al., 2017). Studies demonstrate rapid initiation and propagation of internal micro-cracks in metallic materials (e.g., SAC305 solder balls), culminating in brittle fracture under shock conditions (Jenq et al., 2009; Long et al., 2020). Dynamic responses of materials encompass critical mechanisms such as crack initiation sites, propagation paths, and fracture modes (Gross and Seelig, 2011). Failure mechanisms in specific structural components also involve plastic deformation of connector pins, predominantly influenced by gold-plating thickness; insufficient plating thickness can result in permanent bending of copper substrates under shock, causing electrical connection failure (Ling et al., 2019). Furthermore, stress concentrations at package corners render chip encapsulation particularly susceptible to fracture during mechanical shock events (Lu et al., 2012). Consequently, the stress concentration effects induced by mechanical shocks significantly accelerate crack initiation and propagation within electronic packaging interfaces, leading directly to brittle structural failure. This damage mechanism severely compromises the long-term reliability and operational stability of electronic packaging materials in marine environments (Gao and Oterkus, 2018; Hu et al., 2022).
3 Reliability impacts on shipboard electronic packaging materials in marine environments
In marine environments, the reliability of electronic packaging materials is simultaneously affected by multiple factors, including salt-spray corrosion, high humidity, thermal cycling, and mechanical shock. To quantitatively assess these impacts, Physics-of-Failure (PoF) based approaches are employed to analyze material reliability under various environmental stresses. The following section introduces physical models tailored to different failure mechanisms and discusses their applicability within marine conditions.
High humidity and salt spray in marine environments accelerate the degradation of electronic devices, adversely affecting gate oxide layers, metal interconnects, solder joints, and packaging materials. High humidity alters the charge distribution within oxide layers, accelerating Time-Dependent Dielectric Breakdown (TDDB), while ionic contamination from salt spray decreases dielectric insulation, prompting premature failures. Furthermore, temperature fluctuations exacerbate Hot Carrier Injection (HCI), intensifying device deterioration. Metal interconnects and solder joints are particularly susceptible to electromigration (EM) and corrosion; salt-spray corrosion reduces metallic stability, and elevated humidity accelerates ionic migration, aggravating electromigration-induced failures that eventually lead to interconnect fractures. Thermal fatigue failures in solder joints and packaging materials can be modeled using Coffin-Manson, Norris-Landzberg, and Arrhenius models, as high humidity combined with temperature cycling intensifies expansion mismatches and elevates failure risks. Additionally, mechanical shocks induce crack propagation, often analyzed by the Paris Law model, while salt spray exacerbates interfacial cracking, and humid conditions soften packaging materials, reducing their mechanical strength and accelerating failure. Table 1 summarizes these failure-physics models widely used for reliability assessment and accelerated test design to predict the service life of electronic packaging devices in marine environments.
To enhance reliability under marine conditions, significant progress has been achieved through material modifications targeting extreme humidity, salinity, and temperature fluctuations. For instance, polymers with low moisture absorption, such as modified epoxy resins, have demonstrated significantly reduced hygroscopic plasticization in experiments, thus improving the stability and long-term reliability of electronic packaging materials (Bone et al., 2022; He and Kabiri, 2022). Additionally, novel corrosion-resistant metal alloys have increasingly been utilized in marine electronic systems due to their enhanced resistance to galvanic corrosion, effectively reducing corrosion-related failures in metallic components (Adesina et al., 2021). Beyond these material modifications, researchers have explored various advanced coatings, including nano-coatings and self-healing coatings, aimed at improving corrosion resistance in packaging materials and extending their service lifetimes. Surface modifications enabled by nanotechnology have provided superior protection by effectively blocking moisture and ionic ingress, slowing corrosion processes (Son et al., 2023). Moreover, modified ceramic materials have demonstrated exceptional stability under high-temperature and corrosive conditions, particularly suitable for marine applications demanding extremely high reliability (Yang et al., 2022).
It is noteworthy that currently there are no standards or guidelines specifically dedicated to electronic packaging materials used in marine environments. Although some existing standards, such as ISO 9227 and ASTM B117, provide cyclic salt-spray test methods capable of simulating marine salt-spray corrosion, they lack specific regulations addressing the reliability of electronic equipment operating under marine conditions. Standards like IPC-SM-785 provide accelerated reliability testing procedures for PCBs and related electronic interconnect products, and JEP122H addresses failure mechanisms and modeling for semiconductor devices under accelerated testing conditions. However, while these standards effectively simulate extreme temperature and humidity conditions, they do not directly address the unique demands posed by marine environments. The specificity of marine conditions—including high salinity, elevated humidity, temperature fluctuations, and mechanical shock—necessitates the establishment of specialized standards and guidelines tailored explicitly for electronic packaging materials in marine applications. Consequently, focused research on reliability assessment methods and testing procedures for electronic packaging materials under marine conditions is critically important for filling this standards gap.
In conclusion, factors such as high humidity, salt-spray corrosion, temperature cycling, and mechanical shock significantly affect the reliability of electronic packaging materials in marine environments. The application of PoF models enables quantitative evaluation of these environmental stresses, facilitating accurate predictions of device service lifetimes in marine conditions. To address these reliability challenges, researchers have notably enhanced material stability and durability through modifications such as low moisture-absorption polymers, corrosion-resistant metal alloys, and advanced coating materials. However, current standards and guidelines predominantly focus on terrestrial conditions, leaving a critical need for the development of specialized marine-environment standards and reliability assessment methods. Thus, establishing such standards will significantly contribute to improving the long-term stability and reliability of electronic systems deployed in marine environments.
4 Conclusion
This paper systematically reviews the primary failure mechanisms and reliability implications of extreme environmental factors—such as salt-spray corrosion, high humidity, thermal cycling, and mechanical shock—on electronic packaging materials in marine environments. Research indicates that salt-spray corrosion initiates localized galvanic corrosion through Cl− ion penetration, creating internal corrosion pits and stress concentrations that trigger pronounced electrochemical-mechanical coupling failures. High humidity induces moisture-induced plasticization within packaging materials, significantly weakening interface strength and causing interfacial delamination failures. Under thermal cycling, mismatches in coefficients of thermal expansion (CTE) accelerate interfacial IMC layer growth and induce creep-fatigue damage in solder joints, deteriorating interface reliability. Mechanical shock loading notably exacerbates brittle interface crack initiation and rapid propagation, leading to structural failure. Although current Physics-of-Failure (PoF)-based reliability models can partly describe and predict the degradation behavior under single environmental factors, the complexity arising from multi-factor coupling in marine environments limits their comprehensive accuracy for evaluating long-term material service performance. Therefore, it is critically necessary to establish and develop more precise multi-physics coupled models that integrate material degradation behaviors and damage mechanisms, as well as specialized reliability standards and assessment methodologies tailored specifically to marine environments, to improve the long-term stability and reliability of electronic packaging materials in extreme marine conditions.
Author contributions
FL: Writing – review and editing. ZeZ: Investigation, Writing – original draft, Writing – review and editing. JL: Methodology, Supervision, Writing – review and editing. MC: Methodology, Writing – review and editing. ZoZ: Conceptualization, Supervision, Writing – review and editing.
Funding
The author(s) declare that financial support was received for the research and/or publication of this article. This work was supported by the Qin Chuang Yuan high-level innovation and entrepreneurship talent project (No. QCYRCXM-2022-306), and the Natural Science Foundation of Chongqing (No. CSTB2022NSCQ-MSX0574).
Conflict of interest
The authors declare that the research was conducted in the absence of any commercial or financial relationships that could be construed as a potential conflict of interest.
Generative AI statement
The authors declare that no Generative AI was used in the creation of this manuscript.
Publisher’s note
All claims expressed in this article are solely those of the authors and do not necessarily represent those of their affiliated organizations, or those of the publisher, the editors and the reviewers. Any product that may be evaluated in this article, or claim that may be made by its manufacturer, is not guaranteed or endorsed by the publisher.
References
Abdel-Samad, A., Soud, Y., and Zaki, M. (2014). Influence of paint on steel corrosion for marine applications. JSEMAT 04 04, 189–195. doi:10.4236/jsemat.2014.44022
Adesina, O. S., Oki, M., Farotade, G. A., Adesina, O. T., Ogunbiyi, O. F., and Adeleke, A. A. (2021). Effect of nickel-based laser coatings on phase composition and corrosion behaviour of titanium alloy for offshore application. Mater. Today Proc. 38, 830–834. doi:10.1016/j.matpr.2020.04.671
Anolick, E. S., and Nelson, G. R. (2009). Low-field time-dependent dielectric integrity. IEEE Trans. Reliab. 29, 217–221. doi:10.1109/tr.1980.5220804
Black, J. R. (1969). Electromigration failure modes in aluminum metallization for semiconductor devices. Proc. IEEE 57, 1587–1594. doi:10.1109/proc.1969.7340
Bone, J. E., Sims, G. D., Maxwell, A. S., Frenz, S., Ogin, S. L., Foreman, C., et al. (2022). On the relationship between moisture uptake and mechanical property changes in a carbon fibre/epoxy composite. J. Compos. Mater. 56, 2189–2199. doi:10.1177/00219983221091465
Chang, W., Luo, J., Qi, Y., and Wang, B. (2010). “Reliability and failure analysis in designing a typical operation amplifier,” in 2010 17th IEEE international symposium on the physical and failure analysis of integrated circuits (IEEE), 1–4.
Chen, J., Lv, W., An, B., Zhou, L., and Wu, Y. (2013). “Influence of rapid thermal cycling on the microstructures of single SnAgCu and SnPb solder joints,” in 2013 14th international conference on electronic packaging Technology (Dalian, China: IEEE), 848–852. doi:10.1109/ICEPT.2013.6756596
Comizzoli, R. B., Frankenthal, R. P., Milner, P. C., and Sinclair, J. D. (1986). Corrosion of electronic materials and devices. Science 234, 340–345. doi:10.1126/science.234.4774.340
Crook, D. L. (1979). “Method of determining reliability screens for time dependent dielectric breakdown,” in 17th international reliability physics symposium, (IEEE), 1–7.
Darveaux, R. (2002). Effect of simulation methodology on solder joint crack growth correlation and fatigue life prediction. J. Electron. Packag. 124, 147–154. doi:10.1115/1.1413764
Deflorian, F., Rossi, S., Fedel, M., Zanella, C., Ambrosi, D., and Hlede, E. (2015). Crevice corrosion study of materials for propulsion applications in the marine environment. Corros. Sci. Technol. 14, 288–295. doi:10.14773/CST.2015.14.6.288
De Orio, R. L., Ceric, H., and Selberherr, S. (2010). Physically based models of electromigration: from Black’s equation to modern TCAD models. Microelectron. Reliab. 50, 775–789. doi:10.1016/j.microrel.2010.01.007
Ding, R., Chen, S., Lv, J., Zhang, W., Zhao, X., Liu, J., et al. (2019). Study on graphene modified organic anti-corrosion coatings: a comprehensive review. J. Alloys Compd. 806, 611–635. doi:10.1016/j.jallcom.2019.07.256
Fan, X., Zhou, J., and Chandra, A. (2008). “Package structural integrity analysis considering moisture,” in 2008 58th electronic Components and Technology conference, (lake buena vista, FL, USA: ieee), 1054–1066. doi:10.1109/ECTC.2008.4550106
Gao, Y., and Oterkus, S. (2018). Peridynamic analysis of marine composites under shock loads by considering thermomechanical coupling effects. JMSE 6, 38. doi:10.3390/jmse6020038
García-Rodríguez, S., Torres, B., Pulido-González, N., Otero, E., and Rams, J. (2019). Corrosion behavior of 316L stainless steel coatings on ZE41 magnesium alloy in chloride environments. Surf. Coatings Technol. 378, 124994. doi:10.1016/j.surfcoat.2019.124994
Gharaibeh, M. A. (2022). Shock and impact reliability of electronic assemblies with perimeter vs full array layouts: a numerical comparative study. J. Mech. Behav. Mater. 31, 535–545. doi:10.1515/jmbm-2022-0058
Grassini, S., Matteis, P., Scavino, G., Rossetto, M., and Firrao, D. (2011). “Salt spray corrosion of mechanical junctions of magnesium castings,” in Magnesium Technology 2011. Editors W. H. Sillekens, S. R. Agnew, N. R. Neelameggham, and S. N. Mathaudhu (Cham: Springer International Publishing), 493–499. doi:10.1007/978-3-319-48223-1_92
Gross, D., and Seelig, T. (2011). “Dynamic fracture mechanics,” in Fracture mechanics (Berlin, Heidelberg: Springer Berlin Heidelberg), 207–228. doi:10.1007/978-3-642-19240-1_7
Guedon-Gracia, A., Fremont, H., Deletage, J.-Y., and Weide-Zaage, K. (2016). “Corrosion study on BGA assemblies,” in 2016 Pan pacific microelectronics symposium (Pan pacific), (big island, HI: ieee), 1–7. doi:10.1109/PanPacific.2016.7428402
Hartler, G. (1987). Estimation and test for the parameters of the arrhenius model. Qual. and Reliab. Eng 3, 219–225. doi:10.1002/qre.4680030404
He, Y., and Kabiri, M. (2022). In-situ characterization of moisture absorption and hygroscopic swelling of an epoxy molding compound for electronic packaging. J. Therm. Anal. Calorim. 147, 5667–5675. doi:10.1007/s10973-021-10941-w
He, Y., Liu, Y., Wang, C., Cao, G., He, C., and Wang, Z. (2024). Corrosion behavior of 700L automotive beam steel in marine atmospheric environment. Materials 17, 4964. doi:10.3390/ma17204964
Hu, X., Liu, G., Liu, Q., Zhu, W., Liu, S., and Ma, Z. (2022). Failure mechanism of EB-PVD thermal barrier coatings under the synergistic effect of thermal shock and CMAS corrosion. Coatings 12, 1290. doi:10.3390/coatings12091290
Istrate, G. G., and Mureșan, A. C. (2021). Corrosion behavior of materials Al5083 alloy, 316L stainless steel and A681 carbon steel in seawater. Ann_UGAL_MMS 44, 39–46. doi:10.35219/mms.2021.2.07
Jenq, S. T., Chang, H.-H., Lai, Y.-S., and Tsai, T.-Y. (2009). High strain rate compression behavior for Sn–37Pb eutectic alloy, lead-free Sn–1Ag–0.5Cu and Sn–3Ag–0.5Cu alloys. Microelectron. Reliab. 49, 310–317. doi:10.1016/j.microrel.2009.01.002
Jia, X. (2021). “Influence of marine climate on corrosion of common materials for electronic equipment,” in 2021 CIE international conference on radar (radar), 3331–3335. doi:10.1109/Radar53847.2021.10028025
Jia, X., Wu, L., Chen, X., Zhou, J., and Zhu, J. (2020). “Corrosion control of airborne electronic equipment in environment of marine climate,” in Proceedings of the seventh asia international symposium on mechatronics. Editors B. Duan, K. Umeda, and W. Hwang (Singapore: Springer), 559–563. doi:10.1007/978-981-32-9437-0_58
Jiang, T., Nong, H., and Du, C. (2008). “Study of interface reliability in QFN device under hygro-thermal environment,” in 2008 international conference on electronic packaging Technology and high density packaging (Shanghai: IEEE), 1–5. doi:10.1109/ICEPT.2008.4607146
Jiang, X., Zhang, C., Pan, Z., Wang, J., and Liu, Y. (2024). “Failure characteristics and mechanisms of Sn-based solder interconnections under vibrational conditions,” in 2024 25th international conference on electronic packaging Technology (ICEPT) (Tianjin, China: IEEE). 01–04. doi:10.1109/ICEPT63120.2024.10668776
Khatibi, G., Betzwar Kotas, A., and Lederer, M. (2018). Effect of aging on mechanical properties of high temperature Pb-rich solder joints. Microelectron. Reliab. 85, 1–11. doi:10.1016/j.microrel.2018.03.009
Lall, P., Arunachalam, D., and Suhling, J. (2011). “Ridge regression based development of norris-landzberg acceleration factors and goldmann constants for leadfree electronics,” in ASME 2011 pacific rim technical conference and exhibition on packaging and integration of electronic and photonic systems, MEMS and NEMS (Portland, Oregon, USA: ASMEDC), 1, 537–547. doi:10.1115/IPACK2011-52195
Li, Z., Xiao, K., Dong, C., Cheng, X., Xue, W., and Yu, W. (2019). Atmospheric corrosion behavior of low-alloy steels in a tropical marine environment. J. Iron Steel Res. Int. 26, 1315–1328. doi:10.1007/s42243-019-00316-9
Ling, S., Xu, L., Li, D., Wu, S., and Zhai, G. (2019). “Research on composite fretting behaviour of Au-plated copper wire spring contacts used in electrical connectors,” in 2019 IEEE holm conference on electrical contacts (Milwaukee, WI, USA: IEEE), 211–217. doi:10.1109/HOLM.2019.8924029
Liu, B., Lee, T.-K., and Liu, K.-C. (2011). Impact of 5% NaCl salt spray pretreatment on the long-term reliability of wafer-level packages with Sn-Pb and Sn-Ag-Cu solder interconnects. J. Elec Materi 40, 2111–2118. doi:10.1007/s11664-011-1705-y
Liu, N., Jiang, B., Ji, Z., Cui, P., Wang, Y., and Song, H. (2022). Salt spray corrosion and electrochemical corrosion property of anodic oxide films on ADC12 aluminum alloy. Mod. Phys. Lett. B 36, 2150571. doi:10.1142/S0217984921505710
Liu, Y., Long, X., Wang, H., Lu, J., Sun, R., and Wong, C.-P. (2021). “Sequential analysis of drop impact and thermal cycling of electronic packaging structures,” in 2021 22nd international conference on electronic packaging Technology (ICEPT) (Xiamen, China: IEEE), 1–4. doi:10.1109/ICEPT52650.2021.9568020
Liu, Y., Meerwijk, J., Luo, L., Zhang, H., Sun, F., Yuan, C. A., et al. (2014). Formation and evolution of intermetallic layer structures at SAC305/Ag/Cu and SAC0705-Bi-Ni/Ag/Cu solder joint interfaces after reflow and aging. J. Mater Sci. Mater Electron 25, 4954–4959. doi:10.1007/s10854-014-2257-8
Long, X., Xu, J., Wang, S., Tang, W., and Chang, C. (2020). Understanding the impact response of lead-free solder at high strain rates. Int. J. Mech. Sci. 172, 105416. doi:10.1016/j.ijmecsci.2020.105416
Lu, N., Zhang, Z., Yoon, J., and Suo, Z. (2012). Singular stress fields at corners in flip-chip packages. Eng. Fract. Mech. 86, 38–47. doi:10.1016/j.engfracmech.2012.02.010
Mei, Y., and Yao, X. (2011). “A numerical method on thermal-humidity behavior of electronic packaging,” in 2011 12th international conference on electronic packaging Technology and high density packaging (Shanghai, China: IEEE), 1–5. doi:10.1109/ICEPT.2011.6067007
Neville, A. (1995). An investigation of the corrosion behaviour of a range of engineering materials in marine environments (Doctoral thesis). Glasgow, United Kingdom: University of Glasgow. Available online at: https://eleanor.lib.gla.ac.uk/record=b1550658 (Accessed February 10, 2025).
Nguyen, Q., Roberts, J. C., Suhling, J. C., and Jaeger, R. C. (2017). “A study of moisture and thermally induced die stresses in plastic ball grid array packages,” in 2017 16th IEEE intersociety conference on thermal and thermomechanical phenomena in electronic systems (ITherm) (Orlando, FL: IEEE), 1185–1196. doi:10.1109/ITHERM.2017.7992622
Nousiainen, O., Putaala, J., Kangasvieri, T., Rautioaho, R., and Vähäkangas, J. (2007). Failure mechanisms of thermomechanically loaded SnAgCu/plastic core solder ball composite joints in low-temperature Co-fired ceramic/printed wiring board assemblies. J. Elec Materi 36, 232–241. doi:10.1007/s11664-006-0066-4
Pongvittayapanu, K., Srisrual, A., and Fakpan, K. (2022). Effect of thermal cycling and vibration on cracking in Sn-3.0Ag-0.5Cu solder bump. Mater. Today Proc. 52, 2372–2376. doi:10.1016/j.matpr.2021.10.222
Qin, Q., Tang, B., Wei, C., Zhang, F., and Wei, B. (2024). Corrosion behavior of riveted joints with electrophoretic treatment in neutral salt spray environment. Mater. and Corros. 75, 665–677. doi:10.1002/maco.202314097
Rabiei, E., White, M., Mosleh, A., Lyer, S., and Woo, J. (2018). “Component reliability modeling through the use of bayesian networks and applied physics-based models,” in 2018 annual Reliability and maintainability symposium (RAMS), (reno, NV: ieee), 1–7. doi:10.1109/RAM.2018.8462986
Rong, H., Zhao, X., Zhao, Z., Sun, H., Fu, Q., Ding, R., et al. (2021). Effect of acinetobacter sp. on corrosion behavior of 10MnNiCrCu steel in simulated marine environment. Int. J. Electrochem. Sci. 16, 210638. doi:10.20964/2021.06.55
Rongen, R., O’Halloran, G., Mavinkurve, A., Goumans, L., and Farrugia, M.-L. (2014). “Lifetime prediction of Cu-Al wire bonded contacts for different mould compounds,” in 2014 IEEE 64th electronic components and Technology conference (ECTC) (IEEE), 411–418.
Schmitz, D., Shirazi, S., Wentlent, L., Hamasha, S., Yin, L., Qasaimeh, A., et al. (2014). “Towards a quantitative mechanistic understanding of the thermal cycling of SnAgCu solder joints,” in 2014 IEEE 64th electronic components and Technology conference (ECTC) (Orlando, FL, USA: IEEE), 371–378. doi:10.1109/ECTC.2014.6897314
Shi, D., Fan, X., and Xie, B. (2008). “A new method for equivalent acceleration of JEDEC moisture sensitivity levels,” in 2008 58th electronic Components and Technology conference, (lake buena vista, FL, USA: ieee), 907–912. doi:10.1109/ECTC.2008.4550084
Shi, Z., Cao, F., Song, G.-L., Liu, M., and Atrens, A. (2013). Corrosion behaviour in salt spray and in 3.5% NaCl solution saturated with Mg(OH)2 of as-cast and solution heat-treated binary Mg–RE alloys: RE=Ce, La, Nd, Y, Gd. Corros. Sci. 76, 98–118. doi:10.1016/j.corsci.2013.06.032
Son, H. H., Long, N. P., Thanh, N. V., Ngoc, N. T. H., Thuy, D. M., Pham, L. Q., et al. (2023). Polyalphaolefin oil/MgO-20 nanofluids coating shows corrosion resistance, high moisture resistance, and water resistance for electrical and electronic equipment. Coatings 13, 1576. doi:10.3390/coatings13091576
Su, X., Luo, H., and Yang, D. G. (2006). “Investigation of thermal-mechanical and moisture driven delamination in lead-free QFN packages during SMT reflow soldering process,” in 2006 7th international conference on electronic packaging Technology (Shanghai, China: IEEE), 1–6. doi:10.1109/ICEPT.2006.359847
Teo, J. W. R. (2007). Thermal cycling aging effect on the reliability and morphological evolution of SnAgCu solder joints. IEEE Trans. Electron. Packag. Manufact. 30, 279–284. doi:10.1109/TEPM.2007.907575
Tian, W., Chen, X., Zhang, G., Chen, Y., and Luo, J. (2024). Delamination of plasticized devices in dynamic service environments. Micromachines 15, 376. doi:10.3390/mi15030376
Tong, Y. T., and Ng, H. S. (2002). “Whole field vapor pressure modeling of QFN during reflow with coupled hygro-mechanical and thermo-mechanical stresses,” in 52nd electronic components and Technology conference 2002. (Cat. No.02CH37345) (San Diego, CA, USA: IEEE), 1552–1559. doi:10.1109/ECTC.2002.1008314
Wang, J., Niu, Y., Shao, S., Wang, H., Xu, J., Pham, V., et al. (2020). A comprehensive solution for modeling moisture induced delamination in electronic packaging during solder reflow. Microelectron. Reliab. 112, 113791. doi:10.1016/j.microrel.2020.113791
Wang, Y., Deng, E., Wu, L., Yan, Y., Zhao, Y., and Huang, Y. (2022). Influence of humidity on the power cycling lifetime of SiC MOSFETs. IEEE Trans. Components, Packag. Manuf. Technol. 12, 1781–1790. doi:10.1109/TCPMT.2022.3223957
Wu, M.-L., and Chang, Y.-T. (2021). “Simulation study of the high-G drop impact testing of microelectronic packaging products,” in 2021 20th IEEE intersociety conference on thermal and thermomechanical phenomena in electronic systems (iTherm) (San Diego, CA, USA: IEEE), 751–755. doi:10.1109/ITherm51669.2021.9503272
Xiao, H., Li, X. Y., Hu, Y., Guo, F., and Shi, Y. W. (2013). Damage behavior of SnAgCu/Cu solder joints subjected to thermomechanical cycling. J. Alloys Compd. 578, 110–117. doi:10.1016/j.jallcom.2013.05.026
Xiao, L., Peng, J., Zhang, J., Ma, Y., and Cai, C. S. (2020). Comparative assessment of mechanical properties of HPS between electrochemical corrosion and spray corrosion. Constr. Build. Mater. 237, 117735. doi:10.1016/j.conbuildmat.2019.117735
Xie, X., Bi, X., and Li, G. (2009). “Thermal-mechanical fatigue reliability of pbsnag solder layer of die attachment for power electronic devices,” in 2009 international conference on electronic packaging Technology and high density packaging (Beijing, China: IEEE), 1181–1185. doi:10.1109/ICEPT.2009.5270615
Xu, C., Du, L., Yang, B., and Zhang, W. (2011). Study on salt spray corrosion of Ni–graphite abradable coating with 80Ni20Al and 96NiCr–4Al as bonding layers. Surf. Coatings Technol. 205, 4154–4161. doi:10.1016/j.surfcoat.2011.03.007
Xu, D., Huang, J., Zhu, Q., Chen, X., Xu, Y., and Wang, S. (2012). Residual fatigue life prediction of ball bearings based on Paris law and RMS. Chin. J. Mech. Eng. 25, 320–327. doi:10.3901/CJME.2012.02.320
Xu, J., Guo, Y., Su, Y., Tang, R., and Long, X. (2021). “Growth kinetics of intermetallic compound in solder joints during thermal cycling: a review,” in 2021 IEEE 23rd electronics packaging Technology conference (EPTC) (Singapore, Singapore: IEEE), 464–468. doi:10.1109/EPTC53413.2021.9663867
Yan, L., Xiao, K., Yi, P., Dong, C., Wu, J., Mao, C., et al. (2016). Surface analysis of silver-plated circuit boards in a salt-spray environment. J. Alloys Compd. 688, 301–312. doi:10.1016/j.jallcom.2016.07.207
Yang, Z., Yu, X., Liu, L., and Wang, D. (2022). “Research on ceramic package for high temperature electronic devices,” in 2022 23rd international conference on electronic packaging Technology (ICEPT) (Dalian, China: IEEE), 1–4. doi:10.1109/ICEPT56209.2022.9873506
Yi, P., Xiao, K., Ding, K., Wang, X., Yan, L., Mao, C., et al. (2015a). Electrochemical corrosion failure mechanism of M152 steel under a salt-spray environment. Int. J. Min. Metall. Mater 22, 1183–1189. doi:10.1007/s12613-015-1183-y
Yi, P., Xiao, K., Dong, C., Ding, K., Liu, M., and Li, X. (2015b). Field studies of corrosion behaviour of printed circuit board and hot air solder levelling during the marine environment of industrial pollution. Int. J. Electrochem. Sci. 10, 7754–7770. doi:10.1016/S1452-3981(23)17387-1
Zhang, P. C., Zhang, J., Jie, J. C., Gao, Y., Dong, Y., Wang, T. M., et al. (2014). Electrochemical and salt spray corrosion behavior of copper alloy contact wires in chloride solution. MSF 789, 622–626. doi:10.4028/www.scientific.net/MSF.789.622
Zhendong, Z., Zhigang, Li, Yu, Z., and Xuefeng, S. (2008). “Moisture absorption and void growing effects on failure of electronic packaging,” in 2008 international conference on electronic packaging Technology and high density packaging (Shanghai: IEEE), 1–4. doi:10.1109/ICEPT.2008.4607128
Zheng, L., Deng, Y.-K., Wang, H.-G., Lyu, G.-C., Yang, B.-X., Hu, W.-L., et al. (2022). “Moisture desorption and hygro-vapor-thermal induced interfacial delamination in underfilled FCBGA package during reflow process,” in 2022 23rd international conference on electronic packaging Technology (ICEPT) (Dalian, China: IEEE), 1–5. doi:10.1109/ICEPT56209.2022.9873388
Zheng, Z., Wang, E., Liu, X., Duan, Z., Zhang, L., Ou, Z., et al. (2017). The dynamic response of brittle materials under impact loading. Int. J. Nonlinear Sci. Numer. Simul. 18, 115–127. doi:10.1515/ijnsns-2016-0019
Zhou, X., Li, Y., Yang, X., Xie, A., Wang, H., and Cao, C. (2022). “Research on reliability of SnAg1.8/Cu solder joints in extreme environment,” in 2022 23rd international conference on electronic packaging Technology (ICEPT) (Dalian, China: IEEE), 1–6. doi:10.1109/ICEPT56209.2022.9872622
Zhou, Z., Chen, J., Yu, C., Wang, Y., and Zhang, Y. (2023). Failure analysis of printed circuit board solder joint under thermal shock. Coatings 13, 572. doi:10.3390/coatings13030572
Keywords: marine environment, electronic packaging materials, salt spray corrosion, moisture-induced plasticization, temperature cycling, mechanical shock
Citation: Lang F, Zhou Z, Liu J, Cui M and Zhang Z (2025) Review on the impact of marine environment on the reliability of electronic packaging materials. Front. Mater. 12:1584349. doi: 10.3389/fmats.2025.1584349
Received: 27 February 2025; Accepted: 03 April 2025;
Published: 15 April 2025.
Edited by:
Chuantong Chen, Osaka University, JapanReviewed by:
Bowen Zhang, Tianjin Polytechnic University, ChinaCanyu Liu, Huazhong University of Science and Technology, China
Copyright © 2025 Lang, Zhou, Liu, Cui and Zhang. This is an open-access article distributed under the terms of the Creative Commons Attribution License (CC BY). The use, distribution or reproduction in other forums is permitted, provided the original author(s) and the copyright owner(s) are credited and that the original publication in this journal is cited, in accordance with accepted academic practice. No use, distribution or reproduction is permitted which does not comply with these terms.
*Correspondence: Zhongqing Zhang, YmVja2VldEAxMjYuY29t