- 1Department of Entomology, College of Plant Protection, Nanjing Agricultural University, Nanjing, China
- 2Department of Landscape Architecture, College of Biological and Agricultural Engineering, Weifang University, Weifang, China
- 3Jinshanbao Experimental Class, College of Agriculture, Nanjing Agricultural University, Nanjing, China
- 4Department of Phytology, College of Life Sciences, Nanjing Agricultural University, Nanjing, China
The promotion and application of transgenic Bt crops provides an approach for the prevention and control of target lepidopteran pests and effectively relieves the environmental pressure caused by the massive usage of chemical pesticides in fields. However, studies have shown that Bt crops will face a new risk due to a decrease in exogenous toxin content under elevated carbon dioxide (CO2) concentration, thus negatively affecting the ecological sustainability of Bt crops. Arbuscular mycorrhizal fungi (AMF) are important beneficial microorganisms that can effectively improve the nutrient status of host plants and are expected to relieve the ecological risk of Bt crops under increasing CO2 due to global climate change. In this study, the Bt maize and its parental line of non-transgenic Bt maize were selected and inoculated with a species of AMF (Funneliformis caledonium, synonyms: Glomus caledonium), in order to study the secondary defensive chemicals and yield of maize, and to explore the effects of F. caledonium inoculation on the growth, development, and reproduction of the pest Mythimna separata fed on Bt maize and non-Bt maize under ambient carbon dioxide concentration (aCO2) and elevated carbon dioxide concentration (eCO2). The results showed that eCO2 increased the AM fungal colonization, maize yield, and foliar contents of jasmonic acid (JA) and salicylic acid (SA), but decreased foliar Bt toxin content and Bt gene expression in Bt maize leaves. F. caledonium inoculation increased maize yield, foliar JA, SA contents, Bt toxin contents, and Bt gene expression in Bt maize leaves, and positively improved the growth, development, reproduction, and food utilization of the M. separata fed on non-Bt maize. However, F. caledonium inoculation was unfavorable for the fitness of M. separata fed on Bt maize, and the effect was intensified when combined with eCO2. It is indicated that F. caledonium inoculation had adverse effects on the production of non-Bt maize due to the high potential risk of population occurrence of M. separata, while it was just the opposite for Bt maize. Therefore, this study confirms that the AMF can increase the yield and promote the expression levels of its endogenous (JA, SA) and exogenous (Bt toxin) secondary defense substances of Bt maize under eCO2, and finally can enhance the insect resistance capacity of Bt crops, which will help ensure the sustainable utilization and safety of Bt crops under climate change.
Introduction
In recent years, many transgenic Bt crops, such as Bt maize and Bt cotton, have been grown around the world and have shown high resistance to specific target pests, mainly Lepidoptera insects (Wu et al., 2008; Liu et al., 2016). As a result, Bt crops have been used to control a wider range of pests, such as Helicoverpa armigera (Hübner), Heliothis virescens, and Mythimna separata (Riddick et al., 1998; Chen F. J. et al., 2011; Chang et al., 2013). Meanwhile, human activities, specifically fossil fuel burning and land-use change, are rapidly increasing the level of carbon dioxide (CO2) in the atmosphere (Yu and Chen, 2019; Yao et al., 2020). Specifically, it has been reported that the atmospheric CO2 concentration increased from 288 to 405 ppm from 1800 to 2018 (www.esrl.noaa.gov/gmd/ccgg/trends/). With the acceleration of industrialization, it is estimated that the concentration of CO2 in the atmosphere will increase from 800 to 1,000 ppm by the end of the twenty-first century (Pachauri and Reisinger, 2014).
Plant productivity is fundamentally tied to atmospheric CO2 by photosynthesis, and the increase in atmospheric CO2 concentration can improve the photosynthetic capacity of plants and undoubtedly affect the plant physiology with profound impacts on all aspects, including the increase in photosynthetic rate, biomass, and seed production (Dietterich et al., 2015; Johnson and Hartley, 2018; Zhu et al., 2018). Most studies reported that elevated CO2 (eCO2) increased the C/N ratio in plant tissues; thus, the content of carbohydrates in plant tissues increased and the content of N-containing compounds decreased (Chen et al., 2005a; Xu et al., 2015; Dai et al., 2018). All these changes in turn affect the production of plant secondary metabolites (Stiling and Cornelissen, 2007). Elevated CO2 may enhance or weaken plant defense against herbivorous insects, at least partly due to the changes in C- and N-based defensive metabolites, as well as plant nutrients, especially protein content (Kretzschmar et al., 2009). Some studies have shown that jasmonic acid (JA), ethylene (ET), and salicylic acid (SA) are secondary defense substances of plants against aphids under eCO2 (Sun et al., 2013; Guo et al., 2014), and reported that eCO2 caused a significant reduction of N-based compounds (i.e., Bt toxin proteins) in Bt crops (Chen et al., 2005b; Wu et al., 2011a; Liu et al., 2019). Hence, it is speculated that under the condition of climate change, transgenic Bt crops will face a new risk that the effective control of the target pests will be reduced.
Arbuscular mycorrhizal fungi (AMF) can form associations with the roots of about 80% of terrestrial plant species (Smith and Read, 2008) and exchange soil-derived nutrients (Marschner and Dell, 1994) for plant-derived hexoses and lipids (Helber et al., 2011; Keymer and Lankau, 2017). AMF improve the supply of inorganic nutrients, especially phosphate (Rillig and Mummey, 2006). However, since AMF can also enhance the nitrogen uptake and utilization of plants (Hawkins et al., 2000) and improve their resistance to external biotic and abiotic stresses (Jung et al., 2012; Frew and Price, 2019), we hypothesize that they can be used to alleviate the problems of Bt toxin protein decline under eCO2, and reduce the risk of Bt crops under future climate change. In order to test this hypothesis, we inoculated Bt maize with AMF (Funneliformis caledonium) under elevated CO2 concentration to explore the interaction between eCO2 and AM fungal inoculation on plant growth and secondary defense metabolites of Bt maize, and the effects on the growth and development and food utilization of the main maize pest armyworm from 2017 to 2018. We further hypothesize that F. caledonium inoculation under eCO2 could (a) increase the biomass and yield of maize; (b) promote the expression of endogenous (JA, SA) and exogenous (Bt toxin) secondary defense substances in Bt maize leaves; and (c) decrease indices of growth, development, and reproduction of M. separata.
Materials and Methods
CO2 Setting
A 2-year experiment (2017–2018) was conducted in six open-top chambers (OTCs) in Ningjin County, Shandong Province of China (37.64° N, 116.8° E). OTCs are 2.5 m in height × 3.2 m in diameter. Two concentration levels of CO2 are applied successively, namely, the ambient level (aCO2, 375 μl/l) and the elevated level (eCO2, 750 μl/l). Each CO2 treatment uses three OTCs. During the experiment, OTCs were continuously filled with CO2. The average CO2 concentration is shown in Supplementary Table 1.
Arbuscular Mycorrhizal Fungi and Bt Maize
Funneliformis caledonium (strain number 90036, referred to as FC, synonyms: Glomus caledonium) was provided by the State Key Laboratory of Soil & Sustainable Agriculture, Institute of Soil Science, the Chinese Academy of Sciences. The inoculum consisted of spores, mycelium, maize root fragments, and soil (storage: under normal temperature, keep in dry, cool place). The Bt maize cultivar (line IE09S034 with Cry1Ie, namely, Bt) and the parental line of non-transgenic Bt maize (cv. Xianyu 335, namely, Xy) were provided by The Institute of Crop Sciences of the Chinese Academy of Agricultural Science. Bt maize and non-Bt maize were planted in plastic barrels (the height of 45 cm and the diameter of 30 cm) filled with 20 kg soils and 10 g compound fertilizer (N: P: K = 18: 15: 12), respectively. Soil pH was 7.2, organic carbon 11.7 g/kg, total nitrogen 2.27 g/kg, and total phosphorus 0.56 g/kg. On June 10 of each sampling year, 100 g inoculum of F. caledonium (namely, FC in figure) and 100 g sterilized strain (namely, CK in figure) were evenly spread at 4 cm under maize seed as control; three maize seeds were sown in each barrel with a sowing depth of 2 cm, and two maize were reserved after emergence. Maize were irrigated every two to three days to ensure the water demand for maize growth.
There were eight treatments [two CO2 concentration (aCO2 and eCO2), two maize treatments (Bt and Xy), and two AMF inoculations (F. caledonium and CK)]. Each treatment included three OTCs; each OTC included four planting patterns (Bt + AMF, Bt + CK, Xy + AMF, and Xy + CK); each planting pattern included five repeats; that is, each treatment contained a total of 15 repeats.
Arbuscular Mycorrhizal Fungi Colonization and AMF Phospholipid Fatty Acid (PLFA) Content
In two sampling years, AMF colonization was determined on heading stage (BBCH-59), and it was determined by the method of trypan blue staining and grid counting (Phillips and Hayman, 1970). The fresh plant roots were washed with distilled water and then blotted dry with absorbent paper. One hundred one centimeter roots were randomly cut and placed in a 10% KOH solution at 30°C for 30 min, and then, the KOH was discarded and rinsed with distilled water. After acidification in 2% HCl for 60 min, the HCl was discarded, rinsed with distilled water, and stained in 5% trypan blue dye solution (w/v, lactic acid: glycerol: water = 1: 1: 1). Then, the dye solution was discarded, and the roots were rinsed with distilled water and transferred to a square with a grid at the bottom. We observed the number of infected and uninfected root segments under the microscope. Colonization (%) = number of infected root segments/total root segments (Mcgonigle et al., 1990). The improved Bligh-Dyer method was used to extract microbial PLFA from soil (Bossio and Scow, 1998; Ruess and Chamberlain, 2010). About 8.0 g of the freeze-dried soil sample was weighed into a Teflon tube, and the lipids from soil were extracted by multiple oscillation centrifugation with a Bligh-Dyer mixed extract prepared in a ratio of 1: 2: 0.8 with chloroform: methanol: citrate buffer. The aqueous phase and the organic phase were separated by keeping away from light overnight, and the lower organic-phase supernatant containing the phospholipid was taken up and then dried with nitrogen in a water bath. The sample was dissolved and dried with a small amount of chloroform and acetone several times and passed through a SPE silica gel column to remove neutral lipids and glycolipids from the sample, followed by separation and purification with methanol to collect PLFA, and dried with nitrogen again. The separated PLFA is methylated by a liquid (methanol: toluene 1:1 mixture) and a liquid of KOH–methanol solution, and then the reaction was terminated with acetic acid. Finally, it was extracted with n-hexane and dried with nitrogen to obtain PLFA methyl ester. With C19: 0 as the internal standard, the content of methyl ester of characteristic fatty acids was analyzed by MIDI identification system, and the soil PLFA content was expressed in nmol/g through peak area and internal standard curve.
Foliar Bt Toxin Content and Bt Gene Expression in the Leaves of Bt Maize
During the heading stage (BBCH-59) of maize, the foliar content of Bt toxin protein was measured by using plant Bt-Cry1Ie protein ELISA Kit (mlbio, China). Moreover, the real-time quantitative reverse transcription PCR was performed on a 7500 real-time PCR system (Applied Biosystems Inc.) for Bt gene expression analysis. Total RNA was extracted from the leaf tissues by using TRIzol® reagent (Invitrogen). The concentration and quality of samples were determined by NanoDrop™ spectrophotometer (Thermo Scientific) and 1.5% agarose gel electrophoresis. The cDNA synthesis was carried out with 100 ng of total RNA by using PrimeScript™ RT reagent Kit with gDNA Eraser (Takara, Japan). Reverse transcriptase reactions were performed in a reaction volume of 20 μl. Quantitative real-time PCR was performed with a 7500 real-time PCR detection system (Applied Biosystems) using 1 × SYBR® Premix Ex Taq™ (TaKaRa, Japan), 2 μl cDNA products (diluted from 20 to 200 μl with RNase-free water), and 0.2 μM primers in a final volume of 20 μl. Reaction conditions are 95°C, 30 s pre-denaturation; 95°C, 5 s, 60°C, 34 s, 40 cycles. The cDNA was amplified by PCR using the primers shown in Supplementary Table 2. Quantification of the transcript levels of target genes was conducted by following the 2−ΔΔCt normalization method. The relative expression level was represented as the fold change by comparing three treatments (aCO2 + AMF, aCO2 +CK, and eCO2 +AMF) and the treatment of eCO2 + CK, respectively. Three technical replicates were performed on each sample of cDNA.
Jasmonic Acid and Salicylic Acid Contents in Maize Leaves
During the heading stage (BBCH-59) of maize, the foliar contents of JA and SA were measured in our laboratory by using plant JA ELISA Kit (YaJi Biological, China) and plant SA ELISA Kit (YaJi Biological, China).
Insect Development and Food Utilization
The colony of armyworm, M. separata, was collected in maize fields in Kangbao County, Hebei (China), and continuously reared on artificial diet for more than 15 generations in growth chamber (GDN-400D-4; Ningbo Southeast Instrument CO., LTD, Ningbo, China) (Song et al., 2020). The third-instar larvae with uniform size were randomly selected and were individually fed on fresh maize leaves, which were selected from each treatment at the heading stage (BBCH-59). The feeding trials were conducted in a plastic dish (6 cm in diameter) in 2017 and 2018. Each treatment consisted of five replicates (a total of 20 larvae per replicate).
The initial weights of third-instar larvae were individually measured with an electronic balance (AL104; METTLER-TOLEDO, Greifensee). Larvae feces, pupal weight, and the remaining parts of leaves were also carefully weighed. At the same time, dry weight of larvae and maize leaves was calculated during the experiment. Moreover, the food utilization indices, including relative consumption rate (RCR), relative growth rate (RGR), efficiency of conversion of digested food (ECD), and efficiency of conversion of ingested food (ECI), were measured (Chen et al., 2005b).
where I is the feeding amount (the weight of maize leaves before feeding minus the weight of maize leaves before feeding and after feeding); B is the average larval weight during the experiment (the average larval weight before feeding and after feeding); T is the experiment time (d); G is the added larval weight (the larval weight after feeding minus the larval weight before feeding); and F is the weight of total feces.
The larval lifespan, pupation rate/duration, and emergence of M. separata fed on leaves of Bt and non-Bt maize inoculated with and without F. caledonium were recorded every 12 h. Pairs of novel moths, including female: male ratio of 1: 1, were transferred to metal screen cages for oviposition and fed on 10% honey. The survivorship and fecundity of M. separata were observed every day until death.
Data Analysis
Data were analyzed using IBM-SPSS v.20.0 software (IBM, Armonk, NY). Three-way ANOVAs were used to test the effects of sampling years (2017 vs. 2018), CO2 levels (elevated vs. ambient), AMF inoculation (F. caledonium vs. CK), transgenic treatment (Bt maize vs. non-Bt maize), and their interactions on the indices of Bt toxin and Bt gene expression in the leaves of Bt maize. Four-way ANOVAs were used to test the effects of sampling years (2017 vs. 2018), CO2 levels (elevated vs. ambient), AMF inoculation (F. caledonium vs. CK), transgenic treatment (Bt maize vs. non-Bt maize), and their interactions on the indices of AMF colonization and AMF-PLFA content, foliar contents of JA and SA, and growth, development, and reproduction of M. separata. Significant differences between or among treatments were analyzed by Tukey's test at P < 0.05.
Results
Arbuscular Mycorrhizal Fungi Colonization and AMF-PLFA Content of Bt and Non-Bt Maize Influenced by CO2 Levels and F. caledonium Inoculation
Four-way ANOVAs showed that AMF inoculation, CO2 level, and sampling years, and the interactions between AMF inoculation and CO2 level (F ≥ 4.47, P ≤ 0.042 < 0.05) significantly affected the AMF colonization on Bt and non-Bt maize (Supplementary Table 3). Compared with control, AMF inoculation significantly increased the AMF colonization of Bt and non-Bt maize in two sample years no matter under aCO2 or eCO2 (Figures 1A,C). Compared with aCO2, eCO2 significantly increased the AMF colonization of non-Bt maize inoculated with F. caledonium in 2017 and 2018, that of Bt maize inoculated with F. caledonium in 2017, and that of Bt maize not inoculated in 2018 (P < 0.05; Figures 1A,C).
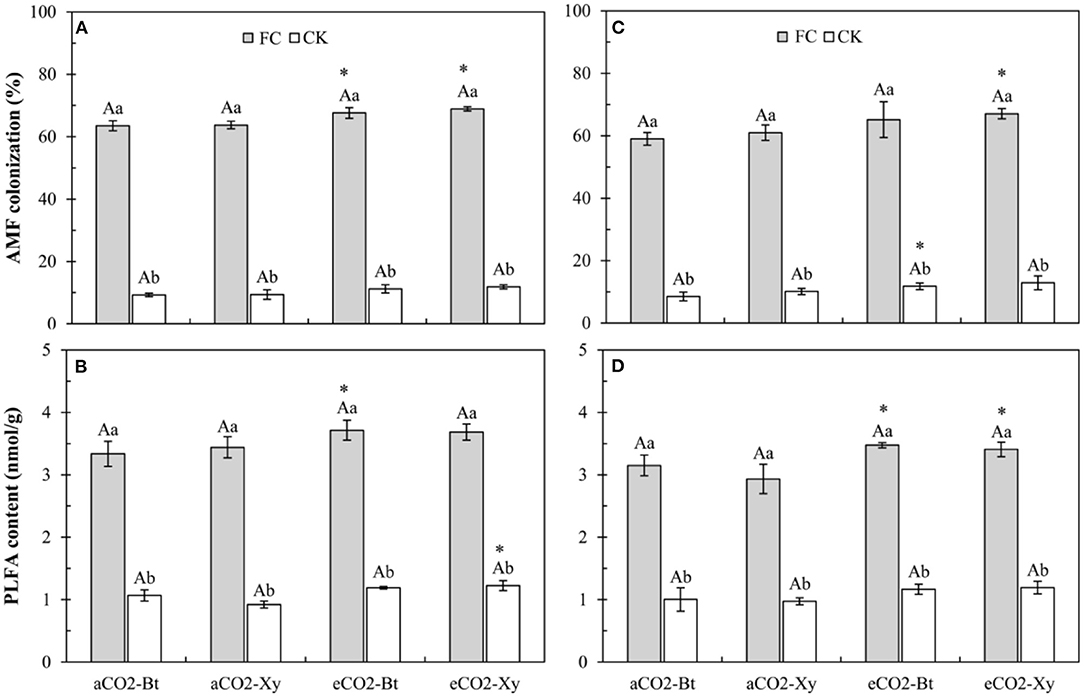
Figure 1. Effects of the inoculation with arbuscular mycorrhizal fungi (AMF), F. caledonium, on the AMF colonization and AMF-PLFA content of Bt maize (Bt) and its parental line of non-Bt maize (Xy) under ambient CO2 (aCO2) and elevated CO2 (eCO2) at the heading stage (BBCH-59) in 2017 (A,B) and 2018 (C,D). (FC, F. caledonium inoculation; CK, the control without F. caledonium inoculation; aCO2-Bt, Bt maize under aCO2; aCO2-Xy, non-Bt maize under aCO2; eCO2-Bt, Bt maize under eCO2; eCO2-Xy, non-Bt maize under eCO2; different uppercase, lowercase letters, and * indicate significant difference between Bt maize and non-Bt maize, between F. caledonium inoculation and non-inoculation of F. caledonium, and between aCO2 and eCO2 under the same other conditions as revealed by Tukey's test) (P < 0.05; n = 15).
Moreover, four-way ANOVAs also showed that AMF inoculation (F = 3385.14, P < 0.001), CO2 level (F = 52.68, P < 0.001), and sampling years (F = 17.27, P < 0.001) significantly affected the AMF-PLFA content of Bt and non-Bt maize (Supplementary Table 3). Compared with control, AMF inoculation significantly increased the AMF-PLFA content of Bt and non-Bt maize in two sample years no matter under aCO2 or eCO2 (Figures 1B,D). Compared with aCO2, eCO2 significantly increased the AMF-PLFA content of Bt maize inoculated with F. caledonium in 2017 and 2018, and also significantly increased the AMF-PLFA content of non-Bt maize inoculated with F. caledonium in 2018 and non-Bt maize not inoculated in 2017 (P < 0.05; Figures 1B,D).
Foliar Bt Protein Content and Bt Gene Relative Expression Level in Leaves of Bt Maize Influenced by CO2 Levels and F. caledonium Inoculation
Three-way ANOVAs showed that AMF inoculation (F ≥ 275.07, P < 0.001), CO2 level (F ≥ 5.89, P ≤ 0.027), and their interaction (F ≥ 18.88, P < 0.001) significantly affected the foliar Bt protein content and Bt gene relative expression level in the leaves of Bt maize (Supplementary Table 4).
For the foliar Bt protein content, compared with aCO2, eCO2 significantly decreased the foliar Bt protein content of Bt maize without F. caledonium inoculation, but significantly increased the foliar Bt protein content of Bt maize inoculated with F. caledonium (Figures 2A,C). Compared with control, AMF inoculation significantly increased the foliar Bt protein content of Bt maize under aCO2 and eCO2 (P < 0.05; Figures 2A,C).
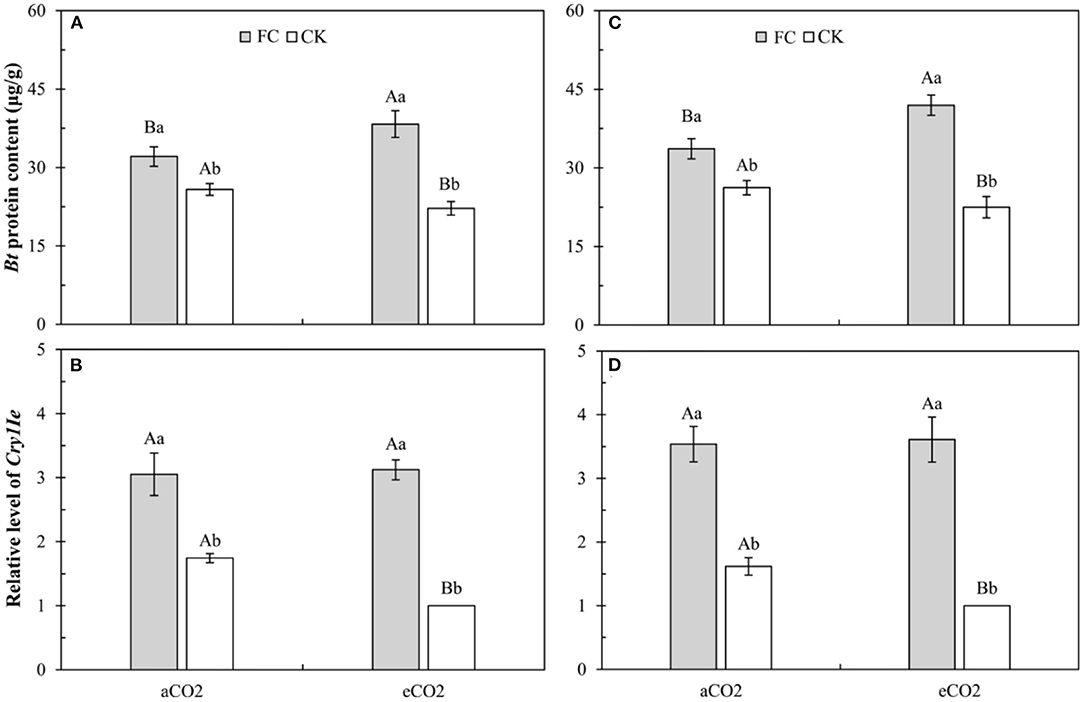
Figure 2. Effects of the inoculation with arbuscular mycorrhizal fungi (AMF), F. caledonium, on foliar Bt protein content and Bt gene relative expression level in leaves of Bt maize under ambient CO2 (aCO2) and elevated CO2 (eCO2) at the heading stage (BBCH-59) in 2017 (A,B) and 2018 (C,D). (Note: Different uppercase and lowercase letters indicate a significant difference between aCO2 and eCO2, and between F. caledonium inoculation and non-inoculation of F. caledonium under the same other conditions as revealed by Tukey's test) (P < 0.05; n = 15).
For the Bt gene relative expression level, compared with aCO2, eCO2 significantly decreased the Bt gene relative expression level in the leaves of Bt maize without F. caledonium inoculation (Figures 2B,D). Compared with control, AMF inoculation significantly increased the Bt gene relative expression level of Bt maize under aCO2 and eCO2 (P < 0.05; Figures 2B,D).
Foliar JA and SA Contents in Leaves of Bt and Non-Bt Maize Influenced by CO2 Levels and F. caledonium Inoculation
Four-way ANOVAs showed that AMF inoculation (F ≥ 216.16, P < 0.001) and CO2 level (F ≥ 99.02, P < 0.001) significantly affected the foliar JA and SA contents of Bt and non-Bt maize (Supplementary Table 4).
For the foliar JA content, compared with control, AMF inoculation significantly increased the foliar JA content of Bt and non-Bt maize in two sample years no matter under aCO2 or eCO2 (Figures 3A,C). Compared with aCO2, eCO2 significantly increased the foliar JA content of Bt and non-Bt maize without F. caledonium inoculation in 2017 and 2018, and Bt maize inoculated with F. caledonium in 2017 and non-Bt maize inoculated with F. caledonium in 2018 (P < 0.05; Figures 3A,C).
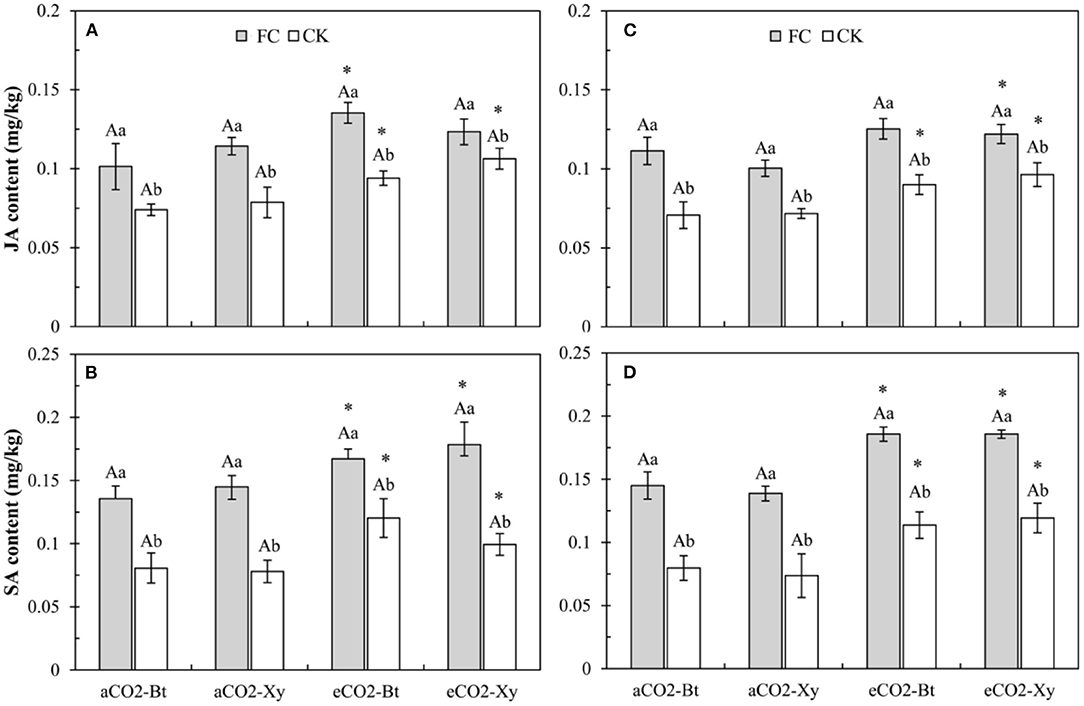
Figure 3. Effects of the inoculation with arbuscular mycorrhizal fungi (AMF), F. caledonium, on the foliar JA and SA contents of Bt maize (Bt) and its parental line of non-Bt maize (Xy) under ambient CO2 (aCO2) and elevated CO2 (eCO2) at the heading stage (BBCH-59) in 2017 (A,B) and 2018 (C,D). (Note: FC, F. caledonium inoculation; CK, the control without F. caledonium inoculation; aCO2-Bt, Bt maize under aCO2; aCO2-Xy, non-Bt maize under aCO2; eCO2-Bt, Bt maize under eCO2; eCO2-Xy, non-Bt maize under eCO2; Different uppercase, lowercase letters and * indicate significant difference between Bt maize and non-Bt maize, between F. caledonium inoculation and non-inoculation of F. caledonium, and between aCO2 and eCO2 under the same other conditions as revealed by Tukey's test, the same in the following figures) (P < 0.05; n = 15).
For the foliar SA content, compared with control, AMF inoculation significantly increased the foliar SA content of Bt and non-Bt maize in two sample years no matter under aCO2 or eCO2 (Figures 3B,D). Compared with aCO2, eCO2 significantly increased the foliar SA content of Bt and non-Bt maize in two sample years regardless of F. caledonium inoculation or not (Figures 3B,D).
Food Utilization of M. separata Larvae Fed on Bt and Non-Bt Maize Influenced by CO2 Levels and F. caledonium Inoculation
Four-way ANOVAs showed that AMF inoculation (F ≥ 4.20, P ≤ 0.048), CO2 level (F ≥ 4.24, P ≤ 0.047), transgenic treatment (F ≥ 36.98, P < 0.001), and their interactions (F ≥ 12.19, P < 0.0014) significantly affected all the food utilization indices of M. separata larvae, except for the interaction between CO2 level and AMF inoculation on RGR (F = 0.72, P = 0.40 > 0.05; Supplementary Table 5). Moreover, the interaction between CO2 level, AMF inoculation, and transgenic treatment also significantly affected the ECD (F = 32.63, P < 0.001) and ECI (F = 9.78, P = 0.004 < 0.01) of M. separata larvae (Supplementary Table 5).
Compared with non-Bt maize, the ECD of M. separata larvae fed on Bt maize inoculated with and without F. caledonium was significantly decreased in two sample years no matter under aCO2 or eCO2. Compared with control, AMF inoculation significantly increased the ECD of M. separata larvae fed on non-Bt maize under eCO2 in 2017 and 2018. Compared with aCO2, eCO2 significantly decreased the ECD of M. separata larvae fed on Bt maize inoculated with and without F. caledonium in two sample years, while significantly increased the ECD of M. separata larvae fed on non-Bt maize inoculated with F. caledonium in 2017 and 2018, and significantly decreased the ECD of M. separata larvae fed on non-Bt maize without F. caledonium inoculation in 2018 (P < 0.05; Figures 4A,E).
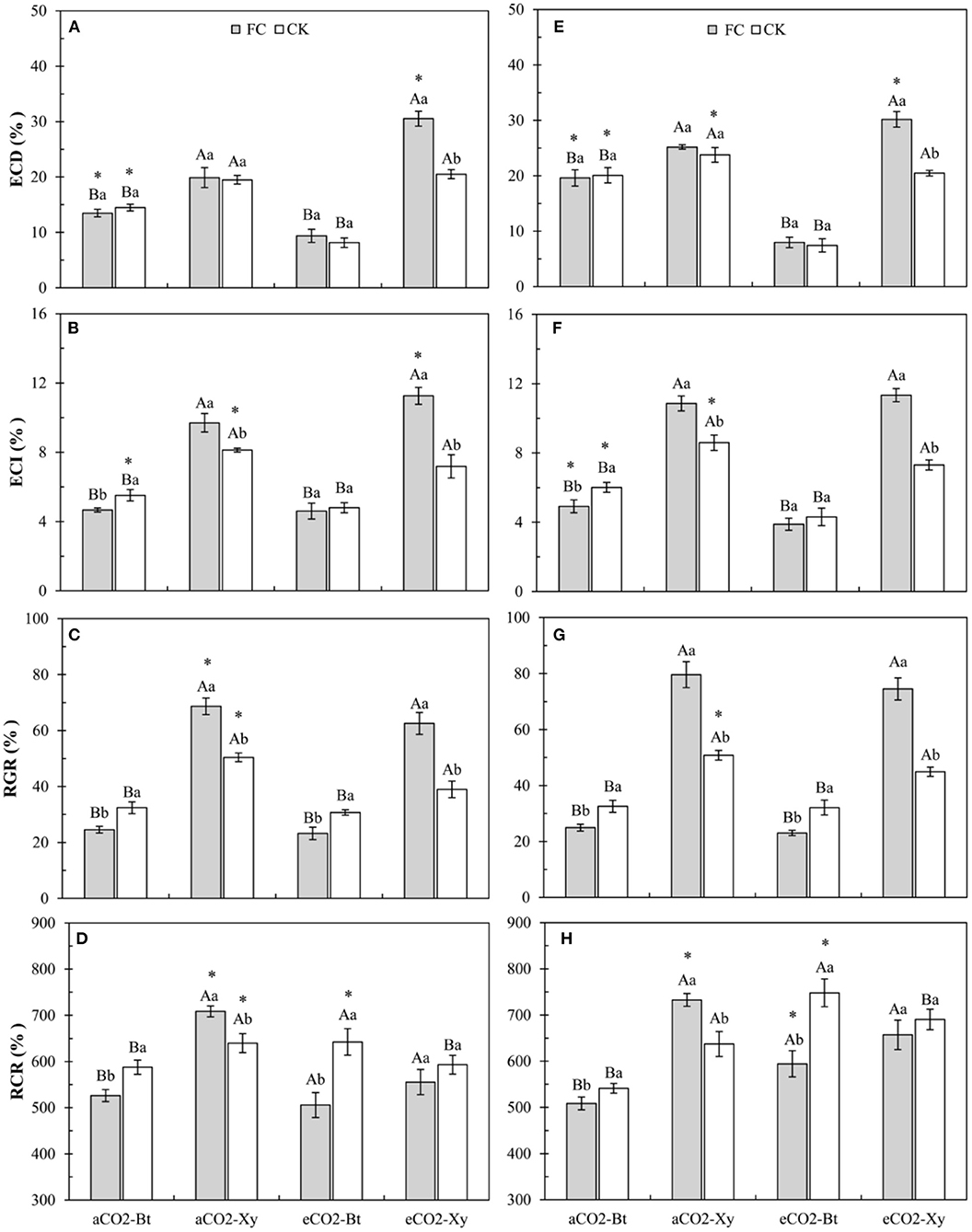
Figure 4. Effects of the inoculation with arbuscular mycorrhizal fungi (AMF), F. caledonium, on the food utilization of M. separata larvae fed on Bt maize (Bt) and its parental line of non-Bt maize (Xy) under ambient CO2 (aCO2) and elevated CO2 (eCO2) at the heading stage (BBCH-59) in 2017 (A–D) and 2018 (E–H). (Note: the same as Figure 3) (P < 0.05; n = 5).
Compared with non-Bt maize, the ECI of M. separata larvae fed on Bt maize inoculated with and without F. caledonium was significantly decreased in two sample years no matter under aCO2 or eCO2. Compared with control, AMF inoculation significantly increased the ECI of M. separata larvae fed on non-Bt maize under aCO2 and eCO2 in two sample years, while significantly decreased the ECI of M. separata larvae fed on Bt maize under aCO2 in 2017 and 2018. Compared with aCO2, eCO2 significantly decreased the ECI of M. separata larvae fed on Bt and non-Bt maize without F. caledonium inoculation in 2017 and 2018, while significantly increased the ECI of M. separata larvae fed on non-Bt maize with F. caledonium inoculation in 2017 and significantly decreased the ECI of M. separata larvae fed on Bt maize with F. caledonium inoculation in 2018 (P < 0.05; Figures 4B,F).
Compared with non-Bt maize, the RGR of M. separata larvae fed on Bt maize inoculated with and without F. caledonium was significantly decreased in two sample years no matter under aCO2 or eCO2. Compared with control, AMF inoculation significantly increased the RGR of M. separata larvae fed on non-Bt maize under aCO2 and eCO2 in 2017 and 2018, while significantly decreased the RGR of M. separata larvae fed on Bt maize under aCO2 and eCO2 in two sample years. Compared with aCO2, eCO2 significantly decreased the RGR of M. separata larvae fed on non-Bt maize without F. caledonium inoculation in 2017 and 2018, and that of M. separata larvae fed on non-Bt maize with F. caledonium inoculation in 2017 (P < 0.05; Figures 4C,G).
Compared with non-Bt maize, the RCR of M. separata larvae fed on Bt maize inoculated with and without F. caledonium was significantly decreased under aCO2 in 2017 and 2018, while the RCR of M. separata larvae fed on Bt maize without F. caledonium inoculation significantly increased under eCO2 in two sample years. Compared with control, AMF inoculation significantly decreased the RCR of M. separata larvae fed on Bt maize in two sample years no matter under aCO2 or eCO2, while significantly increased the RCR of M. separata larvae fed on non-Bt maize under aCO2 in 2017 and 2018. Compared with aCO2, eCO2 significantly decreased the RCR of M. separata larvae fed on non-Bt maize inoculated with F. caledonium in 2017 and 2018, while significantly increased the RCR of M. separata larvae fed on Bt maize without F. caledonium inoculation in two sample years (P < 0.05; Figures 4D,H).
Growth, Development, and Reproduction of M. separata Fed on Bt and Non-Bt Maize Influenced by CO2 Levels and F. caledonium Inoculation
Four-way ANOVAs showed that sampling year (F ≥ 7.97, P ≤ 0.008), CO2 level (F ≥ 24.20, P < 0.001), transgenic treatment (F ≥ 164.88, P < 0.001), and the interaction between transgenic treatment and AMF inoculation (F ≥ 4.23, P ≤ 0.047) significantly affected all measured indices of M. separata, except the interaction between transgenic treatment and AMF inoculation on pupation rate of M. separata (F = 0.23, P = 0.63; Supplementary Table 6). Moreover, AMF inoculation significantly affected pupal weight and pupal duration of M. separata (F ≥ 15.07, P < 0.001), the interaction between CO2 level and transgenic treatment significantly affected larval lifespan of M. separata (F = 89.64, P < 0.001), and the interactions between CO2 level and AMF inoculation affected fecundity of M. separata (F = 12.65, P = 0.001) (Supplementary Table 6).
Compared with non-Bt maize, the larval lifespan of M. separata larvae fed on Bt maize inoculated with and without F. caledonium was significantly extended in two sample years no matter under aCO2 or eCO2. Compared with control, AMF inoculation significantly shortened the larval lifespan of M. separata fed on non-Bt maize under aCO2 and eCO2 in 2017 and 2018, while significantly prolonged the larval lifespan of M. separata fed on Bt maize under aCO2 and eCO2 in 2017 and 2018. Compared with aCO2, eCO2 significantly extended the larval lifespan of M. separata larvae fed on Bt maize inoculated with and without F. caledonium in two sample years (P < 0.05; Figures 5A,E).
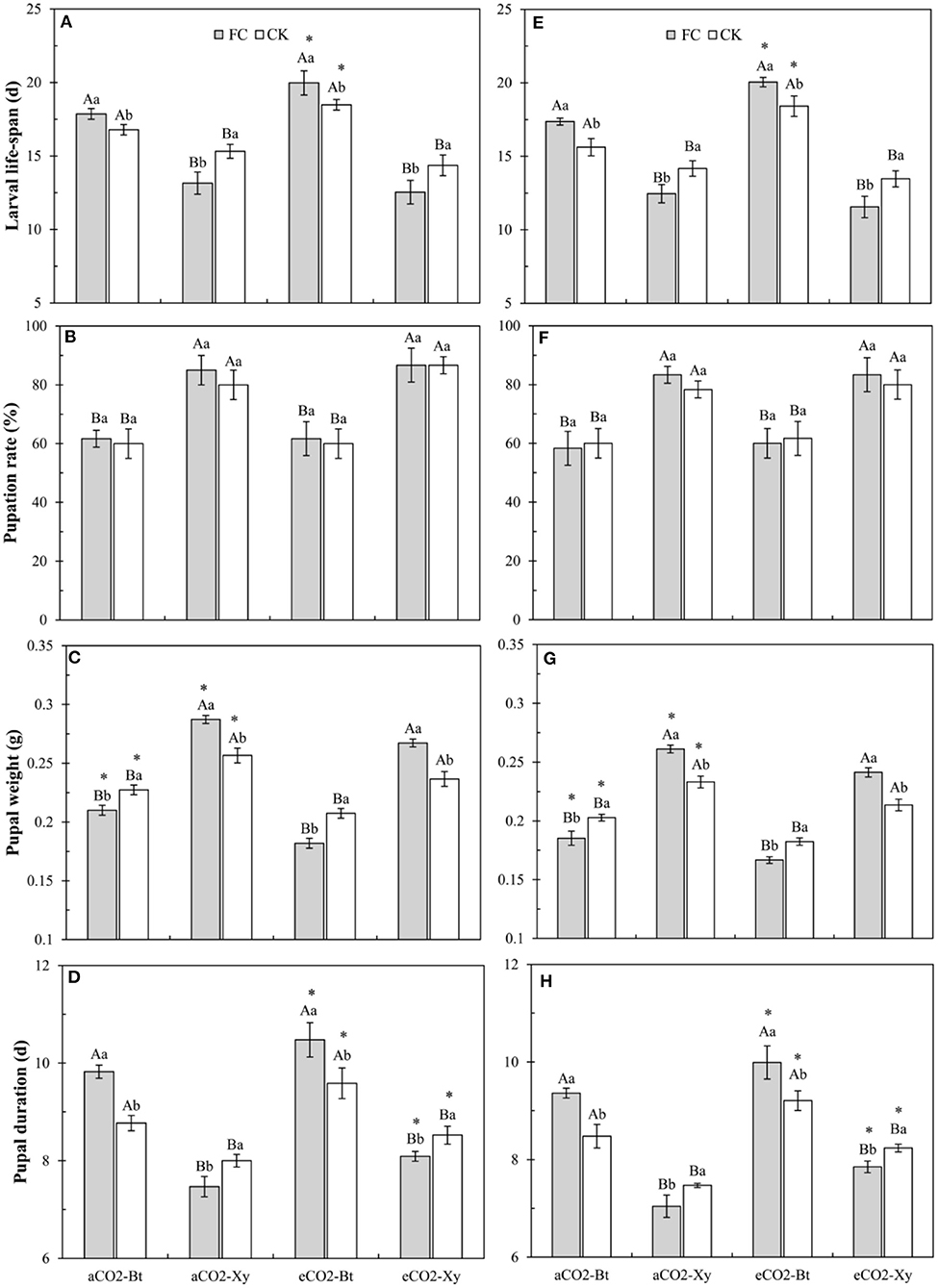
Figure 5. Effects of the inoculation with arbuscular mycorrhizal fungi (AMF), F. caledonium, on the growth and development of M. separata larvae fed on Bt maize (Bt) and its parental line of non-Bt maize (Xy) under ambient CO2 (aCO2) and elevated CO2 (eCO2) at the heading stage (BBCH-59) in 2017 (A–D) and 2018 (E–H). (Note: the same as Figure 3) (P < 0.05; n = 5).
Compared with non-Bt maize, the pupation rate of M. separata fed on Bt maize inoculated with and without F. caledonium was significantly decreased under aCO2 in two sample years no matter under aCO2 or eCO2 (P < 0.05; Figures 5B,F).
Compared with non-Bt maize, the pupal weight of M. separata fed on Bt maize inoculated with and without F. caledonium was significantly decreased in two sample years no matter under aCO2 or eCO2. Compared with control, AMF inoculation significantly increased the pupal weight of M. separata fed on non-Bt maize under aCO2 and eCO2 in 2017 and 2018, while significantly decreased the pupal weight of M. separata fed on Bt maize under aCO2 and eCO2 in two sample years. Compared with aCO2, eCO2 significantly decreased the pupal weight of M. separata fed on Bt and non-Bt maize in two sample years regardless of F. caledonium inoculation or not (P < 0.05; Figures 5C,G), respectively.
Compared with non-Bt maize, the pupal duration of M. separata fed on Bt maize inoculated with and without F. caledonium was significantly extended in two sample years no matter under aCO2 or eCO2. Compared with control, AMF inoculation significantly shortened the pupal duration of M. separata fed on non-Bt maize under aCO2 and eCO2 in 2017 and 2018, while significantly extended the pupal duration of M. separata fed on Bt maize under aCO2 and eCO2 in two sample years. Compared with aCO2, eCO2 significantly extended the pupal duration of M. separata fed on Bt and non-Bt maize in two sample years regardless of F. caledonium inoculation or not (P < 0.05; Figures 5D,H).
Compared with non-Bt maize, the eclosion rate of M. separata fed on Bt maize inoculated with and without F. caledonium was significantly decreased in two sample years no matter under aCO2 or eCO2 (P < 0.05; Figures 6A,D).
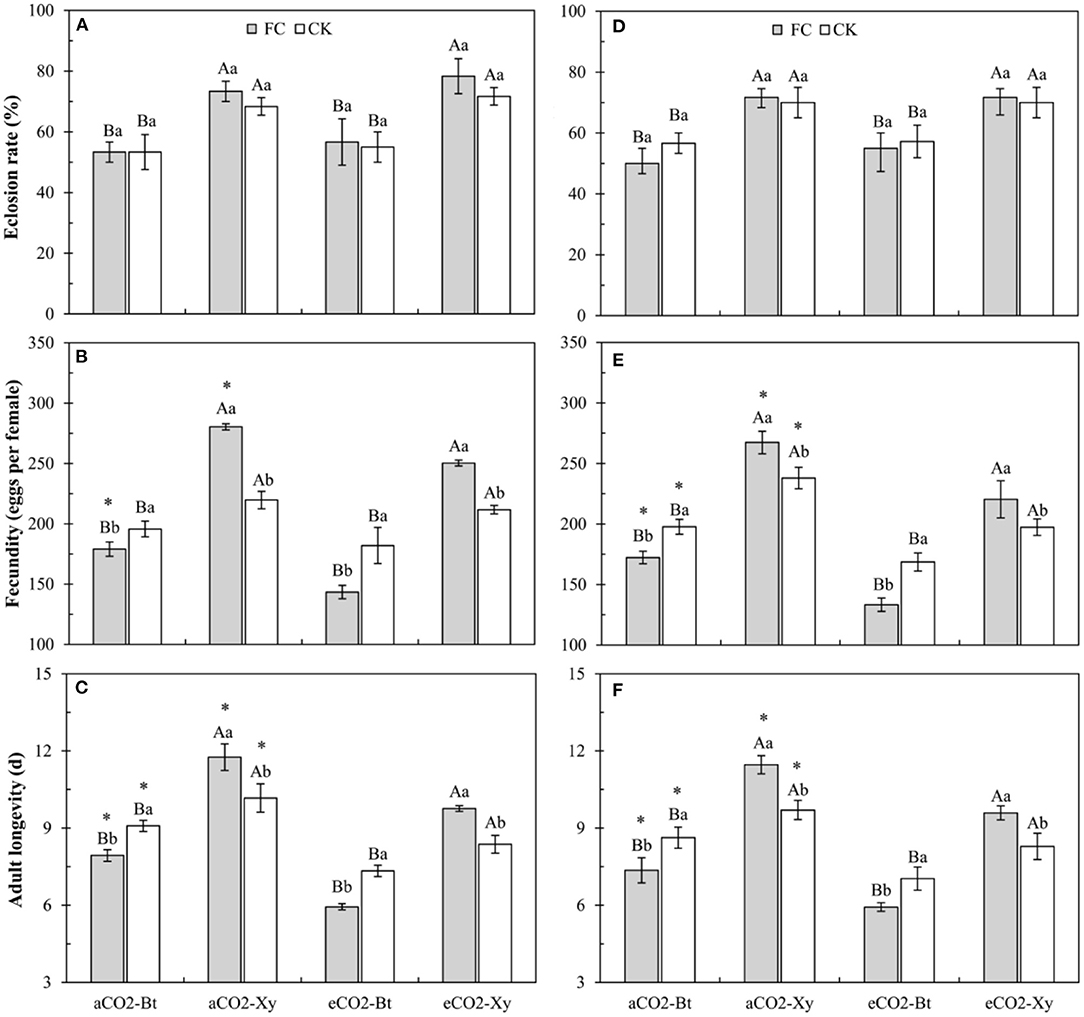
Figure 6. Effects of the inoculation with arbuscular mycorrhizal fungi (AMF), F. caledonium, on the growth and development of M. separata adults fed on Bt maize (Bt) and its parental line of non-Bt maize (Xy) under ambient CO2 (aCO2) and elevated CO2 (eCO2) at the heading stage (BBCH-59) in 2017 (A–C) and 2018 (D–F). (Note: the same as Figure 3) (P < 0.05; n = 5).
Compared with non-Bt maize, the fecundity of M. separata fed on Bt maize inoculated with and without F. caledonium was significantly decreased in two sample years no matter under aCO2 or eCO2. Compared with control, AMF inoculation significantly increased the fecundity of M. separata fed on non-Bt maize under aCO2 and eCO2 in 2017 and 2018, while significantly decreased the fecundity of M. separata fed on Bt maize under aCO2 and eCO2 in two sample years. Compared with aCO2, eCO2 significantly decreased the fecundity of M. separata fed on Bt and non-Bt maize in two sample years regardless of F. caledonium inoculation or not (P < 0.05; Figures 6B,E).
Compared with non-Bt maize, the adult longevity of M. separata fed on Bt maize inoculated with and without F. caledonium was significantly shortened in two sample years no matter under aCO2 or eCO2. Compared with control, AMF inoculation significantly extended the adult longevity of M. separata fed on non-Bt maize under aCO2 and eCO2 in 2017 and 2018, while significantly shortened the adult longevity of M. separata fed on Bt maize under aCO2 and eCO2 in two sample years. Compared with aCO2, eCO2 significantly shortened the adult longevity of M. separata fed on Bt and non-Bt maize in two sample years regardless of F. caledonium inoculation or not (P < 0.05; Figures 6C,F).
Yield of Bt and Non-Bt Maize Influenced by CO2 Levels and F. caledonium Inoculation
Four-way ANOVAs showed that AMF inoculation significantly affected all indices of maize yield (F ≥ 10.23, P ≤ 0.003), CO2 level significantly affected ear weight per plant and grain weight per ear (CO2 level: F ≥ 45.24, P < 0.001), transgenic treatment significantly affected ear weight per plant (F = 10.89, P = 0.002), and the interaction between CO2 level and AMF inoculation significantly affected the grain weight per ear (F = 4.48, P = 0.042 < 0.05) (Supplementary Table 3).
Compared with non-Bt maize, the dry ear weight per plant of Bt maize without F. caledonium inoculation was significantly enhanced under eCO2 in 2017, and that with F. caledonium inoculation was significantly increased under aCO2 in 2018. Compared with control, AMF inoculation significantly increased the dry ear weight per plant of Bt and non-Bt maize in two sample years no matter under aCO2 or eCO2. Compared with aCO2, eCO2 significantly increased the dry ear weight per plant of Bt and non-Bt maize in two sample years regardless of F. caledonium inoculation or not (P < 0.05; Figures 7A,D).
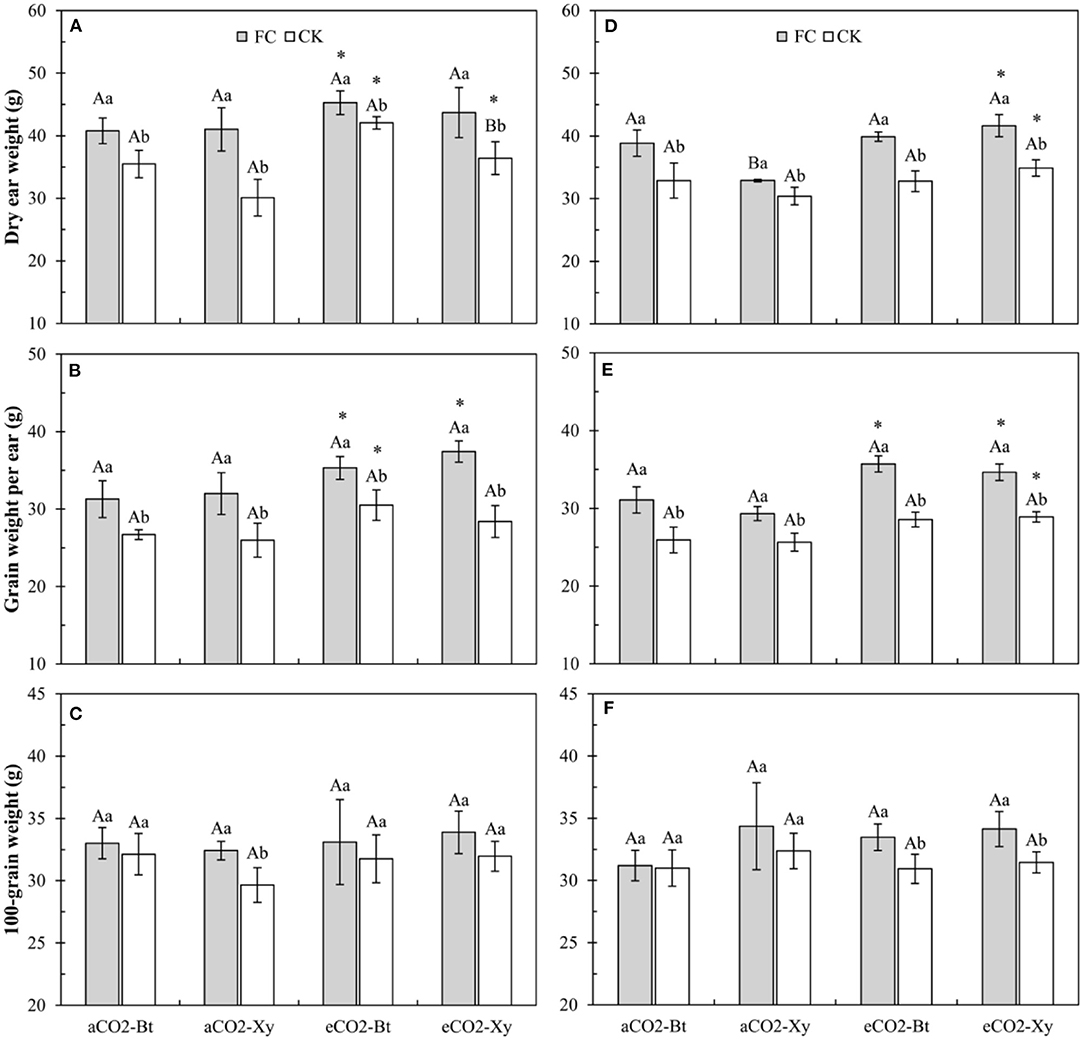
Figure 7. Effects of the inoculation with arbuscular mycorrhizal fungi (AMF), F. caledonium, on the yield of Bt maize (Bt) and its parental line of non-Bt maize (Xy) under ambient CO2 (aCO2) and elevated CO2 (eCO2) in 2017 (A–C) and 2018 (D–F). (Note: the same as Figure 3) (P < 0.05; n = 15).
Compared with control, AMF inoculation significantly increased the grain weight per ear of Bt and non-Bt maize in two sample years no matter under aCO2 or eCO2. Compared with aCO2, eCO2 significantly increased the grain weight per ear of Bt and non-Bt maize inoculated with F. caledonium in two sample years, and significantly increased that of Bt maize without F. caledonium inoculation in 2017 and that of non-Bt maize without F. caledonium inoculation in 2018 (P < 0.05; Figures 7B,E). Compared with control, AMF inoculation significantly increased the 100-grain weight of non-Bt maize under aCO2 in 2017, and that of Bt and non-Bt maize under eCO2 in 2018 (P < 0.05; Figures 7C,F).
Discussion
The values of AMF colonization and AMF-PLFA content indicate the colonization efficiency of AMF on maize. In this experiment, F. caledonium inoculation significantly increased the AMF colonization and AMF-PLFA content no matter what maize variety or CO2 level, and this ensured the validity of the following research work. Meanwhile, elevated CO2 can increase photosynthesis of plants, improve plant growth, and promote the transfer of carbon source substances from host plants to the root-symbiotic AMF, which is beneficial for AMF colonization and growth (Diaz et al., 1993; Cheng et al., 2012). The colonization was increased by ~10% in Medicago truncatula and by as much as 50% in Brachypodium distachyon (Jakobsen et al., 2016). Treseder (2004) also reported that the AMF colonization reflected an increased colonization speed under eCO2. Alberton et al. (2005) found that mycorrhizal fungi and mycorrhizal plants to elevated CO2 were significantly positive, and the response ratio for AM fungi was 1.21 (an increase of 21%), indicating a significantly different response, and AM colonization percentage also had a certain degree of improvement. In this study, the AMF colonization and the AMF-PLFA content of Bt and non-Bt maize under eCO2 were generally higher than those under aCO2; therefore, it is presumed that elevated CO2 did have a positive facilitation on the colonization of F. caledonium on maize (Diaz et al., 1993; Drigo et al., 2013; Becklin et al., 2016). Besides, there were no differences in the AMF colonization and AMF-PLFA content between Bt maize and its parental line of non-Bt maize; the results showed that the presence of the cry1Ie protein in maize did not affect the colonization of the AMF community, and it is consistent with the research report of Cheeke et al.; Cheeke et al. (2014; 2015; Zeng et al., 2018).
Overall, the plant biomass and grain yield increased with the increasing level (200–400 ppm) of atmospheric CO2 for most crops (Chen M. et al., 2011; Wang et al., 2018). In this study, the results showed that eCO2 significantly increased maize ear weight per plant and grain weight per ear, while it did not significantly affect the 100-grain weight. It is mainly because eCO2 can enhance photosynthesis and in turn, has a positive effect on crop biomass and production (Guo et al., 2016; Liu et al., 2019). Although the maize yield was improved, the comprehensive nutritional quality of maize grain (100-grain weight) could not be improved; it may be due to the decreased nitrogen content at high CO2 levels. Moreover, F. caledonium inoculation significantly increased all the yield indices (ear weight per plant, grain weight per ear, and 100-grain weight). The main function of AMF is to enhance the uptake of nutrient elements (e.g., N, P, K, Ca, Mg, Zn, and Fe) by host plants, improve the nutrient metabolism capacity and nutrient level of plant tissues, and then promote the growth and fruiting of plants (Sharifi et al., 2007; Terrer et al., 2016; Turrini et al., 2017). In addition, under the combined effects of eCO2 and F. caledonium inoculation, the ear weight per plant and grain weight per ear of Bt and non-Bt maize showed a further significant increase, which benefit from the improvement of AMF colonization under eCO2.
Insects are sensitive to environmental variations, and environmental stresses can cause changes in their growth, development, fecundity, food utilization, and the occurrence and distribution of populations as a result of metabolic rate fluctuation (Bloom et al., 2010). Usually, endogenously secondary defensive chemicals (e.g., JA and SA) and nutrient components (e.g., C, N, P, and K) are the two main factors that affect the population fitness of pests; the balance between secondary defensive chemicals and nutrient components determines the development trend of pests after feeding. If the pests feed on transgenic Bt plants, in addition to the above-mentioned two influencing factors, the Bt toxin protein will also have a significant adverse effect on the growth and food utilization of pests and occupy the dominant position among the three. Prutz and Dettner (2005) reported that the transgenic Bt maize decreased the growth rate and increased the mortality of Chilo partellus, which might be attributed to the termination of larval metamorphosis fed on Bt maize. Most studies showed adverse effects of Cry proteins on lifetable parameters of different herbivores (Lawo et al., 2010), which might be due to the interaction of feeding inhibitors and growth inhibitors. In this study, we found that Bt maize obviously decreased almost all measured indices of food utilization (ECD, ECI, and RGR), and it showed that the ability of food digestion and absorption of pests has caused serious damage by Bt toxin. Meanwhile, lifetable parameters (growth, development, and reproduction of M. separata larvae; pupation rate, pupal weight, eclosion rate, and fecundity of M. separata adult) markedly decreased feeding on Bt maize, and Bt toxin also prolonged the larval lifespan and shortened the adult longevity of M. separata regardless of the CO2 level and F. caledonium inoculation or not in 2017 and 2018. These results showed that Bt maize obviously retarded the growth and development of M. separata and were similar to those of previous studies.
Previous studies have examined that in most plants, elevated CO2 tends to promote plant photosynthesis and also leads to a decrease in foliar nitrogen content and an increase in C: N ratio (Johns et al., 2003; Li et al., 2018). Meanwhile, nitrogen is the main component of exogenous Bt protein in Bt crops. Plant nitrogen uptake, nitrogen-level status, and C: N ratio could affect the production of exogenous Bt toxins for Bt crops (Gao et al., 2009; Jiang et al., 2013). Numerous studies have shown that eCO2 can significantly reduce the exogenous Bt protein content of Bt cotton and Bt rice, while increasing their yield (Coviella et al., 2000, 2002; Chen et al., 2005b; Wu et al., 2011a,b), and also found the “dilution effect” on exogenous Bt protein or inhibition on Bt-transgene expression (Chen F. J. et al., 2011; Jiang et al., 2017; Liu et al., 2019). Moreover, elevated CO2 also affected the production of primary and secondary metabolites, and the defense mechanisms of crop plants (e.g., JA and SA) (Stiling and Cornelissen, 2007; Sun et al., 2016). In this study, eCO2 significantly decreased the ECI and RGR of M. separata larvae fed on non-Bt maize without F. caledonium inoculation, and was almost adverse to all the measured indices of growth and development of M. separata; it is mainly due to the increased secondary defense substances (i.e., JA and SA) in plants and the declined food nutrient level (e.g., fewer N) under elevated CO2 (Armstrong et al., 1995; Coviella et al., 2002; Liu et al., 2019). Many studies found that elevated CO2 had adverse effects on the developmental duration, pupation, and eclosion of cotton bollworm, H. armigera (Akbar et al., 2016), and reduced the oviposition number of borers and semilooper (Stange, 1997; Rao et al., 2013). On the other hand, elevated CO2 decreased the RGR of the Spodoptera litura and H. armigera (Hattenschwiler and Schafellner, 2004), and also significantly reduced the ECD and ECI of H. armigera (Yin et al., 2010). Meanwhile, eCO2 also significantly reduced the ECD, ECI, pupal weight, fecundity, and adult longevity, and significantly extended the development duration of M. separata fed on Bt maize with and without F. caledonium inoculation. This shows that despite the decrease in exogenous Bt toxin content, the increase in secondary defense substances and the decline in nutrient quality can also make a relatively adverse effect on M. separata.
The effect of F. caledonium inoculation on M. separata fed on Bt maize and non-Bt maize was just the opposite; that is, F. caledonium inoculation significantly reduced the ECI, RGR, and RCR of M. separata larvae fed on Bt maize under aCO2 and eCO2, while significantly increased the ECI and RGR of M. separata larvae fed non-Bt maize under aCO2 and eCO2, and RCR of M. separata larvae fed on non-Bt maize under aCO2, and ECD of M. separata larvae fed on non-Bt maize under eCO2. In addition, F. caledonium inoculation and transgenic treatment had a significant interaction on the growth, development, and reproduction of M. separata. Specifically, as fed on Bt maize, F. caledonium inoculation significantly extended the developmental duration of larvae and pupae of M. separata under aCO2 and eCO2, significantly reduced the pupal weight and fecundity, and significantly shortened the adult longevity of M. separata. On the contrary, as fed on non-Bt maize, F. caledonium inoculation significantly shortened the developmental duration of larvae and pupae of M. separata under aCO2 and eCO2, and significantly increased the pupal weight of M. separata under aCO2 and eCO2. Thus, it was observed that F. caledonium inoculation had diametrically opposite effects on the growth, development, and food utilization of M. separata fed on Bt maize and non-Bt maize; that is, F. caledonium inoculation reduced the food utilization efficiency and had adverse effects on the growth, development, and reproduction of M. separata fed on Bt maize, while it improved the food utilization efficiency and had positive effects on the growth, development, and reproduction of M. separata fed on non-Bt maize. This is mainly due to the promotion of the absorption and utilization of soil nutrients in maize plants by F. caledonium inoculation, thus improving the nutrient level of maize plant tissues (Sharifi et al., 2007; Rodriguez and Sanders, 2015). So it is concluded that M. separata larvae can get more plant nutrition when fed on non-Bt maize inoculated with F. caledonium. When fed on Bt maize inoculated with F. caledonium, M. separata larvae not only obtained more plant nutrients, but also ingested more doses of exogenous Bt toxin, which improved the target resistance ability of Bt crops based on the exogenous Bt toxin, and further reduced the food utilization efficiency, growth, development, and reproduction of M. separata, and at the same time, the promotion of AMF colonization by eCO2 further enhanced the target resistance level of Bt maize to M. separata.
Overall, our research showed that eCO2 was beneficial for AMF colonization on roots, and maize yield, but it had negative effects on the growth, development, reproduction, and food utilization of M. separata. F. caledonium inoculation was positive for maize yield and nutrient quality, and in favor of the growth, development, reproduction, and food utilization of the M. separata fed on non-Bt maize. However, F. caledonium inoculation was unfavorable for the population fitness of M. separata fed on Bt maize. Namely, regardless of the CO2 level, inoculation of F. caledonium had a detrimental effect on the production of non-Bt maize as the result of a high potential risk of M. separata production hazard, while its effects on Bt maize were just the opposite; that is, F. caledonium inoculation had positive effects on the production of Bt maize especially under eCO2 due to the lower potential risk of population occurrence of M. separata. Ultimately, the results proved that all of our hypotheses were confirmed: It showed that the AMF inoculation of F. caledonium under eCO2 was more effective in improving the control efficiency of Bt maize on the target insect pest, M. separata, promoted the expression of endogenous (JA, SA) and exogenous (Bt toxin) secondary defense substances in Bt maize leaves, and also increased the biomass and yield of maize (Figure 8). We have reason to expect this friendly and effective biological way serving for mitigating the ecological risk of Bt maize and improving its ecological sustainable utilization capacity under global climate change.
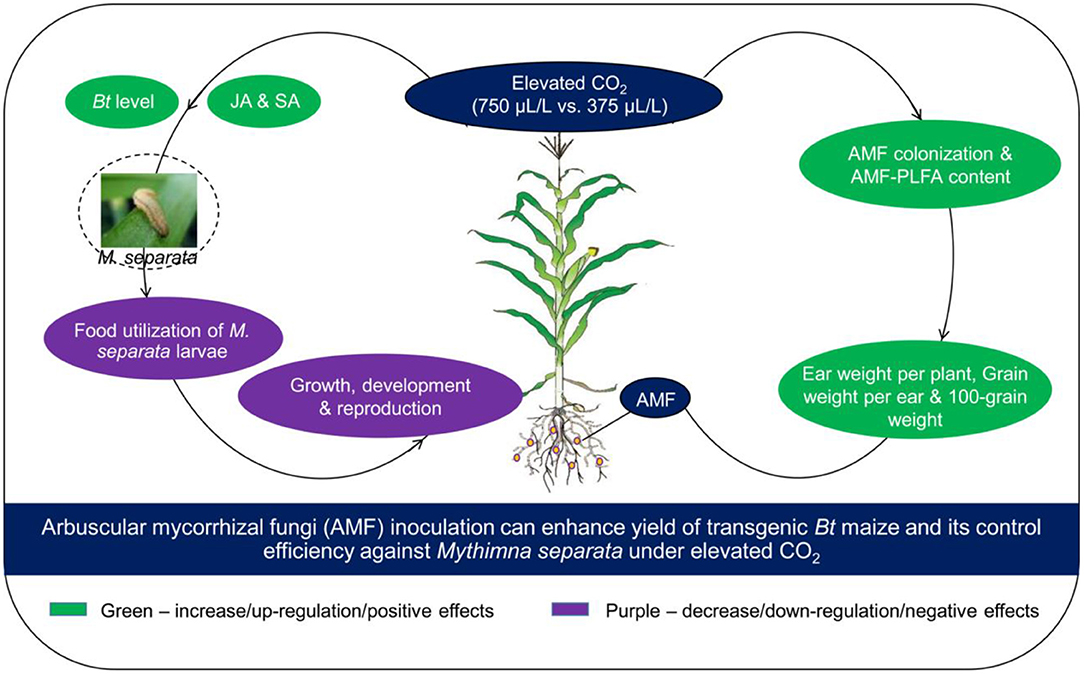
Figure 8. A schematic model that AMF inoculation can enhance the yield of transgenic Bt maize and its control efficiency against M. separata under elevated CO2.
Data Availability Statement
The original contributions presented in the study are included in the article/Supplementary Materials, further inquiries can be directed to the corresponding author/s.
Author Contributions
LW and FC conceived research. LW, XW, and TH conducted the experiments. LW, FG, CL, and LL analyzed data and conducted statistical analysis. LW and FC wrote the manuscript. FC secured funding. All authors read and approved the manuscript.
Funding
This study was supported by the National Nature Science Foundation of China (NSFC) (31871963), the National Key Research and Development Program of China (2017YFD0200400), the Fundamental Research Funds for the Central Universities (KYZ201818), the Qing-Lan Project of Jiangsu Province of China, and the Postgraduate Research and Practice Innovation Program of Jiangsu Province (KYCX17_0574).
Conflict of Interest
The authors declare that the research was conducted in the absence of any commercial or financial relationships that could be construed as a potential conflict of interest.
Supplementary Material
The Supplementary Material for this article can be found online at: https://www.frontiersin.org/articles/10.3389/fpls.2021.655060/full#supplementary-material
References
Akbar, S. M., Pavani, T., Nagaraja, T., and Sharma, H. C. (2016). Influence of CO2 and temperature on metabolism and development of Helicoverpa armigera (Noctuidae: Lepidoptera). Environ. Entomol. 45, 229–236. doi: 10.1093/ee/nvv144
Alberton, O., Kuyper, T. W., and Gorissen, A. (2005). Taking mycocentrism seriously: mycorrhizal fungal and plant responses to elevated CO2. New Phytol. 167, 859–868. doi: 10.1111/j.1469-8137.2005.01458.x
Armstrong, C. L., Parker, G. B., and Pershing, J. C. (1995). Field evaluation of European corn borer control in progeny of 173 transgenic corn events expressing an insecticidal protein from Bacillus thuringiensis. Crop Sci. 35, 550–557. doi: 10.2135/cropsci1995.0011183X003500020045x
Becklin, K. M., Mullinix, G. W. R., and Ward, J. K. (2016). Host plant physiology and mycorrhizal functioning shift across a glacial through future CO2 gradient. Plant Physiol. 172, 789–801. doi: 10.1104/pp.16.00837
Bloom, A. J., Burger, M., Asensio, J. S. R., and Cousins, A. B. (2010). Carbon dioxide enrichment inhibits nitrate assimilation in wheat and Arabidopsis. Science 328, 899–903. doi: 10.1126/science.1186440
Bossio, D. A., and Scow, K. M. (1998). Impacts of carbon and flooding on soil microbial communities: phospholipid fatty acid profiles and substrate utilization patterns. Microb. Ecol. 35, 265–278. doi: 10.1007/s002489900082
Chang, X., Liu, G. G., He, K. L., Shen, Z. C., Peng, Y. F., and Ye, G. Y. (2013). Efficacy evaluation of two transgenic maize events expressing fused proteins to CrylAb-susceptible and -resistant Ostrinia furnacalis (Lepidoptera: Crambidae). J. Econ. Entomol. 106, 2548–2556. doi: 10.1603/EC13100
Cheeke, T. E., Darby, H., Rosenstiel, T. N., Bever, J. D., and Cruzan, M. B. (2014). Effect of Bacillus thuringiensis (Bt) maize cultivation history on arbuscular mycorrhizal fungal colonization, spore abundance and diversity, and plant growth. Agric. Ecosyst. Environ. 195, 29–35. doi: 10.1016/j.agee.2014.05.019
Cheeke, T. E., Schütte, U. M., Hemmerich, C. M., Cruzan, M. B., Rosenstiel, T. N., and Bever, J. D. (2015). Spatial soil heterogeneity has a greater effect on symbiotic arbuscular mycorrhizal fungal communities and plant growth than genetic modification with Bacillus thuringiensis toxin genes. Mol. Ecol. 24, 2580–2593. doi: 10.1111/mec.13178
Chen, F. J., Ge, F., and Parajulee, M. N. (2005a). Impact of elevated CO2 on tri-trophic interaction of Gossypium hirsutum, Aphis gossypii, and Leis axyridis. Environ. Entomol. 34, 37–46. doi: 10.1603/0046-225X-34.1.37
Chen, F. J., Wu, G., Ge, F., and Parajulee, M. N. (2011). Relationships between exogenous-toxin quantity and increased biomass of transgenic Bt crops under elevated carbon dioxide. Ecotoxicol. Environ. Saf. 74, 1074–1080. doi: 10.1016/j.ecoenv.2011.02.001
Chen, F. J., Wu, G., Ge, F., Parajulee, M. N., and Shrestha, R. B. (2005b). Effects of elevated CO2 and transgenic Bt cotton on plant chemistry, performance, and feeding of an insect herbivore, the cotton bollworm. Entomol. Exp. Appl. 115, 341–350. doi: 10.1111/j.1570-7458.2005.00258.x
Chen, M., Shelton, A., and Ye, G. Y. (2011). Insect-resistant genetically modified rice in China: from research to commercialization. Annu. Rev. Entomol. 56, 81–101. doi: 10.1146/annurev-ento-120709-144810
Cheng, L., Booker, F. L., Tu, C., Burkey, K. O., Zhou, L. S., Shew, H. D., et al. (2012). Arbuscular mycorrhizal fungi increase organic carbon decomposition under elevated CO2. Science 337, 1084–1087. doi: 10.1126/science.1224304
Coviella, C. E., Morgan, D. J. W., and Trumble, J. T. (2000). Interactions of elevated CO2 and nitrogen fertilization: effects on production of Bacillus thuringiensis toxins in transgenic plants. Environ. Entomol. 29, 781–787. doi: 10.1603/0046-225x-29.4.781
Coviella, C. E., Stipanovic, R. D., and Trumble, J. T. (2002). Plant allocation to defensive compounds: interactions between elevated CO2 and nitrogen in transgenic cotton plants. J. Exp. Bot. 53, 323–331. doi: 10.1093/jexbot/53.367.323
Dai, Y., Wang, M. F., Jiang, S. L., Zhang, Y. F., Parajulee, M. N., and Chen, F. J. (2018). Host-selection behavior and physiological mechanisms of the cotton aphid, Aphis gossypii, in response to rising atmospheric carbon dioxide levels. J. Insect Physiol. 109, 149–156. doi: 10.1016/j.jinsphys.2018.05.011
Diaz, S., Grime, J. P., Harris, J., and McPherson, E. (1993). Evidence of a feedback mechanism limiting plant response to elevated carbon dioxide. Nature 354, 616–617. doi: 10.1038/364616a0
Dietterich, L. H., Zanobetti, A., Kloog, I., Huybers, P., Leakey, A. D., Bloom, A. J., et al. (2015). Impacts of elevated atmospheric CO2 on nutrient content of important food crops. Sci. Data 2:150036. doi: 10.1038/sdata.2015.36
Drigo, B., Kowalchuk, G. A., Knapp, B. A., Pijl, A. S., Boschker, H. T. S., and Veen, J. A. (2013). Impacts of 3 years of elevated atmospheric CO2 on rhizosphere carbon flow and microbial community dynamics. GCB Bioenergy 19, 621–636. doi: 10.1111/gcb.12045
Frew, A., and Price, J. N. (2019). Mycorrhizal-mediated plant-herbivore interactions in a high CO2 world. Funct. Ecol. 33, 1376–1385. doi: 10.1111/1365-2435.13347
Gao, H. J., Xiao, N. W., Li, J. S., Chen, F. J., and Zhai, B. P. (2009). Effects of double atmospheric CO2 concentration on nitrogen metabolism of transgenic Bt cotton under different nitrogen fertilization levels. Chinese J. Ecol. 28, 2213–2219.
Guo, H., Huang, L., Sun, Y., and Gou, H. G. (2016). The contrasting effects of elevated CO2 on TYLCV infection of tomato genotypes with and without the resistance gene, Mi-1.2. Front. Recent Dev. Plant Sci. 7:1680. doi: 10.3389/fpls.2016.01680
Guo, H. J., Sun, Y. C., Li, Y. F., Liu, X. H., Wang, P. Y., Zhu-Salzman, K., et al. (2014). Elevated CO2 alters the feeding behaviour of the pea aphid by modifying the physical and chemical resistance of Medicago truncatula. Plant Cell Environ. 37, 2158–2168. doi: 10.1111/pce.12306
Hattenschwiler, S., and Schafellner, C. (2004). Gypsy moth feeding in the canopy of a CO2-enriched mature forest. GCB Bioenergy 10, 1899–1908. doi: 10.1111/j.1365-2486.2004.00856.x
Hawkins, H. J., Johansen, A., and George, E. (2000). Uptake and transport of organic and inorganic nitrogen by arbuscular mycorrhizal fungi. Plant Soil 226, 275–285. doi: 10.1023/A:1026500810385
Helber, N., Wippel, K., Sauer, N., Schaarschmidt, S., Hause, B., and Requena, N. (2011). A versatile monosaccharide transporter that operates in the arbuscular mycorrhizal fungus Glomus sp is crucial for the symbiotic relationship with plants. Plant Cell 23, 3812–3823. doi: 10.1105/tpc.111.089813
Jakobsen, I., Smith, S. E., Smith, F. A., Watts-Williams, S. J., Clausen, S. S., and Gronlund, M. (2016). Plant growth responses to elevated atmospheric CO2 are increased by phosphorus sufficiency but not by arbuscular mycorrhizas. J. Exp. Bot. 67, 6173–6186. doi: 10.1093/jxb/erw383
Jiang, S. L., Lu, Y. Q., Dai, Y., Qian, L., Muhuammd, A. B., Li, T., et al. (2017). Impacts of elevated CO2 on exogenous Bacillus thuringiensis toxins and transgene expression in transgenic rice under different levels of nitrogen. Sci. Rep. 7:14716. doi: 10.1038/s41598-017-15321-9
Jiang, Y., Huang, S., Cai, M., Li, C., Kong, X., Zhang, F., et al. (2013). Yield changes of Bt-MH63 with Cry1C* or Cry2A* genes compared with MH63 (Oryza sativa) under different nitrogen levels. Field Crop Res. 151, 101–106. doi: 10.1016/j.fcr.2013.06.017
Johns, C. V., Beaumont, L. J., and Hughes, L. (2003). Effects of elevated CO2 and temperature on development and consumption rates of Octotoma championi and O. scabripennis feeding on Lantana camara. Entomol. Exp. Appl. 108, 169–178. doi: 10.1046/j.1570-7458.2003.00076.x
Johnson, S. N., and Hartley, S. E. (2018). Elevated carbon dioxide and warming impact silicon and phenolic-based defences differently in native and exotic grasses. Glob. Change Biol. 24, 3886–3896. doi: 10.1111/gcb.13971
Jung, S. C., Martinez-Medina, A., Lopez-Raez, J. A., and Pozo, M. J. (2012). Mycorrhiza-induced resistance and priming of plant defenses. J. Chem. Ecol. 38, 651–664. doi: 10.1007/s10886-012-0134-6
Keymer, D. P., and Lankau, R. A. (2017). Disruption of plant-soil-microbial relationships influences plant growth. J. Ecol. 105, 816–827. doi: 10.1111/1365-2745.12716
Kretzschmar, F. D. S., Aidar, M. P. M., Salgado, I., and Braga, M. R. (2009). Elevated CO2 atmosphere enhances production of defense-related flavonoids in soybean elicited by no and a fungal elicitor. Environ. Exp. Bot. 65, 319–329. doi: 10.1016/j.envexpbot.2008.10.001
Lawo, N. C., Wäckers, F. L., and Romeis, J. (2010). Characterizing indirect prey-quality mediated effects of a Bt crop on predatory larvae of the green lacewing, Chrysoperla carnea. J. Insect Physiol. 56, 1702–1710. doi: 10.1016/j.jinsphys.2010.06.012
Li, Z., Parajulee, M. N., and Chen, F. J. (2018). Influence of elevated CO2 on development and food utilization of armyworm Mythimna separata fed on transgenic Bt maize infected by nitrogen-fixing bacteria. PeerJ 6:5138. doi: 10.7717/peerj.5138
Liu, Q. S., Hallerman, E., Peng, Y. F., and Li, Y. H. (2016). Development of Bt rice and Bt maize in China and their efficacy in target pest control. Int. J. Mol. Sci. 17:1561. doi: 10.3390/ijms17101561
Liu, Y. M., Dang, Z. H., Parajulee, M. N., and Chen, F. J. (2019). Interactive effects of [CO2] and temperature on plant chemistry of transgenic Bt rice and population dynamics of a non-target planthopper, Nilaparvata lugens (Stål) under different levels of soil nitrogen. Toxins 11:261. doi: 10.3390/toxins11050261
Marschner, H., and Dell, B. (1994). Nutrient uptake in mycorrhizal symbiosis. Plant Soil 159, 89–102. doi: 10.1007/BF00000098
Mcgonigle, T. P., Evans, D. G., and Miller, M. H. (1990). Effect of degree of soil disturbance on mycorrhizal colonization and phosphorus absorption by maize in growth chamber and field experiments. New Phytol. 116, 629–636. doi: 10.1111/j.1469-8137.1990.tb00548.x
Pachauri, R. K., and Reisinger, A. (2014). Climate Change 2014: Synthesis Report. Contribution of Working Groups I, II and III to the fifth assessment report of the Intergovernmental Panel on Climate Change. IPCC.
Phillips, J. M., and Hayman, D. S. (1970). Improved procedures for clearing roots and staining parasitic and vesicular-arbuscular mycorrhizal fungi for rapid assessment of infection. Q. Rev. Biol. 55, 158–161. doi: 10.1016/S0007-1536(70)80110-3
Prutz, G., and Dettner, K. (2005). Effects of transgenic Bacillus thuringiensis-maize on larval food consumption, utilization and growth in the grass-moth species Chilo partellus under laboratory conditions (Lepidoptera: Crambidae). Entomol. Gen. 28, 161–172. doi: 10.1111/j.0013-8703.2005.00229.x
Rao, M. S., Srinivas, K., Vanaja, M., Manimanjari, D., Rama Rao, C. A., and Venkateswarlu, B. (2013). Response of multiple generations of semilooper, Achaea janata feeding on castor to elevated CO2. J. Environ. Biol. 34, 877–883. doi: 10.1177/1363459312465973
Riddick, E. W., Dively, G., and Barbosa, P. (1998). Effect of a seed-mix deployment of Cry3A-transgenic and nontransgenic potato on the abundance of Lebia grandis (Coleoptera: Carabidae) and Coleomegilla maculata (Coleoptera: Coccinellidae). Ann. Entomol. Soc. Am. 91, 647–653. doi: 10.1093/aesa/91.5.647
Rillig, M. C., and Mummey, D. L. (2006). Mycorrhizas and soil structure. New phytol. 171, 41–53. doi: 10.2307/3694482
Rodriguez, A., and Sanders, I. R. (2015). The role of community and population ecology in applying mycorrhizal fungi for improved food security. ISME J. 9, 1053–1061. doi: 10.1038/ismej.2014.207
Ruess, L., and Chamberlain, P. M. (2010). The fat that matters: soil food web analysis using fatty acids and their carbon stable isotope signature. Soil Biol. Biochem. 42, 1898–1910. doi: 10.1016/j.soilbio.2010.07.020
Sharifi, M., Ghorbanli, M., and Ebrahimzadeh, H. (2007). Improved growth of salinity-stressed soybean after inoculation with salt pre-treated mycorrhizal fungi. J. Plant Physiol. 164, 1144–1156. doi: 10.1016/j.jplph.2006.06.016
Smith, S. E., and Read, D. J. (2008). Mycorrhizal symbiosis. Q. Rev. Biol. 3, 273–281. doi: 10.1016/B978-012370526-6.50018-0
Song, Y. Y., Liu, J. W., and Chen, F. J. (2020). Azotobacter chroococcum inoculation can improve plant growth and resistance of maize to armyworm, Mythimna separata even under reduced nitrogen fertilizer application. Pest Manag. Sci. 76, 4131–4140. doi: 10.1002/ps.5969
Stange, G. (1997). Effects of changes in atmospheric carbon dioxide on the location of hosts by the moth, Cactoblastis cactorum. Oecologia 110, 539–545. doi: 10.2307/4221642
Stiling, P., and Cornelissen, T. (2007). How does elevated carbon dioxide (CO2) affect plant–herbivore interactions? A field experiment and meta-analysis of CO2-mediated changes on plant chemistry and herbivore performance. Glob. Change Biol. 13, 1823–1842. doi: 10.1111/j.1365-2486.2007.01392.x
Sun, Y. C., Guo, H. J., and Ge, F. (2016). Plant–aphid interactions under elevated CO2: some cues from aphid feeding behavior. Front Plant Sci. 7:502. doi: 10.3389/fpls.2016.00502
Sun, Y. C., Guo, H. J., Zhu-Salzman, K., and Ge, F. (2013). Elevated CO2 increases the abundance of the peach aphid on Arabidopsis by reducing jasmonic acid defenses. Plant Sci. 210, 128–140. doi: 10.1016/j.plantsci.2013.05.014
Terrer, C., Vicca, S., Hungate, B. A., Phillips, R. P., and Prentice, I. C. (2016). Mycorrhizal association as a primary control of the CO2 fertilization effect. Science 353, 72–74. doi: 10.1126/science.aaf4610
Treseder, K. K. (2004). A meta-analysis of mycorrhizal responses to nitrogen, phosphorus, and atmospheric CO2 in field studies. New Phytol. 164, 347–355. doi: 10.1111/j.1469-8137.2004.01159.x
Turrini, A., Bedini, A., Loor, M. B., Santini, G., Sbrana, C., Giovannetti, M., et al. (2017). Local diversity of native arbuscular mycorrhizal symbionts differentially affects growth and nutrition of three crop plant species. Biol. Fert. Soils 54, 203–217. doi: 10.1007/s00374-017-1254-5
Wang, L., Li, L. K., Song, Y. Y., Han, T., Li, Z., Wan, G. J., et al. (2018). Elevated CO2 and temperature alter specific-species population dynamic and interspecific competition of three wheat aphids. J. Appl. Entomol. 142, 863–872. doi: 10.1111/jen.12536
Wu, G., Chen, F. J., Ge, F., and Xiao, N. W. (2011a). Impacts of elevated CO2 on expression of plant defensive compounds in Bt-transgenic cotton in response to infestation by cotton bollworm. Agric. For. Entomol. 13, 77–82. doi: 10.1111/j.1461-9563.2010.00508.x
Wu, G., Chen, F. J., Xiao, N. W., and Ge, F. (2011b). Influences of elevated CO2 and pest damage on the allocation of plant defense compounds in Bt-transgenic cotton and enzymatic activity of cotton aphid. Insect Sci. 18, 401–408. doi: 10.1111/j.1744-7917.2011.01429.x
Wu, K. M., Lu, Y. H., Feng, H. Q., Jiang, Y. Y., and Zhao, J. Z. (2008). Suppression of cotton bollworm in multiple crops in china in areas with Bt toxin-containing cotton. Science 321, 1676–1678. doi: 10.1126/science.1160550
Xu, Z. Z., Jiang, Y. L., and Zhou, G. S. (2015). Response and adaptation of photosynthesis, respiration, and antioxidant systems to elevated CO2 with environmental stress in plants. Front. Plant Sci. 6:701. doi: 10.3389/fpls.2015.00701
Yao, Z. S., Wang, R., Zheng, X. H., Mei, B. L., Zhou, Z. X., Xie, B. H., et al. (2020). Elevated atmospheric CO2 reduces yield-scaled N2O fluxes from subtropical rice systems: six site-years field experiments. Glob. Change Biol. 27, 327–339. doi: 10.1111/gcb.15410
Yin, J., Sun, Y. C., and Wu, G. (2010). Effects of elevated CO2 associated with maize on multiple generations of the cotton bollworm, Helicoverpa armigera. Entomol. Exp. Appl. 136, 12–20. doi: 10.1111/j.1570-7458.2010.00998.x
Yu, T., and Chen, Y. (2019). Effects of elevated carbon dioxide on environmental microbes and its mechanisms: a review. Sci. Total Environ. 655, 865–879. doi: 10.1016/j.scitotenv.2018.11.301
Zeng, H., Zhong, W., Tan, F., Shu, Y., Feng, Y., and Wang, J. (2018). The influence of Bt maize cultivation on communities of arbuscular mycorrhizal fungi revealed by MiSeq sequencing. Front Microbiol. 9:3275. doi: 10.3389/fmicb.2018.03275
Zhu, C. W., Kobayashi, K., Loladze, I., Zhu, J. G., Jiang, Q., Xu, X., et al. (2018). Carbon dioxide (CO2) levels this century will alter the protein, micronutrients, and vitamin content of rice grains with potential health consequences for the poorest rice-dependent countries. Sci. Adv. 4:eaaq1012. doi: 10.1126/sciadv.aaq1012
Keywords: elevated CO2, transgenic Bt maize, arbuscular mycorrhizal fungi, Mythimna separata, control efficiency, yield
Citation: Wang L, Wang X, Gao F, Lv C, Li L, Han T and Chen F (2021) AMF Inoculation Can Enhance Yield of Transgenic Bt Maize and Its Control Efficiency Against Mythimna separata Especially Under Elevated CO2. Front. Plant Sci. 12:655060. doi: 10.3389/fpls.2021.655060
Received: 18 January 2021; Accepted: 10 May 2021;
Published: 08 June 2021.
Edited by:
Youssef Rouphael, University of Naples Federico II, ItalyReviewed by:
Philipp Franken, Friedrich Schiller University Jena, GermanyElisa Pellegrino, Sant'Anna School of Advanced Studies, Italy
Copyright © 2021 Wang, Wang, Gao, Lv, Li, Han and Chen. This is an open-access article distributed under the terms of the Creative Commons Attribution License (CC BY). The use, distribution or reproduction in other forums is permitted, provided the original author(s) and the copyright owner(s) are credited and that the original publication in this journal is cited, in accordance with accepted academic practice. No use, distribution or reproduction is permitted which does not comply with these terms.
*Correspondence: Fajun Chen, fajunchen@njau.edu.cn