- 1Division of Quality and Sensory of Plant Products, Department of Crop Sciences, Georg-August-Universität Göttingen, Göttingen, Germany
- 2Department of Plant Physiology, Faculty of Agrobiology and Food Resources, Slovak University of Agriculture, Nitra, Slovakia
Introduction: Providing fresh and healthy vegetables, produced locally and under climate-friendly conditions, is a major challenge for future agriculture. The usage of foil tunnels prolongs cultivation periods in colder climates and reduces abiotic and biotic stress factors during crop growth, but it may also affect nutritional value and consumer perception due to the altered light spectrum.
Methods and results: Three different foils, one with low UV transmission, another with reduced UVB transmission, and a control foil with high UV transmission, were used to modulate light conditions for three different lettuce cultivars [radicchio type (cv. Indigo), romaine type (cv. Attico), and butterhead type (cv. Larissa)]. Taste-relevant sesquiterpene lactones and health-relevant carotenoids and polyphenols were measured by high-performance liquid chromatography, which revealed that light conditions are widely irrelevant for carotenoid concentrations. However, when UV-shielding foils were used, there was an up to 66% decrease in total polyphenol concentration. Less reduction could be achieved through the use of partially UV-transmissive foils. Sesquiterpene lactone concentrations were higher in plants under UV-blocking foils, when radicchio-type lettuce, naturally rich in sesquiterpene lactones, was cultivated.
Discussion: It is noteworthy that the sesquiterpene lactone lactucopicrin had a negative correlation with UVB intensities, while lactucin was unaffected. The nutritional value, measured by three different antioxidant activity assays, also benefited from an optimized foil choice with higher UV transmission.
1 Introduction
Lettuce is an important leafy vegetable worldwide and reached an annual harvest of 29 million tons in 2019 (Food and Agriculture Organization of the United Nations, 1997). The common term lettuce does not only refer to Lactuca sativa L. plants but also often includes Cichorium intybus L. plants. The storability of such leafy vegetables is limited, and during cold seasons, distributors and consumers in temperate climates depend on imports from warmer climates or indoor production facilities. However, significant amounts of fossil energy are used, leading to the emission of greenhouse gases that contribute to climate change. Polytunnels are used in temperate regions to extend the growth period of locally produced lettuce in cold seasons (Robson et al., 2022). Polytunnels heat up under solar radiation and provide climate-neutral higher temperatures for the plants, as well as controlled humidity and protection from extreme weather events such as hailstorms and winds. Such an unheated growth system might drastically reduce climate gas release during vegetable production (Theurl et al., 2014; Theurl et al., 2017). However, polytunnels are only semi-permeable to light, and a substantial share of the total light spectrum is absorbed. Commercially available foils do reduce photosynthetically active radiation (PAR) and UVB radiation to different ratios, while standard glass greenhouses do not transmit UVB radiation at all (Kotilainen et al., 2018). Thus, altered light patterns reaching the plant are expected when growing plants under diverse foils, i.e., the contribution of UVB radiation may vary from 0.003% to 0.062%, while UVA may correspond to 0.5%–3.5% of the total radiation. Consequently, the ratios of UV to PAR radiation differ between foils (Kotilainen et al., 2018).
Physiological responses to differing doses of PAR and UV radiation cause morphological changes and alter the accumulation of secondary plant metabolites in lettuce (Assumpção et al., 2019; Chen et al., 2019). The causal response to UVB radiation is the UVR8 photoreceptor, while UVA- and PAR-modulated light responses are controlled by cryptochromes, phototropins, and Zeitlupe (ZTL) proteins (Heijde and Ulm, 2012). UVB reduces plant height and leaf area, among other effects (Jenkins, 2009). Most importantly, concentrations of secondary plant metabolites, including phenolic acids caffeoyltartaric acid, caffeoylquinic acid, and caffeoylmalic acid, are increased in lettuce plants under UVB exposure (Assumpção et al., 2019). More recently, dicaffeoyltartaric acid reductions under consistent UVB exposure have been reported, while quercetin and kaempferol glycosides increased 3–7 fold under the same conditions (Weiland et al., 2023). The accumulation of polyphenols in lettuce under natural UV exposure is due to a regulation of the transcriptional level, namely, a higher chalcone synthase expression, compared to lettuce grown under foil (Harbart et al., 2023). In contrast to polyphenol accumulation, post-transcriptional regulation has been assumed for carotenoids (Harbart et al., 2023). High concentrations of polyphenols are regarded as an important factor for a healthy lifestyle, contributing to the prevention of metabolic-, aging-, or lifestyle-associated illnesses (Leri et al., 2020). Carotenoid concentrations also increase under exposure to supplemental UVB light for 2 weeks (Assumpção et al., 2019), thus improving lettuce as a valuable source of these health-beneficial compounds (Kim et al., 2016). Sesquiterpene lactones are less in the focus of research but also contribute decisively to lettuce quality. Their bitter taste is often disliked by consumers, but several health-beneficial effects, such as tumor inhibition in human cancer cell lines, anti-inflammatory effects, or antimicrobial function, have been reported (Chadwick et al., 2013; Chadwick et al., 2016). In addition, reports on sesquiterpene lactone concentrations after UVB exposure are missing. Consumers are reported to prefer lettuce grown under natural light conditions. When sunlight-exposed lettuce was compared to lettuce grown under artificial light conditions, based on supplemental light in the red, far-red, and blue spectra, consumers reported a bitter taste in lettuce grown under artificial light (Zhang et al., 2019). This study aimed to evaluate the impact of different light conditions provoked by different foils, capable of transmitting different intensities of UV light, in general, or UVB, in particular, on the quality of lettuce. The fresh weight of lettuce and secondary plant metabolites—carotenoids, polyphenols, and sesquiterpene lactones—were measured to elucidate the effect of UV light on the quality parameters of lettuce.
2 Materials and methods
2.1 Chemical and plant seeds
Acetonitrile, methanol, acetic acid, tert-butyl methyl ether (tBME), tetrahydrofuran (THF), ethyl acetate, β-carotene, caftaric acid, and formic acid were purchased from Roth (Karlsruhe, Germany). Standards of zeaxanthin and lutein were purchased from Extrasynthese (Genay, France). Ammonium acetate, sodium sulfate, gallic acid monohydrate, sodium carbonate, chicoric acid, and Folin–Ciocalteu´s phenol reagent were purchased from Merck (Darmstadt, Germany). Furthermore, 2,2-diphenyl-1-picrylhydrazyl (DPPH) free radicals (Alfa Aesar, Kandel, Germany), Trolox (Thermo Fisher, Kandel, Germany), 2,2′-azino-bis-3-ethylbenzothiazoline-6-sulfonic acid (ABTS), potassium persulfate, and cellulase enzymes from Aspergillus niger (Merck) were used. Additional standards of chlorogenic acid, quercetin-3-glucoside, and kaempferol-3-glucoside were purchased from PhytoLab (Vestenbergsgreuth, Germany).
2.2 Cultivation and harvest of samples
Radicchio-type lettuce cultivar Indigo (C. intybus L.), romaine-type lettuce cultivar Attico (L. sativa var. longifolia (Lam.) Helm.), and butterhead-type lettuce cultivar Larissa (L. sativa var. capitata L.) (all from Kiepenkerl, Everswinkel, Germany) were sown in pots with Fruhstorfer soil (Hawita, Vechta, Germany) on 11 August 2020 and grown in a ventilated air chamber with halogen light (12 h day/night) at a temperature of 20°C/16°C. After 20 days, plants were transferred outdoors (21 August 2020) and subsequently grown under ambient light conditions, including the three treatments with foils “Rosco” (UV-absorbing foil; Rosco, Stamford, CT, United States), “CT5” (UVB-absorbing foil; Gabler Druck-und Werbetechnikbedarf, Bochum, Germany), and “Polythene” (UV-transmitting foil; Etra Oy, Helsinki, Finland) as well as one treatment without foil. Light conditions were measured with a DataHog 2 data logger (Skye Instruments, Llandrindod Wells, United Kingdom), equipped with UVA, UVB, and PAR light sensors (Table 1). Each of the four treatments consisted of six plants from each cultivar, planted with 30 cm of spacing between plants. Harvest was carried out on 05 October 2020, and four plants from each treatment were used for fresh weight determination and chemical analyses. Meteorological data were gathered at the Göttingen weather station (www.dwd.de, N 51° 30.027176 E 9° 57.029615).
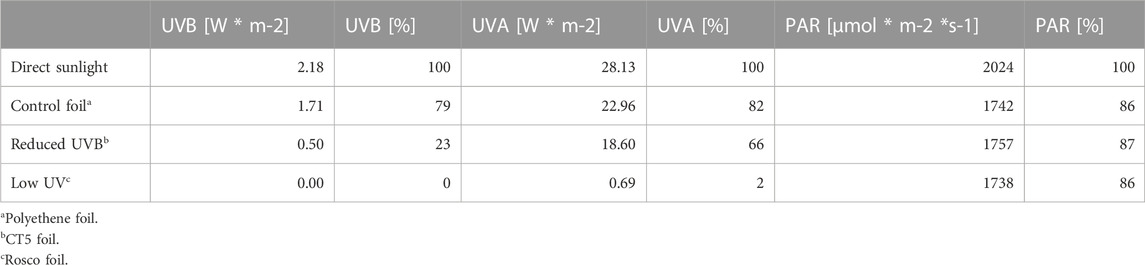
TABLE 1. Relative light intensity (%) of PAR, UVA, and UVB light under the applied conditions in comparison to direct sunlight.
2.3 Determination of fresh weight and lyophilization
Whole lettuce heads were weighed directly after harvest to determine lettuce fresh weight. Subsequently, lettuce heads were cut in half along the longitudinal axis, and one half was lyophilized to total dryness. Dry weight was determined based on the ratio of the weight loss of samples after lyophilization to their fresh weight. Lyophilized leaf samples were milled in a ball mill (Retsch, Haan, Germany) at 30 Hz for 30 s to a fine powder for subsequent analyses.
2.4 Extraction and determination of carotenoids by HPLC-DAD
Carotenoids and chlorophylls were extracted and analyzed according to a slightly modified method reported by Bayer et al. (2022). Sample preparation was conducted under dim light to prevent carotenoids from degradation. In brief, the lyophilized ground material (10 mg) was extracted with 500 µL methanol and tetrahydrofuran (50:50, v/v) and mixed for 10 min with a thermomixer (Eppendorf Hamburg, Deutschland) at a rotational speed of 1,400 rpm at a temperature of 20°C. Subsequently, the mixture was centrifuged at 10,000 rpm for 10 min at 20°C. The supernatant was collected, and the solid pellet was re-extracted twice. The combined supernatants (approximately 1,500 µL) were evaporated to dryness in an RVC 2-25 CD plus rotary evaporator (Martin Christ, Osterode am Harz, Germany) with a PC 3001 Vario select membrane vacuum pump (Vacuubrand, Wertheim, Germany) and re-dissolved in 100 µL methyl tert-butyl ether and 150 µL methanol in the aforementioned thermomixer at 1,400 rpm and 20°C for 10 min. The re-dissolved extract was filtered through a Chromafil® PTFE (0.2-µm) syringe filter (Macherey–Nagel, Düren, Germany) and stored in HPLC vials at −80°C until HPLC analyses. The aforementioned authentic standards of zeaxanthin, β-carotene, and lutein were used to identify and determine carotenoids in all samples.
Analysis of carotenoids and chlorophylls was performed on a Shimadzu Prominence HPLC system (Shimadzu, Kyoto, Japan), equipped with an LC-20AT pump, a CTO-10AS column oven, a DGU-20AS degasser, and a SPD-M20A UV/vis diode array detector. A YMC C30 reversed-phase column (250 × 4.6 mm i.d., 5 µm particle size, YMC Europe, Basel, Switzerland) and a YMC C30 guard column (10 × 4.6 mm i.d., 5 µm particle size, YMC Europe) were used for separation. The mobile phase consisted of methanol/MTBA/water (80:18:2, v/v/v) with 0.4 g/L ammonium acetate (eluent A) and methanol/MTBE/water (8:90:2, v/v/v) with 0.4 g/L ammonium acetate (eluent B). The applied gradient of eluent B was 10% (0 min)–60% (30 min)–100% (35 min)–isocratic 100% (37 min)–10% (40 min), followed by isocratic 10% (40–45 min). The total run time was 45.5 min at a flow rate of 0.600 mL/min and an oven temperature of 20°C. The injection volume was 20 µL. Determination was carried out after obtaining linear calibration curves of the aforementioned authentic standards at 454 nm (β-carotene), 446 nm (lutein), and 452 nm (zeaxanthin).
2.5 Extraction and determination of phenolic substances by HPLC-DAD
Phenolic substances were analyzed according to a previously published method by Bayer et al. (2022) with slight modifications. The lyophilized powder (50 mg) was extracted with 1,500 µL of 60% aqueous methanol and mixed with a thermomixer at a rotational speed of 1,400 rpm at 20°C for 40 min. The supernatant was collected after centrifugation (10,000 rmp for 5 min), and pellets were re-extracted twice. The three corresponding supernatants were combined (approximately 4,500 µL) and filled up to a final volume of 5 mL. Combined extracts were filtered through syringe filters (Chromafil® RC-20/13 MS (0.2 µm); Macherey–Nagel) and stored at −20°C until HPLC analyses. Authentic standards of chlorogenic acid, caftaric acid, chichoric acid, and quercetin-3-glucoside (Carl Roth, Karlsruhe, Germany) were used for the identification and quantitation of polyphenols. All extraction protocols were carried out in duplicate.
HPLC analyses were carried out on the aforementioned Prominence HPLC system. For separation, a Supelco Ascentis Express F5 column (150 mm × 3.0 mm i.d., 5 µm particle size, Supelco, Bellefone, PA, United States) equipped with a Supelco Ascentis Express F5 (5 mm × 3.0 mm i.d., 5 µm particle size) guard column was used. The mobile phase consisted of acetic acid (1%) in water (eluent A) and acetonitrile (eluent B). The gradient applied was as follows: B conc. 5% (0 min)—12% (3 min)—25% (46 min)—isocratic 90% (49.5 min)—90% (52 min)—5% (52.7 min)—5% (59 min). The total run time was 59 min at a flow rate of 0.300 mL/min and an oven temperature of 20°C. The injection volume was 20 µL. Polyphenol chicoric acid, caffeoylmalic acid, and caffeoyltartaric acid were monitored at 330 nm. 3-5-dicaffeoylquinic acid, meso-dicaffeoyltartaric acid, quercetin-3-glucoronide, quercetin-3-malonylglucoside, and kaempferol-3-glucoronide were detected at 350 nm, and additionally, UV–VIS spectra were recorded in the range from 200 to 800 nm. Quantification was carried out after obtaining linear calibration curves of authentic standards of chlorogenic acid, chicoric acid, caftaric acid, quercetin-3-glucoside, and kaempferol-3-glucoside. 4-caffeoylqunic acid, 5-caffeoyluinic acid, caffeoylmalic acid, and 3-5-dicaffeoylquinic acid were quantitated as chlorogenic acid equivalents. Caffeoyltartaric acid was quantitated as the caftaric acid equivalent. Chichoric acid was quantitated as the chichoric acid equivalent. Quercetin-3-glucuronide and quercetin-3-malonyl glucoside were quantitated as quercetin-3-glucoside equivalents. Kaempferol-3-glucuronide was quantitated as the kaempferol-3-glucosid equivalent.
2.6 Extraction and determination of sesquiterpene lactones by HPLC-DAD
Sesquiterpenes were extracted and analyzed according to the method reported by Wulfkuehler et al. (2013) with slight modifications. A total of 300 mg of the lyophilized ground material of samples was weighed into 15-mL tubes and rehydrated by adding 8 mL of H2O (pH 5, adjusted with HCL and NaOH). Samples were liquefied using 400 µL of cellulase from Aspergillus niger (Sigma Aldrich, St. Louis, MO, United States). Liquefaction took place under continuous stirring at 37°C for 2 h. After filtration through paper filters (MN 615 1/4, 12 μm, Macherey–Nagel, Düren, Germany) and the addition of 20 mL of NaCl solution (20% w/v), the mixture was extracted twice with 5 mL of ethyl acetate in 50-mL tubes. Thereafter, combined mixtures were shaken in a thermomixer at 700 rpm for 10 min and subsequently centrifuged at 5,000 rpm and 20°C for 5 min. The combined supernatants of both extractions were dried over sodium sulfate and filtered through paper filters (MN 615 1/4; 12 μm, Macherey–Nagel). Combined extracts were evaporated to dryness in the aforementioned rotary evaporator RVC 2-25 CD Plus, dissolved in 500 µL methanol, filtered with Chromafil® PTFE (0.2 µL) syringe filters (Macherey–Nagel), and stored in HPLC vials at −80°C until HPLC analyses. All extraction protocols were carried out in duplicate.
For analysis of sesquiterpene lactones, a Jasco LC-4000 HPLC system (Jasco, Tokyo, Japan), equipped with an AS-4150 autosampler, a CO-4060 column oven, a PU-4185 semi-micro pump, a UV-4070 UV/Vis detector, and ChromNAV software, was used. Separation was performed on a NUCLEODUR Sphinx RP (150 × 4.6 i.d.; particle size 5 µm) column (Macherey-Nagel) with a NUCLEODUR Universal RP 4 × 3 mm i.d.; particle size 5 µm) guard column (Macherey-Nagel). The mobile phase consisted of 5% formic acid in ultrapure water (eluent A) and acetonitrile (eluent B). The gradient applied was as follows: B conc: 99% (0 min)—99% (10 min)—40% (50 min)—0% (55 min)—0% (60 min)—99% (65 min)—99% (72 min). The total run time was 72 min at a flow rate of 0.5 mL/min and a column temperature of 20°C. The injection volume was 10 µL. Sesquiterpenes were monitored at 259 nm. Quantitation was carried out after obtaining linear calibration curves of lactucin and lactucopicrin at 259 nm.
2.7 Mass spectrometric analysis of phenolic substances and sesquiterpene lactones
The same aforementioned columns and methods for phenolic substances and sesquiterpene lactones were used for tentative confirmation of the compound’s identity. Acidic concentrations in all eluents were reduced to 0.5%. Measurements were performed on an Agilent 1290 Infinity II HPLC system (Agilent Technologies, Palo Alto, CA, United States), coupled to an Agilent 6545 LC/Q-TOF mass spectrometer, with a G6545A Q-TOF, a G7104A pump, a G7116B column compartment, and a G7167A multisampler. The measurement was performed in the ESI negative mode for phenolic compounds and positive mode for sesquiterpene lactones in a range from m/z 100 to 920 with a rate of 4 spectra/sec. The gas temperature was set to 320°C at a flow rate of 8 L/min. Nebulizer gas was 35 psi, and sheath gas temperature and flow rate were 350°C and 11 L/min, respectively. Source parameters were a capillary voltage of 3500 V, a nozzle voltage of 1,000 V, and a fragmentor voltage of 150 V. The injection volume was 5 µL for all samples.
2.8 Antioxidant TEAC, DPPH, and TPC assays
For the determination of antioxidant activity with the TEAC assay, the aforementioned methanolic extracts for phenolic substances were used according to a previously published method by Engelhardt et al. (2021). In brief, stock solutions of 2,2′-azino-bis(3-ethylbenzothiazoline-6-sulfonic acid (ABTS; 9.6 mg) and potassium persulfate (K2S2O8; 1.66 mg) were dissolved in 25 mL of ultrapure water and used, after 12–16 h of incubation in the dark, throughout the analysis. A total of 3.13 mg of Trolox® was dissolved in 5 mL of methanol and used within 2 h. In 96-well microplates, 10 µL of the sample, 10 µL of the Trolox® calibration mix, or 10 µL of blank (60% methanol), as well as 150 µL of the TEAC solution, were pipetted. Mixtures were incubated in darkness for 5 min before being placed inside a microplate reader (Synergy HTX multi-mode microplate reader, Bad Friedrichshall, Germany) and measured at 734 nm after 30 s of orbital shaking. For DPPH measurements, 7.88 mg of 2,2-diphenyl-1-picryl-hydrazyl-hydrate (DPPH) was dissolved in 100 mL of methanol and incubated for 2 h in darkness. Furthermore, the same aforementioned Trolox® mixture was used. In 96-well microplates, 20 µL of the sample, 20 µL of the Trolox® calibration mix, or 20 µL of blank (60% methanol), as well as 180 µL of the DPPH solution, were mixed. The incubation time was 30 min in darkness; afterward, the measurement was performed on the aforementioned microplate reader at 515 nm. For TPC analyses, 5 g of Na2CO3 was dissolved in 250 mL of ultrapure water, and 26.43 mg of gallic acid monohydrate was dissolved in 10 mL of ultrapure water. The gallic acid working solution was further diluted by factor 5. A 50 μL sample, 50 µL of the gallic acid calibration mix, or 50 µL of blank (60% methanol), as well as 100 µL of Na2CO3, were combined in 96-well microplates. The absorbance was measured at 736 nm in the aforementioned microplate reader after 15 min of orbital shaking and 1 min of resting.
2.9 Statistics
All analytical procedures were performed in duplicate. Results are expressed as means ± standard deviation and were calculated across cultivars and UV treatments. The number of biological replicas (n) was 4, if not stated otherwise. All statistical analyses were carried out with R Core Team (2019). Analysis of variance (ANOVA) with a subsequent Tukey’s test was applied for identifying significant differences (p ≤ 0.05).
3 Results
3.1 Growth conditions and biomass
Lettuce plants were transferred to the field after 20 days and harvested in early October of 2020. During that period, mean temperatures decreased from an average of 18.6°C in the first week to 12.6°C in the last 4 days before harvest, all measured to a height of 2 m. The lowest daily average temperatures were 13.6°C in the first week and 6.5°C in the last week before harvest, also measured to a height of 2 m. It is noteworthy that minimum temperatures at 5 cm were below 0°C for 3 days in the last 3 weeks and were, on average, below 10°C most of the time throughout the whole experiment. The fresh weights of romaine-type cvs. Attico and Larissa were higher when plants were grown under foils, i.e., the fresh weights of plants grown without foil shelter were 41.6 ± 7.7 g and 52.8 ± 9.8 g. Under foil treatments, fresh weights were considerably higher, ranging between 143.2 ± 15.2 g and 199.1 ± 30.7 g for cv. Attico and between 159.8 ± 17.3 g and 189.8 ± 29.5 g for cv. Larissa. Within the different treatments of radicchio-type cv. Indigo, there were no differences, with plant biomasses ranging from 37.8 ± 12.7 g to 56.8 ± 10.4 g. Cultivar Attico contained between 5.9% ± 0.3% and 7.0% ± 0.3% dry matter, cv. Indigo contained between 7.3% ± 0.5% and 10.7% ± 4.9%, and cv. Larissa contained between 4.8% ± 0.2% and 6.8% ± 0.1%. Consequently, there were differences between cultivars but not within treatments of the cvs. Attico and Indigo. Within cv. Larissa, the dry matter was highest in samples grown without foil (Table 2).
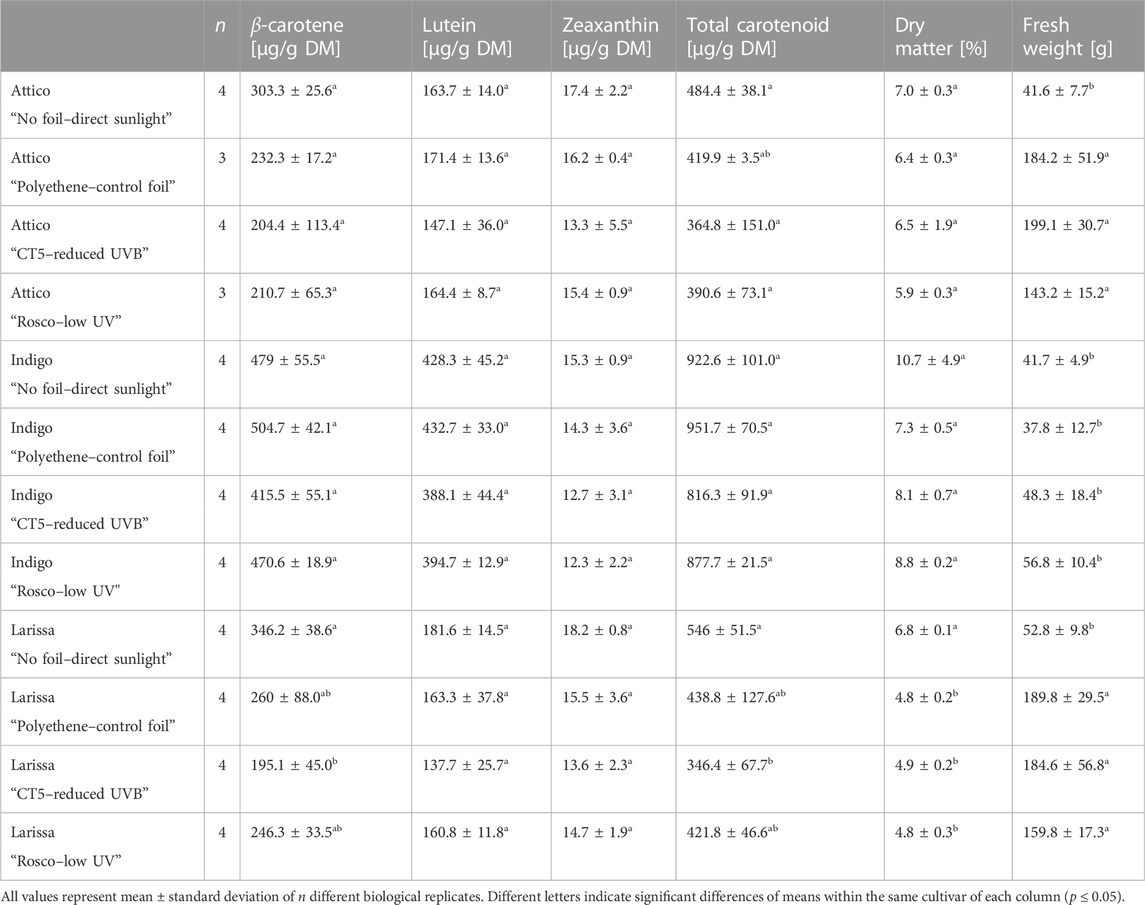
TABLE 2. Dry matter, fresh weight, and carotenoid concentrations of three lettuce cultivars (Lactuca sativa L. and Cichorium intybus L.) grown under three different foils, control foil, reduced UVB foil, and low UV foil, compared to direct sunlight.
3.2 Carotenoids
The carotenoids β-carotene, lutein, and zeaxanthin were found in all lettuce samples. The highest concentrations of total carotenoids were found in cultivar Indigo, ranging from 816.3 ± 91.9 to 951.7 ± 70.5 µg per g dry matter (DM). Cvs. Attico and Larissa contained lower carotenoid concentrations below 370 µg per g DM. Those differences originated from higher lutein concentrations in cultivar Indigo up to 432.7 ± 33 µg per g DM, while the other two cultivars contained less than 200 µg lutein per g dry matter. Similar observations were made for β-carotene, where concentrations were highest in cv. Indigo, being higher than most samples of cv. Larissa (195.1 ± 45.0 to 346.2 ± 38.6 µg per g DM) and all samples of cv. Attico (204.4 ± 113.4 to 303.3 ± 25.6 µg per g DM). Concentrations of zeaxanthin were equally low among all cultivars and treatments, not surpassing 20 µg per g DM (Table 2). Differences based on foil treatments were not observed in this study. In addition, there are few differences between plants of the same cultivar grown under foils and those grown in direct sunlight. Only, within cv. Larissa, β-carotene concentration was lower under UVB reducing foils, causing a lower overall carotenoid concentration.
3.3 Phenolic substances
In total, seven phenolic substances were identified and quantitated during our experiments. Compound 1 (caffeoyltartaric acid), at a retention time of 6.3 min, had a deprotonated molecular ion of m/z 311.04169 and fragment ions of m/z 179.03259, 149.00841, and 135.04549, which were identified as deprotonated caffeic acid and tartaric acid. Compound 2 (caffeoylmalic acid; retention time: 9.8 min) had a deprotonated molecular ion of m/z 295.04684, with fragment ions of m/z 135.04198 and 115.00678 m/z, representing deprotonated malic acid and an unidentified fragment, respectively. Compound 3 was identified as chicoric acid due to its molecular ion of m/z 473.0735 and fragment ions of m/z 311.04077, 179.03500 (deprotonated caffeic acid), and 149.00978 (deprotonated tartaric acid). Compound 4 was identified as quercetin-3-glucuronide with a molecular ion of m/z of 477.06726 and fragment ions of m/z 301.03436 (deprotonated quercetin) and 151.00365 (characteristic A− ring). Compound 5 was identified as kaempferol-3-glucuronide with a molecular ion of m/z 461.07239 and a fragment ion of m/z 285.03986 (deprotonated kaempferol). Compound 6 was identified as quercetin-3-malonylglucoside, based on the molecular ion of m/z of 549.0885 and its fragment ion of m/z 300.02664 (twice deprotonated quercetin). Compound 7 was identified as 3-5-dicaffeoylquinic acid due to its molecular ion of m/z of 515.11859, with fragment ions of m/z of 191.0656 (deprotonated quinic acid) and 179.03479 (deprotonated caffeic acid) (Table 3).
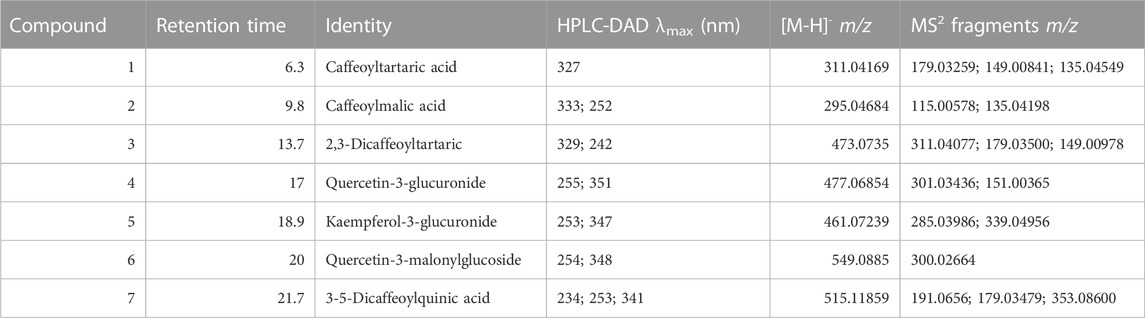
TABLE 3. UV spectra and mass spectrometric data (deprotonated molecular ions and fragment ions in MS2) of characteristic phenolic substances from lettuce (Lactuca sativa L. and Cichorium intybus L.).
Phenolic substances varied largely between cultivars, with cv. Indigo having the highest concentration in total phenols, reaching up to 164.2 ± 10.9 mg per g DM. The highest concentrations within other cultivars were considerably lower, reaching 93.7 ± 9.1 mg per g DM in cv. Larissa and 63.0 ± 7.7 in cv. Attico. It is noteworthy that the highest concentrations were always reached in samples in full sunlight. Cv. Indigo had the highest concentration in phenols under UVB-shielding foils, while the lowest concentrations were found under control foils and UV-shielding foils. The concentration of all flavonoids, including that of kaempferol-3-glucuronide, was highest in treatments under full sunlight and under UVB radiation-shielding foil. The concentration of phenolic acids, other than chicoric acid, was considerably lower, with only caffeoylmalic acid surpassing 10 mg per g DM. However, in contrast to flavonoid concentrations, we could not observe any differences in phenolic acid concentrations within the treatments. Within cvs. Attico and Larissa, there were no differences between different foil treatments. The most abundant phenol in cv. Indigo was chicoric acid, reaching 61.0 ± 5.0 mg per g DM, followed by the flavonoids quercetin-3-glucuronide and quercetin-3-malonylglucoside, reaching a maximum concentration of 44.1 ± 5.3 and 34.1 ± 1.4 mg per g DM, respectively. Regarding the cvs. Attico and Larissa, being low in phenolic substances, concentrations of quercetin-3-glucuronide, quercetin-3-malonylglucoside, and kaempferol-3-glucuronide were 16.4 mg per g DM or lower, regardless of the applied treatment. Still, plants grown under foils were lower in quercetin-3-glucoronide and quercetin-3-manonylglucoside than plants grown in direct sunlight. Chicoric acid was found in the highest concentrations of 28.9 ± 3.6 and 44.7 ± 2.6 mg per g DM in untreated plants of cvs. Attico and Larissa, respectively. In cvs. Attico and Larissa, concentrations of chicoric acid and caffeoyltartaric acid were highest under full sunlight, while in cv. Indigo, concentrations were highest in samples grown under full sunlight and under UVB-shielding foils. Furthermore, in cv. Indigo, concentrations of quercetin-3-glucuronide and kaempferol-3-glucuronide were highest under UVB-shielding foils, reaching values comparable to those under full sunlight (Table 4).
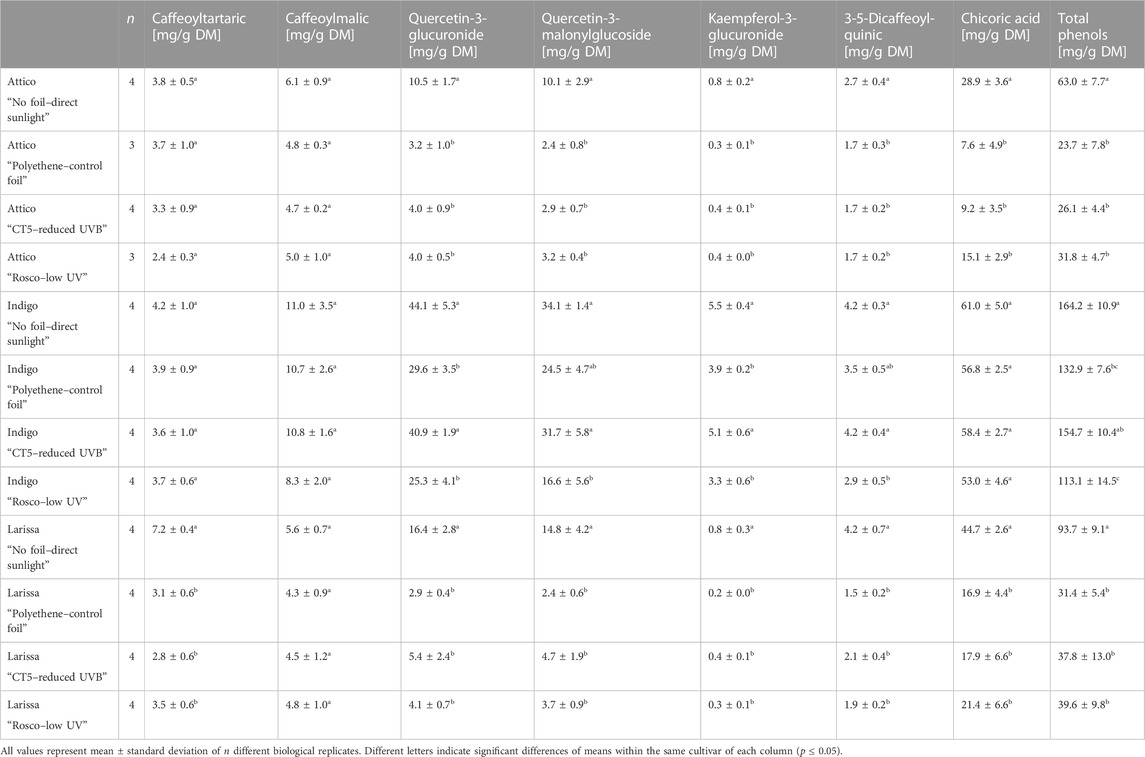
TABLE 4. Phenolic substance concentrations of three lettuce cultivars (Lactuca sativa L. and Cichorium intybus L.) grown under three different foils, control foil, reduced UVB foil, and low UV foil, compared to direct sunlight.
3.4 Sesquiterpene lactones
In total, six sesquiterpene lactones (STLs) were identified and quantitated. Compound 1 (1(S),13-dihydrolactucin) was identified due to its characteristic mass of m/z 279.12231 (a protonated molecular ion; theoretical mass m/z 279.12270) and its fragment ions of m/z 161.09557 and m/z 169.096116. Peaks 2 and 3 were identified as lactucin and 8-deoxylactucin due to their characteristic masses of m/z 277.10992 12231 (a protonated molecular ion; theoretical mass m/z 277.10705) and m/z 261.11102 (a protonated molecular ion; theoretical mass m/z 261.11214) with their fragments of m/z 185.06290 and m/z 213.08601, as well as m/z 188.55617 and m/z 197.09373. Additionally, the fragmentation of both parent ions led to a loss of CO2 from the lactone ring, H2O, and two protons (m/z 213.08601 and m/z 197.09373) and C2H2 (m/z 185.06290 and m/z 188.55617). For compound 4, a mass of m/z 263.12872 (a protonated molecular ion; theoretical mass m/z 263.12779) and a fragment ion of 199.11098 m/z (loss of CO2 from the lactone ring, H2O, and two protons) were found. Compounds 5 and 6, identified as 11(S),13-dihydrolactucopicrin and lactucopicrin, respectively, were identified according to their characteristic masses of m/z 413.15958 (a protonated molecular ion; theoretical mass m/z 413.16007) and m/z 411.14243 (a protonated molecular ion; theoretical mass m/z 411.14493). The loss of 4-hydroxyphenylacetic acid (152.0473 u) was only observed for 11(S),13-dihydrolactucopicrin and resulted in a m/z 261.11237 fragment (Table 5). STL concentrations were highest in radicchio-type lettuce cv. Indigo with concentrations up to 127.0 ± 23.0 µg per g DM. In butterhead- and romaine-type lettuce cvs. Larissa and Attico, STL concentrations did not surpass 30 µg per g DM. 11(S),13-dihydrolactucin, lactucin, and lactucopicrin were found in all samples, while in cv. Indigo, all six different aforementioned STLs were tentatively identified. Lactucopicrin was the most abundant STL in cvs. Indigo, reaching up to 46.3 ± 7.2 µg per g DM. In cvs. Larissa and Attico, concentrations of 11(S),13-dihydrolactucin, lactucin, and lactucopicrin reached a maximum of 5.5 ± 2.7, 8.4 ± 5.6, and 11.3 ± 1.9 µg per g DM, respectively. Overall, the highest STL concentrations were reached under UV-shielding foils, while differences between other treatments were not significant in cvs. Attico and Indigo. However, in cv. Larissa, concentrations of STLs were highest in direct sunlight and control foils. In contrast to other STLs, concentrations of lactucopicrin were highest under UV-reduced conditions in cv. Indigo, surpassing all other treatments. Furthermore, high concentrations of 11(S),13-dihydrolactucopicrin were reached under the same conditions. In detail, within cv. Indigo, differences between UV-shielding foils and UVB-shielding foils were observed for total STLs, 11(S),13-dihydrolactucopicrin, and lactucopicrin, while differences between other treatments were less pronounced (Table 6). Within the same cultivar, concentrations of 11(S),13-dihydro-8-deoxylactucin were highest in plants grown in direct sunlight, whereas there was no difference between foil treatments. No further differences in concentrations of 11(S),13-dihydrolactucin, lactucin, and 8-deoxylactucin regarding the foliar treatments were observed. Cvs. Attico and Larissa were so low in STLs overall, and their composition was limited to 11(S),13-dihydrolactucin, lactucin, and lactucopicrin (Table 6). Within cv. Attico, there were no differences between all four treatments. In cv. Larissa, the concentrations of 11(S),13-dihydrolactucin and lactucin were higher in direct sunlight and control foils when compared to UV- and UVB-shielding foils. Concentrations of lactucin and total STLs were also highest in direct sunlight and control foils, but differences from the other treatments were smaller (Table 6).
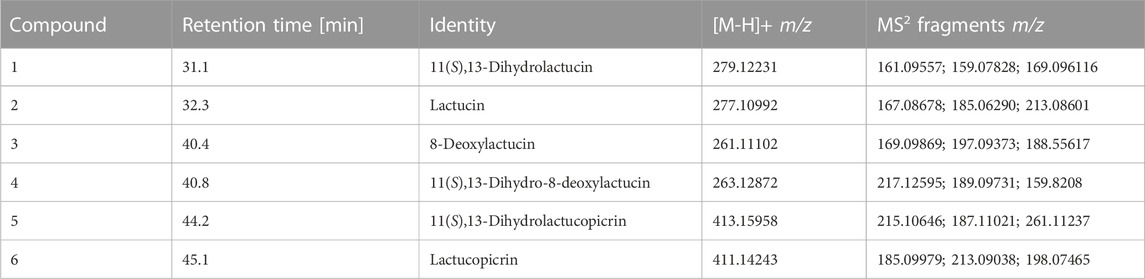
TABLE 5. Mass spectrometric data (protonated molecular ions and fragment ions in MS2) of characteristic sesquiterpene lactones from lettuce (Lactuca sativa L. and Cichorium intybus L.).
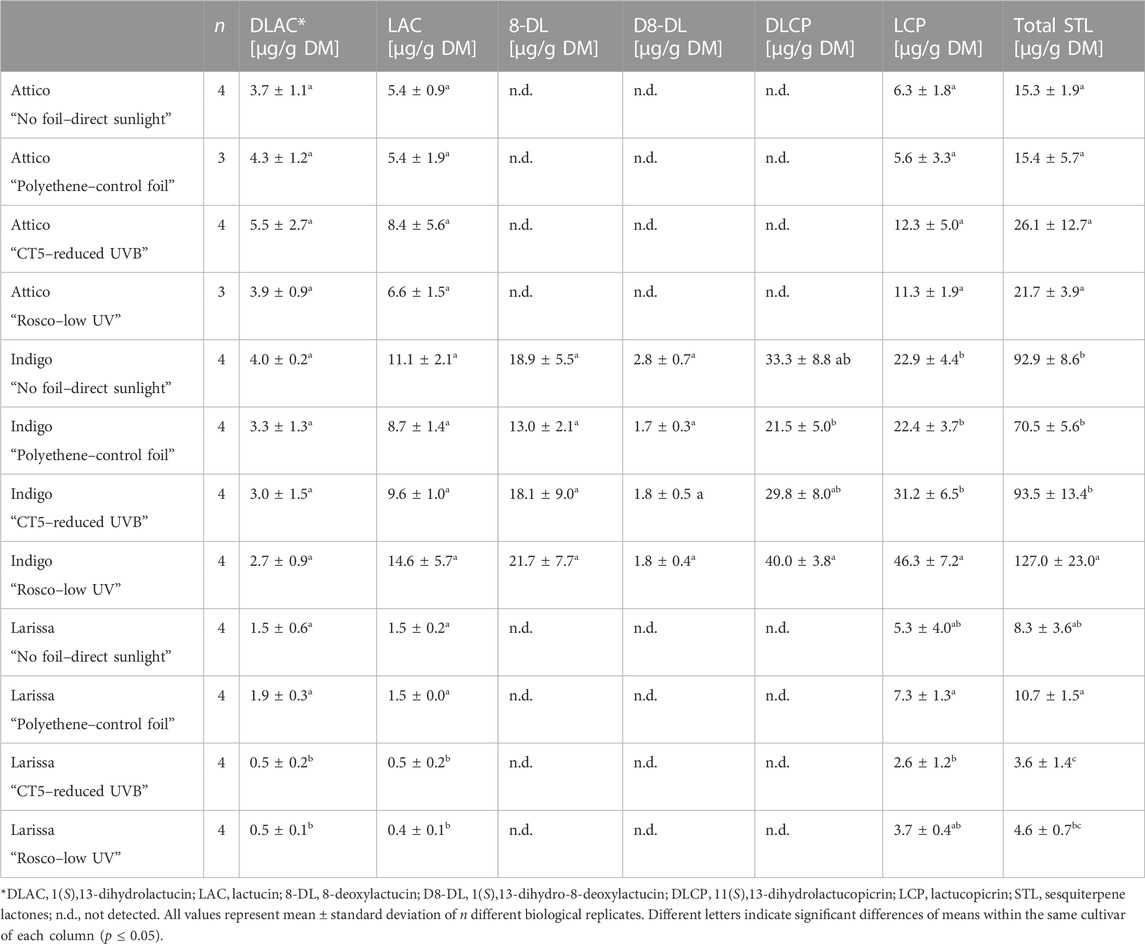
TABLE 6. Sesquiterpene lactone concentrations of three lettuce cultivars (Lactuca sativa L. and Cichorium intybus L.) grown under three different foils, control foil, reduced UVB foil, and low UV foil, compared to direct sunlight.
3.5 Antioxidant activity measured by DPHH, TEAC, and TPC assays
Antioxidant activity, measured by DPPH, was highest in cv. Indigo, ranging between 1.2 ± 0.1 and 1.8 ± 0.8 mmol Trolox equivalent per 100 g FW, while the antioxidant activity of cvs. Larissa and Attico remained at a maximum of 0.8 ± 0.1 and 0.6 ± 0.1 mmol Trolox equivalent per 100 g FW, respectively. It is noteworthy that there were no differences between different treatments of the cv. Indigo, while antioxidant activity was measured highest in samples grown in direct sunlight in cvs. Attico and Larissa. Analogously, the highest antioxidant activity was measured in samples grown in direct sunlight in cv. Larissa, while no differences in cvs. Indigo and Attico were observed in the TEAC assay. The results of the TPC assay were different from those of the used DPPH and TEAC assays. The highest concentrations of 38.6 ± 2.2 to 64.1 ± 7.5 mmol Trolox equivalent per 100 g FW were observed in cv. Attico, being higher than those of most samples of cvs. Indigo and Larissa (up to 16.6 ± 7.5 and up to 37.4 ± 7.2 mmol Trolox equivalent per 100 g FW, respectively). It is noteworthy that within cv. Larissa, antioxidant activity was highest in samples grown under UV- and UVB-shielding foils (Table 7).
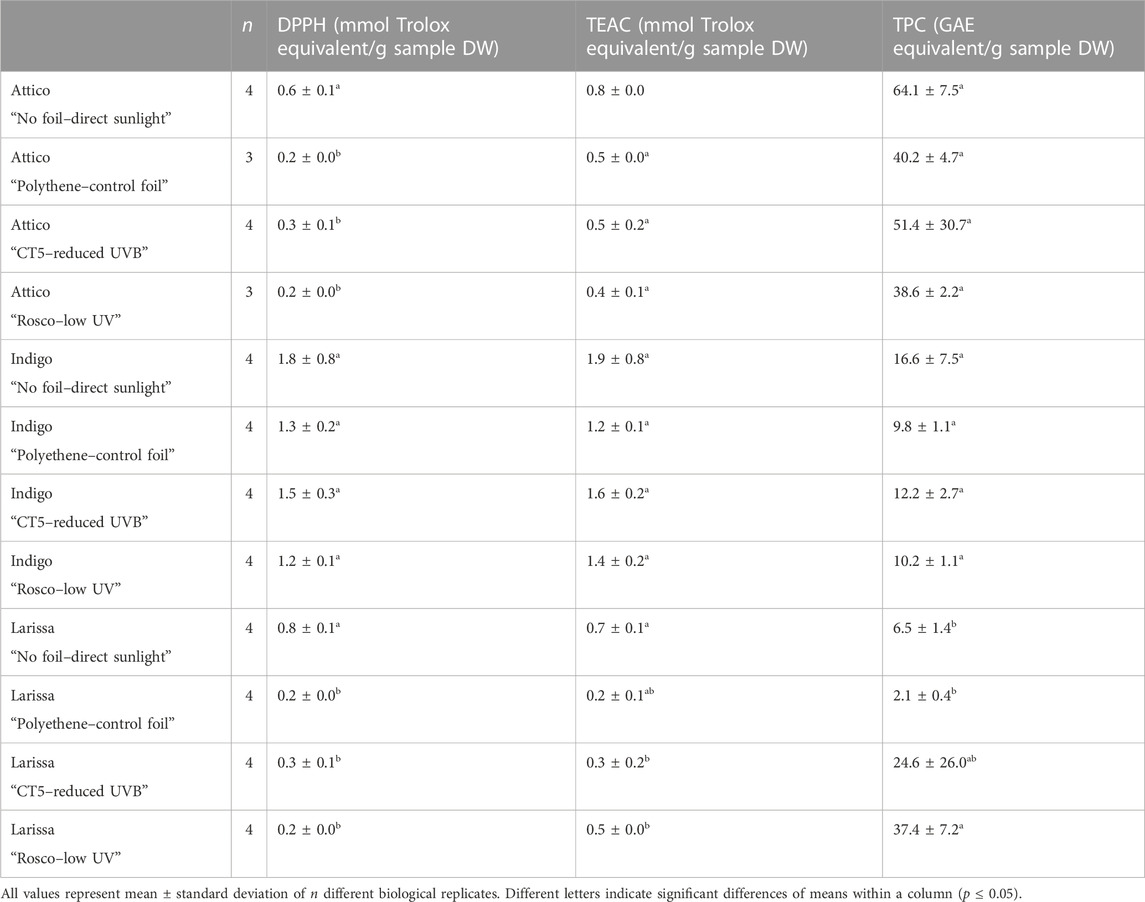
TABLE 7. Antioxidant activity measured by DPPH, TEAC, and TPC assays of three lettuce cultivars (Lactuca sativa L. and Cichorium intybus L.) grown under three different foils, control foil, reduced UVB foil, and low UV foil, compared to direct sunlight.
4 Discussion
4.1 Plant size
Lettuce plants of the cvs. Attico and Larissa were heavier by factors 2 and 4, respectively, when grown under foils compared to plants grown under direct sunlight, clearly indicating the growth advantage of such systems under unfavorable late summer to autumn conditions. The transfer of plants was conducted in August with still high night and day temperatures. However, average temperatures dropped drastically within the short 6-week cultivation period. Especially, night temperatures were below 10°C for most nights during the experiment and did occasionally drop below 5°C. Those plants grown under foils could directly benefit from limited heat loss under foils. However, plants of the cv. Indigo did not differ in weight between the treatments. Comparable observations were made by Yordanova et al. (2021), who found lettuce cultivars to differ in their response to foil treatments with UV-shielding geotextile, green polyethylene, and reinforced and stabilized polyethylene foils during the winter season, with some foils providing an advantage over other foils only for some cultivars. Thus, those foil systems can increase the productivity of lettuce production when facing unfavorable environmental conditions (Santos et al., 2009; Wallace et al., 2012). However, temperature differences between foil treatments and direct sunlight are usually limited to 2°C–3°C (Harbart et al., 2023). During our study, differences in dry matter were widely irrelevant, and contrary results were also published by Santos et al. (2009), who found higher dry matter in lettuce cultivated under foils.
4.2 Carotenoids
The carotenoids β-carotene, lutein, and zeaxanthin were quantitated in lettuce, which are in accordance with Mou (2005), Park et al. (2014), and Burns et al. (2003). The highest concentrations of 951.7 ± 70.5 µg per g DM in cv. Indigo were comparable to those concentrations found by Park et al. (2014), who found up to 856.41 ± 24.12 µg per g DM in green lettuce. Radicchio-type lettuce cv. Indigo clearly surpassed the carotenoid concentrations of butterhead- and romaine-type lettuce cvs. Larissa and Attico. Those cultivar- or type-dependent differences were previously found by Mou (2005), who reported the highest concentrations in cultivated romaine lettuce, followed by red leaf- and butterhead-type lettuce. These findings are in contrast to our results, which were limited to one cultivar per lettuce type. However, a correlation between β-carotene and lutein concentrations was found, both being analogously higher in radicchio-type lettuce Indigo. Concentrations of zeaxanthin were low throughout the experiment and did not differ between cultivars or treatments. Furthermore, only in cv. Larissa, an effect of the treatments was observed for β-carotene. This is in contrast to Caldwell and Britz (2006) and Harbart et al. (2023), who found increased β-carotene, lutein, and neoxanthin concentrations after a supplemental UVA and UVB treatment of 16 lettuce cultivars, and under polytunnel treatment. In contrast to our experiment, Caldwell and Britz (2006) used supplemental light under controlled greenhouse conditions to add UV radiation, while we used outdoor conditions with foils with filtering capacities to reduce UV radiation. Ferrón-Carrillo et al. (2021) also found altered carotenoid concentrations in lettuce after different LED treatments. In a previous study, different shading intensities (based on the cardinal directions) had little to no effect on carotenoids in kale (Bayer et al., 2022). It is noteworthy that Harbart et al. (2023) reported no differences between foil treatments and their control on a transcriptional level (phytoene synthase), suggesting a post-transcriptional regulated effect that increased carotenoid concentrations but was not observed in our study. However, such post-transcriptional regulation might explain the diverse results reported in the literature.
4.3 Phenolic compounds
In agreement with Materska et al. (2019), who provided additional 1H and 13C NMR spectra, compound 1 was tentatively identified as caffeoyltartaric acid, while compound 2 was tentatively identified as caffeoylmalic acid. Compound 3 was tentatively identified, due to the characteristic fragmentation pattern, as chicoric acid (Schütz et al., 2005; Materska et al., 2019). Analogously, compound 4 was tentatively identified as quercetin-3-glucuronide in agreement with Materska et al. (2019), while compound 5 was tentatively identified as kaempferol-3-glucuronide in accordance with Llorach et al. (2008) and Weiland et al. (2023). Compounds 6 and 7, due to their characteristic masses and fragmentation patterns, were tentatively identified as quercetin-3-malonylglucoside and 3-5-dicaffeoylquinic acid (Materska et al., 2019). Phenolic substances in lettuce comprised flavonoid glycosides based on kaempferol, quercetin, and caffeic acid derivatives chicoric acid, caffeoyltartaric acid, caffeoylmalic acid, and dicaffeoylquinic acid. Total phenol concentrations were cultivar-dependent, with radicchio-type lettuce Indigo clearly surpassing the concentrations in butterhead- and romaine-type cvs. Larissa and Attico. Low concentrations of total phenols have previously been reported by Llorach et al. (2008) in romaine-type lettuce. In romaine- and butterhead-type cvs. Attico and Larissa, phenolic acids represented 65% of all phenols, with flavonoids being present in lower proportions. This is in agreement with the findings of Llorach et al. (2008), who found phenolic acids to account for 70% of all phenols in romaine-type cultivars. In radicchio-type lettuce, concentrations of phenolic acids and flavonoids were 50% each. Such differences in phenol composition between lettuce types are common in lettuce, but might favor phenolic acids by up to 95% (Llorach et al., 2008; Becker et al., 2013). Concentrations were overall comparable with concentrations found in other research studies (Llorach et al., 2008). Important and highly concentrated phenolic substances were chicoric acid, quercetin-3-glucuronide, and quercetin-3-malonylglucoside (Table 4), while Romani et al. (2002) found additionally high concentrations of chlorogenic acid. In the same study, Romani et al. (2002) reported an increased flavonoid concentration in open-air cultivated lettuce in comparison to greenhouse-grown lettuce. This is in agreement with our findings, where relevant phenolic substances, such as chicoric acid, quercetin-3-glucuronide, and quercetin-3-malonylglucoside, were higher when grown under direct sun exposure in all cultivars. Furthermore, Harbart et al. (2023) found a more than 2-fold decrease in flavonoids when growing lettuce under foils with or without an antifog additive, which was due to a decrease in quercetin glycoside concentrations. Behn et al. (2010) also found increased quercetin concentrations in lettuce plants grown under additional UVB light. It is noteworthy that those increased quercetin concentrations were limited to cultivation periods where different UVB conditions were applied and vanished after the treatment. The lower concentrations of quercetin derivatives under UV-shielding foils are in agreement with previous findings that UVB radiation enhances the accumulation of those polyhydroxylated flavonoids (Neugart and Schreiner, 2018; Assumpção et al., 2019). However, concentrations under UVB-shielding foils were higher and lowest under UV-shielding foils. This might be explained by the actual proportional UV light reduction of the used foil. UVB-shielding foils reduced UVB radiation to 23% of the original terrestrial conditions, while UVA was transmitted to 66%. However, the UV-shielding foil was more effective against both wavelengths, blocking almost all UV light (Table 1). However, monohydroxylated kaempferol flavonoids were also found in elevated concentrations under UVB-shielding foils and were reported to be less affected by UVB radiation (Neugart and Schreiner, 2018; Weiland et al., 2023). It is noteworthy that, within cvs. Attico and Larissa, differences were only observed for chicoric acid, which was more concentrated in direct sunlight. However, within cv. Indigo, differences in concentrations were observed for chichoric acid only in plants grown under UV-shielding foils. Consequently, these results are only partly in agreement with those of Assumpção et al. (2019), who found an accumulation of caffeoyltartaric acid, caffeoylquinic acid, and caffeoylmalic acid after additional LED-emitted UVB exposure. However, the used foils were generally efficient in absorbing UVB light but were not able to block all UV radiation (Table 1). Additionally, the results suggest that some cultivars, presumably those low in polyphenols, are not affected by UV-blocking foils. In general, concentrations of phenolic substances were reduced by 20%–60% by applying foils. Thus, to achieve high concentrations of phenolic substances under foils, suitable and partly UV-transmissive foils should be used for some cultivars. However, acquiring special foils to specifically allow certain wavelengths to pass through can be quite challenging and may not be specific enough to target only phenolic substances. For scientific research, however, this uncertainty is a clear disadvantage against indoor experiments with targeted LED applications. The clear response of polyphenols under foil treatment is due to a reduced expression of chalcone synthase in lettuce, which was recently reported by Harbart et al. (2023), also leading to reduced concentrations of phenolic substances.
4.4 Sesquiterpene lactones (STLs)
In agreement with Wulfkuehler et al. (2013), all STLs were tentatively identified according to their characteristic protonated molecular ion and their fragment ions (Table 5). Additionally, mass spectrometric data were compared to the results of Sessa et al. (2000), who additionally confirmed lactucin and compound lactucopicrin by 1H NMR data. Sesquiterpene lactone concentrations distinguished clearly between sesquiterpene lactone-rich radicchio-type lettuce Indigo, reaching up to 127.0 ± 23.0 µg per g DM, with a reputed bitter taste, and mild romaine- and butterhead-type lettuce cvs. Attico and Larissa, reaching only concentrations below 30 µg per g DM. Those differences are in accordance with those found by Seo et al. (2009), who found 14.6–67.7 µg per g DM STLs. The most prominent STLs were 11(S),13-dihydrolactucopicrin, lactucopicrin, and lactucin in cv. Indigo and lactucopicrin and lactucin in cvs. Attico and Larissa in accordance with Abu-Reidah et al. (2013). Most notably, STL concentrations in cv. Indigo were highest under UV-shielding foils. This effect was due to a high concentration of 11(S),13-dihydrolactucopicrin and lactucopicrin. Analogously, lactucin concentrations were not affected by foliar treatment. Thus, there was a shift in overall STL composition from lactucin toward lactucopicrin dependent on reduced UVB radiation. This observation was observed in all three cultivars. However, romaine-type cv. Attico was not affected by its total STL concentration. Thus, the effects of our treatments were mainly observed when cultivars with a high STL concentration were used. Within cv. Indigo, UV-shielding foils were more effective in increasing STL concentrations, and 11(S),13-dihydrolactucopicrin and lactucopicrin in particular, than UVB-shielding foils. It is noteworthy that UV-shielding foils were most effective in shielding UVA and UVB radiation. Thus, those results suggest a negative correlation between UV radiation and lactucopicrin and its derivatives. Reports on the influence of light conditions and UV radiation, in particular, are rare in the literature. However, Kitazaki et al. (2018) reported an increase in several STLs after exposing lettuce to green light, and Scavo et al. (2020) reported manifold increases in STL concentrations in cardoon leaf extracts (Cynara cardunculus L.) after shading. In agreement with our phenolic substance results, an exposure to artificial UV light under indoor conditions might be favorable. For sensory experience of lettuce, such differences between shading and an open field can result in an increased bitterness (Zhao and Carey, 2009) and need to be addressed in sensory studies in the future.
4.5 Antioxidant activity
Antioxidant activity was comparable to the previously found results for phenolic substances. The phenol-rich cv. Indigo also had higher DPPH and TEAC values than the phenol-poor cvs. Attico and Larissa. Reduced antioxidant activity was observed across varieties based on reduced light intensity during the foil treatment, as a direct consequence of the reduced concentrations of polyphenols. This is in agreement with Samuolienė et al. (2013), who found increased antioxidant activity after exposure to supplemental UV light, while we found decreasing antioxidant activities after reducing the UV light dose by foil treatments. However, the TPC assays differed from our DPPH and TEAC assays by displaying extremely low values for cultivar Indigo. Similar results were also reported by Sutulienė et al. (2022), who found higher antioxidant activity in DPPH, ABTS, and FRAP assays, while the results of TPC assays were less conclusive. Presumably, other substances, such as STLs, might interfere with this rather unspecific assay.
5 Conclusion
The application of foil tunnels is a widely accepted method of prolonging cultivation periods for different horticultural crops. We could show that these foils influence health-related phenolic substances and taste-relevant STLs, while carotenoids remained widely unaffected. Furthermore, we could show that light conditions are important for STL accumulation in lettuce and that UVB-shielding foils can increase STL concentrations, namely, lactucopicrin concentrations. While the results and metabolic explanations for phenolic substances are quite clear, for carotenoids, there are still contradictory results and open research questions regarding their accumulation under different light conditions. Sesquiterpene lactones, however, are underrepresented in this field of research. For more practical applications, foils with a specific transmission potential for physiologically active wavelengths of the terrestrial spectrum are still limited, and foils suitable for agriculture are lacking. Therefore, the application of supplemental and LED-emitted light might be more suitable for scientific research. Those findings might be of significance for researchers working on consumer acceptance, insect repellent effects of STLs, and health-beneficial effects of STLs in the focus of growing indoor cultivation and foil-protected agriculture.
Data availability statement
The original contributions presented in the study are included in the article/Supplementary Material, further inquiries can be directed to the corresponding author.
Author contributions
TP and SN designed the study. TP and NL handled field samples and set up the analytical methods. NL conducted the lab work. TP and NL analyzed the results with help from SN. MZ supported NL with statistical analysis and supervision. NL and TP prepared the manuscript. MZ and SN assisted with the discussion. All authors contributed to the article and approved the submitted version.
Funding
The stay of NL at the Georg-August-University Göttingen was supported by the Erasmus+ programme of the European Union and did not include work materials or laboratory expenses of any kind.
Acknowledgments
The authors want to thank Layla Engelhard for providing them with the methods for antioxidant activities and Ulf Jäger for the support with HPLC measurements. We acknowledge support by the Open Access Publication Funds of the Göttingen University.
Conflict of interest
The authors declare that the research was conducted in the absence of any commercial or financial relationships that could be construed as a potential conflict of interest.
Publisher’s note
All claims expressed in this article are solely those of the authors and do not necessarily represent those of their affiliated organizations, or those of the publisher, the editors, and the reviewers. Any product that may be evaluated in this article, or claim that may be made by its manufacturer, is not guaranteed or endorsed by the publisher.
Supplementary material
The Supplementary Material for this article can be found online at: https://www.frontiersin.org/articles/10.3389/frfst.2023.1208100/full#supplementary-material
References
Abu-Reidah, I. M., Contreras, M. M., Arráez-Román, D., Segura-Carretero, A., and Fernández-Gutiérrez, A. (2013). Reversed-phase ultra-high-performance liquid chromatography coupled to electrospray ionization-quadrupole-time-of-flight mass spectrometry as a powerful tool for metabolic profiling of vegetables: lactuca sativa as an example of its application. J. Chromatogr. A 1313, 212–227. doi:10.1016/j.chroma.2013.07.020
Assumpção, C. F., Assis, R. Q., Hermes Poletto, V. S., Castagna, A., Ranieri, A., Neugart, S., et al. (2019). Application of supplemental UV-B radiation in pre-harvest to enhance health-promoting compounds accumulation in green and red lettuce. J. Food Process. Preserv. 43, 14213. doi:10.1111/jfpp.14213
Bayer, M., Neugart, S., and Pöhnl, T. (2022). Influence of urban gardening conditions on the concentration of antioxidant secondary plant metabolites in kale. J. Appl. Bot. Food Qual. 95, 85–93. doi:10.5073/JABFQ.2022.095.011
Becker, C., Kläring, H. P., Kroh, L. W., and Krumbein, A. (2013). Temporary reduction of radiation does not permanently reduce flavonoid glycosides and phenolic acids in red lettuce. Plant Physiol. biochem. 72, 154–160. doi:10.1016/j.plaphy.2013.05.006
Behn, H., Tittmann, S., Walter, A., Schurr, U., Noga, G., and Ulbrich, A. (2010). UV-B transmittance of greenhouse covering materials affects growth and flavonoid content of lettuce seedlings. Eur. J. Hortic. Sci. 75, 259–268.
Burns, J., Fraser, P. D., and Bramley, P. M. (2003). Identification and quantification of carotenoids, tocopherols and chlorophylls in commonly consumed fruits and vegetables. Phytochemistry 62, 939–947. doi:10.1016/S0031-9422(02)00710-0
Caldwell, C. R., and Britz, S. J. (2006). Effect of supplemental ultraviolet radiation on the carotenoid and chlorophyll composition of green house-grown leaf lettuce (Lactuca sativa L) cultivars. J. Food Compost. Anal. 19, 637–644. doi:10.1016/j.jfca.2005.12.016
Chadwick, M., Gawthrop, F., Michelmore, R. W., Wagstaff, C., and Methven, L. (2016). Perception of bitterness, sweetness and liking of different genotypes of lettuce. Food Chem. 197, 66–74. doi:10.1016/j.foodchem.2015.10.105
Chadwick, M., Trewin, H., Gawthrop, F., and Wagstaff, C. (2013). Sesquiterpenoids lactones: benefits to plants and people. Int. J. Mol. Sci. 14, 12780–12805. doi:10.3390/ijms140612780
Chen, Y., Li, T., Yang, Q., Zhang, Y., Zou, J., Bian, Z., et al. (2019). UVA radiation is beneficial for yield and quality of indoor cultivated lettuce. Front. Plant Sci. 10, 1563. doi:10.3389/fpls.2019.01563
Engelhardt, L., Pöhnl, T., and Neugart, S. (2021). Interactions of ascorbic acid, 5-caffeoylquinic acid, and quercetin-3-rutinoside in the presence and absence of iron during thermal processing and the influence on antioxidant activity. Molecules 26, 7698. doi:10.3390/molecules26247698
Ferrón-Carrillo, F., Guil-Guerrero, J. L., González-Fernández, M. J., Lyashenko, S., Battafarano, F., da Cunha-Chiamolera, T. P. L., et al. (2021). LED enhances plant performance and both carotenoids and nitrates profiles in lettuce. Plant Foods Hum. Nutr. 76, 210–218. doi:10.1007/s11130-021-00894-8
Food and Agriculture Organization of the United Nations (1997). FAOSTAT statistical database. [Rome]: FAO.
Harbart, V., Frede, K., Fitzner, M., and Baldermann, S. (2023). Regulation of carotenoid and flavonoid biosynthetic pathways in Lactuca sativa var capitate L. in protected cultivation. Front. Plant Sci. 14, 1124750. doi:10.3389/fpls.2023.1124750
Heijde, M., and Ulm, R. (2012). UV-B photoreceptor-mediated signalling in plants. Trends Plant Sci. 17, 230–237. doi:10.1016/j.tplants.2012.01.007
Jenkins, G. I. (2009). Signal transduction in responses to UV-B radiation. Annu. Rev. Plant Biol. 60, 407–431. doi:10.1146/annurev.arplant.59.032607.092953
Kim, M. J., Moon, Y., Tou, J. C., Mou, B., and Waterland, N. L. (2016). Nutritional value, bioactive compounds and health benefits of lettuce (Lactuca sativa L). J. Food Compost. Anal. 49, 19–34. doi:10.1016/j.jfca.2016.03.004
Kitazaki, K., Fukushima, A., Nakabayashi, R., Okazaki, Y., Kobayashi, M., Mori, T., et al. (2018). Metabolic reprogramming in leaf lettuce grown under different light quality and intensity conditions using narrow-band LEDs. Sci. Rep. 8, 7914. doi:10.1038/s41598-018-25686-0
Kotilainen, T., Robson, T. M., and Hernández, R. (2018). Light quality characterization under climate screens and shade nets for controlled-environment agriculture. PLoS ONE 13, e0199628. doi:10.1371/journal.pone.0199628
Leri, M., Scuto, M., Ontario, M. L., Calabrese, V., Calabrese, E. J., Bucciantini, M., et al. (2020). Healthy effects of plant polyphenols: molecular mechanisms. Int. J. Mol. Sci. 21, 1250. doi:10.3390/ijms21041250
Llorach, R., Martínez-Sánchez, A., Tomás-Barberán, F. A., Gil, M. I., and Ferreres, F. (2008). Characterisation of polyphenols and antioxidant properties of five lettuce varieties and escarole. Food Chem. 108, 1028–1038. doi:10.1016/j.foodchem.2007.11.032
Materska, M., Olszówka, K., Chilczuk, B., Stochmal, A., Pecio, Ł., Pacholczyk-Sienicka, B., et al. (2019). Polyphenolic profiles in lettuce (Lactuca sativa L) after CaCl2 treatment and cold storage. Eur. Food Res. Technol. 245, 733–744. doi:10.1007/s00217-018-3195-0
Mou, B. (2005). Genetic variation of beta-carotene and lutein contents in lettuce. J. Am. Soc. Hortic. 130, 870–876. doi:10.21273/JASHS.130.6.870
Neugart, S., and Schreiner, M. (2018). UVB and UVA as eustressors in horticultural and agricultural crops. Sci. Hortic. 234, 370–381. doi:10.1016/j.scienta.2018.02.021
Park, S. Y., Choi, S. R., Lim, S. H., Yeo, Y., Kweon, S. J., Bae, Y. S., et al. (2014). Identification and quantification of carotenoids in paprika fruits and cabbage, kale, and lettuce leaves. J. Korean Soc. Appl. Biol. Chem. 57, 355–358. doi:10.1007/s13765-014-4081-5
R Core Team (2019). R: A language and environment for statistical computing. Vienna, Austria: R Core Team.
Robson, T. M., Pieristè, M., Durand, M., Kotilainen, T. K., and Aphalo, P. J. (2022). The benefits of informed management of sunlight in production greenhouses and polytunnels. Plants People Planet 4, 314–325. doi:10.1002/ppp3.10258
Romani, A., Pinelli, P., Galardi, C., Sani, G., Cimato, A., and Heimler, D. (2002). Polyphenols in greenhouse and open-air-grown lettuce. Food Chem. 79, 337–342. doi:10.1016/S0308-8146(02)00170-X
Samuolienė, G., Brazaitytė, A., Sirtautas, R., Viršilė, A., Sakalauskaitė, J., Sakalauskienė, S., et al. (2013). LED illumination affects bioactive compounds in romaine baby leaf lettuce. J. Sci. Food Agric. 93, 3286–3291. doi:10.1002/jsfa.6173
Santos, B., Lobato, A., Da Silva, R., Schimidt, D., Costa, R., Alves, G., et al. (2009). Growth of lettuce (Lactuca sativa L) in protected cultivation and open field. J. Appl. Sci. Res. 5, 529–533.
Scavo, A., Rial, C., Molinillo, J. M. G., Varela, R. M., Mauromicale, G., and Macı As, F. A. (2020). Effect of shading on the sesquiterpene lactone content and phytotoxicity of cultivated cardoon leaf extracts. J. Agric. Food Chem. 68, 11946–11953. doi:10.1021/acs.jafc.0c03527
Schütz, K., Kammerer, D. R., Carle, R., and Schieber, A. (2005). Characterization of phenolic acids and flavonoids in dandelion (Taraxacum officinale WEB. ex WIGG) root and herb by high-performance liquid chromatography/electrospray ionization mass spectrometry. Rapid Commun. Mass Spectrom. 19, 179–186. doi:10.1002/rcm.1767
Seo, M. W., Yang, D. S., Kays, S. J., Lee, G. P., and Park, K. W. (2009). Sesquiterpene lactones and bitterness in Korean leaf lettuce cultivars. HortScience 44, 246–249. doi:10.21273/HORTSCI.44.2.246
Sessa, R. A., Bennett, M. H., Lewis, M. J., Mansfield, J. W., and Beale, M. H. (2000). Metabolite profiling of sesquiterpene lactones from Lactuca species. J. Biol. Chem. 275, 26877–26884. doi:10.1074/jbc.M000244200
Sutulienė, R., Laužikė, K., Pukas, T., and Samuolienė, G. (2022). Effect of light intensity on the growth and antioxidant activity of sweet basil and lettuce. Plants 11, 1709. doi:10.3390/plants11131709
Theurl, M. C., Haberl, H., Erb, K. H., and Lindenthal, T. (2014). Contrasted greenhouse gas emissions from local versus long-range tomato production. Agron. Sustain. Dev. 34, 593–602. doi:10.1007/s13593-013-0171-8
Theurl, M. C., Hörtenhuber, S. J., Lindenthal, T., and Palme, W. (2017). Unheated soil-grown winter vegetables in Austria: greenhouse gas emissions and socio-economic factors of diffusion potential. J. Clean. Prod. 151, 134–144. doi:10.1016/j.jclepro.2017.03.016
Wallace, R. W., Wszelaki, A. L., Miles, C. A., Cowan, J. S., Martin, J., Roozen, J., et al. (2012). Lettuce yield and quality when grown in high tunnel and open-field production systems under three diverse climates. Horttechnology 22, 659–668. doi:10.21273/HORTTECH.22.5.659
Weiland, M., Weßler, C. F., Filler, T., Glaab, J., Ploch, N. L., Winterwerber, U., et al. (2023). A comparison of consistent UV treatment versus inconsistent UV treatment in horticultural production of lettuce. Photochem. Photobiol. Sci. 22, 1611–1624. doi:10.1007/s43630-023-00402-8
Wulfkuehler, S., Gras, C., and Carle, R. (2013). Sesquiterpene lactone content and overall quality of fresh-cut witloof chicory (Cichorium intybus L. var. foliosum Hegi) as affected by different washing procedures. J. Agric. Food Chem. 61, 7705–7714. doi:10.1021/jf402189v
Yordanova, M., Petrova, V., and Kirilov, D. (2021). Evaluation of the different type of tunnels coverings applied at lettuce cultivation. Sci. Pap. Ser. B Hortic. LXV, 590–598.
Zhang, M., Whitman, C. M., and Runkle, E. S. (2019). Manipulating growth, color, and taste attributes of fresh cut lettuce by greenhouse supplemental lighting. Sci. Hortic. 252, 274–282. doi:10.1016/j.scienta.2019.03.051
Keywords: lettuce, sesquiterpene lactones, polyphenols, carotenoids, UV radiation, foil tunnels, light conditions
Citation: Laurenčíková N, Živčák M, Neugart S and Pöhnl T (2023) Influence of UV radiation-absorbing foils on secondary plant metabolites in three lettuce cultivars (Lactuca sativa L. and Cichorium intybus L.). Front. Food. Sci. Technol. 3:1208100. doi: 10.3389/frfst.2023.1208100
Received: 24 April 2023; Accepted: 10 August 2023;
Published: 14 September 2023.
Edited by:
Rama Chandra Pradhan, National Institute of Technology Rourkela, IndiaReviewed by:
Maria Dulce Carlos Antunes, University of Algarve, PortugalHua Zhou, Jiangxi Academy of Sciences, China
Copyright © 2023 Laurenčíková, Živčák, Neugart and Pöhnl. This is an open-access article distributed under the terms of the Creative Commons Attribution License (CC BY). The use, distribution or reproduction in other forums is permitted, provided the original author(s) and the copyright owner(s) are credited and that the original publication in this journal is cited, in accordance with accepted academic practice. No use, distribution or reproduction is permitted which does not comply with these terms.
*Correspondence: Tobias Pöhnl, dG9iaWFzLnBvZWhubEB1bmktZ29ldHRpbmdlbi5kZQ==