- 1Department of Pulmonary Medicine, Hospital Clínic, University of Barcelona, Barcelona, Spain
- 2Fundació de Recerca Clínic Barcelona-Institut d'Investigacions Biomèdiques August Pi i Sunyer (IDIBAPS), Barcelona, Spain
- 3Department of Physical Therapy, Faculty of Medicine, University of Chile, Santiago, Chile
- 4Centro Médico Familiar Integral y Especialidades Diálisis “LA MARISCAL”, Instituto Ecuatoriano de Seguridad Social, Quito, Ecuador
- 5Programa de Doctorat-Facultat Ciències de la Salut Blanquerna, Universitat Ramon Llull, Barcelona, Spain
- 6Barcelona Institute for Global Health (ISGlobal), Barcelona, Spain
- 7Biomedical Research Networking Center on Respiratory Diseases, CIBERES, Madrid, Spain
- 8Departamento de Apoyo en Rehabilitación Cardiopulmonar Integral, Instituto Nacional del Tórax, Santiago, Chile
- 9Exercise and Rehabilitation Sciences Institute, Faculty of Rehabilitation Sciences, Universidad Andres Bello, Santiago, Chile
- 10INTRehab Research Group, Instituto Nacional del Tórax, Santiago, Chile
- 11Departamento de Ciencias de la Rehabilitación, Facultad de Medicina, Universidad de La Frontera, Temuco, Chile
- 12Centro de Excelencia CIGES, Universidad de La Frontera, Temuco, Chile
- 13Department of Research and Development, Ciro, Horn, Netherlands
- 14Department of Respiratory Medicine, School of Nutrition and Translational Research in Metabolism, Faculty of Health Medicine and Life Sciences, Maastricht University Medical Centre, Maastricht, Netherlands
- 15ERN-LUNG (European Reference Network on Rare Respiratory Diseases), Spain
- 16Blanquerna School of Health Sciences, Global Research on Wellbeing (GRoW), Universitat Ramon Llull, Barcelona, Spain
Introduction: There has been inconclusive findings regarding the effectiveness of inspiratory muscle training (IMT) in chronic respiratory diseases (CRDs). Our objective was to determine the effectiveness of IMT on exercise tolerance, maximum respiratory pressure, lung function, symptoms and quality of life in different CRDs.
Methods: We conducted an overview of systematic reviews (SRs) in adults with CRDs who underwent IMT. We reviewed five databases in March 2025. We chose the most comprehensive SRs to report on the analysed outcomes.
Results: Twenty-three SRs were included. In chronic obstructive pulmonary disease (COPD), IMT increased the six-minute walk distance (6MWD) by 35.7 m (95% CI 25.7, 45.7), maximum inspiratory pressure (MIP) by 10.9 cmH2O (95% CI 8.0, 13.9). In asthma, IMT increased the forced expiratory volume in the first second (FEV1) by 3.3%pred (95% CI 1.4, 5.1), forced vital capacity (FVC) by 4.1%pred (95% CI 1.0, 7.3), MIP by 21.9 cmH2O (95% CI 15.0, 28.8), and dyspnoea was reduced (standard mean difference −0.8, 95% CI −1.3,−0.2). In obstructive sleep apnoea (OSA), IMT increased MIP by 29.6 cmH2O (95% CI 6.0, 53.1). In pulmonary hypertension (PH), IMT increased 6MWD by 39.0 m (95% CI 20.7, 57.4), MIP in 21.2 cmH2O (95% CI 11.3, 31.1), maximum expiratory pressure by 14.4 cmH2O (95% CI 6.9, 21.9), and dyspnoea was reduced by 0.5 (95% CI 0.1, 0.9) in modified Medical Research Council scale. In lung resection (LR), IMT increased MIP by 8.1 cmH2O (95% CI 1.3, 14.9). In bronchiectasis, IMT increased MIP by 6.1 cmH2O (95% CI 1.4, 10.8). Overall, the most consistent effect of IMT across different CRDs was an increase in MIP.
Conclusion: IMT improved several clinically relevant outcomes, including MIP, exercise capacity, and dyspnoea in different CRDs. However, the limited evidence for certain outcomes and populations highlights the need for further high-quality studies.
Introduction
Chronic respiratory diseases (CRDs) are a major public health problem, with nearly 4 million deaths in 2017, accounting for 7% of all deaths worldwide (1). Some of the most common CRDs are asthma, chronic obstructive pulmonary disease (COPD), and occupational lung diseases (2).
Individuals with CRDs often experience limitations in exercise tolerance due to a combination of factors, including alterations in ventilation and gas exchange, cardiovascular comorbidities, and peripheral muscle abnormalities (3). They also commonly have inspiratory muscle dysfunction, which is linked to symptoms such as dyspnoea and reduced exercise capacity (4). The causes of respiratory muscle dysfunction in CRDs patients are diverse and vary depending on the specific disease. For example, static lung hyperinflation in patients with COPD or asthma (5), and reduced lung compliance in patients with interstitial lung diseases (ILD) (6). both contributed to an impaired ability to meet the increased ventilatory demands during physical activity (7). In these conditions, the respiratory system operates outside of the ideal pressure-volume relationship, compromising the force-generating capacity of the inspiratory muscles (7). CRDs pose distinct physiological challenges that impact exercise tolerance and overall respiratory function. In conditions like COPD and pulmonary hypertension (PH), the reduced capacity to generate adequate respiratory muscle force impairs ventilation, thereby limiting exercise capacity (8–10).
Inspiratory muscle training (IMT), defined as a rehabilitative intervention that strengthens the inspiratory muscles through the use of inspiratory threshold loading devices that provide a fixed resistance to inhalation (11), has emerged as a promising intervention, enhancing aerobic capacity by strengthening the respiratory muscles, exercise capacity is improved due to the potential increases thoracic expansion and operating lung volumes reducing dynamic hyperinflation, and to the improved work of breathing of the primary inspiratory muscles (diaphragm), thereby reducing respiratory muscle fatigue, dyspnea, and attenuating the concomitant 'stealing' effect of blood flow from the exercising limb to the respiratory muscles (12–14).
Most studies explaining the potential mechanisms of IMT have been conducted in healthy subjects. These studies have shown that IMT can: (1) decrease the inspiratory muscle motor drive while preserving pressure generation; (15) (2) promote hypertrophy of the diaphragm and increase the proportion of type I fibres and the size of type II fibres in the external intercostal muscles; (16) (3) decrease the rating of perceived dyspnoea or rating of perceived exertion; (17) and (4) improve respiratory muscle endurance (18).
These physiological findings provide a foundation for understanding how IMT can benefit individuals with CRDs, but they also underscore the need for further research to better define its impact across different disease stages and types. However, there have been inconclusive findings regarding the effectiveness of IMT in improving exercise tolerance in patients with CRDs (19). Our objective was to determine the effectiveness of IMT on exercise tolerance, maximum respiratory pressure, lung function, symptoms and quality of life in different CRDs.
Methods
Protocol and registration
We performed an overview of systematic reviews (SRs) according to the methodology proposed by the Cochrane Handbook for Systematic Reviews of Interventions (20). This overview was reported using the preferred reporting items for overviews of reviews (PRIOR) statement (21). The review was registered in the International Prospective Register of Systematic Reviews (PROSPERO) with the identifier CRD42022350564.
Eligibility criteria
We included SRs that focused on interventions with or without meta-analysis, which considered primary studies with a randomised controlled trials (RCTs) design. Network meta-analyses were excluded. The studies included in must have involved adults with CRDs. Furthermore, only SRs that examined IMT interventions, excluding pulmonary rehabilitation, were considered. Regarding comparators, we included SRs where control groups in the primary studies received usual care, placebo, or sham treatments. Lastly, we focused on SRs that addressed the effectiveness of IMT interventions in at least one of the following outcomes: exercise tolerance, pulmonary function, maximum inspiratory or expiratory pressure, dyspnoea, or quality of life.
Search strategies and data resources
We reviewed five databases: Embase, PubMed/MEDLINE, Web of Science, Cochrane Central Register of Controlled Trials Cochrane Database of Systematic Reviews (CDSR), and Epistemonikos from their inception to March 8, 2025. We imposed no language or publication restrictions. See Supplementary Material S1.
The terms selected were combined using Boolean logical operators (OR, AND, NOT). Moreover, we did a manual search of the references that were included in the selected articles.
Study selection
Two investigators (RTC-SCT) performed the selection independently. The first step involved reviewing the titles and abstracts of all the references retrieved by the database searches (RTC-SCT) and identifying the SRs that met the inclusion criteria. Next, we selected all articles deemed potentially eligible by at least one of the reviewers. In the second step, we reviewed the full texts, and a decision on inclusion or exclusion was made according to the predefined selection criteria (RTC-SCT). A third reviewer (LVC) solved any disagreement in any step. We excluded studies that did not fulfil the inclusion criteria, and their bibliographic details were listed with the specific reason for exclusion.
Data extraction and methodological quality assessment
Two authors (RTC-SCT) extracted the data independently and in duplicate, using a standardised protocol and reporting forms. The following information was extracted from each included study: bibliometric characteristics of the publication, general characteristics of the SRs, reported outcome data, quality, or risk of bias of the primary studies included, and certainty of evidence. In addition, the methodological quality of the included SRs was rated in this form.
Two reviewers (RTC-SCT) independently assessed the methodological quality of the SRs included in this overview using “A MeaSurement Tool to Assess systematic Reviews 2” (AMSTAR 2) (22). Disagreements were resolved by consensus or by a third reviewer (LVC). SRs were classified according to the overall confidence in their results as High (no or one non-critical weakness), Moderate (more than one non-critical weakness), Low (one critical flaw with or without non-critical weaknesses), and Critically Low (more than one critical flaw with or without non-critical weaknesses).
Certainty of the evidence
We used the reported data from one SR chosen for each outcome (“best” SR) to determine the certainty of the evidence using the “Grades of Recommendation, Assessment, Development, and Evaluation” (GRADE) framework (23). Study limitations, inconsistency of results, indirectness of evidence, imprecision, and reporting bias are assessed by the GRADE approach.
Data analysis
The unit of analysis of this overview was the SRs. Therefore, the primary studies included by each SR were not accessed in case of missing data. Due to the possible existence of redundant SRs, strategies were applied to visualise (24), calculate (25), and manage the overlap (26). In the first instance, a matrix was created with cross-references of the SRs included in this overview with the primary studies included by these SRs. This was done at the outcome level. In addition, from these matrices, the corrected covered area (CCA) (25) were calculated without considering any structural missing data and considering the chronological structural missing data and by primary study design. The Graphical Representation of Overlap for OVErviews (GROOVE) tool was used (27). To select the “best” SR for reporting the effectiveness of each outcome, we prioritise the SR that contains the highest number of primary studies included, rated with a higher rating in their results according to AMSTAR 2.
Results
Study selection
From the 608 identified references, we removed 291 duplicated and screened 317 SRs. We had 71 SRs assessed based on the full text. We excluded 21 SRs for other intervention, 15 for other study design, seven for other population, four for other publication type, and two SRs for being conference abstracts. Finally, 23 SRs were included (Figure 1).
Characteristics of the included SRs
We included ten SRs in patients with COPD (28–37), five in patients with asthma (17, 38–41), four with obstructive sleep apnoea (OSA) patients (42–45), two with PH (46, 47), one in patients with lung resection (48), and one in patients with bronchiectasis (49). Out of the 23 SRs, 21 were SRs with meta-analysis, and two did not pool outcome data from primary studies (Table 1).
Risk of bias (RoB) assessment
Only two of the SRs was rated as high quality (37, 46), while six were rated as low quality (38, 40, 42–45) and 14 were rated critically low quality (17, 28–36, 39, 41, 47–49). If we analysed by publication years, 90% of 10 articles published before 2020 was critically low quality; indeed, in 13 articles after 2020, only 50% was critically low. The items that the authors failed to answer the most were: (10) Did the review authors report on the funding sources for the studies included in the review? (4.3%), (15) If they performed quantitative synthesis, did the review authors carry out an adequate investigation of publication bias (small study bias) and discuss its likely impact on the results of the review? (8.6%), and (13) Did the review authors account for RoB in individual studies when interpreting/discussing the results of the review? (39.3%) (Table 2). The RoB of each SR reported by the authors is in Supplementary Material S2.
Main findings
COPD
We selected ten SRs, eight of them performed meta-analyses. All SRs reported exercise capacity and MIP as outcomes, nine reported dyspnoea and quality of life, seven reported respiratory resistance, and six reported lung function. There was a very high overlap between the SRs in exercise tolerance and MIP, high overlap in forced expiratory volume in the first second (FEV1), dyspnoea and quality of life, and moderate overlap in forced vital capacity (FVC) (Table 3). IMT increased exercise tolerance by 35.7 (95% CI 25.7, 45.7) metres in the 6MWT (37), FEV1 by 2.6%pred (95% CI 0.2, 5.0), and MIP by 10.9 cmH2O (95% CI 8.0, 13.9) (37). Dyspnoea was reduced by 0.6 (95% CI 0.4, 0.8) points in the Borg Scale and 0.9 (95% CI 0.51–1.36) points in modified Medical Research Council (mMRC), and quality of life was improved by 3 (95% CI 2.1, 3.9) points in COPD assessment test (CAT), but the Saint George Respiratory Questionnaire (SGRQ) had not changes (−3.9, 95% CI −8.2, −0.5) (37). The FVC had no changes (−0.3%pred, CI 95% −0.6, 0.1) (29). Maximum expiratory pressure (MEP) was not reported. The certainty of the evidence according to the GRADE methodology was moderate for exercise tolerance, and quality of life (measured with CAT) low for MIP, FEV1, and dyspnoea (measured with Borg scale), and very low for FVC, dyspnoea (measured with mMRC), and quality of life (measured with SGRQ), (Table 4) (Figure 2).
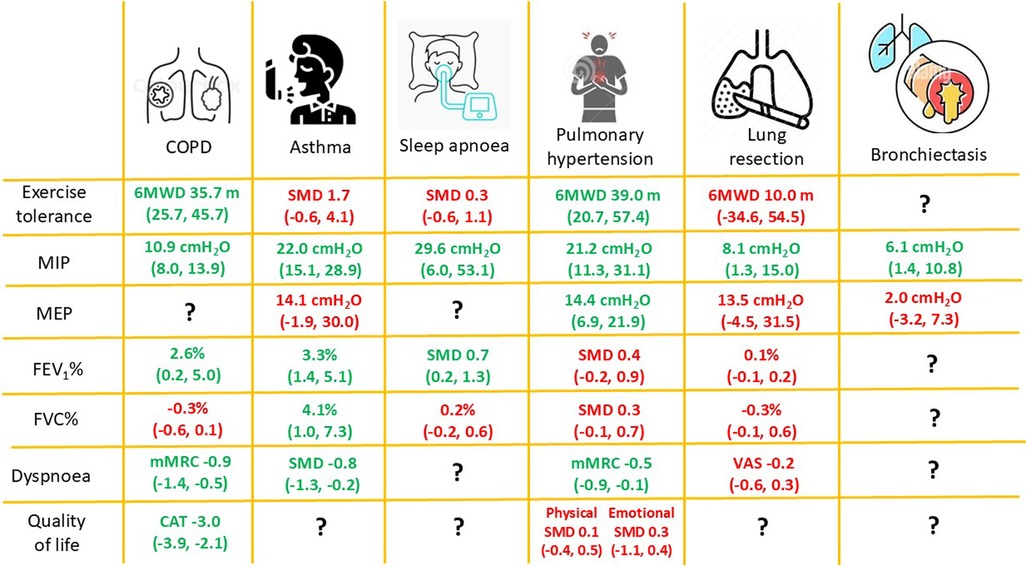
Figure 2. Representation of main findings. 6MWD, six-minute walk distance; COPD, chronic obstructive pulmonary disease; CRQ, chronic respiratory disease questionnaire; FEV1, forced expiratory volume in the first second; FVC, forced vital capacity; MEP, maximum expiratory pressure; MIP, maximum inspiratory pressure; mMRC, modified medical research council; SMD, standard mean difference; VAS, visual analogue scale.
Asthma
We selected five SRs that included patients diagnosed with asthma. All of them performed meta-analyses. All SRs reported MIP and lung function as outcomes, three reported maximum expiratory pressure (MEP), two reported dyspnoea, and one reported exercise tolerance. There was a very high overlap between SRs in FEV1, FVC, MIP, and MEP (Table 3). IMT increased FEV1 by 3.3%pred (95% CI 1.4, 5.1) (41), FVC by 4.1%pred (95% CI 1.0, 7.3) (41), MIP by 22.0 cmH2O (95% CI 15.1, 28.9) (38). Dyspnoea was reduced (SMD −0.8, 95% CI −1.3, −0.2) (17). No changes were found in exercise tolerance (SMD 1.7, 95% CI −0.6, 4.1) (38) and MEP (14.1 cmH2O, 95% CI −1.9, 30.0) (41). The quality of life was not reported. The certainty of the evidence, according to the GRADE methodology, was moderate for MIP, low for exercise tolerance, FEV1 and FVC, and very low for MEP and dyspnoea (Table 4; Figure 2).
Other outcomes reported, but not pooled, were: (1) use of rescue medication, of which, three of four SRs found a significant decrease in β2-agonist consumption un the IMT group; (38) (2) asthma-related symptoms and asthma control with different results (38).
Obstructive sleep apnoea (OSA)
We selected four SRs that included patients with OSA (42–45). All were performed meta-analyses. There was a very high overlap between SRs in exercise tolerance and MIP (Table 3). IMT increased MIP by 29.6 cmH2O (95% CI 6.0, 53.1) (44), and FEV1 by 0.7 SMD (95% CI 0.2, 1.3) (43). The exercise tolerance did not improve (SMD 0.3, 95% CI −0.6, 1.1) (44). No changes were found in FVC (0.2%pred, 95% CI −0.2, 0.6) (42). The MEP, dyspnoea and quality of life were not reported. The certainty of the evidence according to the GRADE methodology was moderate for MIP, and low for exercise tolerance, FEV1 and FVC (Table 4; Figure 2).
Other outcomes reported were: (1) Apnoea/hypopnea index, with all SRs found no changes; (42–45) (2) Sleepiness and sleep quality with an improvement in all SRs; (42–45) and (3) Blood pressure with two SRs that on analysis was found to show an improvement in systolic (42, 45) and diastolic blood pressures (42).
Pulmonary hypertension
We selected two SRs that included patients with PH (46, 47). Both performed meta-analyses. There was a very high overlap between SRs in all analysed outcomes (Table 3). IMT increased exercise tolerance by 39.0 metres (95% CI 20.7, 57.4) in the 6MWT, MIP by 21.2 cmH2O (95% CI 11.3, 31.1), MEP by 14.4 cmH2O (95% CI 6.9, 21.9) (46). Dyspnoea was reduced by 0.5 (95% CI 0.1, 0.9) points in the mMRC scale (46). No changes were found in FEV1 (SMD 0.4, 95% CI −0.2, 0.9), FVC (SMD 0.3, 95% CI −0.1, 0.7), and quality of life in physical (SMD 0.1, 95% CI −0.4, 0.5) and emotional dimension (SMD 0.3, 95% CI −1.1, 0.4) (46). The certainty of the evidence, according to the GRADE methodology, was low for MIP, and very low for all other outcomes (Table 4; Figure 2).
Another outcome reported was physical activity. One individual study selected by one SR found that the IMT group increased by 195.2 MET/min/day (95% CI 102.9, 287.6) compared to the sham IMT group (50). In addition, it reported that the sham IMT group increased by 403.8 steps per day (95% CI −1,241.5, 2,049.1) compared to the IMT group (50).
Lung resection
We selected one SR that included patients undergoing LR. This SR reported all analysed parameters including studies before and after surgery. IMT increased MIP by 8.1 cmH2O (95% CI 1.3, 15.0) (48). Dyspnoea was reduced by SMD −0.8 (95% CI −1.3, −0.2) points (48). No changes were found in exercise tolerance (10.0 m by 6MWT, 95% CI −34.6, 54.5), FEV1 (0.1%pred, 95% CI −0.1, 0.2), FVC (−0.3%pred, 95% CI −0.1, 0.6) (48), MEP (13.5, 95% CI −4.5, 31.5), and dyspnoea (VAS −0.2, 95% CI −0.6, 0.3) (41). The quality of life was not reported. The certainty of the evidence, according to the GRADE methodology, was low for lung function, MIP and MEP, and very low for exercise tolerance and dyspnoea (Table 4; Figure 2).
Other outcomes reported were: (1) pain with no significant differences; (48) and (2) physical activity with higher physical activity in the IMT group (48).
Bronchiectasis
Only one study included patients with bronchiectasis. This study reported only MIP and MEP. IMT increased MIP by 6.1 cmH2O (95% CI 1.4, 10.8) (49). No changes were found in MEP (2.1, 95% CI −3.2, 7.3) (49). Exercise tolerance, lung function, dyspnoea and quality of life were not reported. The certainty of the evidence, according to the GRADE methodology, was low for MIP and very low for MEP (Table 4; Figure 2).
Discussion
IMT showed beneficial effects on several clinically relevant outcomes across different CRDs. Improvements MIP were observed in asthma, OSA, COPD, PH, lung resection, and bronchiectasis. Exercise tolerance increased in COPD and PH, and lung function improved in asthma and OSA. Additionally, reductions in dyspnoea and improvements in quality of life were noted, particularly in COPD patients. These findings highlight the effectiveness of IMT in CRDs.
Exercise tolerance was increased in COPD and PH, close to the minimal clinically important difference (MCID) reported for CRD (51). Our results show that this improvement may be clinically significant. A possible explanation for this improvement is provided by Welch et al., who hypothesized that IMT enhances the aerobic capacity of the respiratory muscles, thereby reducing their demand for blood flow during exercise (12). As a result, a greater proportion of cardiac output could be redirected to the locomotor muscles, potentially delaying peripheral fatigue and improving exercise performance. This mechanism is supported by findings showing that unloading the inspiratory muscles can reduce diaphragm and locomotor muscle fatigue, and prolong exercise duration, particularly during high-intensity efforts (12). In patients with PH, it is proposed that improving MIP, reducing the severity of exertional dyspnea, and enhancing quadriceps muscle strength through IMT play a crucial role in enhancing exercise performance (52). Asthma and OSA did not improve exercise tolerance, coinciding that these were the meta-analyses with fewer studies (three each) so they should be analysed with caution.
Improved lung function was observed in asthma and OSA, specifically FEV1. A possible explanation of this small increase is due to lung function parameters are force-dependent and reflect lung capacity and mainly affected by respiratory muscle strength, airway resistance and lung compliance (41). It is possible that, as a result of IMT, the respiratory muscles were able to perform more work, which resulted in an improvement in respiratory capacity and an increase in thoracic expansion and, therefore, had a role in increasing lung volumes (13). Although in the case of COPD, FEV1 increased significantly, the change is only 2.6%, this result is statistically significant, however, its clinical impact is debatable (and likely low) and it is smaller even than the margin of error of this measurement (53).
MIP was increased in all CRDs analysed. However, in asthma, lung resection and bronchiectasis the change was lower than the MCID established in COPD (54). Unfortunately, to date there is no established MCID for these populations (38). Regarding the changes in MEP, with the exception of patients with OSA, we did not find significant differences in the other CRDs, probably due to the specificity of the IMT that complies with the physiological principles of training (55).
Evidence of dyspnoea diminution was found only in COPD, asthma and PH. A possible explanation is due that IMT can have a possible effect on dynamic hyperinflation, commonly observed in obstructive diseases, allowing the diaphragm to work with a better force-length relationship and allowing for the generation of a given pressure with less respiratory motor drive (14). Individuals with different levels of lung obstruction have less dyspnoea when they have higher MIP (56). On the other hand, the literature has shown that inspiratory muscle fatigue causes the metaboreflex, resulting in vasoconstriction of the blood vessels in the peripheral muscles, which leads to a decrease in respiratory performance (57). IMT might minimise the effects of the activation of the inspiratory muscle metaboreflex.
In COPD patients, the reduction in diaphragmatic electromyographic activity (EMGdi) and the EMGdi/EMGdimax ratio after IMT suggests an improvement in diaphragmatic efficiency, leading to less muscular effort during breathing (58). This could translate into a decrease in the perception of dyspnea, even during intense exercise, as the body no longer needs to recruit as much effort from the diaphragm to maintain minute ventilation (VE) during physical activity (58).
In PH, the primary symptom is progressively worsening dyspnea, often occurring with minimal effort (59). The heightened ventilation demand, coupled with reduced respiratory muscle function, leads to an increased neural respiratory drive, thereby intensifying the sensation of breathlessness (59). Additionally, patients with PH have been found to experience respiratory muscle weakness and atrophy, which contribute to the sensation of dyspnea and exercise-induced fatigue (8).
Evidence of quality of life improvement was found for COPD. There is a close relationship between dyspnoea and quality of life. It has been reported that patients who perceive severe dyspnoea have a worse quality of life (60). However, the CRQ had a change lower than the MCID (61), so it must be analysed with caution since it does not represent a clinically significant change. In PH, however, it did not improve, although it was only analysed in three studies (46), so future studies in this disease should explore the change in this outcome.
We found a very high overlap in the studies included in the SRs for MIP and lung function in asthma, OSA and PH, and dyspnoea and quality of life in COPD, however, there was no overlap in the studies related to dyspnea in asthma. The articles selected in this overview were aimed at answering the same research question, which is why it is striking that there was no overlap in some outcomes. On the other hand, we found many SRs aimed at answering the same question in COPD. This should make us reflect on the meaning of carrying out the same SRs with little time between them.
Most of the SRs included in the study were of “low” quality or “critically low”. AMSTAR 2 has previously been used in several fields, and many publications have reported a substantial number of SRs with low or critically low quality (62). AMSTAR 2, a critical appraisal tool, was introduced in 2017, at a time when most SRs were utilizing different guidelines for their methodologies (62). Consequently, until 2020, fewer than 200 publications in PubMed implemented AMSTAR 2 in their study approaches (62). This lack of adoption and adherence to AMSTAR 2 guidelines may explain certain instances of non-compliance in the conduct and reporting of research studies (62). For this reason, the risk of bias of the results should be carefully assessed along with the risk of bias assessment of each SR individually. Given the high prevalence of low and critically low-quality SRs, the implications of these findings should be carefully considered. The lack of adherence to established guidelines, such as AMSTAR 2, likely contributes to the inconsistencies and limitations observed in the SRs, which must be taken into account when interpreting the results.
There is significant uncertainty regarding the evidence on IMT, highlighting the need for better-designed studies with adequate statistical power rather than continuing to accumulate inconclusive evidence. This overview provides a snapshot of the current landscape and suggests that future studies should focus on less explored CRDs such as interstitial lung diseases or bronchiectasis, to avoid extrapolating findings from COPD. Additionally, the need for new SRs, should be carefully assessed, as many reliable ones already exist; improving the evidence base will require high-quality primary studies (63). Our GRADE-based analysis also revealed discrepancies among SRs, suggesting inconsistent application of criteria when determining the certainty of evidence.
Strengths and limitations
The great strength of this overview is that it allows analysing a broad spectrum of chronic diseases with relevant outcomes, including patient reported outcome measures (PROMs), and, in this way, clinicians can quickly see if the intervention is helpful for the population they treat. On the other hand, we only consider SRs with meta-analyses of RCTs, which strengthens the conclusions. Nevertheless, since we did not perform a meta-analysis, some RCTs could have been left out of the results and findings if not in the “best” SR.
Among the limitations we found is the lack of SRs of other CRDs, such as interstitial diseases and cystic fibrosis (excluded for mixing children and adults). Another important limitation not addressed by this review is the variety of devices and different training protocols used. This issue has been reported and is probably one of the aspects that determines the heterogeneity of the results of the included studies and the discussion of the effectiveness of IMT in some pathologies. The variation in training protocols, devices, duration, and intensities may influence the results and limit the ability to draw firm conclusions.
Conclusion
IMT improved several clinically relevant outcomes, including MIP, exercise capacity, and dyspnoea in different CRDs. However, the limited evidence for certain outcomes and populations highlights the need for further high-quality studies. Additionally, the interpretation of these results should be approached with caution, given the variable quality of the SRs included in this study.
Author contributions
RT-C: Conceptualization, Data curation, Formal analysis, Investigation, Project administration, Supervision, Writing – original draft, Writing – review & editing. SC-T: Conceptualization, Data curation, Formal analysis, Investigation, Writing – original draft, Writing – review & editing. EG-S: Conceptualization, Investigation, Methodology, Software, Supervision, Validation, Visualization, Writing – original draft, Writing – review & editing. RG-A: Formal analysis, Investigation, Methodology, Writing – original draft, Writing – review & editing. XA-R: Data curation, Investigation, Writing – original draft, Writing – review & editing. LV-C: Data curation, Investigation, Writing – original draft, Writing – review & editing. PS: Investigation, Methodology, Writing – original draft, Writing – review & editing. MS: Conceptualization, Formal analysis, Validation, Visualization, Writing – original draft, Writing – review & editing. IB: Conceptualization, Formal analysis, Investigation, Supervision, Validation, Writing – original draft, Writing – review & editing. JV: Conceptualization, Investigation, Supervision, Validation, Writing – original draft, Writing – review & editing.
Funding
The author(s) declare that financial support was received for the research and/or publication of this article. The study was supported by grants from the Fondo de Investigación Sanitaria, Instituto de Salud Carlos III (PI17/1515), Fondo Europeo de Desarrollo Regional (FEDER), Unión Europea “Una manera de hacer Europa”. Sociedad Española de Neumología y Cirugía Torácica (SEPAR) and Societat Catalana de Pneumologia (SOCAP). Rodrigo Torres-Castro is funded by a grant from the National Agency for Research and Development (ANID)/Scholarship Program/DOCTORADO BECAS CHILE/2018–72190117.
Conflict of interest
RTC has received educational material from Biolaster, Spain.
The remaining authors declare that the research was conducted in the absence of any commercial or financial relationships that could be construed as a potential conflict of interest.
Generative AI statement
The author(s) declare that no Generative AI was used in the creation of this manuscript.
Publisher's note
All claims expressed in this article are solely those of the authors and do not necessarily represent those of their affiliated organizations, or those of the publisher, the editors and the reviewers. Any product that may be evaluated in this article, or claim that may be made by its manufacturer, is not guaranteed or endorsed by the publisher.
Supplementary material
The Supplementary Material for this article can be found online at: https://www.frontiersin.org/articles/10.3389/fspor.2025.1549652/full#supplementary-material
References
1. Li X, Cao X, Guo M, Xie M, Liu X. Trends and risk factors of mortality and disability adjusted life years for chronic respiratory diseases from 1990 to 2017: systematic analysis for the global burden of disease study 2017. Br Med J. (2020) 368:m234. doi: 10.1136/bmj.m234
2. Collaborators GCRD. Prevalence and attributable health burden of chronic respiratory diseases, 1990–2017: a systematic analysis for the global burden of disease study 2017. Lancet Respir Med. (2020) 8(6):585–96. doi: 10.1016/S2213-2600(20)30105-3
3. O’Donnell DE, Milne KM, James MD, de Torres JP, Neder JA. Dyspnea in COPD: new mechanistic insights and management implications. Adv Ther. (2020) 37(1):41–60. doi: 10.1007/s12325-019-01128-9
4. Barreiro E, Gea J. Respiratory and limb muscle dysfunction in COPD. COPD. (2015) 12(4):413–26. doi: 10.3109/15412555.2014.974737
5. van der Meer AN, de Jong K, Hoekstra-Kuik A, Bel EH, Ten Brinke A. Dynamic hyperinflation impairs daily life activity in asthma. Eur Respir J. (2019) 53(4):1801500. doi: 10.1183/13993003.01500-2018
6. Plantier L, Cazes A, Dinh-Xuan A-T, Bancal C, Marchand-Adam S, Crestani B. Physiology of the lung in idiopathic pulmonary fibrosis. Eur Respir Rev. (2018) 27(147):170062. doi: 10.1183/16000617.0062-2017
7. Hoffman M. Inspiratory muscle training in interstitial lung disease: a systematic scoping review. J Bras Pneumol. (2021) 47(4):e20210089. doi: 10.36416/1806-3756/e20210089
8. Kabitz HJ, Schwoerer A, Bremer HC, Sonntag F, Walterspacher S, Walker D, et al. Impairment of respiratory muscle function in pulmonary hypertension. Clin Sci. (2008) 114(2):165–71. doi: 10.1042/CS20070238
9. Orozco-Levi M. Structure and function of the respiratory muscles in patients with COPD: impairment or adaptation? Eur Respir J Suppl. (2003) 22:41s–51. doi: 10.1183/09031936.03.00004607
10. McKenzie DK, Butler JE, Gandevia SC. Respiratory muscle function and activation in chronic obstructive pulmonary disease. J Appl Physiol. (2009) 107(2):621–9. doi: 10.1152/japplphysiol.00163.2009
11. McConnell AK, Romer LM. Respiratory muscle training in healthy humans: resolving the controversy. Int J Sports Med. (2004) 25(4):284–93. doi: 10.1055/s-2004-815827
12. Welch JF, Kipp S, Sheel AW. Respiratory muscles during exercise: mechanics, energetics, and fatigue. Curr Opin Physiol. (2019) 10:102–9. doi: 10.1016/j.cophys.2019.04.023
13. Hoffman M, Augusto VM, Eduardo DS, Silveira BMF, Lemos MD, Parreira VF. Inspiratory muscle training reduces dyspnea during activities of daily living and improves inspiratory muscle function and quality of life in patients with advanced lung disease. Physiother Theory Pract. (2021) 37(8):895–905. doi: 10.1080/09593985.2019.1656314
14. Shei R-J, Paris HLR, Wilhite DP, Chapman RF, Mickleborough TD. The role of inspiratory muscle training in the management of asthma and exercise-induced bronchoconstriction. Phys Sportsmed. (2016) 44(4):327–34. doi: 10.1080/00913847.2016.1176546
15. Huang CH, Martin AD, Davenport PW. Effect of inspiratory muscle strength training on inspiratory motor drive and RREP early peak components. J Appl Physiol. (2003) 94(2):462–8. doi: 10.1152/japplphysiol.00364.2002
16. Downey AE, Chenoweth LM, Townsend DK, Ranum JD, Ferguson CS, Harms CA. Effects of inspiratory muscle training on exercise responses in normoxia and hypoxia. Respir Physiol Neurobiol. (2007) 156(2):137–46. doi: 10.1016/j.resp.2006.08.006
17. Chen Y, Fu HB, Chen Y, Fu H. Inspiratory muscle training for asthmatic patients: a meta-analysis of randomized controlled studies. Phys Med Rehab Kuror. (2022) 32(05):285–90. doi: 10.1055/a-1510-3422
18. Sales AdN, Fregonezi GdF, Ramsook AH, Guenette JA, Lima INDF, Reid WD. Respiratory muscle endurance after training in athletes and non-athletes: a systematic review and meta-analysis. Phys Ther Sport. (2016) 17:76–86. doi: 10.1016/j.ptsp.2015.08.001
19. Shei R-J. Recent advancements in our understanding of the ergogenic effect of respiratory muscle training in healthy humans: a systematic review. J Strength Cond Res. (2018) 32(9):2665–76. doi: 10.1519/JSC.0000000000002730
20. Pollock M, Fernandes R, Becker L, Pieper D, Hartling L. Overviews of reviews. In: Higgins JPT, Thomas J, Chandler J, Cumpston M, Li T, Page MJ, Welch VA, editors. Cochrane Handbook for Systematic Reviews of Interventions Version 6.3. Cochrane (2022).
21. Gates M, Gates A, Pieper D, Fernandes RM, Tricco AC, Moher D, et al. Reporting guideline for overviews of reviews of healthcare interventions: development of the PRIOR statement. Br Med J. (2022) 378:e070849. doi: 10.1136/bmj-2022-070849
22. Shea BJ, Reeves BC, Wells G, Thuku M, Hamel C, Moran J, et al. AMSTAR 2: a critical appraisal tool for systematic reviews that include randomised or non-randomised studies of healthcare interventions, or both. Br Med J. (2017) 358:j4008. doi: 10.1136/bmj.j4008
23. Guyatt GH, Oxman AD, Vist GE, Kunz R, Falck-Ytter Y, Alonso-Coello P, et al. GRADE: an emerging consensus on rating quality of evidence and strength of recommendations. Br Med J. (2008) 336(7650):924–6. doi: 10.1136/bmj.39489.470347.AD
24. Bougioukas KI, Vounzoulaki E, Mantsiou CD, Savvides ED, Karakosta C, Diakonidis T, et al. Methods for depicting overlap in overviews of systematic reviews: an introduction to static tabular and graphical displays. J Clin Epidemiol. (2021) 132:34–45. doi: 10.1016/j.jclinepi.2020.12.004
25. Pieper D, Antoine S-L, Mathes T, Neugebauer EAM, Eikermann M. Systematic review finds overlapping reviews were not mentioned in every other overview. J Clin Epidemiol. (2014) 67(4):368–75. doi: 10.1016/j.jclinepi.2013.11.007
26. Lunny C, Pieper D, Thabet P, Kanji S. Managing overlap of primary study results across systematic reviews: practical considerations for authors of overviews of reviews. BMC Med Res Methodol. (2021) 21(1):140. doi: 10.1186/s12874-021-01269-y
27. Bracchiglione J, Meza N, Bangdiwala SI, Niño de Guzmán E, Urrútia G, Bonfill X, et al. Graphical representation of overlap for OVErviews: GROOVE tool. Res Synth Methods. (2022) 13(3):381–8. doi: 10.1002/jrsm.1557
28. Crowe J, Reid WD, Geddes EL, O’Brien K, Brooks D. Inspiratory muscle training compared with other rehabilitation interventions in adults with chronic obstructive pulmonary disease: a systematic literature review and meta-analysis. COPD. (2005) 2(3):319–29. doi: 10.1080/15412550500218072
29. Figueiredo RIN, Azambuja AM, Cureau FV, Sbruzzi G. Inspiratory muscle training in COPD. Respir Care. (2020) 65(8):1189–201. doi: 10.4187/respcare.07098
30. Geddes EL, Reid WD, Crowe J, O’Brien K, Brooks D. Inspiratory muscle training in adults with chronic obstructive pulmonary disease: a systematic review. Respir Med. (2005) 99(11):1440–58. doi: 10.1016/j.rmed.2005.03.006
31. Geddes EL, O’Brien K, Reid WD, Brooks D, Crowe J. Inspiratory muscle training in adults with chronic obstructive pulmonary disease: an update of a systematic review. Respir Med. (2008) 102(12):1715–29. doi: 10.1016/j.rmed.2008.07.005
32. Gosselink R, De Vos J, van den Heuvel SP, Segers J, Decramer M, Kwakkel G. Impact of inspiratory muscle training in patients with COPD: what is the evidence? Eur Respir J. (2011) 37(2):416–25. doi: 10.1183/09031936.00031810
33. Lötters F, Tol B van, Kwakkel G, Gosselink R. Effects of controlled inspiratory muscle training in patients with COPD: a meta-analysis. Eur Respir J. (2002) 20(3):570–7. doi: 10.1183/09031936.02.00237402
34. Nakamiti MO, Guerra RO, Freitas D, Nogueira PM, Mendonça K. Effects of inspiratory muscle training on pulmonary function, exercise tolerance, dyspnea and quality of life in patients with COPD. Rev Ter Man. (2011) 9(44):559–68.
35. O’Brien K, Geddes EL, Reid WD, Brooks D, Crowe J. Inspiratory muscle training compared with other rehabilitation interventions in chronic obstructive pulmonary disease: a systematic review update. J Cardiopulm Rehabil Prev. (2008) 28(2):128–41. doi: 10.1097/01.HCR.0000314208.40170.00
36. Shoemaker MJ, Donker S, Lapoe A. Inspiratory muscle training in patients with chronic obstructive pulmonary disease: the state of the evidence. Cardiopulm Phys Ther J. (2009) 20(3):5–15. doi: 10.1097/01823246-200920030-00002
37. Ammous O, Feki W, Lotfi T, Khamis AM, Gosselink R, Rebai A, et al. Inspiratory muscle training, with or without concomitant pulmonary rehabilitation, for chronic obstructive pulmonary disease (COPD). Cochrane Database Syst Rev. (2023) 1(1):CD013778. doi: 10.1002/14651858.CD013778.pub2
38. Lista-Paz A, Bouza Cousillas L, Jácome C, Fregonezi G, Labata-Lezaun N, Llurda-Almuzara L, et al. Effect of respiratory muscle training in asthma: a systematic review and meta-analysis. Ann Phys Rehabil Med. (2023) 66(3):101691. doi: 10.1016/j.rehab.2022.101691
39. Ram FSF, Wellington SR, Barnes NC. Inspiratory muscle training for asthma. Cochrane Database Syst Rev. (2003) (4):CD003792. doi: 10.1002/14651858.cd003792
40. Silva IS, Fregonezi GAF, Dias FAL, Ribeiro CTD, Guerra RO, Ferreira GMH. Inspiratory muscle training for asthma. Cochrane Database Syst Rev. (2013) (9):CD003792. doi: 10.1002/14651858.CD003792.pub2
41. Wang Q, Yang F, Gao L, Gao W. Effects of inspiratory muscle training and high-intensity interval training on lung function and respiratory muscle function in asthma. Respir Care. (2022) 67(11):1465–75. doi: 10.4187/respcare.09813
42. Chen T-A, Mao S-T, Lin H-C, Liu W-T, Tam K-W, Tsai C-Y, et al. Effects of inspiratory muscle training on blood pressure- and sleep-related outcomes in patients with obstructive sleep apnea: a meta-analysis of randomized controlled trials. Sleep Breath. (2023) 27(5):1953–66. doi: 10.1007/s11325-022-02773-1
43. Dar JA, Mujaddadi A, Moiz JA. Effects of inspiratory muscle training in patients with obstructive sleep apnoea syndrome: a systematic review and meta-analysis. Sleep Sci. (2022) 15(4):480–9. doi: 10.5935/1984-0063.20220081
44. Torres-Castro R, Solis-Navarro L, Puppo H, Alcaraz-Serrano V, Vasconcello-Castillo L, Vilaró J, et al. Respiratory muscle training in patients with obstructive sleep apnoea: a systematic review and meta-analysis. Clocks Sleep. (2022) 4(2):219–29. doi: 10.3390/clockssleep4020020
45. Silva de Sousa A, Pereira da Rocha A, Brandão Tavares DR, Frazão Okazaki JÉ, de Andrade Santana MV, Fernandes Moça Trevisani V, et al. Respiratory muscle training for obstructive sleep apnea: systematic review and meta-analysis. J Sleep Res. (2024) 33:e13941. doi: 10.1111/jsr.13941
46. Gutierrez-Arias R, Hinojosa-Riadi J, Sandoval-Cañío A, Santana-Garrido H, Valdovinos-Guerrero N, Seron P. Effectiveness of respiratory muscle training in adults with pulmonary hypertension. A systematic review and meta-analysis. Heart Lung Circ. (2023) 32(3):315–29. doi: 10.1016/j.hlc.2022.11.010
47. Luo Z, Qian H, Zhang X, Wang Y, Wang J, Yu P. Effectiveness and safety of inspiratory muscle training in patients with pulmonary hypertension: a systematic review and meta-analysis. Front Cardiovasc Med. (2022) 9:999422. doi: 10.3389/fcvm.2022.999422
48. Yang M-X, Wang J, Zhang X, Luo Z-R, Yu P-M. Perioperative respiratory muscle training improves respiratory muscle strength and physical activity of patients receiving lung surgery: a meta-analysis. World J Clin Cases. (2022) 10(13):4119–30. doi: 10.12998/wjcc.v10.i13.4119
49. Martín-Valero R, Jimenez-Cebrian AM, Moral-Munoz JA, De-la-Casa-Almeida M, Rodriguez-Huguet M, Casuso-Holgado MJ. The efficacy of therapeutic respiratory muscle training interventions in people with bronchiectasis: a systematic review and meta-analysis. J Clin Med. (2020) 9(1):231. doi: 10.3390/jcm9010231
50. Aslan GK, Akıncı B, Yeldan I, Okumus G. A randomized controlled trial on inspiratory muscle training in pulmonary hypertension: effects on respiratory functions, functional exercise capacity, physical activity, and quality of life. Heart Lung. (2020) 49(4):381–7. doi: 10.1016/j.hrtlng.2020.01.014
51. Singh SJ, Puhan MA, Andrianopoulos V, Hernandes NA, Mitchell KE, Hill CJ, et al. An official systematic review of the European respiratory society/American thoracic society: measurement properties of field walking tests in chronic respiratory disease. Eur Respir J. (2014) 44(6):1447–78. doi: 10.1183/09031936.00150414
52. Naeije R. Breathing more with weaker respiratory muscles in pulmonary arterial hypertension. Eur Respir J. (2005) 25(1):6–8. doi: 10.1183/09031936.04.00121004
53. Donohue JF. Minimal clinically important differences in COPD lung function. COPD. (2005) 2(1):111–24. doi: 10.1081/COPD-200053377
54. Iwakura M, Okura K, Kubota M, Sugawara K, Kawagoshi A, Takahashi H, et al. Estimation of minimal clinically important difference for quadriceps and inspiratory muscle strength in older outpatients with chronic obstructive pulmonary disease: a prospective cohort study. Phys Ther Res. (2021) 24(1):35–42. doi: 10.1298/ptr.E10049
55. Romer LM, McConnell AK. Specificity and reversibility of inspiratory muscle training. Med Sci Sports Exerc. (2003) 35(2):237–44. doi: 10.1249/01.MSS.0000048642.58419.1E
56. Killian KJ, Jones NL. Respiratory muscles and dyspnea. Clin Chest Med. (1988) 9(2):237–48. doi: 10.1016/S0272-5231(21)00502-5
57. Moreno AM, Toledo-Arruda AC, Lima JS, Duarte CS, Villacorta H, Nóbrega ACL. Inspiratory muscle training improves intercostal and forearm muscle oxygenation in patients with chronic heart failure: evidence of the origin of the respiratory metaboreflex. J Card Fail. (2017) 23(9):672–9. doi: 10.1016/j.cardfail.2017.05.003
58. Langer D, Ciavaglia C, Faisal A, Webb KA, Neder JA, Gosselink R, et al. Inspiratory muscle training reduces diaphragm activation and dyspnea during exercise in COPD. J Appl Physiol. (2018) 125(2):381–92. doi: 10.1152/japplphysiol.01078.2017
59. Ozcan Kahraman B, Tanriverdi A, Savci S, Odaman H, Akdeniz B, Sevinc C, et al. Effects of inspiratory muscle training in patients with pulmonary hypertension. Am J Cardiol. (2023) 203:406–13. doi: 10.1016/j.amjcard.2023.06.097
60. Akinci AC, Pinar R, Demir T. The relation of the subjective dyspnoea perception with objective dyspnoea indicators, quality of life and functional capacity in patients with COPD. J Clin Nurs. (2013) 22(7–8):969–76. doi: 10.1111/j.1365-2702.2012.04161.x
61. Chauvin A, Rupley L, Meyers K, Johnson K, Eason J. Outcomes in cardiopulmonary physical therapy: chronic respiratory disease questionnaire (CRQ). Cardiopulm Phys Ther J. (2008) 19(2):61. doi: 10.1097/01823246-200819020-00007
62. Li L, Asemota I, Liu B, Gomez-Valencia J, Lin L, Arif AW, et al. AMSTAR 2 appraisal of systematic reviews and meta-analyses in the field of heart failure from high-impact journals. Syst Rev. (2022) 11(1):147. doi: 10.1186/s13643-022-02029-9
Keywords: inspiratory muscle training, chronic respiratory diseases, overview, exercise tolerance, symptoms
Citation: Torres-Castro R, Caicedo-Trujillo S, Gimeno-Santos E, Gutiérrez-Arias R, Alsina-Restoy X, Vasconcello-Castillo L, Seron P, Spruit MA, Blanco I and Vilaró J (2025) Effectiveness of inspiratory muscle training in patients with a chronic respiratory disease: an overview of systematic reviews. Front. Sports Act. Living 7:1549652. doi: 10.3389/fspor.2025.1549652
Received: 21 December 2024; Accepted: 28 April 2025;
Published: 21 May 2025.
Edited by:
Felipe Contreras–Briceño, Pontificia Universidad Católica de Chile, ChileReviewed by:
Maximiliano Espinosa-Ramírez, The University of Iowa, United StatesGregory Cuvelier, Haute École provinciale de Hainaut Condorcet, Belgium
Copyright: © 2025 Torres-Castro, Caicedo-Trujillo, Gimeno-Santos, Gutiérrez-Arias, Alsina-Restoy, Vasconcello-Castillo, Seron, Spruit, Blanco and Vilaró. This is an open-access article distributed under the terms of the Creative Commons Attribution License (CC BY). The use, distribution or reproduction in other forums is permitted, provided the original author(s) and the copyright owner(s) are credited and that the original publication in this journal is cited, in accordance with accepted academic practice. No use, distribution or reproduction is permitted which does not comply with these terms.
*Correspondence: Elena Gimeno-Santos, ZWxlbmFnaW1lbm9AZ21haWwuY29t