- 1National Food Institute (DTU Food), Kgs Lyngby, Denmark
- 2Royal Greenland Seafood A/S, Svenstrup J, Denmark
- 3Ifremer, MASAE Microbiologie Aliment Santé Environnement, Nantes, France
The aims of this study were firstly, to determine if the removal of heads and storage in a modified atmosphere (MA) extended the sensory shelf-life of chilled shell-on cooked Northern shrimp (Pandalus borealis). Secondly, the safe shelf-life of products was determined in regard to Listeria monocytogenes. Shrimp were kept whole or as tails (head region removed) at 5°C in laminar-film-bags with or without MA (40% CO2/60% N2) for 21 days. Analyses included changes in sensory properties (shelf-life), headspace CO2/O2, drip loss, pH, microbiota, total volatile nitrogen (TVN), trimethylamine-oxide (TMAO), and trimethylamine (TMA). Shrimp tails stored in MA reached the end-of-sensory-shelf-life after 21 days, followed by 18 days for whole shrimp in MA, and 14 and 11 days, respectively, for shrimp tails and whole shrimp without MA. For all four treatments, aerobic plate counts reached >8 log CFU/g at the time of sensory spoilage with Carnobacterium spp. and Pseudoalteromonas spp. dominating for shrimp with or without MA, respectively. Sensory spoilage coincided with TVN levels of 32–41 mg-N/100 g for shrimp in MA, while higher levels (66–143 mg-N/100 g) were observed for shrimp without MA. Pseudoalteromonas spp. had the strongest spoilage potential and likely contributed to the observed conversion of TMAO to TMA in shrimp stored without MA. Safe shelf-life was studied by testing the growth rate of L. monocytogenes, and the applicability of existing models to predict its growth. At 2°C–15°C, L. monocytogenes grew faster in whole shrimp than in shrimp meat. Moreover, the studied predictive model slightly underestimated growth of the pathogen. Taken together, our results show that to avoid unacceptable growth of L. monocytogenes, the safe shelf-life should be limited to less than 10 days at 5°C in MA for these ready-to-eat, cooked shell-on Northern shrimp products. Extension of product shelf-life would require research into stabilization of the products to inhibit the growth of L. monocytogenes.
1 Introduction
Commercially, the Northern shrimp (Pandalus borealis) is one of the most important crustaceans in the Northern Hemisphere, with more than 250,000 metric tons being caught and processed each year (FAO, 2024). Products of refreshed (thawed and chilled) shrimp have recently appeared on the Scandinavian market, where shrimp is marketed in modified atmosphere packaging (MAP) with a shelf-life of up to 20 days at 2°C–5°C, depending on the producer. MAP is widely used for retail distribution and extension of seafood shelf-life (Dewitt and Oliveira, 2016; Peng et al., 2022; Yu et al., 2023). Specifically for whole, shell-on cooked P. borealis it is previously shown that a gas mixture with 60% CO2 and 40% N2 increased sensory shelf-life to more than 21 days at 3°C, compared to 7 days for ice-stored samples in atmospheric air (Sivertsvik et al., 1997). However, more detailed studies into shelf-life limiting reactions including the spoilage microbiota and the safe shelf-life of refreshed cooked shell-on Northern shrimp are lacking.
Removal of the head and, thereby, also the majority of the gastrointestinal tract, is common practice for warm-water shrimp species (Chandrasekaran, 1994) and has previously been shown to extend the shelf-life of raw spotted shrimp (Pandalus platyceros) (Matches and Layrisse, 1985). This is likely due to the removal of the gut microbiota and enzymes responsible for the deterioration of raw shrimp (Sriket et al., 2010; 2011; Sriket, 2014). Moreover, removing the head would solve the issue of the head region turning dark brown or black following the cooking of the Northern shrimp (Supplementary Figure S1; Company communication, Royal Greenland Seafood A/S). The cause of this discoloration has been suggested to be due to the cooking-induced release of the stomach content (Hansen and Aagard, 1968). Currently, no studies have investigated the quantitative effect of the removal of the head on the sensory shelf-life and spoilage microbiota of cooked, Northern shrimp stored at refrigeration temperatures. Microbial spoilage is important for the shelf-life of various raw and cooked shrimp products (Das and Mishra, 2023; Mejlholm et al., 2008; Peng et al., 2022). Identification of the bacteria, which are most active in the spoilage reactions, i.e., specific spoilage organism (SSO) (Dalgaard, 1995a; Gram and Dalgaard, 2002), may help producers develop products that are formulated to reduce the growth of the SSOs. The SSOs for cooked and peeled Northern shrimp in MAP are identified as Brochothrix thermosphacta and Carnobacterium maltaromaticum, which lead to the production of sour, wet-dog and chlorine-like off-odors (Mejlholm et al., 2005; Laursen et al., 2006). In contrast, for whole, cooked, and peeled brown shrimp (Crangon) stored in atmospheric air, the spoilage microbiota was dominated by Psychrobacter spp. and Pseudoalteromonas spp. (Broekaert et al., 2013a). Currently, the spoilage microbiota for shell-on-cooked Northern shrimp is unknown.
While SSO is the determining factor for microbial spoilage, the shelf-life of the product might be reduced due to the potential growth of relevant pathogenic microorganisms. Listeria monocytogenes is highly persistent in food processing environments, and Carpentier and Cerf (2011) even conclude their review on the persistence of L. monocytogenes in food processing environments, saying that the “total control of Listeria in a ready-to-eat processing plant is extremely difficult, perhaps impossible.” Moreover, in 2016 the Danish Veterinary and Food Agency (DVFA) launched a national Listeria awareness campaign in Denmark to educate both producers of high-risk ready-to-eat (RTE) products and consumers to reduce the prevalence and risk of L. monocytogenes. The results of a recently published article concluded that the campaign had little effect on reducing the prevalence of the pathogen in the processing environments nor reduced the number of human cases of listeriosis (Takeuchi-Storm et al., 2023), underlining the conclusion by Carpentier and Cerf (2011). As it is obvious that cleaning the processing facilities does not prevent or eliminate the prevalence and outbreaks caused by L. monocytogenes, producers of high-risk RTE products must find other strategies to ensure that their products do not pose a risk to consumers. Refrigerated, shell-on-cooked Northern shrimp falls under the category of high-risk RTE products, as L. monocytogenes has been shown to grow in similar products of warm-water shrimp species (Wang and Johnson, 1997; Zhao et al., 2020) and since the product is not stabilized against the growth of the pathogen. Furthermore, Listeria spp. has been observed and isolated from the processing environments of Northern shrimp (Valdimarsson et al., 1998; Gudbjörnsdóttir et al., 2004; Gudmundsdóttir et al., 2006). No studies have investigated the growth of L. monocytogenes in shell-on-cooked Northern shrimp during refrigerated storage. Previous studies on the safe shelf-life of cooked and peeled (C&P) Northern shrimp products led to the development and validation of a cardinal parameter model for the growth of L. monocytogenes in lightly preserved and RTE C&P shrimp. The developed model is freely available and incorporated into the Food Safety and Spoilage Predictor (FSSP, www.fssp.food.dtu.dk). FSSP can be used to predict L. monocytogenes growth in various foods, and it is suggested by the DVFA to utilize this tool to improve food safety. The performance of the existing model on shell-on-cooked Northern shrimp is unknown.
The objectives of this study were first to study the effect of head removal and MA on the chilled sensory shelf-life and microbial spoilage of refreshed shell-on-cooked Northern shrimp. Secondly, the safe shelf-life of products was evaluated using data from challenge experiments and predictions for the growth of L. monocytogenes.
2 Materials and methods
2.1 Raw material
Northern shrimp (P. borealis) was caught by a commercial fishing vessel with a bottom otter trawl in the FAO 21 fishing area (Northwestern Atlantic Ocean). Within 3 hours of being caught, whole shrimp were cooked in seawater for 3 minutes, reaching average core temperatures of 95°C. Shrimp (90–120 individuals per kg, average weight 10 g each) were then individually quick frozen (IQF) and packed in boxes, each containing 5 kg. Boxes were stored frozen, onboard the shrimp trawler the storage temperature was approx. −30°C, while during the shipment to Denmark and until experimental processing at DTU Food (Kgs. Lyngby, Denmark), the temperature was approx. −20°C. The shrimp used for the storage trial was kept frozen for approximately 4 months at −20°C (see Section 2.2). The shrimp used for the challenge tests were transferred to −40°C directly after arrival at DTU Food to increase the stability of the product’s characteristics and stored for up to 3 months (see Section 2.3).
2.2 Storage trial for determination of sensory shelf-life
2.2.1 Preparation of shell-on shrimp samples with and without heads
A total of 1,120 thawed shell-on shrimp (approximately 11.2 kg) were used for the storage trial, of which half (560) shrimp had the head removed with a clean knife, which was disinfected with 70% ethanol between samples, and the remaining (560) were kept as whole shrimp. This meant that for tail samples, the entire cephalothorax (head) region was removed. The remaining shell-on tail includes the entire edible meat portion of the shrimp.
2.2.2 Packaging and storage of shell-on shrimp samples
Individual tray samples were prepared by placing seven shrimp with heads (whole, approximately 70 g per sample) or without heads (tail, approximately 35 g per sample) in clean 14 × 14 cm plastic trays (11-513-432, Fisherbrand, Fisher Scientific, Hampton, United States). A total of 160 trays were prepared, 80 of each type of shrimp. 80 trays, 40 of each type of shrimp (n = 40 for whole and n = 40 for tail), were packed in atmospheric air in sealed plastic bags made from 70 μm thick polyethylene film with high permeability for water vapor (>6 g m−2 d−1), O2 (>3,000 cm3 m−2 d−1 atm−1) and CO2 (>14,000 cm3 m−2 d−1 atm−1) according to information from the supplier (H102017, Topiplast A/S, Greve, Denmark). The remaining trays (MAP, n = 40 each for whole and tail) were packed in a modified atmosphere consisting of 40% CO2 and 60% N2 (AGA, Copenhagen, Denmark) and plastic bags made from a 117 ± 6 μm laminate film (NEN 40 HOB/LLPDE 75, Amcore, Horsens, Denmark) with low gas permeability for O2 (0.45 cm3 m−2 d−1 atm−1) and CO2 (1.8 cm3 m−2 d−1 atm−1). The gas-to-product ratio was approximately 4:1 for whole shrimp samples and 6:1 for tail samples. All samples were stored at 5.0°C ± 0.3°C for 21 days. Storage temperature was followed using four data loggers (Tinytag, Gemini Data Loggers Ltd, Chichester, United Kingdom).
2.2.3 Sampling plan and sensory shelf-life determination
To determine the shelf-life of the Northern shrimp, with or without heads at two different gas compositions, a storage trial was designed. The odor subsection of the Quality Index Method (QIM) for “deep water and fjord shrimp” was used to determine a sensory shelf-life. The odor scoring category was as follows; 0 (fresh, seaweedy), 1 (faint odor, reminds of tar), 2 (faint ammonia odor) and three (obvious ammonia odor, putrid) (Martinsdóttir et al., 2001) and were determined for shell-on and peeled samples. The first evaluation was 24 h after the shrimp was packed and thawed (5°C) within the packages. Sampling was performed after 1, 4, 7, 11, 14, 18, and 21 days. On each sampling day, five trays from each treatment were randomly withdrawn and evaluated for sensory (n = 2), microbial, chemical, and physical changes (n = 3).
The sensory evaluation was performed for each individual shrimp obtained from two trays from each treatment. The shrimp were given random three-digit numbers and placed on cooling plates. On sampling days, a total of 14 individual shrimp were assessed during the sensory evaluation for each treatment. Two reference samples (freshly thawed whole shrimp) were included to test the evaluators’ ability to differentiate test samples from fresh reference samples. Samples were evaluated by a trained panel consisting of five to six assessors. Based on QIM scores, samples were deemed to be spoiled on the first day, where the combined odor score for shell-on and peeled samples was three or above.
2.2.4 Chemical and physical changes
The headspace gas composition was determined for each tray (n = 3) using a gas analyzer (Checkmate3, MOCON Dansensor®, Ringsted, Denmark). Drip loss was measured as previously described (Dalgaard et al., 1993) for each tray (n = 3, averaging value of seven shrimp). Briefly, without squeezing the trays, liquid was allowed to drip from the trays and the weight of the liquid was expressed as % of the initial weight of product inside the package. Four shrimp were randomly taken from each tray (n = 3) and homogenized into a single sample using a kitchen blender to determine the chemical changes. The pH level was measured in a solution consisting of a 12.5 g blended shell-on sample and 37.5 mL distilled water. Total volatile nitrogen (TVN), trimethylamine-oxide (TMAO), and trimethylamine (TMA) were determined in triplicates at sampling times using a microdiffusion method (Conway and Byrne, 1933). TVN was determined on all sampling days, whereas TMAO and TMA were exclusively determined on days 1 and 21 to investigate if any increases in TVN could be explained by changes in TMAO and TMA. The color was assessed for all samples during storage by Hyperspectral imaging (HSI). HSI was performed with a VideometerLab 2 installed with 18 channels from 375 nm to 1,050 nm. Before the experiment, a series of HSI of shrimp from different production dates and storage conditions were taken to optimize the exposure time of each channel and saved as the systematic light setting for the color analysis. The system was calibrated radiometrically using a diffuse white, dark, and geometrical disc before each sampling point. Shrimp were placed in a plastic petri dish, and the HSI picture was taken using the software provided by Videometer. The HSIs were transformed into an image cube with the coordinates corresponding to the CIEL × a × b × color space, using the daylight50 correction in the Videometer software (VideometerLab 2, version 2.13.54, Videometer A/S, Denmark). To obtain the average L × (lightness), a × (+a, redness; −a, greenness) and b × (+b, yellowness; −b, blueness) coordinates for each shrimp, an in-house MATLAB script was applied (R2020b, The Mathworks Inc., MA, United States). Seven shrimp were analyzed per sample.
2.2.5 Microbiological plate counts
At the individual sampling day, three shrimp from each tray (n = 3 per treatment) were randomly chosen and aseptically weighed, approx. 30 g and 15 g of shrimp with and without heads, respectively, into sterile stomacher bags. The shrimp sample was then tenfold diluted in chilled physiological saline (PS) (0.85% NaCl and 0.1% Bacto-peptone, Oxoid, Basingstoke, United Kingdom) and homogenization for 60 s in a stomacher. Further tenfold dilutions in PS were performed as required. Aerobic Plate Count (APC) was determined by spread plating (100 μL) of suitable dilutions on prechilled plates of modified Long and Hammer agar (LH, NMKL, 2006) and by pour plating (1 mL) suitable dilutions in Iron Agar (IA) Lyngby (CM0964, Oxoid) with L-cysteine hydrochloride (Sigma Aldrich, St. Louis, United States). LH plates were incubated for 7 days at 15°C and IA plates for 3 days at 25°C. Presumptive Pseudomonas was determined by spread plating (100 µL) on Pseudomonads agar (CM0559, Oxoid) with selective supplement (CFC, SR0103, Oxoid) and incubation for 2 days at 25°C. Yeast and molds were determined by spread plating on Oxytetracycline-Glucose-Yeast Extract (OGYE) agar (CM0545, Oxoid) with Oxytetracycline Selective supplement (SR0073A, Oxoid) and incubation at 25°C for 5 days. APC and presumptive Pseudomonas sp. were enumerated on all sampling days. Concentrations of H2S-producing organisms, yeast, and mold were determined on the first day and the last day (21) of storage. The number of colony forming units (CFU) was enumerated following the indicated incubation periods and converted to log CFU/g. For the APC determined by IA, both white and black (H2S-producing organisms) colonies were counted, while a second CFU count was obtained from the same plates, by only counting the black colonies, to determine the H2S-producing organisms.
2.2.6 Identification of bacteria isolated from spoiled shrimp
To identify the dominating culturable microbiota for each treatment at the time of sensory spoilage, i.e., culture dependent analysis, all colonies on countable LH plates (n = 3 for each treatment) were divided into groups based on colony morphology. The proportion of each group was calculated, and of these ten proportionally representative colonies from each of the three plates were picked for each treatment, resulting in a total of 120 colonies, and directly streaked onto LH agar. Colonies on LH agar were characterized by biochemical tests (Gram-reaction, OF-test, catalase, oxidase as described by Dalgaard (1995a) and morphology leading to the selection of 76 representative bacterial isolates. The selected isolates were sub-cultured in brain heart infusion broth (BHI, CM1135B, Oxoid) supplemented with 1.5% NaCl for 48 h at 15°C and streaked onto BHI agar plates (CM1136, Oxoid). Isolates, which showed limited growth when incubated aerobically, were incubated in a modified atmosphere (40% CO2/60% N2) at 15°C.
For sequencing of the 16S rRNA gene of isolates, DNA was extracted by suspending colony mass in chilled PS followed by centrifugation (10,000 × g, 1 min) and removal of the supernatant. The pellet was resuspended in 200 µL 10× TE buffer and boiled for 10 min (MHR 23, Hettich Benelux, Geidermaisen, Netherlands) followed by centrifugation (10,000 x g, 5 min, Mikro 120, Hettich, Germany). The supernatant was used in the PCR for amplification of the 16S rRNA gene. Each reaction was composed of 17 µL nuclease free water, 25 µL DreamTaqTM PCR Master Mix, 2 µL of 10 µM forward primer (27F: AGAGTTTGATCCTGGCTCAG) 2 µL of 10 µM reverse primer (1492F: GGTTACCTTGTTACGACTT) and 4 µL supernatant (DNA). The PCR was composed of an activation step at 95°C for 10 min followed by 35 cycles of denaturering at 95°C for 30 s, annealing at 51°C for 60 s, and elongation at 72°C for 90 s. The PCR was concluded by a final elongation at 72°C for 7 min. The PCR product was purified by using the MinElute PCR Purification Kit (Qiagen, Hilden, Germany). The purified PCR product was sent to Eurofins Genomic for Sanger sequencing (Mix2Seq, Eurofins Genomics). Sequences were trimmed with the CLC workbench, by removing part of the sequences with low quality score (limit of 0.05) and the trimmed sequences were not allowed to have more than two ambiguous nucleotides (CLC workbench 8.1, Qiagen, Aarhus, Denmark). The trimmed sequences were assembled to reads with a minimum of 50 aligned base pairs and lengths of between 900 and 1,400 bp, and compared to the NCBI 16S ribosomal RNA sequence Database using their website service tool with the standard megablast option (https://blast.ncbi.nlm.nih.gov/Blast.cgi). The identified isolates (n = 76) were used to calculate the composition of the microbiota (% of each genus). Based on this identification, 24 representative isolates were further analyzed for their sensory spoilage potential (i.e., ability to produce off-odors in shrimp, described in Section 2.2.8).
2.2.7 Microbiota in fresh and spoiled samples determined by next-generation sequencing
Culture independent analysis, i.e., analysis which does not rely on the microbiota being culturable, was performed by 16S rRNA amplicon sequencing. Culture independent analysis was performed to investigate the qualitative composition of the microbiota at the start of the storage trial (all treatments) and at the point of sensory spoilage [whole shrimp in air (day 11), whole shrimp in MAP (day 18), and tails in air (day 14)] or at the end of the storage period [tails in MAP (day 21)]. Bacterial cells were harvested by centrifugation (10 min at 10,000 x g at 4°C) of aliquots of 10 mL of the stomacher bags (10−1 dilution) for all the treatments (n = 3 for each treatment). DNA was extracted from the resulting pellet using the PowerFood® Kit (Qiagen, Hilden, Germany) according to the manufacturer’s instructions. Library preparation was performed targeting the V3-V4 region of the 16S rRNA gene following the recommended method for 16S rRNA amplicon sequencing on the MiSeq Illumina platform (Illumina, 2021). In short, the extracted DNA was amplified using the 16S Amplicon PCR Forward Primer = 5′TCGTCGGCAGCGTCAGATGTGTATAAGAGACAGCCTACGGGNGGCWGCAG and 16S Amplicon PCR Reverse Primer = 5′GTCTCGTGGGCTCGGAGATGTGTATAAGAGACAGGACTACHVGGGTATCTAATCC. The PCR product was washed with 80% ethanol and transferred to a new amplicon plate in 10 mM Tris pH 8.5. A second PCR was performed using the Nextera index kit primers, following a second wash step with ethanol. The final PCR product was quality checked using the Bioanalyzer DNA 1000 chip (Agilent, Santa Clara, CA, United States), and normalized before sample pooling. The resulting sequences were analyzed using the Quantitative Insights Into Microbial Ecology 2 (QIIME2) (Bolyen et al., 2019) with the DADA2 package (Callahan et al., 2016) and the standard operating procedure [https://github.com/LangilleLab/microbiome_helper/wiki/Amplicon-SOP-v2-(qiime2-2020.8)]. The SILVA 138.1 SSU Ref NR 99 database (Quast et al., 2013) was used to assign taxonomical classification for the amplicon sequence variants (ASVs), following filtration and trimming of the reads with the amplicon region primers using RESCRIPt (Robeson et al., 2021).
2.2.8 Spoilage potential of representative bacterial isolates
The spoilage potential was determined for 24 isolates as their ability to produce off-odors following growth on whole, shell-on cooked shrimp stored at 5°C. Isolates were pre-cultured in BHI with 1.5% NaCl at 15°C and harvested in the late exponential phase after 2–3 days at 15°C. Whole shell-on shrimp were boiled for 5 min to reduce the endogenous microbiota, and three aliquots of 100 g were inoculated by 1% diluted preculture (4 × 0.25%, v/v) to achieve initial concentrations of ∼4 log CFU/g. After each addition, the samples were manually tumbled to ensure an even distribution of the inoculum. Non-inoculated control samples were prepared by adding sterile PS (1% v/w). Samples inoculated with isolates originally from shrimp or tails packed in bags with atmospheric air were packed and stored similarly (see section 2.2.2) and evaluated after 7 days at 5°C. Similarly, shrimp inoculated with isolates derived from shrimp or tails packed in MA, was MAP and stored for 18 days at 5°C before assessment. Non-inoculated control samples were subjected to both storage treatments. The ability of the isolates to produce off-odors was assessed by five to ten assessors using a three-point scale: (1) no off-odor, (2) weak off-odor, or (3) strong off-odor. The spoilage potential was recorded as present for isolates with off-odor scores of 2.0 or above. Off-odor characteristics were noted.
The quantitative spoilage activity was determined for 14 isolates (two Carnobacterium, ten Pseudoalteromonas, one Psychromonas and one Vibrionaceae) using the same inoculation and storage conditions as described above. Non-inoculated control samples were also prepared. For each isolate and uninoculated controls, the cell concentration (LH agar) and the concentrations of TVN and TMA (see above) were determined immediately after inoculation (n = 1) and at the end of storage (n = 2, at 7 and 18 days at 5°C for samples packed in air and MA, respectively).
2.3 Challenge tests for the growth of Listeria monocytogenes
2.3.1 Growth of L. monocytogenes in whole shrimp, shrimp meat and shrimp shell
Cooked shrimp were either kept whole (shell-on) or peeled and divided into meat and shell (the shrimp head was discarded). These shrimp and shrimp parts were inoculated with an equal mixture of four bacterial isolates of L. monocytogenes (94-203D, 94-54A, 95-442A and 94-167B), originating from seafood including shrimps (Jørgensen and Huss, 1998). Individual strains were precultured in Brain Heart Infusion broth at 8°C (BHI, Oxoid CM225), pH 7.5, 0.5% NaCl, and then incubated for 1–2 days. Precultures were harvested in the late exponential growth phase, defined as a relative change in absorbance of 0.05–0.2 at 540 nm (Novaspec II, Pharmacia LKB Biochrom, Cambridge, United Kingdom). An inoculum of L. monocytogenes with a concentration of 104 CFU/mL were prepared by mixing the four precultures and diluting in 0.85% NaCl. The inoculum (1% v/w) was added to bags with the whole shrimp or shrimp parts to achieve an initial level of approx. 102 L. monocytogenes per gram. The inoculum was added as four portions (4 × 0.25% v/w) to the bags. After each addition, the samples were manually tumbled, by thoroughly shaking the bags for 2 min each time, to ensure an even distribution of L. monocytogenes. The samples (approx. 20 g) were placed in trays and packed in modified atmosphere initially containing 40% CO2 and 60% N2 (AGA Ltd., Copenhagen, Denmark). This was performed using a Multivac A300/16 packaging machine (Multivac, Vejle, Denmark) and packaging film (NEN 40 HOB/LLPDE 75, Amcore Flexibles, Horsens, Denmark) with low gas permeability (See Section 2.2.2). The gas/shrimp ratio was approximately 1:2 for all samples.
2.3.1.1 Storage conditions, sampling and product characteristics
The temperature was recorded throughout storage by data loggers (Tinytag, Gemini Data Loggers Ltd, Chichester, United Kingdom). At regular intervals during the storage period, three packs (n = 3) from each treatment were analyzed by microbiological methods. pH was measured in the stomacher bags (dilution 10−1). The dry matter (DM) content was measured as the percentage of remaining mass after 24 h drying of 2 g shrimp at 105°C and performed in duplicates. The salt content was determined using an automated potentiometric titration method. The salt and dry matter content were used to calculate water phase salt (WPS). Finally, the headspace gas composition was measured in the packs at regular intervals during storage using a Combi Check 9800-1 gas analyzer (PBI, Dansensor, Ringsted, Denmark).
2.3.1.2 Microbiological analysis
Twenty grams of shrimp were diluted 10-fold in chilled, physiological saline (0.85% NaCl) with 0.1% peptone (PS) and homogenized 60 s in a Stomacher 400 (Seward Medical, London, United Kingdom). Further appropriate 10-fold dilutions of the homogenates were made in chilled PS. L. monocytogenes was enumerated by spread plating (37°C, 2 days) onto Palcam agar (CM0877, Oxoid) with Palcam Selective Supplement (SR0150, Oxoid). The colony forming unit (CFU) was enumerated following the indicated incubation period and converted to log CFU/g.
2.3.2 Effect of pH on growth of L. monocytogenes in shrimp meat and shell
Cooked shrimp were peeled and divided into meat and shell (with cephalothorax). Samples were frozen with liquid N2 and finely chopped (Waring commercial blender 38BL41, CT, United States). The pH of the shell samples was adjusted with HCl to lower pH to approx. 7, whereas meat samples were adjusted with NaOH to achieve pH values of approx. 8–8.5. Non-adjusted samples were used as controls. Samples were inoculated and packed as described above, and product characteristics and microbiological analysis were performed as described in Section 2.3.1.
2.4 Data analysis
Mean and standard deviation (SD) were calculated for the storage trial for all results. The two-sample Student’s t-test or 2-way ANOVA was used to analyze for significant differences (p < 0.05) among treatments. Before the ANOVA the homoscedasticity and normality of the data was verified. GraphPad Prism version 9.5.0 was used to conduct statistical analyses and to visualize data.
For the amplicon sequencing data, the Shannon entropy was used to express the alpha diversity (Shannon, 1948), and the beta diversity was calculated using the Jaccard similarity index to express the drastic taxonomic difference in the microbiota between pairs of samples (Jaccard, 1912). The calculations of the Shannon entropy and Jaccard Similarity index were performed as part of the bioinformatic pipeline [https://github.com/LangilleLab/microbiome_helper/wiki/Amplicon-SOP-v2-(qiime2-2020.8)].
A MultiBlock Common Dimension (ComDim) analysis was performed to investigate relationships in data or highlight common structures between these different blocks of data. The relationships between the four different data blocks, i.e., plate counts, ASVs, chemical and physical characteristics, and sensory scores observed in samples of fresh and spoiled whole shrimp and tails (head removed) following storage in air or MAP were explored using the multivariate unsupervised MultiBlock ComDim analysis. This unsupervised method aims at simultaneously exhibiting common dimensions shared by the various blocks of data and associated with each common dimension, a vector of saliences depicting the degree of agreement of each block with this common dimension (Cariou et al., 2018; Luong et al., 2021).
According to Luong et al. (2021), pre-processing to reduce low count ASVs (to remove background noise and focus on the most abundant ASVs) was done on rarefied 16S rRNA sequencing data prior to the ComDim analysis. ASVs with an abundance of ≤0.1% in the samples were removed, reducing the number of reads by 1.2% and the number of unique genera from the original 76 to 26. The Total Sum Scaling was performed on ASVs to normalize data (Bodein et al., 2019; Cardinal et al., 2022).
The modeling strategy linked plate counts (LH, IA, and CFC), the filtered ASVs, and sensory scores with the chemical and physical block (TVN, drip loss, pH) as suggested by Cardinal et al. (2022) and using a customized R-script. The salience, i.e., the importance of the different data blocks investigated, was analyzed as part of the analysis. The MultiBlock ComDim analysis was prepared in R-studio (version 3.6.3, R Foundation for Statistical Computing, 2021) and the plot was visualized using the ggplot2 package (Wickham, 2016).
To determine the lag phase (days) and maximum specific growth rate (μmax/h) of L. monocytogenes, the logistic growth model with delay (Dalgaard, 1995b) was used to fit the data, and a Student’s t-test was used to determine differences between samples. Growth rates were predicted by the cardinal parameter model of Mejlholm and Dalgaard (2009) included in the Food Spoilage and Safety Predictor (FSSP v.4) software (Dalgaard, 2014) with a relative lag time of 4.5. To evaluate the performance of this growth model, bias- and accuracy factors values were calculated from observed and predicted maximum specific growth rates (µmax) (Ross, 1996). The µref–value of the cardinal parameter model was then calibrated to data for whole cooked shrimps as previously described (Koukou et al., 2021).
3 Results
3.1 Storage trial for determination of sensory shelf-life
3.1.1 Sensory changes and shelf-life
Freshly thawed samples (blind control) never reached scores above 2. Air-stored samples spoiled for whole shrimp and tails after 11 and 14 days, respectively. In contrast, storage in MAP yielded shelf-lives of 18 and 21 days, respectively, for whole shrimp and tails (Figure 1; Table 1). This showed that storage in MAP increased the sensory shelf-life by 7 days, whereas removal of the head increased the sensory shelf-life by 3 days. The spoilage off-odor attributes of aerobically stored samples were described as ammonia-like, while the MAP-stored samples harbored off-odors described as sour, with an occasional hint of ammonia (Table 1).
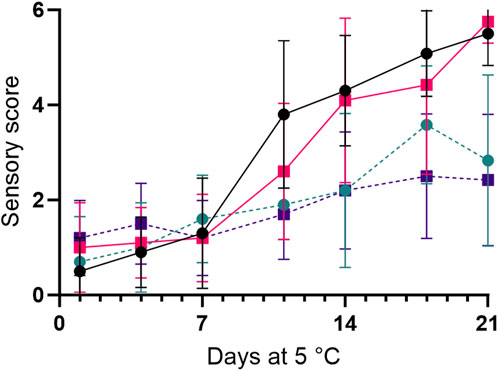
Figure 1. Sensory scores for odor for shell-on and peeled whole shrimp packed in air (—,

Table 1. Sensory shelf-life and spoilage attributes of shell-on whole shrimp and tails stored in air or MAP at 5°C.
3.1.2 Physical and chemical changes
After 7 and 11 days of storage in air, the microbiota of whole shrimp and tail samples, respectively, had consumed the O2 (decrease from 20% to 1%) in the packages and produced CO2 (final levels 4%). For shrimp in MAP, the initial CO2 level (40%) decreased more for whole shrimp (to 15%) compared to tails (24%; Supplementary Figure S3). The initial drip loss was similar for samples stored in air and MAP. The drip loss remained unchanged for MAP-stored shrimp (4%–6%) during storage, whereas reabsorption of the drip was observed for shrimp packed in air during the 21 days of storage (Figure 2). The initial pH was slightly higher in whole shrimp (7.7 ± 0.02) than in tails (7.5 ± 0.04) and increased until values for all four treatments reached 8.4 ± 0.05 on day 11 (data not shown). The initial content of TVN was low at 2–5 mg-N/100 g for whole shrimp and tails (Figure 3). After 11 days of refrigerated aerobic storage, levels of TVN rose to more than 60 mg-N/100 g for both whole shrimp and tails. The increase in TVN was significantly (p < 0.05) higher for tails than for whole shrimp in air after 14 days of storage (Figure 3). In contrast, much less TVN was formed in MAP shrimp (whole and tails) where close to 35 mg-N TVN/100 g were observed at sensory spoilage. Concentrations of TMAO at the beginning of the storage were 73 ± 9 and 66 ± 6 mg-N/100 g for whole shrimp and tails, respectively, while levels of TMA were 1–3 mg-N/100 g. After 21 days in aerobic storage, TMA concentrations rose to levels of 67 ± 16 and 60 ± 13 mg-N/100 g for whole shrimp and tails, respectively. For whole MAP shrimp, approximately 35% of the TMAO became converted to TMA to reach a final level of 44 ± 12 mg-N/100 g, whereas 5 ± 7 mg-N TMA/100 g was formed after 21 days in MAP tails.
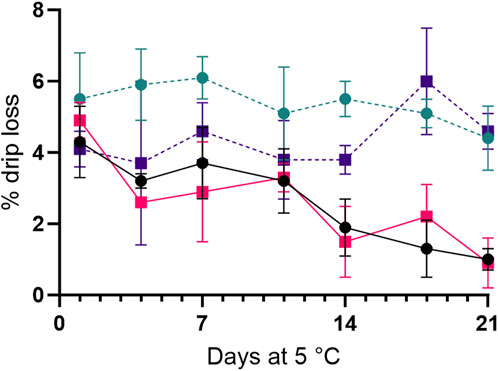
Figure 2. Drip loss (%) for shell-on whole shrimp packed in air (—,
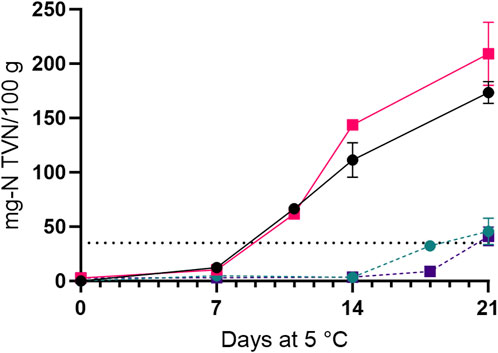
Figure 3. Content of total volatile nitrogen (TVN, mg-N/100 g) in whole shrimp in air (—,
The measured color of the shrimp did not change during storage using the HSI-technology (Supplementary Figure S2).
3.1.3 Microbial changes
Initial APCs determined by LH were 2.6 ± 0.2 and 2.4 ± 0.2 log CFU/g for whole shrimp and shrimp tails, respectively. APC on LH and IA reached >8 log CFU/g after 7 days of aerobic storage for both whole shrimp and tails, while this level was not attained until day 18 and day 21 for whole shrimp and tails stored in MAP, respectively (Figures 4A, B). Concentrations of presumptive Pseudomonas on CFC agar reached significantly (p < 0.05) higher levels, 7–8 log CFU/g, in aerobic storage compared to 4–5 log CFU/g reached for samples stored in MA. CFC counts were generally lower compared to APC based on LH (Figures 4A, C). Regardless of the storage and packaging conditions, low concentrations of H2S-producing bacteria were sporadically seen in IA, and no yeast or molds concentrations were above the detection level (2 log CFU/g, data not shown).
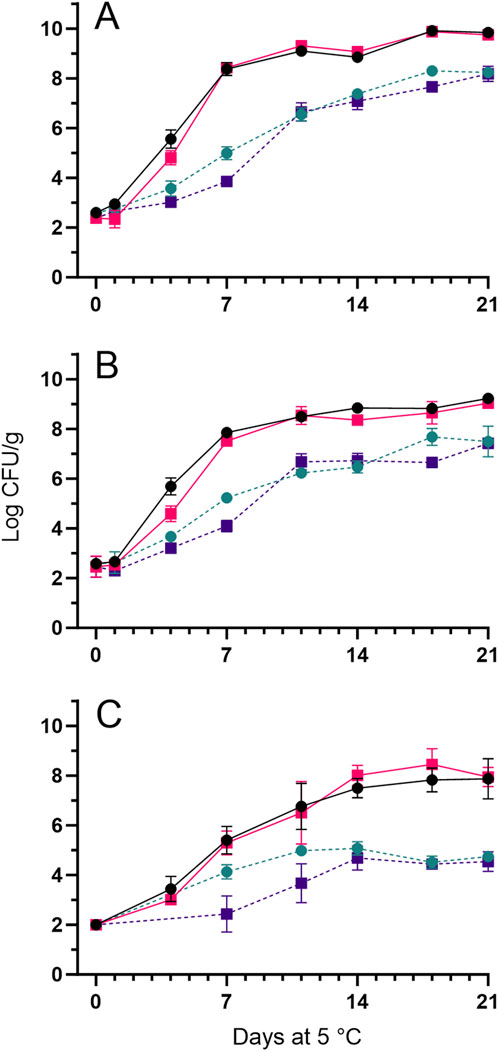
Figure 4. Microbial growth during storage at 5°C of whole shrimp in air (—,
Characterization of the 76 bacterial isolates obtained on the day of sensorial spoilage revealed that Pseudoalteromonas spp. dominated the microbiota (78%–92%) for shrimp products packed in air (Table 2). For whole shrimp and tails stored in MAP, the microbiota was dominated by Carnobacterium spp. with proportions of 79% and 99%, respectively (Table 2).
3.1.4 Microbiota in fresh and spoiled samples determined by next-generation sequencing
The 16S rRNA amplicon sequencing of the 18 samples resulted in 5,022,851 reads, of which 66.9% were successfully merged. After filtration, 57.7% of non-chimaera merged reads were used for further analysis. The sampling depth was set to 10,000 reads, at which point rare fraction curves leveled off for all samples. One fresh tail sample had insufficient reads (<10,000) and was filtered out during the analysis. The Shannon entropy was between 3.2 ± 1.4 and 4.5 ± 0.5 for fresh whole shrimp and tail samples. Aerobic storage reduced the index to 2.4 ± 0.1 and 1.9 ± 0.2 in spoiled whole shrimp and tail samples, respectively (Figure 5). Similar values of 2.2 ± 0.3 and 2.1 ± 0.3 were calculated for spoiled whole shrimp and tail samples in MAP, respectively. The beta diversity (Figure 6) was mainly affected by storage time (PCoA1, 44.87%), while storage conditions (air versus MAP) explained 12.35% of the variation (PCoA2, Figure 6). Removing the head of the shrimp caused no difference in the microbiota in the air-stored samples, while a noticeable difference in the beta diversity was observed for the MAP products with the appearance of Vibrionaceae in tail samples (Figures 5, 6).
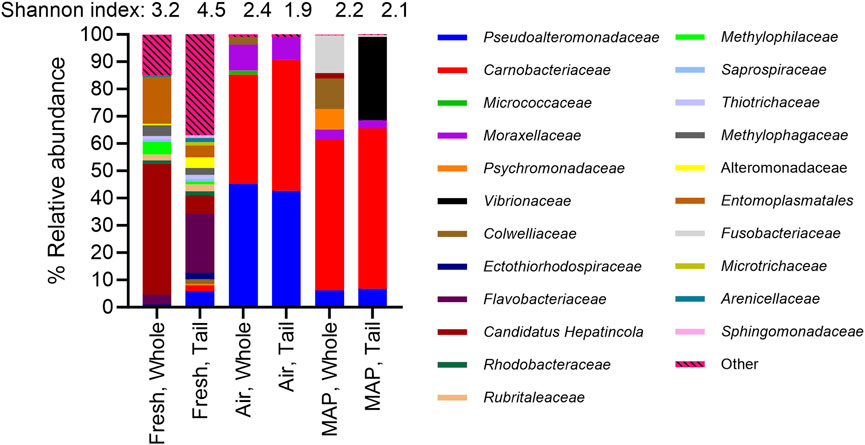
Figure 5. Comparison of the relative abundance (%) of the bacterial families found in fresh and spoiled samples of shell-on whole shrimp and tails following storage in air or MAP at 5°C for up to 21 days, by culture-independent analysis (16S rRNA amplicon sequencing). Other categories contain bacterial families with an abundance of less than 0.2%. The Shannon index of samples is also shown as measure of species richness (alpha diversity).
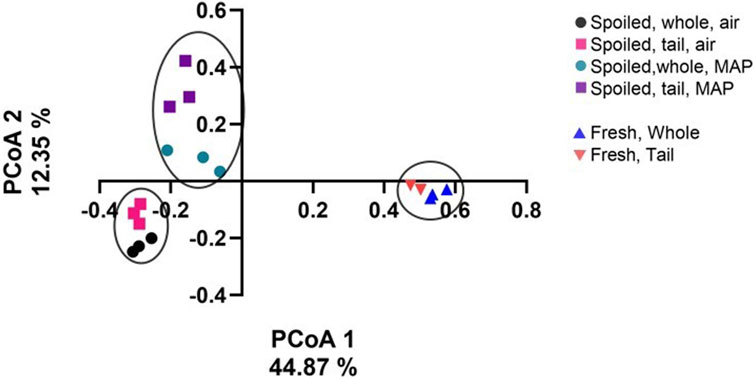
Figure 6. Beta diversity shown by using the Jaccard similarity index and Principal Coordinate Analysis of the microbiota in fresh and spoiled samples of shell-on whole shrimp and tails following stored in air or MAP at 5°C for up to 21 days. Circles have no statistical properties.
Differences between the microbiota-derived from amplicon sequencing (culture-independent) and the identification of isolates (culture-dependent) were especially noted for air-stored samples, where a larger proportion of ASVs was assigned to Carnobacteriaceae (40%–47%) compared to isolates from culture-dependent microbiology (0%–21%). Several genera found by the culture-independent analysis were not found in the culture-dependent analysis, e.g., Colwelliaceae, Fusobacteriaceae, and Candidatus Hepatincola. A higher abundance of Vibrionaceae were found in MAP-stored tail samples using the amplicon sequencing compared to the 16S rRNA sanger sequencing of isolates.
3.1.5 Spoilage potential and amine formation by the bacterial isolates
Eight of the nine isolates of Pseudoalteromonas sp. produced off-odors and formed high levels of TVN (>100 mg-N/100 g) and TMA (>50 mg-N/100 g) (Table 3). Carnobacterium sp (n = 1), Psychrobacter sp. (n = 1), Glutamicibacter sp (n = 1) and one isolate of Pseudoalteromonas sp. did not produce off-odors, TVN or TMA (Table 3). Of the 12 isolates tested for spoilage potential in modified atmosphere, one isolate of Pseudoalteromonas sp. and one isolate of Photobacterium sp. were found to have a spoilage potential and to produce TVN levels of >40 and >80 mg-N/100 g and TMA levels of >40 and 60 mg-N/100 g, respectively. Neither Carnobacterium sp., nor Psychromonas sp. produced off-odors in MAP. However, both species produced TVN, and Psychromonas sp. also produced TMA.
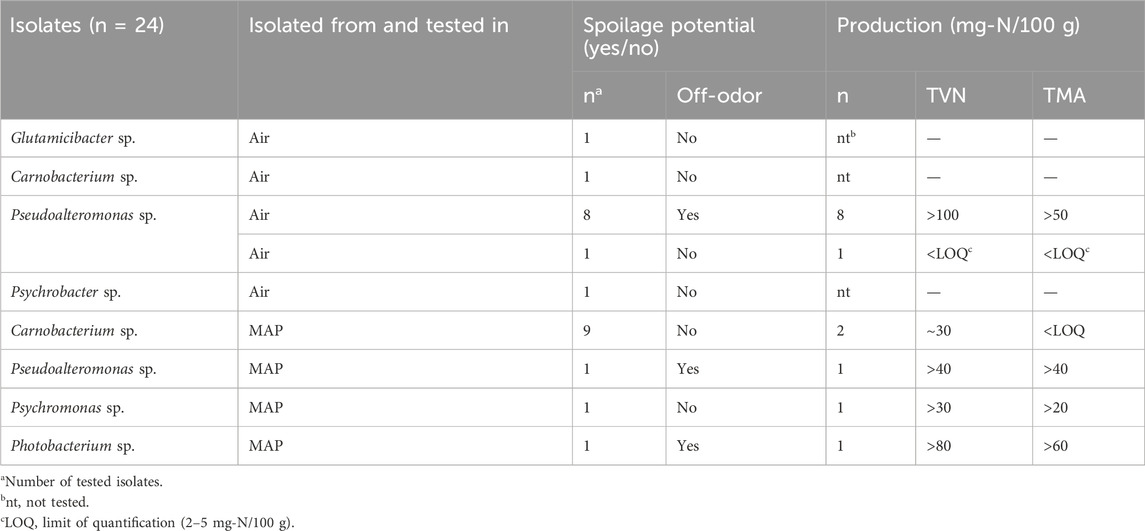
Table 3. Spoilage potential and average production of TVN and TMA for selected bacterial isolates during growth in shrimp at 5°C in air or MAP.
3.1.6 Multiblock ComDim analysis
The Multiblock ComDim salience analysis of the four data blocks (sensory, microbial plate counts, ASVs and chemical/physical data) showed that >97% of the variability among fresh and spoiled samples of whole shrimp and tails stored in air or MAP was explained by the first three dimensions (Supplementary Table S1). Based on the salience, it was chosen to show the results of dimension 1 against dimension 3 (Figure 7). Dimension 1 showed that increases in the plate counts on LH, CFC and IA (green dots), pH and Carnobacterium ASVs separated fresh and spoiled samples on the left and right side of the plot, respectively (Figure 7A). Also, variances among fresh and spoiled samples (dimension 1) were explained by changes in the microbiota, with more genera (ASVs) being present in fresh (blue dots) than in spoiled samples. Spoiled MAP samples were explained by variances in ASVs belonging to Psychromonas, Psychrilyobacter and Colwellia, together with drip loss (dimension 3), while variances in spoiled air samples were explained by ASVs of Pseudoalteromonas, Glutamicibacter and Psychrobacter, together with TVN, putative Pseudomonas counts on CFC and odor of the samples (Figure 7B).
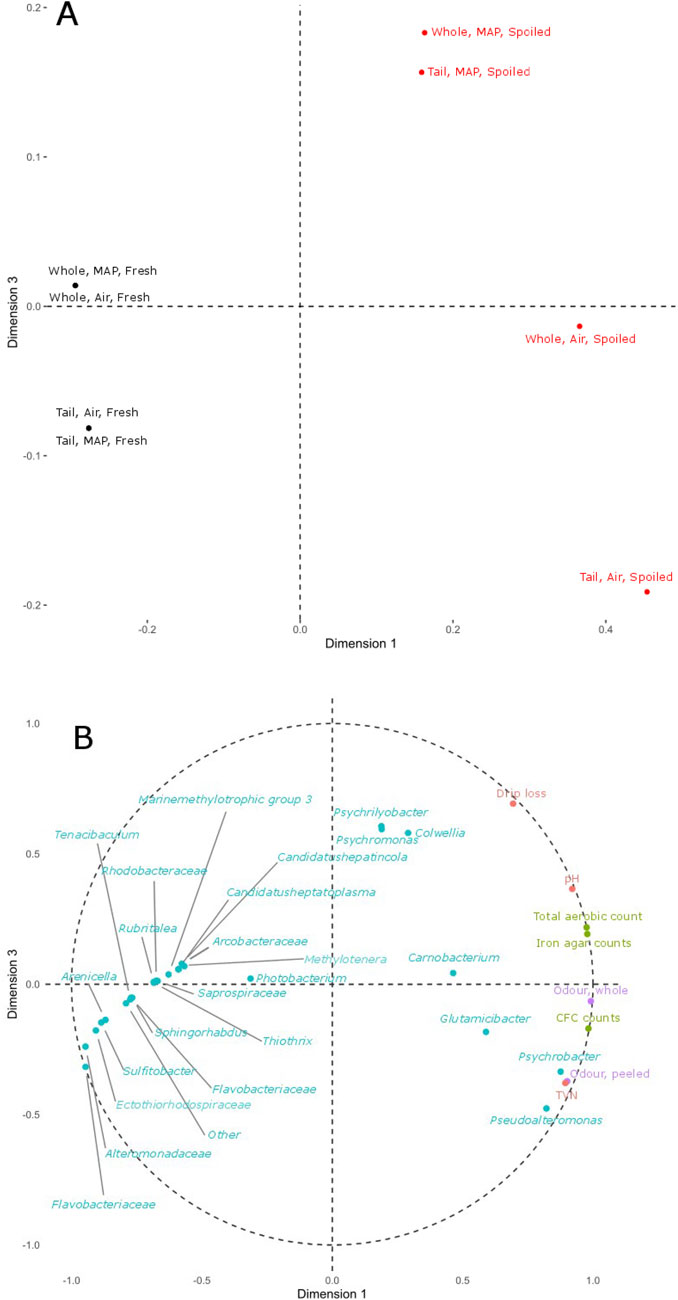
Figure 7. Score plot (A) with fresh and spoiled samples of whole shrimp and tails in air or MAP as dimension 1 (71.8%) and dimension 3 (10.9%). Black font indicate samples which contributed to less than 0.025% of the variance, whereas red font indicate samples which contributed to more than 0.025% of variance explained on dimension 1 and 3. Circle plot of loadings (B) of variables from sensory (purple), chemical/physical (red), culture-dependent (green) and culture-independent (blue) analysis.
3.2 Challenge tests for the growth of L. monocytogenes
3.2.1 Growth of L. monocytogenes in whole shrimp, shrimp meat and shrimp shell
The growth of L. monocytogenes was studied at 2°C because this is the typical storage temperature for seafood in Danish supermarkets. In contrast, 15°C was chosen to speed up the growth of psychrotrophic bacteria, which thrive at low temperatures but grow markedly faster at higher temperatures. At 2°C, L. monocytogenes reached significantly (p < 0.05) higher cell concentrations and growth rates (>6 log CFU/g; 0.022 1/h) in the whole shrimp compared to the shrimp meat or shell (∼5 log CFU/g and 0.011–0.013 1/h). This pattern was not observed at 15°C and the faster growth of L. monocytogenes in whole shrimp compared to shrimp meat did not seem to result from faster growth in shrimp shell that had a slightly higher pH (Figure 8; Table 4). It was attempted to change pH in shrimp shell and meat to study the effect on growth rates of L. monocytogenes, but no clear effect was observed (data not shown). However, the authors could not adjust pH as much as initially planned. Thus, average values are reported in Table 4.
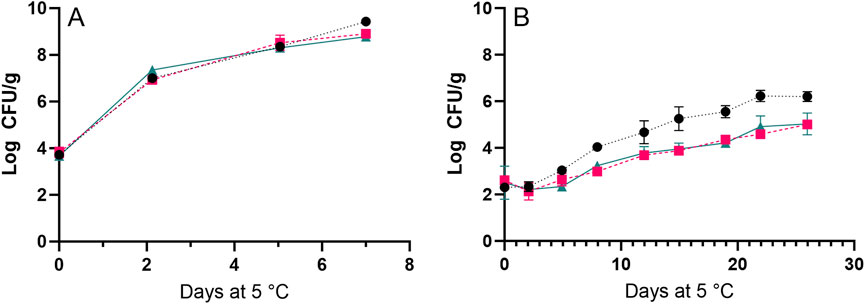
Figure 8. Growth of Listeria monocytogenes on the whole shrimp (
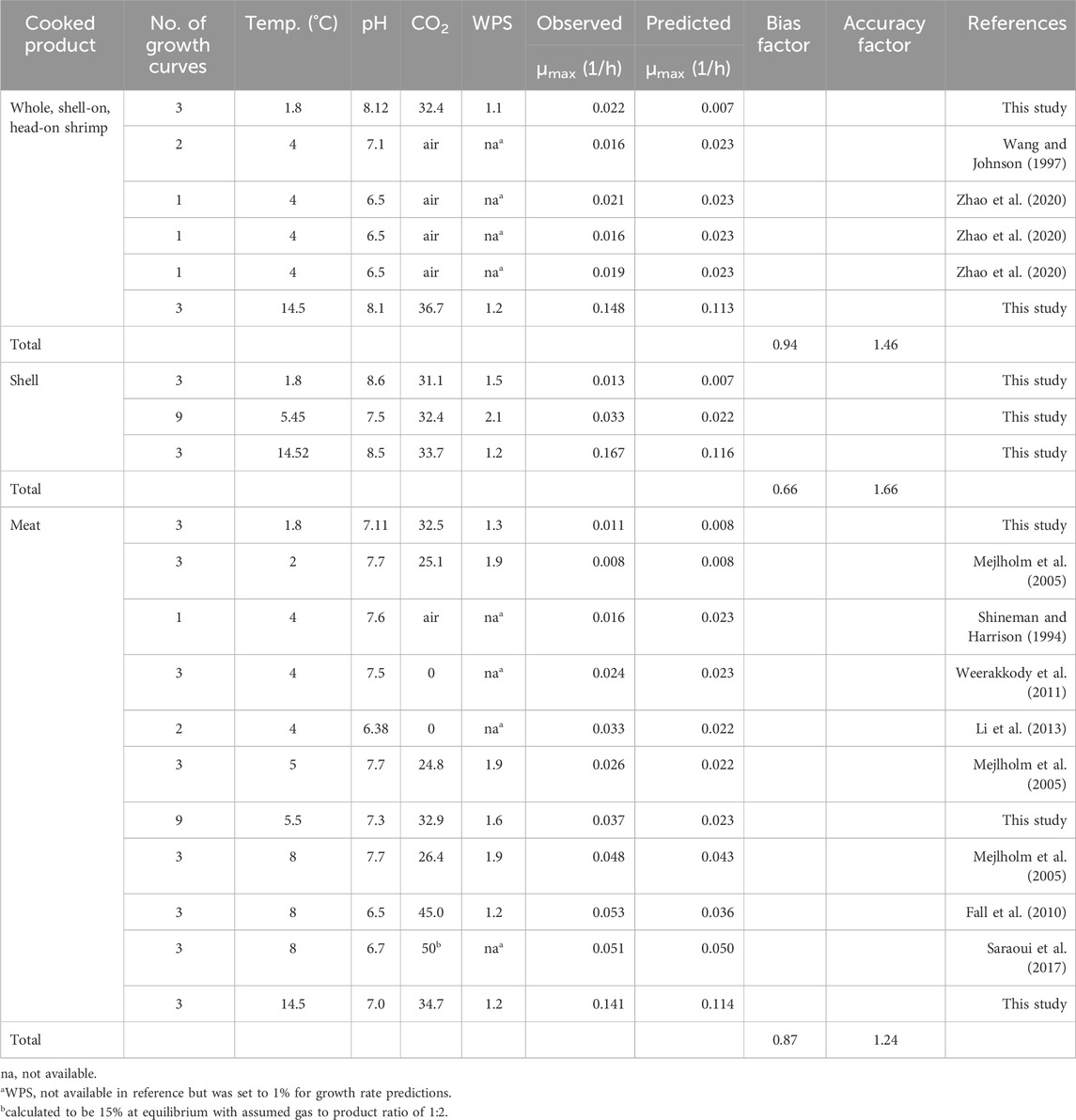
Table 4. Comparison of observed growth rates of Listeria monocytogenes from the present and the literature study and growth rates predicted with FSSP based on temperature, pH, %CO2, and water phase salt (WPS), together with calculated Bias- and Accuracy factors.
3.2.2 Evaluation of the Food Safety and Spoilage Predictor (FSSP) to predict growth of L. monocytogenes in shrimp products
The FSSP software predicted lower growth rates than observed for all samples investigated in this study resulting in a Bf–value of 0.65 (n = 36) (data not shown). When using data from the literature in addition to the data from this study, FSSP predicted growth rates of L. monocytogenes in whole shrimp and shrimp meat more accurately with a Bf–value of 0.94 (n = 11) and 0.87 (n = 33), respectively (Table 4).
3.2.3 Estimations of safe shelf-life
The FSSP software allows for calibration of the current model by updating µref. This was done based on the average Bf observed for whole shrimp (Bf = 0.94, n = 11) and the shrimp meat (Bf = 0.87, n = 33) of the current study. A standard relative lag time of 4.5 was added to the predicted time for a 100-fold increase. The results gave predictions of 9 days at 5°C and 24 days at 2°C based on data from whole shrimp (pH 8, 1.1% WPS, and 32% CO2), whereas the safe shelf-life from the observed growth in the tail meat was 8 days at 5°C and 23 days at 2°C (pH 7.1, 1.3% WPS and 32% CO2).
Once the model was calibrated, FSSP was used to predict the growth under realistic storage temperatures in the food supply chain, i.e., storage at retail followed by storage at the consumer. Predictions of the time for a 100-fold increase during 7 days of storage in retail at 2°C, followed by storage at 5°C at the consumer level, resulted in safe shelf-lives of 13–14 and 12–13 for the calibrated models of whole shrimp and shrimp meat respectively (Table 5). As expected, increasing the storage temperatures in the two parts of the food supply chain caused the safe shelf-life to shorten.
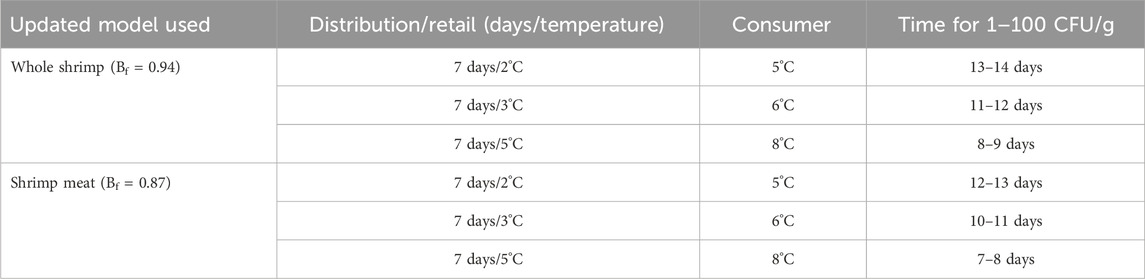
Table 5. Different scenarios with different storage times and temperatures at two different steps in the food supply chain, i.e., distribution/retail and at the consumer, with the corresponding predicted time for L. monocytogenes to increase a 100-fold (CFU/g).
4 Discussion
4.1 Storage trials
4.1.1 Sensory shelf-life determinations
Whole shrimp stored aerobically spoiled after 11 days of storage, whereas tails spoiled after 14 days of storage. Whole shrimp stored in MAP spoiled after 18 days of storage whereas tails in MAP spoiled after 21 days at 5°C. The extended shelf-life of tail samples is likely due to their composition, which is primarily meat, unlike whole shrimp samples that include the gastrointestinal system. The beneficial effect of removing the head shown in this study for cooked shrimp is supported by similar results for raw shrimp by Shakila et al. (1995) for raw Parapenaeopsis stylifera shrimp stored in ice and by Layrisse and Matches (1984) for raw P. platyceros stored in modified atmospheres. In contrast, Alvarez and Koburger (1979) found that the head removal affected neither microbial counts nor the sensory acceptability of raw Penaeus shrimp, which may, however, be due to a high initial APC (6 log CFU/g). In addition to the 3-day shelf-life extension, beheading effectively prevents the quality issue of the dark-colored shrimp heads, where industry guidelines demote batches with high level of dark-colored heads to a lower value and quality grade (company communication, Royal Greenland Seafood). The marked shelf-life increase observed for samples stored in MAP was similar to observations by Sivertsvik et al. (1997) for shell-on cooked P. borealis at 3°C and for cooked and peeled P. borealis stored in MAP at 5°C (Mejlholm et al., 2005). The shelf-life extension of MAP is more pronounced for cooked shrimp than for fresh seafood where psychrotolerant and CO2 resistant spoilage bacteria limit the product shelf-life (Dalgaard, 2006). Interestingly, similar off-odors have been observed in C&P and raw shrimp stored under comparable conditions suggesting common spoilage reactions (Mejlholm et al., 2005; Qian et al., 2013).
4.1.2 Physical and chemical changes
During storage, the CO2 content in aerobically stored samples increased to about 4%, as O2 was depleted after 11 days of storage. Similar results were obtained by Noseda et al. (2012) for cooked and peeled Cr. crangon stored in different modified atmospheres. This change is likely caused by the consumption of O2 by the microbiota, which produces CO2 by respiration. For shrimp stored in MAP, the initial CO2 levels (40%) decreased to 15% and 24% for whole and tails, respectively, due to the CO2 being dissolved in the water phase, which amounts to approx. 80% of the product. Similar decreases in CO2 were seen by Mejlholm et al. (2005) for cooked and peeled P. borealis with similar gas-to-product ratio and by Sivertsvik et al. (1997) for whole cooked P. borealis with a gas-to-product ratio of 1:1. Drip loss during aerobically stored shrimp decreased of storage, while drip loss of shrimp stored in MA remained stable at 5%–6%. The effect of MAP on drip loss was also observed by Kimbuathong et al. (2020) for Litopenaeus vannamei. Matches and Layrisse (1985) similarly observed a greater drip loss for MAP-stored, raw P. platyceros compared to air storage for 20 days. We found no studies reporting on the reabsorption of liquid in cooked air-stored shrimp. In contrast to the results of this study, an increasing drip loss is commonly reported during storage in air for other fishery products (Fagan et al., 2003; Kaale et al., 2014). The drip loss occurs due to autolysis and bacterial activity during storage (Huss, 1995). The authors hypothesize that the formation of biofilm in the packages may retain the drip and thereby reduce the measurable drip loss.
Whole shrimp and tails reach high TVN levels (>100 mg-N/TVN) when stored in air compared to MAP (<50 mg-N/TVN) after 21 days of storage. TMA levels reached >60 mg-N/100 g and between 5 and 44 mg-N/100 g for shrimp in air and MAP, respectively. The initial low content of TVN (2–5 mg-N/100 g) is likely a result of the shrimp being cooked and frozen within hours of being caught. Notably, for shrimp stored in air, TVN concentrations at sensory spoilage was not higher than the EU critical limits of 25-35 mg-N TVN/100 for finfish (EU, 1022/2008) not the limit of 50 mg-N TVN/100 g of peeled shrimp suggested by Nesbakken and Solberg (1981). For shrimp stored in MAP, the EU limit was reached at the point of sensory spoilage. High contents of TVN of 126 mg-N/100 g after 14 days at 5°C have previously been shown in aerobically stored whole, cooked P. borealis (Bøe et al., 1982). In that study, TVN levels correlated with pH increases. However, we found TVN levels to increase later than pH, and a pH increase was also seen for MAP samples with lower TVN levels (data not shown), indicating that non-volatile compounds contributed to the change in pH. The high concentration of TVN corresponds to the ability of Pseudoalteromonas spp. (Table 3) to produce ammonia from amino acid and urea as previously documented in shrimp, skate and squid (Paarup et al., 2002; Laursen et al., 2006; Reynisson et al., 2012; Broekaert et al., 2013b). It is unlikely that Carnobacterium spp. have contributed to the reduction of 66–73 mg-N TMAO to form 60–67 mg-N TMA/100 g of shrimp samples in air (Table 3; Mejlholm et al., 2005). Paarup et al. (2002) found Pseudoalteromonas spp. to be unable to produce TMA from TMAO in a soft agar assay. However, in the present study, nine out of 10 isolates of Pseudoalteromonas spp. produced TMA when growing in shrimp (Table 3). These results correspond to data from Yin et al. (2019) and Zhang et al. (2020), where the growth yield for five of 16 Pseudoalteromonas shioyasakiensis isolates increased when cultured with TMAO. Moreover, DNA sequencing revealed the presence of the catalytic subunit of TMAO reductase within the genomes of these Pseudoalteromonas. Taken together, it seems relevant to further study the role of Pseudoalteromonas spp. as an SSO involved in sensory spoilage, including TMA formation, in shrimp and seafood products. Furthermore, Vibrionaceae/Photobacterium are known TMA producers (Dalgaard, 1995a; Sørensen et al., 2020) and together with Pseudoalteromonas spp. may have formed the TMA and TVN observed in the MAP shrimp (Table 3).
4.1.3 Microbial changes
The observed change in the microbiota from a predominant Gram-negative microbiota (CFC agar) in air-packaged, to a microbiota dominated by Gram-positive bacteria in MAP was also observed by Noseda et al. (2012) for refrigerated brown shrimp, indicating that Gram-positive species were more tolerant to the presence of CO2. Studies on whole, cooked Cr. crangon also found Pseudoalteromonas to be present in large numbers during aerobic iced storage, however, Psychrobacter became the most abundant genus at the time of microbial spoilage (Broekaert et al., 2013a). Studies on refrigerated cooked and peeled P. borealis (Mejlholm et al., 2005) and cooked, peeled and brined P. borealis (Dalgaard et al., 2003) in MAP found Carnobacterium spp. to dominate the spoilage microbiota. The finding of Vibrionaceae in the cooked MAP tail samples was unexpected as members of this genus are known to be heat-labile and inactivated by frozen storage (Emborg et al., 2002; Dalgaard, 2006). While its presence could be due to a contamination event, future studies should elucidate whether this isolate is more tolerant to frozen storage. The ability of other seafood-related Gram-negative bacteria to grow on the Pseudomonas CFC media was recently reported by Thomassen et al. (2022) indicating the limitation of this agar substrate for presumptive counts of Pseudomonas sp. in seafood. Counts on CFC may, therefore, not represent Pseudomonas spp. as also suggested by the lack of Pseudomonas isolates or abundance in the 16S rRNA sequencing results. A study by Jensen et al. (2024) also reported growth on CFC agar (four to five log CFU/g), without reporting the presence of Pseudomonas spp. in the product.
4.1.4 Changes in the microbiota of fresh and spoiled samples
Results from the amplicon sequencing (Figure 2) show that the Shannon entropy and the species richness is higher for fresh samples than for spoiled samples, a well-known phenomenon during the storage of foods, where only a part of the original microbiota grows to high levels as these are best adapted to the environment. The reduction in the number of species during the storage of shrimp was also demonstrated by Chaillou et al. (2015) for cooked MAP Pe. vannamei and by Parlapani et al. (2020) for air-stored Parapenaues longirostris. We found no other studies investigating the effect of the beheading of shrimp on microbial community composition.
Members of the genus Vibrionaceae were detected by the amplicon sequencing, and the 16S rRNA Sanger sequencing identified an isolate to be Photobacterium sp. in samples of the tails stored in MAP. The identification was unexpected as members of this genus are both heat-labile and expected to be inactivated during frozen storage of shrimp (Dalgaard, 2006; Dalgaard et al., 2006). A study by Emborg et al. (2002) showed that freezing at temperatures of −30°C was less inhibiting on Photobacterium phosphoreum compared to frozen storage at −20°C. It could, therefore, be possible that the species survived during the initial frozen transport and had not been completely inactivated during the final storage prior to the experimental start.
4.1.5 Spoilage potential
Of the 24 isolates tested, 10 isolates were found to have spoilage potential (Table 3), including Pseudoalteromonas sp. in air and MAP and Photobacterium sp. in MAP. This study is not the first to report on the spoilage potential of Pseudoalteromonas sp. in cooked shrimp. Macé et al. (2014) showed the potential for a combination of Pseudoalteromonas spp. and Psychrobacter spp. to cause slight sensory spoilage (sour off-odors) of whole, cooked tropical shrimp during MAP storage. Notably, one isolate of Pseudoalteromonas did not produce off-odors, TVN, or TMA (Table 3), indicating variable activity among members of this genus and the need for further studies of the role of Pseudoalteromonas spp. as an SSO for shrimp and other seafood products. The lack of spoilage potential, despite some TVN formation (∼30 mg-N/100 g), by Carnobacterium sp. in our study was unexpected. In fact, isolates of C. divergens and C. maltaromaticum have previously been shown to form both TVN and off-flavors in cooked and peeled MAP P. borealis at 5°C (Mejlholm et al., 2005; Laursen et al., 2006). However, other isolates belonging to C. mobile, C. maltaromaticum, and C. divergens have been demonstrated not to produce off-flavors in shrimp products (Laursen et al., 2006; Saraoui et al., 2017), indicating variability in the spoilage potential between groups and strains within Carnobacteriaceae.
4.1.6 Multiblock ComDim as a tool for investigating relationships in data
The use of the Multiblock ComDim tool allowed for the discovery of major changes in the complexity of the microbiota and sensory and chemical/physical product characteristics that were relatable to the main treatments, i.e., storage time, removal of the head, and packaging in MA. Wiernasz et al. (2021) similarly used the Multiblock ComDim tool to highlight differences in the spoilage patterns among three different, lightly preserved salmon-based products. Other multivariate statistics, e.g., non-metric multidimensional scaling analysis, have been shown to be useful in investigating relationships between sequencing data and volatile compounds during storage of beef (Jääskeläinen et al., 2016) and redundancy analysis for relationships between chemical/physical changes (color, drip loss, pH, etc.) and sequencing data during storage of tuna (Wang and Xie, 2020). The potential for using Multiblock ComDim to investigate relationships in large data sets shows promising results and could aid researchers when investigating the causes of sensory spoilage in the future.
4.2 Challenge tests with L. monocytogenes
4.2.1 Growth of L. monocytogenes in shrimp at 2°C and 15°C
At 15°C the growth rates were higher in the shell than in both pure meat and whole shrimp, whereas lowering the temperature to 2°C changed the picture. At 2°C the growth was faster in the whole shrimp than in both the meat and the shell. The higher growth rate in the shell at ∼15°C was unexpected as fewer nutrients are expected to be available in the shell. The shrimp shell consists of chitin which is a polymer of the amino sugar N-acetylglucosamine. The faster growth in the shell at 15°C, could potentially be explained by the chitinolytic properties of L. monocytogenes as investigated by Leisner et al. (2008). They explained that Listeria spp. can hydrolyze the chitin in the shrimp shell and use it for growth. The higher levels and growth rate of L. monocytogenes in whole shrimp at 2°C suggest that the nutrients in the whole shrimp promote bacterial growth more effectively than the nutrients in just the meat and shell. However, this effect might be significant only at lower temperatures. Additional studies are needed to validate these results.
We found no other data on the growth of L. monocytogenes in whole, cooked Northern shrimp. In contrast to the results of the present study, Wan Norhana et al. (2010) did not find strains of L. monocytogenes to be able to grow on raw shrimp carapace (shell of the shrimp head-region). However, the cells persisted on the surface of the carapace throughout storage at 4°C and were found to grow on cooked shrimp meat. The authors did not propose a cause for these differences. More studies are needed to determine the causes of the differences in growth between the parts of the shrimp. Data for other shrimp species shows a large variation between the growth rates of studies on whole (shell-on), cooked shrimp. Wang and Johnson (1997) reported a growth rate of 0.016 1/h at 4°C, Zhao et al. (2020) reported growth rates of 0.016–0.021 for three strains of L. monocytogenes at 4°C (Table 4) and Niu et al. (2018) reported growth rates of 0.048 1/h and 0.125 1/h at 4°C and 10°C, respectively. A packaging atmosphere of 100% CO2 did not permit growth of L. monocytogenes at 3°C during 15 days of storage. In the same study, using the same experimental design, L. monocytogenes grew with a rate of 0.041 1/h when stored in air (Rutherford et al., 2007). Mejlholm et al. (2005) similarly investigated the growth of L. monocytogenes in cooked and peeled (C&P) Northern shrimp in MAP (50% CO2/30% N2/20% O2) at 2°C. They reported a growth rate of 0.0074 1/h, much lower than the 0.022 1/h observed for whole shrimp and lower than the 0.011 1/h observed for the shrimp meat in our study. A similar tendency was seen for the growth at 5°C, when compared with the results from the study investigating the effect of pH on the growth. The growth observed in shrimp meat in our study should be comparable to the results of Mejlholm et al. (2005) as both are products of shrimp meat from the Northern shrimp. However, the processing of the two products is very different. After catch shrimp destined for the C&P shrimp product are stored on ice during the transport to the shore. Subsequently, the product is matured for several days in ice or salt brine, followed by steam cooking for 90 s at 100° C (Dang et al., 2018; Gringer et al., 2020). The cooked and cooled shrimp are then peeled, IQF, and glazed with water. In contrast, there is no maturation step in the processing of whole, cooked shrimp. Thus, the leaching of potentially important nutrients during the maturation in ice or salt brine (Hoegh, 1989) could be the cause for the lower observed growth in C&P shrimp compared to the meat of whole, cooked shrimp. The lower growth rate in C&P shrimp observed by Mejlholm et al. (2005) was not assumed to be caused by the effect of CO2, as equilibrium CO2 levels were lower (∼25%) than observed for shrimp meat in the present study (∼32%). Similar to results from Mejlholm et al. (2005), Paranjpye et al. (2008) reported growth rates of 0.021 1/h at 5°C in air and vacuum for C&P P. jordani, indicating that O2 levels are of little importance to the growth of L. monocytogenes in shrimp.
4.2.2 Effect of pH
Results revealed no clear effect of pH, likely caused by the limited range of pH investigated. This was due to difficulties with adjusting pH of shrimp meat and shell. However, as growth rates in the shell (pH 8.6) and growth in the meat (pH 7.0–7.3) at lower temperatures were comparable (Table 4), the pH is likely not the main cause for the differences in growth rates of L. monocytogenes. More studies should be carried out to thoroughly investigate the importance of pH. It would be interesting to conduct additional experiments to investigate whether the chitinolytic properties of Listeria are responsible for the observed growth, or if Listeria thrives on the tissue that remains attached to the shell after peeling.
4.2.3 Evaluation of FSSP for prediction of growth in cooked shrimp
According to European legislation (EU, 2073/2005), food producers must ensure that L. monocytogenes does not exceed 100 CFU/g in RTE food products like whole, cooked shrimp. Predictive modeling is less time-consuming and more cost-effective than performing challenge tests for determining the product shelf-life. An example of predictive modeling is the FSSP software, which incorporates a model validated on C&P, brined Northern shrimp (Mejlholm and Dalgaard, 2009). Currently, it is not known if the model accurately predicts L. monocytogenes growth in whole, shell-on, cooked Northern shrimp. In the present study, data (shrimp pH, storage temperature, etc.) from the challenge tests were used to obtain predicted growth rates using the generic model in FSSP. Due to the model limitations, a maximum pH of 8 was used for predictions. Only Wang and Johnson (1997) and Zhao et al. (2020) reported on the product characteristics of the whole, shell-on cooked shrimp, and are included in Table 4. The predicted growth rates for L. monocytogenes based on data from this study (Table 4) are lower than what is observed in the challenge tests. Thus, using the model to predict the time for L. monocytogenes to grow from 1 CFU/g to 100 CFU/g (i.e., the maximum allowable amount; EC, 2073/2005), would produce fail-dangerous predictions. This means that L. monocytogenes would reach 100 CFU/g earlier than predicted and the product may then pose a health risk to the consumers. A comparison of observed growth rates from the literature with the predicted growth rates from FSSP shows that the model is acceptable for whole, cooked shrimp of other species. In some cases, FSSP even predicts faster growth than observed in the respective studies. This could indicate that either L. monocytogenes grows differently in other shrimp species or that the information on the product characteristics was incorrect, or that the differences are caused by strain variances. More growth rates (>20) are needed to completely validate/calibrate the model for it to be used for shell-on cooked shrimp.
4.2.4 Estimations of safe shelf-life
The updated model predicts the time to 100 CFU/g to be 20 days at 2°C, including the lag phase. However, as the Bf was calculated as an average Bf of the Bf from only the two temperatures, the time to 100 CFU/g is longer (20 days) than when using only the results of the challenge test performed at 2°C (7–11 days) as is evident from the growth shown in Figure 8B. To be able to sufficiently calibrate the model, more growth curves at a wider range of temperatures should be performed and used for final calibration.
The temperature varies along the food supply chain (FSC) (Göransson et al., 2018), and these temperature variations will undoubtedly affect the safe shelf-life of the products. Furthermore, the temperatures of the refrigerators in the homes of consumers vary to a great extent (Kennedy et al., 2005; Roccato et al., 2017). The important effect of storage temperature is also seen by the difference in the estimated shelf-lives where the shelf-life is reduced by 7–10 days by storing the shrimp at 5°C rather than 2°C Additionally, when taking the fluctuating storage temperatures into account, the estimated safe shelf-life is shortened even further (Table 5). These estimations are consistent with United Kingdom guidelines of implementation of shelf-lives of less than 10 days at 3°C–8°C to mitigate the risk of non-proteolytic Clostridium botulinum in MAP seafood products (ACMSF, 2020).
5 Conclusion
Removal of the head region increases the sensory shelf-life with up to 3 days for shell-on cooked Northern shrimp stored at 5°C. Storage in a modified atmosphere prolongs this shelf-life by an additional 7 days. However, these sensory shelf-lives are shortened due to the potential growth of L. monocytogenes during refrigerated storage. Pseudoalteromonas and Carnobacterium spp. dominated the spoilage microbiota in air and MAP-stored shrimp. Further studies of Pseudoalteromonas spp. are relevant to elucidate their growth, metabolism, and spoilage activity in Northern shrimp products. This study provides vital information on both the sensory and safe shelf-lives of frozen and thawed cooked Northern shrimp. It describes the beneficial effect of the removal of the head-region of the shrimp, which could additionally reduce shipping costs. With the combination of MAP, an even longer shelf-life can be obtained and thereby reducing the food loss in the food supply chain. However, the current study is an example of how the sensory shelf-life is longer than the safe shelf-life for an RTE product. In this case, the safe shelf-life is 9–10 days at 5°C or 16–20 days at 2°C. Our results highlight the need for companies to incorporate safety aspects into all product development to ensure the safety of consumers, as numerous Listeria outbreaks are caused by cooked RTE meat products indicating that reliance on cooking and hygiene is inadequate (SSI, 2025; Takeuchi-Storm et al., 2023). Research into ways to stabilize the products against the growth of L. monocytogenes is highly recommended.
Data availability statement
The datasets presented in this study can be found in online repositories. The names of the repository/repositories and accession number(s) can be found below: https://www.ncbi.nlm.nih.gov/, SUB13521083.
Ethics statement
Ethical approval was not required for the studies involving humans because Participants volunteered to taste products prior to the tasting. The studies were conducted in accordance with the local legislation and institutional requirements. Written informed consent for participation was not required from the participants or the participants’ legal guardians/next of kin in accordance with the national legislation and institutional requirements because it was not considered to be needed at the time of study start.
Author contributions
HJ: Conceptualization, Formal Analysis, Investigation, Visualization, Writing – original draft, Writing – review and editing. KS: Investigation, Writing – review and editing. SG: Investigation, Writing – review and editing. JS: Formal Analysis, Investigation, Visualization, Writing – review and editing. SM: Formal Analysis, Methodology, Writing – original draft. NB: Conceptualization, Funding acquisition, Resources, Supervision, Writing – review and editing. OM: Conceptualization, Funding acquisition, Resources, Supervision, Writing – review and editing. PD: Conceptualization, Funding acquisition, Methodology, Supervision, Writing – review and editing.
Funding
The author(s) declare that financial support was received for the research and/or publication of this article. This work was funded by Innovation Fund Denmark [grant no. 9065-00263B].
Acknowledgments
We thank laboratory technicians Giulia Martini, Nanna H. Scheel and Rannvá H. Houmann for their help with storage trials. Researcher Martin Laage Kragh is thanked for skillful help with preparations for the Sanger sequencing work. We thank the sensory panel for their contribution.
Conflict of interest
Authors HJ, NB, and OM were employed by Royal Greenland Seafood A/S.
The remaining authors declare that the research was conducted in the absence of any commercial or financial relationships that could be construed as a potential conflict of interest.
Generative AI statement
The author(s) declare that no Generative AI was used in the creation of this manuscript.
Publisher’s note
All claims expressed in this article are solely those of the authors and do not necessarily represent those of their affiliated organizations, or those of the publisher, the editors and the reviewers. Any product that may be evaluated in this article, or claim that may be made by its manufacturer, is not guaranteed or endorsed by the publisher.
Supplementary material
The Supplementary Material for this article can be found online at: https://www.frontiersin.org/articles/10.3389/frfst.2025.1572265/full#supplementary-material
References
ACMSF (2020). Subgroup on non-proteolytic Clostridium botulinum and vacuum and modified atmosphere packaged foods. Advis. Comm. Microbiol. Saf. Food. Available online at: https://acmsf.food.gov.uk/sites/default/files/acmsf-vpmap-subgroup-report_1.pdf. (Accessed September 14, 2024).
Alvarez, R. J., and Koburger, J. A. (1979). Effect of delayed heading on some quality attributes of Penaeus shrimp. J. Food Prot. 42, 407–409. doi:10.4315/0362-028x-42.5.407
Bodein, A., Chapleur, O., Droit, A., and Lê Cao, K. A. (2019). A generic multivariate framework for the integration of microbiome longitudinal studies with other data types. Front. Genet. 10, 963–1018. doi:10.3389/fgene.2019.00963
Bøe, B., Losnegaard, N., and Xu, X. L. (1982). Determination of indole as a freshness assessment of shrimp. Fisk. Dir. Skr. Ser. Ernær. II, 35–38.
Bolyen, E., Rideout, J. R., Dillon, M. R., Bokulich, N. A., Abnet, C. C., Al-Ghalith, G. A., et al. (2019). Reproducible, interactive, scalable and extensible microbiome data science using QIIME 2. Nat. Biotechnol. 37, 852–857. doi:10.1038/s41587-019-0209-9
Broekaert, K., Heyndrickx, M., Herman, L., Devlieghere, F., and Vlaemynck, G. (2013a). Molecular identification of the microbiota of peeled and unpeeled brown shrimp (Crangon crangon) during storage on ice and at 7.5°C. Food Microbiol. 36, 123–134. doi:10.1016/j.fm.2013.04.009
Broekaert, K., Noseda, B., Heyndrickx, M., Vlaemynck, G., and Devlieghere, F. (2013b). Volatile compounds associated with Psychrobacter spp. and Pseudoalteromonas spp., the dominant microbiota of brown shrimp (Crangon crangon) during aerobic storage. Int. J. Food Microbiol. 166, 487–493. doi:10.1016/j.ijfoodmicro.2013.08.013
Callahan, B. J., McMurdie, P. J., Rosen, M. J., Han, A. W., Johnson, A. J. A., and Holmes, S. P. (2016). DADA2: high-resolution sample inference from Illumina amplicon data. Nat. Methods 13, 581–583. doi:10.1038/nmeth.3869
Cardinal, M., Bézier, L., Máce, S., Courcoux, P., and Vigneau, E. (2022). “Quality of smoked salmon in factory: relationship between sensory data, microbiological counts and sequencing data using ComDim analysis,” in Conference for the west European fisheries technology association, rotterdam, The Netherlands.
Cariou, V., Qannari, E. M., Rutledge, D. N., and Vigneau, E. (2018). ComDim: from multiblock data analysis to path modeling. Food Qual. Prefer 67, 27–34. doi:10.1016/j.foodqual.2017.02.012
Carpentier, B., and Cerf, O. (2011). Review - persistence of Listeria monocytogenes in food industry equipment and premises. Int. J. Food Microbiol. 145, 1–8. doi:10.1016/j.ijfoodmicro.2011.01.005
Chaillou, S., Chaulot-Talmon, A., Caekebeke, H., Cardinal, M., Christieans, S., Denis, C., et al. (2015). Origin and ecological selection of core and food-specific bacterial communities associated with meat and seafood spoilage. ISME J. 9, 1105–1118. doi:10.1038/ismej.2014.202
Chandrasekaran, M. (1994). Methods for preprocessing and freezing of shrimps: a critical evaluation. J. Food Sci. Technol. 31, 441–452.
Conway, E. J., and Byrne, A. (1933). An absorption apparatus for the micro-determination of certain volatile substances. The micro-determination of ammonia. J. Biochem. 27, 419–429.
Dalgaard, P. (1995a). Qualitative and quantitative characterization of spoilage bacteria from packed fish. Int. J. Food Microbiol. 26, 319–333. doi:10.1016/0168-1605(94)00137-U
Dalgaard, P. (1995b). Modelling of microbial activity and prediction of shelf life for packed fresh fish. J. Food Microbiol. 26, 305–317. doi:10.1016/0168-1605(94)00136-t
Dalgaard, P. (2006). Microbiology of marine muscle foods. Handb. Food Sci. Technol 999–1017. doi:10.1201/b15995-58
Dalgaard, P. (2014). Food safety and spoilage predictor. Available online at: http://fssp.food.dtu.dk/. (Accessed September 11, 2024).
Dalgaard, P., Gram, L., and Huss, H. H. (1993). Spoilage and shelf-life of cod fillets packed in vacuum or modified atmospheres. Int. J. Food Microbiol. 19, 283–294. doi:10.1016/0168-1605(93)90020-H
Dalgaard, P., Madsen, H. L., Samieian, N., and Emborg, J. (2006). Biogenic amine formation and microbial spoilage in chilled garfish (Belone belone belone) - effect of modified atmosphere packaging and previous frozen storage. J. Appl. Microbiol. 101, 80–95. doi:10.1111/j.1365-2672.2006.02905.x
Dalgaard, P., Vancanneyt, M., Vilalta, N. E., Swings, J., Fruekilde, P., and Leisner, J. J. (2003). Identification of lactic acid bacteria from spoilage associations of cooked and brined shrimps stored under modified atmosphere between 0°C and 25°C. J Appl Microbiol 94, 80–89. doi:10.1046/j.1365-2672.2003.01806.x
Dang, T. T., Gringer, N., Jessen, F., Olsen, K., Bøknæs, N., Nielsen, P. L., et al. (2018). Emerging and potential technologies for facilitating shrimp peeling: a review. Innov. Food Sci. Emerg. Technol. 45, 228–240. doi:10.1016/j.ifset.2017.10.017
Das, J., and Mishra, H. N. (2023). A comprehensive review of the spoilage of shrimp and advances in various indicators/sensors for shrimp spoilage monitoring. Food Res. Int. 173, 113270. doi:10.1016/j.foodres.2023.113270
Dewitt, C. A. M., and Oliveira, A. C. M. (2016). Modified atmosphere systems and shelf life extension of fish and fishery products. Foods 5, 48–27. doi:10.3390/foods5030048
Emborg, J., Laursen, B. G., Rathjen, T., and Dalgaard, P. (2002). Microbial spoilage and formation of biogenic amines in fresh and thawed modified atmosphere-packed salmon (Salmo salar) at 2°C. J. Appl. Microbiol. 92, 790–799. doi:10.1046/j.1365-2672.2002.01588.x
EU (2005). Commision regulation (EC) No 2073/2005 of 15 November 2005 on microbiological criteria for foodstuffs. Official Journal of the European Union.
EU (2008). Commission Regulation (EC) No 1022/2008 of 17 October 2008 amending Regulation (EC) No 2074/2005 as regards the total volatile basic nitrogen (TVB-N) limits. Official Journal of the European Union.
Fagan, J. D., Ronan Gormley, T., and Mhuircheartaigh, M. U. (2003). Effect of freeze-chilling, in comparison with fresh, chilling and freezing, on some quality parameters of raw whiting, mackerel and salmon portions. LWT - Food Sci. Technol. 36, 647–655. doi:10.1016/S0023-6438(03)00084-7
Fall, P. A., Leroi, F., Chevalier, F., Guerin, C., and Pilet, M. F. (2010). Protective effect of a non-bacteriocinogenic Lactococcus piscium CNCM I-4031 strain against Listeria monocytogenes in sterilized tropical cooked peeled shrimp. J. Aquat. Food Prod. Technol. 19, 84–92. doi:10.1080/10498850.2010.486910
FAO (2024). Species fact sheets: Pandalus borealis (Krøyer, 1838). Rome, Italy: Food and Agriculture Organisation. Available online at: https://www.fao.org/fishery/en/aqspecies/3425/en (accessed September 10, 2024).
Göransson, M., Nilsson, F., and Jevinger, A. (2018). Temperature performance and food shelf-life accuracy in cold food supply chains – Insights from multiple field studies. Food Cont. 86, 332–341. doi:10.1016/j.foodcont.2017.10.029
Gram, L., and Dalgaard, P. (2002). Fish spoilage bacteria - problems and solutions. Curr. Opin. Biotechnol. 13, 262–266. doi:10.1016/S0958-1669(02)00309-9
Gringer, N., Skytte, J. L., Dang, T. T., Orlien, V., Olsen, K., Schlippè-Steffensen, K., et al. (2020). Effect of ice maturation, freezing and heat treatment on the peelability and quality of cold water shrimps (Pandalus borealis). LWT 134, 110139. doi:10.1016/j.lwt.2020.110139
Gudbjörnsdóttir, B., Suihko, M. L., Gustavsson, P., Thorkelsson, G., Salo, S., Sjöberg, A. M., et al. (2004). The incidence of Listeria monocytogenes in meat, poultry and seafood plants in the Nordic countries. Food Microbiol. 21, 217–225. doi:10.1016/S0740-0020(03)00012-1
Gudmundsdóttir, S., Gudbjörnsdóttir, B., Einarsson, H., Kristinsson, K. G., and Kristjánsson, M. (2006). Contamination of cooked peeled shrimp (Pandalus borealis) by Listeria monocytogenes during processing at two processing plants. J. Food Prot. 69, 1304–1311. doi:10.4315/0362-028X-69.6.1304
Hansen, P., and Aagard, J. (1968). Dybhavsrejen (Pandalus). Kongens Lyngby: Fiskeriministeriets forsøgslaboratorium.
Hoegh, L. (1989). Quality index for shrimp [in Danish]. PhD thesis. Denmark: Technical University of Denmark.
Huss, H. H. (1995). Quality and quality changes in fresh fish. Rome: FAO Fisheries and Aquaculture. Technical Paper no. 348.
Illumina (2021). 16S metagenomic sequencing library preparation preparing 16S ribosomal RNA gene amplicons for the illumina MiSeq system. San Diego, California: Illumina.
Jääskeläinen, E., Hultman, J., Parshintsev, J., Riekkola, M. L., and Björkroth, J. (2016). Development of spoilage bacterial community and volatile compounds in chilled beef under vacuum or high oxygen atmospheres. Int. J. Food Microbiol. 223, 25–32. doi:10.1016/j.ijfoodmicro.2016.01.022
Jaccard, P. (1912). The distribution of the flora in the alpine zone. New Phytol. 11, 37–50. doi:10.1111/j.1469-8137.1912.tb05611.x
Jensen, H. A., Plaza, L. G., Houmann, R. H., Andreasen, P. B., Bøknæs, N., Kragh, M. L., et al. (2024). Microbial and chemical stability of lumpfish (Cyclopterus lumpus) roe during refrigerated storage in acid-salt brines with or without antioxidants. Front. Food. Sci. Technol. 4, 1498035. doi:10.3389/frfst.2024.1498035
Jørgensen, L. V., and Huss, H. H. (1998). Prevalence and growth of Listeria monocytogenes in naturally contaminated seafood. Int. J. Food Microbiol. 42, 127–131. doi:10.1016/S0168-1605(98)00071-3
Kaale, L. D., Eikevik, T. M., Rustad, T., and Nordtvedt, T. S. (2014). Changes in water holding capacity and drip loss of Atlantic salmon (Salmo salar) muscle during superchilled storage. LWT - Food Sci. Technol. 55, 528–535. doi:10.1016/j.lwt.2013.10.021
Kennedy, J., Jackson, V., Blair, I. S., Mcdowell, D. A., Cowan, C., and Bolton, A. D. J. (2005). Food safety knowledge of consumers and the microbiological and temperature status of their refrigerators. J. Food Prot. 68, 1421–1430. doi:10.4315/0362-028x-68.7.1421
Kimbuathong, N., Leelaphiwat, P., and Harnkarnsujarit, N. (2020). Inhibition of melanosis and microbial growth in Pacific white shrimp (Litopenaeus vannamei) using high CO2 modified atmosphere packaging. Food Chem. 312, 126114. doi:10.1016/j.foodchem.2019.126114
Koukou, I., Mejlholm, O., and Dalgaard, P. (2021). Cardinal parameter growth and growth boundary model for non-proteolytic Clostridium botulinum – effect of eight environmental factors. Internat. J. Food. Microbio. 346, 109162. doi:10.1016/j.ijfoodmicro.2021.109162
Laursen, B. G., Leisner, J. J., and Dalgaard, P. (2006). Carnobacterium Species: effect of metabolic activity and interaction with Brochothrix thermosphacta on sensory characteristics of modified atmosphere packed shrimp. J. Agric. Food Chem. 54, 3604–3611. doi:10.1021/jf053017f
Layrisse, M. E., and Matches, J. R. (1984). Microbiological and chemical changes of spotted shrimp (Pandalus platyceros) stored under modified atmospheres. J. Food Prot. 47, 453–457. doi:10.4315/0362-028x-47.6.453
Leisner, J. J., Larsen, M. H., Jørgensen, R. L., Brøndsted, L., Thomsen, L. E., and Ingmer, H. (2008). Chitin hydrolysis by Listeria spp., including L. monocytogenes. Appl. Environ. Microbiol. 74, 3823–3830. doi:10.1128/AEM.02701-07
Li, M., Wang, W., Fang, W., and Li, Y. (2013). Inhibitory effects of chitosan coating combined with organic acids on Listeria monocytogenes in refrigerated ready-to-eat shrimps. J. Food Prot. 76, 1377–1383. doi:10.4315/0362-028X.JFP-12-516
Luong, N. D. M., Membré, J. M., Coroller, L., Zagorec, M., Poirier, S., Chaillou, S., et al. (2021). Application of a path-modelling approach for deciphering causality relationships between microbiota, volatile organic compounds and off-odour profiles during meat spoilage. Int. J. Food Microbiol. 348, 109208. doi:10.1016/j.ijfoodmicro.2021.109208
Macé, S., Cardinal, M., Jaffrès, E., Cornet, J., Lalanne, V., Chevalier, F., et al. (2014). Evaluation of the spoilage potential of bacteria isolated from spoiled cooked whole tropical shrimp (Penaeus vannamei) stored under modified atmosphere packaging. Food. Microbio. 40, 9–17. doi:10.1016/j.fm.2013.11.018
Martinsdóttir, E., Sveinsdóttir, K., Luten, J., Schelvis-Smit, G., and Hyldig, R. (2001). Reference manual for the fish sector: Sensory evaluation of fish freshness. Ijmuiden, Netherlands: QIM Eurofish.
Matches, J. R., and Layrisse, M. E. (1985). Controlled atmosphere storage of spotted shrimp (Pandalus platyceros). J. Food Prot. 48, 709–711. doi:10.4315/0362-028x-48.8.709
Mejlholm, O., Bøknæs, N., and Dalgaard, P. (2005). Shelf life and safety aspects of chilled cooked and peeled shrimps (Pandalus borealis) in modified atmosphere packaging. J. Appl. Microbiol. 99, 66–76. doi:10.1111/j.1365-2672.2005.02582.x
Mejlholm, O., and Dalgaard, P. (2009). Development and validation of an extensive growth and growth boundary model for Listeria monocytogenes in lightly preserved and ready-to-eat shrimp. J. Food Prot. 72, 2132–2143. doi:10.4315/0362-028X-72.10.2132
Mejlholm, O., Kjeldgaard, J., Modberg, A., Vest, M. B., Bøknæs, N., Koort, J., et al. (2008). Microbial changes and growth of Listeria monocytogenes during chilled storage of brined shrimp (Pandalus borealis). Int. J. Food Microbiol. 124, 250–259. doi:10.1016/j.ijfoodmicro.2008.03.022
Nesbakken, T., and Solberg, T. (1981). Quality changes in iced shrimps (Pandalus borealis). I. Changes in the contents of trimethylamide oxide and volatile nitrogen bases and bacteria in raw shrimps after different storage periods compared with organoleptic examinations. Nord. Veterinærmed. 33, 250–259.
Niu, B., Mu, L., Xiao, L., Zhang, Z., Malakar, P. K., Liu, H., et al. (2018). Reduction of infection risk mediated by co-culturing Vibrio parahaemolyticus and Listeria monocytogenes in refrigerated cooked shrimp. J. Sci. Food Agric. 98, 4454–4461. doi:10.1002/jsfa.8969
Noseda, B., Goethals, J., De Smedt, L., Dewulf, J., Samapundo, S., Van Langenhove, H., et al. (2012). Effect of O2CO2 enriched atmospheres on microbiological growth and volatile metabolite production in packaged cooked peeled gray shrimp (Crangon crangon). Int. J. Food Microbiol. 160, 65–75. doi:10.1016/j.ijfoodmicro.2012.09.018
Paarup, T., Sanchez, J. A., Moral, A., Christensen, H., Bisgaard, M., and Gram, L. (2002). Sensory, chemical and bacteriological changes during storage of iced squid (Todaropsis eblanae). J. Appl. Microbiol. 92, 941–950. doi:10.1046/j.1365-2672.2002.01604.x
Paranjpye, R. N., Peterson, M. E., Poysky, F. T., and Eklund, M. W. (2008). Incidence, growth, and inactivation of Listeria monocytogenes in cooked and peeled cold-water shrimp. J. Aquat. Food Prod. Technol. 17, 266–284. doi:10.1080/10498850802187118
Parlapani, F. F., Ferrocino, I., Michailidou, S., Argiriou, A., Haroutounian, S. A., Kokokiris, L., et al. (2020). Microbiota and volatilome profile of fresh and chill-stored deepwater rose shrimp (Parapenaeus longirostris). Food Res. Int. 132, 109057–109058. doi:10.1016/j.foodres.2020.109057
Peng, S., Wei, H., Zhan, S., Yang, W., Lou, Q., Deng, S., et al. (2022). Spoilage mechanism and preservation technologies on the quality of shrimp: an overview. Trends Food Sci. Technol. 129, 233–243. doi:10.1016/j.tifs.2022.09.024
Qian, Y. F., Xie, J., Yang, S. P., and Wu, W. H. (2013). Study of the quality changes and myofibrillar proteins of white shrimp (Litopenaeus vannamei) under modified atmosphere packaging with varying CO2 levels. Eur. Food Res. 236, 629–635. doi:10.1007/s00217-013-1918-9
Quast, C., Pruesse, E., Yilmaz, P., Gerken, J., Schweer, T., Yarza, P., et al. (2013). The SILVA ribosomal RNA gene database project: improved data processing and web-based tools. Nucleic Acids Res. 41, 590–596. doi:10.1093/nar/gks1219
R Foundation for Statistical Computing (2021). R: a language and environment for statistical computing. Vienna, Austria: R Foundation for Statistical Computing. Available online at: https://www.R-project.org/. (Accessed September 10, 2024).
Reynisson, E., Thór Marteinsson, V., Jónsdóttir, R., Magnússon, S. H., and Hreggvidsson, G. O. (2012). Bacterial succession during curing process of a skate (Dipturus batis) and isolation of novel strains. J. Appl. Microbiol. 113, 329–338. doi:10.1111/j.1365-2672.2012.05349.x
Robeson, M. S., O’Rourke, D. R., Kaehler, B. D., Ziemski, M., Dillon, M. R., Foster, J. T., et al. (2021). RESCRIPt: reproducible sequence taxonomy reference database management. PLoS Comput. Biol. 17, e10095811–e1009637. doi:10.1371/journal.pcbi.1009581
Roccato, A., Uyttendaele, M., and Membré, J. M. (2017). Analysis of domestic refrigerator temperatures and home storage time distributions for shelf-life studies and food safety risk assessment. Food Res. Int. 96, 171–181. doi:10.1016/j.foodres.2017.02.017
Ross, T. (1996). Indices for performance evaluation of predictive models in food microbiology. J. Appl. Bact. 81, 501–508. doi:10.1111/j.1365-2672.1996.tb03539.x
Rutherford, T. J., Marshall, D. L., Andrews, L. S., Coggins, P. C., Wes Schilling, M., and Gerard, P. (2007). Combined effect of packaging atmosphere and storage temperature on growth of Listeria monocytogenes on ready-to-eat shrimp. Food Microbiol. 24, 703–710. doi:10.1016/j.fm.2007.03.011
Saraoui, T., Cornet, J., Guillouet, E., Pilet, M. F., Chevalier, F., Joffraud, J. J., et al. (2017). Improving simultaneously the quality and safety of cooked and peeled shrimp using a cocktail of bioprotective lactic acid bacteria. Int. J. Food Microbiol. 241, 69–77. doi:10.1016/j.ijfoodmicro.2016.09.024
Shakila, R. J., Vasundhara, T. S., and Rao, D. V. (1995). Rapid quality assessment of shrimps during storage by monitoring amines. J. Food Sci. Technol. 32, 310–314.
Shannon, C. E. (1948). A mathematical theory of communication. Bell Syst. Tech. J. 27 (27), 379–423. doi:10.1002/j.1538-7305.1948.tb01338.x
Shineman, T. L., and Harrison, M. A. (1994). Growth of Listeria monocytogenes on different muscle tissues. J. Food Prot. 57, 1057–1062. doi:10.4315/0362-028X-57.12.1057
Sivertsvik, M., Rosnes, J. T., and Bergslien, H. (1997). “Shelf-life of whole cooked shrimp (Pandalus borealis) in CO2-enriched atmosphere,” in Seafood from producer to consumer, integrated approach to quality, developments in food science. Editors J. B. Luten, T. Børresen, and J. Oehlenschläger (Amsterdam, Netherlands: Elsevier Science), 221–230.
Sørensen, J. S., Bøknæs, N., Mejlholm, O., and Dalgaard, P. (2020). Superchilling in combination with modified atmosphere packaging resulted in long shelf-life and limited microbial growth in Atlantic cod (Gadus morhua L.) from capture-based-aquaculture in Greenland. Food Microbiol. 88, 103405. doi:10.1016/j.fm.2019.103405
Sriket, C. (2014). Proteases in fish and shellfish: role on muscle softening and prevention. Int. Food Res. J. 21, 433–445.
Sriket, C., Benjakul, S., and Visessanguan, W. (2010). Characterisation of proteolytic enzymes from muscle and hepatopancreas of fresh water prawn (Macrobrachium rosenbergii). J. Sci. Food Agric. 91, 52–59. doi:10.1002/jsfa.4145
Sriket, C., Benjakul, S., Visessanguan, W., and Kishimura, H. (2011). Collagenolytic serine protease in fresh water prawn (Macrobrachium rosenbergii): characteristics and its impact on muscle during iced storage. Food Chem. 124, 29–35. doi:10.1016/j.foodchem.2010.05.098
SSI (2025). Outbreak archive. Statens serum institut. Available online at: https://www.ssi.dk/sygdomme-beredskab-og-forskning/sygdomsudbrud/arkiv (Accessed January 20, 2025).
Takeuchi-Storm, N., Hansen, L. T., Nielsen, N. L., and Andersen, J. K. (2023). Presence and persistence of Listeria monocytogenes in the Danish Ready-to-Eat food production environment. Hygiene 3, 18–32. doi:10.3390/hygiene3010004
Thomassen, G. M. B., Reiche, T., Tennfjord, C. E., and Mehli, L. (2022). Antibiotic resistance properties among Pseudomonas spp. associated with salmon processing environments. Microorganisms 10, 1420. doi:10.3390/microorganisms10071420
Valdimarsson, G., Einarsson, H., Gudbjörnsdottir, B., and Magnusson, H. (1998). Microbiological quality of Icelandic cooked-peeled shrimp (Pandalus borealis). Int. J. Food Microbiol. 45, 157–161. doi:10.1016/S0168-1605(98)00149-4
Wang, L.-L., and Johnson, E. A. (1997). Control of Listeria monocytogenes by monoglycerides in foods. J. Food Prot. 60, 131–138. doi:10.4315/0362-028X-60.2.131
Wang, X. Y., and Xie, J. (2020). Water dynamics and microbial communities of bigeye tuna (Thunnus obesus) during simulated cold chain logistics. J. Food Saf. 40, e12766. doi:10.1111/jfs.12766
Wan Norhana, M. N., Poole, S. E., Deeth, H. C., and Dykes, G. A. (2010). The effects of temperature, chlorine and acids on the survival of Listeria and Salmonella strains associated with uncooked shrimp carapace and cooked shrimp flesh. Food Microbiol. 27, 250–256. doi:10.1016/j.fm.2009.10.008
Weerakkody, N. S., Caffin, N., Dykes, G. A., and Turner, M. S. (2011). Effect of antimicrobial spice and herb extract combinations on Listeria monocytogenes, Staphylococcus aureus, and spoilage microflora growth on cooked ready-to-eat vacuum-packaged shrimp. J. Food Prot. 74, 1119–1125. doi:10.4315/0362-028X.JFP-11-052
Wickham, H. (2016). ggplot2: elegant graphics for data analysis. Available online at: https://ggplot2.tidyverse.org. (Accessed September 4, 2024).
Wiernasz, N., Gigout, F., Cardinal, M., Cornet, J., Rohloff, J., Courcoux, P., et al. (2021). Effect of the manufacturing process on the microbiota, organoleptic properties and volatilome of three salmon-based products. Foods 10, 2517. doi:10.3390/foods10112517
Yin, Q., Zhang, W., Li, X., Zhou, L., Qi, X., Zhang, C., et al. (2019). Contribution of trimethylamine N-oxide on the growth and pressure tolerance of deep-sea bacteria. J. Oceanol. Limnol. 37, 210–222. doi:10.1007/s00343-019-7377-9
Yu, Q., Liu, J., Yang, J., Lou, Y., Li, Y., and Zhang, M. (2023). Postharvest preservation technologies for marine-capture shrimp: a review. Food Bioprocess Technol. 16, 2343–2358. doi:10.1007/s11947-023-03049-6
Zhang, C., Zhang, W. J., Yin, Q., Li, X., Qi, X., and Wu, L. F. (2020). Distinct influence of trimethylamine N-oxide and high hydrostatic pressure on community structure and culturable deep-sea bacteria. J. Oceanol. Limnol. 38, 364–377. doi:10.1007/s00343-019-9076-y
Keywords: spoilage microbiota, spoilage potential, storage trial, total volatile nitrogen (TVN), Pseudoalteromonas, Carnobacterium, food safety, Listeria monocytogenes
Citation: Jensen HA, Skytthe KB, Güde SH, Sparvath JS, Macé S, Bøknæs N, Mejlholm O and Dalgaard P (2025) Frozen and thawed cooked shell-on shrimp (Pandalus borealis) – effect of microbial and chemical changes on sensory and safe shelf-life. Front. Food Sci. Technol. 5:1572265. doi: 10.3389/frfst.2025.1572265
Received: 06 February 2025; Accepted: 21 March 2025;
Published: 22 April 2025.
Edited by:
Aris E. Giannakas, University of Patras, GreeceReviewed by:
Dimitrios A. Anagnostopoulos, University of Thessaly, GreeceYénoukounmè Euloge Kpoclou, Université Nationale d'Agriculture, R. Benin, Benin
Laura Leilén Cocito, CONICET Centro Austral de Investigaciones Científicas (CADIC), Argentina
Copyright © 2025 Jensen, Skytthe, Güde, Sparvath, Macé, Bøknæs, Mejlholm and Dalgaard. This is an open-access article distributed under the terms of the Creative Commons Attribution License (CC BY). The use, distribution or reproduction in other forums is permitted, provided the original author(s) and the copyright owner(s) are credited and that the original publication in this journal is cited, in accordance with accepted academic practice. No use, distribution or reproduction is permitted which does not comply with these terms.
*Correspondence: Hanne Aarslev Jensen, aGFhakBmb29kLmR0dS5kaw==
†Deceased as of 5th January 2024