- 1Department of Agricultural Sciences, University of Helsinki, Helsinki, Finland
- 2Agriculture Flagship, Commonwealth Scientific and Industrial Research Organisation, Canberra, ACT, Australia
Timothy is a perennial forage grass grown commonly in Boreal regions. This study explored the effect of vernalization and photoperiod (PP) on flowering and growth characteristics and how this related to changes in expression of three flowering related genes in accessions from different geographic origin. Large variation was found in accessions in their vernalization and PP responses. In southern accessions vernalization response or requirement was not observed, the heading date remained unchanged, and plants flowered without vernalization. On the contrary, northern types had obligatory requirement for vernalization and long PP, but the tiller elongation did not require vernalization at 16-h PP. Longer vernalization or PP treatments reduced the genotypical differences in flowering. Moreover, the vernalization saturation progressed stepwise from main tiller to lateral tillers, and this process was more synchronized in southern accessions. The expression of PpVRN1 was associated with vernalization while PpVRN3 accumulated at long PP. A crucial role for PpVRN3 in the transition to flowering was supported as in southern accession the transcript accumulated in non-vernalized plants after transfer to 16-h PP, and the apices transformed to generative stage. Differences in vernalization requirements were associated with variation in expression levels of PpVRN1 and PpVRN3, with higher expression levels in southern type. Most divergent transcript accumulation of PpMADS10 was found under different vernalization conditions. These differences between accessions can be translated into agronomic traits, such as the tiller composition of canopy, which affects the forage yield. The southern types, with minimal vernalization response, have fast re-growth ability and rapidly decreasing nutritive value, whereas northern types grow slowly and have better quality. This information can be utilized in breeding for new cultivars for longer growing seasons at high latitudes.
Introduction
Timothy (Phleum pratense L.) belongs to Poaceae family, which includes some of the world’s most important cereal and pasture grasses (Stewart et al., 2011). It is a perennial cool-season forage grass that is commonly used in grass leys in temperate regions. It is well adapted for cultivation in Boreal climate due to its winter hardiness and adaptation to wide range of PP conditions (e.g., Andrews and Gudleifsson, 1983; Jönsson et al., 1992; Stewart et al., 2011). The level of genetic diversity in timothy accessions of different origin is high (Tanhuanpää and Manninen, 2012; Fjellheim et al., 2015). High levels of genetic variation are also found, within individual accessions, likely due to the wind pollinating outcrossing behavior and hexaploid nature of timothy (Tanhuanpää and Manninen, 2012).
There is considerable variation in flowering behavior amongst different accessions of timothy. Fiil et al. (2011) have showed that heading time (HD) is correlated to geographical origin of timothy. One component of the variation in flowering behavior is differences in vernalization requirement; the need for prolonged winter cold to stimulate flowering (Fiil et al., 2011; Jokela et al., 2014). When tested at natural Danish day length conditions (15–18 h), Fiil et al. (2011) identified considerable variation in vernalization response for timothy accessions of different origins, collected from latitudes between 35° N and 70°N. Vernalization accelerated flowering and development of timothy in all tested cultivars and accessions (Seppänen et al., 2010; Fiil et al., 2011; Jokela et al., 2014). For two Finnish timothy cultivars, ‘Iki’ (originated 63°N) and ‘Tuure’ (bred for cultivation 60–64°N), 10 weeks vernalization was required for proper flowering, although more southern ‘Tuure’ was able to flower also without vernalization in 16-h PP (Jokela et al., 2014). There exists wide genetic diversity in timothy cultivars and accessions for PP sensitivity (Heide, 1982; Junttila, 1985). For this study, PP treatments were selected based on the earlier results, where 12 h was shown to delay flowering (Jokela et al., 2014), and since the critical PP for accelerating flowering of timothy is typically being between 14 and 18 h (Heide, 1982). The length of vernalization was chosen based on previous studies of the effects of 0–15 weeks vernalization on flowering of timothy (Seppänen et al., 2010; Fiil et al., 2011; Jokela et al., 2014).
Timothy canopy is comprised of three different tiller types of different ages (Seppänen et al., 2010; Gustavsson, 2011; Virkajärvi et al., 2012; Supplementary Figure S1). The stem formation has an important role in the spring yield formation, because the vernalized spring growth consists mainly of stem producing GEN and ELONG tillers, which have a true stem with palpable nodes and apex at vegetative stage and high from soil surface (Virkajärvi et al., 2012). The second and third yields are composed of more vegetative tillers and leaves, and then leaf characters have larger importance (Virkajärvi et al., 2012). The stem producing GEN tillers are heaviest and thus, can contribute more to the silage yield than ELONG or VEG tillers (Virkajärvi et al., 2012). In forage grasses flowering was thought to be strictly associated with rapid decrease of stem digestibility. Our earlier study showed, however, that the lignified sclerenchyma ring forms similarly in time to all stem forming tillers irrespective of the developmental stage of apex (Seppänen et al., 2010). Thus, the ideotypic timothy canopy would consist of heavy stem forming tillers where the lignified sclerenchyma ring has not yet fully developed. In addition, an ideotypic timothy genotype would produce stem forming tillers rapidly without vernalization throughout the growing season. Doing so, the critical LAI could be high in all harvests and thus, the biomass accumulation would reach its maximum.
In winter cereals the saturation of vernalization requirement can be measured as FLN (Berry et al., 1980). Mahfoozi et al. (2001) noticed that in wheat plants where vernalization requirement was not fulfilled, the apex remained longer at vegetative stage producing new leaves instead of generative structures, which was seen as higher FLN. When vernalization requirement is saturated, the number of leaves will remain at constant level even when vernalization time is extended (Mahfoozi et al., 2001). In forage grasses the connection of FLN and vernalization saturation has not completely understood. Understanding the relationship between FLN and vernalization would bring important information on the development of the growth characteristics of different tiller types.
Variation in vernalization and PP requirements is important for breeding timothy cultivars with flowering behaviors suited to different geographical regions. How this variation can impact on other aspects of agronomic performance, e.g., tiller composition and yield formation under different PP or vernalization conditions has not yet been resolved. In timothy breeding programs, genetic variation between accessions of different origin has traditionally been utilized to combine desired traits (Tanhuanpää and Manninen, 2012). Southern accessions tend to grow faster after cutting (Larsen and Marum, 2006), whereas northern accessions have better winter hardiness (Jørgensen et al., 2010). Rapid stem growth is associated with development of lignified sclerenchyma ring and decrease in the digestibility of the stem (Seppänen et al., 2010). Therefore, the use of southern accessions in breeding programs accelerates growth, but can also decrease the nutritive value of the harvested forage yield.
The simplified model of molecular flowering-time regulation by vernalization and PP in monocots has been reviewed by several authors (e.g., Distelfeld et al., 2009; Kamran et al., 2014; Fjellheim et al., 2014). VERNALIZATION1 (VRN1) is a major vernalization response gene in several studied monocots (e.g., Hemming et al., 2008; Sasani et al., 2009) and similar to APETALA1(AP1) in Arabidopsis (Kaufmann et al., 2010), whereas VRN3 is an integrator of the vernalization and PP pathways, and a strong flowering promoter (Yan et al., 2006), and similar to FLOWERING LOCUS T (FT) in Arabidopsis (Kardailsky et al., 1999). In cereals flowering is repressed by VRN2 (Hemming et al., 2008), and in grasses (e.g., Lolium perenne) by LpMADS10 homologs (Ciannamea et al., 2006) under SD conditions. Seppänen et al. (2010) showed that vernalization genes, especially VRN1, are regulated similarly in timothy as in cereals, and the apex transition from vegetative to reproductive stage is connected to the expression peak of VRN1 also in timothy. PpVRN1, PpVRN3, and PpMADS10 genes were also identified and the expression patterns were shown to be similar to equivalent genes in other Poaceae species (Jokela et al., 2014).
Ecotypic variation in vernalization requirement of flowering has been reported in many species including Arabidopsis thaliana (Grillo et al., 2013), A. lyrata (Quilot-Turion et al., 2013), barley (Loscos et al., 2014), Brachypodium distachyon (Ream et al., 2014), Poa sp. (Heide, 2001), and wheat (Iwaki et al., 2001). Mutations, insertions or deletions in the promoter of first intron of VRN1 may result rapid flowering without vernalization (Yan et al., 2004; Fu et al., 2005), and generally for spring growth habit. Also variations in VRN3 due to copy number or structural rearrangements can have effect on the flowering time of cereals (Yan et al., 2006; Chen et al., 2013). At epigenetic level winter types are more methylated compared to spring types (Sherman and Talbert, 2002). The structural changes in FRIGIDA (FRI; Johanson et al., 2000) and FLOWERING LOCUS C (FLC; Grillo et al., 2013), provide an example of genetic variation underlying the ecotypical differences in Arabidopsis, whereas in cereals epigenetic changes in VRN1 (Oliver et al., 2009) and in FT/VRN3 (Diallo et al., 2012) explain differences. To date it has not been able to show any clear epigenetic changes in cereal’s flowering repressor TaVRN2 (Diallo et al., 2012).
The objective of this study was to investigate how timothy accessions from different geographic origin respond to vernalization and PP treatments relative to growth characteristics, and how these differences can be translated into agronomic parameters. These differences were characterized at molecular level by studying the expression of major vernalization genes in relation to release of stem growth and flowering.
Materials and Methods
Plant Material and Experimental Conditions
Fiil et al. (2011) tested 38 timothy accessions for vernalization responses, and three of those accessions with strong (NGB14403, NGB 17198, 51998), one with intermediate (6116) and two with weak vernalization response (NGB4053, PI204909) were selected for this study (Table 1). In addition, two breeding lines of northern (BOR N) and southern (BOR S) origin and cultivars characterized previously for their agronomic performance in Boreal climate (Grindstad, Donatello, BOR1) were included.
Timothy accessions were tested for vernalization and PP responses in two separate experiments (Table 1). The propagation of the plant material was timed so that vernalization treatments finished at the same time, and plants with different treatments were transferred to greenhouse together. In the first experiment plants were propagated on 14.10.2010, 4.11.2010, 18.11.2010, 6.1.2011, and 20.1.2011, and transferred to greenhouse on 17.2.2011. In the second experiment plants were propagated on 17.11.2011, 21.12.2011, and 21.2.2012, and transferred to greenhouse on 21.3.2012. Clonal material was propagated using vegetative plants (stages 22–28; Gustavsson, 2011) and grown for 4 weeks in SD conditions (20/15°C day/night, light intensity was between 200 and 500 μmol m-2s-1, 12 h day length) in fertilized and limed peat (Kekkilä B2, Finland).
Clonally propagated plants having one to two leaves (and a single vegetative apex) were transferred to growth chamber (Weiss Technik, Germany) and vernalized at 6/4°C (day/night) and 8-h PP for 0, 2, 10, 12, or 15 weeks (Experiment 1) and 0, 10 or 15 weeks (Experiment 2). In both experiments, non-vernalized plants were grown in SD conditions (20/15°C day/night, light intensity was between 200 and 500 μmol m-2s-1, 12-h PP) for 4 weeks, and transferred to the LD greenhouse simultaneously with all vernalized plants. Thus, there was difference in the PP conditions for the control and vernalized plants, but based on earlier studies we assume that this should not have any effect on the results, because the PP after vernalization should have more important effect. In growth chamber, pots with three individual plants were arranged in a completely randomized design with three replicates, and rotated once a week in order to minimize the effects of possible environmental variation within the growth chamber. After vernalization plants were transferred at the same time with non-vernalized plants to greenhouse (20°C/15°C day/night, light intensity was between 200 and 500 μmol m-2s-1), to 16 h PP (Experiment 1), and to 12, 16, or 20 h PP (Experiment 2) conditions.
Flowering was expressed as HD which was recorded for individual plants separately when the first spikelets had emerged. Plants which did not produce flowering tillers at all, a set value of 200 days was given for the calculations. The height of the three individual main tillers in three (Experiment 1) or two (Experiment 2) replications were measured after 3 weeks’ time in greenhouse conditions. FLN, the type of main tiller, and the number and type of lateral tillers were calculated at the end of the experiments, ca. 100 days in greenhouse conditions. Tiller types (VEG, ELONG, GEN) were defined as described in Jokela et al. (2014; Supplementary Figure S1).
Leaf material for RNA samples were collected 0, 7, and 28 days after the transfer to greenhouse (Experiment 1), or 0 and 14 days after the transfer (Experiment 2). The developmental stages of the apical meristem of three individual plants were determined at RNA sampling time. The scale of apical meristem by Sweet et al. (1991) classifies apices as vegetative (stages 1–2), with double ridge stages (stages 3–4), and as generative (stages 5–11). The sampling and determination of apices was conducted as removing the plant tissue (leaves and part of the stem) around the apex from three individual plants. The developmental stage was observed using light microscope.
Gene Expression Analysis
Total RNA was extracted from timothy leaves from both experiments using Pine Tree Method (Chang et al., 1993) and diluted in 12 μl of DEPC- water. RNA was cleaned up using NucleoSpin XS (Macherey-Nagel, Düren, Germany) kit according to manufacturer’s instructions and diluted in 10 μl of DEPC- water. cDNA synthesis with DNase treatment was performed using the SuperScript III (Invitrogen, Karlsruhe, Germany) one-step cDNA kit, where 1 μg of total RNA was used per reaction, according to the manufacturer’s instructions.
Quantitative real time PCR analysis was conducted in a Roche Light Cycler 480 (Roche Applied Science, Basel, Switzerland) in a 96-well plate one reaction containing 0.5 μM forward and reverse primer (actin, PpVRN1, PpVRN3, PpMAS10; Supplementary Table S1), 10 μl SYBR Green master mix (Roche, Basel, Switzerland), 3 μl water and 5 μl diluted cDNA. The PCR program was: an initial denaturation step at 95°C for 10 min, following 40 cycles of 10 s at 95°C, 20 s at 59°C and 30 s at 72°C. Expression levels were calculated by the 2-ΔΔCt method (Livak and Schmittgen, 2001) actin for normalization of the data, and results were reported as a fold change relative to a calibrator sample (non-vernalized BOR S and BOR N samples). The efficiency for the primers was calculated using a decreasing series of cDNA concentrations, and all primer efficiencies were close to 2.
Statistical Analyses
Analysis of variance (ANOVA) was performed using PASW 18 software (SPSS Inc., Chicago, IL, USA; replicates as random effects, and environmental and accessions, and their interaction were as fixed effects), and multiple comparisons of means were performed with Tukey’s test.
Results
Genetic Variation of Vernalization Response
Wide variation in flowering time was found among the tested 11 accessions (p < 0.001; Figure 1). The most southern and northern accessions could be identified based on their divergent response to vernalization (Figures 1 and 2). Southern accessions (BOR S, Saltum) did not show vernalization response or requirement as the HD remained unchanged (HD ~40 days) irrespective of vernalization treatments. In comparison, Northern accessions (BOR N, Karasjok, Närekumpu) required 2–10 weeks vernalization for flowering. Moreover, HD occurred earlier in two northern accessions (Närekumpu, Karasjok) as the vernalization time got longer (Figure 1). The acceleration of heading was accession dependent being strongest in Närekumpu (from 200 to 40 days after 10 weeks) and slowest in BOR N (from 200 to 148 days in 12 weeks; Figure 1). After 15 weeks of vernalization the genotypic differences in HD were diminished (HD ca. 35 days) with exception of BOR N for which no flowering tillers were observed when plants were vernalized for 15 weeks and then grown in 16-h PP (Figure 1). The rest of the studied accessions were classified as intermediates as the HD decreased in response to vernalization (from ca. 75–40; Figure 1). These accessions, however, did not have an absolute vernalization requirement at this PP similarly to northern accessions and flowering tillers were eventually produced in non-vernalized plants within 60–90 days. The ANOVA showed significant differences between vernalization treatments, accessions and the interaction of these in HD (for all p < 0.001, Table 2).
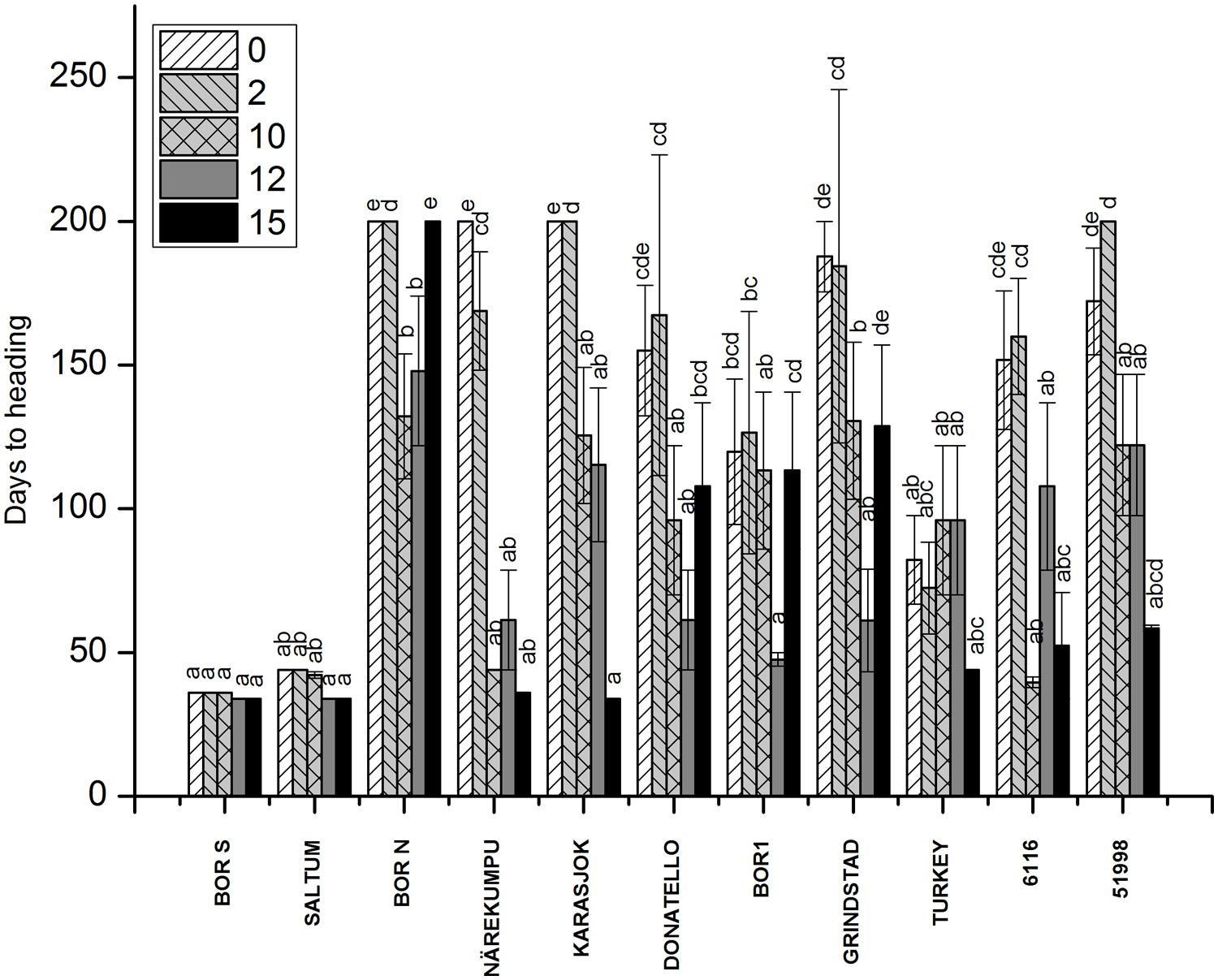
FIGURE 1. The effect of vernalization on HD in timothy accessions (n = 9). Plants were vernalized for 2, 10, 12, or 15 weeks in growth chamber [at 6/4°C (day/night), 8 h DL, control plants in 20/15°C (day/night), 12 h DL] and grown in 16-h DL in greenhouse for over 3 months. Some of the individual plants did not flower at all, and the HD was set for 200 for those plants. Data shows mean of three replicates of three plants ± SE. Distinct letters above bars indicate the significant differences in one vernalization treatment between accessions calculated by Tukey’s test.
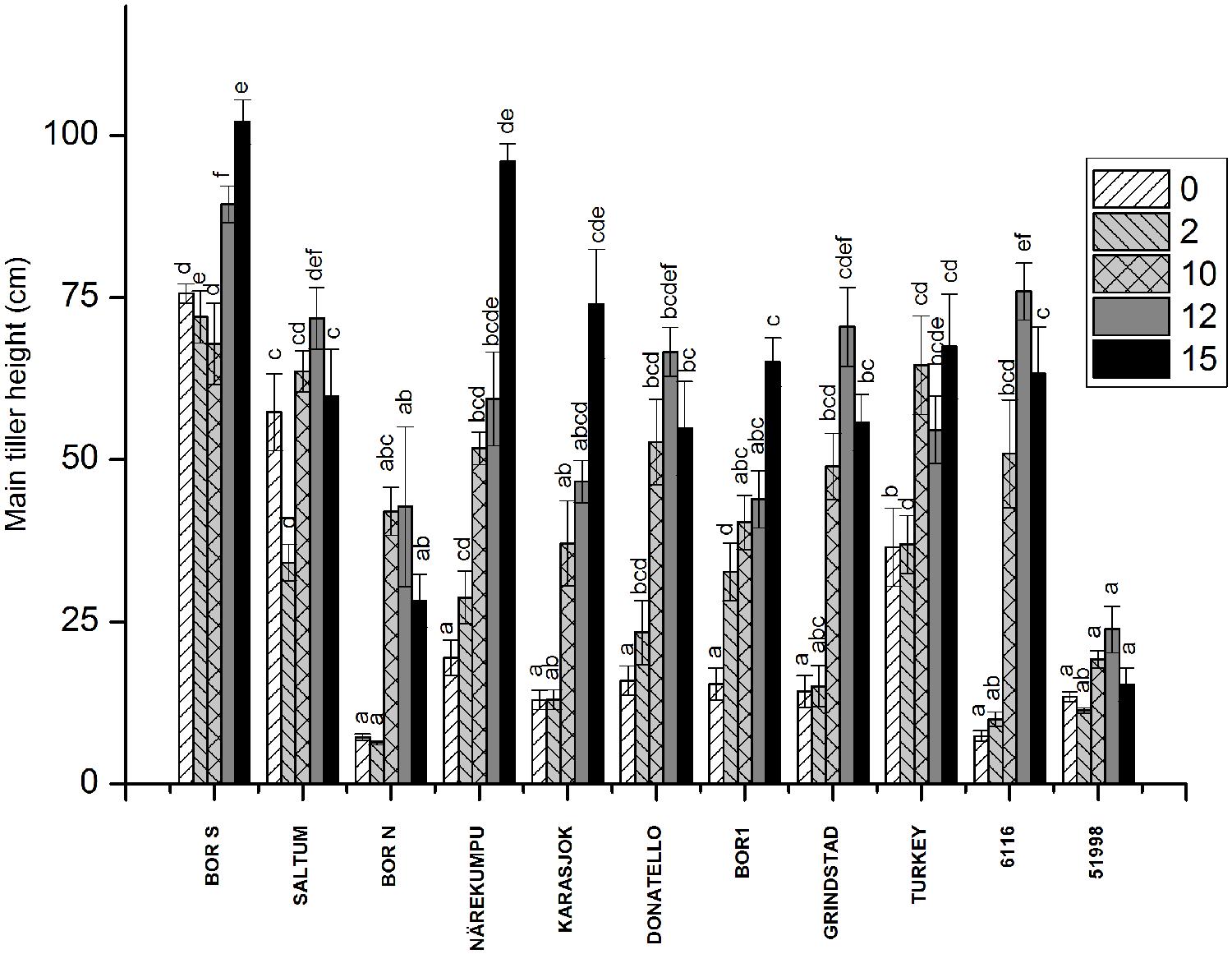
FIGURE 2. The effect of vernalization on height of the main tiller in timothy accessions (n = 9). Plants were vernalized for 2, 10, 12, or 15 weeks in growth chamber [at 6/4°C (day/night), 8 h DL, control plants in 20/15°C (day/night), 12 h DL] and grown in 16-h DL in greenhouse, and the height of the main tiller was measured after 3 weeks growth. Data shows mean of three replicates of three plants ± SE. Distinct letters above bars indicate the significant differences in one vernalization treatment between accessions calculated by Tukey’s test.
The fulfillment of vernalization requirement was not clearly connected to FLN in timothy (FLN; Supplementary Figure S2). A relationship between the duration of vegetative stage of apices and FLN of the main tiller was not as clear, for instance BOR N remained vegetative and the FLN was also low (Supplementary Figure S2). Decreased leaf number was detected only in Närekumpu indicating that faster transition of apices in main tiller from vegetative to reproductive development resulted in fewer leaf primordia and thus less leaves (Supplementary Figure S2). In all other studied accessions, the number of developing leaves per tiller remained quite constant throughout vernalization treatments (Supplementary Figure S2). The ANOVA showed significant differences between the used vernalization treatments (p < 0.001), but the average of FLN in all studied accessions after 15 weeks vernalization was only 1, 5 leaves lower than in 0 weeks vernalized plants (Supplementary Figure S2).
Interaction of Vernalization and Accession on Growth Characteristics
The release of stem growth was monitored by measuring the height of the individual plants 3 weeks after vernalization treatments (Figure 2). Rapid development and stem elongation was measured in the non-vernalized plants of southern accessions (BOR S, Saltum), in contrast to the majority of the northern accessions, which required vernalization for the initiation of stem elongation. Geographic origin did not, however, determine the response of height growth to vernalization although in two northern accessions (Närekumpu and Karasjok) the growth response to vernalization was notable (Figure 2). For example, accession 6116 originating from Italy required 10-weeks of vernalization for the initiation of rapid stem growth (Figure 2). On the contrary, in accession 51998 (England) stem elongation was arrested and was not affected by vernalization. Weakest response to height growth was seen in BOR N, which may be due to the restricting 16-h PP used in this experiment.
Timothy canopy consists of three tiller types and the proportions of these types vary depending on the physiological stage of the plant. Northern accessions were able to produce more tillers during vernalization, whereas southern accessions produced more tillers after vernalization (data not shown). At the end of the experiment each main tiller was categorized as VEG, ELONG, or GEN tillers. In BOR S all main tillers developed into GEN tillers without vernalization (Table 3A). Similarly, in Saltum and Turkey most of the main tillers in non-vernalized plants were generative (89%) whereas the northern accessions (BOR N, Närekumpu, Karasjok) were unable to produce GENs without vernalization in 16-h PP (Table 3A). In general, prolonged vernalization increased the proportion of GEN and ELONG main tillers so that after 15-weeks of vernalization none of the main tillers had remained VEG, expect in BOR N, where VEG tillers were present (Table 3A). The proportions of the main tiller type were significantly different between accessions and vernalization treatments (for both p < 0.001). The number of lateral tillers differed significantly between the studied accessions (p < 0.001, Table 3B). There were no interactions between vernalization time or observed vernalization response and total tiller number (p = 0.165). Most of the lateral tillers were VEG among accessions and vernalization treatments (Table 3B). In general, the number of GEN lateral tillers increased as the vernalization time prolonged (Table 3B). BOR S and Turkey produced significant proportion of GEN lateral tillers also in non-vernalized plants, whereas few flowering lateral tillers were observed in the rest of the southern and intermediate accessions (Table 3B). All three northern accessions (BOR N, Närekumpu, Karasjok) required 10 weeks vernalization for the development of GEN lateral tillers. The ANOVA showed significant differences between accessions in the number of VEG, ELONG, and GEN tillers (in all cases p < 0.001) and vernalization treatments (p = 0.005, p = 0.001, p < 0.001 respectively).
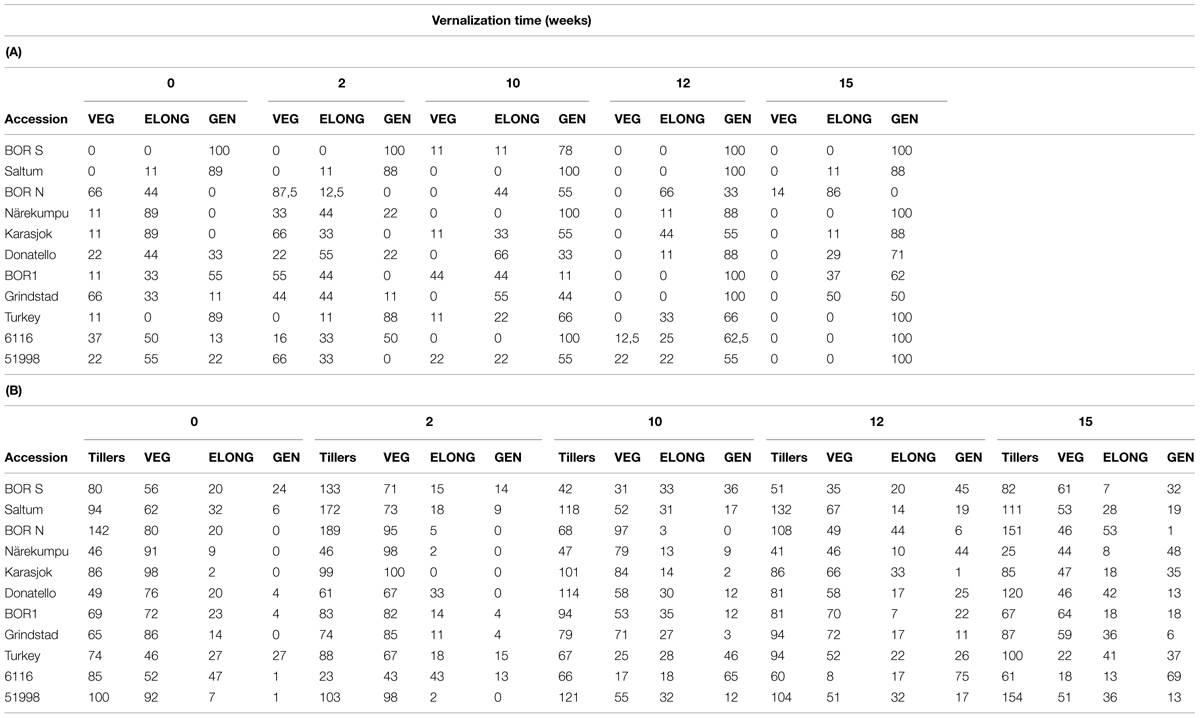
TABLE 3. (A) The percentage of the main tiller type (VEG, ELONG, and GEN) n = 9, and (B) the total number of side tillers and percentage of the side tiller type (VEG, ELONG, and GEN) in 11 timothy accessions (n = 9) after 0–15 weeks vernalization and 100 days (no change visible anymore) in greenhouse.
Interaction between Accession, Photoperiod and Vernalization on Flowering and Growth Characteristics
The interaction between vernalization time and PP on flowering and growth characteristics was studied in four selected accessions having either strong, weak, or intermediate vernalization response (Table 1). At 12-h PP none of the tested plants produced flowering tillers, but flowering did occur in longer PPs (Figure 3). 20-h PP accelerated heading of all tested accessions by ca. 5 days compared to 16 h PP (Figure 3). In BOR N the HD shortened from 175 to 34 days at 20-h PP in non-vernalized plants (Figure 3) indicating that vernalization requirement was not obligatory at long enough DL. In fact, the genotypic differences in vernalization response were diminished at 20-h PP, but they existed at 16-h PP (Figures 3B,C). In BOR S and Grindstad neither vernalization nor change in PP from 16 to 20-h affected flowering time (Figures 3B,C). ANOVA showed significant differences between tested accessions, vernalization and PP treatments in HD (p = 0.004, p = 0.001, and p < 0.001, respectively, Table 2).
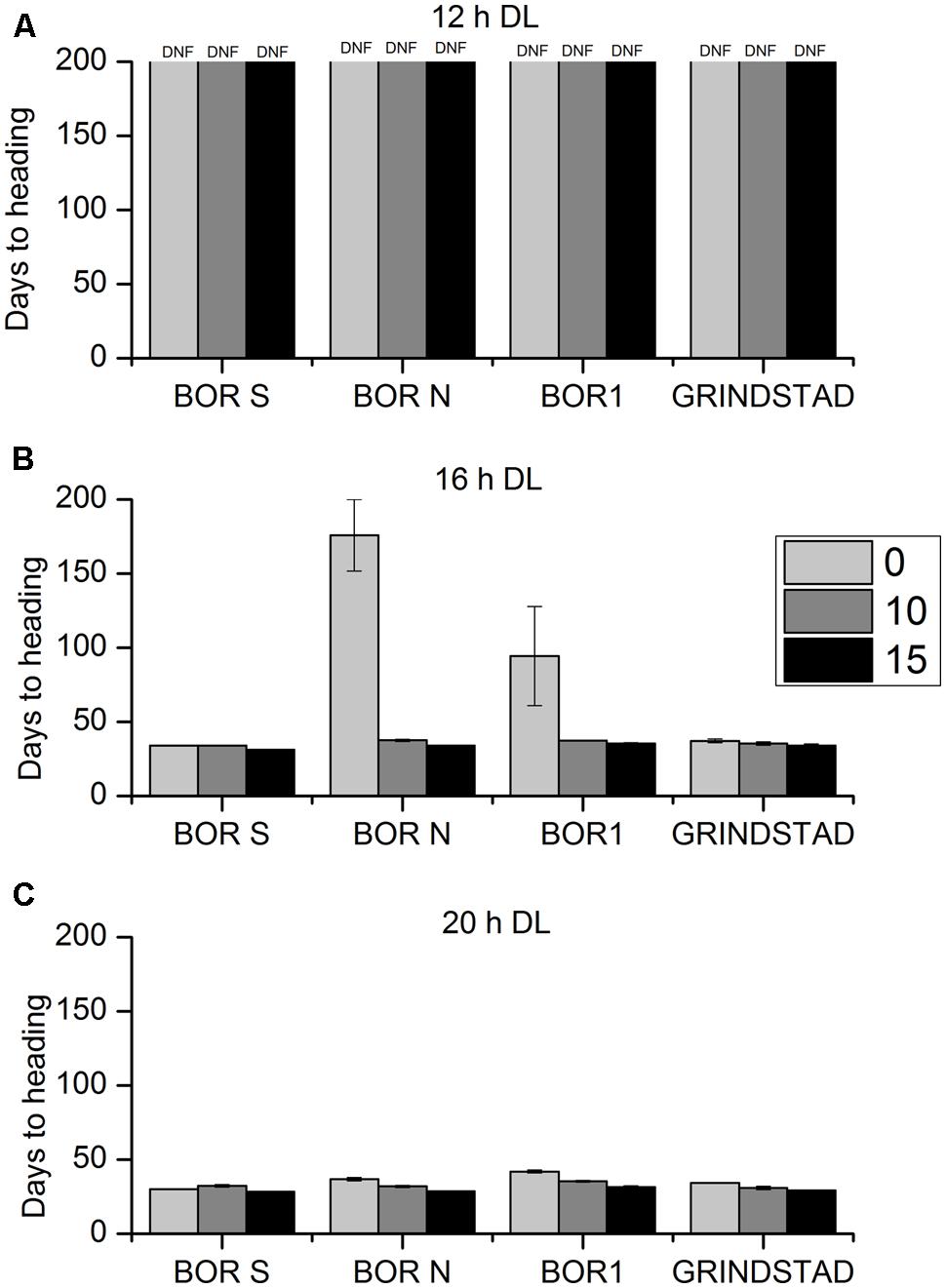
FIGURE 3. The effect of different vernalization and PPs on heading time (HD) in four timothy accessions (n = 6). Plants were vernalized 0 (light gray), 10 (dark gray), or 15 (black) weeks in growth chamber [at 6/4°C (day/night), 8 h DL; control plants in 20°C/15°C (day/night), 12 h DL] and transferred to (A) 12-h, (B) 16-h DL or (C) 20-h DL in greenhouse. Some of the individual plants did not head at all, and the HD was set 200 for those plants. Data shows mean of two replicates of three plants ± SE. DNF, did not flower.
There were clear differences in height growth between accessions, vernalization and PP treatments (Figure 4, p = 0.006, p = 0.016, p = 0.001, respectively). Short day (12-h) arrested height growth in all studied accessions (Figure 4A). Modest growth at 12-h PP was, however, detected in BOR1 after long vernalization treatment (Figure 4A). In 16 h day length BOR N had strongest respond to PP, which was also seen in Experiment 1 (Figures 2 and 4B). Twenty hours PP was long enough to activate height growth also in non-vernalized BOR N, and the different vernalization treatments did not affect height in this PP (Figure 4C).
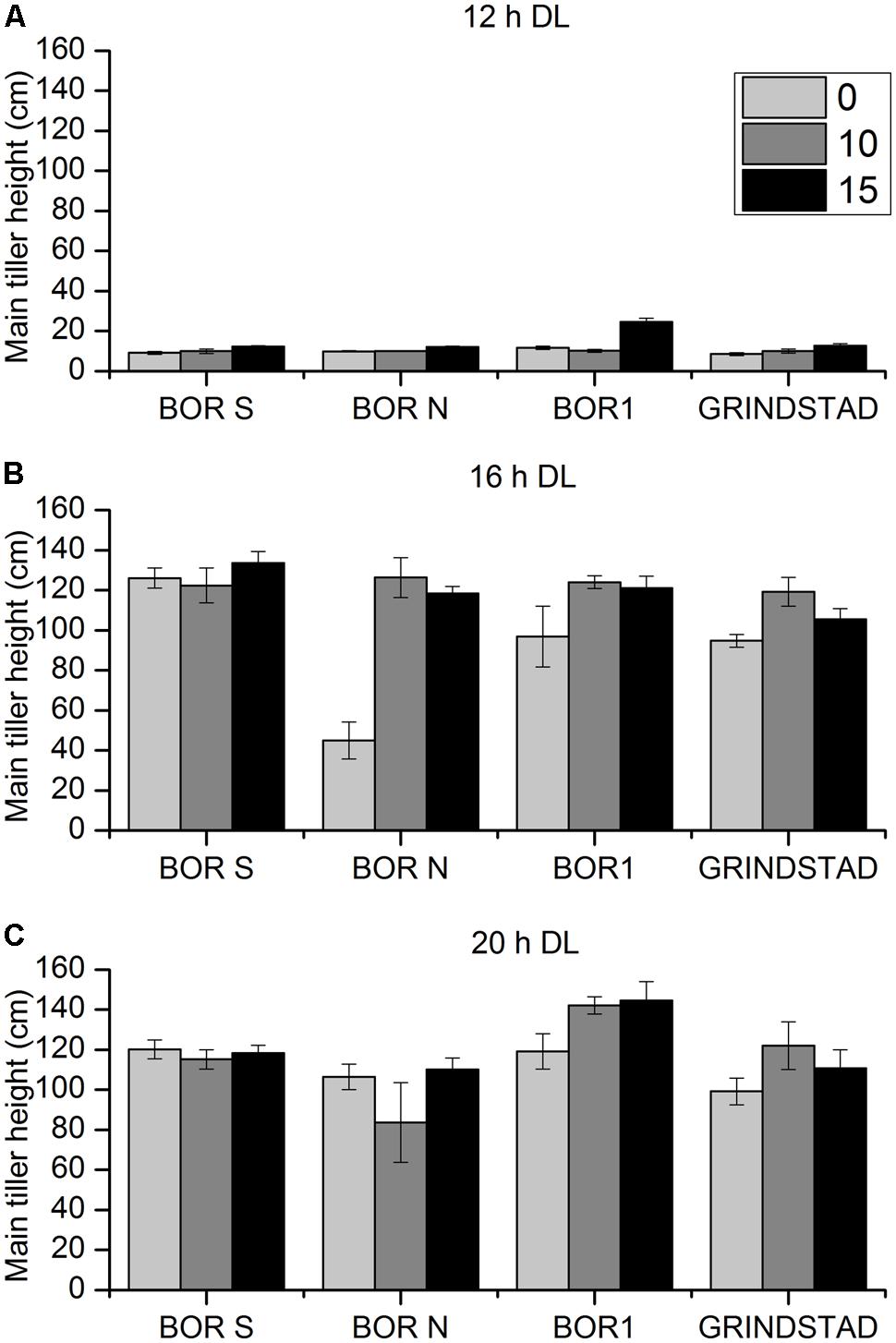
FIGURE 4. The effect of different vernalization and PPs on main tiller height in four timothy accessions (n = 6). Plants were vernalized 0 (light gray), 10 (dark gray), or 15 (black) weeks in growth chamber [at 6/4°C (day/night), 8 h DL; control plants in 20°C/15°C (day/night), 12 h DL] and transferred to (A) 12-h, (B) 16-h DL or (C) 20-h DL in greenhouse. Data shows mean of two replicates of three plants ± SE.
In 12 h PP only 15 weeks vernalized BOR1 was able to produce ELONG tillers (Table 4A). In 16-h PP only BOR N required vernalization for producing GEN tillers, whereas other accessions flowered without vernalization. However, BOR N was able to produce ELONG tillers without vernalization (Table 4A). In longer PPs development of tillers was shifted from VEG to GEN tillers in all accessions (Table 4A). The number of lateral tillers was highest in 12-h PP (Table 4B). All lateral tillers in 12-h PP were VEG, except BOR1 was able to produce few ELONG tillers after 15 weeks vernalization (Table 4B). The shift from VEG and ELONG tillers to GEN tillers was seen in most of the lateral tillers after all treatments in longer PP (Table 4B). Similar to Experiment 1, FLN did not correlate with the phenological development or vernalization response in timothy (Supplementary Figure S3).
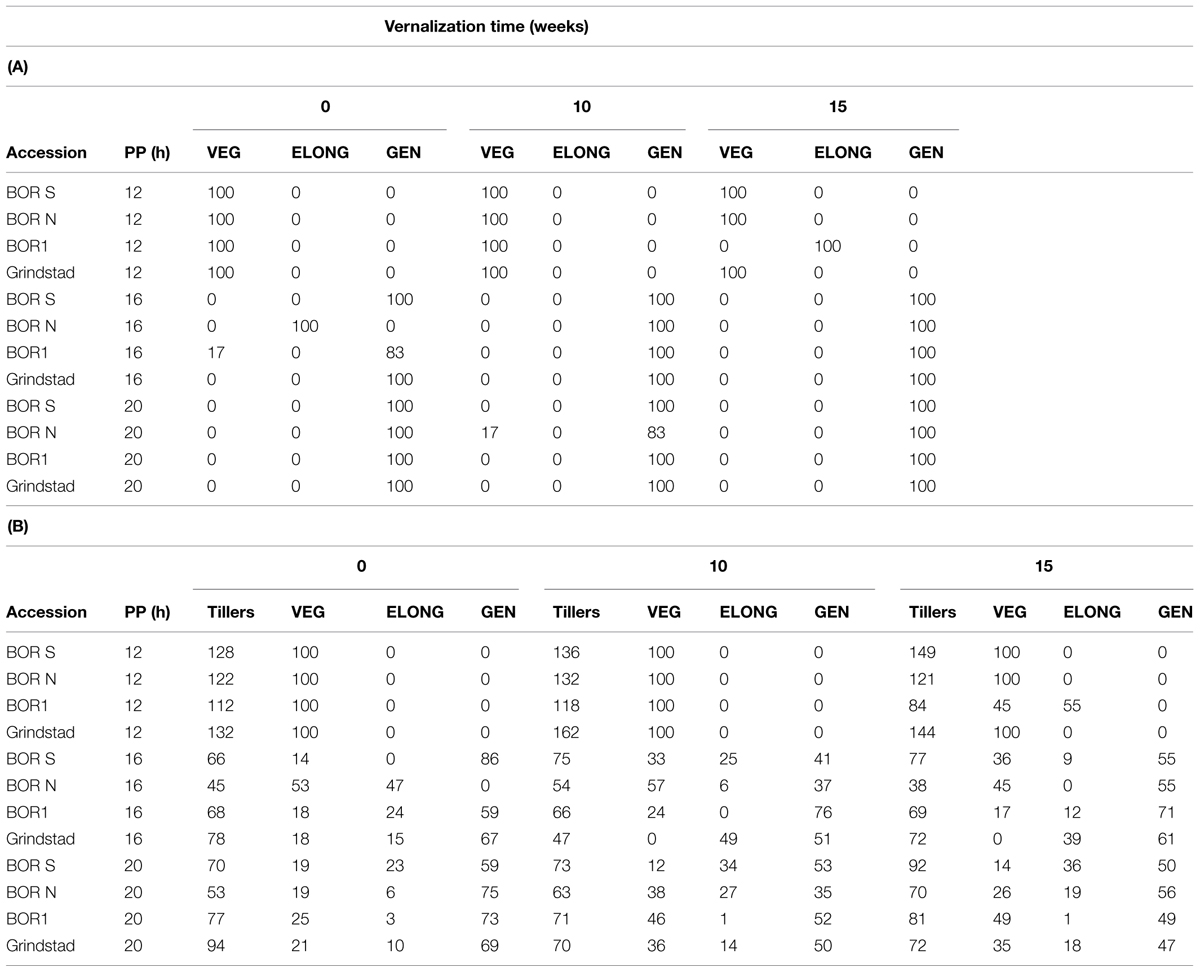
TABLE 4. (A) The percentage of the main tiller type (VEG, ELONG, and GEN; n = 6), and (B) the total number of side tillers and percentage of the side tiller type in four timothy accessions (n = 6) after 0, 10, or 15 weeks vernalization and 100 days in greenhouse.
Expression of Vernalization Genes after Vernalization and PP Treatments in Southern and Northern Accessions and Connection to Apex Development
The behavior of genes that regulate vernalization and PP flowering responses was studied in the most divergent northern (BOR N) and southern accessions (BOR S). The ability of southern accession to initiate stem elongation and produce flowering tillers without vernalization was associated with more rapid apex development and more intense transcript accumulation of PpVRN1 and PpVRN3 (Figure 5). After transfer from short day (12 h) to long day (16 h), apices of BOR S developed within two weeks from vegetative (apex 1) to generative stage (apex 6–10; Figure 5). This response occurred independently of vernalization treatment. The transition to generative growth in BOR S was accompanied by elevated transcript accumulation of PpVRN1 (0 vern +4; Figure 5A) and PpVRN3 (0 vern +2; Figure 5C). Reduced transcript levels for PpMADS10 were detected after short vernalization (2 vern +2) and it occurred simultaneously with rapid development of apices (Figure 5E). In BOR S PpVRN3 accumulation was detected in non-vernalized plants after transfer to 16-h PP and the level was slightly higher after 10–15 weeks vernalization (Figure 5D). PpVRN1 transcript accumulation was responsive to vernalization (p < 0.001), with higher levels detected after 10–15 weeks vernalization versus after 0 or 2-weeks vernalization in both accessions (Figures 5A,B). In BOR N, development of apices was accelerated after 10-weeks of vernalization simultaneously with elevated transcript accumulation of PpVRN1 (Figure 5B). However, PpVRN1 and PpVRN3 transcript accumulation was clearly less intensive in BOR N than in BOR S (Figures 5A–D), which was also shown by the ANOVA (p = 0.019 and p < 0.001, respectively). The accumulation of PpMADS10 in BOR S was decreased after growth in greenhouse, but contradictory in BOR N this trend was not seen (Figures 5E,F).
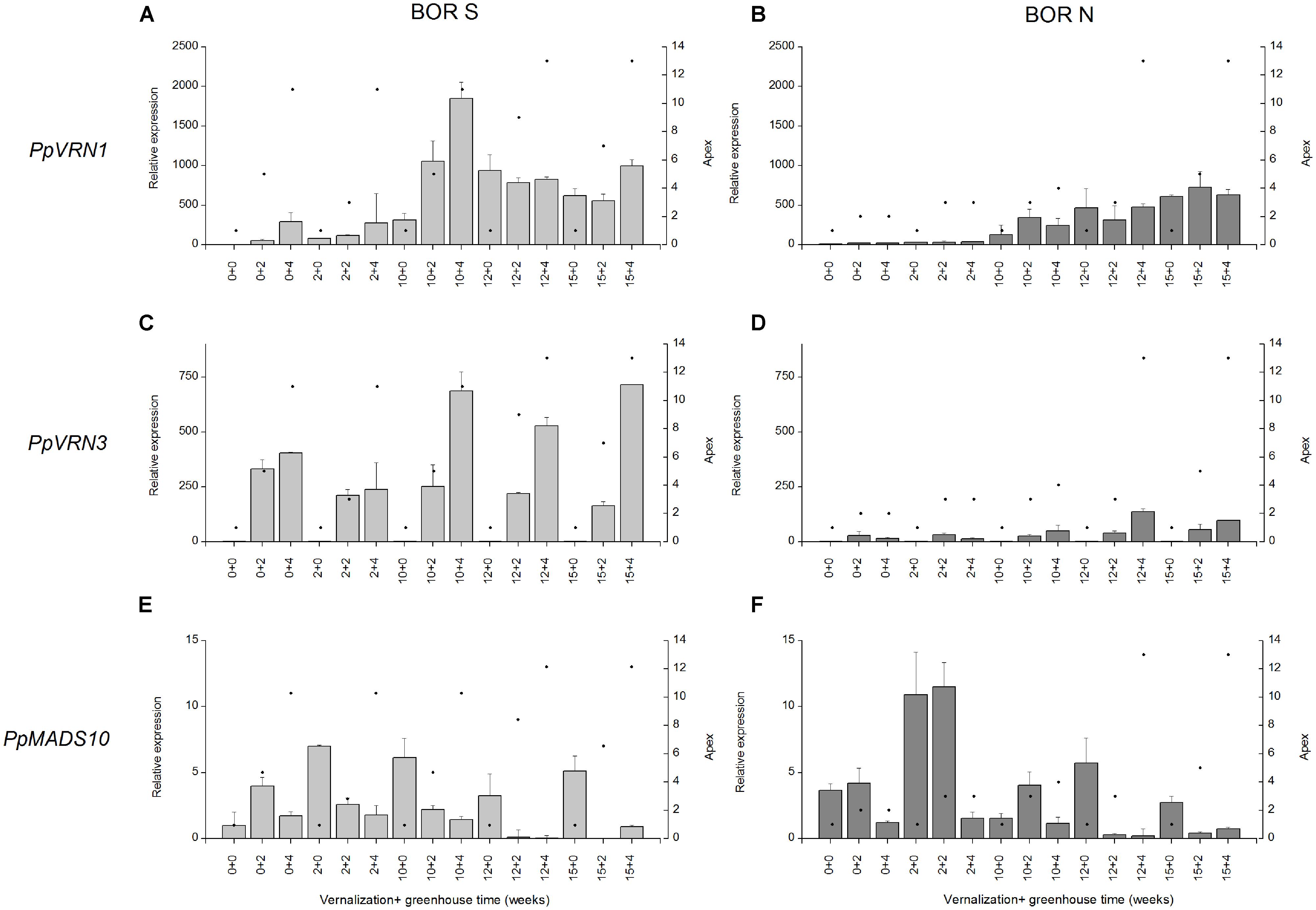
FIGURE 5. (A,B) PpVRN1, (C,D) PpVRN3, and (E,F) PpMADS10 transcript levels in accessions BOR S and BOR N after 0–15 weeks vernalization [at 6/4°C (day/night), 8 h DL; control plants in 20/15°C (day/night), 12 h DL], and 0–4 weeks in a greenhouse (16 h DL). The expression levels are shown as the averages of three biological replicates ± SE. Relative expression is expressed as fold difference relative to transcript level of non-vernalized plants. Black spots on the right side y-axis describe the apex developmental stage in scale of Sweet et al. (1991).
We then examined gene expression after 2 weeks growth in different PPs following 0, 10, or 15 weeks vernalization (Figure 6). There was a correlation between elevated PpVRN1 and PpVRN3 expression levels and accession by treatment combinations that resulted in rapid flowering. However, there was higher PpVRN1 accumulation in BOR S plants vernalized for 15 weeks and grown in 12-h PP without flowering. In BOR S PpVRN1 transcript accumulated extensively during all vernalization treatments and the levels were lower when growing at 12-h (Figure 6A), whereas in BOR N decreased levels of PpVRN1 transcript accumulated (Figure 6B). Increasing PpVRN1 did not result in transcript accumulation of PpVRN3 or development of GEN tillers at 12-h PP (Table 4, Figures 6C,D), whereas in 16-h and 20-h PP that occurred. Genotypic differences that were observed in apices development as well as in height growth (Figure 4) and tiller types (Table 4) at 16-h were associate with divergent transcript accumulation pattern of PpMADS10 (p < 0.001; Figures 6E,F). In 16-h PP divergent accumulation of PpMADS10 was seen in studied accessions; in BOR S the expression level was only high in non-vernalized plants, whereas in BOR N 15 weeks of vernalization was required for the low level (Figures 6E,F). At 20-h PP PpMADS10 was at low level and genotypic differences were no longer visible (Figures 6E,F). At 20-h PP PpMADS10 accumulation and the development of apices did not correlate as low levels of PpMADS10 was detected although apices were at stage 8 in BOR S and 3 in BOR N (Figures 6E,F). Arrested apices development was associated with high levels of PpMADS10 in both accessions (Figures 6E,F).
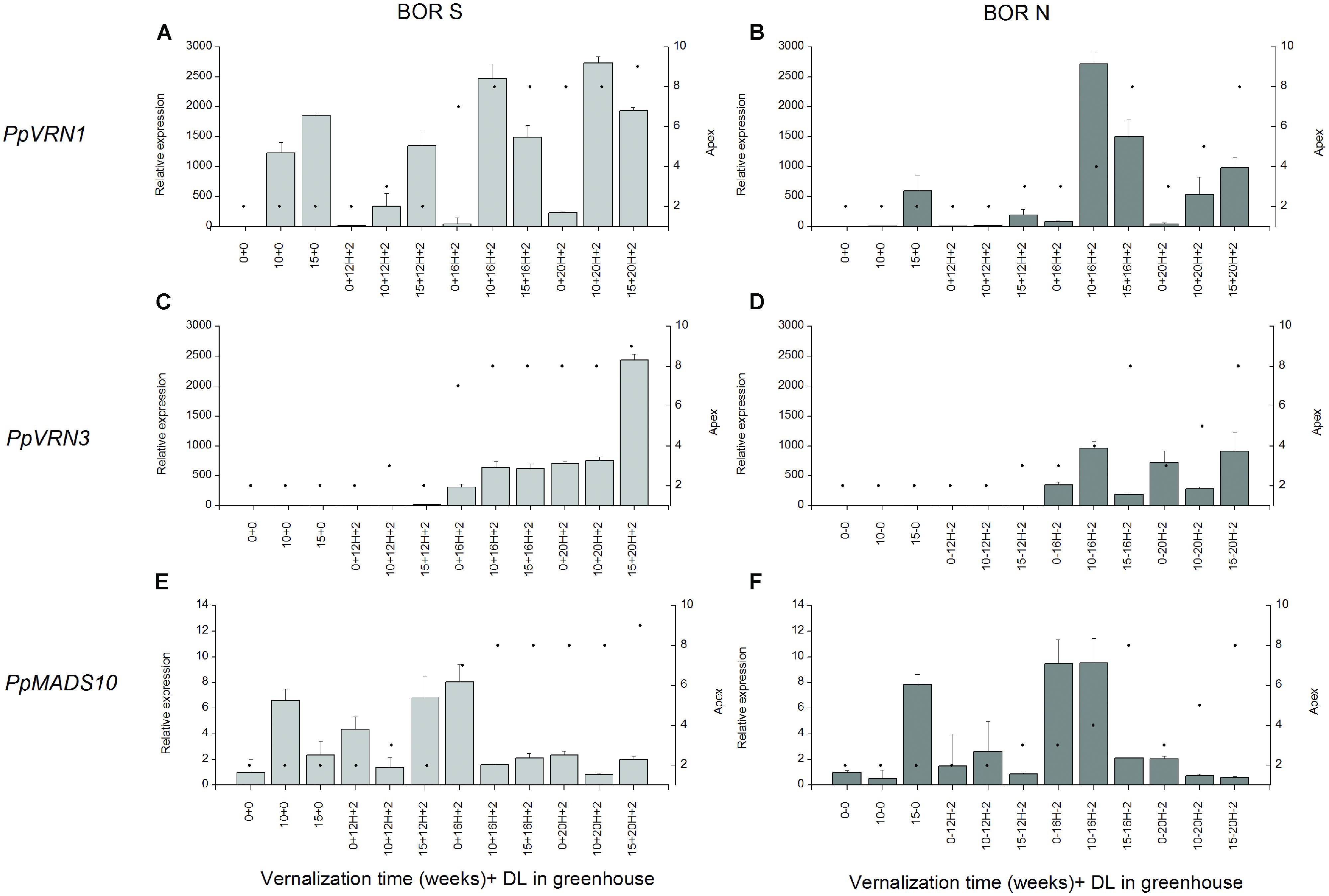
FIGURE 6. (A,B) PpVRN1, (C,D) PpVRN3, (E,F) PpMADS10 transcript levels in accessions BOR S and BOR N after 0, 10, or 15 weeks vernalization [at 6/4°C (day/night), 8 h DL; control plants in 20°C/15°C (day/night), 12 h DL], and 0 or 2 weeks in a greenhouse (in 12, 16, or 20-h DL). The expression levels are shown as the averages of three biological replicates ± SE. Relative expression is expressed as fold difference relative to transcript level of non-vernalized plants. Black spots on the right side y-axis describe the apex developmental stage in scale of Sweet et al. (1991).
Discussion
Climate change will dramatically affect forage production in the future. Adaptation to extended growing period and elevated temperatures in Northern hemisphere will require new cultivars, where the responses to PP and vernalization have been optimized for yield formation in novel growing conditions. Timothy ecotypes of different origin provide interesting genetic variation in their PP and vernalization responses that could be utilized in the future for breeding. In shorter PP the southern accessions with shorter critical PP and lack of vernalization response can produce higher dry matter (DM) yields as the tiller height growth, stem elongation or flowering are not restricted by environmental cues. In this study, vernalization was observed to progress stepwise so that first the main tillers were saturated and later the lateral tillers. Moreover, the development of GEN tillers in main and lateral tillers was more synchronized in southern accessions resulting in higher proportion of GEN tillers in canopy. On the contrary, in accessions 51998 (England) and 6116 (Italy) stem elongation was arrested and was not affected by vernalization. These plants remained short indicating probably adaptation for grazing. In silage production, the entire canopy is harvested several times during growing season and the proportion of tillers with true stem (either ELONG or GEN tillers) determines the quantity and quality of the yield. While in winter cereals the timing of flowering has a major role in adaptation (Shimizu et al., 2011), for forage grasses the major consideration with respect to cultivation is the influence of flowering behavior on growth rate and biomass accumulation in the short days of autumn.
In this study, wide variation in flowering time among timothy accessions in vernalization response was reported. Northern accessions typically showed a strong vernalization response, which was seen as decreased HD after vernalization, whereas southern accessions did not respond to vernalization and HD remained unchanged. In cereals rapid flowering without vernalization is typical for spring cultivars (e.g., Fowler et al., 1996; Danyluk et al., 2003) and it seems that in timothy both spring and winter type accessions exists within same species similar to Arabidopsis and cereals. In many species ecotypic variation in vernalization response has been seen, e.g., in A. thaliana (Grillo et al., 2013), A. lyrata (Quilot-Turion et al., 2013), barley (Loscos et al., 2014), Brachypodium (Ream et al., 2014), Poa sp. (Heide, 2001), and wheat (Iwaki et al., 2001). Fiil et al. (2011) reported that in timothy HD was correlated with geographic origin in non-vernalized plants, but after 15 weeks of vernalization differences were diminished. Also in this study flowering behavior was to some extent associated with the geographic origin of accessions studied. Vernalization response, expressed as a shortening of HD, was strongest in northern accessions and was absent in some southern accessions. It should be noted, however, that PP has the most important role in the regulation of flowering in timothy (Jokela et al., 2014) and that critical PP requirement varies between cultivars by their geographic origin (Heide, 1982, 1994). The 12 h PP has been shown to be too short for the flower initiation here, similar to earlier studies (e.g., Heide, 1982; Jokela et al., 2014). Here the most northern accession BOR N required longer vernalization for flowering in 16 h-PP. The genetic variation in HD diminished between accessions of different origin as the vernalization time or PP was long enough, and even BOR N was able to flower without vernalization in 20-h PP. Similar dependence on PP was not observed in Arabidopsis (Grillo et al., 2013) referring to a particular PP sensitivity of flowering in timothy, which has been observed to some degree also in Brachypodium (Ream et al., 2014). The possibility of SD induction for flowering during the pre-growth period in greenhouse can be rejected based on our earlier study where timothy plants were grown in 16-h PP following a similar pre- treatment, without vernalization, and were not able to flower (Seppänen et al., 2010). For the future research it should be noted that the experimental conditions, especially the greenhouse temperature and natural light, might have considerable effect on the growth of timothy plants. Here, the second experiment was conducted later in the spring when greenhouse temperature rose and PP was longer, which resulted in faster heading and taller plants compared to first experiment. However, the ability of accessions to flower was similar between them in both experiments. In addition, BOR S flowered constantly after ca. 45 days in both experiments, and thus it seems that the reaction to changing environmental conditions differs between accessions.
Final leaf number is useful characteristic to define the fulfillment of vernalization requirement of cereals and grasses (Frank and Bauer, 1995). This was first time to our knowledge when FLN was used to determine the vernalization fulfillment/response in forage grasses. However, it seems that FLN is unsuitable tool to define the vernalization saturation in timothy, because in several studied accessions the FLN was at constant level after all vernalization treatments despite the growth status. Only northern Närekumpu reacted similarly as winter cereals: when vernalization saturation was reached also the FLN was lower. It can be summarized that based on these experiments FLN cannot be used to determine timothy accessions having vernalization requirement. The typical growth habit for grasses is to produce more lateral tillers, and thus the relationship between FLN and vernalization might not be as significant as in winter cereals. So, whereas winter cereals show increasing leaf number with delayed flowering, FLN of timothy remains constant and there are instead increases in tiller number. In addition, the used experimental conditions may have kept some of the accessions in dormancy and thus, the leaf development had not started properly.
A clear connection between flowering and expression of PpVRN1 and PpVRN3 was detected in this study in timothy (Figure 7). In temperate cereals VRN1 is activated by vernalization, which in turn blocks VRN2 (Trevaskis et al., 2006; Hemming et al., 2008), and VRN3 acts as an integrator of vernalization and PP pathways (reviewed, e.g., Distelfeld et al., 2009; Kamran et al., 2014). Ream et al. (2014) suggested that there exists a positive feedback loop between VRN1 and VRN3/FT, and it is conserved throughout Pooideae species including Brachypodium. Recent study by Deng et al. (2015) revealed that PP and vernalization response pathways have a direct connection, and moreover FT1 (VRN3) is a direct target of VRN1. Ergon et al. (2013) proposed that, however, the role of VRN1 in outcrossing forage grass species may be less important than in cereals. This hypothesis was supported by the present results and our earlier study, where we suggested that PpVRN1 alone is insufficient to trigger flowering stimulus, and PpVRN3 is required for flowering (Jokela et al., 2014). Diallo et al. (2012) suggested that TaFT1, homolog of PpVRN3, is involved at the last stages of the transition to reproductive growth in wheat, and it is not required after the flowering initiation. The major role of VRN3/FT genes connected to PP sensitivity has been shown earlier also, e.g., in barley (Hemming et al., 2008), oats (Nava et al., 2012), and wheat (Diallo et al., 2012), where the expression of TaFT1 was significantly higher in spring cultivar. In our earlier study, we speculated that the PP sensitivity in two studied cultivars could be seen in the expression levels of PpVRN3 (Jokela et al., 2014). Similar results were obtained in the present study, where the PpVRN3 expression levels were constantly higher in BOR S compared to BOR N, probably due the potential role of PpVRN3 as an integrator of vernalization and PP pathways. Interestingly, here the expression peak of PpVRN3 was detected in BOR S plants grown 2 weeks in 16 and 20 h PP, also without vernalization.
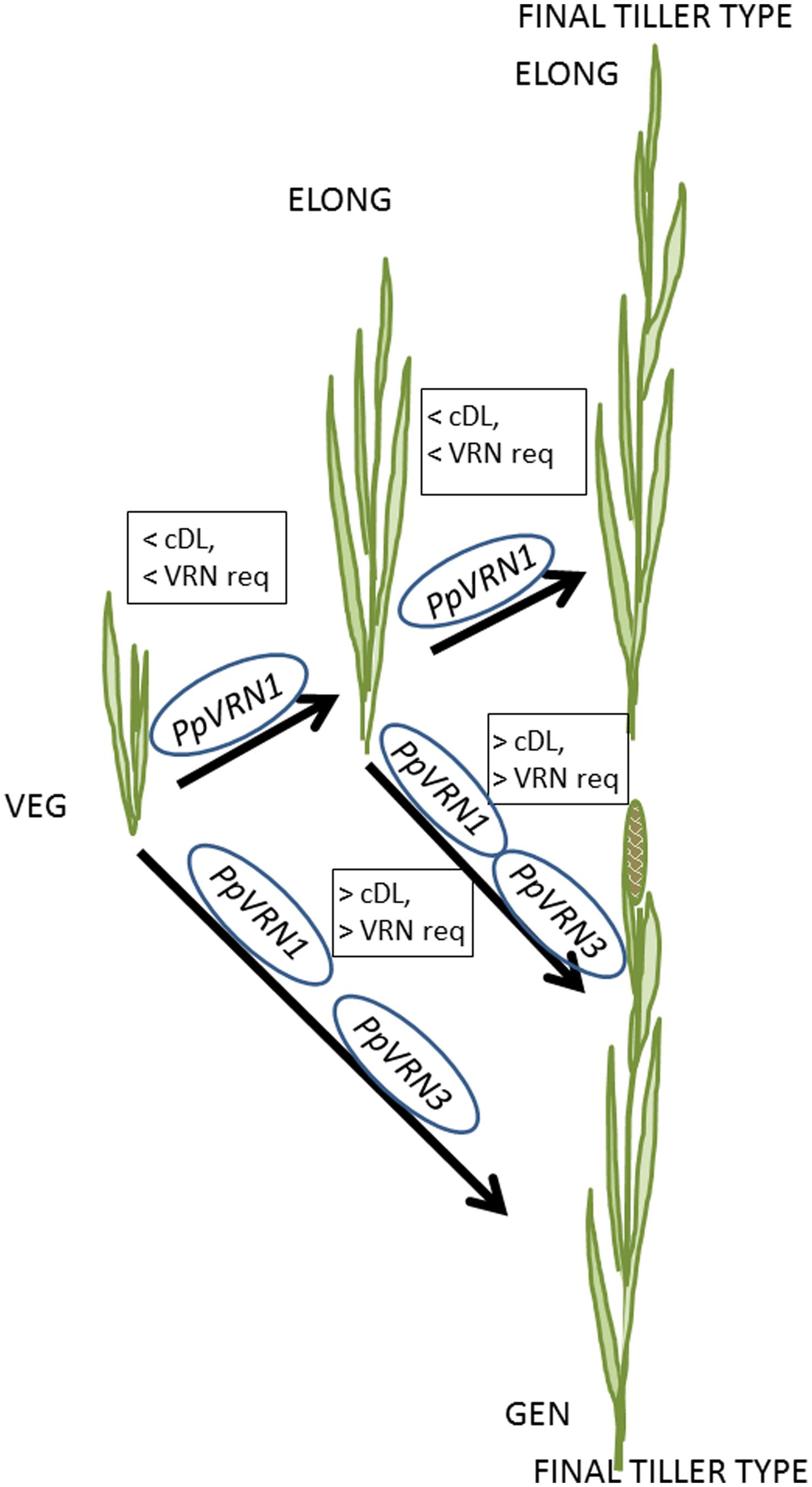
FIGURE 7. A simplified schematic model to illustrate how critical day length (cDL) and vernalization requirement (VRN req) regulate the formation of vegetative (VEG), vegetative elongating (ELONG) and generative (GEN) tiller types in timothy. The final tiller type is either ELONG or GEN tiller. In addition, the proposed roles of PpVRN1 and PpVRN3 in tiller formation are presented.
Studies done with cereals have shown that especially rapid flowering accessions holding dominant alleles of VRN1 and FT (VRN3) can bypass the vernalization requirement (Yan et al., 2006; Faure et al., 2007). Based on the results in this paper, it seems that this kind of bypassing the vernalization requirement is also possible in timothy if PP is long enough. Taken together, these observations enable the speculations of the crucial role of PP in the flowering of the timothy both at physiological and molecular level. The transcript accumulation of PpVRN1 and PpVRN3 were at different levels, and the vernalization saturation occurred at divergent rate in two separate experiments. Although, the vernalization saturation was always connected to higher expression of the studied genes, and if plants were not saturated, also the levels of PpVRN1 and PpVRN3 remained low. The allele form of VRN1 has been shown to classify cereal types to spring (dominant), and winter (recessive) types (Fu et al., 2005; Chu et al., 2011). Further, vernalization has promoting effect on the level of active state of TaFT1 chromatin in winter types, whereas in spring types vernalization reduces it (Diallo et al., 2012). In timothy accessions, on the contrary, vernalization does not seem to have similar effect on the expression of PpVRN3. Hence, the more detailed analysis and grouping timothy accessions to spring and winter types would require allelic and epigenetic studies of PpVRN1 and PpVRN3.
In Arabidopsis genetic variation and linkage of flowering time and adaptation has been analyzed in locally adapted populations (Grillo et al., 2013). In general, Arabidopsis ecotypes can be characterized either as winter annuals, summer annuals germinating in spring and flowering without vernalization, or overwintering as rosettes requiring vernalization (Alonso-Blanco and Koornneef, 2000). Grillo et al. (2013) found that winter annuals originating from Sweden failed to flower without vernalization, whereas populations from Italy flowered. Moreover, the northern populations had longer time to flower after vernalization, i.e., they could be characterized as late flowering types (Grillo et al., 2013). The northern Arabidopsis accessions have strict vernalization requirement for flowering, whereas in timothy PP can diminish vernalization requirement, as it has been shown in this study. Mutant studies have revealed that in Arabidopsis winter annuals allelic variation in FRI and FLC is the main regulators of flowering (Lee et al., 1993; Clarke and Dean, 1994; Johanson et al., 2000; Lempe et al., 2005). In the presence of functional FRI alleles, latitudinal decline in flowering time is correlated with FLC expression (Caicedo et al., 2004; Lempe et al., 2005; Shindo et al., 2005). Results on the QTL mapping of F2 populations produced by crossing northern and southern Arabidopsis populations show that the genetic variation in flowering time was co-localized to FLC locus (Grillo et al., 2013). In timothy divergent transcript accumulation of PpMADS10 was seen between accessions especially at conditions where the response to vernalization was different. The variation in HD in timothy was diminished in long enough PP or vernalization treatments. Furthermore, in growing conditions, where ecotypical differences in flowering behavior between BOR S and BOR N were not seen differences in PpMADS10 were also absent. It is possible that the variation in PpMADS10 expression in southern and northern timothy accessions seems to be comparable to the variation of FLC expression in Arabidopsis ecotypes of different origin. It should be noted, however, that there was also a strong interaction between developmental stage of the shoot apex and PpMADS10 expression level. In most cases at 16- and 20-h PP low PpMADS10 levels were detected in the apices at the reproductive stage, so low PpMADS10 expression might be a consequence of reproductive development rather than the cause.
Our results provide more information on the regulation of flowering and canopy structure in perennial forage grass, timothy. It seems that PP is the key regulator in the initiation of flowering. In addition, in northern accessions also vernalization has important role. However, after long enough vernalization or PP treatments differences in HD between accessions diminished. The expression of flowering promoting genes, PpVRN1 and PpVRN3, were connected to vernalization saturation, and flowering. Both physiological and molecular observations indicate that spring and winter types can be found also in timothy.
Author Contributions
VJ and MS planned the study. VJ carried out the experiments and analyzed the data. All authors wrote, read and approved the final manuscript.
Conflict of Interest Statement
The authors declare that the research was conducted in the absence of any commercial or financial relationships that could be construed as a potential conflict of interest.
Acknowledgments
We thank Juha Luhtanen for the excellent care of timothy plants in greenhouse and data analysis of the Experiment 1. Mika Isolahti from Boreal Plant Breeding is acknowledged for providing clonal material for BOR S, BOR N, BOR 1 and Donatello. Jeppe Andersen and Alice Fiil are acknowledged for providing clonal material for Karasjok, Närekumpu, Saltum, Turkey, 6116 and 51998. Professor Perttu Virkajärvi is acknowledged for providing the photograph showing tiller types (Supplementary Figure S1). The work was partly funded by Finnish Cultural Foundation and Finnish Ministry of Agriculture and Forestry, project NurMi.
Supplementary Material
The Supplementary Material for this article can be found online at: http://journal.frontiersin.org/article/10.3389/fpls.2015.00465
Figure S1 | The tiller types of timothy, (A) generative (GEN), (B) vegetative elongating (ELONG), (C) vegetative (VEG) tiller. Photograph: Perttu Virkajärvi.
Figure S2 | The effect of vernalization on final leaf number (FLN) in timothy accessions (n = 9). Plants were vernalized for 2, 10, 12, or 15 weeks in growth chamber [at 6/4°C (day/night), 8 h DL; control plants in 20/15°C (day/night), 12 h DL] and grown in 16-h DL in greenhouse for over 3 months. FLN was calculated at the end of the experiment. Data shows mean of three replicates of three plants ± SE. Distinct letters above bars indicate the significant differences in one vernalization treatment between accessions calculated by Tukey’s test.
Figure S3 | The effect of different vernalization and PPs on FLN in four timothy accessions (n = 6). Plants were vernalized 0 (light gray), 10 (dark gray), or 15 (black) weeks in growth chamber [at 6/4°C (day/night), 8 h DL; control plants in 20 /15°C (day/night), 12 h DL] and transferred to (A) 12-h, (B) 16-h DL or (C) 20-h DL in greenhouse. FLN was calculated at the end of the experiment. Data shows mean of two replicates of three plants ± SE.
Abbreviations
ELONG, vegetative elongating tiller; FLN, final leaf number; GEN, generative tiller; HD, heading date; LAI, leaf area index; PP, photoperiod; VEG, vegetative tiller; VRN, vernalization genes.
References
Alonso-Blanco, C., and Koornneef, M. (2000). Naturally occurring variation in Arabidopsis: an underexploited resource for plant genetics. Trends Plant Sci. 5, 22–29. doi: 10.1016/S1360-1385(99)01510-1
Andrews, C. J., and Gudleifsson, B. E. (1983). A comparison of cold hardiness and ice encasement tolerance of timothy grass and winter wheat. Can. J. Plant Sci. 63, 429–435. doi: 10.4141/cjps83-049
Berry, G. J., Salisbury, P. A., and Halloran, G. M. (1980). Expression of vernalization genes in near-isogenic wheat lines: duration of vernalization period. Ann. Bot. 46, 235–241.
Caicedo, A. L., Stinchcombe, J. R., Olsen, K. M., Schmitt, J., and Purugganan, M. D. (2004). Epistatic interaction between Arabidopsis FRI and FLC flowering time genes generates a latitudinal cline in a life history trait. Proc. Natl. Acad. Sci. U.S.A. 101, 15670–15675. doi: 10.1073/pnas.0406232101
Chang, S., Puryear, J., and Cairney, J. (1993). A simple and efficient method for isolating RNA from pine trees. Plant Mol. Biol. Rep. 11, 113–116. doi: 10.1007/BF02670468
Chen, F., Gao, M., Zhang, J., Zuo, A., Shang, Z., and Cui, D. (2013). Molecular characterization of vernalization and response genes in bread wheat from the Yellow and Huai Valley of China. BMC Plant Biol. 13:199. doi: 10.1186/1471-2229-13-199
Chu, C. G., Tan, C. T., Tu, G. T., Zhong, S., Xu, S. S., and Yan, L. (2011). A novel retrotransposon inserted in the dominant Vrn-B1 allele confers spring growth habit in tetraploid wheat (Triticum turgidum L.). G3 7, 637–645. doi: 10.1534/g3.111.001131
Ciannamea, S., Kaufmann, K., Frau, M., Tonaco, I., Petersen, K., Nielsen, K., et al. (2006). Protein interactions of MADS box transcription factors involved in flowering in Lolium perenne. J. Exp. Bot. 57, 3419–3431. doi: 10.1093/jxb/erl144
Clarke, J. H., and Dean, C. (1994). Mapping FRI, a locus controlling flowering time and vernalization response in Arabidopsis thaliana. Mol. Gen. Genet. 242, 81–89. doi: 10.1007/BF00277351
Danyluk, J., Kane, N. A., Breton, G., Limin, A. E., Fowler, D. B., and Sarhan, F. (2003). TaVRT-1, a putative transcription factor associated with vegetative to reproductive transition in cereals. Plant Physiol. 132, 1849–1860. doi: 10.1104/pp.103.023523
Deng, W., Casao, C., Wang, P., Sato, K., Hayes, P. M., Finnegan, E. J., et al. (2015). Direct links between the vernalization response and other key traits of cereal crops. Nat. Commun. 6, 5882. doi: 10.1038/ncomms6882
Diallo, A. O., Ali-Benali, M. A., Badawi, M., Houde, M., and Sarhan, F. (2012). Expression of vernalization responsive genes in wheat is associated with histone H3 trimethylation. Mol. Genet. Genomics 289, 575–590. doi: 10.1007/s00438-012-0701-0
Distelfeld, A., Li, C., and Dubcovsky, J. (2009). Regulation of flowering in temperate cereals. Curr. Opin. Plant Biol. 12, 178–184. doi: 10.1016/j.pbi.2008.12.010
Ergon,Å., Hamland, H., and Rognli, O. A. (2013). Differential expression of VRN1 and other MADS-box genes in Festuca pratensis selections with differential vernalization requirements. Biol. Plant. 57, 245–254. doi: 10.1007/s10535-012-0283-z
Faure, S., Higgins, J., Turner, A., and Laurie, D. A. (2007). The FLOWERING LOCUS T-like gene family in barley (Hordeum vulgare). Genetics 176, 599–609. doi: 10.1534/genetics.106.069500
Fiil, A., Jensen, L. B., Fjellheim, S., Lubbersted, T., and Andersen, J. R. (2011). Variation in the vernalization response of a geographically diverse collection of timothy accessions. Crop Sci. 51, 2689–2697. doi: 10.2135/cropsci2010.12.0677
Fjellheim, S., Boden, S., and Trevaskis, B. (2014). The role of seasonal flowering responses in adaptation of grasses to temperate climates. Front. Plant Sci. 5:431. doi: 10.3389/fpls.2014.00431
Fjellheim, S., Tanhuanpää, P., Marum, P., Manninen, O., and Rognli, O. A. (2015). Phenotypic or molecular diversity screening for conservation of genetic resources? An example from a genebank collection of the temperate forage grass timothy. Crop Sci. 55, 1–14. doi: 10.2135/cropsci2014.12.0825
Fowler, D. B., Limin, A. E., Wang, S-Y., Ward, R. W. (1996). Relationship between low-temperature tolerance and vernalization response in wheat and rye. Can. J. Plant Sci. 76, 37–42. doi: 10.4141/cjps96-007
Frank, A. B., and Bauer, A. (1995). Phyllochron differences in wheat, barley, and forage grasses. Crop Sci. 35, 19–23. doi: 10.2135/cropsci1995.0011183X003500010004x
Fu, D., Szucs, P., Yan, L., Helguera, M., Skinner, J. S., von Zitzewitz, J., et al. (2005). Large deletions within the first intron in VRN-1 are associated with spring growth habit in barley and wheat. Mol. Genet. Genomics 273, 54–65. doi: 10.1007/s00438-004-1095-4
Grillo, M. A., Li, C., Hammond, M., Wang, L., and Schemske, D. W. (2013). Genetic architecture of flowering time differentiation between locally adapted populations of Arabidopsis thaliana. New Phytol. 197, 1321–1331. doi: 10.1111/nph.12109
Gustavsson, A.-M. (2011). A developmental scale for perennial forage grasses based on the decimal code framework. Grass Forage Sci. 66, 93–108. doi: 10.1111/j.1365-2494.2010.00767.x
Heide, O. M. (1982). Effects of photoperiod and temperature on growth and flowering in Norwegian and British timothy cultivars (Phleum pratense L.). Acta Agric. Scand. 32, 241–252. doi: 10.1080/00015128209435320
Heide, O. M. (1994). Control of flowering and temperature in temperate grasses. New Phytol. 128, 347–362. doi: 10.1111/j.1469-8137.1994.tb04019.x
Heide, O. M. (2001). Flowering responses of contrasting ecotypes of Poa annua and their putative ancestors Poa informa and Poa supine. Ann. Bot. 87, 795–804. doi: 10.1006/anbo.2001.1406
Hemming, M. N., Peacock, W. J., Dennis, E. S., and Trevaskis, B. (2008). Low-temperature and daylength cues are integrated to regulate FLOWERING LOCUS T in barley. Plant Physiol. 147, 355–366. doi: 10.1104/pp.108.116418
Iwaki, K., Haruna, S., Niwa, T., and Kato, K. (2001). Adaptation and ecological differentiation in wheat with special reference to geographical variation of growth habit and Vrn accession. Plant Breed. 120, 107–114. doi: 10.1046/j.1439-0523.2001.00574.x
Johanson, U., West, J., Lister, C., Michaels, S., Amasino, R., and Dean, C. (2000). Molecular analysis of FRIGIDA, a major determinant of natural variation in Arabidopsis flowering time. Science 290, 344–347. doi: 10.1126/science.290.5490.344
Jokela, V., Virkajärvi, P., Tanskanen, J., and Seppänen, M. M. (2014). Vernalization, gibberellic acid and photo period are important signals of yield formation in timothy (Phleum pratense) Physiol. Plant. 152, 152–163. doi: 10.1111/ppl.12141
Junttila, O. (1985). Experimental control of flowering and vivipary in timothy. Physiol. Plant. 63, 35–42. doi: 10.1111/j.1399-3054.1985.tb02814.x
Jönsson, H. A., Kristiansson, U., Nilsson, C., and Sjödin, J. (1992). Morphological characters in spaced plants associated with forage yield under sward conditions in timothy (Phleum pratense L.). Acta Agric. Scand. 42, 18–25. doi: 10.1080/09064719209410195
Jørgensen, M., Østrem, L., and Höglind, M. (2010). De-hardening in contrasting cultivars of timothy and perennial ryegrass during winter and spring. Grass Forage Sci. 65, 38–48. doi: 10.1111/j.1365-2494.2009.00718.x
Kamran, A., Iqbal, M., and Spaner, D. (2014). Flowering time in wheat (Triticum aestivum L.): a key factor for global adaptability. Euphytica 197, 1–26. doi: 10.1007/s10681-014-1075-7
Kardailsky, I., Shukla, V. K., Ahn, J. H., Dagenais, N., Christensen, S. K., Nguyen, J. T., et al. (1999). Activation tagging of the floral inducer FT. Science 286, 1962–1965. doi: 10.1126/science.286.5446.1962
Kaufmann, K., Wellmer, F., Muino, J. M., Ferrier, T., Wuest, S. E., Kumar, V., et al. (2010). Orchestration of Floral Initiation by APETALA1. Science 328, 85–89. doi: 10.1126/science.1185244
Larsen, A., and Marum, P. (2006). “Breeding goals and possibilities in future timothy breeding,” in Proceedings of the NJF Seminar Timothy Productivity and Forage Quality- Possibilities and Limitations, 10–12 August, Akureyri, 31–39.
Lee, I., Bleecker, A., and Amasino, R. (1993). Analysis of naturally- occurring late flowering in Arabidopsis thaliana. Mol. Gen. Genet. 237, 171–176. doi: 10.1007/BF00282798
Lempe, J., Balasubramanian, S., Sureshkumar, S., Singh, A., Schmid, M., and Weigel, D. (2005). Diversity of flowering responses in wild Arabidopsis thaliana strains. PLoS Genet. 1:e6. doi: 10.1371/journal.pgen.0010006
Livak, K. J., and Schmittgen, T. D. (2001). Analysis of relative gene expression data using real-time quantitative PCR and the 2-ΔΔCT method. Methods 25, 402–408. doi: 10.1006/meth.2001.1262
Loscos, J., Iggartua, E., Contreras-Moreira, B., Gracia, M. P., and Casas, A. M. (2014). HvFT1 polymorphism and effect- survey of barley germplasm and expression analysis. Front. Plant Sci. 5:251. doi: 10.3389/fpls.2014.00251
Mahfoozi, S., Limin, A. E., and Fowler, D. B. (2001). Influence of vernalization and photoperiod responses on cold hardiness in winter cereals. Crop Sci. 41, 1006–1011. doi: 10.2135/cropsci2001.4141006x
Nava, I. C., Wight, C. P., Pacheco, M. T., Federizzi, L. C., and Tinker, N. A. (2012). Tagging and mapping candidate loci for vernalization and flower initiation in hexaploid oat. Mol. Breed. 30, 1295–1312. doi: 10.1007/s11032-012-9715-x
Oliver, S. N., Finnegan, E. J., Dennis, E. S., Peacock, J., and Trevaskis, B. (2009). Vernalization-induced flowering in cereals is associated with changes in histone methylation at the VERNALIZATION1 gene. Proc. Natl. Acad. Sci. U.S.A. 106, 8386–8391. doi: 10.1073/pnas.0903566106
Quilot-Turion, B., Leppälä, J., Leinonen, P. H., Waldmann, P., Savolainen, O., and Kuittinen, H. (2013). Genetic changes in flowering and morphology in response to adaptation to a high-latitude environment in Arabidopsis lyrata. Ann. Bot. 111, 957–968. doi: 10.1093/aob/mct055
Ream, T. S., Woods, D. P., Schwartz, C. J., Sanabria, C. P., Mahoy, J. A, Walters, E. M., et al. (2014). Interaction of photoperiod and vernalization determines flowering time of Brachypodium distachyon. Plant Physiol. 164, 694–709. doi: 10.1104/pp.113.232678
Sasani, S., Hemming, M. N., Oliver, S. N., Greenup, A., Tavakkol-Afshari, R., Mahfoozi, S., et al. (2009). The influence of vernalization and daylength on expression of flowering-time genes in the shoot apex and leaves of barley (Hordeum vulgare). J. Exp. Bot. 60, 2169–2178. doi: 10.1093/jxb/erp098
Seppänen, M., Pakarinen, K., Jokela, V., Andersen, J. R., Fiil, A., Santanen, A., et al. (2010). Vernalization response of Phleum pratense and its relationships to stem lignification and floral transition. Ann. Bot. 106, 697–707. doi: 10.1093/aob/mcq174
Sherman, J. D., and Talbert, L. E. (2002). Vernalization-induced changes of the DNA methylation pattern in winter wheat. Genome 45, 253–260. doi: 10.1139/g01-147
Shimizu, K. K., Kudoh, H., and Kobayashi, M. J. (2011). Plant sexual reproduction during climate change: gene function in natura studied by ecological and evolutionary systems biology. Ann. Bot. 108, 777–787. doi: 10.1093/aob/mcr180
Shindo, C., Aranzana, M. J., Lister, C., Baxter, C., Nicholls, C., Nordborg, M., et al. (2005). Role of FRIGIDA and FLOWERING LOCUS C in determining variation in flowering time of Arabidopsis. Plant Physiol. 138, 1163–1173. doi: 10.1104/pp.105.061309
Stewart, S. V., Ellison, N., and Joachimiak, A. (2011). “Phleum,” in Wild Crop Relatives: Genomic and Breeding Resources, Millets and Grasses, ed. C. Kole (Berlin: Springer-Verlag), 257–274. doi: 10.1007/978-3-642-14255-0_14
Sweet, N., Wiltshire, J. J. J., and Baker, C. K. (1991). A new descriptive scale for early reproductive development in Lolium perenne L. Grass Forage Sci. 46, 201–206. doi: 10.1111/j.1365-2494.1991.tb02223.x
Tanhuanpää, P., and Manninen, O. (2012). High SSR diversity but little differentiation between accessions of Nordic timothy (Phleum pretense L.). Hereditas 149, 114–127. doi: 10.1111/j.1601-5223.2012.02244.x
Trevaskis, B., Hemming, M. N., Peacock, W. J., and Dennis, E. S. (2006). HvVRN2 responds to day length, whereas HvVRN1 is regulated by vernalization and developmental status. Plant Physiol. 140, 1397–1405. doi: 10.1104/pp.105.073486
Virkajärvi, P., Pakarinen, K., Hyrkäs, M., Seppänen, M. M., and Bélanger, G. (2012). Tiller characteristics of timothy and tall fescue in relation to herbage mass accumulation. Crop Sci. 52, 970–980. doi: 10.2135/cropsci2011.01.0039
Yan, L., Helguera, M., Kato, K., Fukuyama, S., Sherman, J., and Dubcovsky, J. (2004). Allelic variation at the VRN-1 promoter region in polyploid wheat. Theor. Appl. Genet. 109, 1677–1686. doi: 10.1007/s00122-004-1796-4
Keywords: flowering, photoperiod, Phleum pratense L., PpMADS10, PpVRN1, PpVRN3, timothy, vernalization
Citation: Jokela V, Trevaskis B and Seppänen MM (2015) Genetic variation in the flowering and yield formation of timothy (Phleum pratense L.) accessions after different photoperiod and vernalization treatments. Front. Plant Sci. 6:465. doi: 10.3389/fpls.2015.00465
Received: 01 February 2015; Accepted: 11 June 2015;
Published: 30 June 2015.
Edited by:
Diego Rubiales, Consejo Superior de Investigaciones Cientificas, SpainReviewed by:
Margherita Irene Beruto, Istituto Regionale per la Floricoltura, ItalyMarcelo Carnier Dornelas, Universidade Estadual de Campinas, Brazil
Rina Kamenetsky, Agricultural Research Organization – The Volcani Center, Israel
Copyright © 2015 Jokela, Trevaskis and Seppänen. This is an open-access article distributed under the terms of the Creative Commons Attribution License (CC BY). The use, distribution or reproduction in other forums is permitted, provided the original author(s) or licensor are credited and that the original publication in this journal is cited, in accordance with accepted academic practice. No use, distribution or reproduction is permitted which does not comply with these terms.
*Correspondence: Venla Jokela, Department of Agricultural Sciences, University of Helsinki, P. O. Box 27, Latokartanonkaari 5, FI-00014 Helsinki, Finland,dmVubGEuam9rZWxhQGhlbHNpbmtpLmZp