- 1Center of Pear Engineering Technology Research, State Key Laboratory of Crop Genetics and Germplasm Enhancement, Nanjing Agricultural University, Nanjing, China
- 2Institute of Horticulture, Zhejiang Academy of Agricultural Sciences, Hangzhou, China
An evaluation of fruit wax components will provide us with valuable information for pear breeding and enhancing fruit quality. Here, we dissected the epicuticular wax concentration, composition and structure of mature fruits from 35 pear cultivars belonging to five different species and hybrid interspecies. A total of 146 epicuticular wax compounds were detected, and the wax composition and concentration varied dramatically among species, with the highest level of 1.53 mg/cm2 in Pyrus communis and the lowest level of 0.62 mg/cm2 in Pyrus pyrifolia. Field emission scanning electron microscopy (FESEM) analysis showed amorphous structures of the epicuticular wax crystals of different pear cultivars. Cluster analysis revealed that the Pyrus bretschneideri cultivars were grouped much closer to Pyrus pyrifolia and Pyrus ussuriensis, and the Pyrus sinkiangensis cultivars were clustered into a distant group. Based on the principal component analysis (PCA), the cultivars could be divided into three groups and five groups according to seven main classes of epicuticular wax compounds and 146 wax compounds, respectively.
Introduction
Cuticular wax is the product of a complex mixture of very-long-chain (VLC) aliphatic compounds and their oxygenated derivatives, including fatty acids, alkanes, alcohols, esters, aldehydes, ketones, and triterpenes (Kolattukudy, 1996). It has been widely reported that wax plays important roles in moderating gas exchange, limiting non-stomatal water loss, protecting plants against ultraviolet (UV) radiation and extreme temperature damage, self-cleaning behavior and providing mechanical support to maintain the integrity of plant organs (Wang et al., 2016). More and more studies have suggested that the fruit cuticular wax layer acts as the first protective barrier against fruit splitting and plays pivotal roles in the reduction of pathogenic and insect attacks, protection against mechanical damage (Chu et al., 2017). Studies also showed that cuticular wax play important roles in maintaining the postharvest quality and delaying fruit senescence. For example, the removal of the natural wax of blueberry fruits can accelerate the postharvest water loss and decay, reduce the sensory and nutritional qualities, and then shorten the fruit shelf-life (Chu et al., 2018). In addition, as one of the most important quality factors determining consumer demand, the apple’s appearance during the postharvest storage were also determined by the physical and chemical properties of wax composition (Glenn et al., 1990).
The chemical composition and morphology of the cuticular wax layer of several fruit species have been detected. For example, triterpenoids and β-diketones were the most prominent compounds in blueberry fruits, and a large amount of tubular wax was deposited on their surfaces (Chu et al., 2017). In the mature fruits of olives, triterpenes were the main compounds, and only epicuticular waxes were observed, arranged in various crystalloid structures, including granules, platelets, plates and rodlets (Lanza and Di Serio, 2015). Aldehydes, triterpenes and secondary alcohols were the most predominant components of cuticular wax from citrus and plum fruits (Ismail et al., 1977; Wang et al., 2014). The wax concentration, chemical composition and morphology were also reported to be positively correlated with disease resistance capabilities (Wu et al., 2017). Therefore, a clear understanding of the components and amounts of fruit wax is important for obtaining better fruit quality, improving disease resistance and developing postharvest treatment strategies. More importantly, the structure, composition and concentration of fruit wax vary among cultivars of the same species; thus, knowledge of the cuticular wax traits from different germplasms will also be helpful for the selection of breeding parents. To the best of our knowledge, the cuticular wax profiles of different cultivars from several fruit and vegetable species have been detected, such as various cultivars of apple (Belding et al., 1998), grape (Pensec et al., 2014), blueberry (Chu et al., 2017), persimmon (Tsubaki et al., 2012), tomato (Bauer et al., 2004), and pepper (Parsons et al., 2012).
As the third most important temperate fruit species, the pear belongs to the Rosaceae family. The Pyrus genus is genetically diverse with 1000s of cultivars, which can be divided into two major groups, Occidental (European) and Oriental (Asiatic) pears. The Oriental pears comprise Pyrus bretschneideri, Pyrus ussuriensis, Pyrus pyrifolia, and Pyrus sinkiangensis and are mainly grown in China, Korea, Japan, and other Asian countries, whereas the Occidental pear (Pyrus communis Linn.) is mainly produced in America, Italy, Spain, and Germany. Previous studies have reported that the amount of wax obtained from four pear varieties (‘Pingguoli’ and ‘Xuehua’, P. bretschneideri; ‘Kuerle’, P. sinkiangensis; ‘Yuluxiang’, hybrid cultivars) varied dramatically, with wax concentrations ranging from 0.32 mg/cm2 (‘Pingguoli’) to 1.43 mg/cm2 (‘Kuerle’) (Chen et al., 2014; Wu et al., 2017); however, in the mature fruit of pears belonging to the other three species, information about the epicuticular wax profile is very limited. More generally, the genetic relationships of several important fruit-related traits of pear have been reported, such as sugar, acid, and flesh color (Liu et al., 2016; Wu et al., 2014), but genetic relationship of pear cuticular wax was less reported. Thus, the investigation of the pear cuticular wax at the germplasm level is of significant value for both the genetic study and the higher wax pear breeding program.
In this study, 35 pear cultivars belonging to five different species and hybrid interspecies were selected for determination of the concentration, composition, structure and genetic relationship of epicuticular wax through GC–MS, FESEM and cluster analysis. The results in the present study will not only help further studies of the potential role of wax components on postharvest quality but also provide a foundation for plant breeding aimed at improving fruit epicuticular wax.
Materials and Methods
Plant Material
The composition, concentration and structure of epicuticular wax compounds of the fruits of 32 pear cultivars (Table 1) were evaluated in 2016, and the data of other three pear cultivars (‘Kuerle’, ‘Xuehua’ and ‘Yuluxiang’) was originated from our previous research (Wu et al., 2017). The fruits at commercial maturity, without disease, infection or physical injuries, were selected for the following experiments. Thirty fruits of each cultivar were packed individually in a plastic net bag and delivered immediately to the laboratory at Nanjing Agricultural University. All the samples were examined immediately.
Extraction of the Epicuticular Wax
The epicuticular wax was extracted according to the method of Li et al. (2014). After being washed with tap water and air dried, three groups of five pear fruits were fully immersed and agitated twice for 1 min in 600 mL chloroform under a fume hood. The solvent containing the waxes was transferred into pre-weighed vials and evaporated by a nitrogen-blowing instrument (JHD-001S, Shanghai Jiheng Industries Company, Ltd.) at 40°C, and the wax weights were recorded.
Determination of the Epicuticular Wax Concentration
The surface area of the pear fruits was calculated according to the method of Yin et al. (2011). The wax concentration (μg/cm2) was calculated with the following formula:
where W1 is the final weight of the vials (μg); W0 is the initial weight of the vials (μg); and Sa is the total surface area of 5 pear fruits (cm-2).
Chemical Analysis by GC-MS
The components present within each wax extraction prepared were analyzed using the method of Li et al. (2014). The wax extraction (1 mg) dissolved with 1.2 mL chloroform was carried out on a Bruker 450-GC, coupled with a Bruker 320-MS and a BR-5MS capillary column (30 m FS, 0.25 μm ID, 0.25 μm df). Helium was used as a carrier gas at a flow rate of 1.2 mL min-1. The following parameters were employed: inlet temperature, 280°C; MS transfer line temperature, 280°C; ion source temperature, 250°C; quadrupole temperature, 150°C; electron impact (EI), 70 eV; and m z-1 range, 50–650.
GC was carried out at the following temperature settings. First, the temperature was set to 50°C for 2 min. Next, it was increased to 200°C at a rate of 40°C min-1 and held at this temperature for 2 min. Finally, it was increased to 320°C at a rate of 3°C min-1 and held at this temperature for 30 min.
Electron Microscope Observations
The epicuticular wax was observed by FESEM according to the method of Wu et al. (2017). Pericarp pieces (3 × 3 × 1 mm) from the equatorial sections of three fruits for each cultivar were excised using a blade, fixed in 2.5% glutaraldehyde. The dehydrated samples were attached to a sample stage with conductive tape and coated with gold particles using a Hitachi E-1045. The coated samples were examined using a Hitachi 4800 field emission scanning electron microscope.
Statistical Analysis
The chemical composition of epicuticular wax was analyzed using the NIST 2013 Library. Box-plot, principal component analysis (PCA) and heatmap analysis of the dataset for wax concentration and composition were used to detect clustering and to establish relationships; these analyses were carried out using the R programming language. For the heatmap and clustering analysis, the scaled data file with Log transformed was calculated, and analyzed by the ‘complete’ algorithm in the heatmap.2 package (Murtagh and Legendre, 2014). We performed the principal component analysis (PCA) by the algorithm of ‘Multivariate Analysis’ in the ‘prcomp’ module of the R package, and 3D plot of PCA was drawn by the scatterplot3d packages (Mardia et al., 1979). The SPSS20 statistical software package (IBM Software Group) was used for all statistical analysis. Data were compared by Student’s t-test, and significant differences are marked with different letters (P < 0.05). Data are shown as the mean ± SD (n = 3).
Results and Discussion
Wax Amounts and VLC Aliphatic Compounds
The total wax of mature fruits from 35 pear cultivars was collected via chloroform extraction (Table 1). The amount of wax obtained from the 35 pear varieties varied dramatically (Figure 1 and Supplementary Tables S1, S2), and the wax concentrations ranged from 0.46 ± 0.03 mg/cm2 (‘Huagai’, P. ussuriensis) to 2.44 ± 0.41 mg/cm2 (‘Docteur Jules Guyot’, P. communis) (Figure 1A). On the species level, the epicuticular wax concentration was the highest in P. communis (1.53 ± 0.77 mg/cm2) and the lowest in P. pyrifolia (0.62 ± 0.12 mg/cm2) (Supplementary Table S2). Similar results have been identified among other species, such as pepper (2.15–7.52 μg/cm2) (Parsons et al., 2012), persimmon (337–770 μg/cm2) (Tsubaki et al., 2012), tomato (27–79 μg/cm2) (Bauer et al., 2004), blueberry (48–172 μg/cm2) (Chu et al., 2017), grape (3.5–5.5 mg/g) (Pensec et al., 2014) and apple (366–1038 μg/cm2) cultivars (Belding et al., 1998). Compared with these species, the average wax concentration of pears (1.05 mg/cm2) was the second highest, lower than only that of grapes. Various methods of wax extraction and surface area calculation were employed in these species; thus, the considerable variation of epicuticular wax obtained from different species was reasonable. In total, 146 epicuticular wax compounds were detected in the fruits of 35 cultivated pear species (Supplementary Table S1 and Supplementary Figure S1), mainly primary alcohols (26 compounds), alkanes (25 compounds), fatty acids (25 compounds), terpenoids (23 compounds), esters (16 compounds) and aldehydes (8 compounds), and some other small amounts of compounds (23 compounds, Supplementary Table S1); the content of these wax compounds accounted for 24.47, 40.72, 7.09, 11.80, 3.59, 3.45, and 8.88% of the total content, respectively (Figure 1A). Although the primary alcohols comprised the highest number of wax compounds, the alkanes were the dominant compounds in the epicuticular wax of pear fruits in terms of amounts. It has also been reported that alkanes are the major wax component in many fruits, including tomato (Leide et al., 2007), mandarin (Sala, 2000) and grapefruit (McDonald et al., 1993). However, limited studies reported that primary alcohols are essential components in fruits. Similar to the total wax content, the amount of each component also varied among the five different species and hybrid interspecies (Supplementary Figure S2). For example, the concentrations of alkanes, primary alcohols, fatty acids, esters and other epicuticular wax compounds were much higher in P. communis than in the other four species and hybrid interspecies (Supplementary Figures S2B,D,F,L,N), whereas the hybrid cultivars and P. ussuriensis had the highest concentrations of terpenoids and aldehydes, respectively (Supplementary Figures S2H,J). Similar results were also reported in blueberry fruits from nine blueberry cultivars belonging to three different species (Chu et al., 2017).
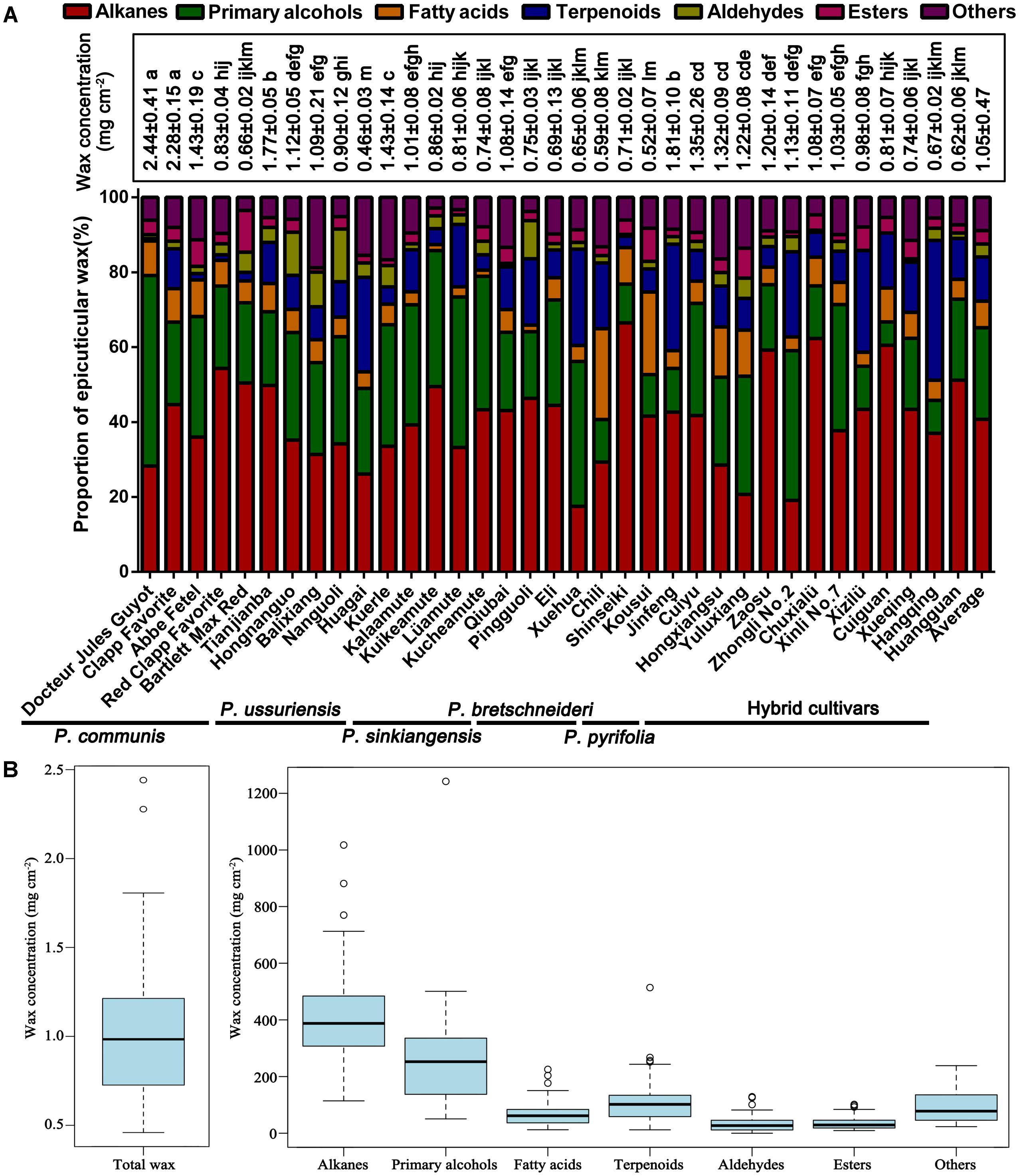
FIGURE 1. Amounts and compositions of epicuticular wax in the mature fruits of 35 pear cultivars. (A) Total epicuticular wax concentration and relative wax compositions of the mature fruits of 35 pear cultivars. Statistical significance was determined using Student’s t-test. Each value is the mean ± SD of three biological repeats. Different letters above each bar indicate significant differences at the 5% level. (B) Range and distribution of total wax and seven wax compounds in 35 pear cultivars. The horizontal lines in the interior of the box are the median values. The height of the box is equal to the interquartile distance, indicating the distribution for 50% of the data. Approximately 99% of cultivars fall inside the whiskers, and the cultivars outside these whiskers are considered outliers or extreme values, indicated by horizontal lines and circles.
Very-long-chain aliphatic compounds were the major epicuticular wax components found in the fruit surfaces of mature pears. Abundant VLC aliphatic compounds were detected in the 35 pear cultivars, and their concentrations varied greatly, ranging from 336.85 μg/cm2 (‘Huagai’, P. ussuriensis) to 769.87 μg/cm2 (‘Kuikeamute’, P. sinkiangensis) (Supplementary Table S2). Our results also showed that the VLC aliphatic compounds of pear fruits are mainly composed of alkanes (C16–C44), primary alcohols (C17–C41), fatty acids (C16–C26), and aldehydes (C16–C18) (Figure 2).
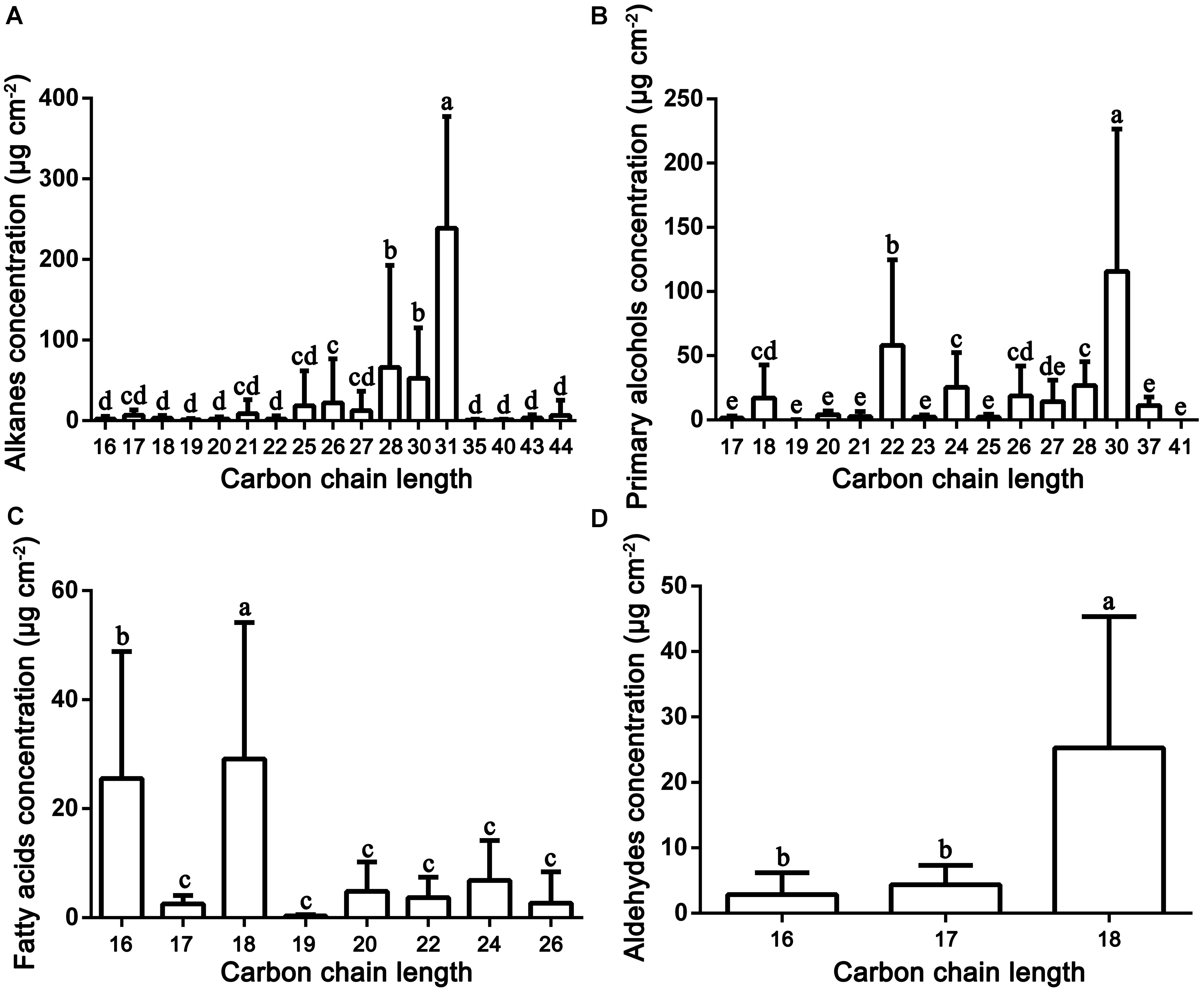
FIGURE 2. Distribution and concentration of the main VLC aliphatic compounds detected in the epicuticular wax of 35 pear cultivars. (A) Alkanes; (B) primary alcohols, (C) fatty acids; and (D) aldehydes. Statistical significance was determined using Student’s t-test. Each value is the mean ± SD of three biological repeats. Different letters above each bar indicate significant differences at the 5% level.
Of all the detected 35 pear cultivars, 20 (10 hybrid combinations) were genetically related (Supplementary Figure S3). This was designed to further understand the regular relationship of the epicuticular wax between the hybrids and their parents. Our results showed that five hybrid cultivars (‘Hongxiangsu’, ‘Yuluxiang’, ‘Xinli No. 7’, ‘Chuxialü’ and ‘Cuiyu’) with higher concentration were consistent with that of their parents (Supplementary Figures S3A–D), other three hybrid cultivars (‘Huangguan’, ‘Xueqing’ and ‘Cuiguan’) were also similar with their parents obtained lower concentration of epicuticular wax (Supplementary Figures S3G,H), and only two hybrid cultivars (‘Jinfeng’ and ‘Zhongli No. 2’) with higher concentration were contrary with that of their parents (Supplementary Figures S3E,F). These indicate that the epicuticular wax concentration of hybrid pear cultivars was positively correlated with that of the parents. Take an example, ‘Kuerle’ (Pyrus sinkiangensis Yü.) with relatively higher wax concentration is the most famous pear cultivar in China, not only for its pleasant appearance, aroma and taste, but also the longer postharvest storage period. Here, three hybrid cultivars (‘Hongxiangsu’, ‘Yuluxinag’ and ‘Xinli No. 7’) have all inherited the higher wax concentration from ‘Kuerle’, making it an excellent parent for pear breeding. In addition, ‘Docteur Jules Guyot’ (P. communis), ‘Tianjianba’ (P. ussuriensis), ‘Qiubai’ (P. bretschneideri) and ‘Shinseiki’ (P. pyrifolia) should be the corresponding optimal parent cultivars in each species for the pear breeding with higher wax concentrations.
Alkanes
Alkanes are important VLC aliphatic compounds in the epicuticular wax of pear fruits, with concentrations ranging from 114.19 μg/cm2 (‘Xuehua’, P. bretschneideri) to 1017.49 μg/cm2 (‘Clapp Favorite’, P. communis), respectively (Figure 1A and Supplementary Figure S2A). On the species level, the alkane concentrations were the highest in P. communis (601.14 μg/cm2), followed by hybrid cultivars (442.75 μg/cm2), P. ussuriensis (408.77 μg/cm2), P. sinkiangensis (578.50 μg/cm2) and P. pyrifolia (408.77 μg/cm2), but they were the lowest in P. bretschneideri (281.72 μg/cm2) (Supplementary Figure S2B). Many studies have shown that alkanes are predominant in epicuticular wax on many fruits, such as mandarin (148–234 mg/g) (Sala, 2000), pepper (1.4–47.4 μg/dm2) (Parsons et al., 2012) and tomato (55%, 13.75 μg/cm2) (Bauer et al., 2004); leaves, including those of Schefflera elegantissima (∼80%, 400 μg/cm2), Garcinia spicata (∼72%, 864 μg/cm2) (Jetter and Riederer, 2016), castor bean (64.1%–70.7%, 88.1–139.4 μg/cm2) (de Araújo Silva et al., 2017) and aloe (34.4%, 8.8 μg/cm2) (Racovita et al., 2015); and both fruits and leaves of cucumber (46.1%, 59.6 μg/cm2; 61.9%, 110.3 μg/cm2) (Wang W. et al., 2015). Compared with these species, the average wax concentration of pears (422.73 μg/cm2) is the second highest, only lower than that of Garcinia spicata. VLC alkanes ranging from hexadecane (C16) to tetratetracontane (C44) were detected in the fruit wax of the 35 pear species, and C28 to C31 were the most abundant. For example, the average amount of hentriacontane (C31, 238.94 μg/cm2) was 3.70 times higher than the amount of the least abundant alkane (Figure 2A). Similarly, hentriacontane (C31) was also detected as the predominant alkane in cuticle wax of ‘Newhall’ navel orange (Wang et al., 2014), tomato (Bauer et al., 2004), pepper, eggplant (Bauer et al., 2005) and aloe (Racovita et al., 2015). It has also been reported that alkanes are associated with limiting water loss (Zhang et al., 2007). Furthermore, low temperature induced the alkane-forming pathway resulting in the accumulation of very long chain alkanes (including C22, C27, C29, C31 alkane) and their derivatives on the surface of apple fruit peel, which may be positive response to the environment signals of temperature and extension of the storage period (Hao et al., 2017). Thus, the higher proportion of alkanes in epicuticular wax of pear fruits may play a significant role in limiting water loss and prolonging the shelf-life to keep the fruits juicy.
Primary Alcohols
Twenty-six primary alcohols were detected as the second dominant component in the epicuticular wax of pear fruits, accounting for 24.47% of the total wax (Figure 1A and Supplementary Table S1). The concentrations of primary alcohols in the epicuticular wax of pear fruits ranged from 50.54 μg/cm2 (‘Cuiguan’, hybrid cultivars) to 1242.11 μg/cm2 (‘Docteur Jules Guyot’, P. communis), and the average concentration was 271.04 μg/cm2 (Figure 2C). On the species level, the primary alcohols were the highest in P. communis (505.53 μg/cm2), followed by P. sinkiangensis (337.77 μg/cm2), P. ussuriensis (259.60 μg/cm2), hybrid cultivars (228.15 μg/cm2) and P. bretschneideri (174.87 μg/cm2), but the primary alcohol levels were the lowest in P. pyrifolia (65.75 μg/cm2) (Supplementary Figure S2D). Higher concentrations of primary alcohols have been detected only in the epicuticular wax of leaves, such as wheat (42.5–72.9%) (Wang Y. et al., 2015), salix (32–51%) (Teece et al., 2008) and citrus plants (23.0–38.4%) (Baker et al., 1975). By contrast, the epicuticular wax of fruits with lower concentrations of primary alcohols have been reported in several species, such as 3.2% in blueberries (Chu et al., 2017), 0–9.1% in apples (Belding et al., 1998) and 6.0–15.0% in citrus fruits (Baker et al., 1975), respectively. A wide distribution of VLC primary alcohol homologues ranging from C17 to C41 was detected in the wax of pear fruits. As shown in Figure 2B and Supplementary Table S1, the C30 alcohols, including triacontanol and triacontane-1,30-diol (average 115.66 μg/cm2), were the most abundant primary alcohols; a similar result has been reported in the epicuticular wax of blueberry fruits (Chu et al., 2017). In addition, tetracosanol (C24), dotriacontanol (C32), and octacosanol (C28) were the dominant primary alcohols in the fruit wax of lemons (Baker et al., 1975) and tomatoes (Bauer et al., 2004) and the leaf wax of wheat (Wang Y. et al., 2015), respectively, whereas hexacosanol (C26) has been detected as the main primary alcohol in the leaf wax of both orange (Baker et al., 1975) and salix plants (Teece et al., 2008).
Fatty Acids
Sixteen VLC fatty acids ranging from C16 to C26 were detected in the fruit wax of 35 pear species. The highest concentration of fatty acids was observed in ‘Docteur Jules Guyot’ (P. communis, 225.27 μg/cm2), whereas the lowest was detected in ‘Kucheamute’ (P. sinkiangensis, 12.28 μg/cm2), and the average concentration was 74.44 μg/cm2 (Supplementary Figure S2E). On the species level, the fatty acids were the highest in P. communis (132.95 μg/cm2), followed by P. pyrifolia (92.00 μg/cm2), hybrid cultivars (74.31 μg/cm2), P. ussuriensis (67.48 μg/cm2), and P. bretschneideri (57.99 μg/cm2), but they were the lowest in P. sinkiangensis (32.66 μg/cm2) (Supplementary Figure S2F). The fatty acid profile was predominantly composed of C16 and C18 carbons, and a similar result has been reported in the epicuticular wax of peach fruits (Figure 2C) (Belge et al., 2014). Fatty acids with moderate concentrations, being a common component, were widespread in the epicuticular wax. Compared with pears (C16–C26), the carbon chain length of fatty acids in other fruit species (C16–C34) is longer, and the prominent components of fatty acids are more than 20 carbon atoms. For example, the fatty acid profiles in fruits of blueberry (C16–C30) (Chu et al., 2017) and lemon (C16–C34) were predominantly composed of C30 chains, and the dominant fatty acids in fruits of orange (C16–C34), clementine (C16–C32) and mandarin (C16–C28) consisted of 28 carbon atoms, while in the leaves of these three citrus species (all C16–C34), chains with 32 carbon atoms were the major fatty acid component (Baker et al., 1975).
Aldehydes
Aldehydes were found in all cultivars but at low concentrations in the epicuticular wax of pear fruits, accounting for 3.45% of the total wax (Figure 1A). The highest concentration of aldehydes was observed in ‘Hongnanguo’ (P. ussuriensis, 128.69 μg/cm2), whereas it was not detected in ‘Cuiguan’ (hybrid cultivars), and the average concentration was 35.32 μg/cm2 (Supplementary Figure S2I). On the species level, the aldehydes were the highest in P. ussuriensis (87.17 μg/cm2), followed by P. sinkiangensis (35.04 μg/cm2), P. communis (32.00 μg/cm2), hybrid cultivars (25.35 μg/cm2) and P. bretschneideri (24.40 μg/cm2), and they were the lowest in P. pyrifolia (6.84 μg/cm2) (Supplementary Figure S2J). A series of aldehydes composed of 16, 17, and 18 carbon atoms were detected in pear wax, and octadecanal, 9-octadecenal,(Z)- and 13-octadecenal,(Z)- (C18, average 25.87 μg/cm2) was the most abundant aldehyde (Figure 2D and Supplementary Table S1). However, similar to the fatty acids, the carbon chain length of aldehydes was longer in other species than in pears, and the concentration and main type of aldehydes in different species showed considerable variation. The aldehydes were the main components in the total wax of citrus fruits including ‘Satsuma’ (C16–C32, 45% of the total wax) and ‘Newhall’ navel orange (C16–C33, 46% of the total wax) fruits (Wang et al., 2014). Aldehydes comprising 24 and 32 carbon atoms were found in olive wax and accounted for the smallest proportion (3–5%) of total wax (Bianchi et al., 1992). As direct precursors of alkanes, aldehydes determine the alkane formation. Similar to alkanes, aldehydes have been identified as playing an important role in avoiding water loss (Wang et al., 2014).
Esters
Similar to aldehydes, the proportion of esters accounted for 3.59% of the total wax. The highest concentration of esters was observed in ‘Abbe Fetel’ (P. communis, 101.63 μg/cm2), whereas the lowest was detected in ‘Huagai’ (P. ussuriensis, 9.34 μg/cm2), and the average concentration was 36.77 μg/cm2 (Supplementary Figure S2K). On the species level, the ester concentrations were the highest in P. communis (75.01 μg/cm2) and the lowest in P. sinkiangensis (21.78 μg/cm2) (Supplementary Figure S2L). It is noteworthy that 1-monopalmitin and 1-monostearin were the only two glycerides compounds simultaneously detected in all 35 pear cultivars in this study (Supplementary Table S1). Glycerides were interesting ester compounds which have been rarely detected in waxes, till now, they have only been detected in the cuticular waxes of potato tuber and olives fruits (Vichi et al., 2016; Guo and Jetter, 2017). In addition, the epicuticular wax of fruits, esters were also detected as being the smallest proportion of the total wax in many species, including plums (8.2%) (Ismail et al., 1977), grapes (<5.1%) (Casado and Heredia, 1999) and apple fruits (1.39–4.85%) (Veraverbeke et al., 2001), while esters were not detected in blueberry (Chu et al., 2017) or peach fruits (Belge et al., 2014).
Terpenoids
In addition to aliphatic compounds, a total of 23 terpenoid compounds, accounting for 11.80% of the total wax, were detected in the epicuticular waxes of the 35 pear cultivars. The highest concentration of terpenoids was observed in ‘Jinfeng’ (hybrid cultivars, 514.02 μg/cm2), whereas the lowest was detected in ‘Red Clapp Favorite’ (P. communis, 14.96 μg/cm2), and the average concentration was 36.77 μg/cm2 (Supplementary Figure S2G). On the species level, the terpenoids were highest in hybrid cultivars (165.91 μg/cm2), followed by P. ussuriensis (118.62 μg/cm2), P. bretschneideri (115.77 μg/cm2), P. sinkiangensis (76.25 μg/cm2) and P. communis (62.58 μg/cm2) and the lowest in P. pyrifolia (26.89 μg/cm2) (Supplementary Figure S2H).
Terpenoids was the predominant component of epicuticular wax in many species, such as the blueberry (64.2%) (Chu et al., 2017), grape (34–49%) (Pensec et al., 2014), peach (44.05–51.92%) (Belge et al., 2014) and sweet cherry (75.6%) (Peschel et al., 2007). Similar to the fruit wax of the tomato (Bauer et al., 2004), grapefruit (Nordby and McDonald, 1994) and citrus (Wang et al., 2014) and the leaf wax of eucalyptus (Viana et al., 2010), we detected lanosterol, farnesene, amyrin, lupeol, squalene, sitosterol and urs-12-en-28-al in the epicuticular wax of pear fruits (Supplementary Table S1). It has been reported that the accumulation of farnesene in the cuticular waxes of apple was the significant cause of greasiness (Christeller and Roughan, 2016). Several certain pear cultivars, such as ‘Yuluxiang’, ‘Kuerle’ and ‘Hongxiangsu’, developing a greasy surface, have accumulated more fluid wax constituents, which were mainly of farnesene. Although ursolic acids and oleanolic acids are the prominent terpenoid components in the cuticular waxes of apple (32.03–69.8%) (Belding et al., 1998), blueberry (16.4–26.0%) (Chu et al., 2017), peach (17.21–29.19%) (Belge et al., 2014), plum (1.0–5.4%) (Ismail et al., 1977), and sweet cherry (7.5–60.0%) (Peschel et al., 2007), they were not detected in epicuticular wax of pear fruits. In addition to the common terpenoid compounds, the pear fruits also contained specific compounds, such as 20α-dihydro pregnenolone (0–7.77 μg/cm2) (Supplementary Table S1).
Terpenoids are of significant importance for maintaining the mechanical strength of the cuticular membrane in persimmon fruit, as well as for defending against biotic and abiotic stress, delaying fruit senescence and being involved in important biological activities. Here, we detected four types of tocopherols in 30 cultivated pear species (except ‘Hongnanguo’, ‘Huangguan’, ‘Nanguo’, ‘Docteur Jules Guyot’ and ‘Kousui’), including delta-tocopherol, beta-tocopherol, gamma-tocopherol and alpha-tocopherol (Supplementary Table S1). As promising sources of biological activities, all four types of tocopherols were simultaneously detected in the mature fruits of ‘Xizilü.’ Tocopherol plays important roles in inhibiting the growth of pathogens and protecting fruits against biotic stresses, and squalene may extend the storage time by removing free radicals and enhancing oxygen loading in citrus fruits (Wang et al., 2014; Wu et al., 2017). Tocopherol and squalene concentrations were 0–81.07 μg/cm2 and 0–7.34 μg/cm2 in the epicuticular waxes of the 35 pear cultivars (Supplementary Table S1). Prior studies have noted that tocopherol and squalene can lead to enhanced resistance to Alternaria rot in pear fruits (Wu et al., 2017).
Crystal Morphology
To elucidate the crystal morphology of the 35 pear cultivars, wax structures were detected by FESEM. Interestingly, the wax morphology showed various amorphous structures in different cultivars, including crystalline plates, irregular ovate crystals, platelets and rodlets with wax crystals (Figure 3 and Supplementary Figures S4, S5). For example, the mature fruits of ‘Qiubai’, ‘Kuerle’, ‘Clapp Favorite’ and ‘Jinfeng’ were covered by wax with irregular ovate crystals (Figures 3D1–D4 and Supplementary Figures S4C1–C4,I1–I4, S5I1–I4), whereas the fruit wax of ‘Chili’, ‘Eli’, ‘Hongnanguo’, ‘Balixiang’, ‘Lüamute’, ‘Abbe Fetel’, ‘Xueqing’ and ‘Yuluxiang’ were composed of numerous transversely polygonal rodlets and platelet crystals (>Figures 3A1–A4 and Supplementary Figures S4A1–A4,G1–G4,H1–H4,K1–K4, S5C1–C4,L1–L4,O1–O4), and glossy transversely polygonal rodlet wax covered the fruit surfaces of the cultivars including ‘Zhongli No. 2’ and ‘Pingguoli’ (Figures 3G1–G4 and Supplementary Figures S5D1–D4). The mature fruits of ‘Kucheamute’ and ‘Hongxiangsu’ were covered by wax crystals with vertically polygonal rodlets with numerous platelets (Figures 3C1–C4,G1–G4), and ‘Nanguoli,’ ‘Huagai’ and ‘Xizilü’ were covered by wax crystals with glossy vertically polygonal rodlets (Figures 3B1–B4 and Supplementary Figures S4E1–E4,S5K1–K4). Remarkably, on the fruit surfaces of ‘Zhongli No. 2’, ‘Eli’, ‘Abbe Fetel’, ‘Bartlett Max Red’ and ‘Yuluxiang’, these structures separated from the wax layer, producing cracks and discontinuity in the outer layer of wax (Figures 3G1–G4 and Supplementary Figures S4A1–A4, S5C1–C4,D1–D4,O1–O4).
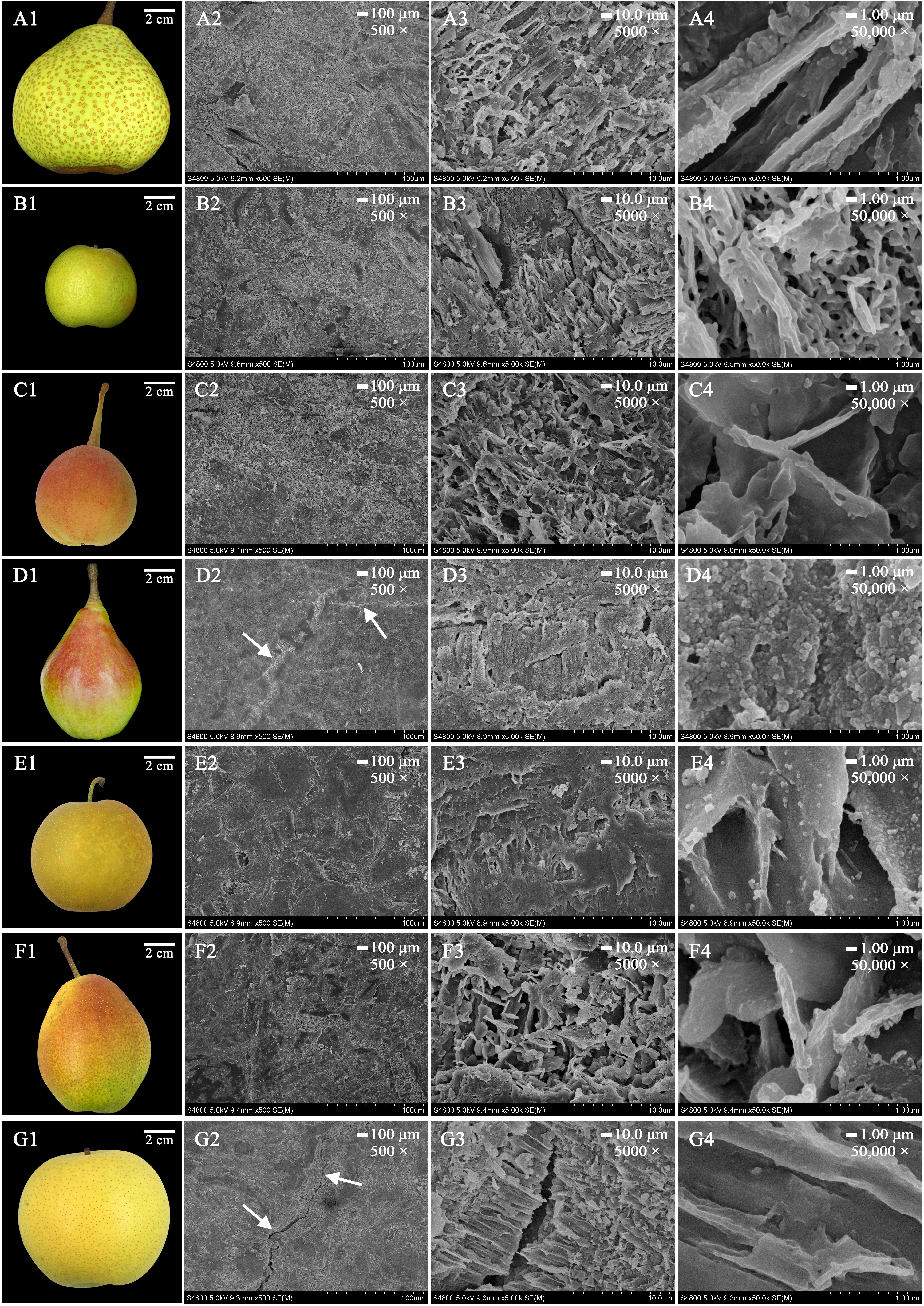
FIGURE 3. The morphology of mature fruits and the magnification series of the FESEM images of epicuticular wax in seven pear cultivars. (A1–G1) Are mature fruits of ‘Chili’, ‘Nanguoli’, ‘Kucheamute’, ‘Clapp Favorite’, ‘Shinseiki’, ‘Hongxiangsu’ and ‘Zhongli No. 2’, respectively. Scale bars represent 100 μm (magnification 500×) in (A2–G2), 10.0 μm (magnification 5000×) in (A3–G3) and 1.00 μm (magnification 50,000×) in (A4–G4). The white arrow denotes the most prominent wax crack.
It is generally believed that the various epicuticular wax crystal types, such as rodlets, tubules and platelets, deriving from self-assembly processes, were mainly based on their different chemical compositions (Chu et al., 2017). It has been reported that the tubule-shaped wax crystal is mainly determined by higher proportions of secondary alcohol tubes and β-diketone wax components in blueberry fruits (Chu et al., 2017), and the platelet wax crystals are caused by a higher proportion of aldehydes and alkanes in the epicuticular waxes of ‘Newhall’ navel orange fruits (Wang et al., 2014). However, it seems that similar wax structures can be formed by different chemical compositions in different species. For example, platelets are the most common wax structures, and wax platelets in Sedum rupestre are characterized by a high amount of triterpenoids, whereas platelets found in the Poaceae (e.g., Triticum, Zea) are generally dominated by primary alcohols (Stevens et al., 1995; Koch et al., 2006). However, many diverse structural and intermediate forms, environmental effects, and erosion of the waxes lead to undetermined shapes (Koch and Ensikat, 2008). Alkanes and primary alcohols were the dominant compounds of the epicuticular wax of pear fruits. The epicuticular wax had an amorphous structure in 35 pear cultivars, which makes classification of the pear fruits unclear. This denotes that wax structures in pear species are not only based on their dominant chemical composition but also influenced by other factors. It has been reported that wax structures on plant surfaces could be influenced by the molecular order (crystallinity) and orientation of the polar groups (polarity) of cutin, and the resulting template effects of the substrates could be used to influence the orientation and preferred direction of crystal growth (Koch et al., 2006). Given the present lack of knowledge about the molecular order and polarity of the cutin network, the factors contributing to the growth of wax structures on the cuticles of pear fruits still need further study.
The Cluster Analysis of the 35 Pear Cultivars
Prior studies have noted that various chemical compositions of epicuticular wax, such as alkanes (Li et al., 2013), terpenes (Medina et al., 2006), flavonoids (Essokne et al., 2012), and fatty acids (Velasco and Goffman, 1999), could be a useful taxonomic marker for classifying the family, genus or species in higher plants and can reflect both ecological and genetic relationships. To elucidate epicuticular wax relationships among 35 pear cultivars, the concentrations of 146 epicuticular wax compounds found in the mature fruits of 35 pear cultivars were clustered through heatmap analysis (Figure 4). The dendrogram generated from the heatmap of wax composition could be divided into two major groups (Figure 4). Group one, including P. bretschneideri, P. ussuriensis, P. communis, P. pyrifolia and the hybrid cultivars, could be further divided into two subgroups (I–II). Subgroup (I) contains all five P. ussuriensis, four P. bretschneideri, seven hybrid cultivars and two P. communis cultivars, whereas the remaining three P. communis, one P. bretschneideri, two P. pyrifolia and four hybrid cultivars were clustered into Subgroup (II). The close genetic relationships between P. bretschneideri, P. ussuriensis, and P. pyrifolia based on our data are consistent with previous results using isozymes, SSR markers and RAPD markers (Bao et al., 2007). Group two was composed of P. sinkiangensis and two hybrid cultivars. All five cultivars of P. sinkiangensis were grouped into the same clade (Figure 4), indicating that the components and contents of their epicuticular wax are similar, and their relationships are phylogenetically close. In addition, ‘Yuluxiang’ and ‘Kuerle’ were clustered into group two (Figure 4). Though ‘Yuluxiang’ is a hybrid cultivar of ‘Kuerle’ (P. ussuriensis) and ‘Xuehua’ (P. bretschneideri), the results of this study indicate that the ‘Yuluxiang’ pear is much more phylogenetically close to the P. sinkiangensis from the hereditary perspective of epicuticular wax. The chemical composition of two major groups (group one and two) and two subgroups (I–II) were compared with t-test. We found that the statistical significant number have 60 and 62 according to two major groups and two subgroups, respectively (Supplementary Table S3).
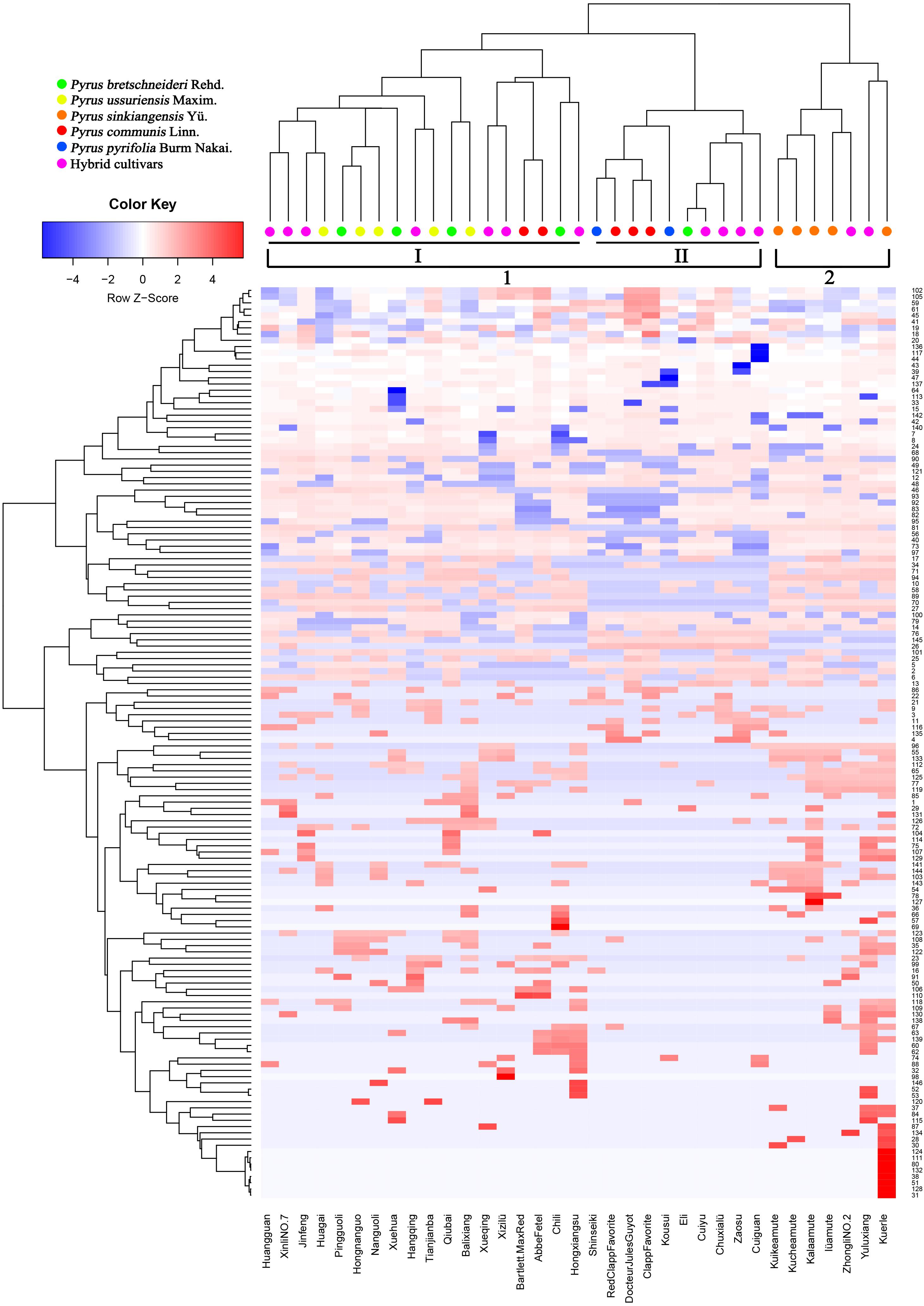
FIGURE 4. Heatmap and clustering of 146 wax components in 35 pear cultivars. Colors indicate chemical composition levels. The numbers of each row represent the wax component numbers corresponding to the accession numbers in Supplementary Table S1. Each column represents a pear cultivar.
Principal Component Analysis of Wax Compounds
The seven classes of epicuticular wax compounds (alkanes, primary alcohols, fatty acids, aldehydes, esters, terpenoids, and others) found in 35 pear cultivars were analyzed through principal component analysis (PCA). The scatter plot of the scores of the PCs of 35 pear cultivars and the corresponding loadings plot of epicuticular wax compounds are shown in Figure 5. PCA1 (47.12%), PCA2 (16.65%) and PCA3 (16.03%) accounted for 79.80% of the total variance, which was high enough to represent all the variables (Figure 5). The 35 pear cultivars could be divided into three groups on the basis of the relationships between cultivars (scores) and their seven classes of wax compounds (loadings). Group one was on the positive side of PCA1 with the longest distance and on the negative side of PCA3 and included cultivars ‘Jinfeng’, ‘Tianjianba’ and ‘Clapp Favorite’, which were characterized by high concentrations of alkanes (Figure 5AI). Cultivars on the positive side of PCA2 with the longest distance formed the second group and contained five pear cultivars (‘Kousui’, ‘Docteur Jules Guyot’, ‘Shinseiki’, ‘Bartlett MaxRed’ and ‘Abbe Fetel’); these cultivars were characterized as cultivars with lower concentrations of terpenoids (Figure 5AII). The third group contained all the other cultivars, and there was no consistency with respect to the composition of wax compounds (Figure 5AIII).
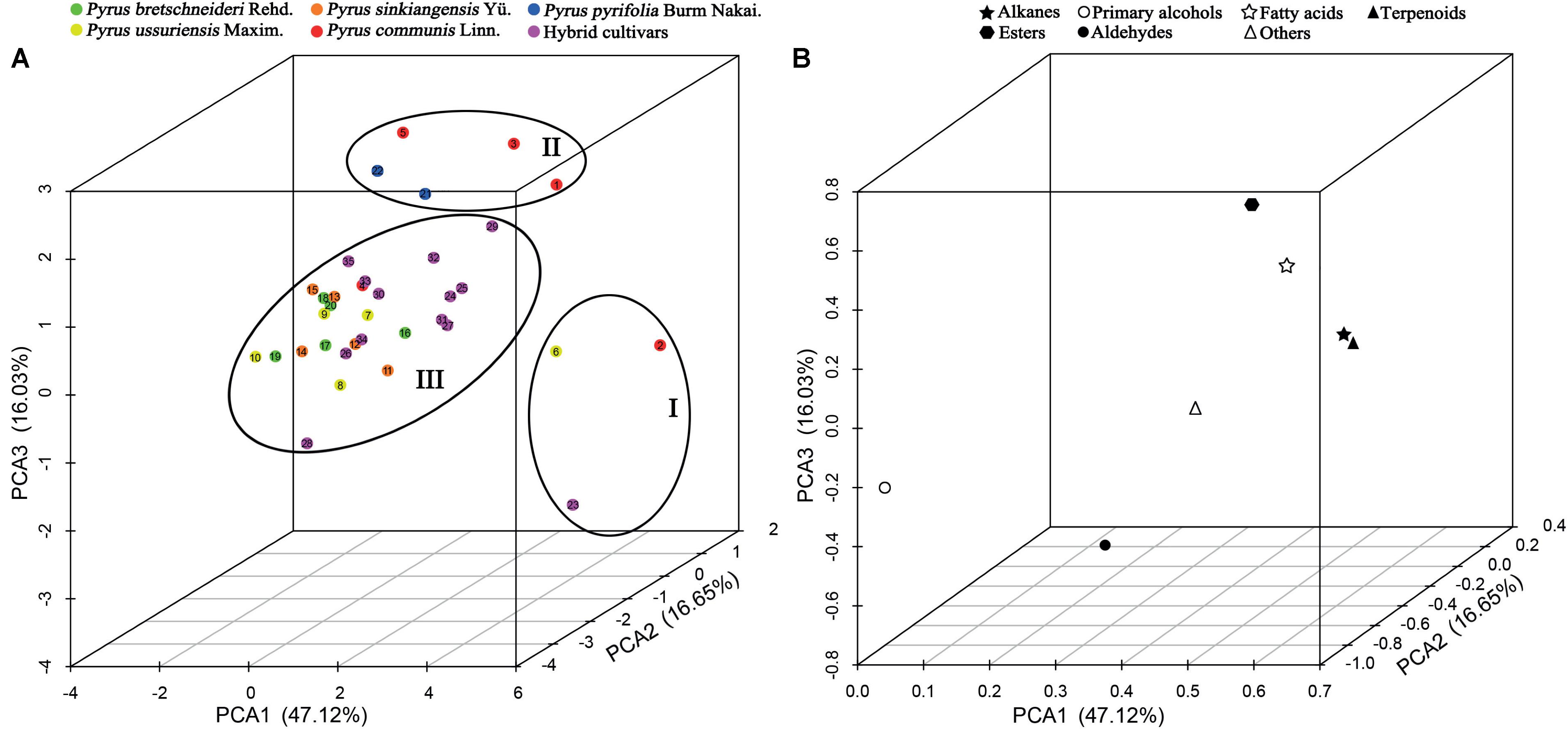
FIGURE 5. PCA of seven main classes of wax compounds in 35 pear cultivars. (A) Scatter plot of PCA scores and (B) loadings plot of the PCA. The numbers in (A) correspond to the sample numbers given in Table 1. Percentages in parentheses are the variance of each component.
To further understand how pear cultivars were affected by cuticular wax compositions, we performed the PCA analysis using 146 wax compounds. Compared with the former PCA analysis using seven classes of epicuticular wax compounds, different similar result showed that the PCA1 (64.45%), PCA2 (8.12%) and PCA3 (6.40%), explained 78.97% of the variation (Supplementary Figure S6). However, the 35 pear cultivars could be divided into five groups based on the relationships between cultivars (scores) and their 146 wax compounds (loadings). ‘Clapp Favorite’ formed the first group, which were characterized by high concentrations of octacosane. Group two contained six pear cultivars (‘Bartlett Max Red’, ‘Hongnanguo’, ‘Nanguoli’, ‘Huagai’, ‘Xuehuali’ and ‘Zhongli No. 2’), which were characterized with high concentrations of triacontane-1,30-diol. Group three including two cultivars (‘Eli’ and ‘Huangguan’), which were mainly characterized by high concentrations of triacontane. ‘Abbe Fetel’, ‘Hongxiangsu’ and ‘Xueqing’ characterized with lack of triacontane and hentriacontane were clustered into group four. The fifth group contained all the other cultivars, and these cultivars were characterized with high concentrations of hentriacontane (Supplementary Figure S6A).
Conclusion
The total wax content of mature fruits was highly variable among 35 pear cultivars, ranging from a high of 2.44 ± 0.41 mg/cm2 (‘Docteur Jules Guyot’, P. communis) to a low of 0.46 ± 0.03 mg/cm2 (‘Huagai’, P. ussuriensis). A total of 146 epicuticular wax compounds were detected through GC-MS analysis. Although the composition and concentration of wax compounds varied greatly, alkanes (422.73 ± 205.79 μg/cm2, 40.72 ± 12.03%), primary alcohols (271.06 ± 210.96 μg/cm2, 24.48 ± 10.57%) and terpenoids (116.39 ± 98.72 μg/cm2, 11.80 ± 8.76%) were the predominant wax compounds in all 35 pear cultivars. FESEM images showed that epicuticular wax crystals of the 35 pear cultivars were mostly amorphous structures, including crystalline plates, irregular ovate crystals, platelets and rodlets. The cluster analysis of wax composition and concentration revealed that the Pyrus bretschneideri cultivars were grouped much closer to Pyrus pyrifolia and Pyrus ussuriensis, whereas Pyrus sinkiangensis were clustered into a distant separate group. The 35 pear cultivars could be divided into three groups and five groups based on the principal component analysis (PCA) using seven main classes of epicuticular wax compounds and all of the 146 wax compounds, respectively. Taken together, ‘Docteur Jules Guyot’ (P. communis), ‘Tianjianba’ (P. ussuriensis), ‘Kuerle’ (Pyrus sinkiangensis Yü.), ‘Qiubai’ (P. bretschneideri) and ‘Shinseiki’ (P. pyrifolia) should be the most representative parent cultivars for the future pear breeding with higher wax concentrations.
Author Contributions
HY and SZ: conceived and designed the experiments. XW, YC, GW, and PC: performed the experiments. XW and YC: analyzed the data. ZS and KQ: contributed reagents, materials, analysis tools or data. XW, XQ, HY, and SZ: wrote the paper.
Funding
This study was supported by the National Natural Science Foundation of China (31701890), Fundamental Research Funds for the Central Universities (KJQN201818), the Key Project for New Agricultural Cultivar Breeding in Zhejiang Province, China (2016C02052-4), Natural Science Foundation of Jiangsu Province in China (BK20160715), and China Postdoctoral Science Foundation funded project (2015M570456).
Conflict of Interest Statement
The authors declare that the research was conducted in the absence of any commercial or financial relationships that could be construed as a potential conflict of interest.
Acknowledgments
We would like to thank many contributors of experimental materials, including Qingyu Li (Yantai Academy of Agricultural Sciences, Yantai, Shandong), Shoufeng Sha (Liaoning Institute of Fruit Sciences, Xiongyue, Liaoning), Yongjie Liu (Research Center of Korla Fragrant Pear, Korla, Xinjiang), Mudan Bai (Pomology Institute, Shanxi Academy of Agricultural Sciences, Taigu, Shanxi), Yingtao Wang (Institute of Fruit Tree Research, Hebei Academy of Agriculture and Forestry Sciences, Shijiazhuang, Hebei), Yufen Cao (National Germplasm Repository of Pear in the Research Institute of Pomology, Chinese Academy of Agricultural Sciences (CAAS), Xingcheng, Liaoning Province), and Long Wang (Zhengzhou Fruit Research Institute, CAAS, Zhengzhou, Henan, China).
Supplementary Material
The Supplementary Material for this article can be found online at: https://www.frontiersin.org/articles/10.3389/fpls.2018.00679/full#supplementary-material
References
Baker, E. A., Procopiou, J., and Hunt, G. M. (1975). The cuticles of Citrus species. Composition of leaf and fruit waxes. J. Sci. Food Agric. 26, 1093–1101. doi: 10.1002/jsfa.2740260807
Bao, L., Chen, K., Zhang, D., Cao, Y., Yamamoto, T., and Teng, Y. (2007). Genetic diversity and similarity of pear (Pyrus L.) cultivars native to East Asia revealed by SSR (simple sequence repeat) markers. Genet. Resour. Crop Evol. 54, 959–971. doi: 10.1007/s10722-006-9152-y
Bauer, S., Schulte, E., and Thier, H.-P. (2004). Composition of the surface wax from tomatoes - II. Quantification of the components at the ripe red stage and during ripening. Eur. Food Res. Technol. 219, 487–491. doi: 10.1007/s00217-004-0944-z
Bauer, S., Schulte, E., and Thier, H.-P. (2005). Composition of the surface waxes from bell pepper and eggplant. Eur. Food Res. Technol. 220, 5–10. doi: 10.1007/s00217-004-1046-7
Belding, R. D., Blankenship, S. M., Young, E., and Leidy, R. B. (1998). Composition and variability of epicuticular waxes in apple cultivars. J. Am. Soc. Hortic. Sci. 123, 348–356.
Belge, B., Llovera, M., Comabella, E., Graell, J., and Lara, I. (2014). Fruit cuticle composition of a melting and a nonmelting peach cultivar. J. Agric. Food Chem. 62, 3488–3495. doi: 10.1021/jf5003528
Bianchi, G., Murelli, C., and Vlahov, G. (1992). Surface waxes from olive fruits. Phytochemistry 31, 3503–3506. doi: 10.1016/0031-9422(92)83716-C
Casado, C. G., and Heredia, A. (1999). Structure and dynamics of reconstituted cuticular waxes of grape berry cuticle (Vitis vinifera L.). J. Exp. Bot. 50, 175–182. doi: 10.1093/jxb/50.331.175
Chen, S.-J., Li, Y.-C., Bi, Y., Yan, Y., Ge, Y.-H., and Yi, W. (2014). Solvent effects on the ultrastructure and chemical composition of cuticular wax and its potential bioactive role against Alternaria alternata in Pingguoli pear. J. Integr. Agric. 13, 1137–1145. doi: 10.1016/S2095-3119(13)60374-3
Christeller, J. T., and Roughan, P. G. (2016). The novel esters farnesyl oleate and farnesyl linoleate are prominent in the surface wax of greasy apple fruit. N. Z. J. Crop Hortic. Sci. 44, 164–170. doi: 10.1080/01140671.2016.1152979
Chu, W., Gao, H., Cao, S., Fang, X., Chen, H., and Xiao, S. (2017). Composition and morphology of cuticular wax in blueberry (Vaccinium spp.) fruits. Food Chem. 219, 436–442. doi: 10.1016/j.foodchem.2016.09.186
Chu, W., Gao, H., Chen, H., Fang, X., and Zheng, Y. (2018). Effects of cuticular wax on the postharvest quality of blueberry fruit. Food Chem. 239, 68–74. doi: 10.1016/j.foodchem.2017.06.024
de Araújo Silva, M. M., Cursino dos Santos, D. Y. A., Melo-de-Pinna, G. F. A., Câmara, T. D. J. R., and Oliveira, A. F. M. (2017). Chemical composition and ultrastructure of the foliar cuticular wax of two Brazilian cultivars of castor bean (Ricinus communis L.). Ind. Crops Prod. 95, 558–563. doi: 10.1016/j.indcrop.2016.11.010
Essokne, R. S., Grayer, R. J., Porter, E., Kite, G. C., Simmonds, M. S., and Jury, S. L. (2012). Flavonoids as chemosystematic markers for the genus Adenocarpus. Biochem. Syst. Ecol. 42, 49–58. doi: 10.1016/j.bse.2011.12.023
Glenn, G., Rom, C., Rasmussen, H., and Poovaiah, B. (1990). Influence of cuticular structure on the appearance of artificially waxed ‘Delicious’ apple fruit. Sci. Hortic. 42, 289–297. doi: 10.1016/0304-4238(90)90052-G
Guo, Y., and Jetter, R. (2017). Comparative analyses of cuticular waxes on various organs of potato (Solanum tuberosum L.). J. Agric. Food Chem. 65, 3926–3933. doi: 10.1021/acs.jafc.7b00818
Hao, S., Ma, Y., Zhao, S., Ji, Q., Zhang, K., Yang, M., et al. (2017). McWRI1, a transcription factor of the AP2/SHEN family, regulates the biosynthesis of the cuticular waxes on the apple fruit surface under low temperature[J]. PLoS One 12:e0186996. doi: 10.1371/journal.pone.0186996
Ismail, H. M., Brown, G. A., Tucknott, O. G., Holloway, P. J., and Williams, A. A. (1977). Nonanal in epicuticular wax of golden egg plums (Prunus domestica). Phytochemistry 16, 769–770. doi: 10.1016/S0031-9422(00)89254-7
Jetter, R., and Riederer, M. (2016). Localization of the transpiration barrier in the epi-and intracuticular waxes of eight plant species: water transport resistances are associated with fatty acyl rather than alicyclic components. Plant Physiol. 170, 921–934. doi: 10.1104/pp.15.01699
Koch, K., Barthlott, W., Koch, S., Hommes, A., Wandelt, K., Mamdouh, W., et al. (2006). Structural analysis of wheat wax (Triticum aestivum, cv ‘Naturastar’ L.): from the molecular level to three dimensional crystals. Planta 223, 258–270. doi: 10.1007/s00425-005-0081-3
Koch, K., and Ensikat, H.-J. (2008). The hydrophobic coatings of plant surfaces: epicuticular wax crystals and their morphologies, crystallinity and molecular self-assembly. Micron 39, 759–772. doi: 10.1016/j.micron.2007.11.010
Kolattukudy, P. (1996). “Biosynthetic pathways of cutin and waxes, and their sensitivity to environmental stresses,” in Plant Cuticles: An Integrated Functional Approach, ed. G. Kerstiens (Oxford: BIOS Scientific Publishers Ltd), 83–108. doi: 10.1016/j.micron.2007.11.010
Lanza, B., and Di Serio, M. G. (2015). SEM characterization of olive (Olea europaea L.) fruit epicuticular waxes and epicarp. Sci. Hortic. 191, 49–56. doi: 10.1016/j.scienta.2015.04.033
Leide, J., Hildebrandt, U., Reussing, K., Riederer, M., and Vogg, G. (2007). The developmental pattern of tomato fruit wax accumulation and its impact on cuticular transpiration barrier properties: effects of a deficiency in a β-ketoacyl-coenzyme A synthase (LeCER6). Plant Physiol. 144, 1667–1679. doi: 10.1104/pp.107.099481
Li, J., Huang, J., Ge, J., Huang, X., and Xie, S. (2013). Chemotaxonomic significance of n-alkane distributions from leaf wax in genus of Sinojackia species (Styracaceae). Biochem. Syst. Ecol. 49, 30–36. doi: 10.1016/j.bse.2013.02.001
Li, Y., Yin, Y., Chen, S., Bi, Y., and Ge, Y. (2014). Chemical composition of cuticular waxes during fruit development of Pingguoli pear and their potential role on early events of Alternaria alternata infection. Funct. Plant Biol. 41, 313–320. doi: 10.1071/FP13184
Liu, L., Chen, C.-X., Zhu, Y.-F., Xue, L., Liu, Q.-W., Qi, K.-J., et al. (2016). Maternal inheritance has impact on organic acid content in progeny of pear (Pyrus spp.) fruit. Euphytica 209, 305–321. doi: 10.1007/s10681-015-1627-5
McDonald, R. E., Nordby, H. E., and McCollum, T. G. (1993). Epicuticular wax morphology and composition are related to grapefruit chilling injury. HortScience 28, 311–312.
Medina, E., Aguiar, G., Gomez, M., Aranda, J., Medina, J., and Winter, K. (2006). Taxonomic significance of the epicuticular wax composition in species of the genus Clusia from Panama. Biochem. Syst. Ecol. 34, 319–326. doi: 10.1016/j.bse.2005.10.009
Murtagh, F., and Legendre, P. (2014). Ward’s hierarchical agglomerative clustering method: which algorithms implement Ward’s criterion? J. Classif. 31, 274–295. doi: 10.1007/s00357-014-9161-z
Nordby, H. E., and McDonald, R. E. (1994). Friedelin, the major component of grapefruit epicuticular wax. J. Agric. Food Chem. 42, 708–713. doi: 10.1021/jf00039a021
Parsons, E. P., Popopvsky, S., Lohrey, G. T., Lü, S., Alkalai-Tuvia, S., Perzelan, Y., et al. (2012). Fruit cuticle lipid composition and fruit post-harvest water loss in an advanced backcross generation of pepper (Capsicum sp.). Physiol. Plant. 146, 15–25. doi: 10.1111/j.1399-3054.2012.01592.x
Pensec, F., Paczkowski, C., Grabarczyk, M., Wozìniak, A., Beìnard-Gellon, M., Bertsch, C., et al. (2014). Changes in the triterpenoid content of cuticular waxes during fruit ripening of eight grape (Vitis vinifera) cultivars grown in the Upper Rhine Valley. J. Agric. Food Chem. 62, 7998–8007. doi: 10.1021/jf502033s
Peschel, S., Franke, R., Schreiber, L., and Knoche, M. (2007). Composition of the cuticle of developing sweet cherry fruit. Phytochemistry 68, 1017–1025. doi: 10.1016/j.phytochem.2007.01.008
Racovita, R. C., Peng, C., Awakawa, T., Abe, I., and Jetter, R. (2015). Very-long-chain 3-hydroxy fatty acids, 3-hydroxy fatty acid methyl esters and 2-alkanols from cuticular waxes of Aloe arborescens leaves. Phytochemistry 113, 183–194. doi: 10.1016/j.phytochem.2014.08.005
Sala, J. M. (2000). Content, chemical composition and morphology of epicuticular wax of Fortune mandarin fruits in relation to peel pitting. J. Sci. Food Agric. 80, 1887–1894. doi: 10.1002/1097-0010(200010)80:13<1887::AID-JSFA730>3.0.CO;2-W
Stevens, J. F., Hart, H. T., and Wollenweber, E. (1995). The systematic and evolutionary significance of exudate flavonoids in Aeonium. Phytochemistry 39, 805–813. doi: 10.1016/0031-9422(93)00074-P
Teece, M. A., Zengeya, T., Volk, T. A., and Smart, L. B. (2008). Cuticular wax composition of Salix varieties in relation to biomass productivity. Phytochemistry 69, 396–402. doi: 10.1016/j.phytochem.2007.08.015
Tsubaki, S., Ozaki, Y., Yonemori, K., and Azuma, J.-I. (2012). Mechanical properties of fruit-cuticular membranes isolated from 27 cultivars of Diospyros kaki Thunb. Food Chem. 132, 2135–2139. doi: 10.1016/j.foodchem.2011.12.039
Velasco, L., and Goffman, F. D. (1999). Chemotaxonomic significance of fatty acids and tocopherols in Boraginaceae. Phytochemistry 52, 423–426. doi: 10.1016/S0031-9422(99)00203-4
Veraverbeke, E. A., Lammertyn, J., Saevels, S., and Nicolaï, B. M. (2001). Changes in chemical wax composition of three different apple (Malus domestica Borkh.) cultivars during storage. Postharvest Biol. Technol. 23, 197–208. doi: 10.1016/S0925-5214(01)00128-4
Viana, R. G., Tuffi Santos, L. D., Demuner, A. J., Ferreira, F. A., Ferreira, L. R., Ferreira, E. A., et al. (2010). Quantification and chemical composition of epicuticular wax of eucalyptus leaves. Planta Daninha 28, 753–758. doi: 10.1590/S0100-83582010000400007
Vichi, S., Corteìs-Francisco, N., Caixach, J., Barrios, G., Mateu, J., Ninot, A., et al. (2016). Epicuticular wax in developing olives (Olea europaea) is highly dependent upon cultivar and fruit ripeness. J. Agric. Food Chem. 64, 5985–5994. doi: 10.1021/acs.jafc.6b02494
Wang, J., Hao, H., Liu, R., Ma, Q., Xu, J., Chen, F., et al. (2014). Comparative analysis of surface wax in mature fruits between Satsuma mandarin (Citrus unshiu) and ‘Newhall’ navel orange (Citrus sinensis) from the perspective of crystal morphology, chemical composition and key gene expression. Food Chem. 153, 177–185. doi: 10.1016/j.foodchem.2013.12.021
Wang, J., Sun, L., Xie, L., He, Y., Luo, T., Sheng, L., et al. (2016). Regulation of cuticle formation during fruit development and ripening in ‘Newhall’ navel orange (Citrus sinensis Osbeck) revealed by transcriptomic and metabolomic profiling. Plant Sci. 243, 131–144. doi: 10.1016/j.plantsci.2015.12.010
Wang, W., Liu, X., Gai, X., Ren, J., Liu, X., Cai, Y., et al. (2015). Cucumis sativus L. WAX2 plays a pivotal role in wax biosynthesis, influencing pollen fertility and plant biotic and abiotic stress responses. Plant Cell Physiol. 56, 1339–1354. doi: 10.1093/pcp/pcv052
Wang, Y., Wang, J., Chai, G., Li, C., Hu, Y., Chen, X., et al. (2015). Developmental changes in composition and morphology of cuticular waxes on leaves and spikes of glossy and glaucous wheat (Triticum aestivum L.). PLoS One 10:e0141239. doi: 10.1371/journal.pone.0141239
Wu, J., Li, L.-T., Li, M., Khan, M. A., Li, X.-G., Chen, H., et al. (2014). High-density genetic linkage map construction and identification of fruit-related QTLs in pear using SNP and SSR markers. J. Exp. Bot. 65, 5771–5781. doi: 10.1093/jxb/eru311
Wu, X., Yin, H., Chen, Y., Li, L., Wang, Y., Hao, P., et al. (2017). Chemical composition, crystal morphology and key gene expression of cuticular waxes of Asian pears at harvest and after storage. Postharvest Biol. Technol. 132, 71–80. doi: 10.1016/j.postharvbio.2017.05.007
Yin, Y., Bi, Y., Chen, S., Li, Y., Wang, Y., Ge, Y., et al. (2011). Chemical composition and antifungal activity of cuticular wax isolated from asian pear fruit (cv. Pingguoli). Sci. Hort. 129, 577–582. doi: 10.1016/j.scienta.2011.04.028
Zhang, J.-Y., Broeckling, C. D., Sumner, L. W., and Wang, Z.-Y. (2007). Heterologous expression of two Medicago truncatula putative ERF transcription factor genes, WXP1 and WXP2, in Arabidopsis led to increased leaf wax accumulation and improved drought tolerance, but differential response in freezing tolerance. Plant Mol. Biol. 64, 265–278. doi: 10.1007/s11103-007-9150-2
Keywords: wax, GC-MS, crystal morphology, PCA, pears
Citation: Wu X, Yin H, Shi Z, Chen Y, Qi K, Qiao X, Wang G, Cao P and Zhang S (2018) Chemical Composition and Crystal Morphology of Epicuticular Wax in Mature Fruits of 35 Pear (Pyrus spp.) Cultivars. Front. Plant Sci. 9:679. doi: 10.3389/fpls.2018.00679
Received: 04 January 2018; Accepted: 03 May 2018;
Published: 23 May 2018.
Edited by:
Guusje Bonnema, Wageningen University & Research, NetherlandsReviewed by:
Pinarosa Avato, Università degli Studi di Bari Aldo Moro, ItalyBrian Farneti, Fondazione Edmund Mach, Italy
Copyright © 2018 Wu, Yin, Shi, Chen, Qi, Qiao, Wang, Cao and Zhang. This is an open-access article distributed under the terms of the Creative Commons Attribution License (CC BY). The use, distribution or reproduction in other forums is permitted, provided the original author(s) and the copyright owner are credited and that the original publication in this journal is cited, in accordance with accepted academic practice. No use, distribution or reproduction is permitted which does not comply with these terms.
*Correspondence: Hao Yin, yinhao85@gmail.com Shaoling Zhang, slzhang@njau.edu.cn
†These authors have contributed equally to this work.