- Key Laboratory of Crop Germplasm Resources and Utilization, Ministry of Agriculture, National Key Facility for Crop Gene Resources and Genetic Improvement, Institute of Crop Sciences, Chinese Academy of Agricultural Sciences, Beijing, China
The heading date is critical in determining the adaptability of plants to specific natural environments. Molecular characterization of the wheat genes that regulate heading not only enhances our understanding of the mechanisms underlying wheat heading regulation but also benefits wheat breeding programs by improving heading phenotypes. In this study, we characterized a late heading date mutant, m605, obtained by ethyl methanesulfonate (EMS) mutation. Compared with its wild-type parent, YZ4110, m605 was at least 7 days late in heading when sown in autumn. This late heading trait was controlled by a single recessive gene named TaHdm605. Genetic mapping located the TaHdm605 locus between the molecular markers cfd152 and barc42 on chromosome 3DL using publicly available markers and then further mapped this locus to a 1.86 Mb physical genomic region containing 26 predicted genes. This fine genetic and physical mapping will be helpful for the future map-based cloning of TaHdm605 and for breeders seeking to engineer changes in the wheat heading date trait.
Introduction
Heading date (flowering time) in crops is associated with the timing of the floral transition and is one of the most important agronomic traits that determines the distribution and regional adaptability of plants, thereby affecting crop production. Plants have evolved multiple genetic pathways that integrate both internal signals and extrinsic stimuli to adapt flowering time to different environmental conditions. Isolation and functional study of heading related genes will provide better understanding of the genetic pathways that control the flowering time of plants and could offer effective strategies for engineering high-yield varieties that can adapt to different climate conditions and changing environments (Jung and Muller, 2009).
Wheat (Triticum aestivum L, 2n = 6x = 42, AABBDD) is grown in a wide range of environments all over the world. Suitable heading date is an important determinant that contributes to precise adjustment for seasonal changes, which is important to maximize crop yield. Many studies have been performed to clarify the genetic control of heading date in wheat and have identified the vernalization requirement and photoperiod sensitivity as two main components in the regulation of heading date in wheat.
Vernalization is the requirement for a plant to undergo cold temperatures before flowering. The wheat vernalization requirement is mainly controlled by the VRN1, VRN2, VRN3, and VRN4 genes, and the characterization of these four genes has advanced our understanding of vernalization regulatory pathways (Yan et al., 2004, 2006; Fu et al., 2005; Li and Dubcovsky, 2008; Yoshida et al., 2010; Kippes et al., 2014). VRN1, isolated in diploid wheat using a map-based cloning method, has been shown to share high sequence similarity with the Arabidopsis MADS-box gene AP1/FRUITFULL. The central role of VRN1 in the leaves is to maintain low transcript levels of the flowering repressor gene VRN2 after vernalization and to ultimately regulate timely flowering in the spring (Chen and Dubcovsky, 2012). The VRN2 gene encodes a zinc finger-CCT domain transcription factor and acts as a dominant flowering repressor that can be down-regulated by vernalization (Yan et al., 2004). It has been postulated that the VRN2 protein suppresses the expression of the flowering-integrator gene FT (VRN3), which is a mobile signaling protein that moves from the leaves to the shoot apical meristem to induce flowering. Transgenic analysis in winter wheat indicated that reduced VRN2 expression leads to increased transcription of VRN1 and accelerated flowering (Yan et al., 2004; Dubcovsky et al., 2006; Li et al., 2011; Chen and Dubcovsky, 2012). Further studies have also demonstrated that VRN3 regulates VRN1 transcription through interactions with FDL2. Most importantly, VRN3 is an integrator of vernalization and photoperiod pathways (Yan et al., 2006; Trevaskis et al., 2007; Li and Dubcovsky, 2008). For these three vernalization genes, the combination of the recessive vrn-1 allele, dominant Vrn-2 allele and recessive vrn-3 allele is associated with winter growth habits, and a mutation in any one or more of these three genes results in a variant with a spring growth habit (Tranquilli and Dubcovsky, 2000; Yan et al., 2006). VRN4 has been found only in the D genome (VRN-D4). The detection of significant interactions between VRN-D4 and other four genes controlling vernalization requirement (Vrn-A1, Vrn-B1, Vrn-D1, and Vrn-B3) confirmed that VRN-D4 acts as part of the vernalization pathway and that it is either upstream of or part of the regulatory feedback loop involving the VRN1, VRN2, and VRN3 genes. Subsequent studies have demonstrated that VRN-D4 originated from the insertion of a ∼290 kb region carrying a copy of VRN-A1 from chromosome arm 5AL into the proximal region of chromosome arm 5DS (Kippes et al., 2015).
The photoperiod response in wheat is primarily determined by the three dominant genes Ppd-A1, Ppd-B1, and Ppd-D1, which belong to three homologous loci on chromosomes 2A, 2B, and 2D, respectively. Wheat is a LD plant, and SD conditions can delay its heading time. The Ppd1 gene acts as a regulator that reduces the delay in heading time under SD conditions (Murai et al., 2003). Recent studies have suggested that diverse alleles and epigenetic modification of the Ppd1 genes may contribute to photoperiod sensitivity and affect the heading date in wheat (Distelfeld et al., 2009; Dhillon et al., 2010; Guo et al., 2010; Diaz et al., 2012; Sun et al., 2014). Another flowering pathway called the GI-CO-FT regulation pathway, which was found in both Arabidopsis and rice, has also been identified in the wheat flowering process. Studies have already demonstrated that VRN3 is the wheat FT gene (Yan et al., 2006), and its upstream genes TaGI1 and TaCO were cloned in wheat using the homology cloning method (Nemoto et al., 2003; Zhao et al., 2005).
When wheat cultivars were exposed to an optimal photoperiod and vernalization treatments, there were still differences in flowering time among the wheat accessions, which is usually referred to as earliness per se (Eps) (Slafer, 1996). The Eps-Am1 locus was identified from the Triticum monococcum chromosome 1Am (Bullrich et al., 2002). However, it exhibited a large effect on the heading date and significant epistatic interactions with the photoperiod and vernalization treatments; Eps-Am1 also exhibited a significant interaction with temperature. Moreover, Eps-Am1 affected spike development and spikelet number (Bullrich et al., 2002; Faricelli et al., 2010). Further study identified EARLY FLOWERING 3 (ELF3) as a candidate gene (Alvarez et al., 2016).
Although these studies provide a basic understanding of the control of the flowering process in wheat, the regulatory networks of flowering in wheat and their underlying molecular mechanisms are not well understood. To solve these problems, more wheat heading date genes need to be discovered. In the present study, we report the identification of a novel heading date gene TaHdm605 and illustrate fine mapping of the heading date gene in bread wheat; these results could serve as a framework for map-based cloning and for marker-assisted selection in wheat breeding programs.
Materials and Methods
Plant Materials
The m605 mutant originated from the seeds of the elite wheat variety YZ4110 (vrn-A1, vrn-B1, Vrn-D1, vrn-2, vrn-3, Ppd-A1b, Ppd-B1b, and Ppd-D1a), which was treated with EMS (Zhao et al., 2009). The F2:3 populations derived from m605 crossed with an early heading variety, Chun47 (vrn-A1, vrn-B1, Vrn-D1, Vrn-2, vrn-3, Ppd-A1b, Ppd-B1b, and Ppd-D1a) and wild-type YZ4110, respectively, were used for genetic analysis. The genotypes of the Vrn and Ppd loci of WT YZ4110, the m605 mutant and Chun47 were detected by the primers listed in Supplementary Table S1 (Fu et al., 2005; Yan et al., 2006; Zhu et al., 2011; Nishida et al., 2013). The F2 population derived from the m605 mutant crossed with wild-type YZ4110 was used to test the segregation ratio of the heading date phenotype. The F2 population from the cross of Chun47 × m605 was used to perform fine mapping. Fifteen seeds from the F2 segregating population as well as the parents were planted in 2-m rows spaced 30 cm apart in fields in Beijing (N39°49’, E 116°39’) and Xinxiang (N35°18’, E113°55’), China, between 2010 and 2013. To ensure the accuracy of the results, the phenotypes of the F2 plants were further validated by using the F3 families. F3 families containing 25 seeds from every F2 plants were planted in rows for verifying the phenotypes of the F2 plants in fields in Beijing and Xinxiang between 2011 and 2014. To avoid the damage caused by cold weather during hard winters, the seedlings planted in Beijing were covered with plastic film. For main shoot apex development analysis, the mutant plants of m605 and YZ4110 were grown in greenhouse with vernalized in 4°C for 3 weeks. The room temperature at the greenhouse was maintained at 22°C during the 16-h light period and at 18°C during the 8-h dark period, and the average relative humidity was 75%. The floral primordium samples of m605 and YZ4110 were collected between 9 and 10 AM after 8 and 10 weeks, respectively.
Data Analysis
For the comparison of days to heading and other agronomical traits between YZ4110 and m605, the data of agronomical traits from 20 plants were tested and recorded from 2009 to 2016. One-way analysis of variance was performed in Excel. The F2 population from the cross of Chun47 × m605, plant height, spike length, effective tiller number, grain number per spike, and 1,000-kernel weight of each individual progeny were recorded. The data analysis was conducted using SPSS software. ∗Indicates 0.01 < p < 0.05, ∗∗indicates p < 0.01.
DNA Extraction and Polymerase Chain Reaction (PCR)
Total genomic DNA from the Chun47 × m605 F2 population was isolated from leaves by using a cetyltrimethylammonium bromide (CTAB) method (Saghai-Maroof et al., 1984). PCR was performed in a Veriti thermal cycler (Life Technologies, United States) in 10 μl reactions containing 25 ng of each primer, 30 ng of genomic DNA, and 5 μL of 2 × PCR MasterMix (Tiangen, Beijing, China). The PCR conditions were as follows: denaturation at 94°C for 10 min, followed by 40 cycles of 94°C for 45 s, 55°C for 45 s, and 72°C for 45 s and a final extension for 10 min at 72°C. PCR products were mixed with 2 μl of loading buffer (98% formamide, 10 mM EDTA, 0.25% bromophenol blue, and 0.25% xylene cyanol), separated on 5% denaturing polyacrylamide gels and visualized using silver staining. Silver staining was performed as follows: rinse with water for 10 s, silver stain (1 g/L AgNO3) for 8 min, rinse with water for 10 s, develop for 5–10 min (16 g/L NaOH, 0.75% HCHO), fix for 1 min (7.5 g/L Na2CO3), and rinse with water for 5 min.
Molecular Marker Analysis and Polymorphic Marker Development
F2 plants from the cross of Chun47 and m605 were used to establish early and late bucks for bulked segregant analysis (BSA). The DNAs of the 10 homozygous plants that exhibited early heading dates and late heading dates, based on a validation of the heading date of the F3 family, were pooled in equal quantities to construct an early bulk sample and a late bulk sample, separately. Wheat genomic simple sequence repeat (SSR) markers from the GrainGenes website1, with an average distance of 10 cM between adjacent markers, were used for screening the polymorphisms between the two parents and two bulk samples. The resulting polymorphic markers were used to genotype the F2 populations.
The marker sequences linked to TaHdm605 were used to perform BLAST searches of the Aegilops tauschii genome sequence (Jia et al., 2013) to develop new polymorphic markers. The obtained sequences were used as templates to design primers with Primer32 with the following parameters: amplification product size of 120–600 bp, primer length of 17–24 bp, Tm of 55–65°C with the optimum at 60°C, and GC content of 40–60%. The designed primers were first screened for polymorphisms between the parental lines, as well as the early and late DNA bulks. The chromosome 3DL-specific polymorphic markers were used for genotyping the F2 populations to construct a high-density genetic linkage map.
High-Density Genetic Linkage Map Construction
Chi-squared (χ2) tests for goodness-of-fit were used to evaluate the deviations of the observed data from the theoretically expected segregation ratios. A linkage map with the genetic distance between the markers and the TaHdm605 gene was determined using Mapmaker 3.0, with a logarithm of odds (LOD) score threshold of 3.0 (Lincoln et al., 1992). A genetic map was constructed with the software Mapdraw V2.1 (Liu and Meng, 2003).
Candidate Gene Analysis and Comparative Genomics Analysis of the TaHdm605 Locus Among Different Species
The nearest marker sequences flanking the TaHdm605 gene were used to perform BLAST searches of the wheat chromosome 3DL survey sequences (International Wheat Genome Sequencing Consortium (IWGSC) RefSeq v1.0). Gene annotation for the obtained genomic sequence surrounding the TaHdm605 gene was conducted by using the TriAnnot pipeline3 and BLAST analysis tools from NCBI4. The genes expression pattern was determined by blasting the predicted CDS sequences to WheatEXP5 with an e-value cutoff of 1e-10. The identified gene sequences were used as query sequences to BLAST search the Brachypodium6, rice7 and sorghum8 genome sequences with an e-value cutoff of 1e-10. The orthologous gene pairs among the different species were compared within the homologous genomic regions. The inversion region of the TaHdm605 locus was identified by projecting the genetic markers of A. tauschii onto the Brachypodium and rice chromosomes (Luo et al., 2013).
Results
Phenotype of the m605 Mutant
The wheat heading date mutant m605 was obtained by mutagenesis of YZ4110 seeds with EMS (Figures 1A,B). To measure the difference in heading date between the m605 mutant and YZ4110, we investigated the days to heading of m605 and YZ4110 in 10 environments. The results show that the average delay in the heading date of m605 was approximately 12.1 days, and the difference between m605 and YZ4110 was significant (Supplementary Table S2). Furthermore, the m605 mutant was characterized by a clustered growth habit accompanied by other characteristics, such as dwarfing and short spike length (Table 1). We also performed main shoot apex development analysis of the m605 and YZ4110, as shown in Supplementary Figure S1, and the development of floral primordium in the m605 was apparently slower than that in YZ4110. Moreover, we compared the differences in heading date between m605 and YZ4110 under controlled conditions. As shown in Figure 1C, and the three-way ANOVA analysis (Supplementary Table S3), in both LD and SD conditions, the differences in heading date between m605 and YZ4110 under non-vernalized conditions were significantly greater than those under vernalized conditions (p < 0.0001), whereas the performance of m605 and YZ4110 under LD and SD conditions was not significantly different (p = 0.5172). These results indicated that TaHdm605 interacted with vernalization treatments but did not follow the photoperiod pathway, implying that temperature may significantly influence the function of the TaHdm605 gene.
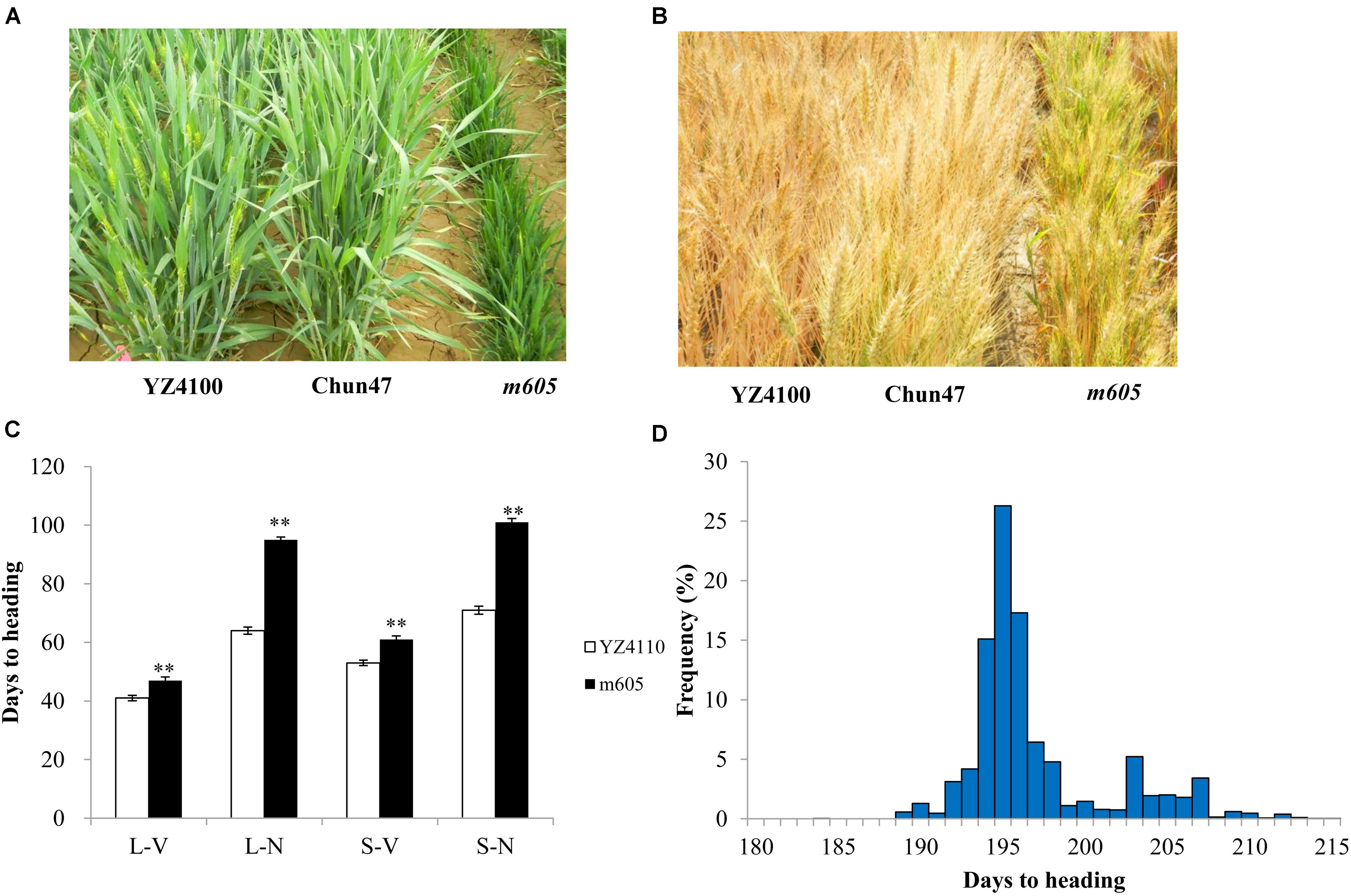
FIGURE 1. Phenotypes of wild-type YZ4110, late heading mutant m605 and early heading parent Chun47 as planted in the field and in controlled conditions. (A) The phenotypes of YZ4110, Chun47, and m605 at the heading stage when planted in the field. (B) The phenotypes of YZ4110, Chun47, and m605 at the ripening stage when planted in the field. (C) Days to heading of YZ4110 and m605 under controlled conditions. L-V, long day and vernalized; L-N, long day and non-vernalized; S-V, short day and vernalized; S-N, short day and non-vernalized. The data in (C) represent the means of three replicates, and the error bars indicate the standard deviation. ∗ and ∗∗ represents 0.01 < p < 0.05 and p < 0.01, respectively, based on a Student’s t-test. (D) Frequency distribution of heading date in the F2 population derived from Chun47 × m605 planted in Xinxiang, Henan province.
Genetic Analysis of the TaHdm605 Gene in Hexaploid Wheat
To test the segregation ratio of the heading date phenotype, the F2 populations derived from the m605 mutant crossed with the wheat cultivar Chun47 were planted in Beijing and Xinxiang (Henan province), respectively. A total of 2,480 F2 plants contained 587 late and 1,893 early heading plants in Xinxiang, in Henan province, and these plants fit a 1:3 ratio (χ2 = 2.34, p > 0.05) (Figure 1D); 746 F2 plants contained 205 late and 541 early heading plants in Beijing, and these plants also fit a 1:3 ratio (χ2 = 2.45, p > 0.05). The agronomic data from the Chun47 × m605 F2 population was listed in Supplementary Table S4. We also tested the segregation ratio of the heading date phenotype by using the F2 population derived from the m605 mutant crossed with wild-type YZ4110. In the 226 F2 plants planted in Beijing, there were 54 late and 172 early plants, as expected of a 1:3 ratio (χ2 = 0.15, p > 0.05). These results suggested that a single recessive gene, temporarily named TaHdm605, controlled the late heading phenotype in the m605 mutant. Additionally, through analyzing the agronomic traits of the F2 population, we found that the heading date of m605 had significantly negative correlations with 1,000-kernel weight, plant weight, grain number per plant, grain number per spike, spikelet number, spike length, and plant height (Supplementary Table S5).
Initial Mapping of the Heading Date Gene TaHdm605 by Using Public Markers
To map the TaHdm605 gene, 871 publicly available SSR markers distributed on the 21 wheat chromosomes, with an average distance of 10 cM, were used to screen the polymorphisms between the two parents as well as between early and late DNA bulk samples. As a result, four chromosome 3D specific markers, barc1119, wmc492, barc42, and cfd152, were found to have polymorphisms between both parents and bulk samples. Then, a genetic linkage map of TaHdm605 was constructed with the four linked marker loci by using 120 F2 plants from the cross between m605 and Chun47. Lastly, TaHdm605 was initially mapped between the markers barc42 and cfd152, with genetic distances of 5.8 and 14.4 cM, respectively (Figure 2A).
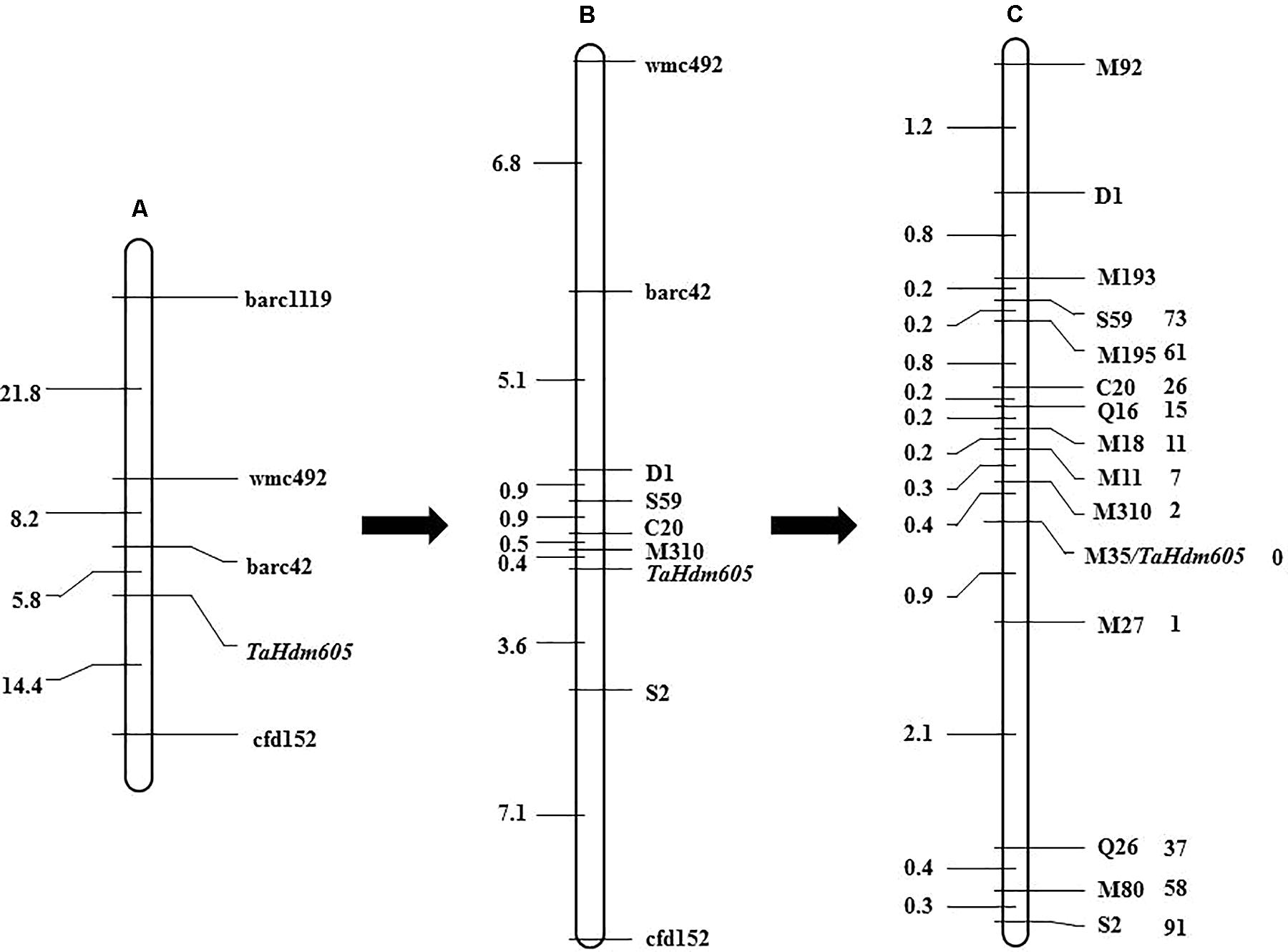
FIGURE 2. Genetic map of the heading date gene TaHdm605. (A) Initial mapping of the heading date gene TaHdm605 obtained with public markers and 120 F2 plants. (B) Genetic mapping of the heading date gene TaHdm605 obtained with markers developed by using the A. tauschii genome sequence (Ver. 0.1). (C) High-resolution genetic map of TaHdm605. The genetic distances in cM are shown on the left, and the markers are shown on the right. The numbers on the right of the markers represent the recombinants of the corresponding markers.
Fine Genetic Mapping of TaHdm605 by Using the A. tauschii Genome Reference Sequence
To obtain markers more closely linked to the TaHdm605 gene, the sequences of the markers barc42 and cfd152 were used to perform a BLAST search against the A. tauschii genome sequence (Ver. 0.1) to develop additional markers (Jia et al., 2013). As a result, a 63 Mb genomic region containing the TaHdm605 locus was identified, and a total of 1,015 new SSR markers were developed. Among these markers, five polymorphic markers, C20, D1, S2, S59, and M310, were screened and deployed to genotype the F2 segregating population of 3,226 plants. The results showed that TaHdm605 was located between markers M310 and S2 with genetic distances of 0.4 and 3.6 cM (Figure 2B). Later in this study, a new version of the A. tauschii genome sequence (Ver. 1.1) with higher quality became available (our unpublished data). By using this new sequence information, nine more markers, Q16, Q26, M11, M18, M27, M35, M80, M92, M193, and M195, were developed and used to screen the recombinants (Supplementary Table S6). Finally, TaHdm605 was mapped between the markers M310 and M27 with genetic distances of 0.4 and 0.9 cM and was found to co-segregate with the marker M35 (Figure 2C). Additionally, we checked the reported QTLs located on chromosome 3D, revealing only one QTL, QEet.fal-3DL, reported for heading date (Paillard et al., 2004). Further analysis indicated that QEet.fal-3DL is located between the markers Xgwm645 and Xgwm383a. By blasting these two markers sequence to the wheat genome reference sequence (CS; IWGSC RefSeq v1.0), we found that this QTL was not located in our candidate region. These results suggested that TaHdm605 is a novel gene for heading date.
Candidate Gene Analysis by Using Wheat Genome Reference Sequences
The reference sequence of the bread wheat Chinese Spring (CS; IWGSC RefSeq v1.0) was released during the process of our mapping work on TaHdm605. To support future identification of the candidate gene of TaHdm605, the sequences of M27 and M310 were used as query sequences to perform BLAST searches of the CS 3DL reference sequence. As a result, a contiguous sequence covering the markers M27 and M310 was identified. The physical interval between the two markers was 1.86 Mb and contained 26 predicted genes (Figures 3A,B). To obtain the functional information for these 26 predicted genes, we first conducted functional annotation for these genes (Supplementary Table S7). For these 26 predicted genes, seven genes (gene8, gene11, gene13, gene15, gene16, gene22, and gene25) were annotated only as expressed proteins with unknown function. The proteins encoded by the remaining 19 genes had a variety of functions. However, none of these 26 predicted genes was a previously reported gene that related to regulation of heading date or flowering time in wheat or any other species. Moreover, we checked the expression patterns of these 26 genes in different tissues by using the sequences of the 26 predicted genes to blast WheatEXP (see footnote 5). As a result, 11 genes were expressed in all of the five test tissues at different levels, four genes were expressed in only one or some of the five test tissues, and the remaining 11 genes were not Blast the hits with high similarity on chromosome 3D (Supplementary Figure S2).
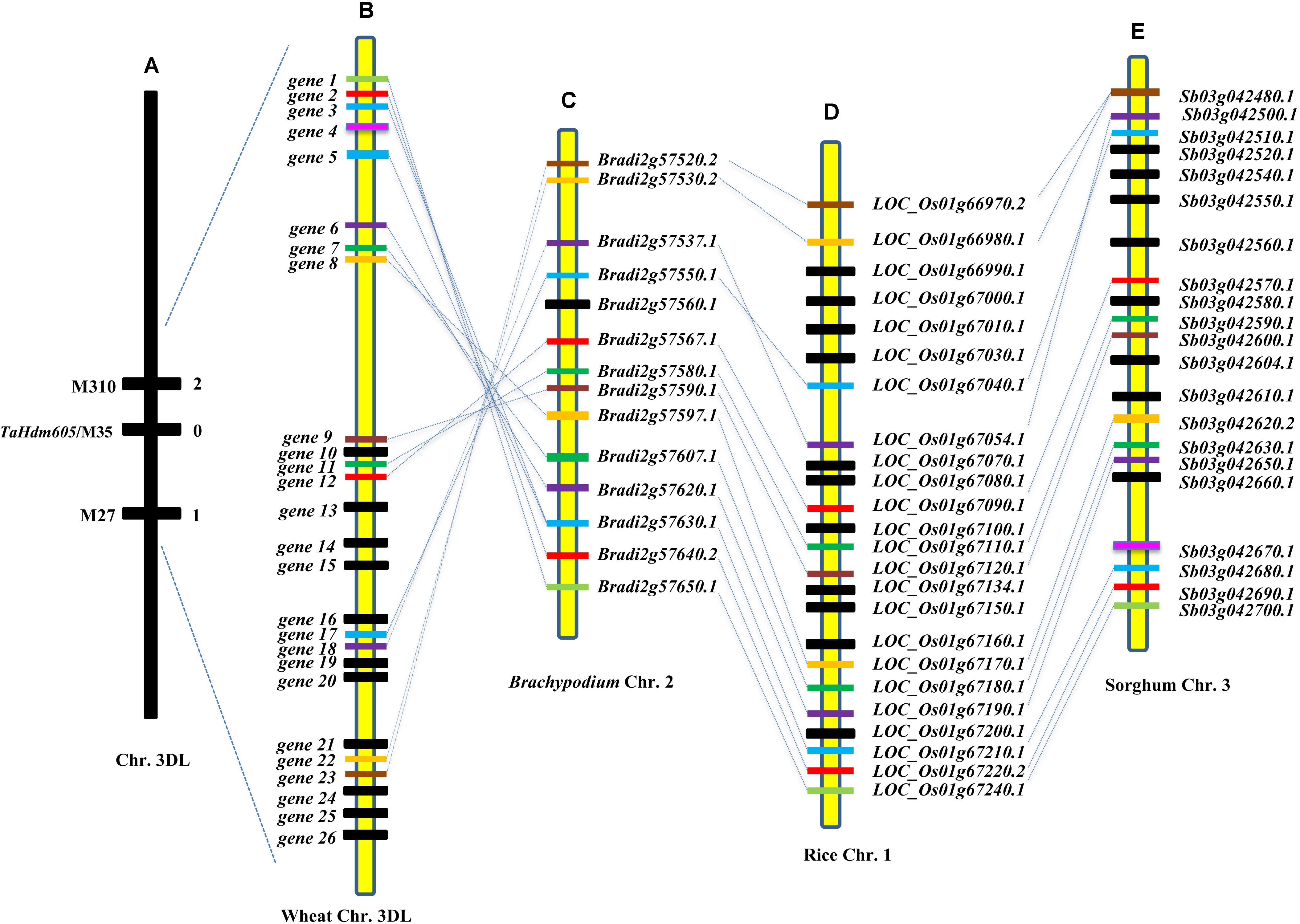
FIGURE 3. Fine mapping of TaHdm605 and comparative genomics analysis of the surrounding region in wheat, Brachypodium, rice, and sorghum. (A) Genetic linkage map of the heading date gene TaHdm605 on wheat chromosome arm 3DL. Horizontal lines indicate mapping marker locations, and the number of recombinants is shown on the right. (B) Physical map of the TaHdm605 locus based on Chinese Spring 3DL sequences. The horizontal lines represent the predicted wheat genes. (C) Orthologous genomic region of TaHdm605 on Brachypodium chromosome 2. (D) Orthologous genomic region of TaHdm605 on rice chromosome 1. (E) Orthologous genomic region of TaHdm605 on sorghum chromosome 3. For (B–E) orthologs are indicated by diagonal lines.
Comparative Genomics Analysis of the TaHdm605 Region Among Wheat, Brachypodium, Rice, and Sorghum
We also assessed the collinearity of the syntenic genomic region around the TaHdm605 locus in wheat compared to Brachypodium, rice, and sorghum. First, collinear regions spanning 150 kb, 123.5 kb, and 148 kb were identified in Brachypodium, rice, and sorghum, respectively (Figures 3C–E). Detailed analysis revealed a 150 kb segment in Brachypodium chromosome 2 containing 14 predicted genes (Bradig2g57520-Bradig2g57650), a 123.5 kb segment in rice chromosome 1 containing 24 predicted genes (Os01g66970–Os01g67240) and a 148 kb segment in sorghum chromosome 3 containing 21 predicted genes (Sb03g042480–Sb03g042700) (Figures 3B–E). Then, the sequences of the 26 predicted wheat genes were used to perform BLAST searches of the genome sequences of Brachypodium, rice, and sorghum to evaluate the collinearity of wheat with the other three species around the TaHdm605 locus. On the whole, these comparative analyses suggested that out of the 26 predicted CS genes, 14 are orthologous to genes in Brachypodium and rice and 15 are orthologous to genes in sorghum. Of the 14 predicted Brachypodium genes, 13 are orthologs of rice genes and 14 are orthologs of sorghum genes. Of the 24 predicted rice genes, 13 are orthologs of sorghum genes (Supplementary Table S7). These results implied that the genomic region around the TaHdm605 locus in wheat did not share a high degree of collinearity with the corresponding regions in the other three genomes.
Moreover, when projecting the high-resolution genetic map of A. tauschii onto the Brachypodium and rice chromosomes, we found that the region around the TaHdm605 locus is part of an ∼15.9 Mb inversion in the A. tauschii genome between the markers AT3D3149 and AT3D3183, and the inversion region in the wheat 3D chromosome is also approximately 15.9 Mb in length (CS; IWGSC RefSeq v1.0). The corresponding regions in Brachypodium and rice were approximately 0.96 Mb and 1.17 Mb, respectively (Figure 4).
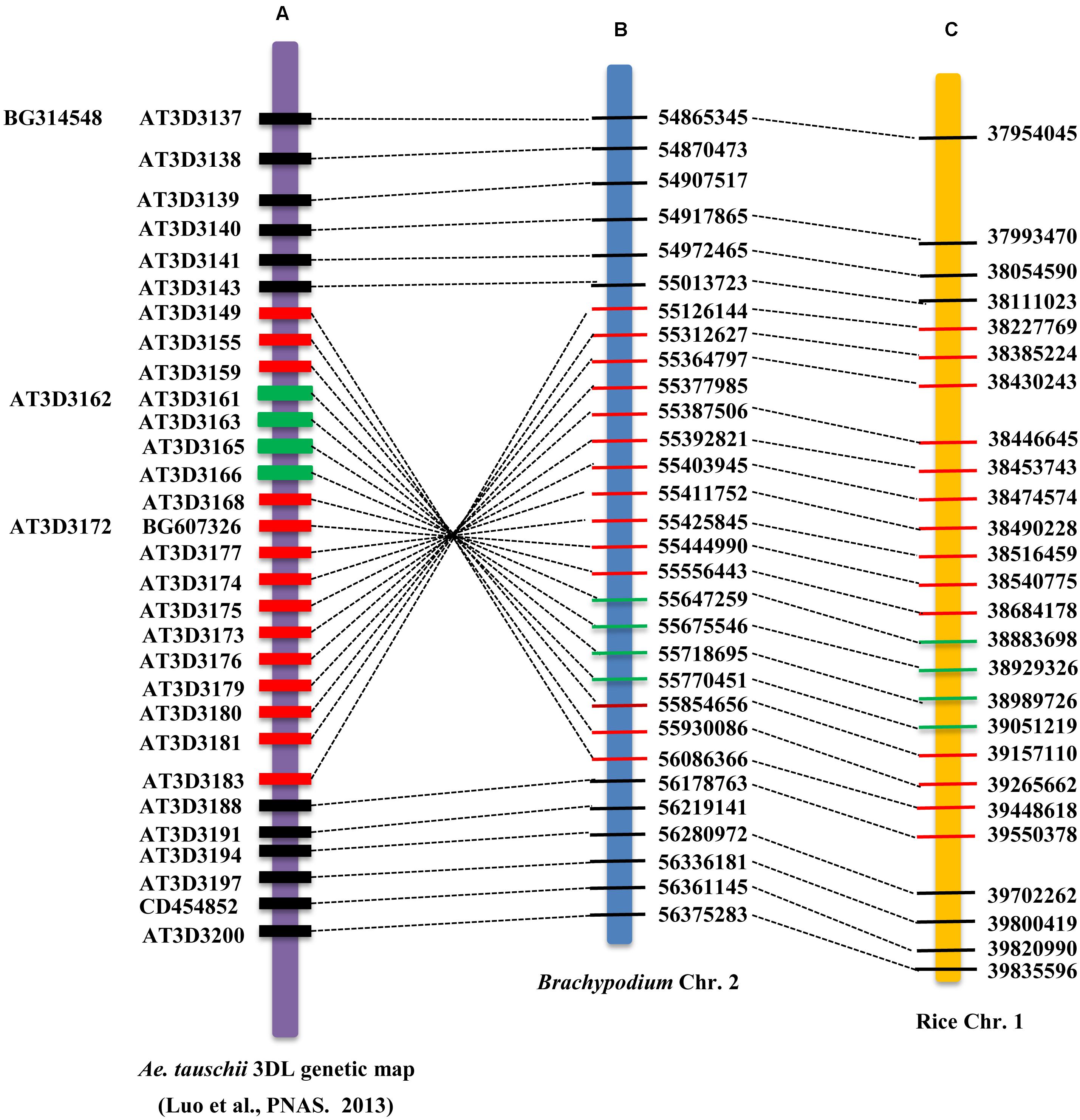
FIGURE 4. Schematic of the border and position of the genomic inversion around the TaHdm605 locus. The dashed lines indicate the collinear positions of the markers from A. tauschii on Brachypodium chromosome 2 and rice chromosome 1. The red rectangles represent the region of the genomic inversion in A. tauschii, Brachypodium and rice. The green rectangles indicate the region of the TaHdm605 locus covered by the markers M27 and M310. (A) High-resolution genetic map of TaHdm605 on A. tauschii, with single nucleotide polymorphism (SNP) markers shown on the left. (B,C) Physical maps of TaHdm605 on Brachypodium chromosome 2 and rice chromosome 1, with physical position on the right.
Discussion
Wheat is one of the world’s most important staple food crops. Heading date (flowering time) is an important agronomic trait that not only determines the distribution and regional adaptability of a crop but also influences its yield (Jung and Muller, 2009). Taking the heading date regulatory gene Ppd1 and rice Ghd7 as examples, these two genes also directly regulate crop growth and yield (Xue et al., 2008; Boden et al., 2015). Therefore, identifying genes that regulate heading is beneficial for crop improvement including appropriate heading date and high yield in the process of modern plant breeding.
Plants have developed multiple mechanisms to ensure that heading or flowering occurs at the correct time in a given environment, which were regulated by a series of genes. In wheat, VRN1, VRN2, VRN3, and VRN-D4 in the vernalization pathway and Ppd-1A, Ppd-1B, and Ppd-1D in the photoperiod pathway have been cloned and functionally studied (Zheng et al., 2013; Kippes et al., 2015), however, very little is known about the other genes that participate in these two pathways or about genes active in other heading date regulatory pathways. Therefore, characterization of heading date mutants and mapping new heading date regulatory genes are of great significance for clarifying the mechanisms regulating wheat heading date. In this work, we characterized a late heading mutant, m605, which was obtained by EMS mutagenesis of YZ4110 seeds. Our results indicate that the mutant m605 exhibited about a 12.1-day delay in heading date compared to YZ4110. In china, the light and temperature conditions vary greatly between different ecological regions, therefore, a delay in the heading date of m605 may be beneficial in some environments. As a mutant, some undesirable changes in several yield components were detected in mutant m605, which will limit its direct and wide use in future breeding research. However, after we clone the causative gene of mutant m605, we will determine its sequence polymorphisms in wheat varieties distributed in different ecological conditions, which allows us to detect the natural variant that could affect wheat growth habits and heading dates. Ultimately, we will select the suitable natural alleles of a specific wheat variety in the breeding programs. Moreover, studies conducted on this gene will also enrich our knowledge and understanding of the regulation of flowering time in wheat.
Genetic analysis suggested that the late heading in the m605 mutant is controlled by a single recessive allele of the TaHdm605 gene. By use of publicly available markers, the late heading gene TaHdm605 was mapped to a region of chromosome 3DL spanned by markers barc42 and cfd152 with genetic distances of 5.8 cM and 14.4 cM, respectively. Constructing the high-resolution genetic linkage map and the physical map containing the candidate gene is among the most important steps toward map-based cloning of the target gene. At the beginning of the mapping of the TaHdm605 gene, the assembled reference of the sequence of hexaploid wheat was not available. However, the genome sequence of the wheat D-genome progenitor A. tauschii was identified during our study (Jia et al., 2013). The availability of the genome sequence resource was of great utility in further marker development for mapping the TaHdm605 gene. Fortunately, the initial mapping using public markers already located the TaHdm605 on the 3DL chromosome, which meant that using the genome sequence of A. tauschii was a feasible and efficient way to develop new markers linked to TaHdm605. With this strategy, 15 polymorphic markers linked to TaHdm605 were successfully developed, and the TaHdm605 gene was mapped to a 1.3 cM interval. To date, none of the heading date regulatory genes or QTLs have been reported in this region, indicating that TaHdm605 is a novel gene related to heading date in wheat.
Due to the great advances in sequencing technologies and assembly strategies, wheat genome sequencing has achieved very remarkable progress, and the new version of the chromosome-based reference sequences with the whole genome assembly and the emmer wheat genome sequence have recently become available (International Wheat Genome Sequencing Consortium [IWGSC], 2014; Avni et al., 2017); these advances have provided valuable sequence information for the cloning of important genes in the future. Later in the study, with the release of the wheat genome assembly (IWGSC RefSeq v1.0), additional sequences could be used to construct the physical map around the TaHdm605 gene. By using this sequence information, we located TaHdm605 in a 1.86 Mb genome region that contained 26 predicted genes. Based on the sequence analysis and functional annotation of these 26 genes, none of the genes were previously reported genes that function in regulating heading date or flowering time in plants.
In the future work, to clone the candidate gene of TaHdm605, we must sequence these 26 genes in YZ4110 and m605 and find the mutated nucleotides in m605. In the meantime, one thing we cannot ignore is that some causative mutant genes may be located in non-coding regions, such as promoter or intergenic regions. If we do not determine the causative mutant site in gene coding regions for m605, different approaches may be required to detect the causative mutation in the non-coding region or promoter region. Once we obtain the causative mutation, the candidate gene of TaHdm605 can be determined based on the composition of the mutated nucleotide sites in the recombinants. Therefore, the results collected here will serve as a valuable framework for future map-based cloning of TaHdm605 and for breeders to engineer changes in this trait.
Author Contributions
XK, JJ, and XL designed the research. GL and TZ collected the plant materials and constructed the populations. GL and XZ performed the experiments. GL, LZ, XZ, CX, JJ, and XK analyzed the data. LZ and XK wrote the paper.
Funding
This work was supported by the National Key Research and Development Program of China (2016YFD0101802), the National Natural Science Foundation of China (31371619), and the National Transgenic Research Project (2016ZX08009001-001-004).
Conflict of Interest Statement
The authors declare that the research was conducted in the absence of any commercial or financial relationships that could be construed as a potential conflict of interest.
Acknowledgments
We would like to thank Ruilian Jing (Institute of Crop Sciences, Chinese Academy of Agricultural Sciences, Beijing, China) for kindly providing the seeds of Chun47 and Yongqiang Gu, Yongqing Jiao, Huihui Li, and Cheng Zou for their helpful comments. We also thank Suxia Zhang, Lingli Zheng, and Yuhong Liu for their work in the field.
Supplementary Material
The Supplementary Material for this article can be found online at: https://www.frontiersin.org/articles/10.3389/fpls.2018.01059/full#supplementary-material
FIGURE S1 | Comparision of floral primordium betweenYZ4110 and m605. (A,C) Floral primordium of YZ4110. (B,D) Floral primordium of m605.
FIGURE S2 | The expression profiles of the candidate genes on WheatEXP.
TABLE S1 | Details for primers used in the phenotype detection.
TABLE S2 | The comparison of days to heading between YZ4110 and m605.
TABLE S3 | The significance test of the interactions between TaHdm605 alleles, photoperiods, and vernalization treatments.
TABLE S4 | Agronomic trait datas of the F2 population in the crossing of Chun47 × m605 in Beijing and Xinxiang.
TABLE S5 | Agronomic trait analysis based on the F2 population from the cross Chun47 × m605.
TABLE S6 | Newly developed polymorphic SSR markers tightly linked to the TaHdm605 locus.
TABLE S7 | Gene annotation of the TaHdm605 genomic region on the Chinese Spring chromosome 3DL.
Abbreviations
EMS, ethyl methanesulfonate; LD, long-day; SD, short-day.
Footnotes
- ^ http://wheat.pw.usda.gov/GG3
- ^ http://bioinfo.ut.ee/primer3-0.4.0/
- ^ https://urgi.versailles.inra.fr/triannot/?pipeline
- ^ https://www.ncbi.nlm.nih.gov
- ^ https://wheat.pw.usda.gov/WheatExp/
- ^ http://mips.helmholtz-muenchen.de/plant/brachypodium/
- ^ http://rice.plantbiology.msu.edu/
- ^ http://mips.helmholtz-muenchen.de/plant/sorghum/
References
Alvarez, M. A., Tranquilli, G., Lewis, S., Kippes, N., Dubcovsky, J. (2016). Genetic and physical mapping of the earliness per se locus Eps-Am1 in Triticum monococcum identifies EARLY FLOWERING3 (ELF3) as a candidate gene. Funct. Intrgr. Genomics. 16, 365–382. doi: 10.1007/s10142-016-0490-3
Avni, R., Nave, M., Barad, O., Baruch, K., Twardziok, S. O., Gundlach, H., et al. (2017). Wild emmer genome architecture and diversity elucidate wheat evolution and domestication. Science 357, 93–97. doi: 10.1126/science.aan0032
Boden, S. A., Cavanagh, C., Cullis, B. R., Ramm, K., Greenwood, J., Jean Finnegan, E, et al. (2015). Ppd-1 is a key regulator of inflorescence architecture and paired spikelet development in wheat. Nat. Plants 1:14016. doi: 10.1038/nplants.2014.16
Bullrich, L., Appendino, M. L., Tranquilli, G., Lewis, S., and Dubcovsky, J. (2002). Mapping of a thermos-sensitive earliness per se gene on Triticum monococcum chromosome 1Am. Theor. Appl. Genet. 105, 585–593. doi: 10.1007/s00122-002-0982-5
Chen, A., and Dubcovsky, J. (2012). Wheat TILLING mutants show that the vernalization gene VRN1 down-regulates the flowering repressor VRN2 in leaves but is not essential for flowering. PLoS Genet. 8:e1003134. doi: 10.1371/journal.pgen.1003134
Dhillon, T., Pearce, S. P., Stockinger, E. J., Distelfeld, A., Li, C., Knox, A. K., et al. (2010). Regulation of freezing tolerance and flowering in temperate cereals, the VRN-1 connection. Plant Physiol. 153, 1846–1858. doi: 10.1104/pp.110.159079
Diaz, A., Zikhali, M., Turner, A. S., Isaac, P., and Laurie, D. A. (2012). Copy number variation affecting the Photoperiod-B1 and vernalization-A1 genes is associated with altered flowering time in wheat Triticum aestivum. PLoS One 7:e33234. doi: 10.1371/journal.pone.0033234
Distelfeld, A., Li, C., and Dubcovsky, J. (2009). Regulation of flowering in temperate cereals. Curr. Opin. Plant Biol. 12, 178–184. doi: 10.1016/j.pbi.2008.12.010
Dubcovsky, J., Loukoianov, A., Fu, D., Valarik, M., Sanchez, A., and Yan, L. (2006). Effect of photoperiod on the regulation of wheat vernalization genes VRN1 and VRN2. Plant Mol. Biol. 60, 469–480. doi: 10.1007/s11103-005-4814-2
Faricelli, M. E., Valárik, M., and Dubcovsky, J. (2010). Control of flowering time and spike development in cereals, the earliness per se Eps-1 region in wheat, rice, and Brachypodium. Funct. Integr. Genomics 10, 293–306. doi: 10.1007/s10142-009-0146-7
Fu, D., Szucs, P., Yan, L., Helguera, M., Skinner, J. S., Hayes, P., et al. (2005). Large deletions in the first intron of the VRN1 vernalization gene are associated with spring growth habit in barley and polyploidy wheat. Mol. Genet. Genomics 273, 54–65. doi: 10.1007/s00438-004-1095-4
Guo, Z., Song, Y., Zhou, R., Ren, Z., and Jia, J. (2010). Discovery, evaluation and distribution of haplotypes of the wheat Ppd-D1 gene. New Phytol. 185, 841–851. doi: 10.1111/j.1469-8137.2009.03099.x
International Wheat Genome Sequencing Consortium [IWGSC] (2014). A chromosome-based draft sequence of the hexaploid bread wheat Triticum aestivum genome. Science 345:1251788. doi: 10.1126/science.1251788
Jia, J., Zhao, S., Kong, X., Li, Y., Zhao, G., He, W., et al. (2013). Aegilops tauschii draft genome sequence reveals a gene repertoire for wheat adaptation. Nature 496, 91–95. doi: 10.1038/nature12028
Jung, C., and Muller, A. E. (2009). Flowering time control and applications in plant breeding. Trends Plant Sci. 14, 563–573. doi: 10.1016/j.tplants.2009.07.005
Kippes, N., Debernardi, J. M., Vasquez-Gross, H. A., Akpinar, B. A., Budak, H., Kato, K., et al. (2015). Identification of the VERNALIZATION 4 gene reveals the origin of spring growth habit in ancient wheats from South Asia. Proc. Natl. Acad. Sci. U.S.A. 112, E5401–E5410. doi: 10.1073/pnas.1514883112
Kippes, N., Zhu, J., Chen, A., Vanzetti, L., Lukaszewski, A., Nishida, H., et al. (2014). Fine mapping and epistatic interactions of the vernalization gene VRN-D4 in hexaploid wheat. Mol. Genet. Genomics 289, 47–62. doi: 10.1007/s00438-013-0788-y
Li, C., Distelfeld, A., Comis, A., and Dubcovsky, J. (2011). Wheat flowering repressor VRN2 and promoter CO2 compete for interactions with NUCLEAR FACTOR-Y complexes. Plant J. 67, 763–773. doi: 10.1111/j.1365-313X.2011.04630.x
Li, C., and Dubcovsky, J. (2008). Wheat FT protein regulates VRN1 transcription through interactions with FDL2. Plant J. 55, 543–554. doi: 10.1111/j.1365-313X.2008.03526.x
Lincoln, S., Daly, M., and Lander, E. (1992). Constructing Genetic Maps with Mapmaker/EXP30, 3rd Edn. Cambridge, MA: Whitehead Institute.
Liu, R. H., and Meng, J. L. (2003). MapDraw, a microsoft excel macro for drawing genetic linkage maps based on given genetic linkage data. Yi Chuan 25, 317–321.
Luo, M. C., Gu, Y. Q., You, F. M., Deal, K. R., Ma, Y., Hu, Y., et al. (2013). A 4-gigabase physical map unlocks the structure and evolution of the complex genome of Aegilops tauschii, the wheat D-genome progenitor. Proc. Natl. Acad. Sci. U.S.A. 110, 7940–7945. doi: 10.1073/pnas.1219082110
Murai, K., Miyamae, M., Kato, H., Takumi, S., and Ogihara, Y. (2003). WAP1, a wheat APETALA1 homolog, plays a central role in the phase transition from vegetative to reproductive growth. Plant Cell Physiol. 44, 1255–1265. doi: 10.1093/pcp/pcg171
Nemoto, Y., Kisaka, M., Fuse, T., Yano, M., and Ogihara, Y. (2003). Characterization and functional analysis of three wheat genes with homology to the CONSTANS flowering time gene in transgenic rice. Plant J. 36, 82–93. doi: 10.1046/j.1365-313X.2003.01859.x
Nishida, H., Yoshida, T., Kawakami, K., Fujita, M., Long, B., Akashi, Y., et al. (2013). Structural variation in the 5’ upstream region of photoperiod-insensitive alleles Ppd-A1a and Ppd-B1a identified in hexaploid wheat Triticum aestivum L., and their effect on heading time. Mol. Breed. 31, 27–37. doi: 10.1007/s11032-012-9765-0
Paillard, S., Schnurbusch, T., Tiawari, R., Messmer, M., Winzeler, M., Keller, B., et al. (2004). QTL analysis of resistance to Fusarium head blight in Swiss winter wheat (Triticum aestivum L.). Theor. Appl. Genet. 109, 323–332. doi: 10.1007/s00122-004-1628-6
Saghai-Maroof, M. A., Soliman, K. M., Jorgensen, R. A., and Allard, R. W. (1984). Ribosomal DNA spacer-length polymorphisms in barley, mendelian inheritance, chromosomal location, and population dynamics. Proc. Natl. Acad. Sci. U.S.A. 81, 8014–8018. doi: 10.1073/pnas.81.24.8014
Slafer, G. A. (1996). Differences in phasic development rate amongst wheat cultivars independent of responses to photoperiod and vernalization. A viewpoint of the intrinsic earliness hypothesis. J. Agric. Sci. 126, 403–419. doi: 10.1017/S0021859600075493
Sun, H., Guo, Z., Gao, L., Zhao, G., Zhang, W., Zhou, R., et al. (2014). DNA methylation pattern of Photoperiod-B1 is associated with photoperiod insensitivity in wheat Triticum aestivum. New Phytol. 204, 682–692. doi: 10.1111/nph.12948
Tranquilli, G., and Dubcovsky, J. (2000). Epistatic interactions between vernalization genes Vrn-Am1 and Vrn-Am2 in diploid wheat. J. Hered. 91, 304–306. doi: 10.1093/jhered/91.4.304
Trevaskis, B., Hemming, M. N., Dennis, E. S., and Peacock, W. J. (2007). The molecular basis of vernalization-induced flowering in cereals. Trends Plant Sci. 12, 352–357. doi: 10.1016/j.tplants.2007.06.010
Xue, W., Xing, Y., Weng, X., Zhao, Y., Tang, W., Wang, L., et al. (2008). Natural variation in Ghd7 is an important regulator of heading date and yield potential in rice. Nat. Genet. 40, 761–767. doi: 10.1038/ng.143
Yan, L., Fu, D., Li, C., Blechl, A., Tranquilli, G., Bonafede, M., et al. (2006). The wheat and barley vernalization gene VRN3 is an orthologue of FT. Proc. Natl. Acad. Sci. U.S.A. 103, 19581–19586. doi: 10.1073/pnas.0607142103
Yan, L., Loukoianov, A., Blechl, A., Tranquilli, G., Ramakrishna, W., SanMiguel, P., et al. (2004). The wheat VRN2 gene is a flowering repressor down-regulated by vernalization. Science 303, 1640–1644. doi: 10.1126/science.1094305
Yoshida, T., Nishida, H., Zhu, J., Nitcher, R., Distelfeld, A., Akashi, Y., et al. (2010). Vrn-D4 is a vernalization gene located on the centromeric region of chromosome 5D in hexaploid wheat. Theor. Appl. Genet. 120, 543–552. doi: 10.1007/s00122-009-1174-3
Zhao, T. X., Kong, X. Y., Zhou, R. H., Gao, S. C., and Jia, J. Z. (2009). Morphological identification and analysis of EMS mutants from hexaploid wheat cultivar Yanzhan 4110. Sci. Agric. Sin. 42, 755–764.
Zhao, X. Y., Liu, M. S., Li, J. R., Guan, C. M., and Zhang, X. S. (2005). The wheat TaGI1, involved in photoperiodic flowering, encodes an Arabidopsis GI ortholog. Plant Mol. Biol. 58, 53–64. doi: 10.1007/s11103-005-4162-2
Zheng, B., Biddulph, B., Li, D., Kuchel, H., and Chapman, S. (2013). Quantification of the effects of VRN1 and Ppd-D1 to predict spring wheat Triticum aestivum heading time across diverse environments. J. Exp. Bot. 64, 3747–3761. doi: 10.1093/jxb/ert209
Keywords: wheat, EMS mutant, heading date gene, fine mapping, physical map
Citation: Zhang X, Liu G, Zhang L, Xia C, Zhao T, Jia J, Liu X and Kong X (2018) Fine Mapping of a Novel Heading Date Gene, TaHdm605, in Hexaploid Wheat. Front. Plant Sci. 9:1059. doi: 10.3389/fpls.2018.01059
Received: 05 December 2017; Accepted: 29 June 2018;
Published: 18 July 2018.
Edited by:
Petr Smýkal, Palacký University, CzechiaReviewed by:
Liezhao Liu, Southwest University, ChinaJan Šafář, Institute of Experimental Botany (ASCR), Czechia
Copyright © 2018 Zhang, Liu, Zhang, Xia, Zhao, Jia, Liu and Kong. This is an open-access article distributed under the terms of the Creative Commons Attribution License (CC BY). The use, distribution or reproduction in other forums is permitted, provided the original author(s) and the copyright owner(s) are credited and that the original publication in this journal is cited, in accordance with accepted academic practice. No use, distribution or reproduction is permitted which does not comply with these terms.
*Correspondence: Xu Liu, bGl1eHUwMUBjYWFzLmNu; Xiuying Kong, a29uZ3hpdXlpbmdAY2Fhcy5jbg==
†These authors have contributed equally to this work.