- 1Beijing Advanced Innovation Center for Tree Breeding by Molecular Design, College of Biological Sciences and Technology, Beijing Forestry University, Beijing, China
- 2Public Analysis and Testing Center, Beijing Forestry University, Beijing, China
- 3Beijing Dabeinong Technology Group Co., Ltd., Beijing, China
- 4College of Life Science, Jiangsu Normal University, Xuzhou, China
Non-invasive micro-test techniques (NMT) were used to analyze NaCl-altered flux profiles of K+, Na+, and H+ in roots and effects of NaHS (a H2S donor) on root ion fluxes in two contrasting poplar species, Populus euphratica (salt-resistant) and Populus popularis (salt-sensitive). Both poplar species displayed a net K+ efflux after exposure to salt shock (100 mM NaCl), as well as after short-term (24 h), and long-term (LT) (5 days) saline treatment (50 mM NaCl, referred to as salt stress). NaHS (50 μM) restricted NaCl-induced K+ efflux in roots irrespective of the duration of salt exposure, but K+ efflux was not pronounced in data collected from the LT salt stress treatment of P. euphratica. The NaCl-induced K+ efflux was inhibited by a K+ channel blocker, tetraethylammonium chloride (TEA) in P. popularis root samples, but K+ loss increased with a specific inhibitor of plasma membrane (PM) H+-ATPase, sodium orthovanadate, in both poplar species under LT salt stress and NaHS treatment. This indicates that NaCl-induced K+ loss was through depolarization-activated K+ channels. NaHS caused increased Na+ efflux and a corresponding increase in H+ influx for poplar roots subjected to both the short- and LT salt stress. The NaHS-enhanced H+ influx was not significant in P. euphratica samples subjected to short term salt stress. Both sodium orthovanadate and amiloride (a Na+/H+ antiporter inhibitor) effectively inhibited the NaHS-augmented Na+ efflux, indicating that the H2S-enhanced Na+ efflux was due to active Na+ exclusion across the PM. We therefore conclude that the beneficial effects of H2S probably arise from upward regulation of the Na+/H+ antiport system (H+ pumps and Na+/H+ antiporters), which promote exchange of Na+ with H+ across the PM and simultaneously restricted the channel-mediated K+ loss that activated by membrane depolarization.
Introduction
High salt content in soil leads to plant growth inhibition due to ion toxicity, water shortage, and nutrient imbalances (Zhu, 2001). The maintenance of K+ and Na+ homeostasis is essential for herbaceous and woody species growing in or adapting to saline environments (Chen et al., 2001, 2002, 2003; Cuin et al., 2003; Ottow et al., 2005; Wang et al., 2006, 2007, 2008; Shabala and Cuin, 2008; Sun et al., 2009a). NaCl-induced K+ loss observed in higher plants is mediated by depolarization-activated KORCs (outward rectifying K+ channels) and NSCCs (non-selective cation channels; Chen et al., 2007; Shabala and Cuin, 2008; Sun et al., 2009b). The Na+/H+ antiport system contributes to Na+ extrusion and vacuolar compartmentation (Blumwald et al., 2000; Shi et al., 2000; Apse et al., 2003; He et al., 2005). Na+ movement is highly dependent on H+-ATPase since the H+ pumps provide a proton gradient to drive the Na+/H+ exchange across the plasma and vacuolar membranes (Blumwald et al., 2000; Zhu, 2003; Sun et al., 2009a,b; Ma et al., 2010). Moreover, H+-ATPase also inhibits the entry of Na+ through NSCCs (Maathuis and Sanders, 2001; Maathuis, 2006).
A variety of stress signals, extracellular ATP, H2O2, cytosolic [Ca2+], and NO, mediate ionic homeostasis under NaCl stress through regulations of the Na+/H+ antiport system (Zhang et al., 2006; Shi et al., 2007; Sun et al., 2010a,b, 2012; Lu et al., 2013). Evidence increasingly shows that hydrogen sulfide (H2S) functions as a molecular signal that also mediates numerous physiological processes. H2S has been shown to regulate photosynthesis (Chen et al., 2011), stomatal movement (García-Mata and Lamattina, 2010; Lisjak et al., 2010; Jin and Pei, 2016; Jin et al., 2017), adventitious rooting (Zhang et al., 2009a), and flower senescence (Zhang et al., 2011). The role of H2S in stress physiology has thus received growing attention among researchers. Researchers have shown that H2S mediates plant adaptive response to boron (Wang et al., 2010), aluminum (Zhang et al., 2010a), copper (Zhang et al., 2008), cadmium (Li et al., 2012; Sun et al., 2013; Qiao et al., 2016), chromium stress (Fang et al., 2016), and general osmotic and drought stresses (Zhang et al., 2009b, 2010b). Interestingly, H2S enhanced alfalfa’s ability to tolerate NaCl during seed germination through a nitric oxide pathway (Wang et al., 2012). Under NaCl stress, Wang et al. (2012) showed that NaHS (a H2S donor) increased the ratio of K+/Na+ in root structures of germinated alfalfa seeds. Endogenous H2S increased salt tolerance by preventing K+ loss and reestablishing redox homeostasis in Medicago sativa seedlings (Lai et al., 2014). Janicka et al. (2017) confirmed the involvement of H2S as a signaling molecule in the modification of plasma membrane (PM) H+-ATPase in salt-exposed cucumber roots. H2S likely interacts with NO in to modify antioxidant and redox defense systems in tomato and tobacco plants (da Silva et al., 2017; da-Silva et al., 2018; da-Silva and Modolo, 2017). Furthermore, exogenous H2S alleviates salt stress by reducing Na+ content in wheat seedlings (Deng et al., 2016). The physiological mechanism by which H2S influences K+/Na+ homeostasis in woody plants, however, remains unclear.
The objectives of present study were to evaluate the roles of H2S to K+/Na+ homeostasis in two poplar species with differing salt tolerances: the salt-resistant Populus euphratica and the salt-sensitive Populus popularis. We used non-invasive micro-test techniques (NMT) to measure the NaCl-induced flux profiles of K+, Na+, and H+ in the presence and absence of H2S. This study also applied both short- and long-term (LT) salt treatments to subjects in order to clarify the effects of H2S on salt tolerance. The effects of PM transport inhibitors on ion flux were characterized in order to test specific physiological mechanisms linking NaHS to K+/Na+ homeostasis in tree plants.
Materials and Methods
Plant Materials
In April, one-year-old seedlings of P. euphratica grown in the Xinjiang Uygur Autonomous Region of China and one-year-old cuttings of P. popularis 35–44 (P. popularis) grown in the nursery of Beijing Forestry University were planted in individual pots (about 10 L) containing loam soil. These were then raised in a greenhouse. Plant subjects were kept well irrigated and supplemented with full-strength Hoagland’s nutrient solution every 2 weeks. Greenhouse air temperature ranged from 25 to 30°C while relative humidity ranged from 60 to 70%. Plants were subjected to a 12-h photoperiod (7:00–19:00) with photosynthetically active radiation varying from 400 to 800 μmol m-2 s-1. Plants grew for one month prior to hydroponic culture. Rooted seedlings and cuttings were then collected, washed free of soil, and transferred to 2 L individual porcelain pots containing diluted (25% strength) Hoagland’s nutrient solution. The plants were continuously aerated during the period of hydroponic acclimation. Nutrient solution was renewed every 2 days.
Treatments Designed for Flux Recordings
Series 1
Root samples of P. euphratica and P. popularis controls were excised, immediately rinsed with re-distilled water, followed by 30 min equilibration in a basic solution [NaCl (0.1 mM), MgCl2 (0.1 mM), CaCl2 (0.1 mM), and KCl (0.5 mM)] supplemented with or without 25, 50, and 200 μM NaHS (Sigma, St. Louis, MO, United States). The pH of the solution was adjusted to 5.7 with NaOH and HCl. Thereafter, steady K+ flux was recorded in the apical region (ca. 300 μm from the root tip) for 10 min before the addition of salt. A salt shock (100 mM NaCl) was applied by adding NaCl stock (0.2 M, pH 6.0 adjusted with NaOH and HCl). Transient ion fluxes were monitored for an additional 40 min. The flux data collected during the first 2–3 min were not included in analysis due to diffusion effects from stock addition (Shabala, 2000). For each dose treatment, NaCl-altered K+ kinetics were recorded from at least five individual plants.
Series 2
Populus euphratica and P. popularis plants were subjected to one of the four treatments: control, control plus NaHS (50 μM; Sigma, St. Louis, MO, United States), NaCl (50 mM), and NaCl (50 mM) plus NaHS (50 μM). NaHS acts as a H2S donor (Zhao et al., 2001; Wang et al., 2010). The NaCl and NaHS salts were added to the full-strength Hoagland’s nutrient solution. A short-term (ST) saline treatment exposed plants to 50 mM NaCl for 24 h while the LT saline treatment exposed plants to 50 mM NaCl for 5 days. Controls were fertilized but not treated with NaCl or NaHS. In the long term treatment of 5 days, the solutions containing NaHS were renewed daily to ensure its concentration. Ion flux measurements were performed on 2–3 cm of apical root segments. The K+, Na+, H+ fluxes were recorded individually with NMT selective electrodes. In our study, fluxes of K+, Na+, and H+ were measured using roots sampled from the same plants. For each treatment (control, control+NaHS, NaCl, NaCl+NaHS), flux recordings of each target ion (K+, Na+, and H+) were recorded from at least five individual plants after ST and LT salt stress treatment.
Series 3
The same three pharmacological agents were applied to LT- and NaHS-treated roots for 30 min (Sun et al., 2009a). These include:
(1) Twenty millimolars TEA (tetraethylammonium chloride, a K+ channel blocker);
(2) Five hundred micromolars sodium orthovanadate (a specific inhibitor of PM H+-ATPase);
(3) Fifty micromolars amiloride (a Na+/H+ antiporter inhibitor).
After inhibitor treatment, roots were immersed in the measuring solution for 30 min equilibration prior to flux recordings (Sun et al., 2009a,b, 2010a; Lang et al., 2014). Steady-state flux of K+ and Na+ were then recorded for 30 min in P. euphratica and P. popularis roots pretreated with or without inhibitors. Fluxes of K+ and Na+ were recorded individually using roots sampled from the same plants. For NaCl, NaHS, and pharmacological treatments, flux recordings of each target ion (K+ and Na+) were recorded from at least five individual plants.
Flux Recording of K+, Na+, and H+
Preparation and Calculation of Microelectrodes
A NMT system (BIO-001A, Younger USA Sci. & Tech. Corp., Amherst, MA, United States) was used to non-invasively measure net K+, Na+, and H+ fluxes in the P. euphratica and P. popularis root samples. The ion-selective microelectrodes were prepared according to Sun et al. (2009a,b). Prior to flux measurements, K+, Na+, and H+ microelectrodes were, respectively, calibrated in following solutions:
(1) K+ calculation series (mM): 0.1, 0.5, and 1.0;
(2) Na+ calculation series (mM): 0.1, 0.5, and 1.0;
(3) H+ calculation series: pH 5.0, 6.0, and 7.0.
Our experiments used only electrodes with Nerstain slopes >50 mV/decade.
Steady-State NMT Measurements
Young roots (3.0 cm) were sampled from control, NaHS, ST, and LT salt stressed poplars (Series 2). The root tips were rinsed with re-distilled water, and then immediately equilibrated for 30 min in the following solutions (Sun et al., 2009a):
(1) K+ and H+ measuring solutions (mM): NaCl (0.1), MgCl2 (0.1), CaCl2 (0.1), and KCl (0.5), pH 5.7 (adjusted with NaOH and HCl);
(2) Na+ measuring solutions (mM): NaCl (0.1), MgCl2 (0.1), CaCl2 (0.1), and KCl (0.1), pH 5.7 (adjusted with NaOH and HCl).
After equilibration, roots samples were transferred to a NMT measuring chamber containing fresh measuring solution (10 mL) and then immobilized at their bases. Ion fluxes were measured at the root meristematic region (300 μm from the tip). Target ion gradients were measured by moving the electrode between two positions close to the root surface (electrode pre-set excursion 30 μM for roots) at a programmed frequency of 0.3–0.5 Hz. DRIREF-2 (World Precision Instruments) was used as the reference electrode. Steady-state fluxes of K+, Na+, and H+ in P. euphratica and P. popularis root samples were continuously recorded for 30 min. For control, ST, and LT treatment, flux recordings of each target ion were recorded from at least five individual plants.
Long-term and inhibitor-treated roots (Series 3) were also used for steady-state flux recordings as described above. The inhibitors were not included in the refreshed measuring solutions. This was to avoid the potential interference of these pharmacological agents on flux recordings. We have noticed that sodium orthovanadate had clear effect on the Nernstian slopes of the microelectrodes (e.g., Na+), although the effects of amiloride and TEA on Na+ and K+ microelectrodes were much less (Sun et al., 2009a,b).
Data Analysis
JCal V3.3 developed by Yue Xu1,2 was used to calculate three-dimensional ionic flux rates. All mean data were subjected to standard analysis of variance (ANOVA) methods. Significant differences between means were determined using Duncan’s multiple range test. Unless otherwise stated, differences are considered statistically significant when P < 0.05.
Results
Effects of NaHS on Transient K+ Kinetics With NaCl Shock
In the two poplar species analyzed, salt-shock induced K+ kinetics were recorded in root meristematic zones (ca. 300 μm from the root tip). These typically exhibited a higher K+ flux compared to the mature zone and apex (Sun et al., 2009a). Na+ kinetics were not examined because of diminished signal/noise ratio from Na+ electrodes in a measuring medium with higher Na+ (Sun et al., 2009a). After exposure to the 100 mM NaCl solution, P. euphratica roots exhibited an instantaneous increase of K+ efflux, reaching maximum values of 500 to 600 pmol cm-2 s-1 (Figure 1). Salt-induced K+ efflux then showed a gradual decline reaching base levels after 30 min of salt treatment (Figure 1). The salt-shocked P. popularis roots showed a trend similar to those of the salt-tolerant poplar species (Figure 1). Pretreatment with NaHS significantly decreased transient K+ efflux induced by salt shock in samples from the two poplar species (Figure 1). The K+ loss inhibition by NaHS was more pronounced in the salt-sensitive P. popularis samples where NaHS exhibited similar inhibitory effects at all test concentrations, (i.e., 25, 50, 200 μM; Figure 1). We adopted a mid-range concentration of 50 μM NaHS for further experiments. This concentration generated clear inhibition of salt-stimulated K+ efflux in both species analyzed.
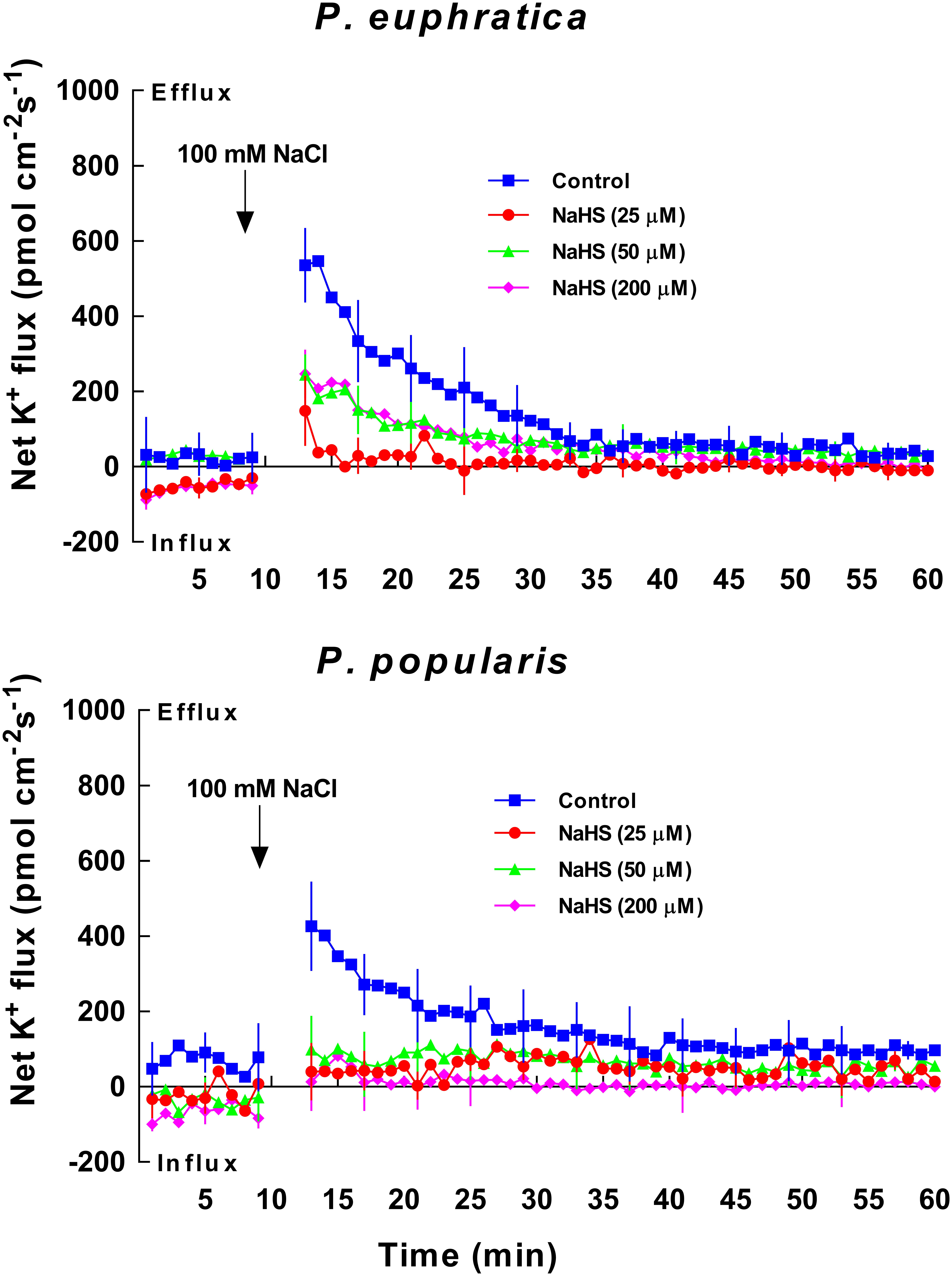
FIGURE 1. Effects of NaHS on NaCl shock-altered transient K+ kinetics within P. euphratica and P. popularis root samples. Young root samples of P. euphratica and P. popularis were equilibrated for 30 min in a basic solution [NaCl (0.1 mM), MgCl2 (0.1 mM), CaCl2 (0.1 mM), and KCl (0.5 mM)] supplemented with or without 25, 50, and 200 μM NaHS. Thereafter, K+ kinetics were recorded at meristems (300 μm from the root tip) for ca. 40 min after NaCl shock. The salt shock (100 mM NaCl) was given by adding acquired amount of NaCl stock (0.2 M, pH 6.0 adjusted with NaOH and HCl) to the measuring solution. Before the NaCl shock, steady-state K+ fluxes were recorded for 10 min. Each point is the mean of five individual plants and bars represent the standard error of the mean.
Effects of NaHS on Steady-State Ion Fluxes Under Short-Term and Long-Term NaCl Stress
Steady-state K+, Na+, and H+ fluxes were examined within root meristems after (ST, 24 h) and (LT, 5 days) exposure to NaCl and NaHS. Samples from different species differed in terms of their ion flux response to agonist and salt treatments (see below).
K+ Flux
Short-term salt stress caused a significant net K+ efflux in roots of P. euphratica and P. popularis, although K+ flux rates varied between the two poplar species (Figure 2). The NaHS (50 μM) markedly reduced salt-induced K+ efflux by 49.4–81.6% in ST salt stressed plants of both species (Figure 2). Under LT salt stress, the NaHS inhibition of K+ loss was evident in NaCl-treated P. popularis samples but not detected in the salt-resistant P. euphratica samples (Figure 2). For P. popularis, NaHS caused a greater reduction in K+ loss in LT-stressed roots than in ST-stressed roots (Figure 2). NaHS also apparently lowered K+ efflux in control samples from P. popularis or even shifted to a net influx in P. euphratica roots (no-salt treatment; Figure 2).
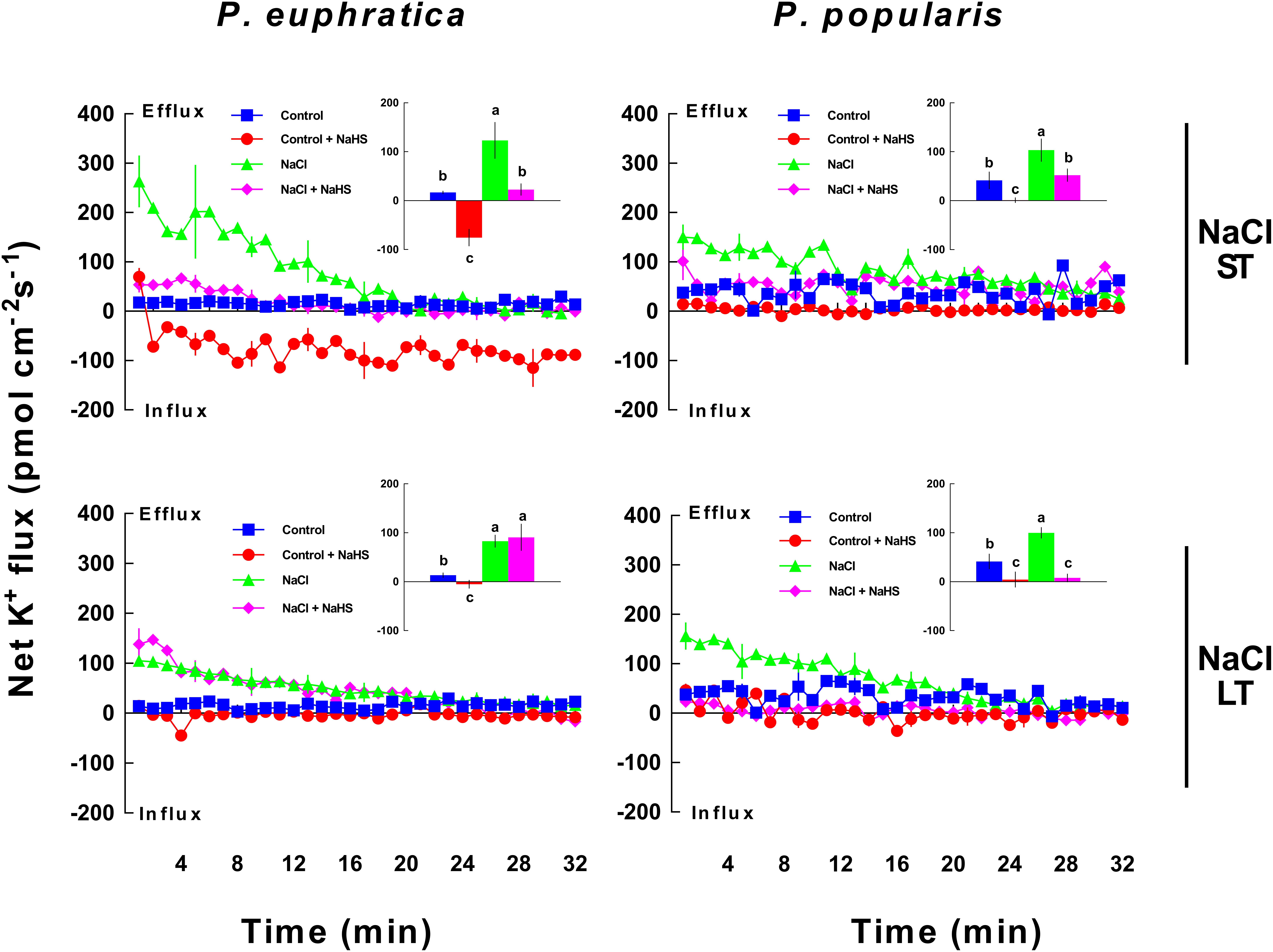
FIGURE 2. Effect of short-term (ST) and long-term (LT) NaCl treatment on K+ fluxes within P. euphratica and P. popularis roots. Steady-state fluxes were measured at meristems (300 μm from the root tip) for ca. 30 min. Plants were treated with short-term (24 h) and long-term (5 days) NaCl (50 mM) supplemented with or without NaHS (50 μM). Control plants were well fertilized but not given additional NaCl or NaHS. Inlays show the mean flux rates of K+ over the first 10 min. Each column shows mean values measured from five individual plants and bars representing the standard error of the mean. Columns labeled with different letters (a, b, and c) denote significant difference at P < 0.05.
Na+ Flux
Steady-state Na+ fluxes were measured in roots of the two poplar species following ST (24 h) and LT (5 days) NaCl and NaHS treatments. Relative to control samples, ST salt stress resulted in an increased Na+ efflux in P. euphratica roots, but not in P. popularis roots (Figure 3). NaHS (50 μM) applications also markedly enhanced the Na+ efflux in P. euphratica and P. popularis roots regardless of control and salt treatment (Figure 3). Na+ stimulation by NaHS was more pronounced in samples of the salt-sensitive P. popularis, in which Na+ efflux increased by 170.1% as compared to the 24.6% increase observed from P. euphratica samples (Figure 3).
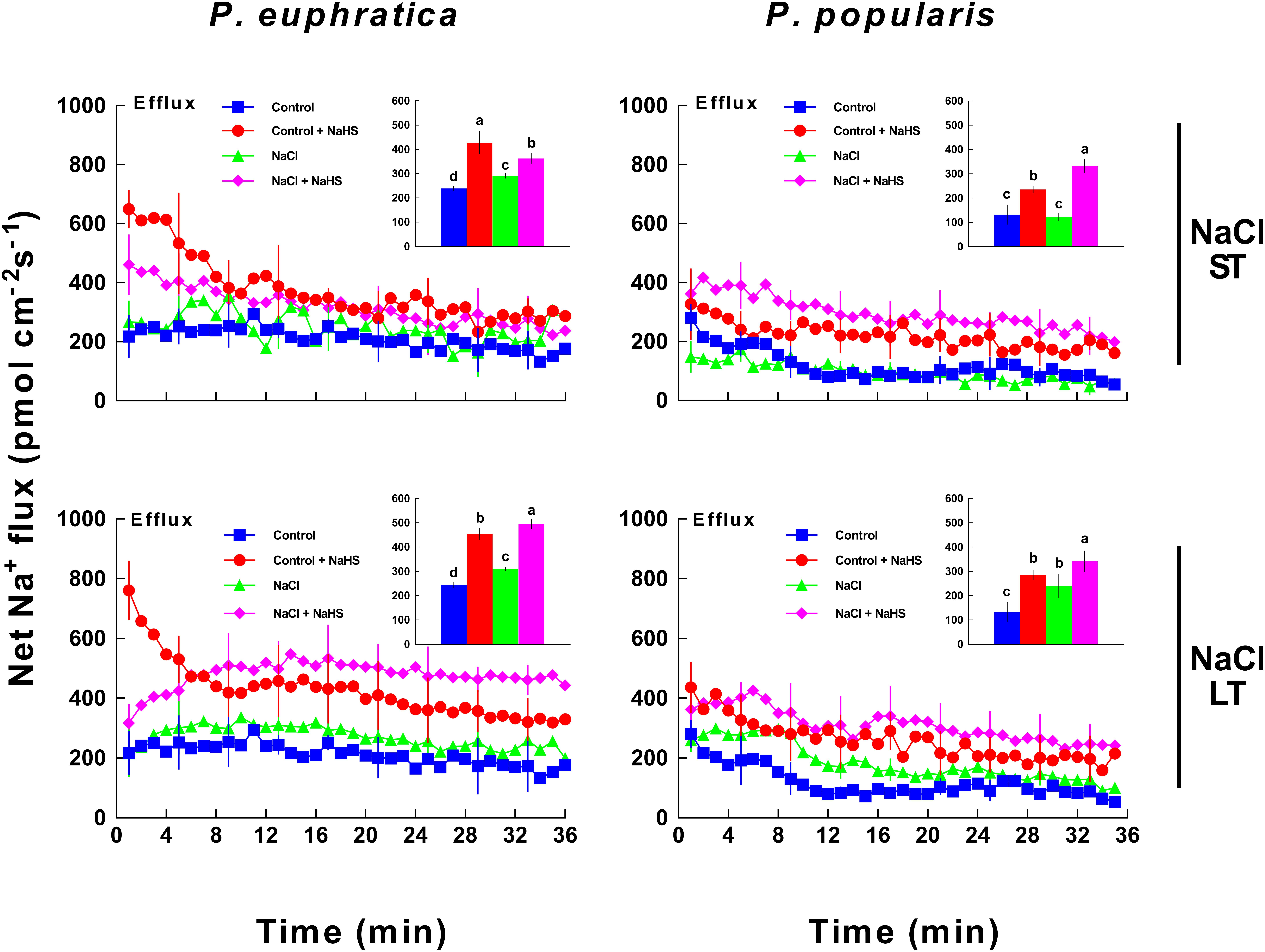
FIGURE 3. Effect of short-term (ST) and long-term (LT) NaCl treatment on Na+ fluxes within P. euphratica and P. popularis roots. Steady-state fluxes were measured in meristems (300 μm from the root tip) for ca. 30 min. Plants were treated with short-term (24 h) and long-term (5 days) NaCl (50 mM) supplemented with or without NaHS (50 μM). Control plants were well fertilized but not given additional NaCl or NaHS. Inserted sections show the mean flux rates of Na+ over the first 10 min. Each column shows mean values measured from five individual plants and bars representing the standard error of the mean. Columns labeled with different letters (a, b, c, and d) denote significant difference at P < 0.05.
A similar trend was observed under LT salt stress. NaHS stimulated Na+ efflux in control and salt stressed root samples of both poplar species, although P. euphratica samples typically displayed higher flux rates within the measured apical region (Figure 3).
H+ Flux
Root tip control samples of P. euphratica exhibited a slight H+ efflux that differed from the evident influx observed in P. popularis control samples (Figure 4). ST salt stress caused an expected shift in H+ efflux toward influx in samples from the salt-resistant poplar species (Figure 4). However, root tip control samples of P. popularis continued to exhibit a net H+ influx, which was not significantly altered by ST salt stress treatments (Figure 4). This finding is consistent with previous results (Sun et al., 2009a). NaHS (50 μM) significantly increased H+ influx in P. popularis with a more pronounced effect in controls than in stressed roots (Figure 4). However, NaHS enhancement was not significant for ST salt stressed P. euphratica samples (Figure 4).
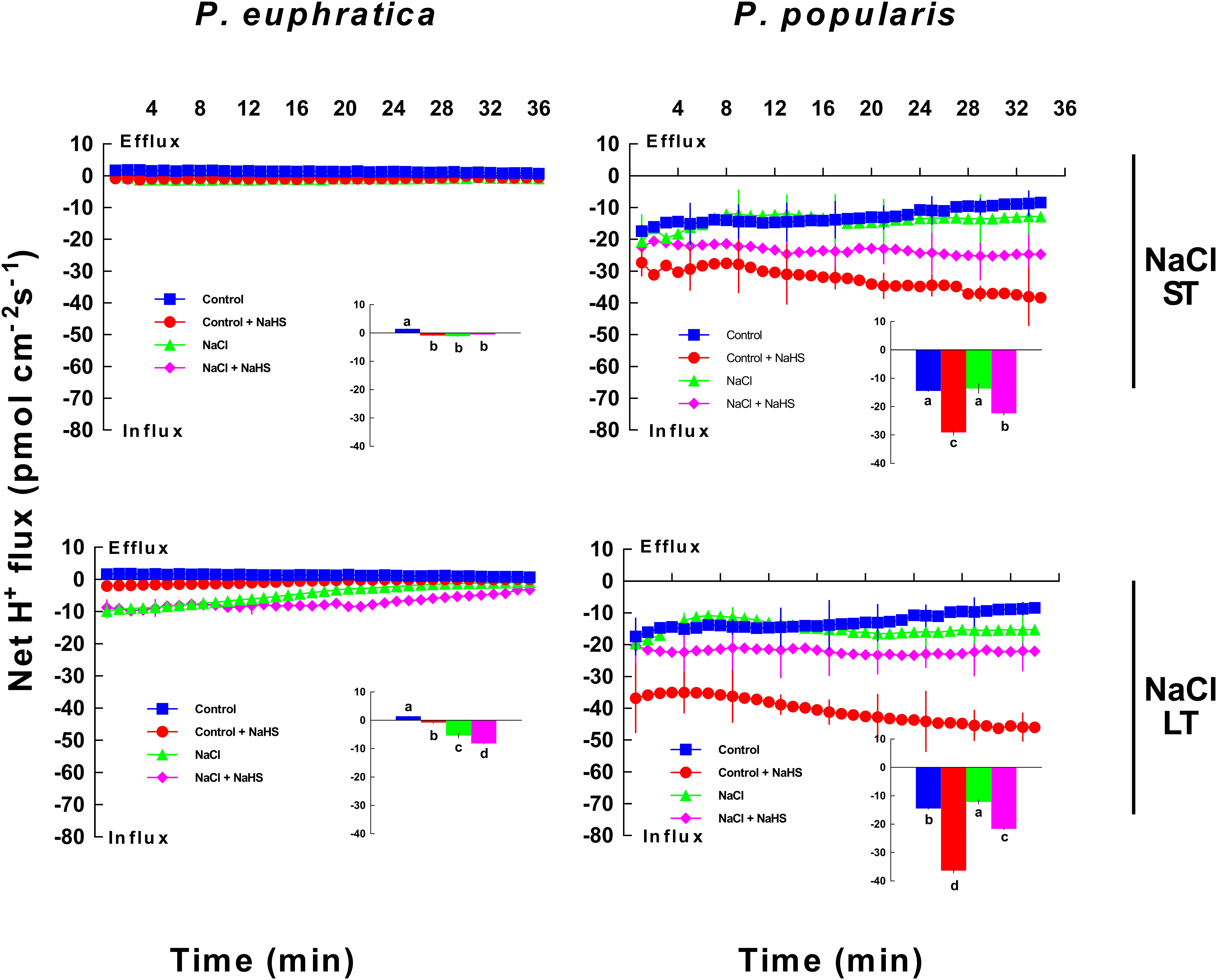
FIGURE 4. Effect of short-term (ST) and long-term (LT) NaCl treatment on H+ fluxes within P. euphratica and P. popularis roots. Steady-state fluxes were measured within root meristems (300 μm from the root tip) for ca. 30 min. Plants were treated with short-term (24 h) and long-term (5 days) NaCl (50 mM) supplemented with or without NaHS (50 μM). Control plants were well fertilized but not given additional NaCl or NaHS. Inlays show the mean flux rates of H+ over the first 10 min. Each column shows mean values measured from five individual plants with bars representing the standard error of the mean. Columns labeled with different letters (a, b, c, and d) denote significant difference at P < 0.05.
Under LT salt stress, NaHS enhanced H+ influx in root samples of both species (Figure 4). A similar effect was also observed for control samples, where NaHS caused the expected shift in H+ efflux to influx in the salt-resistant poplar species, while it enhanced H+ influx in the salt-sensitive poplar species (Figure 4). For P. popularis, the effect of NaHS on H+ influx was more pronounced in no-salt roots compared to LT-stressed ones, similar to the findings in the ST treatment (24 h, Figure 4).
Effects of PM Inhibitors on Root K+ and Na+ Fluxes Under NaHS and LT Salt Stress Treatments
K+ Flux
In our study, TEA was employed to block the PM K+ channels while sodium orthovanadate was used to inhibit H+-ATPase in the PM (Sun et al., 2009b; Lu et al., 2013). Inhibitor experiments indicated that the K+ channel blocker (TEA) did not significantly inhibit the salt-induced K+ efflux in the LT salt stressed P. euphratica root samples, regardless of the presence or absence of NaHS (Figure 5). In the roots of the salt-sensitive poplar species, TEA reduced K+ efflux caused by NaCl (27.0%, Figure 5). However, TEA inhibition of K+ efflux in NaHS-treated root samples was not as pronounced as that observed in root samples not subjected to NaHS treatment (Figure 5). In the former samples, NaHS had markedly reduced K+ efflux under salt stress (Figure 5).
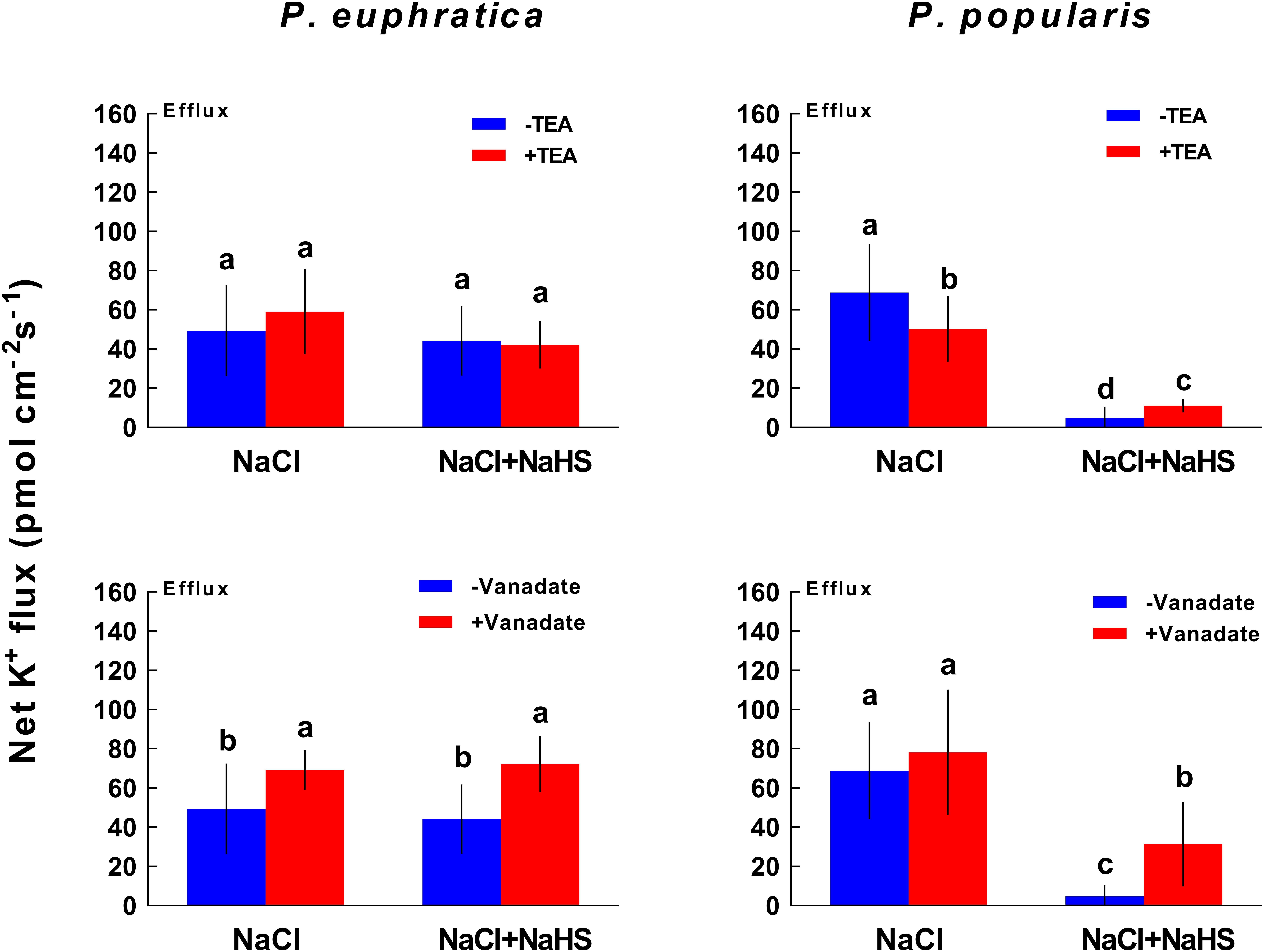
FIGURE 5. Effect of TEA or sodium orthovanadate on K+ fluxes in long-term NaCl-treated roots of P. euphratica and P. popularis. Prior to flux measurements, salinized roots were subjected to TEA (20 mM) or sodium orthovanadate (500 μM) treatments for 30 min. Steady state K+ fluxes were then monitored for another 30 min. Each column shows mean values from five individual plants and bars representing the standard error of the mean. Columns labeled with different letters (a, b, and c) denote significant difference at P < 0.05.
In contrast to TEA, sodium vanadate accelerated K+ efflux in LT salt stressed root samples of P. euphratica and P. popularis, regardless of the presence or absence of NaHS (Figure 5). This indicates that NaHS reduced K+ efflux via the activated H+-ATPase in the PM, which in turn restricted K+ loss through inhibiting depolarization-activated KORCs for both species analyzed (Sun et al., 2009b).
Na+ Flux
To characterize the effects of PM transport inhibitors on Na+ fluxes, we used LT salt-stressed roots of P. euphratica and P. popularis, which exhibited an evident increase in Na+/H+ exchange under NaHS treatment (Figure 3). Figure 6 shows NaHS (50 μM) enhanced Na+ efflux in LT salt stressed samples. However, amiloride (an inhibitor of Na+/H+ exchange) or sodium orthovanadate (the inhibitor of PM H+-ATPase) significantly inhibited Na+ efflux in salinized roots in the presence or absence of NaHS (Figure 6). The effects of amiloride were not pronounced in NaCl-treated P. euphratica roots (Figure 6).
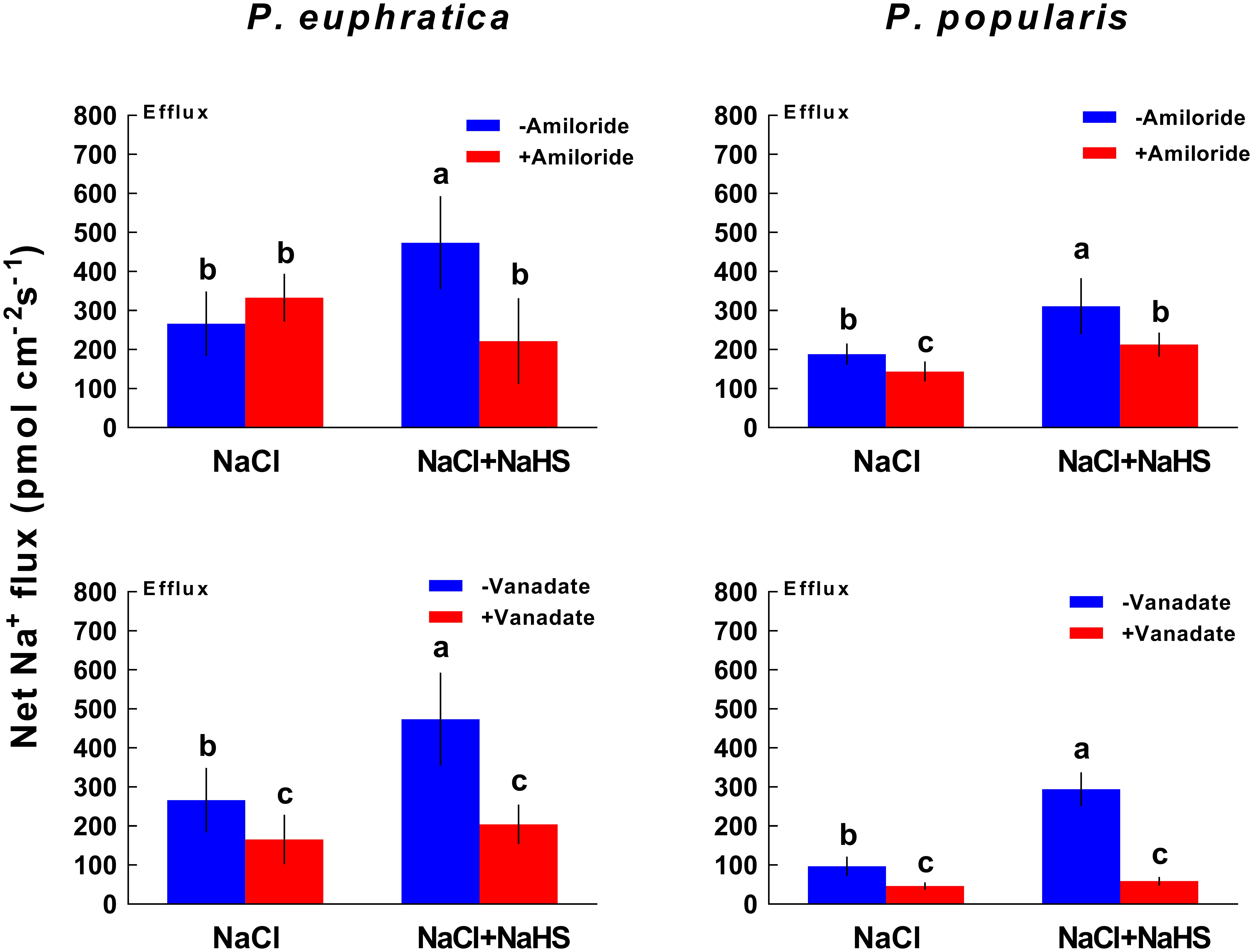
FIGURE 6. Effect of amiloride or sodium orthovanadate on Na+ fluxes in long-term NaCl-treated roots of P. euphratica and P. popularis. Prior to flux measurements, salinized roots were subjected to amiloride (50 μM) or sodium orthovanadate (500 μM) treatments for 30 min. Steady state Na+ fluxes were then monitored for another 30 min. Each column shows mean values from five individual plants and bars representing the standard error of the mean. Columns labeled with different letters (a, b, and c) denote significant difference at P < 0.05.
Discussion
H2S Mediates K+/Na+ Homeostasis
The capacity to retain K+ and Na+ homeostasis is critical for poplar species living in saline soils (Chen et al., 2001, 2003). This study showed that H2S regulates K+/Na+ homeostasis in roots of the salt-resistant poplar, P. euphratica and those of the salt-sensitive poplar, P. popularis (Figures 1–3). H2S treatments similarly increased the ratio of K+ to Na+ in various herbaceous species, including M. sativa (Wang et al., 2012; Lai et al., 2014), Fragaria × ananassa (Christou et al., 2013), Hordeum vulgare (Chen et al., 2015), and Triticum aestivum (Deng et al., 2016). Our NMT data revealed that maintenance of root K+/Na+ homeostasis in NaHS-treated P. euphratica and P. popularis accounted for greater Na+ extrusion and lower K+ loss under NaCl stress.
Na+ Homeostasis
NaHS increased net Na+ efflux in roots of two poplar species under ST and LT salt stress (Figure 3). Moreover, salt stressed P. euphratica and P. popularis samples exhibited a net influx of H+ in NaHS-treated roots (Figure 4). In salt-stressed poplars roots, the H2S-stimulated H+ uptake corresponding to the Na+ efflux suggests that Na+ extrusion primarily results from active Na+/H+ antiport across the PM. This finding resembles those reported by our previous studies of salinized roots belonging to P. euphratica (Sun et al., 2009a,b; Zhao et al., 2016) and mangrove species such as Bruguiera gymnorhiza (Lu et al., 2013), Kandelia obovata and Aegiceras corniculatum (Lang et al., 2014). Pharmacological evidence showed that inhibitors of PM H+-ATPase (sodium orthovanadate) and Na+/H+ antiporter (amiloride) decreased the H2S-stimulated Na+ efflux in LT salt stressed plants (Figure 6). Collectively, the agonist and antagonist data revealed that the H2S enhancement of Na+ efflux results from increased activity of Na+/H+ antiport in NaCl-treated roots of P. euphratica and P. popularis. The Na+/H+ antiport system in the PM (Na+/H+ antiporter and H+-ATPase) contributed to the Na+ extrusion in NaCl stressed roots (Sun et al., 2009a,b). H+-ATPase activity and the H+/ATP coupling ratio of PMs isolated from cucumber roots were enhanced by NaHS treatments (Janicka et al., 2017). Furthermore, NaHS (100 μM) stimulated protein abundance of PM H+-ATPase and the transcription of HvHA1 and HvSOS1 in barley roots (Chen et al., 2015). Therefore, the H2S-activated H+-pumps in the PM maintain electrochemical H+ gradients, which in turn promote secondary Na+/H+ antiport across root PMs for both poplar species (Blumwald et al., 2000; Zhu, 2003). Notably, H2S induced enhancement of Na+/H+ was more pronounced in the salt-sensitive poplar relative to the salt-resistant P. euphratica (Figures 3, 4). The salt-resistant poplar exhibited typically a higher capacity for Na+/H+ exchange in the absence of exogenous NaHS (Figures 3, 4). This indicates that its PM Na+/H+ antiport system extrudes Na+ more effectively than that of the salt-sensitive poplar (Sun et al., 2009a,b). We have shown that the salt signaling network of H2O2, cytosolic Ca2+, extracellular ATP (eATP), and NO help mediate Na+ extrusion in P. euphratica (Sun et al., 2012; Zhang et al., 2015).
In this study, an apparent H+ efflux which indicates activity of H+-ATPase was not recorded in the NaHS-treated roots (Figure 4). Instead, H2S enlarged the influx of H+ in P. popularis roots (Figure 4). The H2S-enhanced H+ influx corresponding to Na+ efflux resulted from the Na+/H+ antiport, which was promoted by PM H+-ATPase (Figures 3, 4, 6). However, the NMT data only show a net flux of H+ across the PM in root cells that undergoing Na+/H+ exchange, instead of the unidirectional flux (Sun et al., 2009b). Using NMT microelectrodes, a net H+ influx was usually recorded in roots of salt-resistant poplar and mangroves after ST (24 h) and LT salt stress (from 7 to 21 days, Sun et al., 2009a,b; Lu et al., 2013). This indicates that the PM H+-pumps had already established a steep H+ gradient upon NaCl exposure, thus promoting the Na+/H+ antiport across the PM in these salt-resistant species. In accordance, H+ efflux promoted by the PM H+-ATPase was observed in salt shock treatment (40 min) in P. euphratica roots (Sun et al., 2009b). Therefore, the H2S-stimulated Na+/H+ antiport was presumably due to the upward-regulated H+-ATPases. Moreover, the effect of NaHS on H+ influx is more pronounced in no-salt P. popularis roots than in NaCl-treated ones (Figure 4). This is presumably due to the salt inhibition of NaCl on Na+/H+ antiport system in this salt-sensitive poplar. We have previously shown that NaCl treatment had an inhibitory effect on the Na+/H+ antiport (Sun et al., 2009a,b) and H+-ATPase activity for P. popularis (Ma et al., 2010).
K+ Homeostasis
K+ flux profiles showed that H2S contributed to K+ homeostasis under NaCl stress in poplars. Salt shock, as well as short- and long-term salt stress resulted in a significant net K+ loss in both the salt-resistant P. euphratica and the salt-sensitive P. popularis (Figures 1, 2). The results are consistent with previous studies of these species (Sun et al., 2009b). Together, these show that salt-induced K+ efflux is mediated by KORCs and NSCCs in poplar (Sun et al., 2009a,b) and herbaceous species (Shabala et al., 2005, 2006; Chen et al., 2007; Shabala and Cuin, 2008). H2S significantly reduced K+ loss evoked by ST and LT salt stress applied to the two species (Figure 2). H2S can similarly enhance salt tolerance by preventing K+ loss in salt-treated M. sativa (Lai et al., 2014) and barley (Chen et al., 2015). A more pronounced reduction of K+ efflux induced by NaHS was observed in the LT salt stressed P. popularis roots compared to those of P. euphratica (Figure 2). The high flux rates of K+ in P. euphratica roots reveal the high concentration of K+ sources under LT salt stress (Sun et al., 2009b). We previously showed that relative to salt-sensitive species, P. euphratica had a higher capacity to maintain K+ uptake and transport under saline conditions (Chen et al., 2001, 2003). The NaCl-induced K+ efflux was inhibited by TEA in P. popularis, but significantly increased by sodium vanadate for both poplars studied under conditions of LT salt stress and NaHS treatment (Figure 5). This indicates that NaCl-induced K+ loss is through depolarization-activated K+ channels (Chen et al., 2007; Shabala and Cuin, 2008). We infer that the H2S-decreased K+ loss likely results from activated H+-pumping activity in the salinized roots. The activated H+-ATPase may inhibit the NaCl-depolarized membrane potential, thus lessening K+ flow through PM-localized DA-KORCs/NSCCs in P. euphratica and P. popularis roots (Sun et al., 2009b). Furthermore, the activated H+-ATPase might contribute to the H2S-induced K+ influx in non-salinized roots, in particular P. euphratica (Figure 2). NaHS activated H+-ATPase in the PM, which could produce a steep H+ gradient, thus promoting the entry of K+ via hyperpolarization-activated potassium channels (Ilan et al., 1996; Sun et al., 2009b).
Species Difference in the Response to Salt and H2S
Our data showed that the response to salt and H2S varied between P. euphratica and P. popularis. NaCl caused a remarkable K+ loss but a less Na+ extrusion in the salt-sensitive poplar (Figures 2, 3). Compared to P. popularis, the salt-resistant P. euphratica exhibited a pronounced effect to extrude Na+ and to retain K+ under salt stress (Figures 2, 3). This is mainly due to its active PM Na+/H+ antiporters and H+ pumps in the PM (Sun et al., 2009a,b, 2010a,b). It is noting to find that H2S was more effective to assist P. popularis to retain K+/Na+ homeostasis under NaCl stress, as compared to the salt-resistant poplar. This is similar to our previous finding that Ca2+ application showed a more pronounced beneficial effect for salt-sensitive poplar to retain ionic homeostasis (Sun et al., 2009b). The difference in the response to salt between contrasting poplars is probably related to their differences in the production of salt signals. The salt-sensitive species, P. popularis, is insensitive to the salinity and unable to produce stress signals, such as H2O2 and NO, leading to uncontrolled conditions of ion toxicity and oxidative damage (Sun et al., 2010b). In contrast to P. popularis, NaCl salinity caused a rapid increase of H2O2 and NO in P. euphratica cells (Sun et al., 2010b). The salt-elicited H2O2 and NO enable P. euphratica cells to regulate ionic and ROS (reactive oxygen species) homeostasis under salinity stress in the longer term (Sun et al., 2010b). In this study, it is likely that the signal molecule H2S contribute to K+/Na+ homeostasis control in P. popularis through activations of both Na+/H+ antiporter and H+-ATPase in the PM. The beneficial effect of H2S was less pronounced in the salt-resistant poplar, due to the abundance of endogenous salt signals. The salt-elicited endogenous signals, such H2O2, cytosolic Ca2+, eATP, and NO, mediated ionic homeostasis in P. euphratica (Sun et al., 2010a,b, 2012; Zhang et al., 2015; Zhao et al., 2016), thus weakening the effect of exogenously applied H2S.
Conclusion
NaHS treatments increased P. euphratica and P. popularis capacity to maintain root K+/Na+ homeostasis under saline conditions. NaHS application effectively limited the NaCl-induced K+ loss and simultaneously increased Na+ extrusion capacity. Beneficial effects of H2S are presumably due to the upward-regulated H+-ATPases, which (1) sustain the H+ gradient that drives the Na+/H+ antiport across the PM, and (2) preserve a less-depolarized membrane potential, which restricts K+ loss through depolarization-activated KORCs and NSCCs in the PM. As a result, K+/Na+ homeostasis in root cells is maintained in P. euphratica and P. popularis subjected to salinized conditions. The effect of H2S was more pronounced for roots of the salt-sensitive P. popularis subjected to higher NaCl conditions. This indicates that H2S has considerable potential for improving salt tolerance in salt-sensitive trees. The molecular mechanisms resulting in different signal transduction responses for both species require further investigation.
Author Contributions
NZ, HpZ, and SC conceived of the original research project and selected methods. SC supervised the experiments. NZ, HpZ, HlZ, JS, CD, and YZ performed most of the experiments. JZ, RZ, XZ, and CL provided technical assistance to NZ, HpZ, HlZ, JS, CD, and YZ. NZ and HpZ designed the experiments and analyzed the data. NZ and HpZ refined the project and wrote the manuscript with contributions from all the authors. SL and SC revised the writing. All authors have read and approved the manuscript.
Funding
This research was supported jointly by National Natural Science Foundation of China (Grant Nos. 31770643 and 31570587), Beijing Natural Science Foundation (Grant Nos. 6182030 and 6172024), the Research Project of the Chinese Ministry of Education (Grant No. 113013A), the Program of Introducing Talents of Discipline to Universities (111 Project, Grant No. B13007), and Fundamental Research Funds for the Central Universities (2017ZY07).
Conflict of Interest Statement
The authors declare that the research was conducted in the absence of any commercial or financial relationships that could be construed as a potential conflict of interest.
Footnotes
References
Apse, M. P., Sottosanto, J. B., and Blumwald, E. (2003). Vacuolar cation/H+ exchange, ion homeostasis, and leaf development are altered in a T-DNA insertional mutant of AtNHX1, the Arabidopsis vacuolar Na+/H+ antiporter. Plant J. 36, 229–239. doi: 10.1046/j.1365-313X.2003.01871.x
Blumwald, E., Aharon, G. S., and Apse, M. P. (2000). Sodium transport in plant cells. Biochim. Biophys. Acta 1465, 140–151. doi: 10.1016/S0005-2736(00)00135-8
Chen, J., Wang, W.-H., Wu, F.-H., He, E.-M., Liu, X., Shangguan, Z.-P., et al. (2015). Hydrogen sulfide enhances salt tolerance through nitric oxide-mediated maintenance of ion homeostasis in barley seedling roots. Sci. Rep. 5, 12516. doi: 10.1038/srep12516
Chen, J., Wu, F., Wang, W., Zheng, C., Lin, G., Dong, X., et al. (2011). Hydrogen sulfide enhances photosynthesis through promoting chloroplast biogenesis, photosynthetic enzyme expression, and thiol redox modification in Spinacia oleracea seedlings. J. Exp. Bot. 62, 4481–4493. doi: 10.1093/jxb/err145
Chen, S., Li, J., Fritz, E., Wang, S., and Hüttermann, A. (2002). Sodium and chloride distribution in roots and transport in three poplar genotypes under increasing NaCl stress. For. Ecol. Manag. 168, 217–230. doi: 10.1016/S0378-1127(01)00743-5
Chen, S., Li, J., Wang, S., Fritz, E., Hüttermann, A., and Altman, A. (2003). Effects of NaCl on shoot growth, transpiration, ion compartmentation, and transport in regenerated plants of Populus euphratica and Populus tomentosa. Can. J. For. Res. 33, 967–975. doi: 10.1139/x03-066
Chen, S., Li, J., Wang, S., Hüttermann, A., and Altman, A. (2001). Salt, nutrient uptake and transport, and ABA of Populus euphratica; a hybrid in response to increasing soil NaCl. Trees 15, 186–194. doi: 10.1007/s004680100091
Chen, Z., Pottosin, I. I., Cuin, T. A., Fuglsang, A. T., Tester, M., Jha, D., et al. (2007). Root plasma membrane transporters controlling K+/Na+ homeostasis in salt stressed barley. Plant Physiol. 145, 1714–1725. doi: 10.1104/pp.107.110262
Christou, A., Manganaris, G. A., Papadopoulos, I., and Fotopoulos, V. (2013). Hydrogen sulfide induces systemic tolerance to salinity and non-ionic osmotic stress in strawberry plants through modification of reactive species biosynthesis and transcriptional regulation of multiple defence pathways. J. Exp. Bot. 64, 1953–1966. doi: 10.1093/jxb/ert055
Cuin, T. A., Miller, A. J., Laurie, S. A., and Leigh, R. A. (2003). Potassium activities in cell compartments of salt-grown barley leaves. J. Exp. Bot. 54, 657–661. doi: 10.1093/jxb/erg072
da Silva, C. J., Fontes, E. P. B., and Modolo, L. V. (2017). Salinity-induced accumulation of endogenous H2S and NO is associated with modulation of the antioxidant and redox defense systems in Nicotiana tabacum L. cv. Havana. Plant Sci. 256, 148–159. doi: 10.1016/j.plantsci.2016.12.011
da-Silva, C. J., and Modolo, L. V. (2017). Hydrogen sulfide: a new endogenous player in an old mechanism of plant tolerance to high salinity. Acta Bot. Brasilica 32, 150–160. doi: 10.1590/0102-33062017abb0229
da-Silva, C. J., Mollica, D. C. F., Vicente, M. H., Peres, L. E. P., and Modolo, L. V. (2018). NO, hydrogen sulfide does not come first during tomato response to high salinity. Nitric Oxide 76, 164–173. doi: 10.1016/j.niox.2017.09.008
Deng, Y. Q., Bao, J., Yuan, F., Liang, X., Feng, Z. T., and Wang, B. S. (2016). Exogenous hydrogen sulfide alleviates salt stress in wheat seedlings by decreasing Na+ content. Plant Growth Regul. 79, 391–399. doi: 10.1007/s10725-015-0143-x
Fang, H., Liu, Z., Jin, Z., Zhang, L., Liu, D., and Pei, Y. (2016). An emphasis of hydrogen sulfide-cysteine cycle on enhancing the tolerance to chromium stress in Arabidopsis. Environ. Pollut. 213, 870–877. doi: 10.1016/j.envpol.2016.03.035
García-Mata, C., and Lamattina, L. (2010). Hydrogen sulphide, a novel gasotransmitter involved in guard cell signalling. New Phytol. 188, 977–984. doi: 10.1111/j.1469-8137.2010.03465.x
He, C., Yan, J., Shen, G., Fu, L., Holaday, A. S., Auld, D., et al. (2005). Expression of an Arabidopsis vacuolar sodium/proton antiporter gene in cotton improves photosynthetic performance under salt conditions and increases fiber yield in the field. Plant Cell Physiol. 46, 1848–1854. doi: 10.1093/pcp/pci201
Ilan, N., Schwartz, A., and Moran, N. (1996). External protons enhance the activity of the hyperpolarization-activated K channels in guard cell protoplasts of Vicia faba. J. Membr. Biol. 154, 169–181. doi: 10.1007/s002329900142
Janicka, M., Reda, M., Czyżewska, K., and Kabała, K. (2017). Involvement of signalling molecules NO, H2O2 and H2S in modification of plasma membrane proton pump in cucumber roots subjected to salt or low temperature stress. Funct. Plant Biol. 45, 428–439. doi: 10.1071/FP17095
Jin, Z., and Pei, Y. (2016). Hydrogen sulfide: the shutter button of stomata in plants. Sci. China Life Sci. 59, 1187–1188. doi: 10.1007/s11427-016-0265-3
Jin, Z., Wang, Z., Ma, Q., Sun, L., Zhang, L., Liu, Z., et al. (2017). Hydrogen sulfide mediates ion fluxes inducing stomatal closure in response to drought stress in Arabidopsis thaliana. Plant Soil 419, 141–152. doi: 10.1007/s11104-017-3335-5
Lai, D., Mao, Y., Zhou, H., Li, F., Wu, M., Zhang, J., et al. (2014). Endogenous hydrogen sulfide enhances salt tolerance by coupling the reestablishment of redox homeostasis and preventing salt-induced K+ loss in seedlings of Medicago sativa. Plant Sci. 225, 117–129. doi: 10.1016/j.plantsci.2014.06.006
Lang, T., Sun, H., Li, N., Lu, Y., Shen, Z., Jing, X., et al. (2014). Multiple signaling networks of extracellular ATP, hydrogen peroxide, calcium, and nitric oxide in the mediation of root ion fluxes in secretor and non-secretor mangroves under salt stress. Aquat. Bot. 119, 33–43. doi: 10.1016/j.aquabot2014.06.009
Li, L., Wang, Y., and Shen, W. (2012). Roles of hydrogen sulfide and nitric oxide in the alleviation of cadmium-induced oxidative damage in alfalfa seedling roots. Biometals 25, 617–631. doi: 10.1007/s10534-012-9551-9
Lisjak, M., Srivastava, N., Teklic, T., Civale, L., Lewandowski, K., Wilson, I., et al. (2010). A novel hydrogen sulfide donor causes stomatal opening and reduces nitric oxide accumulation. Plant Physiol. Biochem. 48, 931–935. doi: 10.1016/j.plaphy.2010.09.016
Lu, Y., Li, N., Sun, J., Hou, P., Jing, X., Deng, S., et al. (2013). Exogenous hydrogen peroxide, nitric oxide and calcium mediate root ion fluxes in two non-secretor mangrove species subjected to NaCl stress. Tree Physiol. 33, 81–95. doi: 10.1093/treephys/tps119
Ma, X., Deng, L., Li, J., Zhou, X., Li, N., Zhang, D., et al. (2010). Effect of NaCl on leaf H+-ATPase and the relevance to salt tolerance in two contrasting poplar species. Trees 24, 597–607. doi: 10.1007/s00468-010-0430-0
Maathuis, F. J. (2006). The role of monovalent cation transporters in plant responses to salinity. J. Exp. Bot. 57, 1137–1147. doi: 10.1093/jxb/erj001
Maathuis, F. J. M., and Sanders, D. (2001). Sodium uptake in Arabidopsis thaliana roots is regulated by cyclic nucleotides. Plant Physiol. 127, 1617–1625. doi: 10.1104/pp.127.4.1617
Ottow, E. A., Brinker, M., Teichmann, T., Fritz, E., Kaiser, W., Brosché, M., et al. (2005). Populus euphratica displays apoplastic sodium accumulation, osmotic adjustment by decreases in calcium and soluble carbohydrates, and develops leaf succulence under salt stress. Plant Physiol. 139, 1762–1772. doi: 10.1104/pp.105.069971
Qiao, Z., Jing, T., Jin, Z., Liang, Y., Zhang, L., Liu, Z., et al. (2016). CDPKs enhance Cd tolerance through intensifying H2S signal in Arabidopsis thaliana. Plant Soil 398, 99–110. doi: 10.1007/s11104-015-2643-x
Shabala, L., Cuin, T. A., Newman, I. A., and Shabala, S. (2005). Salinity-induced ion flux patterns from the excised roots of Arabidopsis sos mutants. Planta 222, 1041–1050. doi: 10.1007/s00425-005-0074-2
Shabala, S. (2000). Ionic and osmotic components of salt stress specifically modulate net ion fluxes from bean leaf mesophyll. Plant Cell Environ. 23, 825–837. doi: 10.1046/j.1365-3040.2000.00606.x
Shabala, S., and Cuin, T. A. (2008). Potassium transport and plant salt tolerance. Physiol. Plant. 133, 651–669. doi: 10.1111/j.1399-3054.2007.01008.x
Shabala, S., Demidchik, V., Shabala, L., Cuin, T. A., Smith, S. J., Miller, A. J., et al. (2006). Extracellular Ca2+ ameliorates NaCl-induced K+ loss from Arabidopsis root and leaf cells by controlling plasma membrane K+-permeable channels. Plant Physiol. 141, 1653–1665. doi: 10.1104/pp.106.082388
Shi, H., Ishitani, M., Kim, C., and Zhu, J. (2000). The Arabidopsis thaliana salt tolerance gene SOS1 encodes a putative Na+/H+ antiporter. Proc. Natl. Acad. Sci. U.S.A. 97, 6896–6901. doi: 10.1073/pnas.120170197
Shi, Q., Ding, F., Wang, X., and Wei, M. (2007). Exogenous nitric oxide protect cucumber roots against oxidative stress induced by salt stress. Plant Physiol. Biochem. 45, 542–550. doi: 10.1016/j.plaphy
Sun, J., Chen, S. L., Dai, S. X., Wang, R. G., Li, N. Y., Shen, X., et al. (2009a). NaCl-induced alternations of cellular and tissue ion fluxes in roots of salt-resistant and salt-sensitive poplar species. Plant Physiol. 149, 1141–1153. doi: 10.1104/pp.108.129494
Sun, J., Dai, S. X., Wang, R. G., Chen, S. L., Li, N. Y., Zhou, X. Y., et al. (2009b). Calcium mediates root K+/Na+ homeostasis in poplar species differing in salt tolerance. Tree Physiol. 29, 1175–1186. doi: 10.1093/treephys/tpp048
Sun, J., Wang, M. J., Ding, M. Q., Deng, S. R., Liu, M. Q., Lu, C. F., et al. (2010a). H2O2 and cytosolic Ca2+ signals triggered by the PM H+-coupled transport system mediate K+/Na+ homeostasis in NaCl-stressed Populus euphratica cells. Plant Cell Environ. 33, 943–958. doi: 10.1111/j.1365-3040.2010.02118.x
Sun, J., Li, L., Liu, M., Wang, M., Ding, M., Deng, S., et al. (2010b). Hydrogen peroxide and nitric oxide mediate K+/Na+ homeostasis and antioxidant defense in NaCl-stressed callus cells of two contrasting poplars. Plant Cell Tissue Org. Cult. 103, 205–215. doi: 10.1007/s11240-010-9768-7
Sun, J., Wang, R., Zhang, X., Yu, Y., Zhao, R., Li, Z., et al. (2013). Hydrogen sulfide alleviates cadmium toxicity through regulations of cadmium transport across the plasma and vacuolar membranes in Populus euphratica cells. Plant Physiol. Biochem. 65, 67–74. doi: 10.1016/j.plaphy.2013.01.003
Sun, J., Zhang, X., Deng, S., Zhang, C. L., Wang, M. J., Ding, M. Q., et al. (2012). Extracellular ATP signaling is mediated by H2O2 and cytosolic Ca2+ in the salt response of Populus euphratica cells. PLoS One 7:e53136. doi: 10.1371/journal.pone.0053136
Wang, B., Shi, L., Li, Y., and Zhang, W. (2010). Boron toxicity is alleviated by hydrogen sulfide in cucumber (Cucumis sativus L.) seedlings. Planta 231, 1301–1309. doi: 10.1007/s00425-010-1134-9
Wang, R., Chen, S., Deng, L., Fritz, E., Hüttermann, A., and Polle, A. (2007). Leaf photosynthesis, fluorescence response to salinity and the relevance to chloroplast salt compartmentation and anti-oxidative stress in two poplars. Trees 21, 581–591. doi: 10.1007/s00468-007-0154-y
Wang, R., Chen, S., Ma, H., Liu, L., Li, H., Weng, H., et al. (2006). Genotypic differences in antioxidative stress and salt tolerance of three poplars under salt stress. Front. For. China 1:82. doi: 10.1007/s11461-005-0019-8
Wang, R., Chen, S., Zhou, X., Shen, X., Deng, L., Zhu, H., et al. (2008). Ionic homeostasis and reactive oxygen species control in leaves and xylem sap of two poplars subjected to NaCl stress. Tree Physiol. 28, 947–957. doi: 10.1093/treephys/28.6.947
Wang, Y., Li, L., Cui, W., Xu, S., Shen, W., and Wang, R. (2012). Hydrogen sulfide enhances alfalfa (Medicago sativa) tolerance against salinity during seed germination by nitric oxide pathway. Plant Soil 351, 107–119. doi: 10.1007/s11104-011-0936-2
Zhang, H., Hu, L., Hu, K., He, Y., Wang, S., and Luo, J. (2008). Hydrogen sulfide promotes wheat seed germination and alleviates oxidative damage against copper stress. J. Integr. Plant Biol. 50, 1518–1529. doi: 10.1111/j.1744-7909.2008.00769.x
Zhang, H., Hu, S., Zhang, Z., Hu, L., Jiang, C., Wei, Z., et al. (2011). Hydrogen sulfide acts as a regulator of flower senescence in plants. Postharvest Biol. Technol. 60, 251–257. doi: 10.1016/j.postharvbio
Zhang, H., Tan, Z., Hu, L., Wang, S., Luo, J., and Jones, R. L. (2010a). Hydrogen sulfide alleviates aluminum toxicity in germinating wheat seedlings. J. Integr. Plant Biol. 52, 556–567. doi: 10.1111/j.1744-7909.2010.00946.x
Zhang, H., Jiao, H., Jiang, C., Wang, S., Wei, Z., Luo, J., et al. (2010b). Hydrogen sulfide protects soybean seedlings against drought-induced oxidative stress. Acta Physiol. Plant 32, 849–857. doi: 10.1007/s11738-010-0469-y
Zhang, H., Tang, J., Liu, X.-P., Wang, Y., Yu, W., Peng, W.-Y., et al. (2009a). Hydrogen sulfide promotes root organogenesis in Ipomoea batatas, Salix matsudana and Glycine max. J. Integr. Plant Biol. 51, 1086–1094. doi: 10.1111/j.1744-7909.2009.00885.x
Zhang, H., Ye, Y., Wang, S., Luo, J., Tang, J., and Ma, D. (2009b). Hydrogen sulfide counteracts chlorophyll loss in sweetpotato seedling leaves and alleviates oxidative damage against osmotic stress. Plant Growth Regul. 58, 243–250. doi: 10.1007/s10725-009-9372-1
Zhang, X., Shen, Z., Sun, J., Yu, Y., Deng, S., Li, Z., et al. (2015). NaCl elicited, vacuolar Ca2+ release facilitates prolonged cytosolic Ca2+ signaling in the salt response of Populus euphratica cells. Cell Calcium 57, 348–365. doi: 10.1016/j.ceca.2015.03.0010
Zhang, Y., Wang, L., Liu, Y., Zhang, Q., Wei, Q., and Zhang, W. (2006). Nitric oxide enhances salt tolerance in maize seedlings through increasing activities of proton-pump and Na+/H+ antiport in the tonoplast. Planta 224, 545–555. doi: 10.1007/s00425-006-0242-z
Zhao, N., Wang, S., Ma, X., Zhu, H., Sa, G., Sun, J., et al. (2016). Extracellular ATP mediates cellular K+/Na+ homeostasis in two contrasting poplar species under NaCl stress. Trees 30, 825–837. doi: 10.1007/s00468-015-1324-y
Zhao, W., Zhang, J., Lu, Y., and Wang, R. (2001). The vasorelaxant effect of H2S as a novel endogenous gaseous KATP channel opener. EMBO J. 20, 6008–6016. doi: 10.1093/emboj/20.21.6008
Zhu, J. K. (2001). Plant salt tolerance. Trends Plant Sci. 6, 66–71. doi: 10.1016/S1360-1385(00)01838-0
Keywords: NaHS, NaCl, root, ion flux, Populus euphratica, Populus popularis, NMT
Citation: Zhao N, Zhu H, Zhang H, Sun J, Zhou J, Deng C, Zhang Y, Zhao R, Zhou X, Lu C, Lin S and Chen S (2018) Hydrogen Sulfide Mediates K+ and Na+ Homeostasis in the Roots of Salt-Resistant and Salt-Sensitive Poplar Species Subjected to NaCl Stress. Front. Plant Sci. 9:1366. doi: 10.3389/fpls.2018.01366
Received: 29 June 2018; Accepted: 28 August 2018;
Published: 19 September 2018.
Edited by:
Yanxi Pei, Shanxi University, ChinaReviewed by:
Zhuping Jin, Shanxi University, ChinaShaowu Xue, Huazhong Agricultural University, China
Copyright © 2018 Zhao, Zhu, Zhang, Sun, Zhou, Deng, Zhang, Zhao, Zhou, Lu, Lin and Chen. This is an open-access article distributed under the terms of the Creative Commons Attribution License (CC BY). The use, distribution or reproduction in other forums is permitted, provided the original author(s) and the copyright owner(s) are credited and that the original publication in this journal is cited, in accordance with accepted academic practice. No use, distribution or reproduction is permitted which does not comply with these terms.
*Correspondence: Shanzhi Lin, szlin@bjfu.edu.cn Shaoliang Chen, Lschen@bjfu.edu.cn
†These authors have contributed equally to this work