- 1Department of Geography and Geosciences, Institute of Geography, Friedrich-Alexander University Erlangen-Nuremberg, Erlangen, Germany
- 2Department of Forestry and Environmental Science, Shahjalal University of Science and Technology, Sylhet, Bangladesh
Xylem hydraulic adjustment to global climatic changes was reported from temperate, boreal, and Mediterranean tree species. Yet, the long-term hydraulic adjustment in tropical tree species has not been studied so far. Here we developed the first standard chronologies of three hydraulic trait variables for three South Asian moist forest tree species to analyze their long-term hydraulic responses to changing climate. Based on wood anatomical measurements, we calculated Hagen–Poiseuille hydraulically weighted vessel diameter (DH), potential specific hydraulic conductivity (KS), and vulnerability index (VX) and developed standard chronologies of these variables for Chukrasia tabularis, Toona ciliata, and Lagerstroemia speciosa which are different in their xylem structure, wood density, shade tolerance, growth rates, and habitat preferences. Bootstrap correlation analysis revealed that vapor pressure deficit (VPD) strongly positively influenced the xylem water transport capacity in C. tabularis, whereas T. ciliata was affected by both temperature and precipitation. The hydraulic conductivity of L. speciosa was mainly affected by temperature. Different adjustment strategies were observed among the species, probably due to the differences in life history strategies and xylem properties. No positive relationship of conductivity and radial growth was found, but a trade-off between hydraulic safety and efficiency was observed in all studied species.
Introduction
The xylem in woody plants serves multiple function such as water and nutrient transport, mechanical support, maintaining stomatal opening and carbon uptake (Carlquist, 2001; Sperry et al., 2003). However, safe and efficient water transport from root to leaf is considered as one of the vital functions (Brodribb and Hill, 1999; Sperry et al., 2003) which determines plant performance, especially during stressful environmental conditions (Tyree and Sperry, 1989; Larcher, 2003; Fonti et al., 2010). Global environmental changes such as rising temperature, changes in temporal precipitation patterns, frequent, and extreme drought events (IPCC, 2014) may have substantial impact on plant growth, phenology, physiology, and other developmental processes (Ceulemans et al., 1997; Crous et al., 2011; Nitschke et al., 2017; He et al., 2018). In order to cope with changing environment particularly related to water availability, vascular plants may adjust their hydraulic architecture so that they can maximize water transportation and can minimize the risk of hydraulic failure (Sperry et al., 2002; Fonti et al., 2010; Martinez-Vilalta et al., 2014). For example, plants produce a small number of large size vessels in response to drought (Hacke et al., 2006; Sperry et al., 2006; Wheeler, 2011; Haworth et al., 2017). This phenotypic plasticity allows plants efficient transportation of water from the root to the leaf (Zimmermann, 1983) since the water flow along a vessel is proportional to the fourth power of its radius according to the Hagen-Poiseuille Law (Tyree and Zimmermann, 2002; Sperry et al., 2006; Steppe and Lemeur, 2007; McCulloh et al., 2010). Likewise, as a resopnse to avoid hydraulic failure due to cavitation, vessel frequency is increased so that a higher number of smaller vessels remain functional in terms of cavitation (Sperry et al., 2008; Zanne et al., 2010; Venturas et al., 2017; Pérez-de-Lis et al., 2018).
The hydraulic adjustment mechanism has been investigated across tree populations, species and at different spatial scales (Borghetti et al., 2017). However, less attention has been paid to get insight into the long-term temporal plasticity particularly in tropical forest trees although tropical forests are supposed to be more vulnerable to climate change as expected before (Clark et al., 2003; Doughty and Goulden, 2009). Studying temporal plasticity in xylem hydraulic structure in response to environmental changes requires developing chronologies of hydraulic traits from long-term wood anatomical time series. Particularly, the necessity to develop time series of hydraulic traits has been emphasized to study climate change adaptability both in temperate (Bryukhanova and Fonti, 2013; Oladi et al., 2014; Rita et al., 2015; Schuldt et al., 2016; Noyer et al., 2017; Granda et al., 2018; Pérez-de-Lis et al., 2018) and Mediterranean forests (Corcuera et al., 2004a, 2006; Gea-Izquierdo et al., 2012; Rita et al., 2016; Castagneri et al., 2017; Martínez-Sancho et al., 2017). So far, no hydraulic conductivity (KS) time series were developed for tropical forest trees. A recent global scale meta-analysis (Borghetti et al., 2017) on the temporal plasticity in xylem hydraulic traits revealed that almost all existing studies were done in high latitude regions (36–52° N), with no studies in tropical and sub-tropical regions (−30° S to 30° N). This points to a clear research gap concerning hydraulic adjustment of tree species in tropical regions.
Tropical broadleaf species are understudied in terms of long-term wood anatomical climate adaptations. Tectona grandis was frequently used to develop vessel chronologies (Pumijumnong and Park, 1999; Bhattacharyya et al., 2007; Venegas-González et al., 2015; Sinha et al., 2017). Besides, some mangrove species such as Heretiera fomes and Rhizophora mucronata were studied to develop wood anatomical time series (Verheyden et al., 2005; Chowdhury et al., 2008). In a preliminary study, we investigated wood anatomical features in 27 tree species from a moist tropical forest in Bangladesh and showed their dendrochronological potential (Islam et al., 2018a). In a further step, we established vessel chronologies of diffuse-porous and intermediate shade tolerant Chukrasia tabularis from a Bangladeshi moist tropical forest and showed that the vessel features are highly sensitive to climate (Islam et al., 2018b). Some other studies have shown the potential of developing wood anatomical time series in moist tropical forests (Ohashi et al., 2011, 2014). Nevertheless, time series of hydraulic conductivity of any of the tropical tree species have not been measured so far. Variations of hydraulic conductivity along a water flow path from root to shoot were studied in an Indonesian tropical forest (Kotowska et al., 2015). Also, radial variations of tree hydraulic properties were studied in a Southeast Asian tropical forest (Rungwattana and Hietz, 2017). However, annually resolved time series of hydraulic traits in tropical tree species that can be related to environmental variable are yet to be developed. Moreover, moist tropical forests are rich in species and functional diversity (Slik et al., 2015). It is reported that different functional groups may show different hydraulic adjustment strategy in response to changing environment (Poorter et al., 2010). Several studies suggested that shade intolerant species have higher hydraulic efficiency than shade tolerant species (Markesteijn et al., 2011a,b; Hoeber et al., 2014; Hietz et al., 2017; Rungwattana and Hietz, 2017). Similarly, ring-porous species are likely to be more vulnarable to cavitation than diffuse porous species (Sperry et al., 1994; Taneda and Sperry, 2008; Ogasa et al., 2014).
Interspecific differences in xylem hydraulic architecture (vessel features) may reflect differences in the way of hydraulic adjustment in response to environmental variability (Fonti et al., 2010), providing valuable information about the plasticity a particular species shows under changing environmental conditions. It is therefore important to test the range of hydrosystem adjustment in species of different functional types. In the present study, we developed long-term chronologies of three hydraulic trait variables for three tropical moist forest trees which differ in their xylem anatomy (diffuse porous, semi ring-porous and ring-porous), shade tolerance (shade tolerant, shade intolerant, and partial shade tolerant), wood density, growth rates, and habitat preferences. We aimed at testing the following hypotheses:
▪ Trees modulate their hydraulic traits in response to inter annual variation in climate variables in moist tropical forests.
▪ Phenotypic plasticity differs among functionally different moist forest trees.
▪ Hydraulic conductivity is positively associated with radial growth since it is assumed that efficient water transport may favor tree growth (Poorter et al., 2010; Fan et al., 2012; Schuldt et al., 2016).
▪ Trees of different functional types maintain the well-known trade-off between hydraulic efficiency and safety through their life time under tropical moist environment.
Materials and Methods
Study Area and Species
The study was carried out within the Rema-Kalenga forest in the north eastern part of Bangladesh (24°06′−24°14′N, and 91°36′−91°39′E). An area of 1,795 ha in Rema-Kalenga forest was declared as Wildlife Sanctuary which is bounded on the east and south by Tripura state of India. The study sites consist of hilly areas of around 100 m elevation and low-lying valleys. Soils of the forest vary from clay loam on the relatively level ground or valleys to sandy loam on the hills (Hassan, 1994). The climate of the study area is characterized as tropical humid monsoonal climate (Holdridge, 1967). According to the climate data recorded at the nearest climate station (Sreemangal, Bangladesh), the mean annual temperature and total annual precipitation over the period 1950–2015 are 24.8°C and 2,363 mm, respectively (Figure 1). A distinct seasonality is present, with a dry season spanning from November to February (monthly rainfall < 100 mm) (Figure 1). May-August is the main monsoon season, March–April is the pre-monsoon and September-October is the late-monsoon season in our study region. A significant increasing trend was observed in mean, minimum, and maximum temperatures, whereas precipitation did not show any clear trend over 1950–2015 (Figure 2).
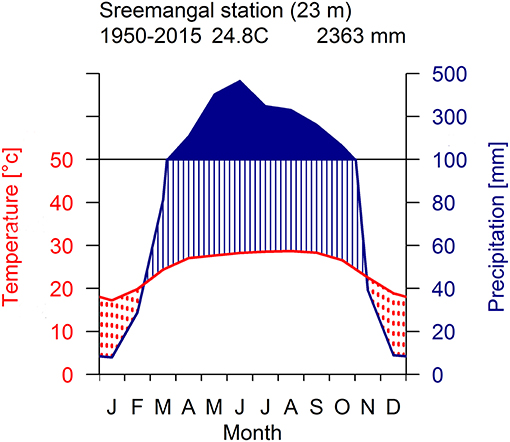
Figure 1. Walter-Leith monthly climate diagram for the study area derived from data collected at meteorological station (Sreemangal, 23 km) for the period 1950–2015.
The studied forests are recognized for the ecological services they provide and are dominated by deciduous and evergreen trees including Chukrasia tabularis, Toona ciliata, Syzygium grandis, Terminalia bellirica, Lagerstroemia speciosa, Dillenia pentagyna, Dipterocarpus turbinatus, Tectona grandis, as well as various species of Ficus and Albizia. We selected three species (Chukrasia tabularis, Toona ciliata, and Lagerstroemia speciosa) based on their wide distribution across the tropics, their functional differences, and the characteristics of growth-ring boundaries (Islam et al., 2018a). C. tabularis comprises diffuse porous wood anatomy with distinct growth-ring boundaries delineated by marginal parenchyma bands. Xylem anatomy of T. ciliata is characterized by semi ring-porous vessel distribution, with distinct growth-ring boundaries defined by marginal parenchyma and large earlywood vessels. L. speciosa is characterized by ring-porous wood anatomy with distinct growth-ring boundaries detected by large early wood vessels following a marginal parenchyma band. All three study species were found to form annual growth rings (Bhattacharyya and Yadav, 1989; Heinrich et al., 2009; Vlam et al., 2014; Rahman et al., 2018). A detailed description of the selected species is given in Table 1.
Wood Sample Collection, Preparation, and Anatomical Measurements
We extracted increment cores from 105 trees at breast height (1.3 m) by using a 5.0 mm diameter increment borer (Vantaa, Finland). In the field, plastic holders were used to store the cores immediately after extraction. Cores were air dried for 24 h to avoid any attack by fungi. In the laboratory, increment cores were mounted on wooden holders and sanded with increasingly finer grain paper up to 2,000 grit to make wood anatomical features clearly visible (Stokes and Smiley, 1968). A high-pressure water blast was used to remove wood dust and tyloses inside the vessels. White chalk was applied on the surface to fill the vessels, thus improving the contrast from the bulk tissues. Ring-width (RW) was measured directly on the core surface by using a Lintab 6 measuring system (Rinntech, Heidelberg, Germany) with a precision of 0.01 mm. Ring-width series were visually crossdated using TSAP-Win (Rinntech, Heidelberg, Germany) and statistically evaluated by t-test and Gleichläufigkeit values (GLK, sign test; Eckstein and Bauch, 1969). Crossdating quality was finally checked using COFECHA (Grissino-Mayer, 2001). A total of 70 trees were successfully crossdated.
Wood anatomical preparation and measurements are laborious and time consuming. Hence, a subset of 9 cross-dated trees from each species (total 27 trees) was finally selected for wood anatomical measurements. We took digital images of the cross sectional surfaces of the cores (5,184 × 3,456 pixels) by using a digital microscope Zeiss Smartzoom 5 (Carl Zeiss Microscopy GmbH 2014, Jena, Germany) for xylem anatomical measurement (Figure 3).To simplify the measurements, Adobe Photoshop was used to further increase the contrast between the vessels and other wood elements and manually edit unclear cases of vessel lumen area due to preparation artifacts. Before the measurement, the image was calibrated from a scale bar of known length in the image. On each image, tree-ring boundaries were identified with their year of formation and an analysis region was created in each tree ring by closing the regions delineated by the ring boundary paths in WinCELL. All vessels (vessel area: 0.002–20.0 mm2, other features smaller or larger were discarded) were measured for each dated tree ring using an image–analysis software WinCELL 2012a (Regent Instruments Inc., Québec, Canada) which is specifically designed for wood cell analysis. We measured four vessel parameters including the number of vessels (NV), mean vessel tangential diameter (MVTD), mean vessel radial diameter (MVRD), and mean vessel area (MVA). NV, MVTD, and MVRD were then used to calculate vessel density (VD) and hydraulic traits such as the Hagen–Poiseuille hydraulically weighted vessel diameter (DH), potential specific hydraulic conductivity (KS), and vulnerability index (VX).
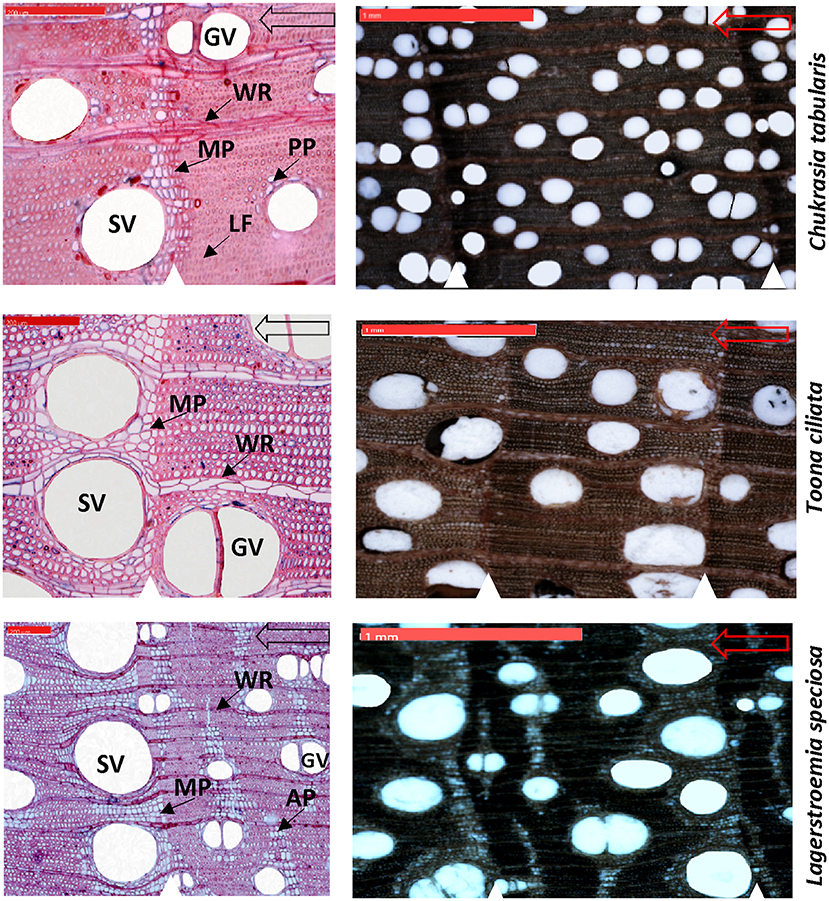
Figure 3. High resolution digital microscopic images of the thin sections (Left) and cross sectional wood surface (Right) showing characteristic wood anatomical features of three South Asian tropical moist forest tree species. White triangles indicate growth-ring boundaries. Hollow arrows indicate growth direction. SV, solitary vessels; GV, grouped vessels; WR, wood rays; MP, marginal parenchyma; LF, latewood fibers; AP, Apotracheal parenchyma; PP, Paratracheal parenchyma. Scale bars are 200 μm in the thin sections and 1 mm in the cross sectional wood surface images.
Xylem Hydraulic Traits Calculations
Since most of the vessels in the studied tree species are not circular but mostly elliptical in shape, radial and tangential diameters (Oladi et al., 2014) of each vessel were considered as major (ai) and minor (bi) axis. The Hagen–Poiseuille hydraulically weighted DH was computed by applying the following equations (Lewis and Boose, 1995; Tyree and Zimmermann, 2002; Steppe and Lemeur, 2007)
Where n is the number of measured vessels, a and b (μm) are the two axes of the vessels
The potential specific hydraulic conductivity is the total estimated conductivity of a group of vessels assuming that their flow is governed by the Hagen–Poiseuille equation. Potential specific hydraulic conductivity KS (kg m−1 s−1 Mpa−1) was calculated using the Hagen–Poiseuille's law (Tyree and Zimmermann, 2002) as follows:
Where η is the viscosity of water (1.002 × 10−9 MPa s−1), ρ the density of water at 20°C (998.21 kg m3), VD (mm−2) is the ratio of NV per analyzed area (mm2), and DH is the Hagen–Poiseuille hydraulically weighted DH (m).
The vulnerability index indicates a rough valuation about the trees sensitivity to the risks of cavitation. An increase in the VX shows higher potential susceptibility of the hydraulic system to damages (Tyree and Zimmermann, 2002). The VX was calculated as follows (Carlquist, 1977; Bauerle et al., 2011; Aref et al., 2013)
where VD is vessel density and DH is the Hagen–Poiseuille hydraulically weighted DH.
Xylem Hydraulic Traits Time Series
For investigating the inter-annual variability of xylem hydraulic traits, we developed chronologies of DH, KS, and VX for the three studied species following standard dendrochronological procedures. We observed an increasing trend in hydraulic traits' time series (Figure S2). It has been shown that DH systematically increases with plant size, including plant height and correlated stem diameter in tropical woody species (Olson and Rosell, 2013) as well as on a global scale (Olson et al., 2014). To avoid any bias of increasing vessel size with increasing stem diameter, we removed age or size related trends from each hydraulic trait time series before chronology development. We used a cubic smoothing spline function with a 50% frequency response at 10 years for detrending, because we aimed at removing multi-decadal variability from our rather short (< 100 years) time series, and to retain high-frequency signals related to inter-annual climate variability. Detrended time series were then obtained by dividing the observed values by the fitted values (Briffa and Jones, 1990). The detrended time series of nine trees of each of the three species were averaged by a bi-weight robust mean to build the chronologies. The chronologies were build using the “chron” function in the Dendrochronology Program Library in R (dplR) (Bunn, 2008, 2010) within the R statistical programming environment (R Development Core Team, 2016). The robustness of the chronologies were assessed using standard statistics commonly used in dendrochronology including the mean inter-series correlation between trees (rbar.bt) (Briffa and Jones, 1990), the expressed population signal (EPS) (Wigley et al., 1984; Buras, 2017) and mean sensitivity (MS) (Briffa and Jones, 1990).
Intra-annual variability of wood anatomical parameters is also a research focus particularly in temperate regions. Such investigations require splitting of a ring into several sections. However, the number of the generally rather large vessels is very low in our studied species particularly in T. ciliata and L. speciosa (Figure 3). Splitting a ring into different sections might further reduce the vessel number per section due to splitting across some vessels. Particularly in narrow rings, sectioning is not feasible due to the very low number or even complete absence of vessels in some sections which does not provide adequate replication for statistical analysis. Hence, we refrained from intra-annual analysis of vessel features and instead considered the complete annual rings.
Dendroclimatic and Statistical Analysis
Bootstrap correlation analysis was performed to assess the influence of climate variables (temperatures, Tmean, Tmin, and Tmax; precipitation; Palmer Drought Severity index, PDSI; and Vapor Pressure Deficit, VPD) on xylem hydraulic traits. The bootstrap procedure generated 1,000 bootstrapped samples from the original hydraulic trait indices to test the significance of correlation coefficients and the stability of the error estimates more precisely (Guiot, 1991). We used the “bootRes” package in R to compute Pearson correlation coefficients between the hydraulic traits and each of the climatic parameters. A 20 months window from May of the previous year to current year December was used to correlate hydraulic conductivity time series with monthly climate data. Previous year's climate was included because current year's tree-ring features might be influenced by previous year climate through carbon carryover effects which is evident from the auto-correlation in the developed chronologies (0.09–0.56) (Fritts, 1976). One way analysis of variance (ANOVA) was performed to test the differences in hydraulic traits (DH, KS, and VX) among the three species. Requirements for normal distribution and statistical methods were checked. In order to assess the relations of hydraulic traits (DH, KS, and VX) with tree radial growth (RW) and various vessel features (MVA), we used simple linear regression analysis. To investigate the relationships between the hydraulically weighted DH and VD we also used regression equation fitting exponential curve.
Temperature, precipitation, and humidity data were obtained from the Bangladesh Meteorological Department (BMD). PDSI data were collected from the KNMI climate explorer (Royal Netherlands Meteorological Institute) (https://climexp.knmi.nl/start.cgi). We calculated vapor pressure deficit (VPD) from the station data following the equations: VPD = [(100 - RH)/100]*SVP, where SVP = 0.610exp*[(17.27*T)/(T+237.3)], T = temperature, RH = relative humidity (Murray, 1967). All analyses were performed using different statistical packages within the R statistical programming environment (R Development Core Team, 2016)
Results
Interspecies Variation in Xylem Hydraulic Traits
Xylem hydraulic traits showed significant differences between species (Figure 4). Highest variations was observed in the hydraulic vulnarability to cavitaiton (VX) [F(2, 243) = 582.51, p < 0.001], followed by the hydraulically weighted vessel diamter (DH) [F(2, 243) = 294.67, p < 0.001]. The lowest but still strongly significant variation was observed in potential hydraulic conductivity (KS) [F(2, 243) = 45.008, p < 0.001]. Intermediate shade tolerant and diffuse porous C. tabularis exhibited the lowest DH, KS, and VX in comparison to the ring posours T. ciliata and L. speciosa. However, semi ring-porous and shade intolerant T. ciliata had higher DH, KS, and VX than shade tolerant and ring-porous L. speciosa (Figure 4). Differences in DH, KS, and VX showed a consistent pattern among the species.
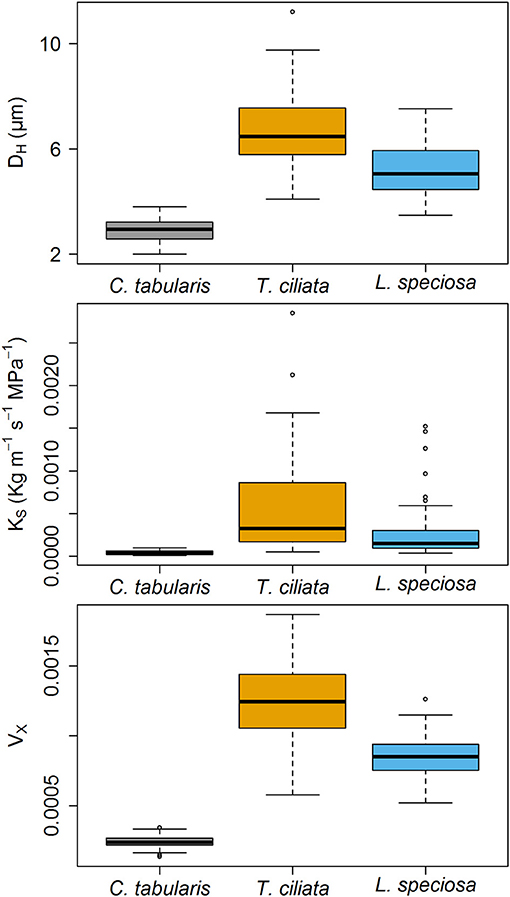
Figure 4. Boxplots of Hagen–Poiseuille hydraulically weighted vessel diameter (DH), Potential specific hydraulic conductivity (KS) and vulnerability index (VX) of three South Asian tropical moist forest tree species. Different letters indicate significant difference (p < 0.01) among the species.
Xylem Hydraulic Traits Chronologies
We developed three hydroulic trait chronologies for all the three species (Figure 5, Figure S1). The statistical parameters indicating the signal strength of the chronologies are described in Table 2. The raw tree ring series used to develop standard chronologies are also shown in Figure S2. Among the three chronologies within a species, specific hydraulic conductivity (KS) showed the highest common signal in all species as indicated by mean inter series correlation (rbar.bt) that varied between 0.17 and 0.22 among the species. The subsequent EPS was also highest in KS among the three hydraulic traits with highest EPS in Toona ciliata (0.72). The lowest rbar.bt and EPS were observed in VX chronology in all species. However, inter annual variation indicated by MS was high in all chronologies except DH. In all species, highest MS was found in KS. Based on the chronlogy statistics we progressed with KS for further dendroclimatic analysis because KS chronologies in all species showed the highest chronology signal which were consistent with values reported for wood anatomical time series (García-González et al., 2016). The Ks chronologies also provide indirect evidence of vulnerability to cavitation since hydraulic efficiency is directly related to hydraulic vulnerability (Markesteijn et al., 2011b).
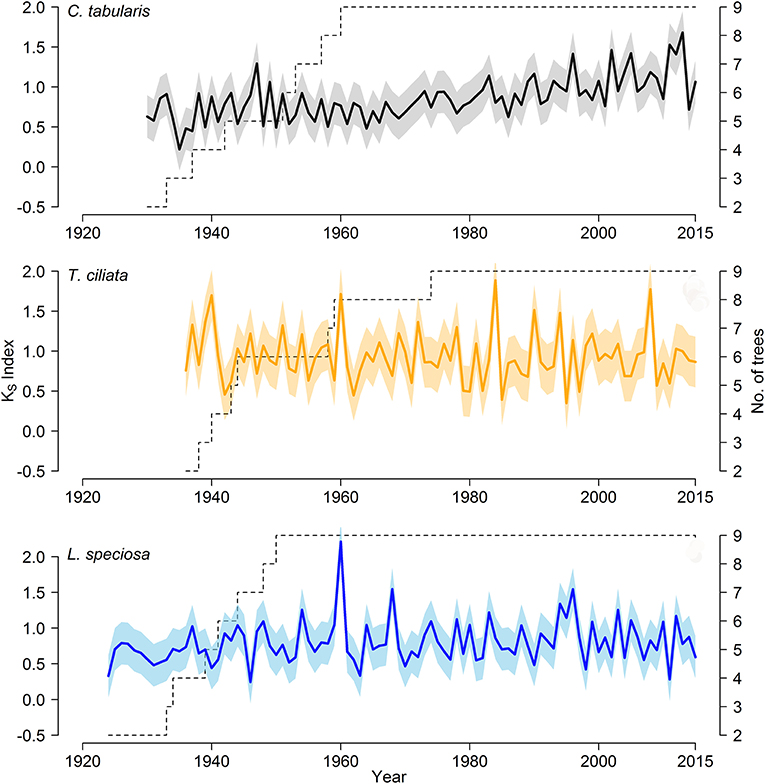
Figure 5. Standard chronologies of hydraulic conductivity (KS) of three South Asian tropical moist forest tree species; Color shading shows a ±1 SD interval around the means of the chronologies.
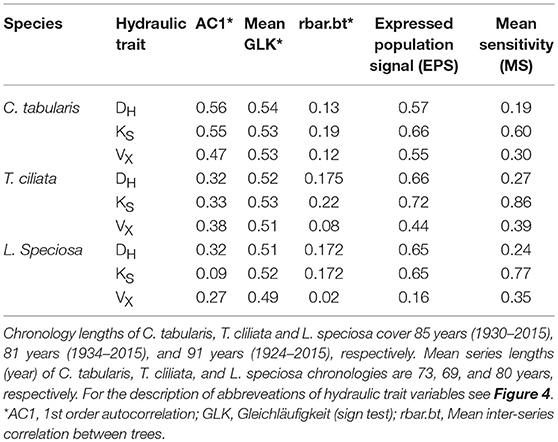
Table 2. Chronology characteristics of three hydraulic traits for three South Asian moist tropical forest tree species.
Xylem Hydraulic Response to Climate Variability
Among the climate variables, VPD had the dominant influence on the variability of hydraulic conductivity (KS) in C. tabularis (Figure 6). Both current year and previous year monsoon and post monsoon season VPD had a positive impact on KS whereas late spring and summer VPD negatively affected KS in C. tabularis (highest correlation with current year September, r = 0.61, p < 0.001). KS was significantly positively correlated with late monsoon season (September) mean and maximum temperatures (Tmean, Tmax) and early monsoon season (May) precipitation (Figure 6). Previous year May temperatures also positively influenced KS whereas temperature in the later growing season (November) negatively affected KS in C. tabularis.
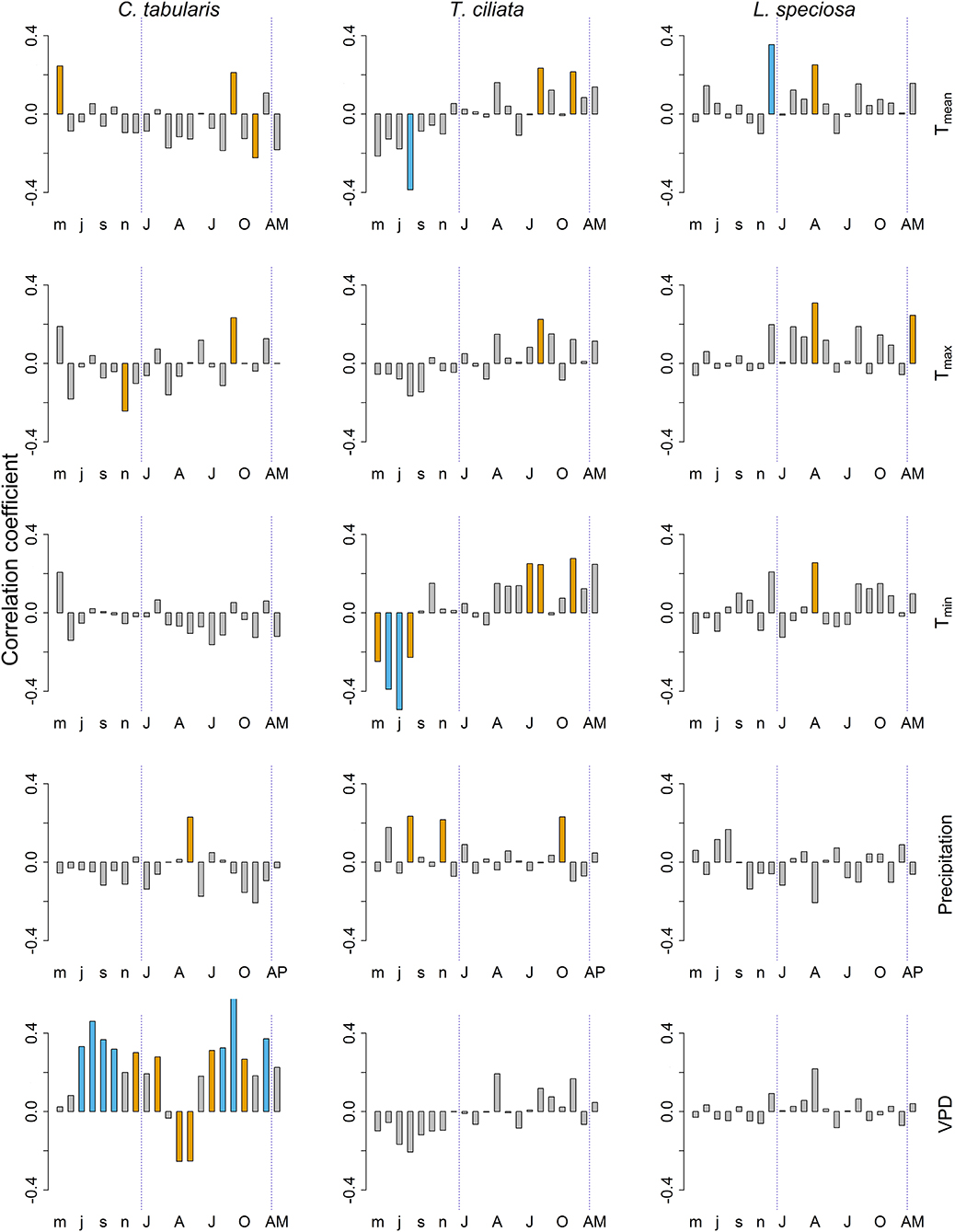
Figure 6. Bootstrap correlations between climate variables and hydraulic efficiency (KS) of three South Asian tropical moist forest tree species. Orange and blue bars indicate correlations significant at p < 0.05 and p < 0.01 levels, respectively. Lower case letters represent months of the previous year. Upper case letters represent months of the current year. AM, Annual mean; AP, Total annual precipitation.
In T.ciliata, KS was positively related to both day and night time temperatures (Tmean, Tmin, and Tmax) and precipitation during the late monsoon season (Figure 6). In contrast, previous year temperatures during the main monsoon and late monsoon season were negatively related to KS, with a strong negative correlation with previous year August Tmean (r = −0.39, p < 0.01) and previous year June and July Tmin (r = −0.49, p < 0.001). Previous year late monsoon precipitation positively affected KS.
KS in L. speciosa was strongly and positively affected by previous year winter temperature mean temperature (December; r = 0.35, p < 0.01; Figure 6). Similarly, late spring and early summer temperatures (April) (Tmean, Tmin, and Tmax) significantly positively influenced KS. Mean annual maximum temperature (Tmax) was also positively related to KS. Nonetheless, Precipitation had no significant influence on the KS in L. speciosa. We found no significant correlation of KS with PDSI in any of the studied species.
Interrelationship Between Hydraulic Traits and Radial Growth
A strong linear and inverse relationship was found between radial growth and hydraulically weighted diameter (DH) in T. ciliata (R2 = 0.19, p < 0.01) and L. speciosa (R2 = 0.29, p < 0.01). However, the relationship was not significant in C. tabularis (Figure 7). The potential hydraulic conductivity (KS) was linearly positively related to radial growth in all three species, with the highest variance explained in T. ciliata (26%) followed by L. speciosa (23%).
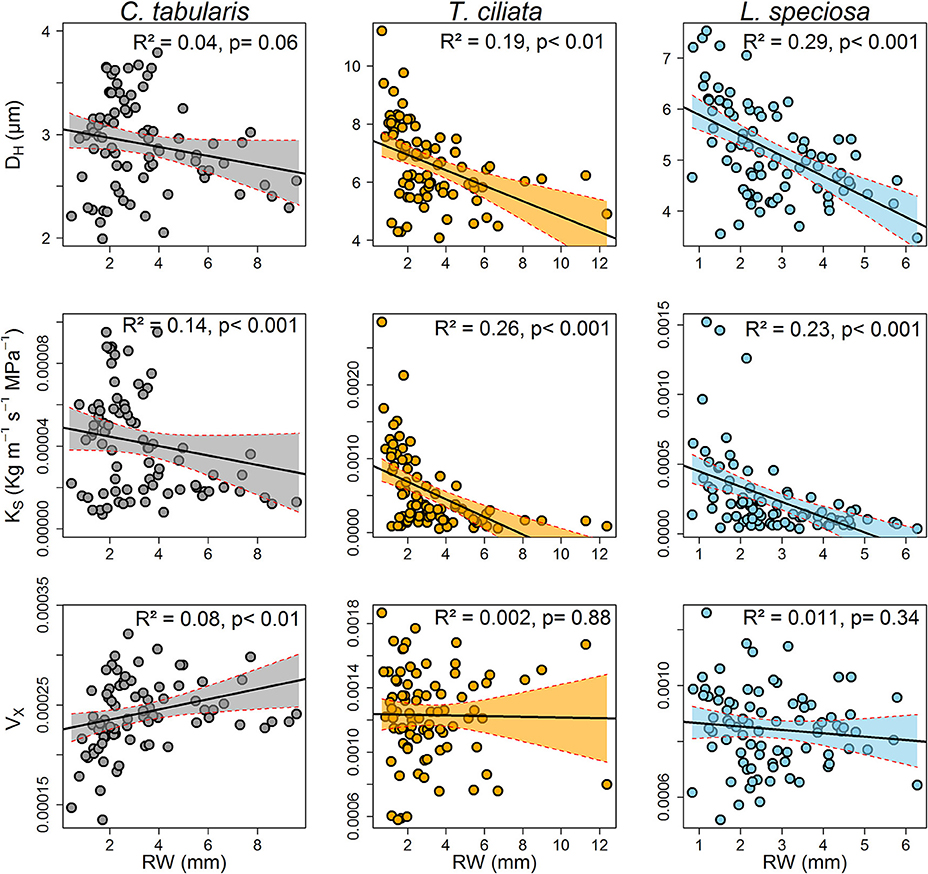
Figure 7. Relationships between ring-width and wood hydraulic traits of three South Asian tropical moist forest tree species. Each data point represents the mean of the respective years of 9 trees. Shaded areas represent 95% confidence intervals.
KS was strongly positively connected to MVA in all the three species, explaining 85–94% of the variations. Likewise, hydraulic vulnerability strongly increased with MVA explaining the highest variation by L. speciosa (62%) (Figure 8). MVA was negatively associated with radial growth in two of the three species except C. tabularis (Figure S3). A strong negative relationship was found between radial growth and VD in all the studied species, with highest variation (56%) explained in L. speciosa (Figure S3). As we expected, hydraulic conductivity was positively related to hydraulic vulnerability in all species (p < 0.001) (Figure S4).
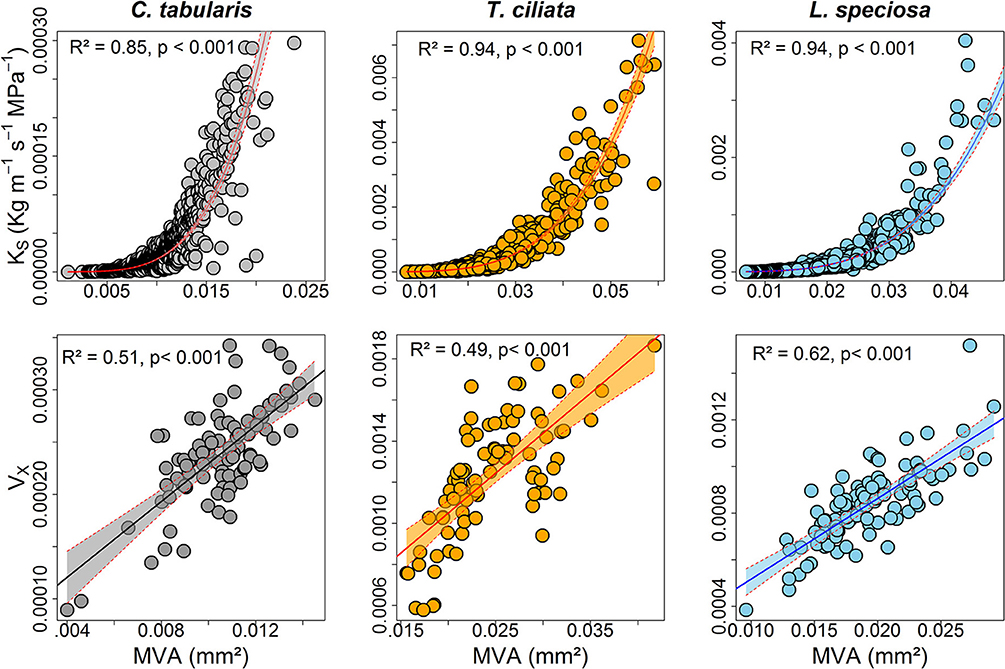
Figure 8. Relationships between hydraulic traits and vessel size (mm2) of three South Asian tropical forest tree species. Shaded areas represent 95% confidence intervals.
Xylem Adjustment: Safety vs. Efficiency
We observed the commonly expected trade-off between hydraulically weighted DH and VD. VD increased when DH decreased, leading to decreased vulnerability to hydraulic failure due to cavitation. This inverse relation was stronger when analyzed on the tree community level (R2 = 0.37, p < 0.001) than on the species level (trees within a species) (Figure 9). Ring-porous T. ciliata and L. speciosa exhibited a stronger trade-off between DH and VD than diffuse porous C. tabularis. The variation explained by the inverse relationship of DH and VD ranged between 5 and 21% among the three species, with T. ciliata explaining the highest variation.
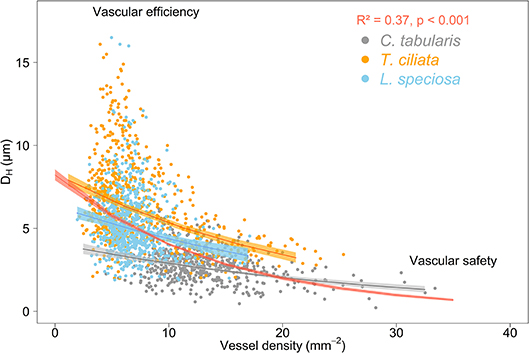
Figure 9. Trade-off between hydraulic efficiency and hydraulic safety in three South Asian tropical moist forest tree species. The red curve represents the average of all 27 measured trees. Shaded areas represent 95% confidence intervals.
Discussion
Long Term Hydraulic Adjustment to Climate Variability
We compared standard chronologies of specific hydraulic conductivity (KS) with climate variables to evaluate the long-term hydraulic adjustment to climate variability. The robustness of the KS chronologies for dendroclimatic analysis was reflected in the chronology statistics because Ks displayed the highest values in all the statistical parameters among the three chronologies in all three species. Although the common signal was rather low and did not pass the recommended threshold for the EPS statistic of 0.85 (Table 2), the values were within the normal range commonly found in wood anatomical time series (García-González et al., 2016). Our chronology statistics are consistent with hydraulic conductivity (KS) time series of other ring-porous (Corcuera et al., 2006; Campelo et al., 2010; Gea-Izquierdo et al., 2012; Pérez-de-Lis et al., 2018) and diffuse-porous (Corcuera et al., 2004b; Oladi et al., 2014; Rita et al., 2015; Schuldt et al., 2016; Noyer et al., 2017) broadleaf trees. Despite of the low common signal, the chronologies exhibited strong relationships with climate variables. Our findings are in line with other studies which reported low common signals but a high correlation with climate in a wide range of species and forest types (Pumijumnong and Park, 1999; García-González and Eckstein, 2003; Fonti et al., 2007; Campelo et al., 2010; González-González et al., 2013; Pritzkow et al., 2016; Martínez-Sancho et al., 2017). Despite of species specific climate responses, we observed some common climate influence on all three species in spring and summer (pre-monsoon) season.
Overall, hydraulic conductivity (KS) of all species positively responded to current year temperatures, precipitation and VPD except the inverse relation of KS in C. tabularis and April-May VPD. VPD in association with mean and minimum temperatures exerted dominant control over the xylem water transportation capacity in C. tabularis, whereas T. ciliata was affected by both temperature and precipitation. Temperature had the dominant effect on the hydraulic conductivity (KS) of L. speciosa. Higher temperature increases VPD, leading to increased evapotranspiration. In order to response to high evaporative demand, trees maximize their water transport efficiency. The underlying mechanism of this increased hydraulic conductivity (KS) lies in the fact that high temperature impairs the cell differentiation processes, particularly during latewood formation. Occasionally, during the post-monsoon season, latewood formation is completely ceased in response to high evaporative demand, which results in a ring with a small number of large size earlywood vessels. Consequently, the MVA increased as an adjustment to support efficient water transport. However, previous year temperatures had a negative influence on hydraulic conductivity (KS). Higher temperatures limited tree growth in the previous year, facilitating storage of carbohydrate reserves which create favorable conditions for tree growth of the following year (Fritts, 1976). This results in a reduced conductivity in the following year since small size latewood vessels reduce mean hydraulically weighted diameter and MVA. The positive influence of precipitation on conductivity is most likely caused by growth reduction due to high soil moisture content, particularly in the main monsoon months and in the post monsoon season (Rahman et al., 2018). Both C. tabularis and T. ciliata are sensitive to water saturation since they prefer well-drained soil conditions (Orwa et al., 2009).
Vulnerability to cavitation is an important hydraulic trait which determines the competitiveness of a particular species under climatic stress. Due to a lower common signal in VX chronologies we were unable to compare them with climate variables. However, we observed that higher hydraulic conductivity (KS) increased hydraulic vulnerability in all species (Figure S4). Consistent with our results many studies have found the positive relationship between hydraulic conductivity and vulnerability (Markesteijn et al., 2011a; Oladi et al., 2014; Pérez-de-Lis et al., 2018). It is also noted that trunk level hydraulic conductivity may be confounded by whole tree architecture such as leaf area to sapwood area and may vary along a height gradient from root to shoot (Kotowska et al., 2015). Considering these factors however, requires huge time and budget and we hope to take them in to account in our next project.
Inter Specific Variations in Phenotypic Plasticity
Plants may show differential growth and wood anatomical adjustment to stressful environmental conditions. The adjustment mechanism may vary depending on tree functional types and their life history strategies (Poorter et al., 2010; Markesteijn et al., 2011a). In our study, semi ring-porous and ring-porous T. ciliata and L. speciosa showed similar responses to water stress, maintaining an inverse relation of radial growth with hydraulically weighted diameter (DH) and MVA (Figure 7 and Figure S3). By the way of increasing DH, ring-porous trees increase the efficiency of water transportation as reflected in increased potential specific hydraulic conductivity (KS). When radial growth decreases during the late growing season, more carbohydrate reserves may be available for earlywood formation in the following year (Lacointe, 2000; Richburg, 2005). Simultaneously, vessel frequency increased to keep a higher number of smaller vessels hydraulically functional and thus to avoid drought induced cavitation (Sperry et al., 2008; Zanne et al., 2010; Venturas et al., 2017; Pérez-de-Lis et al., 2018). Our results are in line with studies on ring-porous tree species in both temperate (Gea-Izquierdo et al., 2012; Pérez-de-Lis et al., 2018) and Mediterranean forests (Corcuera et al., 2006; Gea-Izquierdo et al., 2012; Rita et al., 2016; Castagneri et al., 2017; Martínez-Sancho et al., 2017). Some studies relating hydraulic traits with life history strategies in tropical regions also yielded similar adjustment mechanisms as reported here (Poorter et al., 2010; Zanne et al., 2010; Markesteijn et al., 2011a; Fan et al., 2012).
In contrast, diffuse porous C. tabularis did not show an increase in water transport efficiency since DH and MVA did not significantly increase in water stress conditions, although vessel frequency increased to reduce the risk of hydraulic failure (Figure 7 and Figure S3). The facts that C. tabularis maintains a constant DH and MVA under water stress and shows a strong linear positive relation of MVA to VX (Figure 8), suggest that the species is less vulnerable to cavitation risk than the ring-porous species we studied. Hence, the species specific differences in DH, KS, and VX (Figure 4) clearly support differences in adjustment strategies between ring-porous and diffuse porous trees in our study. In line with our results, diffuse porous Fagus sylvatica and Fagus orientalis showed similar plasticity in order to avoid hydraulic failure due to water stress in temperate forests (Pourtahmasi et al., 2011; Rita et al., 2015; Schuldt et al., 2016; Noyer et al., 2017). Ring-porous and shade tolerant L. speciosa was found to be more effective to prevent hydraulic failure under drought conditions than the shade intolerant T. ciliata. The above findings suggest that ring-porous species are more efficient in water transport than the diffuse porous trees, whereas diffuse porous trees are less vulnerable to cavitation under water stress, as it is clearly reflected in mean KS and VX indices of our studied species (Figure 4).
Does Higher Hydraulic Efficiency Favor Tree Radial Growth?
Efficient water transport is usually expected to favor tree radial growth (Zanne et al., 2010). Numerous studies confirmed the positive association between hydraulic conductivity and tree radial growth across major biomes in the world (Fichot et al., 2009; Zanne et al., 2009; Poorter et al., 2010; Markesteijn et al., 2011a; Fan et al., 2012; Gleason et al., 2012; Hoeber et al., 2014; Rungwattana and Hietz, 2017). Very recently, Hietz et al. (2017) analyzed a larger data set of 325 species from a Panamanian rainforest and revealed that hydraulic conductivity is the best predictor of biomass growth rates. The probable mechanism underlying this positive relationship is attributed to higher leaf level photosynthetic rate due to efficient water transport (Meinzer et al., 2008; Choat et al., 2012). Higher hydraulic efficiency results in plant tissues comprised of higher ratio of vessel lumen area which has lower construction and maintenance costs, which may also explain the positive alignment of hydraulic conductivity to radial growth (Gleason et al., 2016).
Interestingly, we observed a negative relationship between hydraulic conductivity and tree radial growth in all species, with L. speciosa showing the strongest negative relation (Figure 7). However, our results are consistent with findings from many other ring-porous (Gea-Izquierdo et al., 2012; Rita et al., 2016) and diffuse porous species (Oladi et al., 2014; Rita et al., 2015). Our studied species responded to the drought stress conditions (e.g., higher temperature, higher evaporative demand, and higher VPD) by slowing down the cell differentiation process (reduced radial growth), and maintaining plasticity in hydraulic architecture such as increased vessel frequency as a safety mechanism against cavitation. Under stress, carbohydrate accumulation is highly favored rather than investing in biomass growth, which leads to reduced radial growth but following year tree radial growth might be stimulated by the accumulated carbohydrate reserve (Lacointe, 2000; Richburg, 2005). Nonetheless, we cautiously interpret the differences in the conductivity-growth relationships observed in our study from that in other studies which mainly focused on conductivity over the whole lifespan rather than to year-to-year variations. Our findings and the evidence of other studies, however, suggest that the widely expected conductivity-productivity positive relationship may not be consistent and calls for further studies.
Xylem Safety vs. Efficiency: How Consistent at Tree and Species Level Under Humid Environment?
Trees respond to stress by drought or higher VPD by producing a higher number of small vessels to reduce the risk of cavitation (hydraulic safety) and reduce maximum vessel diameters to maintain the efficiency of water transpiration (hydraulic efficiency) (Sperry et al., 2008; Hacke et al., 2017; Haworth et al., 2017; Venturas et al., 2017; Pérez-de-Lis et al., 2018). However, proper knowledge whether this safety-efficiency trade-off occurs only within one individual in response to short-term variations to drought, or whether this trade-off is consistent between trees of the same species or even between individuals of different species is particularly important in the context of long-term global environmental change adaptability. We analyzed hydraulically weighted DH and vessel frequency data derived from a total of 1,429 tree rings of three species over the past nine decades. Our analysis confirmed the well-known trade-off between hydraulic conductivity and safety both in trees within and between species for a long-term (Figure 9). The species-specific hydraulic efficiency-safety relationships fitted exponential functions which reached lower R2 values than a function fitted through the community of studied trees of all species.
Consistent with our results, many studies confirmed this trade-off across temperate (Zanne et al., 2010), Mediterranean (LoGullo and Salleo, 1988; Tognetti et al., 1999; Martínez-Sancho et al., 2017), riparian (Pockman and Sperry, 2000), and tropical tree species (Poorter et al., 2010; Fan et al., 2017; Hietz et al., 2017), although long-term analyses are still absent for the tropics. Many studies found a week or even no trade-off between hydraulic conductivity and hydraulic safety (Maherali et al., 2004; Westoby and Wright, 2006; Gleason et al., 2016; Schuldt et al., 2016) which implies that this relationship is variable. By analyzing a large data set, Gleason et al. (2016) found that many woody species showed low safety and low efficiency, but no acceptable causes were attributed after they have analyzed this trade-off in terms of both species level traits and climate variables at different spatial scales. Gleason et al. (2016) (and references therein) also stated that micro anatomical features such as pit membrane structure or conduit length could be relevant to determine xylem safety although there features were not captured in vessel anatomy parameters measured in cross-sections. Nevertheless, species and climate specific long-term adjustment mechanisms as we investigated in this study can provide important insight into how a particular tree species and its populations will respond to environmental stress in a particular ecosystem.
Conclusions
Overall, we observed differential responses and adjustment mechanisms of hydraulic behavior to climatic stress varying with tree life history strategies and wood traits. The hydraulic adjustment however could not avoid reduced tree growth in any of the three species we studied despite of their functional differences. Growing conditions in our study region are expected to getting worse due to ever increasing temperature and higher frequency of drought and other extreme events. It is however not clear to what extent tropical trees will be able to adjust their hydraulic system under future global changes. To get more insight in to the possible future hydraulic response of tropical trees, such studies should be extended to additional species and sites across the tropics. Future research should also consider intra-annual variations of hydraulic traits which might allow improving our understanding of the seasonality and associated mechanisms driving the hydraulic behavior of tropical trees.
Author Contributions
MI and AB designed the study. MI and MR conducted field work and prepared samples for wood anatomical measurements. MI performed wood anatomical measurements and analyzed data. MI, MR, and AB interpreted the results and wrote the manuscript.
Funding
The research was supported by the German Academic Exchange Service (DAAD) and the University Grant Commission, Bangladesh.
Conflict of Interest Statement
The authors declare that the research was conducted in the absence of any commercial or financial relationships that could be construed as a potential conflict of interest.
The handling Editor declared a past co-authorship with one of the authors AB.
Acknowledgments
We sincerely acknowledge the support of the Forest Department (FD) of Bangladesh during the field work. Special thanks to Bishwajit Gosswami for his valuable support during data processing. We thank Iris Burchardt for her technical supports during sample preparation and measurement of wood anatomical features.
Supplementary Material
The Supplementary Material for this article can be found online at: https://www.frontiersin.org/articles/10.3389/fpls.2018.01761/full#supplementary-material
References
Aref, I. M., Ahmed, A. I., Khan, P. R., El-Atta, H. A., and Iqbal, M. (2013). Drought-induced adaptive changes in the seedling anatomy of Acacia ehrenbergiana and Acacia tortilis subsp. raddiana. Trees Struct. Funct. 27, 959–971. doi: 10.1007/s00468-013-0848-2
Bauerle, T. L., Centinari, M., and Bauerle, W. L. (2011). Shifts in xylem vessel diameter and embolisms in grafted apple trees of differing rootstock growth potential in response to drought. Planta 234, 1045–1054. doi: 10.1007/s00425-011-1460-6
Bhattacharyya, A., Eckstein, D., Shah, S. K., and Chaudhary, V. (2007). Analyses of climatic changes around Perambikulum, South India, based on earlywood mean vessel area of teak. Curr. Sci. 93, 1159–1164.
Bhattacharyya, A., and Yadav, R. R. (1989). Dendroclimatic research in India. Proc. Indian Natl. Sci. Acad. U.S.A. 55, 696–701.
Borghetti, M., Gentilesca, T., Leonardi, S., Van Noije, T., and Rita, A. (2017). Long-term temporal relationships between environmental conditions and xylem functional traits: a meta-analysis across a range of woody species along climatic and nitrogen deposition gradients. Tree Physiol. 37, 4–17. doi: 10.1093/treephys/tpw087
Briffa, K. R., and Jones, P. D. (1990). “Basic chronology statistics and assessment,” in Methods of Dendrochronology: Applications in the Environmental Sciences, eds E. R. Cook and L. A. Kairiuksti (Dordrecht: Kluwer Academic Publishers), 137–152.
Brodribb, T., and Hill, R. S. (1999). The importance of xylem constraints in the distribution of conifer species. New Phytol. 143, 365–372. doi: 10.1046/j.1469-8137.1999.00446.x
Bryukhanova, M., and Fonti, P. (2013). Xylem plasticity allows rapid hydraulic adjustment to annual climatic variability. Trees Struct. Funct. 27, 485–496. doi: 10.1007/s00468-012-0802-8
Bunn, A. G. (2008). A dendrochronology program library in R (dplR). Dendrochronologia 26, 115–124. doi: 10.1016/j.dendro.2008.01.002
Bunn, A. G. (2010). Statistical and visual crossdating in R using the dplR library. Dendrochronologia 28, 251–258. doi: 10.1016/j.dendro.2009.12.001
Buras, A. (2017). A comment on the expressed population signal. Dendrochronologia 44, 130–132. doi: 10.1016/j.dendro.2017.03.005
Campelo, F., Nabais, C., Gutiérrez, E., Freitas, H., and García-González, I. (2010). Vessel features of Quercus ilex L. growing under Mediterranean climate have a better climatic signal than tree-ring width. Trees Struct. Funct. 24, 463–470. doi: 10.1007/s00468-010-0414-0
Carlquist, S. (1977). Ecological factors in wood evolution: a floristic approach. Am. J. Bot. 64, 887–896. doi: 10.2307/2442382
Carlquist, S. (2001). “Methods for comparative wood anatomy studies,” in Comparative Wood Anatomy: Systematic, Ecological, and Evolutionary Aspects of Dicotyledon Wood, ed. T. Timell (Berlin; Heidelberg: Springer Berlin Heidelberg) 1–7. doi: 10.1007/978-3-662-04578-7_1
Castagneri, D., Regev, L., Boaretto, E., and Carrer, M. (2017). Xylem anatomical traits reveal different strategies of two Mediterranean oaks to cope with drought and warming. Environ. Exp. Bot. 133, 128–138. doi: 10.1016/j.envexpbot.2016.10.009
Ceulemans, R., Mohren, G. M. J., Kramer, K., and Sabate, S. (1997). “Direct impacts of CO2 and temperature on physiological processes in trees,” in Impacts of Global Change on Tree Physiology and Forest Ecosystems, eds G. M. J. Mohren, K. Kramer, and S. Sabaté (Dordrecht; Wageningen: Springer), 3–14.
Choat, B., Jansen, S., Brodribb, T. J., Cochard, H., Delzon, S., Bhaskar, R., et al. (2012). Global convergence in the vulnerability of forests to drought. Nature 491, 752–755. doi: 10.1038/nature11688
Chowdhury, M. Q., Schmitz, N., Verheyden, A., Sass-Klaassen, U., Koedam, N., and Beeckman, H. (2008). Nature and periodicity of growth rings in two Bangladeshi mangrove species. IAWA J. 29, 265–276. doi: 10.1163/22941932-90000185
Clark, D. A., Piper, S. C., Keeling, C. D., and Clark, D. B. (2003). Tropical rain forest tree growth and atmospheric carbon dynamics linked to interannual temperature variation during 1984–2000. Proc. Natl. Acad. Sci. U.S.A. 100, 5852–5857. doi: 10.1073/pnas.0935903100
Corcuera, L., Camarero, J. J., and Gil-Pelegrín, E. (2004a). Effects of a severe drought on growth and wood anatomical properties of Quercus faginea. IAWA J. 25, 185–204. doi: 10.1163/22941932-90000360
Corcuera, L., Camarero, J. J., and Gil-Pelegrín, E. (2004b). Effects of a severe drought on Quercus ilex radial growth and xylem anatomy. Trees Struct. Funct. 18, 83–92. doi: 10.1007/s00468-003-0284-9
Corcuera, L., Camarero, J. J., Sisó, S., and Gil-Pelegrín, E. (2006). Radial-growth and wood-anatomical changes in overaged Quercus pyrenaica coppice stands: functional responses in a new Mediterranean landscape. Trees Struct. Funct. 20, 91–98. doi: 10.1007/s00468-005-0016-4
Crous, K. Y., Zaragoza-Castells, J., Löw, M., Ellsworth, D. S., Tissue, D. T., Tjoelker, M. G., et al. (2011). Seasonal acclimation of leaf respiration in Eucalyptus saligna trees: impacts of elevated atmospheric CO2 and summer drought. Glob. Chang. Biol. 17, 1560–1576. doi: 10.1111/j.1365-2486.2010.02325.x
Doughty, C. E., and Goulden, M. L. (2009). Are tropical forests near a high temperature threshold? J. Geophys. Res. Biogeosci. 114:G00B07. doi: 10.1029/2007JG000632
Eckstein, D., and Bauch, J. (1969). Beitrag zur Rationalisierung eines dendrochronologischen Verfahrens und zur Analyse seiner Aussagesicherheit. Forstwissenschaftliches Cent. 88, 230–250. doi: 10.1007/BF02741777
Fan, Z.-X., Sterck, F., Zhang, S.-B., Fu, P.-L., and Hao, G.-Y. (2017). Tradeoff between stem hydraulic efficiency and mechanical strength affects leaf–stem allometry in 28 ficus tree species. Front. Plant Sci. 8:1619. doi: 10.3389/fpls.2017.01619
Fan, Z. X., Zhang, S. B., Hao, G. Y., Ferry Slik, J. W., and Cao, K. F. (2012). Hydraulic conductivity traits predict growth rates and adult stature of 40 Asian tropical tree species better than wood density. J. Ecol. 100, 732–741. doi: 10.1111/j.1365-2745.2011.01939.x
Fichot, R., Laurans, F., Monclus, R., Moreau, A., Pilate, G., and Brignolas, F. (2009). Xylem anatomy correlates with gas exchange, water-use efficiency and growth performance under contrasting water regimes: evidence from Populus deltoides × Populus nigra hybrids. Tree Physiol. 29, 1537–1549. doi: 10.1093/treephys/tpp087
Fonti, P., Solomonoff, N., and García-González, I. (2007). Earlywood vessels of Castanea sativa record temperature before their formation. N. Phytol. 173, 562–570. doi: 10.1111/j.1469-8137.2006.01945.x
Fonti, P., Von Arx, G., García-González, I., Eilmann, B., Sass-Klaassen, U., Gärtner, H., et al. (2010). Studying global change through investigation of the plastic responses of xylem anatomy in tree rings. N. Phytol. 185, 42–53. doi: 10.1111/j.1469-8137.2009.03030.x
García-González, I., and Eckstein, D. (2003). Climatic signal of earlywood vessels of oak on a maritime site. Tree Physiol. 23, 497–504. doi: 10.1093/treephys/23.7.497
García-González, I., Souto-Herrero, M., and Campelo, F. (2016). Ring-porosity and earlywood vessels: a review on extracting environmental information through time. IAWA J. 37, 295–314. doi: 10.1163/22941932-20160135
Gea-Izquierdo, G., Fonti, P., Cherubini, P., Martín-Benito, D., Chaar, H., and Cañellas, I. (2012). Xylem hydraulic adjustment and growth response of Quercus canariensis Willd. to climatic variability. Tree Physiol. 32, 401–413. doi: 10.1093/treephys/tps026
Gleason, S. M., Butler, D. W., Zieminska, K., Waryszak, P., and Westoby, M. (2012). Stem xylem conductivity is key to plant water balance across Australian angiosperm species. Funct. Ecol. 26, 343–352. doi: 10.1111/j.1365-2435.2012.01962.x
Gleason, S. M., Westoby, M., Jansen, S., Choat, B., Hacke, U. G., Pratt, R. B., et al. (2016). Weak tradeoff between xylem safety and xylem-specific hydraulic efficiency across the world's woody plant species. New Phytol. 209, 123–136. doi: 10.1111/nph.13646
González-González, B. D., García-González, I., and Vázquez-Ruiz, R. A. (2013). Comparative cambial dynamics and phenology of Quercus robur L. and Q. pyrenaica Willd. in an Atlantic forest of the northwestern Iberian Peninsula. Trees Struct. Funct. 27, 1571–1585. doi: 10.1007/s00468-013-0905-x
Granda, E., Alla, A. Q., Laskurain, N. A., Loidi, J., Sánchez-Lorenzo, A., and Camarero, J. J. (2018). Coexisting oak species, including rear-edge populations, buffer climate stress through xylem adjustments. Tree Physiol. 38, 159–172. doi: 10.1093/treephys/tpx157
Grissino-Mayer, H. D. (2001). Evaluating crossdating accuracy: A manual and tutorial for the computer program cofecha. Tree-Ring Res. 57, 205–221.
Hacke, U. G., Sperry, J. S., Wheeler, J. K., and Castro, L. (2006). Scaling of angiosperm xylem structure with safety and efficiency. Tree Physiol. 26, 689–701. doi: 10.1093/treephys/26.6.689
Hacke, U. G., Spicer, R., Schreiber, S. G., and Plavcová, L. (2017). An ecophysiological and developmental perspective on variation in vessel diameter. Plant Cell Environ. 40, 831–845. doi: 10.1111/pce.12777
Haworth, M., Centritto, M., Giovannelli, A., Marino, G., Proietti, N., Capitani, D., et al. (2017). Xylem morphology determines the drought response of two Arundo donax ecotypes from contrasting habitats. GCB Bioenergy 9, 119–131. doi: 10.1111/gcbb.12322
He, M., Yang, B., Shishov, V., Rossi, S., Bräuning, A., Ljungqvist, F. C., et al. (2018). Projections for the changes in growing season length of tree-ring formation on the Tibetan Plateau based on CMIP5 model simulations. Int. J. Biometeorol. 62, 631–641. doi: 10.1007/s00484-017-1472-4
Heinrich, I., Weidner, K., Helle, G., Vos, H., Lindesay, J., and Banks, J. C. G. (2009). Interdecadal modulation of the relationship between ENSO, IPO and precipitation: insights from tree rings in Australia. Clim. Dyn. 33, 63–73. doi: 10.1007/s00382-009-0544-5
Hietz, P., Rosner, S., Hietz-Seifert, U., and Wright, S. J. (2017). Wood traits related to size and life history of trees in a Panamanian rainforest. N. Phytol. 213, 170–180. doi: 10.1111/nph.14123
Hoeber, S., Leuschner, C., Kohler, L., Arias-Aguilar, D., and Schuldt, B. (2014). The importance of hydraulic conductivity and wood density to growth performance in eight tree species from a tropical semi-dry climate. For. Ecol. Manag. 330, 126–136. doi: 10.1016/j.foreco.2014.06.039
IPCC (2014). “Part A: global and sectoral aspects. (Contribution of Working Group II to the Fifth Assessment Report of the Intergovernmental Panel on Climate Change),” in Climate Change 2014: Impacts, Adaptation, and Vulnerability, eds C. V. Field, et al. (Cambridge: Cambridge University Press), 1132. Available online at: https://www.ipcc.ch/pdf/assessment-report/ar5/wg2/WGIIAR5-FrontMatterA_FINAL.pdf
Islam, M., Rahman, M., and Bräuning, A. (2018a). Growth-ring boundary anatomy and dendrochronological potential in a moist tropical forest in northeastern Bangladesh. Tree Ring Res. 74, 76–93. doi: 10.3959/1536-1098-74.1.76
Islam, M., Rahman, M., and Bräuning, A. (2018b). Xylem anatomical responses of diffuse porous Chukrasia tabularis to climate in a South Asian moist tropical forest. For. Ecol. Manag. 412, 9–20. doi: 10.1016/j.foreco.2018.01.035
Kotowska, M. M., Hertel, D., Rajab, Y. A., Barus, H., and Schuldt, B. (2015). Patterns in hydraulic architecture from roots to branches in six tropical tree species from cacao agroforestry and their relation to wood density and stem growth. Front. Plant Sci. 6:191. doi: 10.3389/fpls.2015.00191
Lacointe, A. (2000). Carbon allocation among tree organs: a review of basic processes and representation in functional-structural tree models. Ann. For. Sci. 57, 521–533. doi: 10.1051/forest:2000139
Larcher, W. (2003). Physiological Plant Ecology: Ecophysiology and Stress Physiology of Functional Groups. Berlin; Heidelberg: Springer.
Lewis, A. M., and Boose, E. R. (1995). Estimating volume flow rates through xylem conduits. Am. J. Bot. 82, 1112–1116. doi: 10.2307/2446063
LoGullo, M. A., and Salleo, S. (1988). Different strategies of drought resistance in three Mediterranean sclerophyllous trees growing in the same environmental conditions. N. Phytol. 108, 267–276. doi: 10.1111/j.1469-8137.1988.tb04162.x
Maherali, H., Pockman, W. T., and Jackson, R. B. (2004). Adaptive variation in the vulnerability of woody plants to xylem cavitation. Ecology 85, 2184–2199. doi: 10.1890/02-0538
Markesteijn, L., Poorter, L., Bongers, F., Paz, H., and Sack, L. (2011a). Hydraulics and life history of tropical dry forest tree species: coordination of species' drought and shade tolerance. N. Phytol. 191, 480–495. doi: 10.1111/j.1469-8137.2011.03708.x
Markesteijn, L., Poorter, L., Paz, H., Sack, L., and Bongers, F. (2011b). Ecological differentiation in xylem cavitation resistance is associated with stem and leaf structural traits. Plant Cell Environ. 34, 137–148. doi: 10.1111/j.1365-3040.2010.02231.x
Martínez-Sancho, E., Dorado-Liñán, I., Heinrich, I., Helle, G., Menzel, A., and Mencuccini, M. (2017). Xylem adjustment of sessile oak at its southern distribution limits. Tree Physiol. 37, 903–914. doi: 10.1093/treephys/tpx036
Martinez-Vilalta, J., Poyatos, R., Aguade, D., Retana, J., and Mencuccini, M. (2014). A new look at water transport regulation in plants. N. Phytol 204, 105–115. doi: 10.1111/nph.12912
McCulloh, K., Sperry, J. S., Lachenbruch, B., Meinzer, F. C., Reich, P. B., and Voelker, S. (2010). Moving water well: comparing hydraulic efficiency in twigs and trunks of coniferous, ring-porous, and diffuseporous saplings from temperate and tropical forests. N. Phytol. 186, 439–450. doi: 10.1111/j.1469-8137.2010.03181.x
Meinzer, F. C., Campanello, P. I., Domec, J. C., Gatti, M. G., Goldstein, G., Villalobos-Vega, R., et al. (2008). Constraints on physiological function associated with branch architecture and wood density in tropical forest trees. Tree Physiol. 28, 1609–1617. doi: 10.1093/treephys/28.11.1609
Murray, F. W. (1967). On the computation of saturation vapor pressure. J. Appl. Meteorol. 6, 203–204. doi: 10.1175/1520-0450(1967)006<0203:OTCOSV>2.0.CO;2
Nitschke, C. R., Nichols, S., Allen, K., Dobbs, C., Livesley, S. J., Baker, P. J., et al. (2017). The influence of climate and drought on urban tree growth in southeast Australia and the implications for future growth under climate change. Landsc. Urban Plan. 167, 275–287. doi: 10.1016/j.landurbplan.2017.06.012
Noyer, E., Lachenbruch, B., Dlouh,á, J., Collet, C., Ruelle, J., Ningre, F., et al. (2017). Xylem traits in European beech (Fagus sylvatica L.) display a large plasticity in response to canopy release. Ann. For. Sci. 74:46. doi: 10.1007/s13595-017-0634-1
Ogasa, M., Miki, N. H., Okamoto, M., Yamanaka, N., and Yoshikawa, K. (2014). Water loss regulation to soil drought associated with xylem vulnerability to cavitation in temperate ring-porous and diffuse-porous tree seedlings. Trees Struct. Funct. 28, 461–469. doi: 10.1007/s00468-013-0963-0
Ohashi, S., Okada, N., Abdul Azim, A. A., Siripatanadilok, S., Veenin, T., Yahya, A. Z., et al. (2014). Vessel feature changes as a tool for detecting annual rings in tropical trees. Trees Struct. Funct. 28, 137–149. doi: 10.1007/s00468-013-0936-3
Ohashi, S., Okada, N., Azim, A. A. A., Yahya, A. Z., and Nobuchi, T. (2011). Estimation of tree age in the humid tropics by vessel measurement: a preliminary study. Tropics 19, 107–112. doi: 10.3759/tropics.19.107
Oladi, R., Bräuning, A., and Pourtahmasi, K. (2014). “Plastic” and “static” behavior of vessel-anatomical features in Oriental beech (Fagus orientalis Lipsky) in view of xylem hydraulic conductivity. Trees Struct. Funct. 28, 493–502. doi: 10.1007/s00468-013-0966-x
Olson, M. E., Anfodillo, T., Rosell, J. A., Petit, G., Crivellaro, A., Isnard, S., et al. (2014). Universal hydraulics of the flowering plants: vessel diameter scales with stem length across angiosperm lineages, habits and climates. Ecol. Lett. 17, 988–997. doi: 10.1111/ele.12302
Olson, M. E., and Rosell, J. A. (2013). Vessel diameter-stem diameter scaling across woody angiosperms and the ecological causes of xylem vessel diameter variation. N. Phytol. 197, 1204–1213. doi: 10.1111/nph.12097
Orwa, C., Mutua, A., Kindt, R., Jamnadass, R., and Anthony, S. (2009). Agroforestree Database:A Tree Reference and Selection Guide Version 4.0. Nairobi.
Pérez-de-Lis, G., Rozas, V., Vázquez-Ruiz, R. A., and García-González, I. (2018). Do ring-porous oaks prioritize earlywood vessel efficiency over safety? Environmental effects on vessel diameter and tyloses formation. Agric. For. Meteorol. 248, 205–214. doi: 10.1016/j.agrformet.2017.09.022
Pockman, W. T., and Sperry, J. S. (2000). Vulnerability to xylem cavitation and the distribution of Sonoran desert vegetation. Am. J. Bot. 87, 1287–1299. doi: 10.2307/2656722
Poorter, L., McDonald, I., Alarcón, A., Fichtler, E., Licona, J. C., Peña-Claros, M., et al. (2010). The importance of wood traits and hydraulic conductance for the performance and life history strategies of 42 rainforest tree species. N. Phytol. 185, 481–492. doi: 10.1111/j.1469-8137.2009.03092.x
Pourtahmasi, K., Lotfiomran, N., Brauning, A., and Parsapajouh, D. (2011). Tree-ring width and vessel characteristics of oriental beech (Fagus Orientalis) along an altitudinal gradient in the caspian forests, northern Iran. IAWA J. 32, 461–473.
Pritzkow, C., Wazny, T., Heußner, K. U., Słowinski, M., Bieber, A., Liñán, I. D., et al. (2016). Minimum winter temperature reconstruction from average earlywood vessel area of European oak (Quercus robur) in N-Poland. Palaeogeogr. Palaeoclimatol. Palaeoecol. 449, 520–530. doi: 10.1016/j.palaeo.2016.02.046
Pumijumnong, N., and Park, W. K. (1999). Vessel chronologies from teak in northern Thailand and their climatic signal. IAWA J. 20, 285–294. doi: 10.1163/22941932-90000691
R Development Core Team (2016). R: a language and environment for statistical computing. R Found. Stat. Comput. doi: 10.1038/sj.hdy.6800737
Rahman, M., Islam, M., and Bräuning, A. (2018).Tree radial growth is projected to decline in South Asian moist forest trees under climate change. Glob. Planet. Change 170, 106–119. doi: 10.1016/j.gloplacha.2018.08.008
Richburg, J. A. (2005). Timing Treatments to the Phenology of Root Carbohydrate Reserves to Control Woody Invasive Plants, A Ph.D. dissertation submitted to the University of Massachusetts Amherst.
Rita, A., Borghetti, M., Todaro, L., and Saracino, A. (2016). Interpreting the climatic effects on xylem functional traits in two Mediterranean oak species: the role of extreme climatic events. Front. Plant Sci. 7:1126. doi: 10.3389/fpls.2016.01126
Rita, A., Cherubini, P., Leonardi, S., Todaro, L., and Borghetti, M. (2015). Functional adjustments of xylem anatomy to climatic variability: insights from long-term Ilex aquifolium tree-ring series. Tree Physiol. 35, 817–828. doi: 10.1093/treephys/tpv055
Rungwattana, K., and Hietz, P. (2017). Radial variation of wood functional traits reflect size-related adaptations of tree mechanics and hydraulics. Funct. Ecol. 32, 260–272. doi: 10.1111/1365-2435.12970
Schuldt, B., Knutzen, F., Delzon, S., Jansen, S., Müller-Haubold, H., Burlett, R., et al. (2016). How adaptable is the hydraulic system of European beech in the face of climate change-related precipitation reduction? N. Phytol. 210, 443–458. doi: 10.1111/nph.13798
Sinha, S. K., Vijendra Rao, R., and Deepak, M. S. (2017). Influence of climate on the total vessel lumen area in annual rings of teak (Tectona grandis L.f.) from Western Ghats of Central Karnataka, India. Trop. Ecol. 58, 167–175.
Slik, J. W. F., Arroyo-Rodríguez, V., Aiba, S.-I., Alvarez-Loayza, P., Alves, L. F., Ashton, P., et al. (2015). An estimate of the number of tropical tree species. Proc. Natl. Acad. Sci. U.S.A. 112, 7472–7477. doi: 10.1073/pnas.1423147112
Sperry, J. S., Hacke, U. G., Oren, R., and Comstock, J. P. (2002). Water deficits and hydraulic limits to leaf water supply. Plant Cell Environ. 25, 251–263. doi: 10.1046/j.0016-8025.2001.00799.x
Sperry, J. S., Hacke, U. G., and Pittermann, J. (2006). Size and function in conifer tracheids and angiosperm vessels. Am. J. Bot. 93, 1490–1500. doi: 10.3732/ajb.93.10.1490
Sperry, J. S., Meinzer, F. C., and McCulloh, K. A. (2008). Safety and efficiency conflicts in hydraulic architecture: scaling from tissues to trees. Plant Cell Environ. 31, 632–645. doi: 10.1111/j.1365-3040.2007.01765.x
Sperry, J. S., Nichols, K. L., Sullivan, J. E. M., and Eastlack, S. E. (1994). Xylem embolism in ring-porous, diffuse-porous, and coniferous trees of northern Utah and interior Alaska. Ecology 75, 1736–1752. doi: 10.2307/1939633
Sperry, J. S., Stiller, V., and Hacke, U. G. (2003). Xylem hydraulics and the soil-plant-atmosphere continuum: opportunities and unresolved issues. Agron. J. 95, 1362–1370. doi: 10.2134/agronj2003.1362
Steppe, K., and Lemeur, R. (2007). Effects of ring-porous and diffuse-porous stem wood anatomy on the hydraulic parameters used in a water flow and storage model. Tree Physiol. 27, 43–52. doi: 10.1093/treephys/27.1.43
Stokes, M. A., and Smiley, T. L. (1968). An Introduction to Tree-Ring Dating. Chicago, IL: University of Chicago Press.
Taneda, H., and Sperry, J. S. (2008). A case-study of water transport in co-occurring ring- versus diffuse-porous trees: contrasts in water-status, conducting capacity, cavitation and vessel refilling. Tree Physiol. 28, 1641–1651. doi: 10.1093/treephys/28.11.1641
Tognetti, R., Longobucco, A., Miglietta, F., and Raschi, A. (1999). Water relations, stomatal response and transpiration of Quercus pubescens trees during summer in a Mediterranean carbon dioxide spring. Tree Physiol. 19, 261–270. doi: 10.1093/treephys/19.4-5.261
Tyree, M. T., and Sperry, J. S. (1989). Vulnerability of xylem to cavitation and embolism. Annu. Rev. Plant Physiol. Plant Mol. Biol. 40, 19–38. doi: 10.1146/annurev.pp.40.060189.000315
Tyree, M. T., and Zimmermann, M. H. (2002). Xylem Structure and the Ascent of sap, 2nd Edn. Berlin: Springer Berlin Heidelberg.
Venegas-González, A., von Arx, G., Chagas, M. P., and Filho, M. T. (2015). Plasticity in xylem anatomical traits of two tropical species in response to intra-seasonal climate variability. Trees Struct. Funct. 29, 423–435. doi: 10.1007/s00468-014-1121-z
Venturas, M. D., Sperry, J. S., and Hacke, U. G. (2017). Plant xylem hydraulics: what we understand, current research, and future challenges. J. Integr. Plant Biol. 59, 356–389. doi: 10.1111/jipb.12534
Verheyden, A., De Ridder, F., Schmitz, N., Beeckman, H., and Koedam, N. (2005). High-resolution time series of vessel density in Kenyan mangrove trees reveal a link with climate. N. Phytol. 167, 425–435. doi: 10.1111/j.1469-8137.2005.01415.x
Vlam, M., Baker, P. J., Bunyavejchewin, S., and Zuidema, P. A. (2014). Temperature and rainfall strongly drive temporal growth variation in Asian tropical forest trees. Oecologia 174, 1449–1461. doi: 10.1007/s00442-013-2846-x
Westoby, M., and Wright, I. J. (2006). Land-plant ecology on the basis of functional traits. Trends Ecol. Evol. 21, 261–268. doi: 10.1016/j.tree.2006.02.004
Wigley, T. M. L., Briffa, K. R., and Jones, P. D. (1984). On the average value of correlated time series, with applications in dendroclimatology and hydrometeorology. J. Clim. Appl. Meteorol. 23, 201–213. doi: 10.1175/1520-0450(1984)023<0201:OTAVOC>2.0.CO;2
Williams, L. J., Bunyavejchewin, S., and Baker, P. J. (2008). Deciduousness in a seasonal tropical forest in western Thailand: interannual and intraspecific variation in timing, duration and environmental cues. Oecologia 155, 571–582. doi: 10.1007/s00442-007-0938-1
Zanne, A. E., Lopez-Gonzalez, G., Coomes, D. A. A., Ilic, J., Jansen, S., Lewis, S. L., et al. (2009). Data from: towards a worldwide wood economics spectrum. Dryad Digital Repository. doi: 10.5061/dryad.234
Zanne, A. E., Westoby, M., Falster, D. S., Ackerly, D. D., Loarie, S. R., Arnold, S. E. J., et al. (2010). Angiosperm wood structure: global patterns in vessel anatomy and their relation to wood density and potential conductivity. Am. J. Bot. 97, 207–215. doi: 10.3732/ajb.0900178
Keywords: Bangladesh, hydraulic conductivity, tropical forests, climate change, hydraulic safety, xylem anatomy, safety-efficiency trade-off
Citation: Islam M, Rahman M and Bräuning A (2018) Long-Term Hydraulic Adjustment of Three Tropical Moist Forest Tree Species to Changing Climate. Front. Plant Sci. 9:1761. doi: 10.3389/fpls.2018.01761
Received: 12 July 2018; Accepted: 13 November 2018;
Published: 04 December 2018.
Edited by:
Veronica De Micco, Università degli Studi di Napoli Federico II, ItalyReviewed by:
Ignacio García-González, Universidade de Santiago de Compostela, SpainZe-Xin Fan, Xishuangbanna Tropical Botanical Garden (CAS), China
Copyright © 2018 Islam, Rahman and Bräuning. This is an open-access article distributed under the terms of the Creative Commons Attribution License (CC BY). The use, distribution or reproduction in other forums is permitted, provided the original author(s) and the copyright owner(s) are credited and that the original publication in this journal is cited, in accordance with accepted academic practice. No use, distribution or reproduction is permitted which does not comply with these terms.
*Correspondence: Mahmuda Islam, bWFobXVkYS5pc2xhbUBmYXUuZGU=