- 1Centre of Excellence in Rice Value Addition (CERVA), International Rice Research Institute (IRRI)—South Asia Regional Centre (ISARC), Varanasi, India
- 2International Rice Research Institute, Los Baños, Philippines
- 3Max-Planck-Institute of Molecular Plant Physiology, Potsdam-Golm, Germany
Cereal grains and products provide calories globally. The health benefits of cereals attributed to their diverse phenolic constituents have not been systematically explored. Post-harvest processing, such as drying, storing, and milling cereals, can alter the phenolic concentration and influence the antioxidant activity. Furthermore, cooking has been shown to degrade thermo-labile compounds. This review covers several methods for retaining and enhancing the phenolic content of cereals to develop functional foods. These include using bioprocesses such as germination, enzymatic, and fermentation treatments designed to enhance the phenolics in cereals. In addition, physical processes like extrusion, nixtamalization, and parboiling are discussed to improve the bioavailability of phenolics. Recent technologies utilizing ultrasound, micro- or nano-capsule polymers, and infrared utilizing processes are also evaluated for their effectiveness in improving the phenolics content and bio-accessibility. We also present contemporary products made from pigmented cereals that contain phenolics.
Introduction
Genetic diversity present in cereals is an excellent source of a variety of bioactive chemicals. The polyphenolic molecules in cereal grains include phenolic acids (ferulic acid, ferulic, vanillic, p-coumaric, caffeic, protocatechuic, p-hydroxybenzoic, genistic, chlorogenic, and syringic acids), flavonoids (flavones, flavonols, isoflavones, flavanols, flavanones, anthocyanins), and avenanthramides. Given that some of these compounds are water-soluble and others are insoluble antioxidants, strategies for increasing their bioavailability must be investigated (Jones et al., 2019). Cinnamic acid and its derivatives, especially ferulic acid, are the main phenolic acids found in cereal grains (Zhou et al., 2005). Quercetin glycosides, flavones, and flavonols are the major flavonoids found in cereal grains; however, anthocyanins contribute substantially to the overall flavonoid content of dark-colored grains such as purple maize and purple rice (Francavilla and Joye, 2020). Buckwheat grains have also been shown to contain catechins. Sorghum is one of the few kinds of cereal that contains large amounts of oligomeric and polymeric flavonoids known as condensed tannins (Espitia-Hernández et al., 2020). Purple and red pigmentation in cereals is linked to larger amounts of polyphenols covering a wide array of bioactive compounds such as anthocyanins, proanthocyanidins, flavonoids, phenolic acids, and lignins (Lachman et al., 2018; Mbanjo et al., 2020; Loskutov and Khlestkina, 2021).
The majority of phenolic acids exist in conjugated and bound forms, mostly in the bran (Kim et al., 2006; Chandrasekara and Shahidi, 2010). Lignans, a class of polyphenols with significant health benefits, especially as precursors to mammalian lignans, are plentiful in cereal grains. Whole grain cereals and their products are well-known for containing more protein, minerals, vitamins, dietary fiber, and phytochemicals than their polished counterparts. In cereals, a large proportion of phenolic compounds are concentrated in the bran, where they are typically found as soluble conjugates or covalently linked to sugar moieties or cell wall structural components (Mnich et al., 2020). By cross-linking to lignin, extensins, and glucuronoarabinoxylan components, bound phenolic acids are usually implicated in the structure of the cell wall. In white, red, and black rice grains, ferulic, p-coumaric, syringic, and isoferulic acids are found in bound forms (Shao et al., 2014). Further, they may be physically trapped inside cereal matrices and intact cells (Acosta-Estrada et al., 2014; Wang et al., 2014a; Wang Z. et al., 2020). Due to the fact that these chemicals are often found bonded, organic solvents cannot usually remove them. When coupled with increased dietary fiber content in whole-grain foods, increased levels of bioactive phytochemicals such as phenolic compounds, sterols, tocols, and lignans have been found to protect against non-communicable illnesses (Derrien and Veiga, 2017). Given that the bulk of phenolics are contained in the bran or seed coat of grains, it is more beneficial to consume whole grains and intact seed-based meals. Numerous in vivo and in vitro studies on the health benefits of cereals have clearly shown that diets high in whole grain cereals and cereal-based products contribute to the prevention of a range of chronic diseases with significant public health implications (Chen et al., 2019; Francavilla and Joye, 2020).
Numerous variables may affect the content and possible health benefits of meals along the value chain. The concentration of these bioactives in cereals, food components, and dietary supplements, all of which can have an effect on human health and wellbeing, may be influenced by genetics, growing and storage conditions, post-harvest treatments, food formulation, and processing. Postharvest processing of cereal grains is critical to making them preferentially edible and biofunctional and impart distinctive features for better cooking and organoleptic aspects (Oghbaei and Prakash, 2016). These processes include drying, grinding, and storing, contributing to the product’s shelf life, stability, and palatability. Numerous cooking techniques, particularly thermal processing techniques such as microwave heating, roasting, frying, steaming, autoclaving, boiling, extrusion, and baking, have been extensively used to improve the palatability and taste of cereal grains and their products. Several pre-and post-harvest methods have been devised to liberate bound phenolics from the indigestible matrix and boost the free-soluble phenolics content (Wang et al., 2014b; Călinoiu and Vodnar, 2019). The effects of various pretreatment procedures on specific phenolic compounds are shown in Table 1, while Supplementary Table 1 summarizes the change in the antioxidant capacity of the total phenolic content. Understanding the effect of various processing methods on phenolic compounds in grain is critical for maintaining or enhancing these compounds’ health-promoting qualities in processed cereal products (Kadiri, 2017).
Most of the processing approaches alter the polyphenol composition of cereals and their products and improve the availability and digestibility of phenolics due to the chemical or physical modifications that occur during processing (Oghbaei and Prakash, 2016; Ribas-Agustí et al., 2017). In comparison, several of these post-harvest processing methods have been shown to degrade the natural phenolic components in the end products, resulting in decreased bioavailability during in vivo digestion (Ribas-Agustí et al., 2017). Hence in this review article, we detail the chemical diversity of bioactives identified in cereal crops and comprehensively review the implications of (a) bioprocessing methods such as germination, enzymatic treatment, and fermentation, and (b) novel technological processing methods such as ultrasonication, parboiling, micro- and nano-encapsulation, infrared, and pulse electric field methods for improving the stability of phenolic compounds in functional foods and for specific delivery of cereal phenolic compounds with increased bioavailability (Figure 1). To collect as many relevant citations as feasible, a broad variety of scientific databases were searched. Only data from literature published until 31st August 2021 obtained from the following databases (Google Scholar, PubMed, SciELO, and Scopus) were included in this review. To this end, the following keywords and their combinations were used: cereal, grains, wheat, rice, corn, barley, oat, rye, millet, sorghum, phenolics, phenolic acids, flavonoids, anthocyanin, processing, post-harvest, thermal, extrusion, nixtmalization, microwave, ultrasound, microencapsulation, micronization, microfluidization, enzyme, fermentation, baking, pulsed electric field, product, development, cardiovascular disease, obesity, inflammation, diabetes, or glucose.
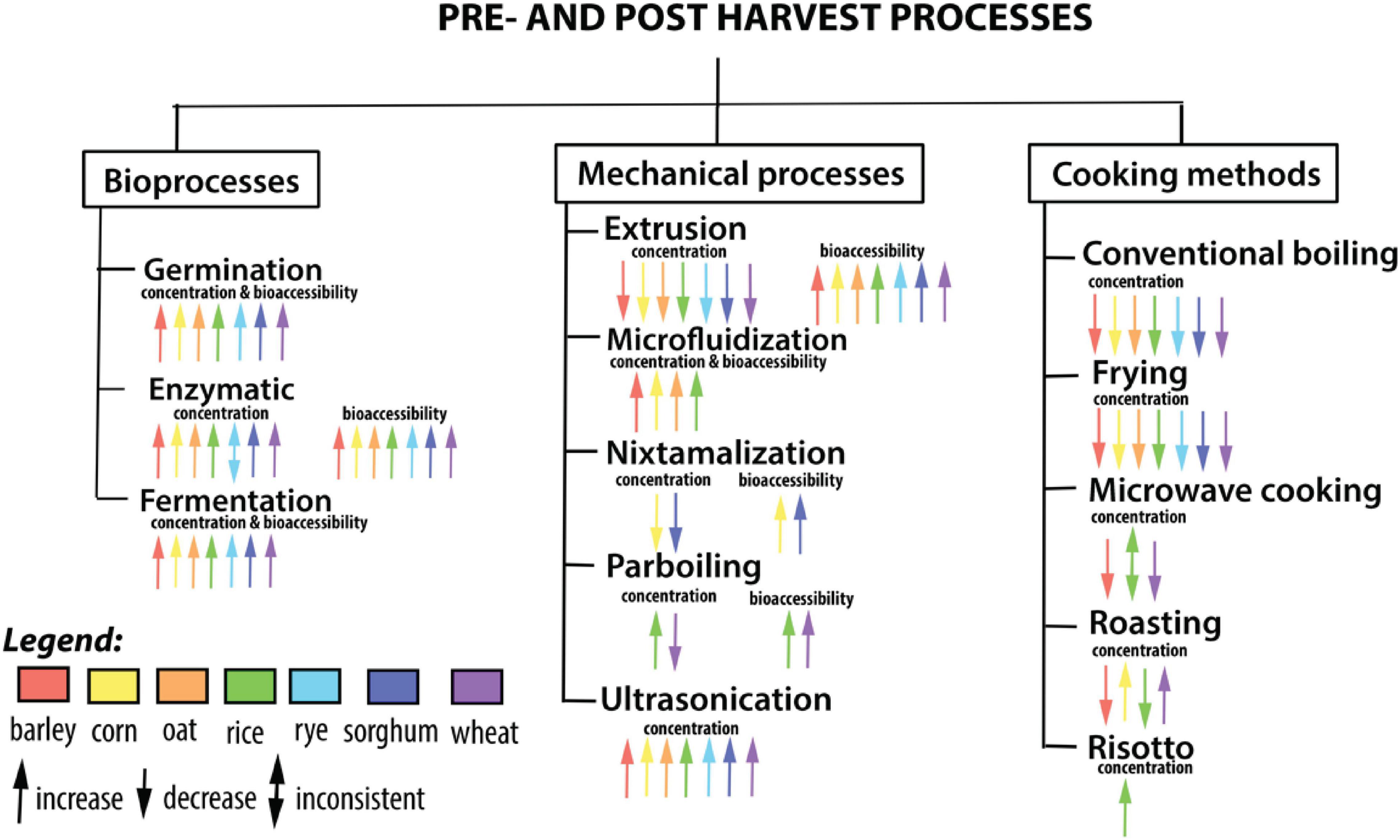
Figure 1. Influence of bioprocesses, mechanical processes, and cooking methods on the concentration and bioavailability of phenolic compounds.
Phenolic Compound-Rich Bioactive Ingredients in Cereals for Sustained Human Health
Recent years have seen the discovery of novel functions for phenolic chemicals, especially flavonoids. Indigenous farmers have preserved the medicinal characteristics of grain types, landraces, cultivars, and wild forebearers for their therapeutic potential. These traits are now attracting considerable scientific attention. For example, Njavara, a Kerala medicinal rice, is widely utilized in Ayurveda to treat neurological disorders and regeneration. Phytochemical assessments and spectroscopic examinations of the diethyl ether extract of methanolic concentrate of Njavara rice bran resulted in the identification of two rare flavonolignanstricins, namely 4′-O-(erythro-β guaiacylglyceryl) ether and tricin 4-O-(threo-β-guaiacylglyceryl) ether (Rao et al., 2010; Jung et al., 2014). It is now recognized that flavonoids may influence cell signaling pathways at physiological doses much below those needed to impact cellular antioxidant activity (Koch et al., 2014). Through their regulation of cell signaling pathways, flavonoids may help prevent cancer by increasing phase II detoxifying enzyme activity, decreasing proliferation, and triggering apoptosis. When human participants were fed pigmented black rice high in flavonoids, serum polyphenols and flavonoids levels were shown to be enhanced compared to baseline values (Vitalini et al., 2020). Bioactive compounds found in cereals possess antioxidative properties, which in turn counter various diseases such as heart disorders, cancer, aging, and inflammatory diseases (Espitia-Hernández et al., 2020). According to Ghasemzadeh et al. (2018), the free fraction of black rice bran had a higher IC50 value than the free fractions of red and white rice bran. Several phytochemicals found in pigmented rice prevent certain types of cancer (Henderson et al., 2012; Forster et al., 2013; Chatthongpisut et al., 2015). Through activation of effector genes, the p53 protein, and caspase enzymes, the proanthocyanidin-rich fraction isolated from red rice germ and bran extract may suppress cell growth and cause death in HepG2 cells (Wongjaikam et al., 2014; Upanan et al., 2019). This may provide a novel target for cancer treatments.
Shan et al. (2020) demonstrated that the bound fraction of foxtail millet bran regulates the expression of miRNAs to exert anti-multidrug resistance against colorectal cancer. Likewise, the enzymatic extraction of rice bran has anti-cancer potentiality against leukemic cell lines (Revilla et al., 2013). Additionally, blue corn and barley reduced the frequency of colon malignancies caused by 1,2-dimethylhydrazine and mammary carcinogenesis caused by N-methyl-N-nitrosourea, respectively (Reynoso-Camacho et al., 2015; Kubatka et al., 2016). Long-term consumption of an anthocyanin-rich extract from black rice on a continuous basis may aid in the stabilization of plaques in elderly apoE-deficient mice (Xia et al., 2006). Furthermore, black rice extracts act as antioxidants by suppressing cellular reactive oxygen species (ROS) and malondialdehyde (Lee et al., 2014).
Cyanidin-3-glucoside, the main anthocyanin compound of black rice, suppresses the occurrence of high-fat-diet-induced obesity (Kongthitilerd et al., 2020), increases hyperglycemia and insulin sensitivity via AMP-activated protein kinase in type 2 diabetic mice (Takikawa et al., 2010). The black rice pigment fraction significantly decreased plasma levels of soluble vascular cell adhesion molecule-1 (sVCAM-1), soluble CD40 ligand (sCD40L), and high sensitivity C-reactive protein (hs-CRP) in patients with coronary heart disease. In terms of the antidiabetic property, oryzanol has been reported to directly correlate with insulin sensitivity and adiponectin, indicating that it plays a critical role in type 2 diabetes (Ohara et al., 2009). Son et al. (2011) showed that oryzanol could control insulin production to maintain glucose homeostasis, correct liver enzymes’ activity, and decrease the risk of hyperglycemia associated with a high-fat diet. A diet intervention supplemented with 0.160% oryzanol and 0.05% ferulic acid alleviated hyperglycemia developed in a group of rats for 13 weeks utilizing a high-fat, high-fructose diet (HFFD) (Wang et al., 2015). Its nutritional quality and medicinal values have made rice unique among cereals. Therefore, some traditional rice varieties and other sources of cereal grains can now be portrayed as functional foods when we deploy novel processing technologies to retain higher antioxidant and anti-cancer properties in the final food products.
Bioprocessing Applications to Attain Optimum Phenolic Compounds in Cereals
Germination
Germination, also known as sprouting, softens the kernel upon imbibition and increases nutritional bioavailability. It is an efficient method of increasing bioactives, such as the phenolic content of grains and pulses (Ti et al., 2014; Xu et al., 2021). It has been found that germinated wheat and brown rice have more phenolic compounds, both free and bound, than ungerminated grains (Ti et al., 2014; Bei et al., 2018; Xu et al., 2021). Among the phenolics, the amount of bound ferulic acid and p-coumaric acid rose significantly during wheat and rice sprouting (Bei et al., 2018). In wheat, it is reported that the germination temperature and length have a beneficial effect on the accumulation of soluble phenolic acids, flavone C-glycosides, and lignans (Tomé-Sánchez et al., 2020). Germinated sweet corn, oat, and buckwheat grains also had much more phenolic compounds than ungerminated grains (Xu et al., 2009; Zhang et al., 2015; Xiang et al., 2017). Germination substantially altered the profile of phenolic compounds in naked barley, and the level of phenolic compounds rose significantly when germination was extended up to 36 h (Ge et al., 2021).
Enzymatic Treatments
Enzymatic hydrolysis is often used to prepare cereal grains and their constituents, such as starch, protein, and bran, with the goal of enhancing their nutraceutical qualities and developing functional foods (Prabhu and Jayadeep, 2015). These enzymatic techniques are claimed to improve the phenolic content and bioavailability of phenolic compounds (Lima et al., 2018). However, it has also been found that the increase in phenolic content depends on the type of bran and enzyme application method used (Prabhu and Jayadeep, 2015). Rice extruded with thermostable α-amylase considerably increased the retention of total phenolics from 50.85 to 87.73% compared to conventionally cooked or extruded rice. Likewise, treatment of rice bran with a complex enzyme hydrolysis (glucoamylase, protease, and cellulase) substantially enhanced the total phenolics (46.24%) and flavonoids (79.13%) contents, respectively. Significant amounts of these phenolic compounds were released in the form of soluble conjugates rather than in their free state. Among the different phenolic acids and flavonoids, ferulic acid was the most abundantly produced, followed by protocatechuic acid and quercetin (Liu et al., 2017). Feruloyl esterase and pentopan have been shown to release ferulic acid from wheat bran selectively, and pretreatment of the bran with alcalase and termamyl enhanced ferulic acid production by up to 20 times (Ferri et al., 2020). Bread enriched with bioprocessed wheat bran through xylanase enzyme and yeast fermentation improved ferulic acid bio-accessibility (Amaya Villalva et al., 2018). Furthermore, wheat aleurone fractions treated with xylanase alone or in combination with feruloyl esterase improved ferulic acid bioavailability in obese mice fed a high-fat diet. Increased aleurone-released metabolites resulted in weight loss, decreased adiposity, increased fasting leptin levels, and better glucose metabolism (Pekkinen et al., 2014).
The cellulase treatment of oats liberated a significant quantity of ferulic acid in the insoluble fraction (Bei et al., 2018). The addition of commercial carbohydrases to maize flour resulted in an increase in the overall phenolic acid content, including ferulic acid (Cho et al., 2018). In vitro multienzymatic digestion of highland barley resulted in a more outstanding phenolic content than chemical extraction (Zhu et al., 2016). In whole rye flour, tannase treatment was found to increase the total phenolics and the amounts of ferulic, sinapic, and vanillic acids. However, phenolic acids’ bio-accessibility and transit efficiency were decreased when whole rye flour was treated with tannase compared to untreated whole rye flour (Lima et al., 2018).
Fermentation
Fermentation has been extensively utilized in the food business to increase cereal grains’ shelf life, nutritional content, and organoleptic characteristics (Frias et al., 2005). Fermentation is also a viable technique for increasing foods’ phenolic content and bioavailability (Adebo and Gabriela Medina-Meza, 2020). Several widely eaten cereals, including rice, wheat, oat, maize, and sorghum, have been enhanced with phenolic content through fermentation (Saharan et al., 2017). For 24 h at 37°C, fermentation of the saccharified solution of extruded brown rice with co-cultures of Lactobacillus plantarum, Lactobacillus fermentum, and Saccharomyces cerevisiae significantly increased the content of free, conjugated, and bound phenolics and flavonoids, including bio-accessible phenolics (Khan et al., 2020). Using solid-state fermentation techniques, wheat grains from different cultivars fermented with a fungal strain Aspergillus awamori, significantly increased their total phenolic content (Sandhu et al., 2016). Likewise, fermentation with probiotic strains Lactobacillus johnsonii LA1, Lactobacillus reuteri SD2112, and Lactobacillus acidophilus LA-5 significantly increased free phenolic acids from 2.55 to 69.91 μg g–1 and 4.13 to 109.42 μg g–1 dry mass in whole grain barley and oat groat, respectively (Hole et al., 2012). In oats, fermentation with Monascus anka significantly increased the phenolics content, especially ferulic acid in the insoluble fraction and the vanillic acid in the soluble fraction. This research demonstrated that Monascus anka carbohydrate-hydrolyzing enzymes mediated the mobilization of phenolic compounds from fermented oats. Additionally, xylanase and cellulase were critical in degrading the cellular structure (Bei et al., 2018). Fermentation of germinated rye raised the amount of free phenolic acids, total phenolic compounds, and lignans by a significant amount (Katina et al., 2007). In maize, solid-state fermentation using Thamnidium elegans CCF 1456 was shown to be helpful for increasing total phenolics (Salar et al., 2012).
As with whole grain, fermentation of rice, wheat, and rye bran fractions increase their phenolic content and bio-accessibility. As defatted rice bran was fermented, it improved the bioavailability of phenolics by 64.4% when compared to raw bran (Chen et al., 2019). Likewise, fermentation of wheat bran with Aspergillus species resulted in the release of bound phenolic acids such as ferulic acid, chlorogenic acid, and syringic acid (Yin et al., 2018). Fermentation of rye bran increases the amount of total phenolics and free ferulic acid that are readily extracted (Katina et al., 2007).
Innovative Processing Technologies to Increase the Phenolic Compounds in Cereal Bran
Micronization and Microfluidization
In the fibrous matrix of cereals, phenolic compounds have limited access for digestion in the upper gastrointestinal tract due to their bound nature. Recently, advanced techniques like micronization (the process of decreasing the average diameter of the particles in a solid substance) and its modified version, microfluidization (high-pressure homogenization that creates very fine emulsion), have been found to be useful in overcoming this issue and improving the functional properties of cereal-based products such as wheat bran, corn bran, and rice bran by reducing particle size and modifying their microstructure (Wang et al., 2013, 2014a; Mert, 2020). It has been found that micronization retained the antioxidant levels of durum wheat kernels even after cooking (Martini et al., 2017), and wheat bran treated with microfluidization process increased the contents of surface-reactive and hydrolyzable phenolics (Wang et al., 2013). Antioxidant activities as measured by FRAP of micronized proso millet bran and buckwheat hulls were 78 and 23.33% higher than control, respectively (Zhu et al., 2014; Čukelj Mustač et al., 2020). Similarly, microfluidization treatment to corn bran considerably increased its antioxidant capacities by increasing the accessibility of phenolic compounds bound to or inside the bran matrix (Wang et al., 2014b). The bioavailability of bound phenolic compounds in cereals was enhanced when subjected to microfluidization. Mert (2020) has extensively reviewed the application of microfluidization in corn, rice, and wheat. However, the application of microfluidization in other cereals like barley, oat, rye, and sorghum remains relatively limited.
Extrusion
Extrusion is a thermomechanical process, which changes the structural and functional properties in the extruded material due to the exposure to high temperature, pressure, and shear forces for a short period of time (Ramos-Enríquez et al., 2018; Zhang et al., 2018). These extrusion processes are useful in increasing the phenolic contents and improving their bio-accessibility in cereal-based products. It has been shown that ingestible phenolic compounds in wheat bran can be increased by optimizing the extrusion process (Ramos-Enríquez et al., 2018). Improved extrusion cooking treatment significantly increased the total bound phenolic acids of brown rice, wheat, and oat by 6.45, 8.78, and 9.10%, respectively. The observed effect depended on the cereal matrix and the sensitivity of free and bound phenolics (Zeng et al., 2016). In black rice bran, the total phenolics and anthocyanins were increased after extrusion processing, whereas these components were significantly reduced in polished and brown rice by extrusion processing (Ti et al., 2014). The bio-accessibility of phenolics was also found to be increased by 40.5% in extruded rice bran after in vitro digestion compared with raw rice bran (Chen et al., 2019). Studies conducted in a pig model showed that the extrusion of barley and oat improved the bio-accessibility of dietary phenolic acids compared with whole-grain barley and dehulled oat (Hole et al., 2012).
Parboiling
Parboiling is a pre-milling hydrothermal process that comprises three main steps, soaking, steaming, and drying. It is generally used to gelatinize starch in the grains to seal fissures to improve the milling yield of cereals (Rocha-Villarreal et al., 2018; Zhu et al., 2020). Parboiling has been found that the crushed fractions of parboiled or bulgur einkorn wheat from Turkey showed minimal loss of phenolic compounds compared to crushed emmer fractions (Giambanelli et al., 2016). In rice, the effect of parboiling on the content of phenolic compounds and their preservation has been more profoundly studied compared with other cereals such as wheat, maize, and barley. Parboiled milled rice was found to have higher free and bound phenolic acids as compared to non-parboiled milled rice (Pal et al., 2018). It has also been found that parboiling allowed the partial preservation of free phenolics content in polished rice (Paiva et al., 2016). However, it is important to note that the parboiling effect on bioactive compounds varies according to the intrinsic properties of the grain and the processing conditions (Rocha-Villarreal et al., 2018). Hu et al. (2017) reported that germinated red rice parboiled for 5 and 15 min had higher total free phenolic content and antioxidant activity than non-parboiled germinated red rice (Hu et al., 2017). With the increase in parboiling time from 5 to 15 min, free p-coumaric acid increased from 0.20 to 0.67 mg/100 g. At 0, 2, and 5 min, bound vanillic (0.17–0.27 mg/100 g) and p-coumaric acid (6.56–8.59 mg/100 g) were at higher levels. These results indicated that thermal treatment deployed in parboiling disrupts the cell wall matrix of bran and endosperm, which helped release the bound phenolic compounds as free form having higher antioxidant capacity.
Ultrasound Processing
Ultrasound technology is widely used in the food processing industry due to its low cost and improved final product quality features. In the food processing industry, ultrasound is used in the 20 kHz to 10 MHz frequency range. High-frequency ultrasound is used to study the physicochemical properties of food, such as acidity, firmness, and sugar. Conversely, low-frequency ultrasound is used to bring changes in the physical and chemical properties of food (Majid et al., 2015). Bonto et al. (2021) recently summarized the use of ultrasound in increasing the nutritional components of rice. In cereal product processing, ultrasound technology is used to improve the stability of products and to extract bioactive compounds, including polyphenols. For instance, ultrasound pre-treatment of wheat-dried distiller’s grain particles, a coproduct from the ethanol production process, increased the extraction yield of phenolic compounds by 14.29% (Izadifar, 2013). In rice grains, rapid extraction of phenolics from rice grains is achieved using ultrasound-assisted extraction (Setyaningsih et al., 2019). Ultrasonication has also activated, and deactivated enzymes related to polyphenolic compounds, affecting the nutritional quality of cereal bran products (Čukelj Mustač et al., 2020). Furthermore, ultrasound can improve the hydration during the germination process, accelerating the sprouting and enhancing the nutritional benefit (Miano et al., 2016). Indeed, ultrasound can be used to improve the extractability of the phenolic compounds from the cereal matrix, which can potentially be used for developing functional food products.
Nixtamalization
Nixtamalization, also known as alkaline cooking, is a pretreatment used to alter the processing characteristics of corn. It is a traditional process in Mexico and Central America to convert corn to other products; however, it has been improved over the last decade. The process involves cooking corn in an oversaturated alkaline solution, typically, calcium hydroxide solution, for 30–40 min followed by steeping for 8–16 h. The cooked corn kernels, called nixtamal, are rinsed to remove excess lime and ground (Serna-Saldivar and Rooney, 2015; Niu and Hou, 2020). This technique dissolves the hemicellulose, alters its rheological properties, promotes protein bonding, reduces antinutrients and aflatoxins to enhance the dietary value of cereals (Schaarschmidt and Fauhl-Hassek, 2019; Cabrera-Ramírez et al., 2020; Kamau et al., 2020; Luzardo-Ocampo et al., 2020; Sunico et al., 2021). The nixtamalization method has also been investigated as a technology for increasing the number of phenolic chemicals in food (Salazar-López et al., 2018). While heat caused a reduction in the total phenolics, a significantly high concentration of ferulic acid was retained in the product, resulting in increased antioxidant activity (Gaxiola-Cuevas et al., 2017). The nixtamalized product called nejayote has been shown to have more phenolic acids and antioxidants than raw corn, which may be attributed to the hydrolysis of ester linkages that then liberated the phenolic acids and increased their bioavailability (Méndez-Lagunas et al., 2020). This technique has been applied to make flour and dough from other cereal grains aside from corn. One such study by Luzardo-Ocampo et al. (2020) demonstrated that nixtamalization improved the bio-accessibility of phenolics and flavonoids in sorghum. Among all phenolic compounds, gallic and chlorogenic acid had the most bioaccessibility. Moving forward, significant effort needs to be made to deploy the effect of nixtamalization on the antioxidant properties of cereal grains and their products in unraveling the contribution of specific phytochemicals to antioxidant potency.
Pulsed Electric Fields
PEFs are a family of non-thermal food processing technologies that have recently transitioned from the laboratory to the food industry. It is primarily based on an electroporation process that includes the formation of holes in cellular membranes (Pérez-Andrés et al., 2018; Zhang et al., 2021). Brief electric pulses (1–100 s) generated by two high-voltage electrodes across a range of electric field strengths ranging from 0.1 to 80 kV/cm result in the reversible permeabilization of plant cells (Wang et al., 2018). These processing methods allow the manufacture of safe, high-quality food items rich in nutritional value, exceptional flavor, and long shelf life (Raso et al., 2014). PEF has been investigated for its ability to enhance the phenolic content of cereal grains. Lohani and Muthukumarappan (2016) showed that optimizing PEF at a flour-to-water ratio of 45% (w/v), a 2 kV/cm electric field strength, and an exposure time of 875 s resulted in a 24.8% increase in total phenolic compounds and a 33.9% increase in antioxidant activity in sorghum flour. Additionally, PEF enhanced the bioactives isolated from brown rice (Quagliariello et al., 2016). Brown rice extracts treated with PEF (2 kV/cm, 1,000 pulses, 64 kJ/kg) showed 50% higher DPPH levels and cytotoxic activity against colorectal cancer cells than untreated samples rice (Quagliariello et al., 2016). Currently, only a few studies have been conducted to determine the suitability of PEF for cereal grain processing. As a result, there is a lack of data on the sensory features, physicochemical impacts, and biochemical reactions of cereals treated with PEF.
Infrared Heating
Infrared (IR) heating has grown in favor in recent years for a number of thermal food preparation procedures, including cereal grain roasting. Processed food products sustain less thermal damage due to the uniformity of IR heating and the short length of the process. As a result, the method has been used lately to enhance polyphenol and antioxidant recovery (Aboud et al., 2019; Faturachman and Indiarto, 2021). Wanyo et al. (2014) determined that IR treatment at an intensity of 2 kW/m2 and temperature of 40°C for 2 h effectively increased the phenolic content and antioxidant activities of rice bran and husk relative to hot air and enzymatic treatment using cellulase (Wanyo et al., 2014). Following IR exposure, the percent inhibition of DPPH from rice bran and husk rose from 88 to 92% and 91 to 93%, respectively. Heated air and cellulase treatments, on the other hand, had no effect on the samples’ antioxidant activities, which supports the hypothesis that the increase was driven by FIR radiation rather than heat or enzymatic activity. Similar findings were made by Irakli et al. (2018) in a study that employed an IR heating of 140°C for 15 min (Irakli et al., 2018). Rice bran samples showed a greater phenolic content and antioxidant activity under these optimal treatment settings. Additionally, the samples lost minimal vitamin E and incurred no change in oryzanol levels or fatty acid composition. These recent findings may serve to further justify the usage of IR as a future food processing alternative. Additional scientific research is needed to elucidate the interaction of food components exposed to IR radiation and its effect on the physicochemical properties, sensory properties, and nutritional values of food components.
Micro-/Nano-Encapsulation
Encapsulation techniques such as micro-and nano-encapsulation have been increasingly used to improve storage stability, bioavailability, and targeted delivery of various food-bioactive compounds, including phenolic compounds (Assadpour and Jafari, 2019). Encapsulation can be achieved by using several physical, chemical, and physicochemical processes such as spray drying, melt extrusion, melt injection, fluid bed coating, emulsification, and liposome entrapment (Faridi Esfanjani et al., 2018; Kasote et al., 2018). Among these, liquid-based encapsulation (emulsion/nanoemulsion, solid lipid nanoparticles, and liposomes/nanoliposomes) is considered as one of the most promising techniques for protection and delivery of polyphenols due to its high-efficiency encapsulation, maintenance of chemical stability, and controlled release (Lu et al., 2016; Ozkan et al., 2020). Moreover, compared with micro-sized carriers, nanocapsules based on lipid formulations provide more surface area and thereby enhance solubility, improve bioavailability, and increase the controlled release of the nano-encapsulated phenolic compounds (Faridi Esfanjani et al., 2018).
Ferulic acid encapsulated in chitosan nanoparticles showed four times enhanced bioavailability in systemic circulation compared to its free form. Moreover, this also had higher antidiabetic potential with minimal toxicity than its free form (Panwar et al., 2018). Similarly, encapsulation of hydroxycinnamic acids such as ferulic, caffeic, sinapic, and coumaric acids in lipid-core nanocapsules protects and releases them in the simulated gastric fluid (Granata et al., 2018). Available literature showed that encapsulation techniques had not been effectively used to encapsulate crude cereals phenolics or their phenolic-rich fractions so far. However, grain components such as starch and phospholipids have been considerably used as encapsulating agents in the food industry. Rice−bran phospholipids were used to encapsulate quercetin in nanoliposomes, and these nanoliposomes were found to enhance the radical−scavenging and anti−angiogenic activities of quercetin (Rodriguez et al., 2019).
Pigmented Cereals as Future Functional Foods and Nutraceuticals
Interest in nutraceuticals and functional foods continues to increase due to growing public interest and consumer demand (AlAli et al., 2021). As a response, food scientists, dietitians, and food industrialists are collaborating to create new functional food products. Pigmented cereal grains such as blue and purple wheat, red and black rice, purple, blue, red, and pink maize, black, blue, and purple barley, and black, purple, red, and lemon-yellow sorghum, all of which contain a variety of functional bioactive components such as polyphenols, anthocyanins, flavonoids contributing to higher antioxidant activity, that can be used to help prevent chronic diseases such as cancer, type 2 diabetes, and hypertension. Colored cereal grains serve as an attractive supplement or whole grain ingredient in the production of functional baked goods such as pan bread, flat bread, buns, rusk, cookies, extruded snacks, breakfast cereals such as pigmented cereal flakes, pigmented popped cereals, snack bars, non-alcoholic beverages, and porridges, as well as in the enhancement and retrofitting of traditional food products. Therefore, the positive health benefits of whole pigmented cereal grains, their bran fraction, phytochemicals, and antioxidant activity as described in this review article may be leveraged by the food industry to create novel nutraceutical cereal-based foods.
Pigmented barley (15.3–132.3 mg Cyn-3-OGlu equiv/kg DM; Suriano et al., 2019), corn (389–7,800 mg Cyn-3-OGlu equiv/kg DM; Suriano et al., 2021), rice (79.5–473.7 mg Cyn-3-OGlu equiv/kg DM; Chen et al., 2012), sorghum (8.62–67.97 mg Cyn-3-OGlu equiv/kg DM; Dykes et al., 2009) and wheat (14.36–27.76 mg Cyn-3-OGlu equiv/kg DM; (Wang X. et al., 2020) contain a relatively higher concentration of anthocyanins and other nutrients than their non-pigmented counterpart. This has resulted in the conversion of colored cereals into a variety of functional foods with increased nutritional value and health advantages. Figure 2 illustrates a variety of food products derived from pigmented cereal grains. Other potential functional foods are shown in Table 2.
Fermented cereal-based meals and beverages have a higher nutritional value owing to an increase in phytochemicals and a reduction in anti-nutrients (Blandino et al., 2003; Das et al., 2011). As shown in Table 3, cereal-based products maintain a high level of phenolics. Recent research has shown the potential for fermented pigmented cereals to increase their nutritional value by boosting phytochemicals such as phenolic compounds and reducing their antinutrient content. Chicha, a traditional Peruvian fermented beverage made from purple maize, has been found to have enhanced phenolics, anthocyanins, and antioxidant capacity while decreasing starch digestibility (Vargas-Yana et al., 2020). A nearly 10-fold rise in gallic acid, catechin, vanillin, and resorcinol was found in pigmented barley (Bangar et al., 2021). The malting process of pigmented rice increases the phenolic acids and GABA (Santos et al., 2020). When several types of fermented rice were examined, black rice had the highest polyphenolic content and antioxidant activity, followed by red and unpigmented rice (Cai et al., 2019). Apart from its nutritional benefits, fermented black rice has good sensory qualities (Jiang et al., 2020), enhancing the product’s use when transformed into fermented snacks and beverages (dela Rosa and Medina, 2021). Pigmented cereals have also been considered in sourdough bread due to the abundance of its phytochemicals and revealed effective anti-inflammatory activities (Luti et al., 2021). Notably, fermentation conditions must be adjusted to get the highest polyphenolic content and antioxidant activity possible. For example, metabolomic analyses of fermented black rice have shown a reduction in phenolic chemicals after 60 h, which may be a result of molecular breakdown and bacterial consumption (Mu et al., 2019). While fermented colored barley, maize, and rice have been widely researched for their nutritional benefits, other pigmented cereals have received less attention. Metabolomics study of fermented pigmented grains has the potential to provide new light on the phenolic diversity and its interaction with microorganisms. Natural pigments such as anthocyanins and proanthocyanidins found in pigmented grains have been used to increase the nutritional content and prolong the shelf life of food items (Chatham et al., 2020). Due to the low price attached to broken-colored cereal, grains may be processed to create food additives (Sapna and Jayadeep, 2020). The encapsulation of phenolic compounds is used as a co-ingredient in bread goods (Papillo et al., 2018; Thiranusornkij et al., 2019), food supplements (Norkaew et al., 2019), beverage hydrogels (Guo et al., 2018), and probiotic products.
Physical mixing of cereal grains rich in phenolic compounds with polymers and food matrix resulted in various product additives and biopolymers (Tiozon et al., 2021). The addition of red sorghum to pasta enhanced the amount of free phenolic acids and resistant starch (Khan et al., 2013). Meanwhile, the inclusion of black rice extracts extends the shelf life of muffins and improves their eating quality (Croitoru et al., 2018). Combining pigmented grain starch has a twofold purpose: starch interacts with other polymers to increase their hydrophilicity, and its phenolic chemicals provide antioxidant qualities. When black rice extracts are combined with a chitosan-starch film, the antioxidant and light barrier characteristics are enhanced (Yong et al., 2019). Additionally, purple corn extracts containing chitosan and silver nanoparticles improved the film’s pH sensitivity and antibacterial activity (Qin et al., 2019). The resultant nanocomposite film based on pigmented grains has been shown to monitor the deterioration of pork (Yong et al., 2019) and seafood items (Ge et al., 2020). The change in pasting, thermal, and rheological characteristics is due to the complexation of phenolic chemicals with starch and other polymers (Zhu, 2015).
Extruded pigmented cereals have gained significant attention due to their nutritive value and ease of consumption. By and large, the overall phenolic content is decreased, but their bioavailability is increased (Hole et al., 2013). For instance, extruded puffed rice increased extractable phenolic acids, namely gallic acid and protocatechuic acid, but significantly decreased cyanidin-3-glucoside (Bagchi et al., 2021). In contrast, extruded blue corn showed cyanidin-3-glucoside stability and retention, which may be related to previous nixtamalization (Escalante-Aburto et al., 2013). Enzymes may be used to enhance the retention of phenolics in extruded goods (Zeng et al., 2018). Additionally, as shown with extruded sorghum, controlling factors such as feed moisture and extrusion zone temperature may avoid additional phenolic loss (Ortiz-Cruz et al., 2020). Other components can be added in cereals like vegetable (Xu et al., 2021) and fruit (Bhat et al., 2019) extracts. Extrusion-based three-dimensional technology has emerged as a new mode to diversify products without sacrificing their nutritional or sensory qualities (Prabha et al., 2021).
Conclusion
In general, promoting wholegrain cereal grain consumption over milled endosperm to prevent non-communicable diseases is widely accepted. However, owing to rancidity, poor shelf-life, and reduced palatability, different processing methods are being used to produce milled goods for broader consumption. As a consequence, we lose the nutritional density found in grains, such as rice bran. The enormous genetic diversity that exists for enriched phenolic chemicals and flavonoids in cereals as purple, variable purple, and red cereal grains has provided new possibilities to market them as future functional food. However, it is critical to evaluate the final concentrations of these free phenolic compounds in pigmented food items after cooking/baking to guarantee bioavailability of these phytochemicals and food safety concerns to remove aflatoxins mycotoxins, and pesticides to translate human health benefits. Identifying the optimal post-harvest processing techniques to maintain greater polyphenolic compounds via traditional cooking and baking processes should be encouraged to preserve the human health advantages. More than 150 million tons of wheat bran and 76 million tons of rice bran nutritional material are produced as a byproduct of milling and are mostly discarded. It can be channeled into technological advancements in processing technologies such as micronization, microfluidization, ultrasound processing, nixtamalization, pulsed electric fields, and micro/nano-encapsulation to extract bioactives and phenolic compounds and produce various functional byproducts with enormous human health benefits.
Furthermore, bioprocessing techniques to produce different functional food products from germination sprouts, fermentation and enzymatic treatments of bran and pigmented grains to enhance the bioaccessibility of phenolic compounds in producing distinct functional byproducts may be used. Implementing holistic strategies to identify donor lines with enriched bioactives using untargeted metabolite profiling to identify novel phenolic compounds with higher antioxidant potential and deploying state-of-the-art processing applications to produce final food products/by-products with higher bioactives will be beneficial in creating novel opportunities to position cereals as staple foods to meet human nutrition needs. Long-term clinical studies for the whole range of functional food items produced from cereals are required to determine long-term benefits. Additional research on the gut-microbiome health advantages of colored grains is needed. We would like to highlight that the consumption of pigmented cereals and their derived functional foods as staples by definition means that improving staples-based diets can deliver maximum benefits, particularly to the poorer sections of society, while also satisfying the urban consumers’ health concerns.
Author Contributions
NS conceptualized the review article and edited the article with inputs from DK, RT, KS, HI, PR, AK, and AR. All authors contributed to the article and approved the submitted version.
Funding
Authors acknowledged funding support from the RICE CGIAR Research Program, AGGRI for BMGF funding, the United Kingdom Biotechnology and the Biological Sciences Research Council United Kingdom Research and Innovation program (Project BB/T008873/1), the Agricultural and Processed Food Products Export Development Authority (APEDA), the Department of Agriculture and Farmers welfare, Government of India.
Conflict of Interest
The authors declare that the research was conducted in the absence of any commercial or financial relationships that could be construed as a potential conflict of interest.
Publisher’s Note
All claims expressed in this article are solely those of the authors and do not necessarily represent those of their affiliated organizations, or those of the publisher, the editors and the reviewers. Any product that may be evaluated in this article, or claim that may be made by its manufacturer, is not guaranteed or endorsed by the publisher.
Supplementary Material
The Supplementary Material for this article can be found online at: https://www.frontiersin.org/articles/10.3389/fpls.2021.771276/full#supplementary-material
Abbreviations
Values are % change from first to last data point, lowest to highest concentration, or control vs. variables: ABTS, 2,2′-azino-bis (3-ethylbenzothiazoline-6-sulfonic acid); AE, Aqueous Extract; DPPH, 2,2-diphenyl-1-picryl-hydrazyl-hydrate; B, Bound; BCR, Black Colored Rice; EE, Ethanolic Extracts; ENCF, Extruded Nixtamalized Corn Flour; F, Free; FRAP, Ferric reducing antioxidant power; UGAL2, L. lactis ssp. Lactis; UGAL1, W. confusa; 15GAL, L. plantarum; 16GAL, L. brevis; L. plantarum; DI-PROX MTTX, L. brevis; LH-B02, L. helveticus; LAF-4, K. Marxianus subsp. Marxianus; ND, Not Detected; RCR, Red Colored Rice; RH, Relative Humidity; RPM, Revolutions Per Minute; SC, Soluble Conjugate; T, Total; TAC, Total Anthocyanin Content; TFC, Total Flavonoid Content; TPC, Total Phenolic Content; UI, Ultrasonic Intensity; WR, White Rice.
References
Aboud, S. A., Altemimi, A. B., Al-HiIphy, A. R. S., Yi-Chen, L., and Cacciola, F. (2019). A Comprehensive Review on Infrared Heating Applications in Food Processing. Molecules 24:4224125. doi: 10.3390/MOLECULES24224125
Acosta-Estrada, B. A., Gutiérrez-Uribe, J. A., and Serna-Saldívar, S. O. (2014). Bound phenolics in foods, a review. Food Chem. 152, 46–55. doi: 10.1016/J.FOODCHEM.2013.11.093
Adebo, O. A., Njobeh, P. B., Adebiyi, J. A., and Kayitesi, E. (2018). Co-influence of fermentation time and temperature on physicochemical properties, bioactive components and microstructure of ting (a Southern African food) from whole grain sorghum. Food Biosci. 25, 118–127. doi: 10.1016/J.FBIO.2018.08.007
Adebo, O. A., and Gabriela Medina-Meza, I. (2020). Impact of fermentation on the phenolic compounds and antioxidant activity of whole cereal grains: A mini review. Molecules 25:927. doi: 10.3390/molecules25040927
Aguayo-Rojas, J., Mora-Rochín, S., Cuevas-Rodríguez, E. O., Serna-Saldivar, S. O., Gutierrez-Uribe, J. A., Reyes-Moreno, C., et al. (2012). Phytochemicals and Antioxidant Capacity of Tortillas Obtained after Lime-Cooking Extrusion Process of Whole Pigmented Mexican Maize. Plant Foods Hum. Nutr. 2, 178–185. doi: 10.1007/S11130-012-0288-Y
AlAli, M., Alqubaisy, M., Aljaafari, M. N., AlAli, A. O., Baqais, L., Molouki, A., et al. (2021). Nutraceuticals: Transformation of Conventional Foods into Health Promoters/Disease Preventers and Safety Considerations. Molecules 26:26092540. doi: 10.3390/MOLECULES26092540
Amaya Villalva, M. F., González-Aguilar, G., Sández, O. R., García, H. A., Osuna, A. I. L., López-Ahumada, G. A., et al. (2018). Bioprocessing of wheat (Triticum aestivum cv. kronstad) bran from northwest Mexico: Effects on ferulic acid bioaccessibility in breads. CYTA - J. Food 16, 570–579. doi: 10.1080/19476337.2018.1440007
Anuyahong, T., Chusak, C., and Adisakwattana, S. (2020). Incorporation of anthocyanin-rich riceberry rice in yogurts: Effect on physicochemical properties, antioxidant activity and in vitro gastrointestinal digestion. LWT 129:109571. doi: 10.1016/J.LWT.2020.109571
Aprodu, I., Milea, S. A., Anghel, R.-M., Enachi, E., Barbu, V., Crăciunescu, O., et al. (2019). New Functional Ingredients Based on Microencapsulation of Aqueous Anthocyanin-Rich Extracts Derived from Black Rice (Oryza sativa L.). Molecules 24, 3324–3389. doi: 10.3390/MOLECULES24183389
Assadpour, E., and Jafari, S. M. (2019). Advances in Spray-Drying Encapsulation of Food Bioactive Ingredients: From Microcapsules to Nanocapsules. Ann. Rev. Food Sci. Technol. 10, 103–131. doi: 10.1146/ANNUREV-FOOD-032818-121641
Ayyash, M., Johnson, S. K., Liu, S. Q., Al-Mheiri, A., and Abushelaibi, A. (2018). Cytotoxicity, antihypertensive, antidiabetic and antioxidant activities of solid-state fermented lupin, quinoa and wheat by Bifidobacterium species: In-vitro investigations. LWT 95, 295–302. doi: 10.1016/J.LWT.2018.04.099
Bagchi, T. B., Chattopadhyay, K., Sivashankari, M., Roy, S., Kumar, A., Biswas, T., et al. (2021). Effect of different processing technologies on phenolic acids, flavonoids and other antioxidants content in pigmented rice. J. Cereal Sci. 100:103263. doi: 10.1016/J.JCS.2021.103263
Bangar, S. P., Sandhu, K. S., Purewal, S. S., Kaur, M., Kaur, P., Siroha, A. K., et al. (2021). Fermented barley bran: An improvement in phenolic compounds and antioxidant properties. J. Food Proc. Preserv. 2021:e15543. doi: 10.1111/JFPP.15543
Banu, I., Vasilean, I., and Aprodu, I. (2010). Effect of Lactic Fermentation on Antioxidant Capacity of Rye Sourdough and Bread. Food Sci. Technol. Res. 16, 571–576.
Bei, Q., Chen, G., Lu, F., Wu, S., and Wu, Z. (2018). Enzymatic action mechanism of phenolic mobilization in oats (Avena sativa L.) during solid-state fermentation with Monascus anka. Food Chem. 245, 297–304. doi: 10.1016/J.FOODCHEM.2017.10.086
Belén Martín-Diana, A., Tomé-Sánchez, I., Jesús García-Casas, M., Martínez-Villaluenga, C., Frías, J., Rico, D., et al. (2021). A Novel Strategy to Produce a Soluble and Bioactive Wheat Bran Ingredient Rich in Ferulic Acid. Antioxidants 2021:10060969. doi: 10.3390/antiox10060969
Bhat, N. A., Wani, I. A., Hamdani, A. M., and Gani, A. (2019). Effect of extrusion on the physicochemical and antioxidant properties of value added snacks from whole wheat (Triticum aestivum L.) flour. Food Chem. 276, 22–32. doi: 10.1016/J.FOODCHEM.2018.09.170
Blandino, A., Al-Aseeri, M. E., Pandiella, S. S., Cantero, D., and Webb, C. (2003). Cereal-based fermented foods and beverages. Food Res. Internat. 36, 527–543. doi: 10.1016/S0963-9969(03)00009-7
Bolea, C.-A., Cotarlet, M., Enachi, E., Barbu, V., and Stanciuc, N. (2021). Co-Microencapsulated Black Rice Anthocyanins and Lactic Acid Bacteria: Evidence on Powders Profile and In Vitro Digestion. Molecules 26:26092579. doi: 10.3390/MOLECULES26092579
Bonto, A. P., Tiozon, R. N., Sreenivasulu, N., and Camacho, D. H. (2021). Impact of ultrasonic treatment on rice starch and grain functional properties: A review. Ultrason. Sonochem. 71:105383. doi: 10.1016/J.ULTSONCH.2020.105383
Bora, P., Ragaee, S., and Marcone, M. (2019). Effect of parboiling on decortication yield of millet grains and phenolic acids and in vitro digestibility of selected millet products. Food Chemistry 274, 718–725. doi: 10.1016/J.FOODCHEM.2018.09.010
Buitimea-Cantúa, N. E., Torres-Chávez, P. I., Ramírez-Wong, B., Ledesma-Osuna, A. I., Gutiérrez-Uribe, J. A., Serna-Guerrero, D. A., et al. (2018). Ferulic, p-coumaric, diferulic and triferulic acids contents of corn tortillas prepared with extruded corn flour and enriched with sorghum (Sorghum bicolor (L.) Moench) bran. J Food Measure. Charac. 3, 1633–1640. doi: 10.1007/S11694-018-9778-4
Cabrera-Ramírez, A. H., Luzardo-Ocampo, I., Ramírez-Jiménez, A. K., Morales-Sánchez, E., Campos-Vega, R., and Gaytán-Martínez, M. (2020). Effect of the nixtamalization process on the protein bioaccessibility of white and red sorghum flours during in vitro gastrointestinal digestion. Food Res. Internat. 134:109234. doi: 10.1016/J.FOODRES.2020.109234
Cai, H., Zhang, Q., Shen, L., Luo, J., Zhu, R., Mao, J., et al. (2019). Phenolic profile and antioxidant activity of Chinese rice wine fermented with different rice materials and starters. LWT 111, 226–234. doi: 10.1016/J.LWT.2019.05.003
Călinoiu, L. F., and Vodnar, D. C. (2019). Thermal Processing for the Release of Phenolic Compounds from Wheat and Oat Bran. Biomolecules 10:21. doi: 10.3390/biom10010021
Călinoiu, L. F., and Vodnar, D. C. (2020). Thermal Processing for the Release of Phenolic Compounds from Wheat and Oat Bran. Biomolecules 10:10021. doi: 10.3390/BIOM10010021
Călinoiu, L. F., Cătoi, A.-F., and Vodnar, D. C. (2019). Solid-State Yeast Fermented Wheat and Oat Bran as A Route for Delivery of Antioxidants. Antioxidants 8:8090372. doi: 10.3390/ANTIOX8090372
Chandrasekara, A., and Shahidi, F. (2010). Content of Insoluble Bound Phenolics in Millets and Their Contribution to Antioxidant Capacity. J. Agricult. Food Chem. 58, 6706–6714. doi: 10.1021/JF100868B
Chatham, L. A., Howard, J. E., and Juvik, J. A. (2020). A natural colorant system from corn: Flavone-anthocyanin copigmentation for altered hues and improved shelf life. Food Chem. 310:125734. doi: 10.1016/J.FOODCHEM.2019.125734
Chatthongpisut, R., Schwartz, S. J., and Yongsawatdigul, J. (2015). Antioxidant activities and antiproliferative activity of Thai purple rice cooked by various methods on human colon cancer cells. Food Chem. 188, 99–105. doi: 10.1016/j.foodchem.2015.04.074
Chen, C., Wang, L., Wang, R., Luo, X., Li, Y., Li, J., et al. (2018). Ultrasound-assisted extraction from defatted oat (Avena sativa L.) bran to simultaneously enhance phenolic compounds and β-glucan contents: Compositional and kinetic studies. J. Food Eng. 222, 1–10. doi: 10.1016/J.JFOODENG.2017.11.002
Chen, X. Q., Nagao, N., Itani, T., and Irifune, K. (2012). Anti-oxidative analysis, and identification and quantification of anthocyanin pigments in different coloured rice. Food Chem. 135, 2783–2788. doi: 10.1016/J.FOODCHEM.2012.06.098
Chen, Y., Ma, Y., Dong, L., Jia, X., Liu, L., Huang, F., et al. (2019). Extrusion and fungal fermentation change the profile and antioxidant activity of free and bound phenolics in rice bran together with the phenolic bioaccessibility. LWT 115:108461. doi: 10.1016/J.LWT.2019.108461
Chmiel, T., Saputro, I. E., Kusznierewicz, B., and Bartoszek, A. (2018). The impact of cooking method on the phenolic composition, total antioxidant activity and starch digestibility of rice (Oryza sativa L.). J. Food Proc. Preserv. 42:e13383. doi: 10.1111/JFPP.13383
Cho, D. H., Kim, M. J., Sim, E. Y., Jeon, Y. H., Lee, C. K., and Woo, K. S. (2018). Effect of carbohydrase treatments on phenolics content and antioxidant activity of maize flour. Korean J. Food Sci. Tech. 50, 132–137. doi: 10.9721/KJFST.2018.50.2.132
Croitoru, C., Muresan, C., Turturica, M., Stanciuc, N., Andronoiu, D. G., Dumitrascu, L., et al. (2018). Improvement of Quality Properties and Shelf-Life Stability of New Formulated Muffins Based on Black Rice. Molecules 23:23113047. doi: 10.3390/MOLECULES23113047
Čukelj Mustač, N., Novotni, D., Habuš, M., Drakula, S., Nanjara, L., Voučko, B., et al. (2020). Storage stability, micronisation, and application of nutrient-dense fraction of proso millet bran in gluten-free bread. J. Cereal Sci. 91:102864. doi: 10.1016/J.JCS.2019.102864
Das, A. B., Goud, V. V., and Das, C. (2019). Microencapsulation of anthocyanin extract from purple rice bran using modified rice starch and its effect on rice dough rheology. Internat. J. Biolog. Macromole. 124, 573–581. doi: 10.1016/J.IJBIOMAC.2018.11.247
Das, A. K., Bhattacharya, S., and Sing, V. (2017). Bioactives-retained non-glutinous noodles from nixtamalized Dent and Flint maize. Food Chem. 217, 125–132. doi: 10.1016/j.foodchem.2016.08.061
Das, A., Raychaudhuri, U., and Chakraborty, R. (2011). Cereal based functional food of Indian subcontinent: a review. J. Food Sci. Tech. 6, 665–672. doi: 10.1007/S13197-011-0474-1
de la Parra, C., Serna Saldivar, S., and Liu, R. H. (2007). Effect of Processing on the Phytochemical Profiles and Antioxidant Activity of Corn for Production of Masa, Tortillas, and Tortilla Chips. J. Agricult. Food Chem. 55, 4177–4183. doi: 10.1021/JF063487P
dela Rosa, J. G. L., and Medina, P. M. B. (2021). Philippine rice wine (Tapuy) made from Ballatinao black rice and traditional starter culture (Bubod) showed high alcohol content, total phenolic content, and antioxidant activity. Food Sci. Technol. 2021:45120. doi: 10.1590/FST.45120
Derrien, M., and Veiga, P. (2017). Rethinking Diet to Aid Human-Microbe Symbiosis. Trends Microbiol. 25, 100–112. doi: 10.1016/j.tim.2016.09.011
Dordević, T. M., Šiler-Marinković, S. S., and Dimitrijević-Branković, S. I. (2010). Effect of fermentation on antioxidant properties of some cereals and pseudo cereals. Food Chem. 119, 957–963. doi: 10.1016/J.FOODCHEM.2009.07.049
Dykes, L., Seitz, L. M., Rooney, W. L., and Rooney, L. W. (2009). Flavonoid composition of red sorghum genotypes. Food Chem. 116, 313–317. doi: 10.1016/J.FOODCHEM.2009.02.052
Escalante-Aburto, A., Ramírez-Wong, B., Torres-Chávez, P. I., Figueroa-Cárdenas, J. D., López-Cervantes, J., Barrón-Hoyos, J. M., et al. (2013). Effect of extrusion processing parameters on anthocyanin content and physicochemical properties of nixtamalized blue corn expanded extrudates. Cyta 11, 29–37. doi: 10.1080/19476337.2013.764929
Espitia-Hernández, P., Chávez González, M. L., Ascacio-Valdés, J. A., Dávila-Medina, D., Flores-Naveda, A., Silva, T., et al. (2020). Sorghum (Sorghum bicolor L.) as a potential source of bioactive substances and their biological properties. Crit. Rev. Food Sci. Nutr. 2020, 1–12. doi: 10.1080/10408398.2020.1852389
Faridi Esfanjani, A., Assadpour, E., and Jafari, S. M. (2018). Improving the bioavailability of phenolic compounds by loading them within lipid-based nanocarriers. Trends Food Sci. Technol. 76, 56–66. doi: 10.1016/J.TIFS.2018.04.002
Faturachman, F., and Indiarto, R. (2021). Food Infrared Heating Technology: A Review of Its Impacts on Rice Bran Quality. Internat. J. Eng. Trends Technol. 69, 218–224. doi: 10.14445/22315381/IJETT-V69I8P227
Ferri, M., Happel, A., Zanaroli, G., Bertolini, M., Chiesa, S., Commisso, M., et al. (2020). Advances in combined enzymatic extraction of ferulic acid from wheat bran. New Biotechnol. 56, 38–45. doi: 10.1016/J.NBT.2019.10.010
Forster, G. M., Raina, K., Kumar, A., Kumar, S., Agarwal, R., Chen, M. H., et al. (2013). Rice varietal differences in bioactive bran components for inhibition of colorectal cancer cell growth. Food Chem. 141, 1545–1552. doi: 10.1016/j.foodchem.2013.04.020
Francavilla, A., and Joye, I. J. (2020). Anthocyanins in Whole Grain Cereals and Their Potential Effect on Health. Nutr 2020:12. doi: 10.3390/nu12102922
Frias, J., Miranda, M. L., Doblado, R., and Vidal-Valverde, C. (2005). Effect of germination and fermentation on the antioxidant vitamin content and antioxidant capacity of Lupinus albus L. var. Multolupa. Food Chem. 92, 211–220. doi: 10.1016/J.FOODCHEM.2004.06.049
Gabaza, M., Shumoy, H., Muchuweti, M., Vandamme, P., and Raes, K. (2016). Effect of Fermentation and Cooking on Soluble and Bound Phenolic Profiles of Finger Millet Sour Porridge. J. Agricult. Food Chem. 64, 7615–7621. doi: 10.1021/ACS.JAFC.6B03090
Gaxiola-Cuevas, N., Mora-Rochín, S., Cuevas-Rodriguez, E. O., León-López, L., Reyes-Moreno, C., Montoya-Rodríguez, A., et al. (2017). Phenolic Acids Profiles and Cellular Antioxidant Activity in Tortillas Produced from Mexican Maize Landrace Processed by Nixtamalization and Lime Extrusion Cooking. Plant Foods Hum. Nutr. 3, 314–320. doi: 10.1007/S11130-017-0624-3
Gaytán-Martínez, M., Cabrera-Ramírez, ÁH., Morales-Sánchez, E., Ramírez-Jiménez, A. K., Cruz-Ramírez, J., Campos-Vega, R., et al. (2017). Effect of nixtamalization process on the content and composition of phenolic compounds and antioxidant activity of two sorghums varieties. J. Cereal Sci. 77, 1–8. doi: 10.1016/J.JCS.2017.06.014
Ge, X., Saleh, A. S. M., Jing, L., Zhao, K., Su, C., Zhang, B., et al. (2021). Germination and drying induced changes in the composition and content of phenolic compounds in naked barley. J. Food Compos. Anal. 95:103594. doi: 10.1016/J.JFCA.2020.103594
Ge, Y., Li, Y., Bai, Y., Yuan, C., Wu, C., and Hu, Y. (2020). Intelligent gelatin/oxidized chitin nanocrystals nanocomposite films containing black rice bran anthocyanins for fish freshness monitorings. Internat. J. Biol. Macromole. 155, 1296–1306. doi: 10.1016/J.IJBIOMAC.2019.11.101
Ghasemzadeh, A., Karbalaii, M. T., Jaafar, H. Z. E., and Rahmat, A. (2018). Phytochemical constituents, antioxidant activity, and antiproliferative properties of black, red, and brown rice bran. Chem. Cent. J. 12, 17. doi: 10.1186/s13065-018-0382-9
Giambanelli, E., Ferioli, F., and D’Antuono, L. F. (2016). Assessing the effect of traditional hulled wheat processing on bioactive compounds retention. J. Cereal Sci. 72, 60–68. doi: 10.1016/J.JCS.2016.10.003
Gong, L., Huang, L., and Zhang, Y. (2012). Effect of Steam Explosion Treatment on Barley Bran Phenolic Compounds and Antioxidant Capacity. J. Agricult. Food Chem. 60, 7177–7184. doi: 10.1021/JF301599A
Granata, G., Consoli, G. M. L., Lo Nigro, R., and Geraci, C. (2018). Hydroxycinnamic acids loaded in lipid-core nanocapsules. Food Chem. 245, 551–556. doi: 10.1016/J.FOODCHEM.2017.10.106
Guo, J., Giusti, M. M., and Kaletunç, G. (2018). Encapsulation of purple corn and blueberry extracts in alginate-pectin hydrogel particles: Impact of processing and storage parameters on encapsulation efficiency. Food Res. Internat. 107, 414–422. doi: 10.1016/J.FOODRES.2018.02.035
Hassan, S., Imran, M., Ahmad, M. H., Muhammad, I., Khan, C., Xu, M., et al. (2020). Phytochemical characterization of ultrasound-processed sorghum sprouts for the use in functional foods. Internat. J. Food Propert. 23, 853–863. doi: 10.1080/10942912.2020.1762644
He, F., Wang, T., Zhu, S., and Chen, G. (2016). Modeling the effects of microfluidization conditions on properties of corn bran. J. Cereal Sci. 71, 86–92. doi: 10.1016/J.JCS.2016.08.002
Henderson, A. J., Ollila, C. A., Kumar, A., Borresen, E. C., Raina, K., Agarwal, R., et al. (2012). Chemopreventive properties of dietary rice bran: current status and future prospects. Adv. Nutr. 3, 643–653. doi: 10.3945/an.112.002303
Hithamani, G., and Srinivasan, K. (2014). Bioaccessibility of Polyphenols from Wheat (Triticum aestivum), Sorghum (Sorghum bicolor), Green Gram (Vigna radiata), and Chickpea (Cicer arietinum) as Influenced by Domestic Food Processing. J. Agricult. Food Chem. 62, 11170–11179. doi: 10.1021/JF503450U
Hole, A. S., Kjos, N. P., Grimmer, S., Kohler, A., Lea, P., Rasmussen, B., et al. (2013). Extrusion of Barley and Oat Improves the Bioaccessibility of Dietary Phenolic Acids in Growing Pigs. J.Agricult. Food Chem. 61, 2739–2747. doi: 10.1021/JF3045236
Hole, A. S., Rud, I., Grimmer, S., Sigl, S., Narvhus, J., and Sahlstrøm, S. (2012). Improved Bioavailability of Dietary Phenolic Acids in Whole Grain Barley and Oat Groat following Fermentation with Probiotic Lactobacillus acidophilus, Lactobacillus johnsonii, and Lactobacillus reuteri. J. Agricult. Food Chem. 60, 6369–6375. doi: 10.1021/JF300410H
Hou, F., Su, D., Xu, J., Gong, Y., Zhang, R., Wei, Z., et al. (2016). Enhanced Extraction of Phenolics and Antioxidant Capacity from Sorghum (Sorghum bicolor L. Moench) Shell Using Ultrasonic-Assisted Ethanol–Water Binary Solvent. J. Food Proc. Preserv. 40, 1171–1179. doi: 10.1111/JFPP.12699
Hu, Z., Tang, X., Liu, J., Zhu, Z., and Shao, Y. (2017). Effect of parboiling on phytochemical content, antioxidant activity and physicochemical properties of germinated red rice. Food Chem. 214, 285–292. doi: 10.1016/J.FOODCHEM.2016.07.097
Iftikhar, M., Zhang, H., Iftikhar, A., Raza, A., Begum, N., Tahamina, A., et al. (2020). Study on optimization of ultrasonic assisted extraction of phenolic compounds from rye bran. LWT 134:110243. doi: 10.1016/J.LWT.2020.110243
Irakli, M., Katsantonis, D., and Kleisiaris, F. (2015). Evaluation of quality attributes, nutraceutical components and antioxidant potential of wheat bread substituted with rice bran. J. Cereal Sci. 65, 74–80. doi: 10.1016/J.JCS.2015.06.010
Irakli, M., Kleisiaris, F., Mygdalia, A., and Katsantonis, D. (2018). Stabilization of rice bran and its effect on bioactive compounds content, antioxidant activity and storage stability during infrared radiation heating. J. Cereal Sci. 80, 135–142. doi: 10.1016/J.JCS.2018.02.005
Irakli, M., Lazaridou, A., and Biliaderis, C. G. (2020). Comparative Evaluation of the Nutritional, Antinutritional, Functional, and Bioactivity Attributes of Rice Bran Stabilized by Different Heat Treatments. Foods 2020:10010057. doi: 10.3390/foods10010057
Itagi, H. N., Rao, B. V. R. S., Jayadeep, P. A., and Singh, V. (2012). Functional and antioxidant properties of ready-to-eat flakes from various cereals including sorghum and millets. Q. Assur. Safety Crops Foods 4, 126–133. doi: 10.1111/J.1757-837X.2012.00136.X
Izadifar, Z. (2013). Ultrasound pretreatment of wheat dried distiller’s grain (DDG) for extraction of phenolic compounds. Ultrason. Sonochem. 20, 1359–1369. doi: 10.1016/J.ULTSONCH.2013.04.004
Jiang, L., Su, W., Mu, Y., and Mu, Y. (2020). Major Metabolites and Microbial Community of Fermented Black Glutinous Rice Wine With Different Starters. Front. Microbiol. 11:593. doi: 10.3389/FMICB.2020.00593
Jones, D., Caballero, S., and Davidov-Pardo, G. (2019). Bioavailability of nanotechnology-based bioactives and nutraceuticals. Adv. Food Nutr. Res. 2019, 235–273. doi: 10.1016/bs.afnr.2019.02.014
Jung, Y. S., Kim, D. H., Hwang, J. Y., Yun, N. Y., Lee, Y. H., Han, S. B., et al. (2014). Anti-inflammatory effect of tricin 4′-O-(threo-β-guaiacylglyceryl) ether, a novel flavonolignan compound isolated from Njavara on in RAW264.7 cells and in ear mice edema. Toxicol. Appl. Pharm. 277, 67–76. doi: 10.1016/J.TAAP.2014.03.001
Kadiri, O. (2017). A review on the status of the phenolic compounds and antioxidant capacity of the flour: Effects of cereal processing. Internat. J. Food Prop. 2017:1315130. doi: 10.1080/10942912.2017.1315130
Kamau, E. H., Nkhata, S. G., and Ayua, E. O. (2020). Extrusion and nixtamalization conditions influence the magnitude of change in the nutrients and bioactive components of cereals and legumes. Food Sci. Nutrit. 8, 1753–1765. doi: 10.1002/FSN3.1473
Kasote, D. M., Jayaprakasha, G. K., and Patil, B. S. (2018). Encapsulation of polyphenols: An effective way to enhance their bioavailability for gut health. ACS Symp. Series 1286, 239–259. doi: 10.1021/BK-2018-1286.CH0013
Kataria, A., Sharma, S., and Dar, B. N. (2021). Changes in phenolic compounds, antioxidant potential and antinutritional factors of Teff (Eragrostis tef) during different thermal processing methods. Internat. J. Food Sci. Technol. 2021:15210. doi: 10.1111/IJFS.15210
Katina, K., Liukkonen, K. H., Kaukovirta-Norja, A., Adlercreutz, H., Heinonen, S. M., Lampi, A. M., et al. (2007). Fermentation-induced changes in the nutritional value of native or germinated rye. J. Cereal Sci. 46, 348–355. doi: 10.1016/J.JCS.2007.07.006
Khan, I., Yousif, A., Johnson, S. K., and Gamlath, S. (2013). Effect of sorghum flour addition on resistant starch content, phenolic profile and antioxidant capacity of durum wheat pasta. Food Res. Internat. 54, 578–586. doi: 10.1016/J.FOODRES.2013.07.059
Khan, S. A., Zhang, M., Liu, L., Dong, L., Ma, Y., Wei, Z., et al. (2020). Co-culture submerged fermentation by lactobacillus and yeast more effectively improved the profiles and bioaccessibility of phenolics in extruded brown rice than single-culture fermentation. Food Chem. 326:126985. doi: 10.1016/J.FOODCHEM.2020.126985
Kim, K. H., Tsao, R., Yang, R., and Cui, S. W. (2006). Phenolic acid profiles and antioxidant activities of wheat bran extracts and the effect of hydrolysis conditions. Food Chem. 95, 466–473. doi: 10.1016/J.FOODCHEM.2005.01.032
Kim, M. J., Kwak, H. S., and Kim, S. S. (2018). Effects of Germination on Protein, γ-Aminobutyric Acid, Phenolic Acids, and Antioxidant Capacity in Wheat. Molecules 2244, 2244. doi: 10.3390/MOLECULES23092244
Klunklin, W., and Savage, G. (2018). Physicochemical Properties and Sensory Evaluation of Wheat-Purple Rice Biscuits Enriched with Green-Lipped Mussel Powder (Perna canaliculus) and Spices. J. Food Q. 2018:769703. doi: 10.1155/2018/7697903
Koch, K., Havermann, S., Büchter, C., and Wätjen, W. (2014). Caenorhabditis elegans as model system in pharmacology and toxicology: effects of flavonoids on redox-sensitive signalling pathways and ageing. ScientificWorldJournal 2014:920398. doi: 10.1155/2014/920398
Koletta, P., Irakli, M., Papageorgiou, M., and Skendi, A. (2014). Physicochemical and technological properties of highly enriched wheat breads with wholegrain non wheat flours. J. Cereal Sci. 60, 561–568. doi: 10.1016/J.JCS.2014.08.003
Kongthitilerd, P., Suantawee, T., Cheng, H., Thilavech, T., Marnpae, M., and Adisakwattana, S. (2020). Anthocyanin-Enriched Riceberry Rice Extract Inhibits Cell Proliferation and Adipogenesis in 3T3-L1 Preadipocytes by Downregulating Adipogenic Transcription Factors and Their Targeting Genes. Nutrients 12, 1–18. doi: 10.3390/NU12082480
Kruma, Z., Tomsone, L., Ķince, T., Galoburda, R., Senhofa, S., Sabovics, M., et al. (2016). Effects of germination on total phenolic compounds and radical scavenging activity in hull-less spring cereals and triticale. Agronomy Res. 14, 1372–1383.
Kubatka, P., Kello, M., Kajo, K., Kruzliak, P., Výbohová, D., Šmejkal, K., et al. (2016). Young Barley Indicates Antitumor Effects in Experimental Breast Cancer In Vivo and In Vitro. Nutr. Cancer 68, 611–621. doi: 10.1080/01635581.2016.1154577
Lachman, S., Boekholdt, S. M., Luben, R. N., Sharp, S. J., Brage, S., Khaw, K.-T., et al. (2018). Impact of physical activity on the risk of cardiovascular disease in middle-aged and older adults: EPIC Norfolk prospective population study. Eur. J. Prev. Cardiol. 25:200. doi: 10.1177/2047487317737628
Lainumngen, N., Saengprakai, J., Tanjor, S., Phanpho, W., and Phodsoongnoen, A. (2020). Suan Sunandha Science and Technology Journal Development of High Anthocyanin Crispy Rice Bar. Suan Sunandha Rajabhat Univ. 7, 34–42. doi: 10.14456/ssstj.2020.6
Laokuldilok, T., and Kanha, N. (2017). Microencapsulation of Black Glutinous Rice Anthocyanins Using Maltodextrins Produced from Broken Rice Fraction as Wall Material by Spray Drying and Freeze Drying. J. Food Proc. Preserv. 41:e12877. doi: 10.1111/JFPP.12877
Lee, S., Choi, Y., Sung, J., Kim, Y., Jeong, H., and Lee, J. (2014). Protective Effects of Black Rice Extracts on Oxidative Stress Induced by tert-Butyl Hydroperoxide in HepG2 Cells. Prevent. Nutr. Food Sci. 19, 348–352. doi: 10.3746/PNF.2014.19.4.348
Lima, F. A., de, Martins, I. M., Faria, A., Calhau, C., Azevedo, J., et al. (2018). Influence of rye flour enzymatic biotransformation on the antioxidant capacity and transepithelial transport of phenolic acids. Food Funct. 9, 1889–1898. doi: 10.1039/C7FO01645J
Liu, L., Wen, W., Zhang, R., Wei, Z., Deng, Y., Xiao, J., et al. (2017). Complex enzyme hydrolysis releases antioxidative phenolics from rice bran. Food Chem. 214, 1–8. doi: 10.1016/J.FOODCHEM.2016.07.038
Lohani, U. C., and Muthukumarappan, K. (2016). Application of the pulsed electric field to release bound phenolics in sorghum flour and apple pomace. Innov. Food Sci. Emerg. Tech. 35, 29–35. doi: 10.1016/J.IFSET.2016.03.012
Loskutov, I. G., and Khlestkina, E. K. (2021). Wheat, Barley, and Oat Breeding for Health Benefit Components in Grain. Plants 86:86. doi: 10.3390/PLANTS10010086
Lu, W., Kelly, A. L., and Miao, S. (2016). Emulsion-based encapsulation and delivery systems for polyphenols. Trends Food Sci. Technol. 47, 1–9. doi: 10.1016/J.TIFS.2015.10.015
Luti, S., Galli, V., Venturi, M., Granchi, L., Paoli, P., and Pazzagli, L. (2021). Bioactive Properties of Breads Made with Sourdough of Hull-Less Barley or Conventional and Pigmented Wheat Flours. Appl. Sci. 3291:3291. doi: 10.3390/APP11073291
Luzardo-Ocampo, I., Ramírez-Jiménez, A. K., Cabrera-Ramírez, A. H., Rodríguez-Castillo, N., Campos-Vega, R., Loarca-Piña, G., et al. (2020). Impact of cooking and nixtamalization on the bioaccessibility and antioxidant capacity of phenolic compounds from two sorghum varieties. Food Chem. 309:125684. doi: 10.1016/J.FOODCHEM.2019.125684
Majid, I., Nayik, G. A., and Nanda, V. (2015). Ultrasonication and food technology: A review. Cogent Food Agricult. 1:1071022. doi: 10.1080/23311932.2015.1071022
Martini, D., Ciccoritti, R., Nicoletti, I., Nocente, F., Corradini, D., D’Egidio, M. G., et al. (2017). From seed to cooked pasta: influence of traditional and non-conventional transformation processes on total antioxidant capacity and phenolic acid content. Internat. J. Food Sci. Nutr. 69, 24–32. doi: 10.1080/09637486.2017.1336751
Mau, J. L., Lee, C. C., Chen, Y. P., and Lin, S. D. (2017). Physicochemical, antioxidant and sensory characteristics of chiffon cake prepared with black rice as replacement for wheat flour. LWT 75, 434–439. doi: 10.1016/J.LWT.2016.09.019
Mbanjo, E. G. N., Kretzschmar, T., Jones, H., Ereful, N., Blanchard, C., Boyd, L. A., et al. (2020). The Genetic Basis and Nutritional Benefits of Pigmented Rice Grain. Front. Genet. 0:229. doi: 10.3389/FGENE.2020.00229
Méndez-Lagunas, L. L., Cruz-Gracida, M., Barriada-Bernal, L. G., and Rodríguez-Méndez, L. I. (2020). Profile of phenolic acids, antioxidant activity and total phenolic compounds during blue corn tortilla processing and its bioaccessibility. J. Food Sci. Technol. 12, 4688–4696. doi: 10.1007/S13197-020-04505-3
Mert, I. D. (2020). The applications of microfluidization in cereals and cereal-based products: An overview. Crit. Rev. Food Sci. Nutr. 60, 1007–1024. doi: 10.1080/10408398.2018.1555134
Miano, A. C., Pereira, J., da, C., Castanha, N., Júnior, M. D., da, M., et al. (2016). Enhancing mung bean hydration using the ultrasound technology: description of mechanisms and impact on its germination and main components. Sci. Rep. 1, 1–14. doi: 10.1038/srep38996
Mnich, E., Bjarnholt, N., Eudes, A., Harholt, J., Holland, C., Jørgensen, B., et al. (2020). Phenolic cross-links: building and de-constructing the plant cell wall. Nat. Prod. Rep. 37, 919–961. doi: 10.1039/C9NP00028C
Mu, Y., Su, W., Yu, X.-T., Mu, Y.-C., Jiang, L., and Wang, H.-L. (2019). Untargeted metabolomics based on GC-TOF-MS reveals the optimal pre-fermentation time for black glutinous rice wine. Internat. J. Food Prop. 22, 2033–2046. doi: 10.1080/10942912.2019.1705481
Muangrat, R., Williams, P. T., and Saengcharoenrat, P. (2017). Subcritical solvent extraction of total anthocyanins from dried purple waxy corn: Influence of process conditions. J. Food Proc. Preserv. 41:13252. doi: 10.1111/JFPP.13252
N’Dri, D., Mazzeo, T., Zaupa, M., Ferracane, R., Fogliano, V., and Pellegrini, N. (2013). Effect of cooking on the total antioxidant capacity and phenolic profile of some whole-meal African cereals. J. Sci. Food Agricult. 93, 29–36. doi: 10.1002/JSFA.5837
Niu, M., and Hou, G. G. (2020). Whole grain noodles. Asian Noodle Manufact. 2020, 95–123. doi: 10.1016/B978-0-12-812873-2.00006-6
Norkaew, O., Thitisut, P., Mahatheeranont, S., Pawin, B., Sookwong, P., Yodpitak, S., et al. (2019). Effect of wall materials on some physicochemical properties and release characteristics of encapsulated black rice anthocyanin microcapsules. Food Chem. 294, 493–502. doi: 10.1016/J.FOODCHEM.2019.05.086
Oghbaei, M., and Prakash, J. (2016). Effect of primary processing of cereals and legumes on its nutritional quality: A comprehensive review. Cogent Food Agricult. 2:1136015. doi: 10.1080/23311932.2015.1136015
Ohara, K., Uchida, A., Nagasaka, R., Ushio, H., and Ohshima, T. (2009). The effects of hydroxycinnamic acid derivatives on adiponectin secretion. Phytomedicine 16, 130–137. doi: 10.1016/J.PHYMED.2008.09.012
Omwamba, M., Li, F., Sun, G., Hu, Q., Omwamba, M., Li, F., et al. (2013). Antioxidant Effect of Roasted Barley (Hordeum vulgare L.) Grain Extract towards Oxidative Stress in Vitro and in Vivo. Food Nutr. Sci. 4, 139–146. doi: 10.4236/FNS.2013.48A017
Ortiz-Cruz, R. A., Ramírez-Wong, B., Ledesma-Osuna, A. I., Torres-Chávez, P. I., Sánchez-Machado, D. I., Montaño-Leyva, B., et al. (2020). Effect of Extrusion Processing Conditions on the Phenolic Compound Content and Antioxidant Capacity of Sorghum (Sorghum bicolor (L.) Moench) Bran. Plant Foods Hum. Nutr. 2, 252–257. doi: 10.1007/S11130-020-00810-6
Ozkan, G., Kostka, T., Esatbeyoglu, T., and Capanoglu, E. (2020). Effects of Lipid-Based Encapsulation on the Bioaccessibility and Bioavailability of Phenolic Compounds. Molecules 25:25235545. doi: 10.3390/MOLECULES25235545
Özkaya, H., Özkaya, B., Duman, B., and Turksoy, S. (2017). Effect of Dephytinization by Fermentation and Hydrothermal Autoclaving Treatments on the Antioxidant Activity, Dietary Fiber, and Phenolic Content of Oat Bran. J. Agricult. Food Chem. 65, 5713–5719. doi: 10.1021/ACS.JAFC.7B01698
Paiva, F. F., Vanier, N. L., Berrios, J. D. J., Pinto, V. Z., Wood, D., Williams, T., et al. (2016). Polishing and parboiling effect on the nutritional and technological properties of pigmented rice. Food Chem. 191, 105–112. doi: 10.1016/J.FOODCHEM.2015.02.047
Pal, P., Singh, N., Kaur, P., and Kaur, A. (2018). Effect of Parboiling on Phenolic, Protein, and Pasting Properties of Rice from Different Paddy Varieties. J. Food Sci. 83, 2761–2771. doi: 10.1111/1750-3841.14347
Pal, S., Bagchi, T. B., Dhali, K., Kar, A., Sanghamitra, P., Sarkar, S., et al. (2019). Evaluation of sensory, physicochemical properties and Consumer preference of black rice and their products. J. Food Sci. Technol. 56:1484. doi: 10.1007/S13197-019-03634-8
Panwar, R., Raghuwanshi, N., Srivastava, A. K., Sharma, A. K., and Pruthi, V. (2018). In-vivo sustained release of nanoencapsulated ferulic acid and its impact in induced diabetes. Mater. Sci. Eng. 92, 381–392. doi: 10.1016/J.MSEC.2018.06.055
Papillo, V. A., Locatelli, M., Travaglia, F., Bordiga, M., Garino, C., Arlorio, M., et al. (2018). Spray-dried polyphenolic extract from Italian black rice (Oryza sativa L., var. Artemide) as new ingredient for bakery products. Food Chem. 269, 603–609. doi: 10.1016/J.FOODCHEM.2018.07.059
Pekkinen, J., Rosa, N. N., Savolainen, O.-I., Keski-Rahkonen, P., Mykkänen, H., Poutanen, K., et al. (2014). Disintegration of wheat aleurone structure has an impact on the bioavailability of phenolic compounds and other phytochemicals as evidenced by altered urinary metabolite profile of diet-induced obese mice. Nutrit. Metabol. 11:1. doi: 10.1186/1743-7075-11-1
Pérez-Andrés, J. M., Charoux, C. M. G., Cullen, P. J., and Tiwari, B. K. (2018). Chemical Modifications of Lipids and Proteins by Nonthermal Food Processing Technologies. J. Agricult. Food Chem. 66, 5041–5054. doi: 10.1021/ACS.JAFC.7B06055
Prabha, K., Ghosh, P. S. A., Joseph, R. M., Krishnan, R., Rana, S. S., et al. (2021). Recent development, challenges, and prospects of extrusion technology. Future Foods 3:100019. doi: 10.1016/J.FUFO.2021.100019
Prabhu, A. A., and Jayadeep, A. (2015). Enzymatic processing of pigmented and non pigmented rice bran on changes in oryzanol, polyphenols and antioxidant activity. J. Food Sci. Technol 10, 6538–6546. doi: 10.1007/S13197-015-1761-Z
Qin, Y., Liu, Y., Yuan, L., Yong, H., and Liu, J. (2019). Preparation and characterization of antioxidant, antimicrobial and pH-sensitive films based on chitosan, silver nanoparticles and purple corn extract. Food Hydrocolloids 96, 102–111. doi: 10.1016/J.FOODHYD.2019.05.017
Quagliariello, V., Iaffaioli, R. V., Falcone, M., Ferrari, G., Pataro, G., and Donsì, F. (2016). Effect of pulsed electric fields – assisted extraction on anti-inflammatory and cytotoxic activity of brown rice bioactive compounds. Food Res. Internat. 87, 115–124. doi: 10.1016/J.FOODRES.2016.07.005
Ramos-Enríquez, J. R., Ramírez-Wong, B., Robles-Sánchez, R. M., Robles-Zepeda, R. E., González-Aguilar, G. A., and Gutiérrez-Dorado, R. (2018). Effect of Extrusion Conditions and the Optimization of Phenolic Compound Content and Antioxidant Activity of Wheat Bran Using Response Surface Methodology. Plant Foods Hum. Nutr. 3, 228–234. doi: 10.1007/S11130-018-0679-9
Rao, A. S., Reddy, S. G., Babu, P. P., and Reddy, A. R. (2010). The antioxidant and antiproliferative activities of methanolic extracts from Njavara rice bran. BMC Complement Alternat. Med. 1, 1–9. doi: 10.1186/1472-6882-10-4
Raso, J., Condón, S., and álvarez, I. (2014). Non-thermal Processing:| Pulsed Electric Field. Encyclop. Food Microbiol. 2014, 966–973. doi: 10.1016/B978-0-12-384730-0.00397-9
Revilla, E., Santa-María, C., Miramontes, E., Candiracci, M., Rodríguez-Morgado, B., Carballo, M., et al. (2013). Antiproliferative and immunoactivatory ability of an enzymatic extract from rice bran. Food Chem. 136, 526–531. doi: 10.1016/j.foodchem.2012.08.044
Reynoso-Camacho, R., Guerrero-Villanueva, G., de Dios Figueroa, J., Gallegos-Corona, M. A., Mendoza, S., Loarca-Piña, G., et al. (2015). Anticarcinogenic Effect of Corn Tortilla Against 1,2-Dimethylhydrazine (DMH)-Induced Colon Carcinogenesis in Sprague–Dawley Rats. Plant Plant Foods Hum. Nutr. 70, 146–152. doi: 10.1007/s11130-015-0471-z
Ribas-Agustí, A., Martín-Belloso, O., Soliva-Fortuny, R., and Elez-Martínez, P. (2017). Food processing strategies to enhance phenolic compounds bioaccessibility and bioavailability in plant-based foods. Crit. Rev. Food Sci. Nutr. 58, 2531–2548. doi: 10.1080/10408398.2017.1331200
Rocha-Villarreal, V., Serna-Saldivar, S. O., and García-Lara, S. (2018). Effects of parboiling and other hydrothermal treatments on the physical, functional, and nutritional properties of rice and other cereals. Cereal Chem. 95, 79–91. doi: 10.1002/CCHE.10010
Rodriguez, E. B., Almeda, R. A., Vidallon, M. L. P., and Reyes, C. T. (2019). Enhanced bioactivity and efficient delivery of quercetin through nanoliposomal encapsulation using rice bran phospholipids. J. Sci. Food Agricult. 99, 1980–1989. doi: 10.1002/JSFA.9396
Saharan, P., Sadh, P. K., and Duhan, J. S. (2017). Comparative assessment of effect of fermentation on phenolics, flavanoids and free radical scavenging activity of commonly used cereals. Biocatal. Agricult. Biotechnol. 201712, 236–240. doi: 10.1016/j.bcab.2017.10.013
Salar, R. K., Certik, M., and Brezova, V. (2012). Modulation of phenolic content and antioxidant activity of maize by solid state fermentation with Thamnidium elegans CCF 1456. Biotechnol. Bioproc. Eng. 1, 109–116. doi: 10.1007/S12257-011-0455-2
Salar, R. K., Purewal, S. S., and Bhatti, M. S. (2016). Optimization of extraction conditions and enhancement of phenolic content and antioxidant activity of pearl millet fermented with Aspergillus awamori MTCC-548. Resource-Efficient Tech. 2, 148–157. doi: 10.1016/J.REFFIT.2016.08.002
Salazar-López, N. J., González-Aguilar, G., Rouzaud-Sández, O., and Robles-Sánchez, M. (2018). Technologies applied to sorghum (Sorghum bicolor L. Moench): changes in phenolic compounds and antioxidant capacity. Food Sci. Technol. 38, 369–382. doi: 10.1590/FST.16017
Sandhu, K. S., Punia, S., and Kaur, M. (2016). Effect of duration of solid state fermentation by Aspergillus awamorinakazawa on antioxidant properties of wheat cultivars. LWT - Food Sci. Technol. 71, 323–328. doi: 10.1016/J.LWT.2016.04.008
Santos, J. P., dos, A., dos, T., Prestes, D. N., Rombaldi, C. V., Halal, S. L. M., et al. (2020). From brown, red, and black rice to beer: Changes in phenolics, γ-aminobutyric acid, and physicochemical attributes. Cereal Chem. 97, 1148–1157. doi: 10.1002/CCHE.10335
Sapna, I., and Jayadeep, A. (2020). Application of pulverization and thermal treatment to pigmented broken rice: insight into flour physical, functional and product forming properties. J. Food Sci. Tech. 6, 2089–2097. doi: 10.1007/S13197-020-04718-6
Schaarschmidt, S., and Fauhl-Hassek, C. (2019). Mycotoxins during the Processes of Nixtamalization and Tortilla Production. Toxins 11:11040227. doi: 10.3390/TOXINS11040227
Serna-Saldivar, S. O., and Rooney, L. W. (2015). Industrial Production of Maize Tortillas and Snacks. Tortillas 2015, 247–281. doi: 10.1016/B978-1-891127-88-5.50013-X
Sethi, S., Nanda, S. K., and Bala, M. (2020). Quality assessment of pasta enriched with anthocyanin-rich black rice bran. J. Food Proc. Preserv. 44:e14952. doi: 10.1111/JFPP.14952
Setyaningsih, W., Saputro, I. E., Carrera, C. A., and Palma, M. (2019). Optimisation of an ultrasound-assisted extraction method for the simultaneous determination of phenolics in rice grains. Food Chem. 288, 221–227. doi: 10.1016/J.FOODCHEM.2019.02.107
Shan, S., Lu, Y., Zhang, X., Shi, J., Li, H., and Li, Z. (2020). Inhibitory effect of bound polyphenol from foxtail millet bran on miR-149 methylation increases the chemosensitivity of human colorectal cancer HCT-8/Fu cells. Mole. Cell. Biochem. 2, 513–523. doi: 10.1007/S11010-020-03906-4
Shao, Y., Xu, F., Sun, X., Bao, J., and Beta, T. (2014). Identification and quantification of phenolic acids and anthocyanins as antioxidants in bran, embryo and endosperm of white, red and black rice kernels (Oryza sativa L.). J. Cereal Sci. 59, 211–218. doi: 10.1016/j.jcs.2014.01.004
Sharma, P., and Gujral, H. S. (2011). Effect of sand roasting and microwave cooking on antioxidant activity of barley. Food Res. Internat. 44, 235–240. doi: 10.1016/J.FOODRES.2010.10.030
Sharma, P., and Gujral, H. S. (2014). Cookie making behavior of wheat–barley flour blends and effects on antioxidant properties. LWT - Food Sci. Technol. 55, 301–307. doi: 10.1016/j.lwt.2013.08.019
Sharma, S., Dar, N., Nayik, G., and Kaur, G. (2016). Total Phenolic Content and Antioxidant Activity of Cereal Bran Enriched Ready to Eat Breakfast Cereal Porridge. Curr. Nutr. Food Sci. 12, 142–149. doi: 10.2174/1573401312666160323000523
Skrajda-Brdak, M., Konopka, I., Tańska, M., and Czaplicki, S. (2019). Changes in the content of free phenolic acids and antioxidative capacity of wholemeal bread in relation to cereal species and fermentation type. Eur. Food Res. Technol. 10, 2247–2256. doi: 10.1007/S00217-019-03331-Y
Son, M. J., Rico, C. W., Nam, S. H., and Kang, M. Y. (2011). Effect of Oryzanol and Ferulic Acid on the Glucose Metabolism of Mice Fed with a High-Fat Diet. J. Food Sci. 76, H7–H10. doi: 10.1111/J.1750-3841.2010.01907.X
Sunico, D. J. A., Rodriguez, F. M., Tuaño, A. P. P., Mopera, L. E., Atienza, L. M., and Juanico, C. B. (2021). Physicochemical and Nutritional Properties of Nixtamalized Quality Protein Maize Flour and its Potential as Substitute in Philippine Salt Bread. Chiang Mai Univ. J. Nat. Sci. 20, 1–15. doi: 10.12982/CMUJNS.2021.035
Suriano, S., Balconi, C., Valoti, P., and Redaelli, R. (2021). Comparison of total polyphenols, profile anthocyanins, color analysis, carotenoids and tocols in pigmented maize. LWT 144, 111257. doi: 10.1016/J.LWT.2021.111257
Suriano, S., Savino, M., Codianni, P., Iannucci, A., Caternolo, G., Russo, M., et al. (2019). Anthocyanin profile and antioxidant capacity in coloured barley. Int. J. Food Sci. Technol. 54, 2478–2486. doi: 10.1111/ijfs.14203
Takikawa, M., Inoue, S., Horio, F., and Tsuda, T. (2010). Dietary Anthocyanin-Rich Bilberry Extract Ameliorates Hyperglycemia and Insulin Sensitivity via Activation of AMP-Activated Protein Kinase in Diabetic Mice. J. Nutr. 140, 527–533. doi: 10.3945/JN.109.118216
Thiranusornkij, L., Thamnarathip, P., Chandrachai, A., Kuakpetoon, D., and Adisakwattana, S. (2019). Comparative studies on physicochemical properties, starch hydrolysis, predicted glycemic index of Hom Mali rice and Riceberry rice flour and their applications in bread. Food Chem. 283, 224–231. doi: 10.1016/J.FOODCHEM.2019.01.048
Ti, H., Zhang, R., Zhang, M., Li, Q., Wei, Z., Zhang, Y., et al. (2014). Dynamic changes in the free and bound phenolic compounds and antioxidant activity of brown rice at different germination stages. Food Chem. 161, 337–344. doi: 10.1016/J.FOODCHEM.2014.04.024
Tiozon, R. N. Jr., Bonto, A. P., and Sreenivasulu, N. (2021). Enhancing the functional properties of rice starch through biopolymer blending for industrial applications: A review. Internat. J. Biol. Macromole. 192, 100–117. doi: 10.1016/J.IJBIOMAC.2021.09.194
Tomé-Sánchez, I., Martín-Diana, A. B., Peñas, E., Bautista-Expósito, S., Frias, J., Rico, D., et al. (2020). Soluble Phenolic Composition Tailored by Germination Conditions Accompany Antioxidant and Anti-Inflammatory Properties of Wheat. Antioxidants 9:9050426. doi: 10.3390/ANTIOX9050426
Upanan, S., Yodkeeree, S., Thippraphan, P., Punfa, W., Wongpoomchai, R., and Limtrakul, P. (2019). The Proanthocyanidin-Rich Fraction Obtained from Red Rice Germ and Bran Extract Induces HepG2 Hepatocellular Carcinoma Cell Apoptosis. Molecules 24:24040813. doi: 10.3390/molecules24040813
Vargas-Yana, D., Aguilar-Morón, B., Pezo-Torres, N., Shetty, K., and Ranilla, L. G. (2020). Ancestral Peruvian ethnic fermented beverage “Chicha” based on purple corn (Zea mays L.): unraveling the health-relevant functional benefits. J. Ethn. Foods 1, 1–12. doi: 10.1186/S42779-020-00063-3
Vitalini, S., Sardella, A., Fracassetti, D., Secli, R., Tirelli, A., Lodi, G., et al. (2020). Polyphenol Bioavailability and Plasma Antiradical Capacity in Healthy Subjects after Acute Intake of Pigmented Rice: A Crossover Randomized Controlled Clinical Trial. J. Clin. Med. 9:9103209. doi: 10.3390/jcm9103209
Walter, M., Marchesan, E., Massoni, P. F. S., da Silva, L. P., Sartori, G. M. S., and Ferreira, R. B. (2013). Antioxidant properties of rice grains with light brown, red and black pericarp colors and the effect of processing. Food Res. Internat. 50, 698–703. doi: 10.1016/J.FOODRES.2011.09.002
Wang, H., Sun, H., Zhang, P., and Fang, Z. (2019). Effects of processing on the phenolic contents, antioxidant activity and volatile profile of wheat bran tea. Internat. J. Food Sci. Technol. 54, 3156–3165. doi: 10.1111/IJFS.14255
Wang, O., Liu, J., Cheng, Q., Guo, X., Wang, Y., Zhao, L., et al. (2015). Effects of Ferulic Acid and γ-Oryzanol on High-Fat and High-Fructose Diet-Induced Metabolic Syndrome in Rats. PLoS One 10:118135. doi: 10.1371/JOURNAL.PONE.0118135
Wang, Q., Li, Y., Sun, D.-W., and Zhu, Z. (2018). Enhancing Food Processing by Pulsed and High Voltage Electric Fields: Principles and Applications. Crit. Rev. Food Sci. Nutr. 58, 2285–2298. doi: 10.1080/10408398.2018.1434609
Wang, T., Raddatz, J., and Chen, G. (2013). Effects of microfluidization on antioxidant properties of wheat bran. J. Cereal Sci. 58, 380–386. doi: 10.1016/J.JCS.2013.07.010
Wang, T., Zhu, Y., Sun, X., Raddatz, J., Zhou, Z., and Chen, G. (2014b). Effect of microfluidisation on antioxidant properties of corn bran. Food Chem. 152, 37–45. doi: 10.1016/J.FOODCHEM.2013.11.059
Wang, T., He, F., and Chen, G. (2014a). Improving bioaccessibility and bioavailability of phenolic compounds in cereal grains through processing technologies: A concise review. J. Funct. Foods 7, 101–111. doi: 10.1016/J.JFF.2014.01.033
Wang, X., Zhang, X., Hou, H., Ma, X., Sun, S., Wang, H., et al. (2020). Metabolomics and gene expression analysis reveal the accumulation patterns of phenylpropanoids and flavonoids in different colored-grain wheats (Triticum aestivum L.). Food Res. Internat. 138:109711. doi: 10.1016/J.FOODRES.2020.109711
Wang, Z., Li, S., Ge, S., and Lin, S. (2020). Review of Distribution, Extraction Methods, and Health Benefits of Bound Phenolics in Food Plants. J. Agricult. Food Chem. 68, 3330–3343. doi: 10.1021/ACS.JAFC.9B06574
Wanyo, P., Meeso, N., and Siriamornpun, S. (2014). Effects of different treatments on the antioxidant properties and phenolic compounds of rice bran and rice husk. Food Chem. 157, 457–463. doi: 10.1016/J.FOODCHEM.2014.02.061
Wongjaikam, S., Summart, R., and Chewonarin, T. (2014). Apoptosis induction in colon cancer cell lines and alteration of aberrant crypt foci in rat colon by purple rice (Oryza sativa L. var. glutinosa) extracts. Nutr. Can. 66, 690–699. doi: 10.1080/01635581.2014.899371
Xia, X., Ling, W., Ma, J., Xia, M., Hou, M., Wang, Q., et al. (2006). An Anthocyanin-Rich Extract from Black Rice Enhances Atherosclerotic Plaque Stabilization in Apolipoprotein E–Deficient Mice. J. Nutr. 136, 2220–2225. doi: 10.1093/jn/136.8.2220
Xiang, N., Guo, X., Liu, F., Li, Q., Hu, J., and Brennan, C. S. (2017). Molecular Sciences Effect of Light-and Dark-Germination on the Phenolic Biosynthesis, Phytochemical Profiles, and Antioxidant Activities in Sweet Corn (Zea mays L.) Sprouts. Internat. J. Mole. Sci. 2017:1806146. doi: 10.3390/ijms18061246
Xu, E., Wu, Z., Long, J., Wang, F., Xu, X., Jin, Z., et al. (2015). Improved bioaccessibility of phenolics and antioxidant activity of glutinous rice and its fermented Chinese rice wine by simultaneous extrusion and enzymatic hydrolysis. J. Funct. Foods 17, 214–226. doi: 10.1016/J.JFF.2015.05.032
Xu, J., Tian, C., Hu, Q., Luo, J., Wang, X., and Tian, X. (2009). Dynamic changes in phenolic compounds and antioxidant activity in oats (Avena nuda L.) during steeping and germination. J. Agricult. Food Chem. 57, 10392–10398. doi: 10.1021/JF902778J
Xu, K., Debelo, H., Roman, L., Guo, M., Ferruzzi, M. G., and Martinez, M. M. (2021). Co-extruded wheat/okra composite blends result in soft, cohesive and resilient crumbs rich in health-promoting compounds. Food Chem. 364:130395. doi: 10.1016/J.FOODCHEM.2021.130395
Yin, Z., Wu, W., Sun, C., Lei, Z., Chen, H., Liu, H., et al. (2018). Comparison of releasing bound phenolic acids from wheat bran by fermentation of three Aspergillus species. Int. J. Food SciTechnol. 53, 1120–1130. doi: 10.1111/ijfs.13675
Yong, H., Liu, J., Qin, Y., Bai, R., Zhang, X., and Liu, J. (2019). Antioxidant and pH-sensitive films developed by incorporating purple and black rice extracts into chitosan matrix. Internat. J. Biol. Macromole. 137, 307–316. doi: 10.1016/J.IJBIOMAC.2019.07.009
Zeng, Z., Hu, X., McClements, D. J., Luo, S., Liu, C., Gong, E., et al. (2019). Hydrothermal stability of phenolic extracts of brown rice. Food Chem. 271, 114–121. doi: 10.1016/J.FOODCHEM.2018.07.180
Zeng, Z., Liu, C., Luo, S., Chen, J., and Gong, E. (2016). The Profile and Bioaccessibility of Phenolic Compounds in Cereals Influenced by Improved Extrusion Cooking Treatment. PLoS One 11:e0161086. doi: 10.1371/JOURNAL.PONE.0161086
Zeng, Z., Luo, S., Liu, C., Hu, X., Gong, E., and Miao, J. (2018). Phenolic retention of brown rice after extrusion with mesophilic α-amylase. Food Biosci. 21, 8–13. doi: 10.1016/J.FBIO.2017.10.008
Zhang, G., Xu, Z., Gao, Y., Huang, X., Zou, Y., and Yang, T. (2015). Effects of Germination on the Nutritional Properties, Phenolic Profiles, and Antioxidant Activities of Buckwheat. J. Food Sci. 80, H1111–H1119. doi: 10.1111/1750-3841.12830
Zhang, R., Khan, S. A., Chi, J., Wei, Z., Zhang, Y., Deng, Y., et al. (2018). Different effects of extrusion on the phenolic profiles and antioxidant activity in milled fractions of brown rice. LWT 88, 64–70. doi: 10.1016/J.LWT.2017.09.042
Zhang, S., Sun, L., Ju, H., Bao, Z., Zeng, X., and Lin, S. (2021). Research advances and application of pulsed electric field on proteins and peptides in food. Food Res. Internat. 139:109914. doi: 10.1016/J.FOODRES.2020.109914
Zhao, G., Zhang, R., Dong, L., Huang, F., Tang, X., Wei, Z., et al. (2018). Particle size of insoluble dietary fiber from rice bran affects its phenolic profile, bioaccessibility and functional properties. LWT 87, 450–456. doi: 10.1016/J.LWT.2017.09.016
Zhou, K., Yin, J.-J., and Yu, L. (2005). Phenolic Acid, Tocopherol and Carotenoid Compositions, and Antioxidant Functions of Hard Red Winter Wheat Bran. J. Agricult. Food Chem. 53, 3916–3922. doi: 10.1021/JF050117C
Zhu, F. (2015). Review: Interactions between starch and phenolic compound. Trends Food Sci. Technol. 43, 129–143. doi: 10.1016/j.tifs.2015.02.003
Zhu, F., Du, B., Li, R., and Li, J. (2014). Effect of micronization technology on physicochemical and antioxidant properties of dietary fiber from buckwheat hulls. Biocataly. Agricult. Biotech. 3, 30–34. doi: 10.1016/J.BCAB.2013.12.009
Zhu, Y., Li, T., Fu, X., Brennan, M., Abbasi, A. M., Zheng, B., et al. (2016). The use of an enzymatic extraction procedure for the enhancement of highland barley (Hordeum vulgare L.) phenolic and antioxidant compounds. Internat. J. Food Sci. Technol. 51, 1916–1924. doi: 10.1111/IJFS.13165
Keywords: cereals, phenolics, flavonoid, anthocyanin, pigmented cereals, post-harvest process
Citation: Kasote D, Tiozon RN Jr, Sartagoda KJD, Itagi H, Roy P, Kohli A, Regina A and Sreenivasulu N (2021) Food Processing Technologies to Develop Functional Foods With Enriched Bioactive Phenolic Compounds in Cereals. Front. Plant Sci. 12:771276. doi: 10.3389/fpls.2021.771276
Received: 06 September 2021; Accepted: 27 October 2021;
Published: 30 November 2021.
Edited by:
Jinsong Bao, Zhejiang University, ChinaReviewed by:
Márcio Carocho, Centro de Investigação de Montanha (CIMO), PortugalErick Paul Gutiérrez-Grijalva, Consejo Nacional de Ciencia y Tecnología (CONACYT), Mexico
Copyright © 2021 Kasote, Tiozon, Sartagoda, Itagi, Roy, Kohli, Regina and Sreenivasulu. This is an open-access article distributed under the terms of the Creative Commons Attribution License (CC BY). The use, distribution or reproduction in other forums is permitted, provided the original author(s) and the copyright owner(s) are credited and that the original publication in this journal is cited, in accordance with accepted academic practice. No use, distribution or reproduction is permitted which does not comply with these terms.
*Correspondence: Nese Sreenivasulu, bi5zcmVlbml2YXN1bHVAaXJyaS5vcmc=