- 1Key Laboratory of Economic Plants and Biotechnology, Kunming Institute of Botany, Chinese Academy of Sciences, Kunming, China
- 2Yunnan Key Laboratory for Wild Plant Resources, Kunming, China
- 3Key Laboratory of Tropical Forest Ecology, Xishuangbanna Tropical Botanical Garden, Chinese Academy of Sciences, Kunming, China
- 4State Key Laboratory of Forest and Soil Ecology, Institute of Applied Ecology, Chinese Academy of Sciences, Shenyang, China
- 5The Arnold Arboretum of Harvard University, Boston, MA, USA
Epiphytes that grow in the canopies of tropical and subtropical forests experience different water regimes when compared with terrestrial plants. However, the differences in adaptive strategies between epiphytic and terrestrial plants with respect to plant water relations remain poorly understood. To understand how water-related traits contrast between epiphytic and terrestrial growth forms within the Cymbidium (Orchidaceae), we assessed leaf anatomy, hydraulics, and physiology of seven terrestrial and 13 epiphytic species using a common garden experiment. Compared with terrestrial species, epiphytic species had higher values for leaf mass per unit area (LMA), leaf thickness (LT), epidermal thickness, saturated water content (SWC) and the time required to dry saturated leaves to 70% relative water content (T70). However, vein density (Dvein), stomatal density (SD), and photosynthetic capacity (Amax) did not differ significantly between the two forms. T70 was positively correlated with LT, LMA, and SWC, and negatively correlated with stomatal index (SI). Amax showed positive correlations with SD and SI, but not with Dvein. Vein density was marginally correlated with SD, and significantly correlated with SI. Overall, epiphytic orchids exhibited substantial ecophysiological differentiations from terrestrial species, with the former type showing trait values indicative of greater drought tolerance and increased water storage capacity. The ability to retain water in the leaves plays a key role in maintaining a water balance in those epiphytes. Therefore, the process of transpiration depends less upon the current substrate water supply and enables epiphytic Cymbidium species to adapt more easily to canopy habitats.
Introduction
Epiphytes are an important component of tropical and subtropical floras, and serve vital ecological functions in forest hydrology and nutrient fluxes (Benzing, 1990; Zotz and Bader, 2009). Approximately 7.5% of all vascular plants are epiphytes, including species from at least 84 families. Epiphytic species comprise more than 70% of the members within the Orchidaceae (Benzing, 1990; Silvera et al., 2009). However, the low availability of substrates in epiphytic habitats results in restricted and irregular moisture supplies, making water shortages the most limiting factor for the establishment and growth of epiphytes (Benzing, 1990; Zotz and Hietz, 2001; Laube and Zotz, 2003). Plants growing in canopy habitats can experience more dramatic fluctuations in vapor pressure deficits (VPDs) than those found in terrestrial habitats (Watkins and Cardelús, 2012). For example, during the dry season, a decrease in leaf water potential has a stronger impact on the conductance of leaf water vapor by epiphytic Anthurium bredemeyeri Schott than by terrestrial plants of the same species (Rada and Jaimez, 1992). These findings indicate that significant differences in water relations may exist between epiphytic and terrestrial growth forms. However, there is a lack of comprehensive understanding of the divergences in water-related traits between closely related epiphytic and terrestrial species (Silvera et al., 2009).
Multiple morphological and physiological adaptations have evolved in epiphytes to deal with drought stress, such as CAM metabolism, low surface-to-volume ratios, and velamentous root tissues capable of rapid water uptake (Benzing, 1990; Zotz and Tyree, 1996; Reyes-García et al., 2008; Sun et al., 2014). The niche differentiation in epiphytic bromeliads (Bromeliaceae) is linked to their capacity for water storage, dependence on fog or dewfall, and physiological plasticity (Reyes-García et al., 2012). This indicates that marked tissue storage capacity is one of the most important mechanisms by which epiphytes adapt to water-limited habitats (Zotz and Bader, 2009; Reyes-García et al., 2012). That is because greater storage capacity leads to the maintenance of more stable hydraulic functioning in plants during drought periods (Goldstein et al., 1998; Stratton et al., 2000; Pivovaroff et al., 2014). In leaves, storage capacity is linked to morphological traits (Ogburn and Edwards, 2010, 2013; Zhang et al., 2012; Pivovaroff et al., 2014). For example, leaves of epiphytic fern species are generally thicker than those of terrestrial species, thereby contributing to higher storage capacity (Watkins et al., 2007; Watkins and Cardelús, 2012). However, epiphytic Paphiopedilum (Orchidaceae) species do not have significantly thicker leaves than terrestrial species (Zhang et al., 2012). In addition, leaf succulence in association with a CAM photosynthetic pathway often favors survival in water-limited habitats (Ogburn and Edwards, 2010, 2012). But CAM has only been observed in a few epiphytic species and not in any terrestrial species in the genus Cymbidium (Orchidaceae; Motomura et al., 2008). This indicates that the strategies by which plants adjust to water stress conditions may be species-specific (Ennajeh et al., 2010).
Leaf hydraulics is another important aspect of plant performance that underlies its adaptability to different habitats (Brodribb et al., 2007; Nardini and Luglio, 2014). Because supplying water to the evaporative surfaces is essential for maintaining the opening of stomata, vein density (Dvein) is often positively correlated with the capacity for water transport, stomatal conductance, and maximum photosynthetic rates across species (Sack and Holbrook, 2006; Brodribb et al., 2007, 2013). Therefore, Dvein often varies across habitats in order to match transpiration demands in a changing environment (Sack and Scoffoni, 2013). For example, epiphytic Paphiopedilum species have higher Dvein than their terrestrial counterparts (Zhang et al., 2012). Moreover, both stomatal density (SD) and dimensions can respond to the environmental conditions under which plants grow because they primarily affect stomatal conductance, potential transpiration demand, and the rate of CO2 uptake (Franks et al., 2009; Brodribb and Jordan, 2011). For example, epiphytic Paphiopedilum species have more stomata than terrestrial species (Zhang et al., 2012). Drought stress leads to more densely packed but smaller stomata (Ennajeh et al., 2010). Those smaller stomata enable plants to respond more quickly to environmental stimuli, and leaves can achieve greater diffusive conductance under favorable conditions (Drake et al., 2012). Both vein density and SD can be quite low in succulent plants (von Willert et al., 1992; North et al., 2013; Ogburn and Edwards, 2013). However, the role of leaf hydraulics (especially water supply) in maintaining a water balance is rarely examined for such plants with high capacity for water storage.
Plants adapt to contrasting environments through simultaneous configurations of multiple characteristics because their ecophysiological traits interact with one another (Dunbar-Co et al., 2009). This may contribute to the optimization of carbon investments toward different functions (Brodribb and Jordan, 2011). Previous studies have found that leaf morphological traits play an important role in maintaining water balances in epiphytes (Zhang et al., 2012; North et al., 2013). For example, a high degree of leaf succulence can contribute to greater storage capacity and expression of CAM metabolism (Ogburn and Edwards, 2010, 2013). The positive correlation between SD and vein density observed in different environments may suggest a functional association between transpiration demand and water supply, and represents an optimization of the carbon investment between those two functions (Brodribb and Jordan, 2011; Murphy et al., 2014). Therefore, being able to identify any correlations between traits and growth forms would provide insight into the changes in leaf traits associated with shifts in habitats, as well as the mechanisms required for those adaptations (Edwards, 2006; Hao et al., 2011).
Vascular epiphytes often show clear habitat preferences between ecosystems or within a given forest because the physiology and establishment of those species are strongly affected by the availability of substrate, water, light, and nutrients (Benzing, 1990; Zotz and Ziegler, 1997; Hietz and Briones, 1998). For example, an epiphytic orchid (Epidendrum magnolia Muhl.) has a strong preference for Magnolia grandiflora L. as a host because the canopy of that host tree provides the most favorable microclimate in terms of shade and humidity (Bergstrom and Carter, 2008). The distribution and density of epiphytic orchids are positively associated with bryophyte cover and bryophyte species richness, indicating that moss cover is an important factor affecting the growth and survival of some orchid species (Crain, 2012; Cancel et al., 2013). Because the performance and distribution of epiphytes can be affected by their interactions with other plant species (Hietz, 1999; Zotz and Vollrath, 2003), a common garden experiment would help modulate the potential effects of different ecological associations, and would ensure that any interspecific differences measured were not merely the result of plastic responses to variable growing conditions (Edwards, 2006).
In this study, we used a common garden experiment to assess 19 leaf traits related to plant water relations in seven terrestrial and 13 epiphytic Cymbidium (Orchidaceae) species. Our main objectives were to clarify the differentiation of leaf traits between those two growth forms and understand the ecological strategies employed by epiphytic species to occupy epiphytic habitats. We hypothesized that epiphytes differ from terrestrials in terms of traits related to drought tolerance, with epiphytes showing more capacity to adapt to the environments within low-moisture canopies.
Materials and Methods
Study Site and Plant Materials
The genus Cymbidium includes some of the most prized and popular ornamental plants in the world because of their highly decorative flowers. This genus comprises 50 species that are widely distributed from subtropical Asia to northern Australia (Yukawa et al., 2002). Although diverse ecological and physiological characteristics have evolved within Cymbidium, few studies have focused on the ecological differentiation among growth forms (Motomura et al., 2008). The wide range of variations in their morphological and physiological traits among species makes Cymbidium an ideal subject for understanding the specific traits that contribute to ecological adaptations among growth forms.
A total of 20 orchid species – seven terrestrial and 13 epiphytic – were selected from nine sections within Cymbidium. Their growth forms, native habitat features, and carbon stable isotope ratios are shown in Supplementary Table S1 (supporting information). Plant materials for 15 species were collected from the nursery of Xishuangbanna Tropical Botanical Garden (XTBG; 21°41′N, 101°25′E, elevation 570 m) in southwestern China, while the other five species were obtained from Luyuan Flower Company (Supplementary Table S1). To minimize the effect that developmental differences might have on experimental results, we used mature individuals of approximately uniform size for all experiments. The plants (50–60 adults per species) were grown in the orchid garden at XTBG beginning in May of 2011. This study site has a mean annual temperature of 21.7°C, an average relative humidity of 66–87%, and an average annual precipitation of 1560 mm, with 80% occurring during the rainy season between May and October. The dry period includes a foggy sub-season from November to February, which is characterized by a high frequency of radiation fog at night and in the morning. Weather during the hot sub-season, from March to April, features dry, hot conditions in the afternoon and dense radiation fog only in the morning (Liu et al., 2013). All plants were grown in wooden boxes filled with a mixture of 70% bark (0.5–2.0 cm in size) and 30% humus. On clear days, the peak light intensity was 280 to 330 μmol m-2 s-1 at noon. Plants were watered at least once a week, and fertilized monthly with a nutrient solution containing 27.6 g L-1 NH4NO3, 17.7 g L-1 KH2PO4, 17.5 g L-1 K2SO4, 0.5 g L-1 MgSO4, and 1.5 g L-1 CaCl2. They produced new ramets from the bases in spring. From June to August in 2012, newly formed, mature leaves were collected from the current-year ramets and used for physiological and anatomical measurements. By the time this sampling began, all plants, regardless of origin, had been growing in the same environment for more than 1 year and were presumed to be fully acclimated to the growing conditions at XTBG (Reyes-García et al., 2008). All traits were measured from six selected plants per species.
Leaf Physiology
Maximum photosynthetic rate (Amax) and transportation rate (Tr) were determined from six mature leaves (for all analyses one leaf each from six plants per species was used), using a Li-Cor 6400 portable photosynthesis system equipped with a 6400-40 fluorescence chamber (Li-Cor, Inc., Lincoln, NE, USA). All measurements were made from 09:00 to 11:30 when CO2 uptake was maximal and water availability was optimal. Leaves were pre-exposed for more than 30 min to a 300 μmol m-2 s-1 irradiance (90% red + 10% blue) to induce the maximum stomatal aperture. The determination of this light level was based on data for light saturation points for photosynthesis in these Cymbidium species (Supplementary Figure S1 in supporting information). During the measurement period, the light intensity was set at 300 μmol m-2 s-1 and the CO2 concentration was maintained at ambient level. The leaf temperature varied between 25 and 27°C and the leaf-to-air VPD ranged from 0.7 to 0.9 kPa.
Leaf water content and the degree of succulence were determined for mature leaves. The leaves were excised in the morning, sealed in plastic bags, and immediately transported to the laboratory. Fresh weight (FW) was recorded to the nearest 0.0001 g on a digital balance. These leaves were then soaked in deionized water for 12 h to achieve full hydration before being re-weighed to obtain their saturated fresh weights (SFW). Afterward, leaves were oven-dried at 70°C for 48 h to determine dry weight (DW). Relative water content (RWC) was calculated as (FW – DW)/(SFW – DW) × 100. SWC, an indicator of water storage capacity, was calculated as (SFW – DW)/DW (Stratton et al., 2000).
The rate of water loss after excision was measured from undamaged, mature leaves. The collected leaves were saturated overnight with distilled water. After the cut petioles were sealed with Parafilm, the leaves were placed on a lab bench under dim light (3–4 μmol m-2 s-1), with an air temperature of ∼25°C and an average VPD of 2.2–2.9 kPa. FWs were measured periodically on a digital balance. At the end of the observation period, the surface area of each leaf was evaluated with a Li-Cor 3000A area meter (Li-Cor, Inc.), and the leaves were then oven-dried at 70°C for 48 h to obtain DW. The rates of water loss between RWCs of 90 and 60% were used to calculate the mean epidermal transpiration rate (Emin), because the rates within that range are considered most stable (Hao et al., 2010). Epidermal conductance (gmin) was calculated by dividing Emin by the daily average value for mole fraction VPD (VPD/atmospheric pressure). As a threshold for physiological damage, the time needed to dry a saturated leaf to 70% RWC (T70) was determined by regressing RWC against the time interval from leaf excision to each measurement of FW (Hao et al., 2010).
Leaf Anatomy and Morphology
After mature leaves were collected, each was divided along its midrib. One half of a leaf was soaked for 1 h in a 5% NaOH aqueous solution to remove mesophyll tissue for analyzing vein density (Dvein), while the other half was used for examining the stomata. For vein observations, after the mesophyll tissues were removed, three sections were excised from the top, middle, and bottom portions of a leaf, and stained with 1% safranin and mounted in glycerol to obtain the vein density. Samples were photographed at 10 × magnification with a digital camera mounted on a Leica DM2500 microscope (Leica Microsystems Vertrieb GmbH, Wetzlar, Germany). Vein lengths were determined from digital images via the IMAGEJ program (http://rsb.info.nih.gov/ij/). Values for Dvein were expressed as vein length per unit area. For stomatal observations, the lower and upper epidermises were peeled from the middle portions of fresh leaves, and the images were captured under the Leica DM2500 microscope. Stomata were observed in 30 randomly selected fields, and SD was calculated as the number of stomata per unit leaf area, and stomatal length (SL) was expressed as the length of guard cells.
Six mature leaves were sampled for assessing anatomy and leaf mass per unit area (LMA). Each was cut along the midrib and one half was scanned with a Li-Cor 3000A area meter before being oven-dried at 70°C for 48 h for assessing LMA. The other half was fixed in FAA (formalin: acetic acid: alcohol: distilled water = 10:5:50:35) for at least 24 h prior to anatomical analysis. Transverse sections were examined and photographed with the Leica DM2500 microscope. Measurements of vessel diameter (Dvessel) were made on 30 vessels randomly chosen from the transverse section of the petiole. Thicknesses of the upper cuticle (UCT), lower cuticle (LCT), upper epidermis (UET), lower epidermis (LET), and the whole leaf (LT) were determined with the IMAGEJ program. Leaf density (LD) was calculated as LMA/LT.
Data Analysis
All statistical analyses were conducted with R software (R Core Team, 2013). Differences in leaf traits between epiphytic and terrestrial growth forms were assessed by independent t-tests. A principal component analysis (PCA) was done with the ‘prcomp’ function of the package ‘vegan’ to characterize the associations among traits or species. Relationships among variables were examined by Pearson’s product-moment correlations (cor. test function in R package).
Results
Although all of the tested leaf traits varied significantly across species, the magnitude of variation differed for each trait (Table 1). The coefficients of variation (CVs) were >50% for LT, epidermal conductance (gmin) and the time required for drying of saturated leaves to 70% RWC (T70), but <10% for RWC and SL. Across all traits, T70 had the highest variation (69.16%), while RWC had the lowest (2.31%). Overall, the magnitude of variation was larger for water-related physiological traits than for structural traits.
Seven of the 18 leaf traits differed significantly between terrestrial and epiphytic species. The latter form had higher values for LMA, upper epidermal thickness (UET), lower epidermal thickness (LET), SWC, LT, T70, and Dvessel, whereas the values for vein density (Dvein), SD, maximum photosynthetic rate (Amax), transpiration rate (Tr) and gmin did not significantly differ between the two forms (Table 2; Figure 1).
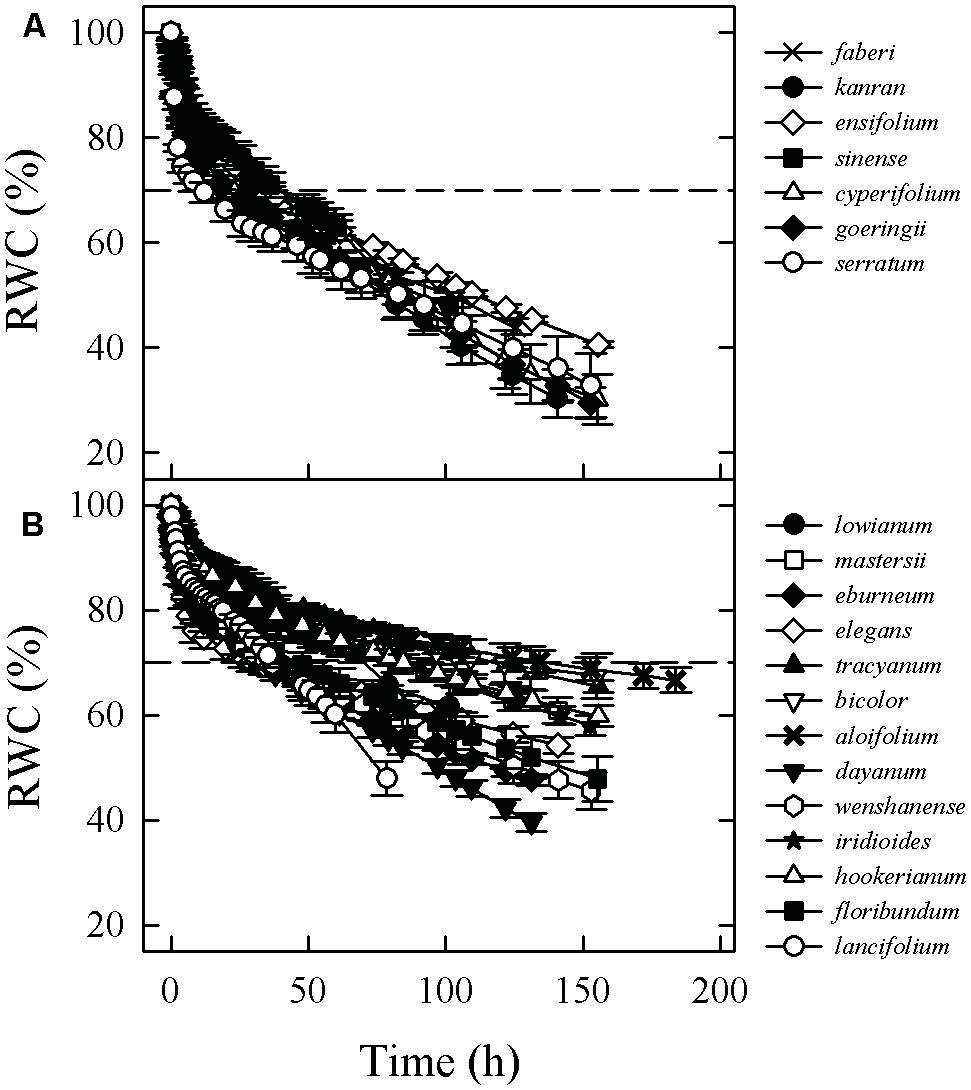
FIGURE 1. Water loss curves for leaves from terrestrial (A) and epiphytic (B) Cymbidium species, showing changes in relative water content (RWC) with time after excision. Dashed lines indicate time required for drying of saturated leaves to 70% RWC.
In our PCA of 18 leaf traits, the first two principal components explained 33.49 and 17.72% of the total variation, respectively (Figure 2). Species-loadings showed that the two growth forms were well-separated along the first PCA axis. Terrestrial species were grouped on the negative side while epiphytic species clustered on the positive side (Figure 2). The first PCA axis was associated positively with reduced LET, LMA, UET, LT, Dvessel, SWC, and T70, but negatively with LD, stomatal index (SI), Dvein, and SD (Table 3). The second PCA axis was associated positively with SL but negatively with LMA, UCT, Dvessel, Dvein, SD, SI, Amax, and RWC (Table 3).
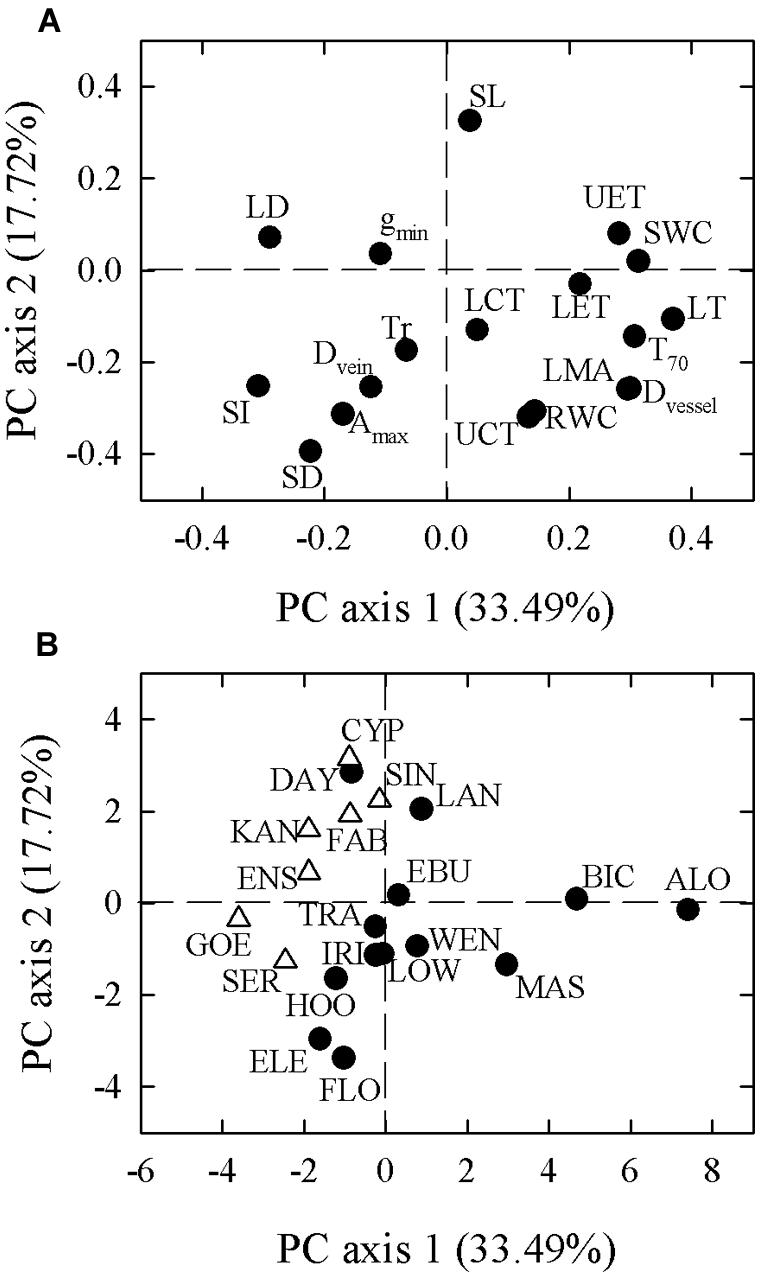
FIGURE 2. Principal component analysis (PCA) based on species trait values (A) for 18 leaf functional traits in 20 Cymbidium species. Loadings of terrestrial (open triangle) and epiphytic (filled circle) species along PCA axes are presented in (B). Values in parentheses along each axis indicate percentage of explained variation. Abbreviations for traits and species are defined in Table 1 and Supplementary Table S1, respectively.
Significant relationships were found between traits associated with drought tolerance, stomatal and leaf morphology. For example, T70 was negatively correlated with SI but positively with LT, LMA, and SWC (Figure 3). SWC was negatively correlated with SD, SI, and LD, but positively with LMA and LT (Figure 4, Supplementary Table S2 in supporting information). By contrast, Amax was positively correlated with SI and SD, but not correlated at all with Dvein (Figure 5). SD was negatively correlated with SWC, but only marginally correlated with Dvein (p = 0.06, Figure 6). SI was correlated positively with Dvein but negatively with LT and SWC (Figure 7).
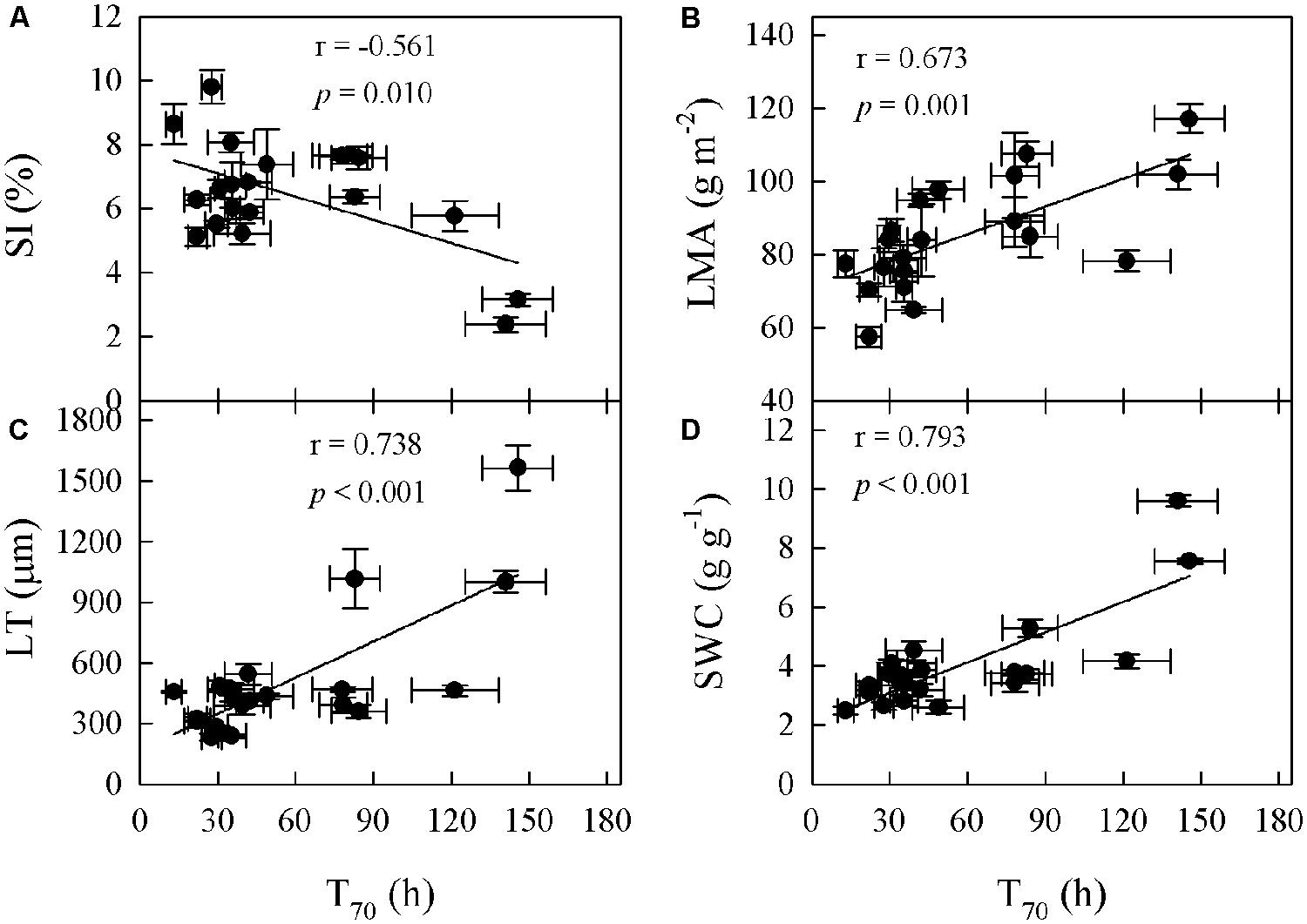
FIGURE 3. Cross-species correlations between time required for drying of saturated leaves to 70% RWC (T70) and stomatal index (SI; A), leaf thickness (LT; C), leaf mass per unit area (LMA; B), and saturated water content (SWC; D) in Cymbidium.
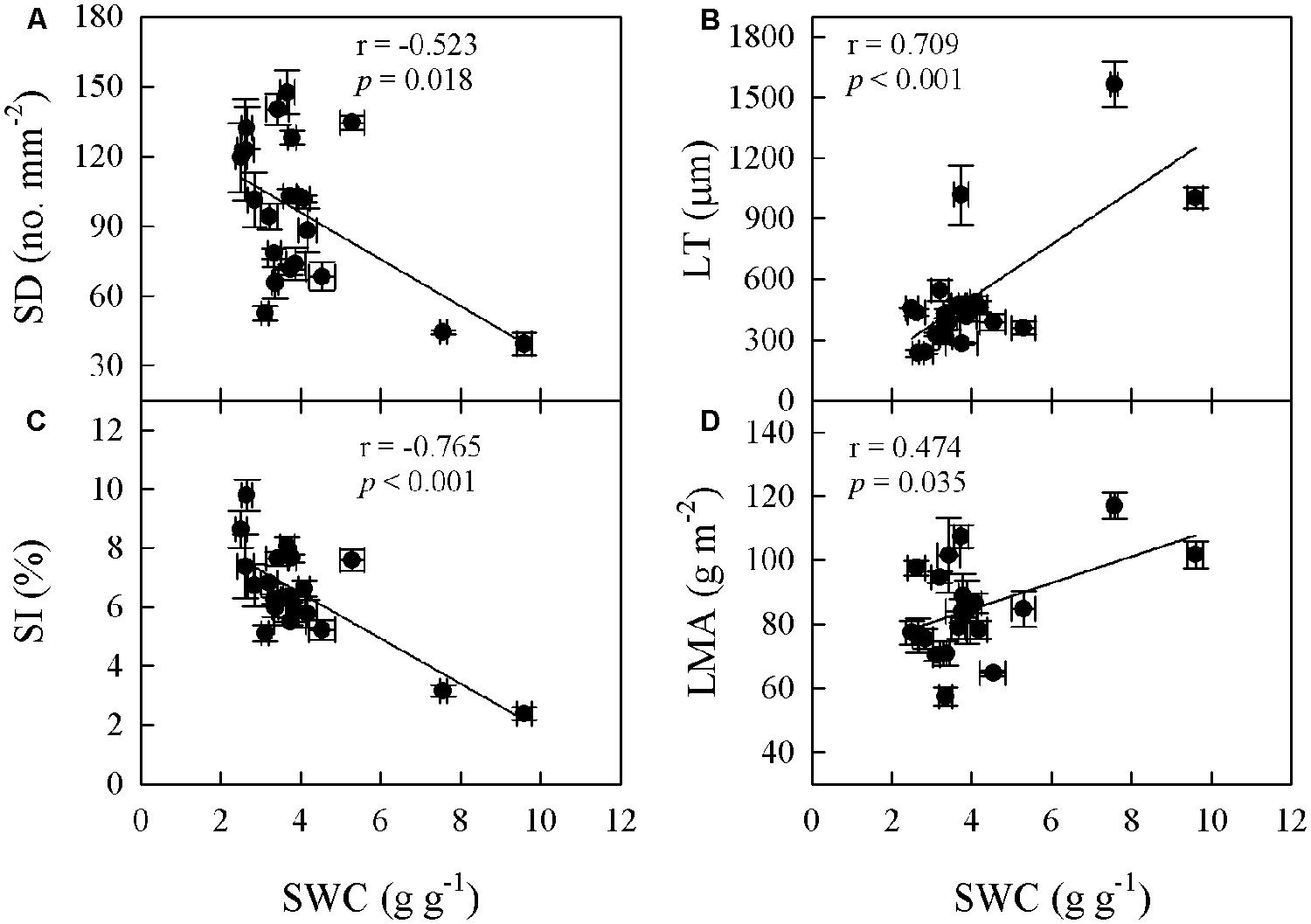
FIGURE 4. Correlations between SWC and stomatal density (SD; A), SI (C), leaf mass per unit area (LMA; D), and LT (B) across 20 Cymbidium species.
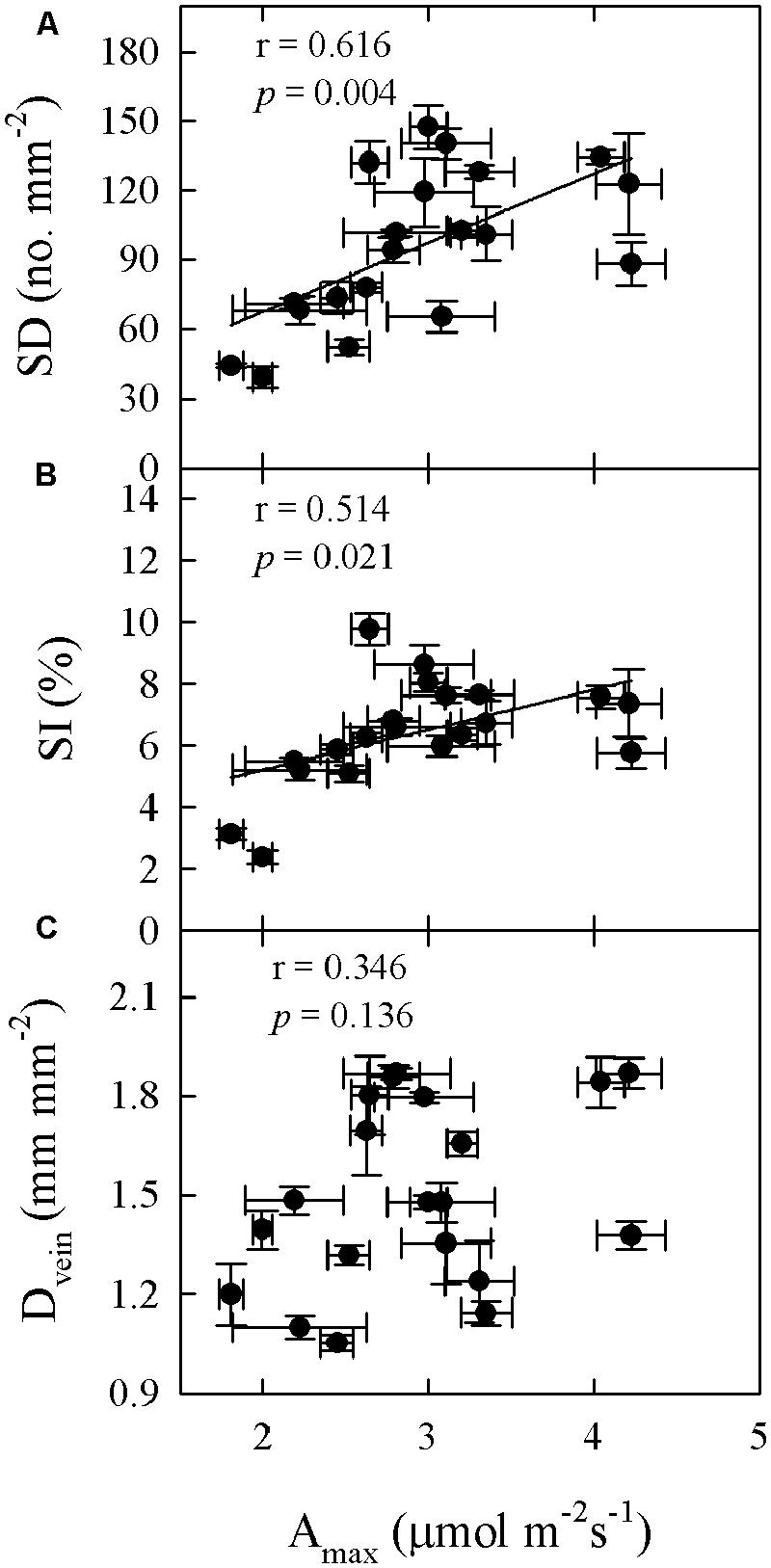
FIGURE 5. Correlations between maximum photosynthetic rate (Amax) and SD (A), SI (B), and leaf vein density (Dvein; C) across 20 Cymbidium species.
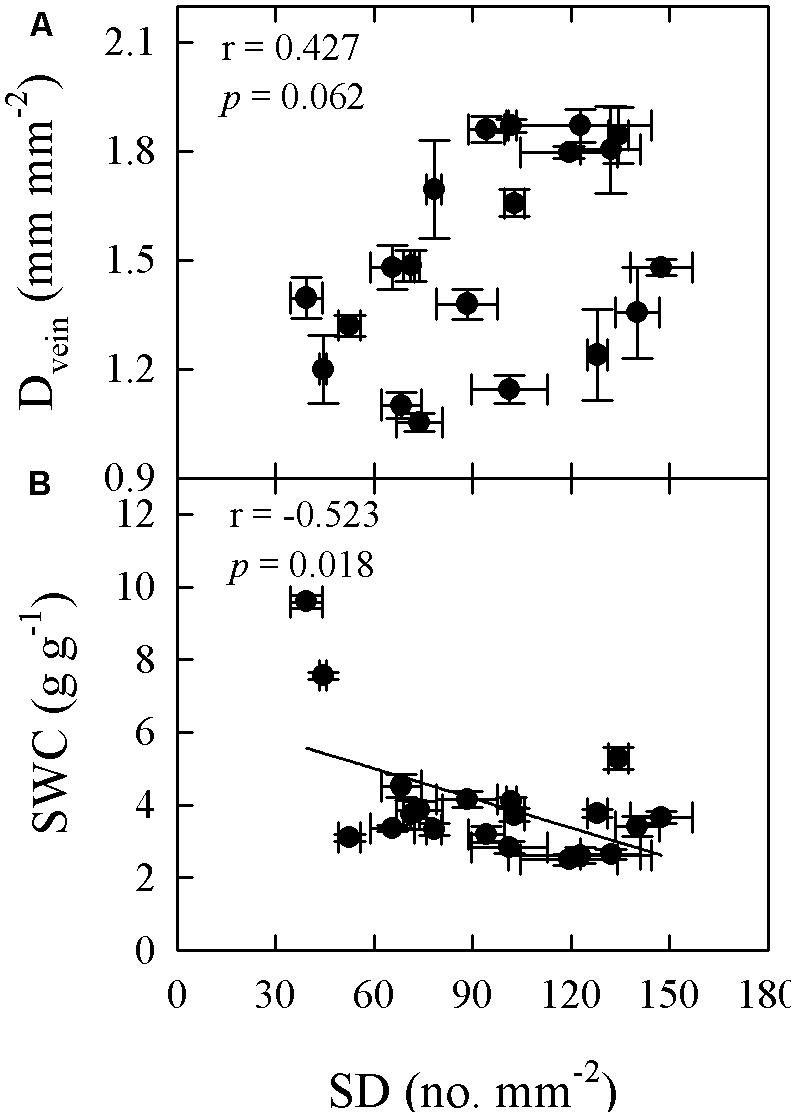
FIGURE 6. Pearson’s correlations of SD with vein density (Dvein; A) and SWC (B) across 20 Cymbidium species.
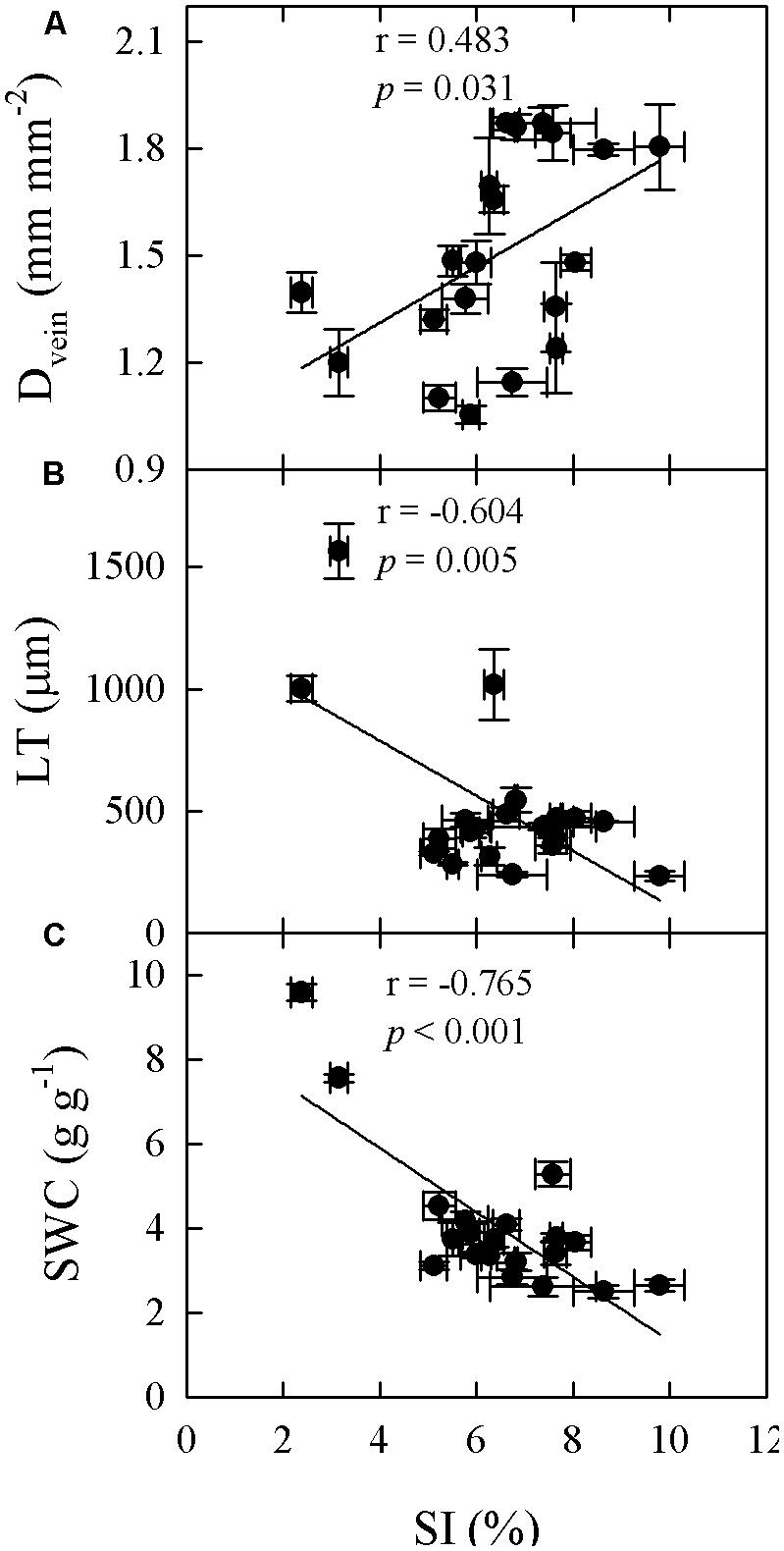
FIGURE 7. Correlations between SI and leaf vein density (Dvein; A), LT (B), and SWC (C) across 20 Cymbidium species.
Discussion
Both drought tolerance and water storage capacity are linked to leaf morphological traits (Ogburn and Edwards, 2010; Pivovaroff et al., 2014), and the results of this study highlight that drought tolerance in epiphytic and terrestrial orchid species is related to T70, SWC, epidermal thickness, and LT. These findings are consistent with previous studies that show significant physiological and morphological differences between terrestrials and epiphytes in several species (Hao et al., 2010; Watkins and Cardelús, 2012).
Previous studies on Ficus spp. (Moraceae) suggested that the value for T70 is related to how resistant the cuticle is to water loss, and is significantly higher in hemiepiphytic species than in non-hemiepiphytic species (Holbrook and Putz, 1996; Hao et al., 2010). Leaves of epiphytes also tend to be more succulent and have greater tolerance to drought than do terrestrial plants (Mantovani, 1999; Pittermann et al., 2013). Our findings and those reported by Watkins and Cardelús (2012) confirm what seems to be a general trend; leaves from epiphytic plants are more succulent and have higher LMA than terrestrial plants. Such leaves have thicker spongy parenchyma that can store more water, thereby enabling them to maintain high water potentials during drought periods (Stratton et al., 2000; Zotz and Bader, 2009; Ogburn and Edwards, 2010). In the epiphytic bromeliad (Guzmania monostachia Rusby ex Mez), the leaf base represents the main water reservoir, which maintains photosynthetic activity in more apical leaf regions when water is not available from external sources (Freschi et al., 2010). This internal storage mechanism can buffer the effects of limited water supplies and help extend the time during which the stomata can remain open, such that normal physiological processes can continue (Ogburn and Edwards, 2013; Ripley et al., 2013). Our results provide evidence that leaf morphological traits contribute to water storage, thereby highlighting the buffering role of storage capacity in improving the water balance in epiphytic Cymbidium species.
The water balance within a leaf depends upon water uptake, supply, and retention (Brodribb and Jordan, 2011; Brodribb et al., 2013; Sack and Scoffoni, 2013). We showed that traits related to xylem water transport and transpiration did not significantly differ between the two growth forms (Table 2). This contradicts previous reports that terrestrial species have greater values for SD, Dvein, and Tr than their epiphytic counterparts (Rada and Jaimez, 1992; Zhang et al., 2012, 2014). A previous study also found that stomatal densities are lower in succulent plants than in non-succulent plants (von Willert et al., 1992). We found that SD was only marginally correlated with Dvein. This is probably because locally stored water can buffer the transpiration stream, decreasing the dependence upon water uptake from the soil (Sinclair, 1983; Ogburn and Edwards, 2013). For this reason, increasing water storage may be an important strategy by which plants respond to periodic shortages, thereby conferring the ability to avoid drought at the cellular level (Ogburn and Edwards, 2010; Pivovaroff et al., 2014). However, increases in leaf succulence and thickness can counterbalance the hydraulic benefits of a high capacity for water transport (Ogburn and Edwards, 2013). In our study, because the high storage capacity found in epiphytic Cymbidium species can temporarily alleviate the challenges associated with periods of low substrate water availability, an increased capacity for water retention in the leaves likely plays a more important role in maintaining the water balance than does a more efficient transport system.
The environmental conditions in which plants grow can affect the range of variation in their functional traits (Cunningham et al., 1999; Sack and Scoffoni, 2013). We observed that although all tested Cymbidium species were grown in the same environment of a common garden, plants actually showed significant differences in traits in terms of water loss rate and water storage capacity (Figure 1; Table 2). This indicated that increased capacities for water storage and retention may represent an important adaptation for the survival of epiphytic Cymbidium species in locations where water is limiting.
Earlier research with members of Orchidaceae from Panama and Costa Rica suggested that the terrestrial growth form is the ancestral state and that epiphytism is a derived characteristic (Silvera et al., 2009). The larger total area of bark surface that epiphytes occupy allows a site to support more species per unit ground surface than could possibly coexist on a forest floor (Benzing, 1990; Gravendeel et al., 2004; Silvera et al., 2009). Because having evolved epiphytism may reduce the niche competition with terrestrial plants for space and light sources, a wider range of habitats then becomes available for diversification (Gravendeel et al., 2004; Phillips et al., 2012). Thus, the movement toward epiphytism may have increased ecological success and species richness in the family. The development of specific morphological and physiological attributes might enable epiphytic plants to cope with an irregular availability of water in canopy habitats (Benzing, 1990). For example, Griffiths and Smith (1983) found that the occurrence of CAM within the Bromeliaceae is linked to epiphytic habit. This leads the authors to suggest a polyphyletic origin in the evolutionary history of the Bromeliaceae. Silvera et al. (2009) also observed a significant correlation between photosynthetic pathway and epiphytism in tropical orchids, suggesting that CAM metabolism may be an adaptive trait acquired later in epiphytic growth forms (Benzing, 1990; Freschi et al., 2010). However, most epiphytic species in Cymbidium perform C3 photosynthesis (Motomura et al., 2008, Supplementary Table S1 in supporting information). It seems, therefore, that CAM metabolism is contributory but not essential for Cymbidium species to occupy tree canopies.
Conclusion
The epiphytic Cymbidium species investigated here exhibit substantial differentiation in their water-related traits when compared with terrestrial congeneric species, with the former type having trait values indicative of greater drought tolerance and higher water storage capacity. Because leaf succulence affects storage capacity, epiphytic Cymbidium species might experience fewer constraints on photosynthetic gas exchange. Such a phenomenon then contributes to their ability to occupy canopy habitats during the niche radiation of those species.
Conflict of Interest Statement
The authors declare that the research was conducted in the absence of any commercial or financial relationships that could be construed as a potential conflict of interest.
Acknowledgments
This work is financially supported by the National Natural Science Foundation of China (31170315, 31370362) and the Natural Science Foundation of Yunnan Province (2013FA044). We thank three anonymous reviewers for their insightful comments and suggestions for our manuscript, and Dr. Kyle Tomlinson and Priscilla Anne Licht for editing the manuscript.
Supplementary Material
The Supplementary Material for this article can be found online at: http://journal.frontiersin.org/article/10.3389/fpls.2015.00260/abstract
References
Benzing, D. H. (1990). Vascular Epiphytes. General Biology and Related Biota. Cambridge: Cambridge University Press. doi: 10.1017/CBO9780511525438
Bergstrom, B. J., and Carter, R. (2008). Host-tree selection by an epiphytic orchid, Epidendrum magnoliae Muhl. (green fly orchid), in an inland hardwood hammock in Georgia. Southeast. Nat. 7, 571–580. doi: 10.1656/1528-7092-7.4.571
Brodribb, T. J., Field, T. S., and Jordan, G. J. (2007). Leaf maximum photosynthetic rate and venation are linked by hydraulics. Plant Physiol. 144, 1890–1898. doi: 10.1104/pp.107.101352
PubMed Abstract | Full Text | CrossRef Full Text | Google Scholar
Brodribb, T. J., and Jordan, G. J. (2011). Water supply and demand remain balanced during leaf acclimation of Nothofagus cunninghamii trees. New Phytol. 192, 437–448. doi: 10.1111/j.1469-8137.2011.03795.x
PubMed Abstract | Full Text | CrossRef Full Text | Google Scholar
Brodribb, T. J., Jordan, G. J., and Carpenter, R. J. (2013). Unified changes in cell size permit coordinated leaf evolution. New Phytol. 199, 559–570. doi: 10.1111/nph.12300
PubMed Abstract | Full Text | CrossRef Full Text | Google Scholar
Cancel, J. G. G., Meléndez-Ackerman, E. J., Olaya-Arenas, P., Merced, A., Flores, N. P., and Tremblay, R. L. (2013). Associations between Lepanthes rupestris orchids and bryophyte presence in the Luquillo experimental forest, Puerto Rico. Caribb. Nat. 4, 1–14.
Crain, B. J. (2012). On the relationship between bryophyte cover and the distribution of Lepanthes spp. Lankesteriana 12, 13–18. doi: 10.15517/lank.v12i1.18270
Cunningham, S. A., Summerhayes, B., and Westoby, M. (1999). Evolutionary divergences in leaf structure and chemistry, comparing rainfall and soil nutrient gradients. Ecol. Monogr. 69, 569–588. doi: 10.1890/0012-9615(1999)069[0569:EDILSA]2.0.CO;2
Drake, P. L., Froend, R. H., and Franks, P. J. (2012). Smaller, faster stomata: scaling of stomatal size, rate of response, and stomatal conductance. J. Exp. Bot. 64, 495–505. doi: 10.1093/jxb/ers347
PubMed Abstract | Full Text | CrossRef Full Text | Google Scholar
Dunbar-Co, S., Sporck, M. J., and Sack, L. (2009). Leaf trait diversification and design in seven rare taxa of the Hawaiian Plantago radiation. Int. J. Plant Sci. 170, 61–75. doi: 10.1086/593111
Edwards, E. J. (2006). Correlated evolution of stem and leaf hydraulic traits in Pereskia (Cactaceae). New Phytol. 172, 479–489. doi: 10.1111/j.1469-8137.2006.01850.x
PubMed Abstract | Full Text | CrossRef Full Text | Google Scholar
Ennajeh, M., Vadel, A. M., Cochard, H., and Khemira, H. (2010). Comparative impacts of water stress on the leaf anatomy of a drought-resistant and a drought-sensitive olive cultivar. J. Hortic. Sci. Biotechnol. 85, 289–294.
Franks, P. J., Drake, P. L., and Beerling, D. J. (2009). Plasticity in maximum stomatal conductance constrained by negative correlation between stomatal size and density: an analysis using Eucalyptus globulus. Plant Cell Environ. 32, 1737–1748. doi: 10.1111/j.1365-3040.2009.002031.x
PubMed Abstract | Full Text | CrossRef Full Text | Google Scholar
Freschi, L., Takahashi, C. A., Cambui, C. A., Semprebom, T. R., Cruz, A. B., Mioto, P. T., et al. (2010). Specific leaf areas of the tank bromeliad Guzmania monostachia perform distinct functions in response to water shortage. J. Plant Physiol. 167, 526–533. doi: 10.1016/j.jplph.2009.10.011
PubMed Abstract | Full Text | CrossRef Full Text | Google Scholar
Goldstein, G., Andrade, J. L., Meinzer, F. C., Holbrook, N. M., Cavelier, J., Jackson, P., et al. (1998). Stem water storage and diurnal patterns of water use in tropical forest canopy trees. Plant Cell Environ. 21, 397–406. doi: 10.1046/j.1365-3040.1998.00273.x
Gravendeel, B., Smithson, A., Slik, F. J. W., and Schuiteman, A. (2004). Epiphytism and pollinator specialization: drivers for orchid diversity. Philos. Trans. R. Soc. Lond. B Biol. Sci. 359, 1523–1535. doi: 10.1098/rstb.2004.1529
PubMed Abstract | Full Text | CrossRef Full Text | Google Scholar
Griffiths, H., and Smith, J. A. C. (1983). Photosynthetic pathways in the Bromeliaceae of Trinidad: relations between life-forms, habitat preference and the occurrence of CAM. Oecologia 60, 176–184. doi: 10.1007/BF00379519
Hao, G.-Y., Goldstein, G., Sack, L., Holbrook, N. M., Liu, Z.-H., Wang, A.-Y., et al. (2011). Ecology of hemiepiphytism in fig species is based on evolutionary correlation of hydraulics and carbon economy. Ecology 92, 2117–2130. doi: 10.1890/11-0269.1
PubMed Abstract | Full Text | CrossRef Full Text | Google Scholar
Hao, G.-Y., Sack, L., Wang, A.-Y., Cao, K.-F., and Goldstein, G. (2010). Differentiation of leaf water flux and drought tolerance traits in hemiepiphytic and non-hemiepiphytic Ficus tree species. Funct. Ecol. 24, 731–740. doi: 10.1111/j.1365-2435.2010.01724.x
Hietz, P. (1999). Diversity and Conservation of Epiphytes in a Changing Environment. Available at: http://www.iupac.org/symposia/proceedings/phuket97/hietz.html
Hietz, P., and Briones, O. (1998). Correlation between water relations and within-canopy distribution of epiphytic ferns in a Mexican cloud forest. Oecologia 114, 305–316. doi: 10.1007/s004420050452
Holbrook, N. M., and Putz, F. E. (1996). From epiphyte to tree: differences in leaf structure and leaf water relations associated with the transition in growth form in eight species of hemiepiphytes. Plant Cell Environ. 19, 631–642. doi: 10.1111/j.1365-3040.1996.tb00398.x
Laube, S., and Zotz, G. (2003). Which abiotic factors limit vegetative growth in a vascular epiphyte? Funct. Ecol. 17, 598–604. doi: 10.1046/j.1365-2435.2003.00760.x
Liu, W., Li, P., Duan, W., and Liu, W. (2013). Dry-season water utilization by trees growing on thin karst soils in a seasonal tropical rainforest of Xishuangbanna, Southwest China. Ecohydrology 7, 927–935. doi: 10.1002/eco.1419
Mantovani, A. (1999). Leaf morpho-physiology and distribution of epiphytic aroids along a vertical gradient in a Brazilian rain forest. Selbyana 20, 241–249.
Motomura, H., Yukawa, T., Ueno, O., and Kagawa, A. (2008). The occurrence of crassulacean acid metabolism in Cymbidium (Orchidaceae) and its ecological and evolutionary implications. J. Plant Res. 121, 163–177. doi: 10.1007/s10265-007-0144-6
PubMed Abstract | Full Text | CrossRef Full Text | Google Scholar
Murphy, M. R. C., Jordan, G. J., and Brodribb, T. J. (2014). Acclimation to humidity modifies the link between leaf size and the density of veins and stomata. Plant Cell Environ. 37, 124–131. doi: 10.1111/pce.12136
PubMed Abstract | Full Text | CrossRef Full Text | Google Scholar
Nardini, A., and Luglio, J. (2014). Leaf hydraulic capacity and drought vulnerability: possible trade-offs and correlations with climate across three major biomes. Funct. Ecol. 28, 810–818. doi: 10.1111/1365-2435.12246
North, G. B., Lynch, F. H., Maharaj, F. D. R., Phillips, C. A., and Woodside, W. T. (2013). Leaf hydraulic conductance for a tank bromeliad: axial and radial pathways for moving and conserving water. Front. Plant Sci. 4:78. doi: 10.3389/fpls.2013.00078
PubMed Abstract | Full Text | CrossRef Full Text | Google Scholar
Ogburn, R. M., and Edwards, E. J. (2010). The ecological water-use strategies of succulent plants. Adv. Bot. Res. 55, 179–255. doi: 10.1016/B978-0-12-380868-4.00004-1
Ogburn, R. M., and Edwards, E. J. (2012). Quantifying succulence: a rapid, physiologically meaningful metric of plant water storage. Plant Cell Environ. 35, 1533–1542. doi: 10.1111/j.1365-3040.2012.02503.x
PubMed Abstract | Full Text | CrossRef Full Text | Google Scholar
Ogburn, R. M., and Edwards, E. J. (2013). Repeated origin of three-dimensional leaf venation releases constraints on the evolution of succulence in plants. Curr. Biol. 23, 722–726. doi: 10.1016/j.cub.2013.03.029
PubMed Abstract | Full Text | CrossRef Full Text | Google Scholar
Phillips, R. D., Dixon, K. W., and Peakall, R. (2012). Low population genetic differentiation in the Orchidaceae: implications for the diversification of the family. Mol. Ecol. 21, 5208–5220. doi: 10.1111/mec.12036
PubMed Abstract | Full Text | CrossRef Full Text | Google Scholar
Pittermann, J., Brodersen, C., and Watkins, J. E. (2013). The physiological resilience of fern sporophytes and gametophytes: advances in water relations offer new insights into an old lineage. Front. Plant Sci. 4:285. doi: 10.3389/fpls.2013.00285
PubMed Abstract | Full Text | CrossRef Full Text | Google Scholar
Pivovaroff, A., Sharifi, R., Scoffoni, C., Sack, L., and Rundel, P. (2014). Making the best of the worst of times: traits underlying combined shade and drought tolerance of Ruscus aculeatus and Ruscus microglossum (Asparagaceae). Funct. Plant Biol. 41, 11–24. doi: 10.1071/FP13047
R Core Team. (2013). R: A Language and Environment for Statistical Computing. R Foundation for Statistical Computing, Vienna. Available at: http://www.R-project.org/
Rada, F., and Jaimez, R. (1992). Comparative ecophysiology and anatomy of terrestrial and epiphytic Anthurium bredemeyeri Schott in a tropical Andean cloud forest. J. Exp. Bot. 43, 723–727. doi: 10.1093/jxb/43.5.723
Reyes-García, C., Mejia-Chang, M., and Griffiths, H. (2012). High but not dry: diverse epiphytic bromeliad adaptations to exposure within a seasonally dry tropical forest community. New Phytol. 193, 745–754. doi: 10.1111/j.1469-8137.2011.03946.x
PubMed Abstract | Full Text | CrossRef Full Text | Google Scholar
Reyes-García, C., Mejia-Chang, M., Jones, G. D., and Griffiths, H. (2008). Water vapour isotopic exchange by epiphytic bromeliads in tropical dry forests reflects niche differentiation and climatic signals. Plant Cell Environ. 31, 828–841. doi: 10.1111/j.1365-3040.2008.01789.x
PubMed Abstract | Full Text | CrossRef Full Text | Google Scholar
Ripley, B. S., Abraham, T., Klak, C., and Cramer, M. D. (2013). How succulent leaves of Aizoaceae avoid mesophyll conductance limitations of photosynthesis and survive drought. J. Exp. Bot. 64, 5485–5496. doi: 10.1093/jxb/ert314
PubMed Abstract | Full Text | CrossRef Full Text | Google Scholar
Sack, L., and Holbrook, N. M. (2006). Leaf hydraulics. Annu. Rev. Plant Physiol. Mol. Biol. 57, 361–381. doi: 10.1146/annurev.arplant.56.032604.144141
PubMed Abstract | Full Text | CrossRef Full Text | Google Scholar
Sack, L., and Scoffoni, C. (2013). Leaf venation: structure, function, development, evolution, ecology and applications in the past, present and future. New Phytol. 198, 983–1000. doi: 10.1111/nph.12253
PubMed Abstract | Full Text | CrossRef Full Text | Google Scholar
Silvera, K., Santiago, L. S., Cushman, J. C., and Winter, K. (2009). Crassulacean acid metabolism and epiphytism linked to adaptive radiations in the Orchidaceae. Plant Physiol. 149, 1838–1847. doi: 10.1104/pp.108.132555
PubMed Abstract | Full Text | CrossRef Full Text | Google Scholar
Sinclair, R. (1983). Water relations of tropical epiphytes: II. Performance during droughting. J. Exp. Bot. 34, 1664–1675. doi: 10.1093/jxb/34.12.1664
Stratton, L., Goldstein, G., and Meinzer, F. C. (2000). Stem water storage capacity and efficiency of water transport: their functional significance in a Hawaiian dry forest. Plant Cell Environ. 23, 99–106. doi: 10.1046/j.1365-3040.2000.00533.x
Sun, M., Yang, S.-J., Zhang, J.-L., Bartlett, M., and Zhang, S.-B. (2014). Correlated evolution in traits influencing leaf water balance in Dendrobium (Orchidaceae). Plant Ecol. 215, 1255–1267. doi: 10.1007/s11258-014-0383-2
von Willert, D. J., Eller, B. M., Werger, M. J. A., Brinckman, E., and Ihlenfeldt, H.-D. (1992). Life Strategies of Succulents in Deserts, with Special Reference to the Namib Desert. Cambridge: Cambridge University Press.
Watkins, J. E., and Cardelús, C. (2012). Ferns in an angiosperm world: cretaceous radiation into the epiphytic niche and diversification on the forest floor. Int. J. Plant Sci. 173, 695–710. doi: 10.1086/665974
Watkins, J. E., Rundel, P. W., and Cardelús, C. L. (2007). The influence of life form on carbon and nitrogen relationships in tropical rainforest ferns. Oecologia 153, 225–232. doi: 10.1007/s00442-007-0723-1
PubMed Abstract | Full Text | CrossRef Full Text | Google Scholar
Yukawa, T., Miyoshi, K., and Yokoyama, J. (2002). Molecular phylogeny and character evolution of Cymbidium (Orchidaceae). Bull. Natl. Sci. Mus. Ser. B Bot. 28, 129–139.
Zhang, S.-B., Guan, Z.-J., Sun, M., Cao, K.-F., and Hu, H. (2012). Evolutionary association of stomatal traits with leaf vein density in Paphiopedilum, Orchidaceae. PLoS ONE 7:e40080. doi: 10.1371/journal.pone.0040080
PubMed Abstract | Full Text | CrossRef Full Text | Google Scholar
Zhang, S.-B., Sun, M., Cao, K.-F., Hu, H., and Zhang, J.-L. (2014). Leaf photosynthetic rate of tropical ferns is evolutionarily linked to water transport capacity. PLoS ONE 9:e84682. doi: 10.1371/journal.pone.0084682
PubMed Abstract | Full Text | CrossRef Full Text | Google Scholar
Zotz, G., and Bader, M. Y. (2009). Epiphytic plants in a changing world-global: change effects on vascular and non-vascular epiphytes. Prog. Bot. 70, 147–170. doi: 10.1007/978-3-540-68421-3_7
Zotz, G., and Hietz, P. (2001). The physiological ecology of vascular epiphytes: current knowledge, open questions. J. Exp. Bot. 52, 2067–2078.
Zotz, G., and Tyree, M. T. (1996). Water stress in the epiphytic orchid, Dimerandra emarginata Hoehne. Oecologia 107, 151–159. doi: 10.1007/BF00327898
Zotz, G., and Vollrath, B. (2003). The epiphyte vegetation of the palm Socratea exorrhiza: correlations with tree size, tree age and bryophyte cover. J. Trop. Ecol. 19, 81–90. doi: 10.1017/S0266467403003092
Keywords: Cymbidium, drought tolerance, epiphytes, photosynthesis, succulence, water loss, water storage, water supply
Citation: Zhang S-B, Dai Y, Hao G-Y, Li J-W, Fu X-W and Zhang J-L (2015) Differentiation of water-related traits in terrestrial and epiphytic Cymbidium species. Front. Plant Sci. 6:260. doi: 10.3389/fpls.2015.00260
Received: 24 November 2014; Accepted: 02 April 2015;
Published online: 22 April 2015.
Edited by:
Sergio Rossi, Université du Québec à Chicoutimi, CanadaReviewed by:
Aurora Gaxiola, Pontificia Universidad Católica de Chile, ChileJacob Vogenberg, University of California, Riverside, USA
Benjamin J. Crain, International Institute of Tropical Forestry, USA
Copyright © 2015 Zhang, Dai, Hao, Li, Fu and Zhang. This is an open-access article distributed under the terms of the Creative Commons Attribution License (CC BY). The use, distribution or reproduction in other forums is permitted, provided the original author(s) or licensor are credited and that the original publication in this journal is cited, in accordance with accepted academic practice. No use, distribution or reproduction is permitted which does not comply with these terms.
*Correspondence: Shi-Bao Zhang, Key Laboratory of Economic Plants and Biotechnology, Kunming Institute of Botany, Chinese Academy of Sciences, Kunming 650201, Yunnan, Chinac2J6aGFuZ0BtYWlsLmtpYi5hYy5jbg==
† These authors share first authorship.