- Department of Biotechnology, Institute of Molecular Biology and Biotechnology, Faculty of Biology, Adam Mickiewicz University, Poznan, Poland
The ubiquitin/26S proteasome system (UPS) has been implicated in the regulation of many physiological processes including hormone signaling. The plant hormone abscisic acid (ABA) employs the UPS to control its own synthesis and signaling and to regulate stress response and tolerance. Among the known effectors of ABA signaling, the ABI1 (abscisic acid-insensitive 1) protein phosphatase, which belongs to group A of the type 2C protein phosphatases, is recognized as a key component of the pathway. Molecular and genetic evidence implicates this protein phosphatase in numerous plant responses. This mini-review discusses recent progress in understanding the role of ABI1 in ABA signaling, with particular emphasis on recent data that link ABI1 to protein degradation via the UPS.
Reversible protein phosphorylation is a key protein modification involved in the regulation of numerous physiological processes. Phosphorylation and dephosphorylation are catalyzed by protein kinases and protein phosphatases, respectively. In Arabidopsis there are over 1000 genes that encode protein kinases and protein phosphatases (Fuchs et al., 2013). These enzymes have critical functions in plant growth, development and stress responses, and ongoing research in Arabidopsis highlights the importance of protein phosphatases type 2C (PP2Cs) from group A as regulatory components of the ABA signaling pathway (Miyazono et al., 2009; Nishimura et al., 2009). Genetic screening experiments indicate nine group A PP2Cs (ABI1, ABI2, HAB1, HAB2, HAI1, HAI2, HAI3, PP2CA/AHG3, and AHG1) as negative regulators of ABA signal (Gosti et al., 1999; Merlot et al., 2001; Saez et al., 2004, 2006; Kuhn et al., 2006; Nishimura et al., 2007; Rubio et al., 2009; Fuchs et al., 2013). Clade A PP2Cs by interaction with multiple proteins enable a wide range of ABA responses in plants. Members of clade A PP2Cs interact with RCAR/PYR/PYLs (Nishimura et al., 2010; Hao et al., 2011; Antoni et al., 2012), SnRK1 (SnRK1.1-2; Rodrigues et al., 2013), SnRK2 (SnRK2.2-3, SnRK2.6; Umezawa et al., 2009), CBL-interacting protein kinases (CIPK8, CIPK14-15, CIPK20; Guo et al., 2002; Ohta et al., 2003; CIPK26, Lyzenga et al., 2013), the b-ZIP transcriptional factor (ATHB6; Himmelbach et al., 2002), and glutathione peroxidase (GPX3; Miao et al., 2006) to regulate ABA signaling or response. Apart from ABA signaling, group A of Arabidopsis PP2Cs have been involved in other pathways to regulate plant growth, development, ion transport and stress acclimation (Chérel et al., 2002; Himmelbach et al., 2002; Yang et al., 2006; Yoshida et al., 2006; Saez et al., 2008; Geiger et al., 2010; Ludwików et al., 2009, 2013, 2014).
Because ABI1 and ABI2 are best known for their key roles in ABA signaling, understanding how these phosphatase activities are regulated in response to ABA is one of the most important goals in plant research. In early studies, H2O2 was proposed to be a reversible inhibitor of ABI1 because of its inhibitory effects on ABI1-dependent phosphatase activity in vitro (Meinhard and Grill, 2001). However, more recent study does not support these findings, showing that in planta ABI1 maintains high phosphatase activity in response to oxidative stress conditions (Ludwików et al., 2014). Moreover, ABI1 is regulated by additional factors that increase or maintain its activity during stress conditions, such as the Rho-like small GTPases, which also protect ABI1 from ABA–PYL/PYR inhibition. Similarly, glutathione peroxidase (GPX3) and FERONIA receptor kinase play an important role in regulating the activity of ABI2 (Miao et al., 2006; Li et al., 2012; Yu et al., 2012; Ludwików et al., 2014).
In addition to the above regulators, the last years have seen notable progress in ABA receptor research. For example, the Arabidopsis PYR/PYL/RCAR family of START proteins have been identified as ABA receptors. PYLs comprise 14 members that fall into two distinct classes, dimeric and monomeric (Melcher et al., 2009; Miyazono et al., 2009; Nishimura et al., 2009; Santiago et al., 2009; Yin et al., 2009; Dupeux et al., 2011), with different affinities for ABA (Miyazono et al., 2009; Santiago et al., 2009; Yin et al., 2009; Szostkiewicz et al., 2010). The current model of the core ABA pathway assumes that ABA receptors in complex with ABA recognize and bind to PP2Cs, releasing SnRK2s from PP2C-dependent regulation (Melcher et al., 2009; Miyazono et al., 2009; Dupeux et al., 2011). Importantly, the above studies clearly demonstrated that PYR/PYL/RCAR receptors show preferences in substrate specificity and selectively inhibit specific PP2Cs in the presence of ABA, although one particular PP2C (AHG1) has been identified as insensitive to ABA-PYL inhibition (Antoni et al., 2012). On the other hand, PYL proteins differ in their ability to inhibit the phosphatase activity of group A PP2Cs even in the absence of the ABA ligand. PYR1 and PYLs 1–3 show only weak inhibitory effects on HAB1, while PYL4 shows clear inhibition of HAB2. PYL5–9 and PYL10 constitutively inhibit particular PP2Cs to a certain degree (Hao et al., 2011). Importantly, low-affinity complexes with PP2Cs generated in the absence of ABA are insufficient to activate ABA signaling (Antoni et al., 2012; Dupeux et al., 2011).
As well as the group A PP2Cs, an additional, underrated but essential component of ABA signaling has emerged in the last decade—the ubiquitin-proteasome system (UPS). The UPS requires the action of three types of enzymes: ubiquitin-activating enzymes (E1), a ubiquitin-conjugating enzyme (E2), and ubiquitin (Ub) ligases (E3). E3 Ub ligases determine the substrate specificity of the ubiquitination reaction and are classified into four groups: HECT, RING, U-box, and cullin-RING ligases (CRLs). Ub ligases function as either monomeric enzymes or multisubunit complexes. The largest and most diverse class of E3s are CRLs comprising the SCF (S-phase kinase-associated protein1-cullin1-F-box), the BTB (bric-a-brac-tramtrak-broad complex), the DDB (DNA damage-binding), and the APC (the anaphase-promoting complex/cyclosome) E3 Ub ligases (Vierstra, 2009; Chen et al., 2013; Stone, 2014).
Abscisic acid employs E3 Ub ligases in the management of its own synthesis and signaling to improve plant growth and development, as well as stress response and tolerance (Ko et al., 2006; Zhang et al., 2008; Raab et al., 2009; Salt et al., 2011; Chen et al., 2013; Lyzenga et al., 2013; Stone, 2014). Several ABA-responsive transcription factors, both positive and negative regulators of ABA signaling, including ABI3, ABI4, ABI5, ATHB6, ABF1, and ABF3, were found to be regulated by the UPS. The ABI3 transcription factor is polyubiquitinated by ABI3-interacting protein (AIP2), a RING-type E3 Ub ligase. ABA up-regulates AIP2 protein abundance, which in turn decreases ABI3 level. In addition, the aip2-1 mutant is hypersensitive to ABA in root growth and seed germination assays. Thus AIP2 ligase is also regarded as a negative regulator of ABA signaling (Zhang et al., 2005). The opposite regulation is observed for the ABI5 transcription factor. ABA increases ABI5 abundance by supervision of KEG (KEEP ON GOING), a RING3-type E3 ligase that targets ABI5 for ubiquitination and subsequent degradation (Liu and Stone, 2010). In this case, ABA controls ABI5 levels by causing KEG to ubiquitinate itself and thereby promote its own destruction (Liu and Stone, 2010). Another regulator of ABI5 stability has been identified recently: ABA-hypersensitive DCAF1 (ABD1), which is a substrate receptor protein, modulates ABI5 turnover in the nucleus (Seo et al., 2014).
Similar mechanisms can be observed in the turnover of the ABF1, ABF3, and ATHB6 transcription factors (Himmelbach et al., 2002; Lechner et al., 2011). ABF1 and ABF3 are ABI5-related transcription factors and positive effectors of multiple ABA responses. Importantly, ABI5, ABF1, and ABF3 interact with ABI3, a transcription factor involved in seed maturation and dormancy, and ABF3 and ABI5 exhibit redundancy (Finkelstein et al., 2005; Chen et al., 2013). ABA probably affects ABF1 and ABF3 accumulation by preventing their degradation. Interestingly, proteolysis of ABF1 and ABF3 is affected by KEG—the same E3 Ub ligase that is involved in ubiquitination of ABI5. The mechanism of ABF1 and ABF3 function is complicated. The conserved C-terminal region (RRTLTGPW motif) required for interaction with 14-3-3 protein is also necessary for ABF1 and ABF3 stabilization. Although degradation of ABF1 and ABF3 is delayed in a keg mutant, KEG ubiquitinates both full-length ABF1/3 proteins and their C-terminal deletion forms. Authors postulate that ABF1 and ABF3 are stabilized by phosphorylation, probably driven by SnRK2 kinases. In addition, interaction with 14-3-3 proteins increases ABF1 and ABF3 stability (Chen et al., 2013).
As already mentioned, ABA prevents the turnover of ATHB6, a homeobox-leucine zipper transcription factor, which is another negative regulator of the ABA response and a target of ABI1 PP2C (Himmelbach et al., 2002; Lechner et al., 2011). ATHB6 directly interacts with, and is a target for degradation by, MATH-BTB proteins. The interaction between MATH-BTB proteins and ATHB6 occurs within the leucine zipper domain of ATHB6, suggesting that this interaction may interfere with the dimerization of ATHB6 with other HD-Zip proteins (Lechner et al., 2011). Furthermore, MYB30 transcription factor, a negative regulator of ABA signaling, is targeted for degradation by MIEL1, the RING-type MYB30-Interacting E3 Ligase 1 (Marino et al., 2013). MIEL1 attenuates cell death and pathogen resistance by promoting the destruction of MYB30.
The UPS is responsible for the rapid breakdown of ABA-regulated protein kinases (Fujii et al., 2009; Lyzenga et al., 2013; Rodrigues et al., 2013). Thus, KEG triggers the degradation of CIPK26, a sucrose non-fermenting-1 (SNF1)-related protein kinase 3 (SnRK3; Lyzenga et al., 2013). Although it has been demonstrated that CIPK26 is a component of ABA signaling downstream of ABI1, it is not clear whether ABI1 affects its kinase activity. Other ABA-regulated SnRKs have also emerged as targets for polyubiquitination (Lee et al., 2008; Fragoso et al., 2009). Importantly, regulation of the kinase activity of SnRK1 and SnRK2 by ABI1 and ABI1-like phosphatases has been clearly demonstrated (Yoshida et al., 2006; Rodrigues et al., 2013). The chloroplast localized SnRK1.2 is specifically degraded in response to phosphate starvation (Fragoso et al., 2009). SnRK1.1 and SnRK1.2 interact with PRL1 (Pleiotropic Regulatory Locus 1), a subunit of a CUL4-based E3 ligase (Bhalerao et al., 1999). In addition, both SnRK1 isoforms interact with SKP1 (S-phase Kinase-associated Protein1), a component of the CUL1-based E3 complex. Nevertheless, only SnRK1.1 has been shown to be degraded by the proteasome, with its breakdown orchestrated by a CUL4-based E3 ligase that uses PRL1 as a substrate receptor (Lee et al., 2008). Other members of the SnRK family, SnRK2.4 and SnRK2.6 (Kulik et al., 2011; Stone, 2014), as well other kinases involved in ABA signaling, including CDPK2, CDPK6, MPK3, and MPK4 (Ichimura et al., 2000; Lu et al., 2002; Mori et al., 2006; Danquah et al., 2014), are also known to be targets for ubiquitination (Kim et al., 2013).
Last but not least, the PYR/PYL/RCAR ABA receptors are directed to the UPS by the proteins De-etiolated1 (DET1) and DDB1-associated1 (DDA1), both of which assemble with CUL4-based E3 Ub ligase (CRL4; Chen et al., 2013; Irigoyen et al., 2014; Figure 1A). DET1 is known as a central regulator of photomorphogenesis and thermomorphogenesis (Delker et al., 2014; Dong et al., 2014; Li et al., 2015; Ly et al., 2015). Very recently, DET1 was identified as a central repressor of light-induced seed germination that controls the stability of phytochrome interacting factor 1 (PIF1) and long hypocotyl in far-red 1 (HFR1) proteins (Shi et al., 2015). Although it is unclear how DET1 controls the stability of ABA receptors, DDA1 binds PYL4 and PYL8-9, and mediates recognition by CRL4. ABA inhibits the degradation of ABA receptor PYL8 by limiting its polyubiquitination. Nevertheless, the exact mechanism of this regulation is unknown (Irigoyen et al., 2014).
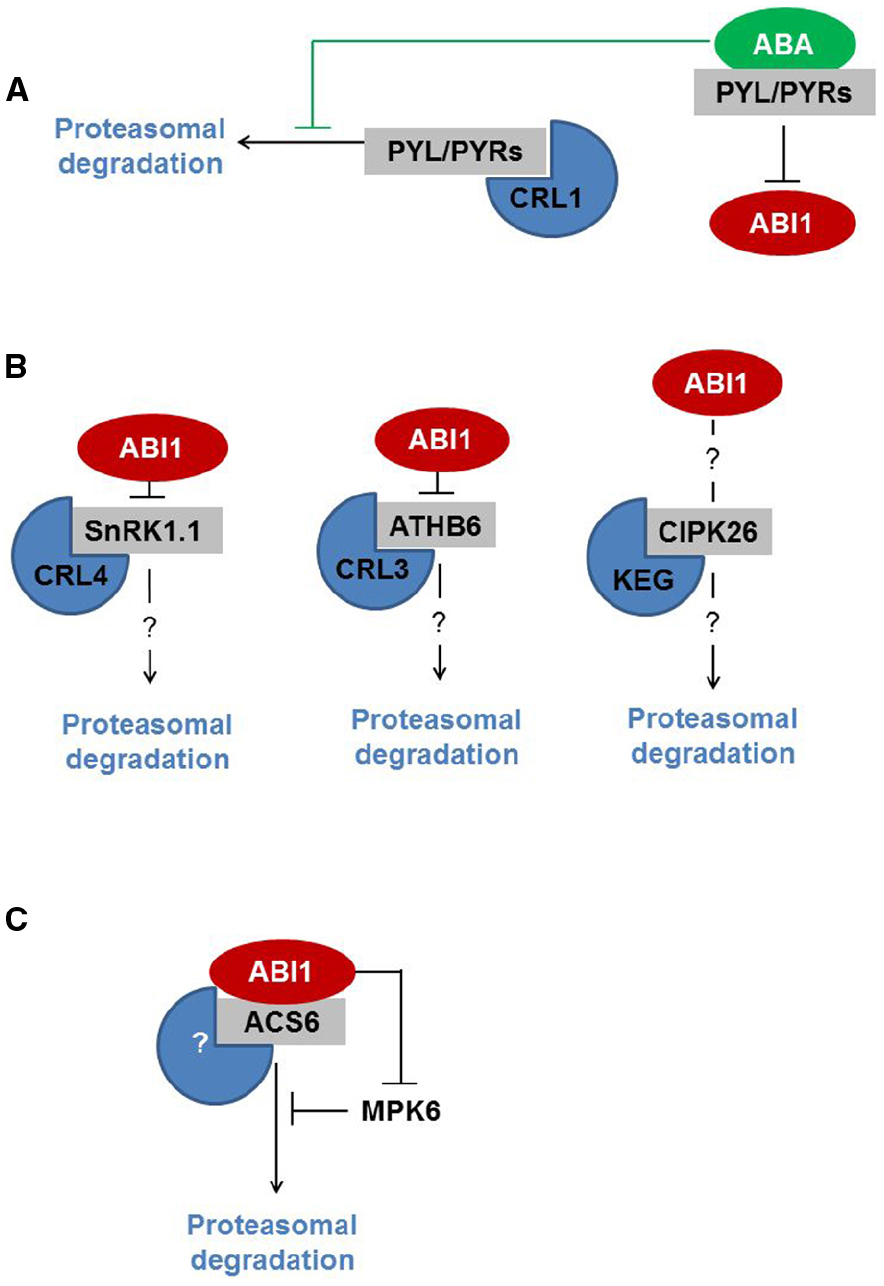
Figure 1. ABI1 interacts with proteins that are subjected to UPS-mediated proteolysis. (A) ABI1 interacts with ABA-bound PYL/PYRs, which inhibits its phosphatase activity. ABI1 downstream targets are therefore not subjected to degradation. (B) ABI1 interacts with SnRK1.1 and ATHB6, thereby modifying downstream signaling. SnRK1.1 and ATHB6 are degraded by CRL4- and CRL3- E3 Ub ligases, respectively. The role of ABI1 in the regulation of SnRK1.1 and ATHB6 is unknown. CIPK26, which is degraded by KEG, also interacts with ABI1. It is largely unclear how ABI1 regulates CIPK26-dependent signaling. (C) ABI1 interacts with ACS6. ABI1-mediated dephosphorylation targets both proteins for degradation by an unknown E3 Ub ligase. ABI1 also regulates the stability of ACS6 by affecting MPK6 kinase activity.
In the context of ABA regulators that are degraded by UPS, two common factors can be seen. Firstly, some UPS targets (like protein kinases and transcription factors) require phosphorylation for functional activation. In general, this is in line with the notion that phosphorylation and proteolysis cross-talks and are essential for ABA signal processing (Liu and Stone, 2010; Stone, 2014). Secondly, some ABA regulators (like ATHB6, SnRKs, and PYR/PYL/RCAR) are indeed the targets of ABI1 protein phosphatase (Himmelbach et al., 2002; Yoshida et al., 2006; Lyzenga et al., 2013; Rodrigues et al., 2013; Irigoyen et al., 2014; Figure 1B). So we might ask: does ABI1 contribute to the regulatory mechanism?
Following the recent report on the role of ABI1 in protein turnover, the answer to this question is positive (Figure 1C). Ludwików et al. (2014) show that under ozone stress conditions, type I ACC synthase (ACS) turnover is controlled by ABI1 at two levels: (i) ABI1 dephosphorylates ACS6 at the C-terminally located MPK6 target-site, thereby promoting ACS6 degradation; (ii) ABI1 inhibits MPK6 activity directly in this way modifying the phosphorylation rate of the ACS6 protein. Based on this report we might hypothesize that ABI1, and possibly more group A PP2Cs, target and control the turnover of other downstream regulators of the ABA signaling pathway.
In conclusion, UPS-mediated proteolysis seems to be a prominent mechanism for removing certain dephosphorylated ABA-signaling elements from the cell. In the context of recent studies on ABI1 (Figure 1), the negative regulation of ABA signaling by ABI1 takes on new meaning: ABI1 resets various signaling pathways to pre-stimulatory status by targeting downstream regulators for degradation by UPS. The future challenge in this research area undoubtedly lies in the identification of ABA-pathway regulators that are controlled by the UPS. Finally, another significant task will be to understand how ABI1 recognizes the protein targets that must be degraded. We might assume that ABI1 binds a particular protein motif, whose dephosphorylation leads to destruction of the target protein; however, currently no such motif is known. Nevertheless, the interplay between ubiquitination and phosphorylation has emerged as a key mechanism regulating protein stability (Yoo et al., 2008; Spoel et al., 2009; He and Kermode, 2010; Wang et al., 2012; Nguyen et al., 2013; Zhai et al., 2013). Whether ABI1 recognizes signals that are encoded in patterns of posttranslational modifications—we are about to learn.
Conflict of Interest Statement
The author declares that the research was conducted in the absence of any commercial or financial relationships that could be construed as a potential conflict of interest.
Acknowledgments
The Author is grateful to Agata C., Filip M., and Małgorzata T. for critical reading of the manuscript. I would like to thank the reviewers for their helpful comments and suggestions for improving the manuscript. This work was supported by a Grant from the National Science Centre (No. DEC-2012/05/B/NZ3/00352) to AL and KNOW Poznan RNA Centre, 01/KNOW2/2014.
References
Antoni, R., Gonzalez-Guzman, M., Rodriguez, L., Rodrigues, A., Pizzio, G. A., and Rodriguez, P. L. (2012). Selective inhibition of clade A phosphatases type 2C by PYR/PYL/RCAR abscisic acid receptors. Plant Physiol. 158, 970–980. doi: 10.1104/pp.111.188623
PubMed Abstract | Full Text | CrossRef Full Text | Google Scholar
Bhalerao, R. P., Salchert, K., Bako, L., Okresz, L., Szabados, L., Muranaka, T., et al. (1999). Regulatory interaction of PRL1 WD protein with Arabidopsis SNF1-like protein kinases. Proc. Natl. Acad. Sci. U.S.A. 96, 5322–5327. doi: 10.1073/pnas.96.9.5322
PubMed Abstract | Full Text | CrossRef Full Text | Google Scholar
Chen, Y. T., Liu, H., Stone, S., and Callis, J. (2013). ABA and the ubiquitin E3 ligase KEEP ON GOING affect proteolysis of the Arabidopsis thaliana transcription factors ABF1 and ABF3. Plant J. 75, 965–976. doi: 10.1111/tpj.12259
PubMed Abstract | Full Text | CrossRef Full Text | Google Scholar
Chérel, I., Michard, E., Platet, N., Mouline, K., Alcon, C., Sentenac, H., et al. (2002). Physical and functional interaction of the Arabidopsis K+ channel AKT2 and phosphatase AtPP2CA. Plant Cell 14, 1133–1146. doi: 10.1105/tpc.000943
PubMed Abstract | Full Text | CrossRef Full Text | Google Scholar
Danquah, A., de Zelicourt, A., Colcombet, J., and Hirt, H. (2014). The role of ABA and MAPK signaling pathways in plant abiotic stress responses. Biotechnol. Adv. 32, 40–52. doi: 10.1016/j.biotechadv.2013.09.006
PubMed Abstract | Full Text | CrossRef Full Text | Google Scholar
Delker, C., Sonntag, L., James, G. V., Janitza, P., Ibañez, C., Ziermann, H., et al. (2014). The DET1-COP1-HY5 pathway constitutes a multipurpose signaling module regulating plant photomorphogenesis and thermomorphogenesis. Cell Rep. 9, 1983–1989. doi: 10.1016/j.celrep.2014.11.043
PubMed Abstract | Full Text | CrossRef Full Text | Google Scholar
Dong, J., Tang, D., Gao, Z., Yu, R., Li, K., He, H., et al. (2014). Arabidopsis DE-ETIOLATED1 represses photomorphogenesis by positively regulating phytochrome-interacting factors in the dark. Plant Cell 26, 3630–3645. doi: 10.1105/tpc.114.130666
PubMed Abstract | Full Text | CrossRef Full Text | Google Scholar
Dupeux, F., Antoni, R., Betz, K., Santiago, J., Gonzalez-Guzman, M., Rodriguez, L., et al. (2011). Modulation of abscisic acid signaling in vivo by an engineered receptor-insensitive protein phosphatase type 2C allele. Plant Physiol. 156, 106–116. doi: 10.1104/pp.110.170894
PubMed Abstract | Full Text | CrossRef Full Text | Google Scholar
Finkelstein, R., Gampala, S. S., Lynch, T. J., Thomas, T. L., and Rock, C. D. (2005). Redundant and distinct functions of the ABA response loci ABA-INSENSITIVE(ABI)5 and ABRE-BINDING FACTOR (ABF)3. Plant Mol. Biol. 59, 253–267. doi: 10.1007/s11103-005-8767-2
PubMed Abstract | Full Text | CrossRef Full Text | Google Scholar
Fragoso, S., Espindola, L., Paez-Valencia, J., Gamboa, A., Camacho, Y., Martinez-Barajas, E., et al. (2009). SnRK1 isoforms AKIN10 and AKIN11 are differentially regulated in Arabidopsis plants under phosphate starvation. Plant Physiol. 149, 1906–1916. doi: 10.1104/pp.108.133298
PubMed Abstract | Full Text | CrossRef Full Text | Google Scholar
Fuchs, S., Grill, E., Meskiene, I., and Schweighofer, A. (2013). Type 2C protein phosphatases in plants. FEBS J. 280, 681–693. doi: 10.1111/j.1742-4658.2012.08670.x
PubMed Abstract | Full Text | CrossRef Full Text | Google Scholar
Fujii, H., Chinnusamy, V., Rodrigues, A., Rubio, S., Antoni, R., Park, S. Y., et al. (2009). In vitro reconstitution of an abscisic acid signalling pathway. Nature 462, 660–664. doi: 10.1038/nature08599
PubMed Abstract | Full Text | CrossRef Full Text | Google Scholar
Geiger, D., Scherzer, S., Mumm, P., Marten, I., Ache, P., Matschi, S., et al. (2010). Guard cell anion channel SLAC1 is regulated by CDPK protein kinases with distinct Ca2+ affinities. Proc. Natl. Acad. Sci. U.S.A. 107, 8023–8028. doi: 10.1073/pnas.0912030107
PubMed Abstract | Full Text | CrossRef Full Text | Google Scholar
Gosti, F., Beaudoin, N., Serizet, C., Webb, A. A., Vartanian, N., and Giraudat, J. (1999). ABI1 protein phosphatase 2C is a negative regulator of abscisic acid signaling. Plant Cell 11, 1897–1910. doi: 10.1105/tpc.11.10.1897
PubMed Abstract | Full Text | CrossRef Full Text | Google Scholar
Guo, Y., Xiong, L., Song, C. P., Gong, D., Halfter, U., and Zhu, J. K. (2002). A calcium sensor and its interacting protein kinase are global regulators of abscisic acid signaling in Arabidopsis. Dev. Cell 3, 233–244. doi: 10.1016/S1534-5807(02)00229-0
PubMed Abstract | Full Text | CrossRef Full Text | Google Scholar
Hao, Q., Yin, P., Li, W., Wang, L., Yan, C., Lin, Z., et al. (2011). The molecular basis of ABA-independent inhibition of PP2Cs by a subclass of PYL proteins. Mol. Cell 42, 662–672. doi: 10.1016/j.molcel.2011.05.011
PubMed Abstract | Full Text | CrossRef Full Text | Google Scholar
He, X., and Kermode, A. R. (2010). Programmed cell death of the megagametophyte during post-germinative growth of white spruce (Picea glauca) seeds is regulated by reactive oxygen species and the ubiquitin-mediated proteolytic system. Plant Cell Physiol. 51, 1707–1720. doi: 10.1093/pcp/pcq130
PubMed Abstract | Full Text | CrossRef Full Text | Google Scholar
Himmelbach, A., Hoffmann, T., Leube, M., Hohener, B., and Grill, E. (2002). Homeodomain protein ATHB6 is a target of the protein phosphatase ABI1 and regulates hormone responses in Arabidopsis. EMBO J. 21, 3029–3038. doi: 10.1093/emboj/cdf316
PubMed Abstract | Full Text | CrossRef Full Text | Google Scholar
Ichimura, K., Mizoguchi, T., Yoshida, R., Yuasa, T., and Shinozaki, K. (2000). Various abiotic stresses rapidly activate Arabidopsis MAP kinases ATMPK4 and ATMPK6. Plant J. 24, 655–665. doi: 10.1046/j.1365-313x.2000.00913.x
PubMed Abstract | Full Text | CrossRef Full Text | Google Scholar
Irigoyen, M. L., Iniesto, E., Rodriguez, L., Puga, M. I., Yanagawa, Y., Pick, E., et al. (2014). Targeted degradation of abscisic acid receptors is mediated by the ubiquitin ligase substrate adaptor DDA1 in Arabidopsis. Plant Cell 26, 712–728. doi: 10.1105/tpc.113.122234
PubMed Abstract | Full Text | CrossRef Full Text | Google Scholar
Kim, D. Y., Scalf, M., Smith, L. M., and Vierstra, R. D. (2013). Advanced proteomic analyses yield a deep catalog of ubiquitylation targets in Arabidopsis. Plant Cell 25, 1523–1540. doi: 10.1105/tpc.112.108613
PubMed Abstract | Full Text | CrossRef Full Text | Google Scholar
Ko, J. H., Yang, S. H., and Han, K. H. (2006). Upregulation of an Arabidopsis RING-H2 gene, XERICO, confers drought tolerance through increased abscisic acid biosynthesis. Plant J. 47, 343–355. doi: 10.1111/j.1365-313X.2006.02782.x
PubMed Abstract | Full Text | CrossRef Full Text | Google Scholar
Kuhn, J. M., Boisson-Dernier, A., Dizon, M. B., Maktabi, M. H., and Schroeder, J. I. (2006). The protein phosphatase AtPP2CA negatively regulates abscisic acid signal transduction in Arabidopsis, and effects of abh1 on AtPP2CA mRNA. Plant Physiol. 140, 127–139. doi: 10.1104/pp.105.070318
PubMed Abstract | Full Text | CrossRef Full Text | Google Scholar
Kulik, A., Wawer, I., Krzywinska, E., Bucholc, M., and Dobrowolska, G. (2011). SnRK2 protein kinases—key regulators of plant response to abiotic stresses. OMICS 15, 859–872. doi: 10.1089/omi.2011.0091
PubMed Abstract | Full Text | CrossRef Full Text | Google Scholar
Lechner, E., Leonhardt, N., Eisler, H., Parmentier, Y., Alioua, M., Jacquet, H., et al. (2011). MATH/BTB CRL3 receptors target the homeodomain-leucine zipper ATHB6 to modulate abscisic acid signaling. Dev. Cell 21, 1116–1128. doi: 10.1016/j.devcel.2011.10.018
PubMed Abstract | Full Text | CrossRef Full Text | Google Scholar
Lee, J. H., Terzaghi, W., Gusmaroli, G., Charron, J. B., Yoon, H. J., Chen, H., et al. (2008). Characterization of Arabidopsis and rice DWD proteins and their roles as substrate receptors for CUL4-RING E3 ubiquitin ligases. Plant Cell 20, 152–167. doi: 10.1105/tpc.107.055418
PubMed Abstract | Full Text | CrossRef Full Text | Google Scholar
Li, K., Gao, Z., He, H., Terzaghi, W., Fan, L. M., Deng, X. W., et al. (2015). Arabidopsis DET1 represses photomorphogenesis in part by negatively regulating DELLA protein abundance in darkness. Mol. Plant 8, 622–630. doi: 10.1016/j.molp.2014.12.017
PubMed Abstract | Full Text | CrossRef Full Text | Google Scholar
Li, Z., Li, Z., Gao, X., Chinnusamy, V., Bressan, R., Wang, Z. X., et al. (2012). ROP11 GTPase negatively regulates ABA signaling by protecting ABI1 phosphatase activity from inhibition by the ABA receptor RCAR1/PYL9 in Arabidopsis. J. Integr. Plant Biol. 54, 180–188. doi: 10.1111/j.1744-7909.2012.01101.x
PubMed Abstract | Full Text | CrossRef Full Text | Google Scholar
Liu, H., and Stone, S. L. (2010). Abscisic acid increases Arabidopsis ABI5 transcription factor levels by promoting KEG E3 ligase self-ubiquitination and proteasomal degradation. Plant Cell 22, 2630–2641. doi: 10.1105/tpc.110.076075
PubMed Abstract | Full Text | CrossRef Full Text | Google Scholar
Lu, C., Han, M. H., Guevara-Garcia, A., and Fedoroff, N. V. (2002). Mitogen-activated protein kinase signaling in postgermination arrest of development by abscisic acid. Proc. Natl. Acad. Sci. U.S.A. 99, 15812–15817. doi: 10.1073/pnas.242607499
PubMed Abstract | Full Text | CrossRef Full Text | Google Scholar
Ludwików, A., Babula-Skowrońska, D., Szczepaniak, M., Belter, N., Dominiak, E., and Sadowski, J. (2013). Expression profiles and genomic organisation of group A protein phosphatase 2C genes in Brassica oleracea. Ann. Appl. Biol. 163, 124–134. doi: 10.1111/aab.12039
Ludwików, A., Ciesla, A., Kasprowicz-Maluski, A., Mitula, F., Tajdel, M., Galganski, L., et al. (2014). Arabidopsis protein phosphatase 2C ABI1 interacts with type I ACC synthases and is involved in the regulation of ozone-induced ethylene biosynthesis. Mol. Plant 7, 960–976. doi: 10.1093/mp/ssu025
PubMed Abstract | Full Text | CrossRef Full Text | Google Scholar
Ludwików, A., Kierzek, D., Gallois, P., Zeef, L., and Sadowski, J. (2009). Gene expression profiling of ozone-treated Arabidopsis abi1td insertional mutant: protein phosphatase 2C ABI1 modulates biosynthesis ratio of ABA and ethylene. Planta 230, 1003–1017. doi: 10.1007/s00425-009-1001-8
PubMed Abstract | Full Text | CrossRef Full Text | Google Scholar
Ly, V., Collister, D. T., Fonseca, E., Liao, T. S., and Schroeder, D. F. (2015). Light and COP1 regulate level of overexpressed DET1 protein. Plant Sci. 231, 114–123. doi: 10.1016/j.plantsci.2014.11.011
PubMed Abstract | Full Text | CrossRef Full Text | Google Scholar
Lyzenga, W. J., Liu, H., Schofield, A., Muise-Hennessey, A., and Stone, S. L. (2013). Arabidopsis CIPK26 interacts with KEG, components of the ABA signalling network and is degraded by the ubiquitin-proteasome system. J. Exp. Bot. 64, 2779–2791. doi: 10.1093/jxb/ert123
PubMed Abstract | Full Text | CrossRef Full Text | Google Scholar
Marino, D., Froidure, S., Canonne, J., Ben Khaled, S., Khafif, M., Pouzet, C., et al. (2013). Arabidopsis ubiquitin ligase MIEL1 mediates degradation of the transcription factor MYB30 weakening plant defence. Nat. Commun. 4, 1476. doi: 10.1038/ncomms2479
PubMed Abstract | Full Text | CrossRef Full Text | Google Scholar
Meinhard, M., and Grill, E. (2001). Hydrogen peroxide is a regulator of ABI1, a protein phosphatase 2C from Arabidopsis. FEBS Lett. 508, 443–446. doi: 10.1016/S0014-5793(01)03106-4
PubMed Abstract | Full Text | CrossRef Full Text | Google Scholar
Melcher, K., Ng, L. M., Zhou, X. E., Soon, F. F., Xu, Y., Suino-Powell, K. M., et al. (2009). A gate-latch-lock mechanism for hormone signalling by abscisic acid receptors. Nature 462, 602–608. doi: 10.1038/nature08613
PubMed Abstract | Full Text | CrossRef Full Text | Google Scholar
Merlot, S., Gosti, F., Guerrier, D., Vavasseur, A., and Giraudat, J. (2001). The ABI1 and ABI2 protein phosphatases 2C act in a negative feedback regulatory loop of the abscisic acid signalling pathway. Plant J. 25, 295–303. doi: 10.1046/j.1365-313x.2001.00965.x
PubMed Abstract | Full Text | CrossRef Full Text | Google Scholar
Miao, Y., Lv, D., Wang, P., Wang, X. C., Chen, J., Miao, C., et al. (2006). An Arabidopsis glutathione peroxidase functions as both a redox transducer and a scavenger in abscisic acid and drought stress responses. Plant Cell 18, 2749–2766. doi: 10.1105/tpc.106.044230
PubMed Abstract | Full Text | CrossRef Full Text | Google Scholar
Miyazono, K., Miyakawa, T., Sawano, Y., Kubota, K., Kang, H. J., Asano, A., et al. (2009). Structural basis of abscisic acid signaling. Nature 462, 609–614. doi: 10.1038/nature08583
PubMed Abstract | Full Text | CrossRef Full Text | Google Scholar
Mori, I. C., Murata, Y., Yang, Y., Munemasa, S., Wang, Y. F., Andreoli, S., et al. (2006). CDPKs CPK6 and CPK3 function in ABA regulation of guard cell S-type anion- and Ca2+-permeable channels and stomatal closure. PLoS Biol. 4:e327. doi: 10.1371/journal.pbio.0040327
PubMed Abstract | Full Text | CrossRef Full Text | Google Scholar
Nishimura, N., Hitomi, K., Arvai, A. S., Rambo, R. P., Hitomi, C., Cutler, S. R., et al. (2009). Structural mechanism of abscisic acid binding and signaling by dimeric PYR1. Science 326, 1373–1379. doi: 10.1126/science.1181829
PubMed Abstract | Full Text | CrossRef Full Text | Google Scholar
Nishimura, N., Sarkeshik, A., Nito, K., Park, S. Y., Wang, A., Carvalho, P. C., et al. (2010). PYR/PYL/RCAR family members are major in-vivo ABI1 protein phosphatase 2C-interacting proteins in Arabidopsis. Plant J. 61, 290–299. doi: 10.1111/j.1365-313X.2009.04054.x
PubMed Abstract | Full Text | CrossRef Full Text | Google Scholar
Nishimura, N., Yoshida, T., Kitahata, N., Asami, T., Shinozaki, K., and Hirayama, T. (2007). ABA-Hypersensitive Germination1 encodes a protein phosphatase 2C, an essential component of abscisic acid signalling in Arabidopsis seed. Plant J. 50, 935–949. doi: 10.1111/j.1365-313X.2007.03107.x
PubMed Abstract | Full Text | CrossRef Full Text | Google Scholar
Nguyen, L., Kolch, W., and Kholodenko, B. (2013). When ubiquitination meets phosphorylation: a systems biology perspective of EGFR/MAPK signalling. Cell Commun. Signal. 11, 52–67. doi: 10.1186/1478-811X-11-52
PubMed Abstract | Full Text | CrossRef Full Text | Google Scholar
Ohta, M., Guo, Y., Halfter, U., and Zhu, J. K. (2003). A novel domain in the protein kinase SOS2 mediates interaction with the protein phosphatase 2C ABI2. Proc. Natl. Acad. Sci. U.S.A. 100, 11771–11776. doi: 10.1073/pnas.2034853100
PubMed Abstract | Full Text | CrossRef Full Text | Google Scholar
Raab, S., Drechsel, G., Zarepour, M., Hartung, W., Koshiba, T., Bittner, F., et al. (2009). Identification of a novel E3 ubiquitin ligase that is required for suppression of premature senescence in Arabidopsis. Plant J. 59, 39–51. doi: 10.1111/j.1365-313X.2009.03846.x
PubMed Abstract | Full Text | CrossRef Full Text | Google Scholar
Rodrigues, A., Adamo, M., Crozet, P., Margalha, L., Confraria, A., Martinho, C., et al. (2013). ABI1 and PP2CA phosphatases are negative regulators of Snf1-related protein kinase1 signaling in Arabidopsis. Plant Cell 25, 3871–3884. doi: 10.1105/tpc.113.114066
PubMed Abstract | Full Text | CrossRef Full Text | Google Scholar
Rubio, S., Rodrigues, A., Saez, A., Dizon, M. B., Galle, A., Kim, T. H., et al. (2009). Triple loss of function of protein phosphatases type 2C leads to partial constitutive response to endogenous abscisic acid. Plant Physiol. 150, 1345–1355. doi: 10.1104/pp.109.137174
PubMed Abstract | Full Text | CrossRef Full Text | Google Scholar
Saez, A., Apostolova, N., Gonzalez-Guzman, M., Gonzalez-Garcia, M. P., Nicolas, C., Lorenzo, O., et al. (2004). Gain-of-function and loss-of-function phenotypes of the protein phosphatase 2C HAB1 reveal its role as a negative regulator of abscisic acid signalling. Plant J. 37, 354–369. doi: 10.1046/j.1365-313X.2003.01966.x
PubMed Abstract | Full Text | CrossRef Full Text | Google Scholar
Saez, A., Robert, N., Maktabi, M. H., Schroeder, J. I., Serrano, R., and Rodriguez, P. L. (2006). Enhancement of abscisic acid sensitivity and reduction of water consumption in Arabidopsis by combined inactivation of the protein phosphatases type 2C ABI1 and HAB1. Plant Physiol. 141, 1389–1399. doi: 10.1104/pp.106.081018
PubMed Abstract | Full Text | CrossRef Full Text | Google Scholar
Saez, A., Rodrigues, A., Santiago, J., Rubio, S., and Rodriguez, P. L. (2008). HAB1-SWI3B interaction reveals a link between abscisic acid signaling and putative SWI/SNF chromatin-remodeling complexes in Arabidopsis. Plant Cell 20, 2972–2988. doi: 10.1105/tpc.107.056705
PubMed Abstract | Full Text | CrossRef Full Text | Google Scholar
Salt, J. N., Yoshioka, K., Moeder, W., and Goring, D. R. (2011). Altered germination and subcellular localization patterns for PUB44/SAUL1 in response to stress and phytohormone treatments. PLoS ONE 6:e21321. doi: 10.1371/journal.pone.0021321
PubMed Abstract | Full Text | CrossRef Full Text | Google Scholar
Santiago, J., Dupeux, F., Round, A., Antoni, R., Park, S. Y., Jamin, M., et al. (2009). The abscisic acid receptor PYR1 in complex with abscisic acid. Nature 462, 665–668. doi: 10.1038/nature08591
PubMed Abstract | Full Text | CrossRef Full Text | Google Scholar
Seo, K. I., Lee, J. H., Nezames, C. D., Zhong, S., Song, E., Byun, M. O., et al. (2014). ABD1 is an Arabidopsis DCAF substrate receptor for CUL4-DDB1-based E3 ligases that acts as a negative regulator of abscisic acid signaling. Plant Cell 26, 695–711. doi: 10.1105/tpc.113.119974
PubMed Abstract | Full Text | CrossRef Full Text | Google Scholar
Shi, H., Wang, X., Mo, X., Tang, C., Zhong, S., and Deng, X. W. (2015). Arabidopsis DET1 degrades HFR1 but stabilizes PIF1 to precisely regulate seed germination. Proc. Natl. Acad. Sci. U.S.A. 112, 3817–3822. doi: 10.1073/pnas.1502405112
PubMed Abstract | Full Text | CrossRef Full Text | Google Scholar
Spoel, S. H., Mou, Z., Tada, Y., Spivey, N. W., Genschik, P., and Dong, X. (2009). Proteasome-mediated turnover of the transcription coactivator NPR1 plays dual roles in regulating plant immunity. Cell 137, 860–872. doi: 10.1016/j.cell.2009.03.038
PubMed Abstract | Full Text | CrossRef Full Text | Google Scholar
Stone, S. L. (2014). The role of ubiquitin and the 26S proteasome in plant abiotic stress signaling. Front. Plant Sci. 5:135. doi: 10.3389/fpls.2014.00135
PubMed Abstract | Full Text | CrossRef Full Text | Google Scholar
Szostkiewicz, I., Richter, K., Kepka, M., Demmel, S., Ma, Y., Korte, A., et al. (2010). Closely related receptor complexes differ in their ABA selectivity and sensitivity. Plant J. 61, 25–35. doi: 10.1111/j.1365-313X.2009.04025.x
PubMed Abstract | Full Text | CrossRef Full Text | Google Scholar
Umezawa, T., Sugiyama, N., Mizoguchi, M., Hayashi, S., Myouga, F., Yamaguchi-Shinozaki, K., et al. (2009). Type 2C protein phosphatases directly regulate abscisic acid-activated protein kinases in Arabidopsis. Proc. Natl. Acad. Sci. U.S.A. 106, 17588–17593. doi: 10.1073/pnas.0907095106
PubMed Abstract | Full Text | CrossRef Full Text | Google Scholar
Wang, F., Liu, P., Zhang, Q., Zhu, J., Chen, T., Arimura, S.-I., et al. (2012). Phosphorylation and ubiquitination of dynamin-related proteins (AtDRP3A/3B) synergically regulate mitochondrial proliferation during mitosis. Plant J. 72, 43–56. doi: 10.1111/j.1365-313X.2012.05052.x
PubMed Abstract | Full Text | CrossRef Full Text | Google Scholar
Vierstra, R. D. (2009). The ubiquitin-26S proteasome system at the nexus of plant biology. Nat. Rev. Mol. Cell Biol. 10, 385–397. doi: 10.1038/nrm2688
PubMed Abstract | Full Text | CrossRef Full Text | Google Scholar
Yang, Y., Sulpice, R., Himmelbach, A., Meinhard, M., Christmann, A., and Grill, E. (2006). Fibrillin expression is regulated by abscisic acid response regulators and is involved in abscisic acid-mediated photoprotection. Proc. Natl. Acad. Sci. U.S.A. 103, 6061–6066. doi: 10.1073/pnas.0501720103
PubMed Abstract | Full Text | CrossRef Full Text | Google Scholar
Yin, P., Fan, H., Hao, Q., Yuan, X., Wu, D., Pang, Y., et al. (2009). Structural insights into the mechanism of abscisic acid signaling by PYL proteins. Nat. Struct. Mol. Biol. 16, 1230–1236. doi: 10.1038/nsmb.1730
PubMed Abstract | Full Text | CrossRef Full Text | Google Scholar
Yoo, S. D., Cho, Y. H., Tena, G., Xiong, Y., and Sheen, J. (2008). Dual control of nuclear EIN3 by bifurcate MAPK cascades in C2H4 signalling. Nature 451, 789–795. doi: 10.1038/nature06543
PubMed Abstract | Full Text | CrossRef Full Text | Google Scholar
Yoshida, T., Nishimura, N., Kitahata, N., Kuromori, T., Ito, T., Asami, T., et al. (2006). ABA-hypersensitive germination3 encodes a protein phosphatase 2C (AtPP2CA) that strongly regulates abscisic acid signaling during germination among Arabidopsis protein phosphatase 2Cs. Plant Physiol. 140, 115–126. doi: 10.1104/pp.105.070128
PubMed Abstract | Full Text | CrossRef Full Text | Google Scholar
Yu, F., Qian, L., Nibau, C., Duan, Q., Kita, D., Levasseur, K., et al. (2012). FERONIA receptor kinase pathway suppresses abscisic acid signaling in Arabidopsis by activating ABI2 phosphatase. Proc. Natl. Acad. Sci. U.S.A. 109, 14693–14698. doi: 10.1073/pnas.1212547109
PubMed Abstract | Full Text | CrossRef Full Text | Google Scholar
Zhai, Q., Yan, L., Tan, D., Chen, R., Sun, J., Gao, L., et al. (2013). Phosphorylation-coupled proteolysis of the transcription factor MYC2 is important for jasmonate-signaled plant immunity. PLoS Genet. 9:e1003422. doi: 10.1371/journal.pgen.1003422
PubMed Abstract | Full Text | CrossRef Full Text | Google Scholar
Zhang, X., Garreton, V., and Chua, N. H. (2005). The AIP2 E3 ligase acts as a novel negative regulator of ABA signaling by promoting ABI3 degradation. Genes Dev. 19, 1532–1543. doi: 10.1101/gad.1318705
PubMed Abstract | Full Text | CrossRef Full Text | Google Scholar
Zhang, Y., Xu, W., Li, Z., Deng, X. W., Wu, W., and Xue, Y. (2008). F-box protein DOR functions as a novel inhibitory factor for abscisic acid-induced stomatal closure under drought stress in Arabidopsis. Plant Physiol. 148, 2121–2133. doi: 10.1104/pp.108.126912
PubMed Abstract | Full Text | CrossRef Full Text | Google Scholar
Keywords: ABI1, PP2C group A, ABA signaling, proteasomal degradation, stress signaling, phosphorylation, dephosphorylation
Citation: Ludwików A (2015) Targeting proteins for proteasomal degradation—a new function of Arabidopsis ABI1 protein phosphatase 2C. Front. Plant Sci. 6:310. doi: 10.3389/fpls.2015.00310
Received: 24 February 2015; Accepted: 19 April 2015;
Published: 05 May 2015.
Edited by:
Hanjo A. Hellmann, Washington State University, USAReviewed by:
Gabriel Schaaf, Zentrum für Molekularbiologie der Pflanzen Tübingen, GermanySutton Mooney, Washington State University, USA
Copyright © 2015 Ludwików. This is an open-access article distributed under the terms of the Creative Commons Attribution License (CC BY). The use, distribution or reproduction in other forums is permitted, provided the original author(s) or licensor are credited and that the original publication in this journal is cited, in accordance with accepted academic practice. No use, distribution or reproduction is permitted which does not comply with these terms.
*Correspondence: Agnieszka Ludwików, Department of Biotechnology, Institute of Molecular Biology and Biotechnology, Faculty of Biology, Adam Mickiewicz University, Umultowska 89 Street, Collegium Biologicum, 61-614 Poznan, Poland,bHVkd2lrYUBhbXUuZWR1LnBs