- 1Departament de Biologia Vegetal, Facultat de Biologia, Universitat de Barcelona, Barcelona, Spain
- 2Centre de Recerca en Agrigenòmica, Consorci CSIC-IRTA-UAB-UB, Campus Universitat Autònoma de Barcelona, Bellaterra, Spain
- 3Departament de Bioquímica i Biologia Molecular, Facultat de Biologia, Universitat de Barcelona, Barcelona, Spain
Chlororespiration is a respiratory process located in chloroplast thylakoids which consists in an electron transport chain from NAD(P)H to oxygen. This respiratory chain involves the NAD(P)H dehydrogenase complex, the plastoquinone pool and the plastid terminal oxidase (PTOX), and it probably acts as a safety valve to prevent the over-reduction of the photosynthetic machinery in stress conditions. The existence of a similar respiratory activity in non-photosynthetic plastids has been less studied. Recently, it has been reported that tomato fruit chromoplasts present an oxygen consumption activity linked to ATP synthesis. Etioplasts and amyloplasts contain several electron carriers and some subunits of the ATP synthase, so they could harbor a similar respiratory process. This review provides an update on the study about respiratory processes in chromoplasts, identifying the major gaps that need to be addressed in future research. It also reviews the proteomic data of etioplasts and amyloplasts, which suggest the presence of a respiratory electron transport chain in these plastids.
Introduction
Plastids likely originated through the process of endosymbiosis, which consisted in the integration of a free-living photosynthetic prokaryote into a eukaryotic cell. This prokaryote was probably an ancestor of current cyanobacteria and provided its host the capacity to obtain energy through oxygenic photosynthesis (Bédard and Jarvis, 2005; Gould et al., 2008). Beside chloroplasts, different types of non-photosynthetic plastids have evolved in plants, for instance chromoplasts, amyloplasts, and elaioplasts. These plastids carry out specialized functions in non-green tissues, mainly the biosynthesis and storage of carotenoids, starch and lipids, respectively (Whatley, 1978; Bédard and Jarvis, 2005; Li and Yuan, 2013).
In cyanobacteria, photosynthetic and respiratory chains are interconnected in the same membrane and share some electron carriers, like plastoquinone (PQ; Bennoun, 1982). Although the endosymbiosis event resulted in a reduction of the metabolic complexity of the free-living prokaryote (Gould et al., 2008), plastids could have retained some relics of its ancestral respiratory pathway (Cournac et al., 2000). The first experimental pieces of evidences confirming the existence of a respiratory chain in chloroplasts were provided by Bennoun (1982), who defined chlororespiration as a light-independent electron transport pathway from NAD(P)H to oxygen in thylakoid membranes. Afterward, the characterization of the Arabidopsis thaliana mutant immutans demonstrated that the oxidase responsible of the oxygen consumption in chlororespiration is the plastid terminal oxidase (PTOX), a monomeric oxidase similar to the mitochondrial alternative oxidase (Carol et al., 1999; Wu et al., 1999). Later studies indicated that the NAD(P)H-PQ oxidoreductase activity might be performed by the thylakoidal NAD(P)H dehydrogenase complex (Ndh; Burrows et al., 1998; Endo et al., 1998; Sazanov et al., 1998) or a type II NAD(P)H dehydrogenase (Desplats et al., 2009).
Chloroplast respiration has been extensively studied and reviewed (Peltier and Cournac, 2002; Rumeau et al., 2007; McDonald et al., 2011; Foudree et al., 2012; Nawrocki et al., 2015), but there is no consensus about its biological role. The most accepted hypothesis is that chlororespiration acts as a safety valve to prevent the over-reduction of the photosynthetic machinery in stress conditions (Laureau et al., 2013; Paredes and Quiles, 2013; Zivcak et al., 2013; Yu et al., 2014). Other proposed roles are photoprotection during dark to light transition (Joët et al., 2002) and balance the ATP/NADPH requirements in chloroplasts (Rumeau et al., 2005). However, the overexpression of PTOX does not result in higher photoprotection on photosystems in stress conditions or during acclimation (Rosso et al., 2006; Heyno et al., 2009). Moreover, chlororespiratory activity is very minor given that the electron flux through PTOX is always two orders of magnitude lower than through cytochrome b6f complex (Trouillard et al., 2012). On the other hand, it has been shown that PTOX has a dual role and also participates in carotenoid biosynthesis, a crucial function during chloroplasts biogenesis (Carol et al., 1999; Aluru et al., 2001; Shahbazi et al., 2007). In any case, chlororespiration has always been considered a complement of photosynthesis, being only an element of a large network of factors involved in stress tolerance and photosynthesis regulation (Foudree et al., 2012).
The study of respiration in non-photosynthetic plastids has received less attention. Nevertheless, growing evidence has accumulated about the presence of some respiratory components in chromoplasts, etioplasts, and amyloplasts of different plant species. As a consequence, a more global role of PTOX in plastid metabolism has been suggested (Aluru et al., 2001; Morstadt et al., 2002; Barr et al., 2004; Nixon and Rich, 2006; McDonald et al., 2011; Foudree et al., 2012). Recently, two studies have provided convincing evidence about the existence of an active respiratory chain in tomato fruit chromoplasts linked to ATP synthesis (Pateraki et al., 2013; Renato et al., 2014). This article aims to review recently published results regarding the presence of respiratory activity in non-photosynthetic plastids and to identify the major gaps that need to be addressed in future research projects.
Respiration in Non-Photosynthetic Plastids
Chromoplasts
Chromoplasts are plastids specialized in the biosynthesis and accumulation of carotenoids. They are found in flowers, fruits, and roots, conferring to these plant tissues their characteristic red, orange, or yellow color. They are originated through the differentiation of other plastids, mainly chloroplasts and amyloplasts (Camara et al., 1995; Li and Yuan, 2013). Among non-photosynthetic plastids, chromoplasts are the most studied since carotenoids are relevant for the nutritional and organoleptic quality of many agricultural products (Li and Yuan, 2013).
The first hints suggesting the presence of a respiratory pathway in chromoplasts were obtained through the study of phytoene desaturase (PDS), an enzyme involved in carotenoid biosynthesis. PDS catalyzes two consecutive dehydrogenation reactions of phytoene and transfers the electrons to PQ (Norris et al., 1995). In daffodil (Narcissus pseudonarcissus) chromoplasts, Mayer et al. (1992) and Beyer et al. (1994) proposed the existence of enzymatic activities which regulate the redox estate of PQs in darkness, using NADH and NADPH as electron donors and oxygen as final acceptor. Nievelstein et al. (1995) reported an oxygen consumption activity in daffodil chromoplast membranes, which was dependent on NAD(P)H and sensitive to respiratory uncouplers, suggesting that this respiration could generate membrane proton gradients. This work was the first to define chromorespiration as a respiratory redox pathway in chromoplast membranes linked to phytoene desaturation. Later, it was described that liposomes containing chromoplast proteins and energized with an acid-base transition were able to produce ATP, suggesting that daffodil chromoplasts contain a functional H+-ATP synthase complex (Morstadt et al., 2002).
Further studies brought molecular support to the enzymatic activities attributed to chromorespiration. The PTOX was found in chromoplasts from tomato (Solanum lycopersicum) and bell pepper (Capsicum annuum) fruits (Josse et al., 2000). In both species, PTOX expression increases during ripening, paralleling PDS expression and the differentiation of chloroplasts into chromoplasts. Thus, PTOX was proposed to participate in carotenoid desaturation in ripening fruits (Josse et al., 2000). The tomato ghost mutant is impaired in PTOX gene and is equivalent to the Arabidopsis mutant immutans. The ghost phenotype is similar to PDS-deficient mutants and, as a consequence, PTOX was considered a PDS cofactor (Josse et al., 2000; Barr et al., 2004).
Recent proteomic studies have reported the presence of several proteins related to electron transport and ATP synthesis in chromoplasts. Subunits of ATP synthase, cytochrome b6f complex and Ndh are present in chromoplasts from tomato fruits (Barsan et al., 2010). Moreover, when comparing the proteome of fruit plastids at different ripening stages, it was found that some electron carriers and the ATP synthase subunits are maintained at significant levels in red tomatoes (Barsan et al., 2012). This was also confirmed in chromoplasts from watermelon (Citrullus lanatus), carrot (Daucus carota), orange cauliflower (Brassica oleracea), red papaya (Carica papaya), red bell pepper, and sweet orange (Citrus sinensis) (Zeng et al., 2011; Wang et al., 2013). Interestingly, these electron carriers and ATP synthase subunits are present even when chromoplasts are not originated from chloroplasts and they have never performed photosynthesis, like in carrots or in watermelon and orange pulp.
On the other hand, a large number of proteins involved in carbohydrate metabolism were found in chromoplasts of several species, for instance some enzymes of glycolysis and the pentose phosphate pathway, translocators of triose-phosphate, glucose-6-phosphate, adenine nucleotides, etc. (Siddique et al., 2006; Zeng et al., 2011; Barsan et al., 2012; Wang et al., 2013). Also, it has been described that isolated chromoplasts are able to synthesize large amounts of lipids without external supply of ATP (Angaman et al., 2012). All these findings suggest an important activity of energy production within chromoplasts and points out a more general role of chromorespiration beyond its contribution to carotenoid desaturation.
Chromoplastic ATP synthesis was further evidenced when an atypical isoform of the ATP synthase complex was identified in tomato fruit chromoplasts (Pateraki et al., 2013). This ATP synthase contains a specific γ-subunit (γ2) which increases its expression during ripening and is absent in green tissues. The silencing of this subunit caused an inhibition of chromoplast ATP synthesis (Pateraki et al., 2013). In photosynthetic tissues, the γ-subunit (γ1) of ATP synthase has a regulatory role. It contains a dithiol domain which provides a redox switch to inactivate the complex in dark conditions, preventing ATP hydrolysis (Samra et al., 2006). However, the γ2 isoform does not have the cysteine residues of the dithiol domain, suggesting that the ATP synthase complex is always active. This atypical γ2-subunit is also found in plastids from other non-photosynthetic tissues, like roots (Kohzuma et al., 2012). It is possible that the γ2-subunit has evolved to work efficiently in the physiological conditions of non-photosynthetic plastids, which may present lower electrochemical potentials than chloroplasts (Pateraki et al., 2013).
Respiration and ATP synthesis were quantified in isolated tomato fruit chromoplasts using NAD(P)H as electron donors (Renato et al., 2014). It was found that octyl gallate, an inhibitor of PTOX, prevented both respiration and ATP synthesis, confirming experimentally that PTOX is involved in chromorespiration (Renato et al., 2014). Also, the use of specific inhibitors suggested the participation of two different NAD(P)H dehydrogenases and the cytochrome b6f complex. Moreover, the existence of proton gradients through chromoplast membranes was evidenced by the study of proton uncouplers and sonicated chromoplasts (Renato et al., 2014).
A Model for Chromorespiration
Even though the components of the chromorespiratory pathway are still unclear, we propose a preliminary model with the aim of summarizing the available data and suggesting future research (Figure 1). The electron transport chain is probably located in the inner membranes of chromoplasts, which form elongated sacs or convoluted compartments (Renato et al., 2014). Both NADH and NADPH transfer electrons to the oxidized PQ pool probably through the Ndh, which is similar to the mitochondrial complex I and is able to pump protons across membranes. Ndh is present in chromoplasts and its dysfunction severely affects fruit ripening (Nashilevitz et al., 2010), so it could play a significant bioenergetic role. However, type II NAD(P)H dehydrogenase may also participate in chromorespiration (Renato et al., 2014). Type II dehydrogenases are monomeric enzymes without proton pumping activity which are targeted to mitochondria, plastids, and peroxisomes (Carrie et al., 2008). The presence of these enzymes in chromoplasts has not been yet tested, and further studies are needed to clarify this issue. Besides, the PQ pool is also reduced by PDS, which transfers the electrons resulting from the desaturation steps of phytoene during carotenoid biosynthesis (Norris et al., 1995).
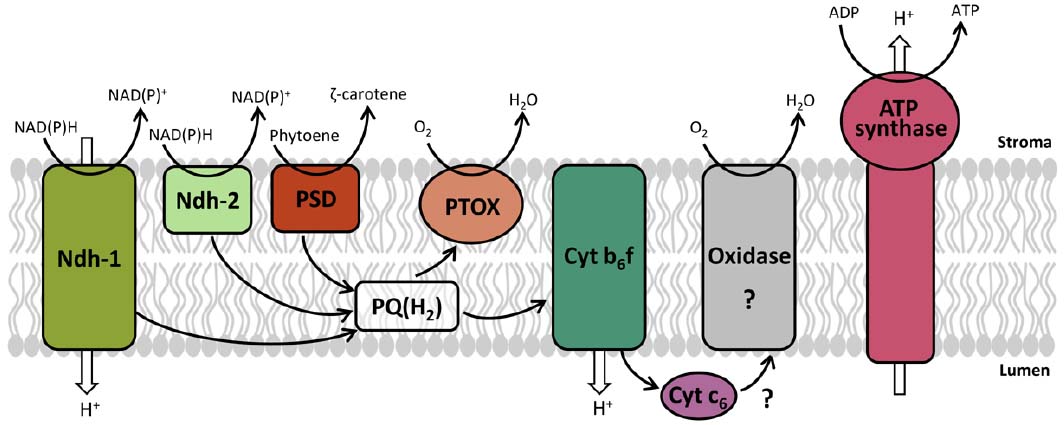
Figure 1. Model proposed for chromorespiration. The electron transport chain is located in the inner membranes of chromoplasts, and the electron entrance through NADH and NADPH takes place by the stromal side. The electron carriers shown are: Ndh-1, NAD(P)H dehydrogenase complex; Ndh-2, type II NAD(P)H dehydrogenase; PDS, phytoene desaturase; PTOX, plastid terminal oxidase; Cyt b6f, cytochrome b6f complex; Cyt c6, cytochrome c6. A possible cytochrome c oxidase or similar (Oxidase) is proposed. ATP synthase complex is also shown. Electron transfer reactions are indicated by black arrows and proton movement across membrane by white arrows.
The reduced PQ pool (PQH2) may transfer the electrons directly to PTOX, the terminal oxidase which is responsible of the oxygen consumption activity in chromoplasts (Renato et al., 2014). Alternatively, the electrons could pass through the cytochrome b6f complex, adding a supplementary proton pumping site. This hypothesis is supported by the marked effect of a cytochrome b6f inhibitor in chromoplast ATP synthesis (Renato et al., 2014). However, the electron acceptor of the cytochrome b6f is unknown. Plastocyanin plays this role in chloroplasts, but it is only found in photosynthetic tissues (Wastl et al., 2002). An alternative electron acceptor could be the cytochrome c6. This cytochrome is present in cyanobacteria and is involved in the interconnection of respiratory and photosynthetic chains: it takes electrons from cytochrome b6f and transfers them to photosystem I or to cytochrome c oxidases (Ho et al., 2011). Cytochrome c6 is also present in higher plants, although its function is not yet known (Wastl et al., 2002). In tomato fruit, cytochrome c6 expression increases during ripening, paralleling PTOX expression and chromoplast differentiation (Renato et al., 2014), suggesting that it may participate in chromorespiration. Further experimental evidences are needed to clarify this point.
As mentioned above, in cyanobacteria the cytochrome c6 can play a respiratory role and transfer the electrons to a cytochrome c oxidase (Hart et al., 2005; Ho et al., 2011). Several proteomic studies have reported the presence of some subunits of cytochrome c oxidase in chromoplasts from tomato fruit (Barsan et al., 2010, 2012; Wang et al., 2013), orange fruit (Zeng et al., 2011), watermelon, carrot, papaya, and bell pepper (Wang et al., 2013). This result may be interpreted as mitochondrial contamination. However, it has been obtained repeatedly in independent studies, so it is possible that chromoplasts could contain a plastidial form of a cytochrome c oxidase. This oxidase could have been inherited from cyanobacteria ancestor and could participate in chromorespiration oxidizing the cytochrome c6 (Figure 1). Nevertheless, more studies are required to elucidate this issue.
One or several components of the electron transport chain in chromoplasts pump protons across membranes (Figure 1) and the proton gradients generated are used by the plastid ATP synthase. Therefore, chromorespiration is a respiratory process that works similarly to the oxidative phosphorylation of mitochondria, where there is an electron transfer from electron donors (NADH and/or NADPH) to an electron acceptor (oxygen) coupled to proton pumping and chemiosmotic ATP synthesis.
Other Non-Photosynthetic Plastids
To our knowledge, dark respiratory processes in non-photosynthetic plastids other than chromoplasts have not been reported. However, published data suggest the presence of several electron carriers and subunits of ATP synthase in etioplasts and amyloplasts. Etioplasts are differentiated from proplastids in photosynthetic tissues grown in darkness. They are characterized by the accumulation of protochlorophyllide (chlorophyll precursor) and the presence of paracrystalline prolamellar bodies (Plöscher et al., 2011). Although etioplasts are a special type of plastid related to chloroplasts, we include them in this work because they could present a light-independent respiratory process when photosynthesis is not yet operative. Amyloplasts are specialized in the synthesis and accumulation of starch (Andon et al., 2002). We were not able to find any information regarding respiratory components in any other non-photosynthetic plastid, like elaioplasts and proteoplasts (specialized in lipid and protein accumulation, respectively).
Proteomic studies have revealed high levels of Ndh in etioplasts from barley (Hordeum vulgare, Catala et al., 1997; Guera et al., 2000), pea (Pisum sativum, Lennon et al., 2003), Arabidopsis (Peng et al., 2008), tobacco (Nicotiana tabacum, Karcher and Bock, 2002), corn (Zea mays), and rice (Oryza sativa) (Fischer et al., 1997). Its accumulation is independent of light and decreases during greening, suggesting a possible role in membrane energization when the photosynthetic electron transport chain is not yet working (Guera et al., 2000). In chloroplasts, the Ndh is assembled with PSI forming a supercomplex (Peng and Shikanai, 2011). Etioplasts are devoid of PSI and the Ndh is found as an independent functional unit (Peng et al., 2008), so its composition could be different in chloroplasts and etioplasts. Regarding this possibility, it has been reported that some subunits of the Ndh complex are modified through post-transcriptional editing. Particularly, the transcript of the ndhB subunit is altered in one nucleotide in photosynthetic tissues, but it is not edited in etiolated tissues, and as a result two different isoforms of this subunit are synthesized in chloroplasts and etioplasts (Karcher and Bock, 2002). Interestingly, this lack of transcript editing in ndhB also happens in tomato fruit chromoplasts (Kahlau and Bock, 2008).
The cytochrome b6f complex is present in etioplasts from pea and barley (Kanervo et al., 2008; Reisinger et al., 2008; Plöscher et al., 2011). Also, one of its subunits has been reported in proteomic analysis of wheat (Triticum aestivum) endosperm amyloplasts (Dupont, 2008).
Several subunits of ATP synthase have been found in significant amounts in etioplasts from pea, corn, Arabidopsis, rice, and barley (Fischer et al., 1997; Kanervo et al., 2008; Plöscher et al., 2011). The α-subunit has also been detected in amyloplasts from wheat endosperm (Andon et al., 2002; Balmer et al., 2006; Dupont, 2008). In addition, the γ2-subunit of the ATP synthase, which participates in chromorespiration, is predominantly expressed in Arabidopsis roots. A knockout of this gene affected root morphology, suggesting that it plays an important role in non-photosynthetic tissues containing amyloplasts (Kohzuma et al., 2012).
The PTOX is ubiquitously expressed in Arabidopsis and tomato (Aluru et al., 2001; Barr et al., 2004), including tissues containing etioplasts and amyloplasts such as etiolated cotyledons and roots. Also, the ultrastructure of etioplasts and amyloplasts is severely affected when PTOX is not functional (Aluru et al., 2001), indicating that some processes in these plastids depend on PTOX activity. Moreover, PTOX expression is strongly upregulated in etioplasts during the de-etiolation of dark-grown seedlings, according to the reported accumulation of carotenoids in this transition (Rodríguez-Villalón et al., 2009).
Therefore, proteomic and genomic data provide several hints about the presence of a respiratory electron transport chain in etioplasts and amyloplasts which could be linked to ATP synthesis. However, further studies are required to better understand its biological function and to identify all the electron carriers involved in this activity.
Conclusions and Future Directions
Dark respiration is one of the less understood processes in plastids. Chlororespiration is mainly considered a mechanism to adapt photosynthesis to changing environmental conditions. Recently, chromorespiration has been shown to be linked to chemiosmotic ATP synthesis, and proteomic studies suggest that a similar respiratory process may be active in etioplasts and amyloplasts.
Future studies should be conducted to address the large number of open questions regarding respiratory processes in chromoplasts and other non-green plastids. For instance, the source of the electron donors NADPH and NADH is unknown. They could be generated inside the plastids or imported from the cytosol instead. Also, the physiological role of the ATP generated by chromorespiration is not known. In ripe tomato, PTOX activity is responsible of one quarter of total fruit oxygen consumption and contribute significantly to tissue ATP content (Renato et al., 2014). It would be interesting to obtain more data about plastid respiration and ATP synthesis in other plant tissues containing chromoplasts or amyloplasts. Further understanding of respiratory processes in these plastids could be useful to overcome limitations in the biosynthesis of carotenoids and starch, and thus to improve the quality of several agricultural products. Besides, further studies about etioplast respiration could provide new data regarding the etiolation and de-etiolation transitions during germination.
Conflict of Interest Statement
The authors declare that the research was conducted in the absence of any commercial or financial relationships that could be construed as a potential conflict of interest.
Acknowledgments
Work from our group was supported by grants of the Spanish Ministerio de Ciencia e Innovación (BIO2009-09523) and Ministerio de Economía y Competitividad (AGL2013-43522-R) and the Generalitat de Catalunya (2014SGR-1434). MR received a predoctoral fellowship FPU from the Spanish Ministerio de Educación.
References
Aluru, M. R., Bae, H., Wu, D., and Rodermel, S. R. (2001). The Arabidopsis immutans mutation affects plastid differentiation and the morphogenesis of white and green sectors in variegated plants. Plant Physiol. 127, 67–77. doi: 10.1104/pp.127.1.67
Andon, N. L., Hollingworth, S., Koller, A., Yates, J. R., Haynes, P. A., and Hill, J. (2002). Proteomic characterization of wheat amyloplasts using identification of proteins by tandem mass spectrometry. Proteomics 2, 1156–1168. doi: 10.1002/1615-9861(200209)2:9<1156::AID-PROT1156>3.0.CO;2-4
Angaman, D. M., Petrizzo, R., Hernandez-Gras, F., Romero-Segura, C., Pateraki, I., Busquets, M., et al. (2012). Precursor uptake assays and metabolic analyses in isolated tomato fruit chromoplasts. Plant Methods 8, 1–10. doi: 10.1186/1746-4811-8-1
Balmer, Y., Vensel, W. H., DuPont, F. M., Buchanan, B. B., and Hurkman, W. J. (2006). Proteome of amyloplasts isolated from developing wheat endosperm presents evidence of broad metabolic capability. J. Exp. Bot. 57, 1591–1602. doi: 10.1093/jxb/erj156
Barr, J., White, W. S., Chen, L., Bae, H., and Rodermel, S. (2004). The GHOST terminal oxidase regulates developmental programming in tomato fruit. Plant Cell Environ. 27, 840–852. doi: 10.1111/j.1365-3040.2004.01190.x
Barsan, C., Sanchez-Bel, P., Rombaldi, C., Egea, I., Rossignol, M., Bouzayen, M., et al. (2010). Characteristics of the tomato chromoplast revealed by proteomic analysis. J. Exp. Bot. 61, 2413–2431. doi: 10.1093/jxb/erq070
Barsan, C., Zouine, M., Maza, E., Bian, W., Egea, I., Rossignol, M., et al. (2012). Proteomic analysis of chloroplast-to-chromoplast transition in tomato reveals metabolic shifts coupled with disrupted thylakoid biogenesis machinery and elevated energy-production components. Plant Physiol. 160, 708–725. doi: 10.1104/pp.112.203679
Bédard, J., and Jarvis, P. (2005). Recognition and envelope translocation of chloroplast preproteins. J. Exp. Bot. 56, 2287–2320. doi: 10.1093/jxb/eri243
Bennoun, P. (1982). Evidence for a respiratory chain in the chloroplast. Proc. Natl. Acad. Sci. U.S.A. 79, 4352–4356. doi: 10.1073/pnas.79.14.4352
Beyer, P., Nievelstein, V., Albabili, S., Bonk, M., and Kleinig, H. (1994). Biochemical aspects of carotene desaturation and cyclization in chromoplast membranes from Narcissus pseudonarcissus. Pure Appl. Chem. 66, 1047–1056. doi: 10.1351/pac199466051047
Burrows, P. A., Sazanov, L. A., Svab, Z., Maliga, P., and Nixon, P. J. (1998). Identification of a functional respiratory complex in chloroplasts through analysis of tobacco mutants containing disrupted plastid ndh genes. EMBO J. 17, 868–876. doi: 10.1093/emboj/17.4.868
Camara, B., Hugueney, P., Bouvier, F., Kuntz, M., and Monéger, R. (1995). Biochemistry and molecular biology of chromoplast development. Int. Rev. Cytol. 163, 175–247. doi: 10.1016/S0074-7696(08)62211-1
Carol, P., Stevenson, D., Bisanz, C., Breitenbach, J., Sandmann, G., Mache, R., et al. (1999). Mutations in the Arabidopsis gene IMMUTANS cause a variegated phenotype by inactivating a chloroplast terminal oxidase associated with phytoene desaturation. Plant Cell 11, 57–68. doi: 10.1105/tpc.11.1.57
Carrie, C., Murcha, M. W., Kuehn, K., Duncan, O., Barthet, M., Smith, P. M., et al. (2008). Type II NAD(P)H dehydrogenases are targeted to mitochondria and chloroplasts or peroxisomes in Arabidopsis thaliana. FEBS Lett. 582, 3073–3079. doi: 10.1016/j.febslet.2008.07.061
Catala, R., Sabater, B., and Guera, A. (1997). Expression of the plastid ndhF gene product in photosynthetic and non-photosynthetic tissues of developing barley seedlings. Plant Cell Physiol. 38, 1382–1388. doi: 10.1093/oxfordjournals.pcp.a029133
Cournac, L., Redding, K., Ravenel, J., Rumeau, D., Josse, E. M., Kuntz, M., et al. (2000). Electron flow between photosystem II and oxygen in chloroplasts of photosystem I-deficient algae is mediated by a quinol oxidase involved in chlororespiration. J. Biol. Chem. 275, 17256–17262. doi: 10.1074/jbc.M908732199
Desplats, C., Mus, F., Cuiné, S., Billon, E., Cournac, L., and Peltier, G. (2009). Characterization of Nda2, a plastoquinone-reducing type II NAD(P)H dehydrogenase in Chlamydomonas chloroplasts. J. Biol. Chem. 284, 4148–4157. doi: 10.1074/jbc.M804546200
Dupont, F. M. (2008). Metabolic pathways of the wheat (Triticum aestivum) endosperm amyloplast revealed by proteomics. BMC Plant Biol. 8:39. doi: 10.1186/1471-2229-8-39
Endo, T., Shikanai, T., Sato, F., and Asada, K. (1998). NAD(P)H dehydrogenase-dependent, antimycin A-sensitive electron donation to plastoquinone in tobacco chloroplasts. Plant Cell Physiol. 39, 1226–1231. doi: 10.1093/oxfordjournals.pcp.a029324
Fischer, M., Fun, K. E., and Steinmuller, K. (1997). The expression of subunits of the mitochondrial complex I-homologous NAD(P)H-plastoquinone-oxidoreductase during plastid development. Z. Naturforsch. C 52, 481–486.
Foudree, A., Putarjunan, A., Kambakam, S., Nolan, T., Fussell, J., Pogorelko, G., et al. (2012). The mechanism of variegation in immutans provides insight into chloroplast biogenesis. Front. Plant Sci. 3:260. doi: 10.3389/fpls.2012.00260
Gould, S. B., Waller, R. F., and McFadden, G. I. (2008). Plastid evolution. Annu. Rev. Plant Biol. 59, 491–517. doi: 10.1146/annurev.arplant.59.032607.092915
Guera, A., de Nova, P. G., and Sabater, B. (2000). Identification of the Ndh (NAD(P)H-plastoquinone-oxidoreductase) complex in etioplast membranes of barley: changes during photomorphogenesis of chloroplasts. Plant Cell Physiol. 41, 49–59. doi: 10.1093/pcp/41.1.49
Hart, S., Schlarb-Ridley, B., Bendall, D., and Howe, C. (2005). Terminal oxidases of cyanobacteria. Biochem. Soc. Trans. 33, 832–835. doi: 10.1042/BST0330832
Heyno, E., Gross, C. M., Laureau, C., Culcasi, M., Pietri, S., and Krieger-Liszkay, A. (2009). Plastid alternative oxidase (PTOX) promotes oxidative stress when overexpressed in tobacco. J. Biol. Chem. 284, 31174–31180. doi: 10.1074/jbc.M109.021667
Ho, K. K., Kerfeld, C. A., and Krogmann, D. W. (2011). “The water-soluble cytochromes of cyanobacteria,” in Bioenergetic Processes in Cyanobacteria, eds G. A. Peschek, C. Obinger, and G. Renger (Dordrecht: Springer Science + Business media), 515–540.
Joët, T., Genty, B., Josse, E. M., Kuntz, M., Cournac, L., and Peltier, G. (2002). Involvement of a plastid terminal oxidase in plastoquinone oxidation as evidenced by expression of the Arabidopsis thaliana enzyme in tobacco. J. Biol. Chem. 277, 31623–31630. doi: 10.1074/jbc.M203538200
Josse, E. M., Simkin, A. J., Gaffé, J., Labouré, A. M., Kuntz, M., and Carol, P. (2000). A plastid terminal oxidase associated with carotenoid desaturation during chromoplast differentiation. Plant Physiol. 123, 1427–1436. doi: 10.1104/pp.123.4.1427
Kahlau, S., and Bock, R. (2008). Plastid transcriptomics and translatomics of tomato fruit development and chloroplast-to-chromoplast differentiation: chromoplast gene expression largely serves the production of a single protein. Plant Cell 20, 856–874. doi: 10.1105/tpc.107.055202
Kanervo, E., Singh, M., Suorsa, M., Paakkarinen, V., Aro, E., Battchikova, N., et al. (2008). Expression of protein complexes and individual proteins upon transition of etioplasts to chloroplasts in pea (Pisum sativum). Plant Cell Physiol. 49, 396–410. doi: 10.1093/pcp/pcn016
Karcher, D., and Bock, R. (2002). The amino acid sequence of a plastid protein is developmentally regulated by RNA editing. J. Biol. Chem. 277, 5570–5574. doi: 10.1074/jbc.M107074200
Kohzuma, K., Dal Bosco, C., Kanazawa, A., Dhingra, A., Nitschke, W., Meurer, J., et al. (2012). Thioredoxin-insensitive plastid ATP synthase that performs moonlighting functions. Proc. Natl. Acad. Sci. U.S.A. 109, 3293–3298. doi: 10.1073/pnas.1115728109
Laureau, C., de Paepe, R., Latouche, G., Moreno-Chacón, M., Finazzi, G., Kuntz, M., et al. (2013). Plastid terminal oxidase (PTOX) has the potential to act as a safety valve for excess excitation energy in the alpine plant species Ranunculus glacialis L. Plant Cell Environ. 36, 1296–1310. doi: 10.1111/pce.12059
Lennon, A. M., Prommeenate, P., and Nixon, P. J. (2003). Location, expression and orientation of the putative chlororespiratory enzymes, Ndh and IMMUTANS, in higher-plant plastids. Planta 218, 254–260. doi: 10.1007/s00425-003-1111-7
Li, L., and Yuan, H. (2013). Chromoplast biogenesis and carotenoid accumulation. Arch. Biochem. Biophys. 539, 102–109. doi: 10.1016/j.abb.2013.07.002
Mayer, M. P., Nievelstein, V., and Beyer, P. (1992). Purification and characterization of a NADPH dependent oxidoreductase from chromoplasts of Narcissus pseudonarcissus: a redox-mediator possibly involved in carotene desaturation. Plant Physiol. Biochem. 30, 389–398.
McDonald, A. E., Ivanov, A. G., Bode, R., Maxwell, D. P., Rodermel, S. R., and Hüner, N. P. A. (2011). Flexibility in photosynthetic electron transport: the physiological role of plastoquinol terminal oxidase (PTOX). Biochim. Biophys. Acta 1807, 954–967. doi: 10.1016/j.bbabio.2010.10.024
Morstadt, L., Gräber, P., Pascalis, L., Kleining, H., Speth, V., and Beyer, P. (2002). Chemiosmotic ATP synthesis in photosynthetically inactive chromoplasts from Narcissus pseudonarcissus L. linked to a redox pathway potentially also involved in carotene desaturation. Planta 215, 134–140. doi: 10.1007/s00425-001-0724-y
Nashilevitz, S., Melamed-Bessudo, C., Izkovich, Y., Rogachev, I., Osorio, S., Itkin, M., et al. (2010). An orange ripening mutant links plastid NAD(P)H dehydrogenase complex activity to central and specialized metabolism during tomato fruit maturation. Plant Cell 22, 1977–97. doi: 10.1105/tpc.110.074716
Nawrocki, W. J., Tourasse, N. J., Taly, A., and Rappaport, F. (2015). The plastid terminal oxidase: its elusive function points to multiple contributions to plastid physiology. Annu. Rev. Plant Biol. 66, 1–26. doi: 10.1146/annurev-arplant-043014-114744
Nievelstein, V., Vandekerckhove, J., Tadros, M. H., Lintig, J. V., Nitschke, W., and Beyer, P. (1995). Carotene desaturation is linked to a respiratory redox pathway in Narcissus pseudonarcissus chromoplast membranes. Involvement of a 23-kDa oxygen-evolving-complex-like protein. Eur. J. Biochem. 233, 864–872. doi: 10.1111/j.1432-1033.1995.864_3.x
Nixon, P. J., and Rich, P. R. (2006). “Chlororespiratory pathways and their physiological significance,” in The Structure and Function of Plastids, eds R. R. Wise and J. K. Hoober (Dordrecht: Springer), 237–248.
Norris, S. R., Barrette, T. R., and DellaPenna, D. (1995). Genetic dissection of carotenoid synthesis in Arabidopsis defines plastoquinone as an essential component of phytoene desaturation. Plant Cell 7, 2139–2149. doi: 10.1105/tpc.7.12.2139
Paredes, M., and Quiles, M. J. (2013). Stimulation of chlororespiration by drought under heat and high illumination in Rosa meillandina. J. Plant Physiol. 170, 165–171. doi: 10.1016/j.jplph.2012.09.010
Pateraki, I., Renato, M., Azcón-Bieto, J., and Boronat, A. (2013). An ATP synthase harboring an atypical γ-subunit is involved in ATP synthesis in tomato fruit chromoplasts. Plant J. 74, 74–85. doi: 10.1111/tpj.12109
Peltier, G., and Cournac, L. (2002). Chlororespiration. Annu. Rev. Plant Biol. 53, 523–550. doi: 10.1146/annurev.arplant.53.100301.135242
Peng, L., and Shikanai, T. (2011). Supercomplex formation with photosystem I is required for the stabilization of the chloroplast NADH dehydrogenase-like complex in Arabidopsis. Plant Physiol. 155, 1629–1639. doi: 10.1104/pp.110.171264
Peng, L., Shimizu, H., and Shikanai, T. (2008). The chloroplast NAD(P)H dehydrogenase complex interacts with photosystem I in Arabidopsis. J. Biol. Chem. 283, 34873–34879. doi: 10.1074/jbc.M803207200
Plöscher, M., Reisinger, V., and Eichacker, L. A. (2011). Proteomic comparison of etioplast and chloroplast protein complexes. J. Proteomics 74, 1256–1265. doi: 10.1016/j.jprot.2011.03.020
Reisinger, V., Hertle, A. P., Plöscher, M., and Eichacker, L. A. (2008). Cytochrome b6f is a dimeric protochlorophyll a binding complex in etioplasts. FEBS J. 275, 1018–1024. doi: 10.1111/j.1742-4658.2008.06268.x
Renato, M., Pateraki, I., Boronat, A., and Azcón-Bieto, J. (2014). Tomato fruit chromoplasts behave as respiratory bioenergetic organelles during ripening. Plant Physiol. 166, 920–933. doi: 10.1104/pp.114.243931
Rodríguez-Villalón, A., Gas, E., and Rodríguez-Concepción, M. (2009). Colors in the dark: a model for the regulation of carotenoid biosynthesis in etioplasts. Plant Signal. Behav. 4, 965–967. doi: 10.4161/psb.4.10.9672
Rosso, D., Ivanov, A. G., Fu, A., Geisler-Lee, J., Hendrickson, L., Geisler, M., et al. (2006). IMMUTANS does not act as a stress-induced safety valve in the protection of the photosynthetic apparatus of Arabidopsis during steady-state photosynthesis. Plant Physiol. 142, 574–585. doi: 10.1104/pp.106.085886
Rumeau, D., Bécuwe-Linka, N., Beyly, A., Louwagie, M., Garin, J., and Peltier, G. (2005). New subunits NDH-M, -N, and -O, encoded by nuclear genes, are essential for plastid Ndh complex functioning in higher plants. Plant Cell 17, 219–232. doi: 10.1105/tpc.104.028282
Rumeau, D., Peltier, G., and Cournac, L. (2007). Chlororespiration and cyclic electron flow around PSI during photosynthesis and plant stress response. Plant Cell Environ. 30, 1041–1051. doi: 10.1111/j.1365-3040.2007.01675.x
Samra, H. S., Gao, F., He, F., Hoang, E., Chen, Z., Gegenheimer, P. A., et al. (2006). Structural analysis of the regulatory dithiol-containing domain of the chloroplast ATP synthase γ subunit. J. Biol. Chem. 281, 31041–31049. doi: 10.1074/jbc.M603315200
Sazanov, L. A., Burrows, P. A., and Nixon, P. J. (1998). The chloroplast Ndh complex mediates the dark reduction of the plastoquinone pool in response to heat stress in tobacco leaves. FEBS Lett. 429, 115–118. doi: 10.1016/S0014-5793(98)00573-0
Shahbazi, M., Gilbert, M., Labouré, A. M., and Kuntz, M. (2007). Dual role of the plastid terminal oxidase in tomato. Plant Physiol. 145, 691–702. doi: 10.1104/pp.107.106336
Siddique, M. A., Grossmann, J., Gruissem, W., and Baginsky, S. (2006). Proteome analysis of bell pepper (Capsicum annuum L.) chromoplasts. Plant Cell Physiol. 47, 1663–1673. doi: 10.1093/pcp/pcl033
Trouillard, M., Shahbazi, M., Moyet, L., Rappaport, F., Joliot, P., Kuntz, M., et al. (2012). Kinetic properties and physiological role of the plastoquinone terminal oxidase (PTOX) in a vascular plant. Biochim. Biophys. Acta 1817, 2140–2148. doi: 10.1016/j.bbabio.2012.08.006
Wang, Y. Q., Yang, Y., Fei, Z., Yuan, H., Fish, T., Thannhauser, T. W., et al. (2013). Proteomic analysis of chromoplasts from six crop species reveals insights into chromoplast function and development. J. Exp. Bot. 64, 949–961. doi: 10.1093/jxb/ers375
Wastl, J., Bendall, D. S., and Howe, C. J. (2002). Higher plants contain a modified cytochrome c6. Trends Plant Sci. 7, 244–245. doi: 10.1016/s1360-1385(02)02280-x
Whatley, J. M. (1978). A suggested cycle of plastid developmental interrelationships. New Phytol. 80, 489–502. doi: 10.1111/j.1469-8137.1978.tb01581.x
Wu, D., Wright, D. A., Wetzel, C., Voytas, D. F., and Rodermel, S. (1999). The IMMUTANS variegation locus of Arabidopsis defines a mitochondrial alternative oxidase homolog that functions during early chloroplast biogenesis. Plant Cell 11, 43–55. doi: 10.2307/3870837
Yu, Q., Feilke, K., Krieger-Liszkay, A., and Beyer, P. (2014). Functional and molecular characterization of plastid terminal oxidase from rice (Oryza sativa). Biochim. Biophys. Acta. 1837, 1284–1292. doi: 10.1016/j.bbabio.2014.04.007
Zeng, Y., Pan, Z., Ding, Y., Zhu, A., Cao, H., Xu, Q., et al. (2011). A proteomic analysis of the chromoplasts isolated from sweet orange fruits [Citrus sinensis (L.) Osbeck]. J. Exp. Bot. 62, 5297–5309. doi: 10.1093/jxb/err140
Keywords: plastid, chromoplast, etioplast, amyloplast, chlororespiration, chromorespiration, PTOX, plastid respiration
Citation: Renato M, Boronat A and Azcón-Bieto J (2015) Respiratory processes in non-photosynthetic plastids. Front. Plant Sci. 6:496. doi: 10.3389/fpls.2015.00496
Received: 31 March 2015; Accepted: 22 June 2015;
Published: 17 July 2015.
Edited by:
Richard Sayre, New Mexico Consortium at Los Alamos National Labs, USACopyright © 2015 Renato, Boronat and Azcón-Bieto. This is an open-access article distributed under the terms of the Creative Commons Attribution License (CC BY). The use, distribution or reproduction in other forums is permitted, provided the original author(s) or licensor are credited and that the original publication in this journal is cited, in accordance with accepted academic practice. No use, distribution or reproduction is permitted which does not comply with these terms.
*Correspondence: Joaquín Azcón-Bieto, Departament de Biologia Vegetal, Facultat de Biologia, Universitat de Barcelona, Avinguda Diagonal 643, Barcelona 08028, Spain,amF6Y29uQHViLmVkdQ==