- Key Laboratory of Crop Gene Resources and Germplasm Enhancement, Ministry of Agriculture, The National Key Facility for Crop Gene Resources and Genetic Improvement, Institute of Crop Science, Chinese Academy of Agricultural Sciences, Beijing, China
NAC transcription factors play diverse roles in plant development and responses to abiotic stresses. However, the biological roles of NAC family members in wheat are not well understood. Here, we reported the isolation and functional characterization of a novel wheat TaNAC47 gene. TaNAC47 encoded protein, localizing in the nucleus, is able to bind to the ABRE cis-element and transactivate transcription in yeast, suggesting that it likely functions as a transcriptional activator. We also showed that TaNAC47 is differentially expressed in different tissues, and its expression was induced by the stress treatments of salt, cold, polyethylene glycol and exogenous abscisic acid. Furthermore, overexpression of TaNAC47 in Arabidopsis resulted in ABA hypersensitivity and enhancing tolerance of transgenic plants to drought, salt, and freezing stresses. Strikingly, overexpression of TaNAC47 was found to activate the expression of downstream genes and change several physiological indices that may enable transgenic plants to overcome unfavorable environments. Taken together, these results uncovered an important role of wheat TaNAC47 gene in response to ABA and abiotic stresses.
Introduction
Abiotic stress is one of the main factors influencing growth, development and yield of plants worldwide. The exploitation and utilization of stress-tolerant plants will become more significant in the saline-alkali land and water shortage areas. Recently, progress has been made in identifying beneficial stress-related genes that can enhance the tolerance of plants to abiotic stresses (Tran et al., 2010; Nakashima et al., 2012). Transcription factors (TFs) are pivotal regulators involving in the response to abiotic stress, and overexpression of TF genes commonly improved a plant’s tolerance to abiotic stress. The NAC protein forms one of the largest families of plant-specific TFs (Olsen et al., 2005). They were derived from three genes containing particular domains of NAM (no apical meristem), ATAF (Arabidopsis transcription activation factor) and CUC (cup-shaped cotyledon) (Souer et al., 1996; Aida et al., 1997). Typically, a NAC transcription factor harbors a highly conserved N-terminal NAC domain and a variable C-terminal transcription regulatory (TR) region (Ernst et al., 2004; Olsen et al., 2005). The nearly invariable N-terminal NAC domain is responsible for nuclear localization, DNA binding, and formation of homodimers or heterodimers. This domain contains approximately 150 amino acids and is classified as an A-E subdomain (Olsen et al., 2005). In contrast, the C-terminal region is diverse and can function as a transcriptional activator or repressor (Delessert et al., 2005; Kim et al., 2007; Yamaguchi et al., 2010; Puranik et al., 2011).
Numerous reports have demonstrated that NAC TFs are involved in a number of biological processes, such as controlling cell division by mediating cytokinin signaling (Kim et al., 2006), regulating the growth of plant cells (Kato et al., 2010), lateral root development (He et al., 2005; Quach et al., 2014), and leaf senescence (Guo and Gan, 2006; Yang et al., 2011; Shah et al., 2013); inducing phytoalexin biosynthesis (Saga et al., 2012), formation of secondary walls (Mitsuda et al., 2007; Zhao et al., 2010), flower formation (Hendelman et al., 2013) and responding to pathogen infection (Voitsik et al., 2013; Yokotani et al., 2014), seed development (Park et al., 2011) and fiber development (Zhao et al., 2014). Additionally, many members of the NAC TF family can coordinate the response to abiotic stress. In rice, expressions of OsNAC5, OsNAC6, OsNAP, and SNAC1 were induced by drought, cold and high salinity. Overexpression of these four genes in transgenic rice improved the tolerance to high salinity and dehydration. Wheat plants expressing the SNAC1 gene exhibited increased tolerance to drought and salinity (Nakashima et al., 2007; Takasaki et al., 2010; Saad et al., 2013; Chen et al., 2014). Similarly, transgenic rice overexpressing OsNAC045 exhibited enhanced resistance to both drought and salt stresses (Zheng et al., 2009). Root-specific overexpression of the OsNAC9 and OsNAC10 genes resulted in enlarged roots and enhanced the drought tolerance of transgenic rice that led to significantly increasing the grain yield under field drought conditions (Jeong et al., 2010; Redillas et al., 2012). Recently, the characterization of the roles of stress-related NAC transcription factors has been reported in wheat. Two genes, TaNAC4 and TaNAC8, were involved in stripe rust pathogen infection and abiotic stresses (Xia et al., 2010a,b). TaNAC69 expression was up-regulated by multiple abiotic stresses in wheat, and overexpression of TaNAC69 in transgenic wheat enhanced the expression levels of stress up-regulated genes and dehydration tolerance (Xue et al., 2011). Moreover, overexpression of TaNAC2, TaNAC2a and TaNAC67 improves the tolerance of transgenic plants to abiotic stresses (Tang et al., 2012; Mao et al., 2012, 2014).
To interpret the possible molecular regulatory mechanisms underlying the plant response to abiotic stress and accelerate the use of the NAC gene to facilitate engineering transgenic wheat, we characterized an abiotic stress-related gene, TaNAC47, from a full-length wheat cDNA library. Its expression profiles in response to polyethylene glycol (PEG), salt, cold, and exogenous ABA treatments were examined in wheat by using the quantitative real-time PCR (RT-qPCR) approach. Its function in abiotic stresses tolerance was evaluated by ectopic expression of TaNAC47 in Arabidopsis. There were no obvious morphological differences between the transgenic and WT Arabidopsis plants under normal growth conditions. Together, results collected in this study indicated that TaNAC47 is likely a candidate gene that will be useful for improving stress tolerance.
Materials and Methods
Plant Materials and Abiotic Stress Treatments
For expression analysis of TaNAC47, young spikes, leaves, stems and roots were sampled from Chinese Spring (CS) wheat. The wheat cv. Hanxuan 10 (drought resistant), Chadianhong (salt resistant) and CS were subjected to drought, salt, low temperature and exogenous ABA treatments. Wheat seeds were germinated and grown with distilled water at 25°C under a 16 h light/8 h dark cycle. Ten-day-old seedlings were treated with 16.1% PEG-6000 (-0.5 MPa), 250 mM NaCl, and 200 μM ABA at 4°C for 0, 1, 3, 6, 12, 24, and 48 h. All of the treated samples were immediately frozen with liquid nitrogen and stored at -80°C for RNA isolation. Arabidopsis thaliana Columbia-0 was used for transgenic study of TaNAC47. CS wheat was used to analyze the genomic sequence of the gene.
Genomic Sequence Isolation and Analysis of TaNAC47
The TaNAC47 cDNA sequence was obtained by sequencing the cDNA plasmid libraries in our laboratory. Gene-specific primers (forward 5′- CCAATGAAGATGAACCCC -3′ and reverse 5′-AATGCTACTGTGAGAGAG-3′) were designed to perform genomic sequencing in CS wheat. The PCR products were cloned into the vector of pEASY-T1 and sequenced. The sequence alignment between the cDNA and the genomic DNA of the gene was used to analyze the exons and introns of the genomic DNA. The theoretical molecular weight and isoelectric point were calculated using ExPASy1. The NAC domain region was identified with SCANPROSITE2.
Cloning of the Promoter Sequences and Analysis of Cis-acting Element
The promoter sequence of TaNAC47 was obtained by using TaNAC47 genomic DNA as the query sequences to blast Ae. tauschii genome sequence database (Jia et al., 2013). The 1,500 bp of sequence upstream from the initiation codon (ATG) of TaNAC47 was used to analyze the cis-acting elements by using the PLACE database3.
Subcellular Localization of the TaNAC47 Protein
The full-length coding sequence of TaNAC47 was fused to the modified pEarleyGate-GFP vector under the control of the cauliflower mosaic virus (CaMV) 35S promoter to generate the 35S::GFP-TaNAC47 fusion construct by the gateway method. The 35S::GFP-TaNAC47 fusion protein and 35S::GFP alone were introduced into epidermal cells of N. benthamiana separately via an Agrobacterium-mediated method (Sheludko et al., 2007). The transformed N. benthamiana leaves were grown under the normal conditions for 2-6 days. The signals were observed and photographed using confocal laser scanning microscopy (Zeiss Lsm 700, Zeiss, Jena, Germany).
Transcription Activation Assay in Yeast
To investigate the transcriptional activity of TaNAC47 protein, the full-length coding sequence of TaNAC47 was amplified using a pair of gene-specific primers containing the attB sites (forward primer 5′ -GGGGACAAGTTTGTACAAAAAAGCAGGCTTAATGGTGATGGCGGCGGCG-3′ and reverse primer 5′-GGGG ACCACTTTGTACAAGAAAGCTGGGTTCAGAAGAAGAATGGGCTGA-3′; attB sites underlined) and then fused with the DNA-binding domain (BD) in a pDEST32 vector. The fused construct pDEST32-TaNAC47, pGAL4 and the pDEST32 vector were transformed into the yeast strain AH109 separately. The transformants were plated on the medium without histidine, leucine, and adenine for the selection of transactivation properties of the reporter constructs.
Yeast One-Hybrid Assays
Full-length TaNAC47 gene was fused in frame with the GAL4 DNA-activation domain (AD) in a vector pDEST22. The bait construct containing the hexamer ABRE/mABRE sequence was provided by Professor Jun Zhao (Biotechnology Research Institute, Chinese Academy of Agricultural Sciences, Beijing, China). The recombinant plasmid pDEST22-TaNAC47 and the ABRE bait plasmid were co-transformed into the yeast strain YM4271 using the Frozen-EZ yeast transformation method (Zymo Research, Irvine, CA, USA). Two different combinations, (i) GAL4-AD-TaNAC47 and mutated forms of ABRE sequence (mABRE); (ii) the GAL4-AD and ABRE sequence were developed, along with GAL4-AD and mABRE used as control were transformed into the yeast strain YM4271 separately. The yeast transformants were cultured on medium of SD/Leu- Trp- at 30°C for about 2 days, and the colonies were then dropped onto the medium of SD/Leu- Trp- His- containing 0.5 mM 3-AT.
Quantitative RT-PCR Analysis
Total RNA extraction and reverse transcription were performed using the TRIZOL reagent and SuperScriptTMII reverse transcriptase (Invitrogen, Carlsbad, CA, USA). The RT-qPCR reaction mix included 5 μL of SYBR Premix EX TaqTM (Takara, Shiga, Japan), 2 μL of 2 μM of each primer, 2 μL of cDNA and distilled water to a final volume of 20 μL, and amplified for 40 cycles with each cycle consisting of 20 s at 95°C; 20 s at 55°C; and 30 s at 72°C on the Applied Biosystems 7500 real time RT-qPCR instrument (Applied Biosystems, Foster City, CA, USA). Wheat Tubulin gene (NCBI accession No. AF251217.1) and Arabidopsis Atactin gene (NCBI accession No. NM_112764) were used as the internal reference, and the 2-ΔΔCT method was used to perform the quantitative analysis. All RT-qPCR reactions data were obtained from three independent experiments. The primers used for the RT-qPCR were listed in Supplementary Table S2.
Generation of TaNAC47 Transgenic Arabidopsis Plants
The full-length coding sequence of TaNAC47 was introduced into the pEarleyGate 100 vector to generate the 35S::TaNAC47 construct. The construct was transformed into the Agrobacterium tumefaciens strain GV3101 (90RK), and then delivered into A. thaliana ecotype Col-0 through the floral dip method (Clough and Bent, 1998). The positive transgenic plants were screened by spraying a 0.002% (v/v) Basta solution and then confirmed by RT-PCR. T3 lines exhibiting 100% Basta resistance were considered to be homozygous and selected for further experiments.
Analysis of Abiotic Stress Tolerance and ABA Sensitivity in Transgenic Arabidopsis Plants
For determining the salt tolerance in transgenic plants, five-day-old seedlings grown vertically on MS plates were moved to new plates with addition of different concentrations of NaCl (0, 150, and 200 mM) and continued for 5 days. The root lengths of the seedlings were recorded.
For freezing tolerance test, 2-week-old transgenic and WT Arabidopsis seedlings were grown under a normal condition and then subjected to -10°C cold treatment for 3 h. Seedlings were then placed at 5°C for 2 h before transferring to a normal condition at 22°C.
To study drought tolerance of transgenic Arabidopsis, ten seedlings were grown in rectangular plates (4 cm deep) with a mixture of vermiculite and humus, and kept well-watered. The seedlings were then cultivated in a phytotron chamber without watering for about 35 days, followed by rehydrating the seedlings for 5 days. The survival rates of transgenic and WT seedlings were statistical analyzed.
For analyzing the sensitivity of transgenic seedlings to ABA, transgenic and WT seedlings grown on the MS plates for 5 days were transferred to new MS plates containing different concentrations of ABA and continued for 7 days. The root lengths of the seedlings were measured. All of the experiments described above were repeated three times.
Measurements of Relative Electrolyte Leakage, Soluble Sugar, Proline Content, and Water Loss Rate
For determining the water loss rate, leaves were harvested from 3-week-old seedlings of transgenic and WT plants and weighed immediately. The leaves were then placed on the lab bench (20-22°C, humidity 45-60%) and weighed at designated time points. The water loss rate was calculated related to the initial fresh weight of the samples. Each measurement was performed in triplicate.
The free proline concentrations were measured according to previously described method (Zhang et al., 2012). The electrolyte leakage and soluble sugar content were evaluated following the method described previously (Lehner et al., 2006; Cao et al., 2007).
All the measurements were performed with ten plants in triplicate, and Student’s t-test was performed for statistical analysis.
Results
Sequence Characterization of the TaNAC47 Gene
The full-length TaNAC47 cDNA was 1,277 bp and was predicted to encode a 271-amino acid protein harboring a molecular weight of 32.37 kD with a pI of 5.13. The deduced TaNAC47 protein contained a conserved NAC domain (19-169 aa). The TaNAC47 gene sequence was submitted to GenBank with the accession number KT345698. Results from blast analysis revealed that TaNAC47 shared 87.6% identity to another wheat abiotic stressed responsive NAC protein TaNAC67.
To analyze the gene structure of TaNAC47, the genomic sequence was cloned from the hexaploid CS wheat. A comparison of the genomic sequence and the corresponding cDNA sequence of TaNAC47 revealed that the TaNAC47 gene did not possess any intron.
Analysis of cis-acting regulatory element in the promoter region of TaNAC47 revealed the presence of basic components and stress-responsive element-binding motifs, including A-boxes, C-boxes, CAAT-boxes, GATA-boxes, and the abiotic stress response cis-elements (ABRE, DRE/CRT, HSE, and LTRE; Supplementary Table S1). Collectively, the presence of these cis-elements suggested that the TaNAC47 gene may play a role in response to abiotic stresses, most likely via an ABA-dependent pathway.
Expression Pattern of TaNAC47 in Wheat
To elucidate the molecular mechanism underlying the responsiveness to various abiotic stresses, RT-qPCR was performed to investigate the expression patterns of TaNAC47 in different wheat tissues. The TaNAC47 gene transcripts accumulated at the highest level in leaf but at low levels in young spike, stem and root (Supplementary Figure S1). Under cold stress conditions, TaNAC47 expression was increased between 1 and 24 h (Figure 1A). Expression of TaNAC47 was induced during the course of 1-48 h in the presence of exogenous ABA (Figure 1B). An increase in the TaNAC47 transcript level was observed within 1 h of PEG-induced osmotic stress (Figure 1C). TaNAC47 expression levels were also increased in response to NaCl treatment within 12 h, although the transcript level returned to background level afterwards (Figure 1D). These results indicated that gene expression for TaNAC47 was significantly induced by all four abiotic stress treatments, and that TaNAC47 was sensitive to cold, ABA and PEG stresses at an early stage of the treatments.
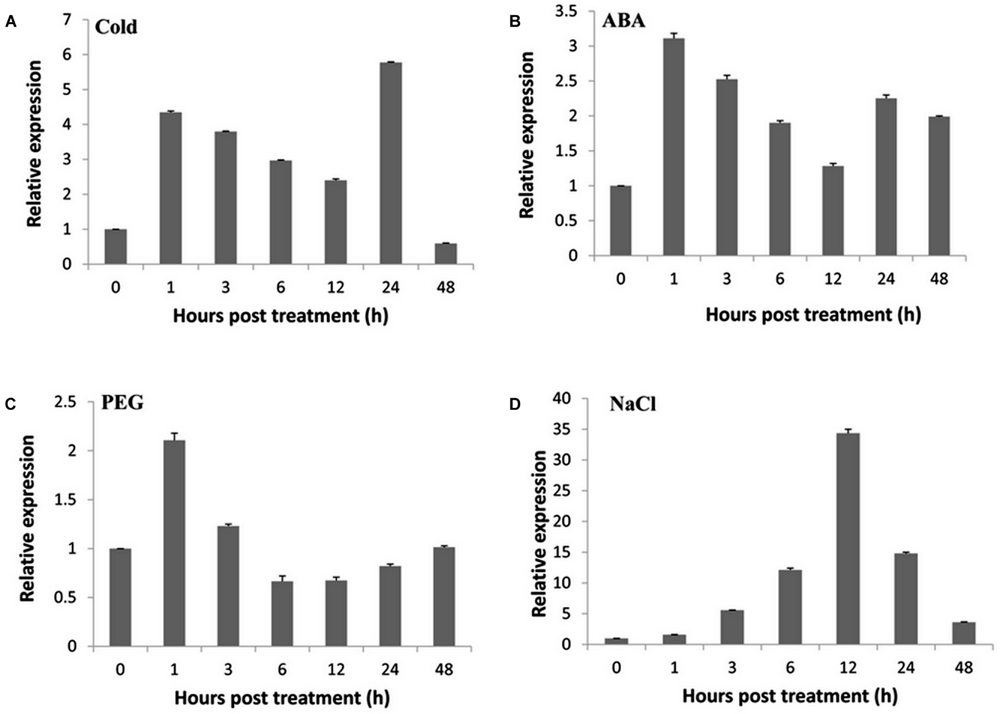
FIGURE 1. Expression of the TaNAC47 gene in wheat. (A) Low-temperature (4°C) treatment; (B) 200 μM ABA treatment; (C) 16.1% PEG6000 treatment; (D) 250 mM NaCl treatment. The y-axis indicates the relative expression levels of the TaNAC47 gene. The data were calculated using the 2-ΔΔCT method. The experiment was performed three times. The bars show the standard errors.
TaNAC47 Encoded Protein Localizes in the Nucleus and Acts as a Transcriptional Activator
To determine the subcellular localization of TaNAC47 encoded protein, the full-length sequence of TaNAC47 was fused to the GFP gene sequence in a pEarleyGate-GFP vector. Recombinant plasmids and pEarleyGate-GFP vector alone were transient expressed in the N. benthamiana leaves separately. Results obtained from confocal microscopy showed that the fluorescence signals from GFP alone were widely distributed throughout the cells, while the green fluorescent signals from transformed cells harboring TaNAC47-GFP were mainly observed in the nuclei (Figure 2). These results implied that the TaNAC47 protein was a nuclear-localized protein.
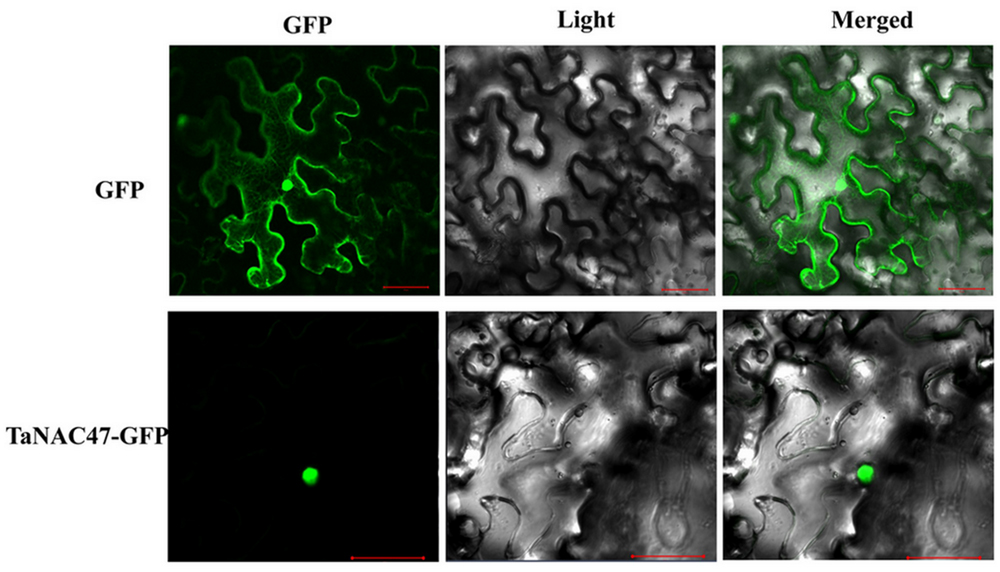
FIGURE 2. Subcellular localization of the TaNAC47 protein in leaf epidermal cells of Nicotiana benthamiana. The p35:TaNAC47-GFP and p35:GFP constructs were separately introduced and expressed instantly in leaf epidermal cells of Nicotiana benthamiana. Bars = 30 μM.
The transactivation assay in yeast showed that all transformants grew well on SD/Leu- medium. While the transformants containing the pDEST32 vector alone did not grow on the transactivation selective SD/Leu-His-Ade- medium, the pDEST32 transformants fused with the ORFs of TaNAC47 and pGAL4 grew on transactivation selective SD/Leu-His-Ade- medium (Figure 3), demonstrating that these transformants were able to activate the transcription of the reporter genes Ade and His in the yeast strain AH109. Therefore, our results provided direct evidence that the TaNAC47 protein has transcriptional activity in yeast.
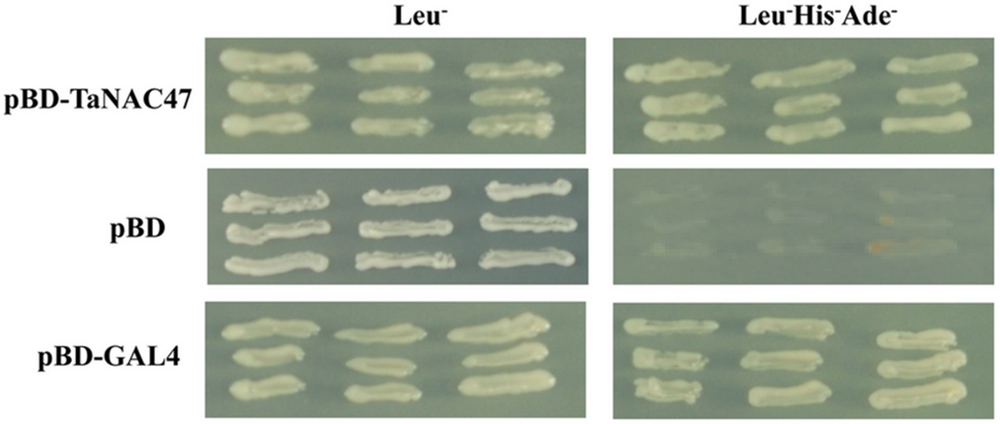
FIGURE 3. Transactivation assay of the TaNAC47 protein in yeast. Full-length TaNAC47 was fused with pDEST32. The pDEST32 vector alone and GAL4 were used as the negative and positive controls, respectively.
Binding of TaNAC47 to the ABRE Cis-acting Element
The ABRE is an important cis-acting element for genes involved in the ABA signaling pathway. To investigate whether TaNAC47 participated in the ABA regulatory pathway, the binding affinity between TaNAC47 and ABRE was assessed by employing an yeast one-hybrid assay. As shown in Figure 4, all the yeast transformants were able to grow on the medium containing SD/Leu-Trp-. When adding 0.5 mM 3-aminotriazole (3-AT) to the medium, only the transformants containing the construct of GAL4-AD-TaNAC47 and the ABRE bait plasmid were able to grow, but the control transformants were not. Therefore, our results strongly suggested that TaNAC47 had a capability to bind to ABRE.
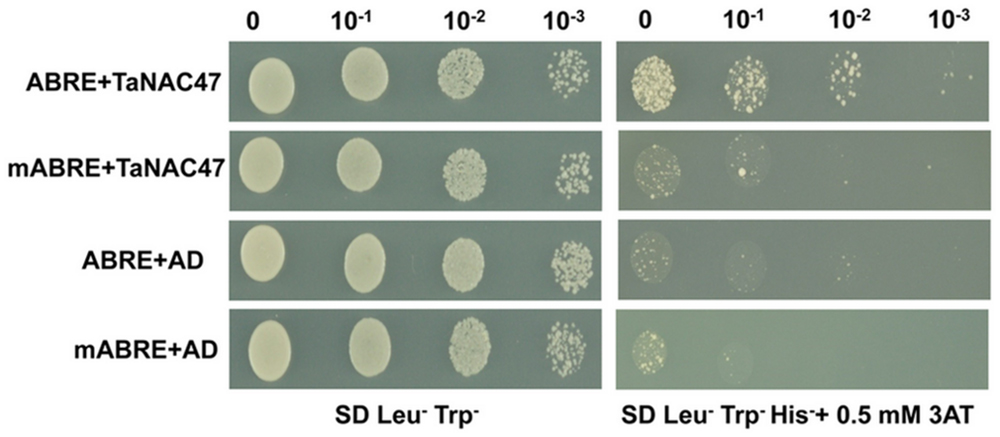
FIGURE 4. The binding activity of TaNAC47 to ABRE element in a yeast one-hybrid system. The hexamer ABRE/mABRE sequence was used as the negative control. Yeast cells grown in liquid medium were diluted in a 10× dilution series. A total of 10 μL of each dilution was spotted onto SD/Leu-Trp-His- medium with 0.5 mM 3-AT.
Overexpression of TaNAC47 in Arabidopsis Increases Tolerance to Multiple Abiotic Stresses and Plant Sensitivity to ABA
To explore the function of TaNAC47 in providing tolerance to abiotic stress in plants, transgenic Arabidopsis plants over-expressing TaNAC47 driven by the CaMV 35S promoter were generated. At least 15 transgenic lines were acquired, and three independent homozygous lines with relatively high expression levels (Supplementary Figure S2) were used for the analysis. For salt tolerance, no obvious difference was observed between WT and transgenic plants under normal conditions. However, leaf growth in WT plants was delayed, their cotyledons turned yellow and some of the cotyledons exhibited albino characteristics in the presence of 150 and 200 mM NaCl. Additionally, root growth remained healthier in transgenic plants than that in WT plants during the time period when raising the NaCl concentration to 150 and 200 mM (Figures 5A,B).
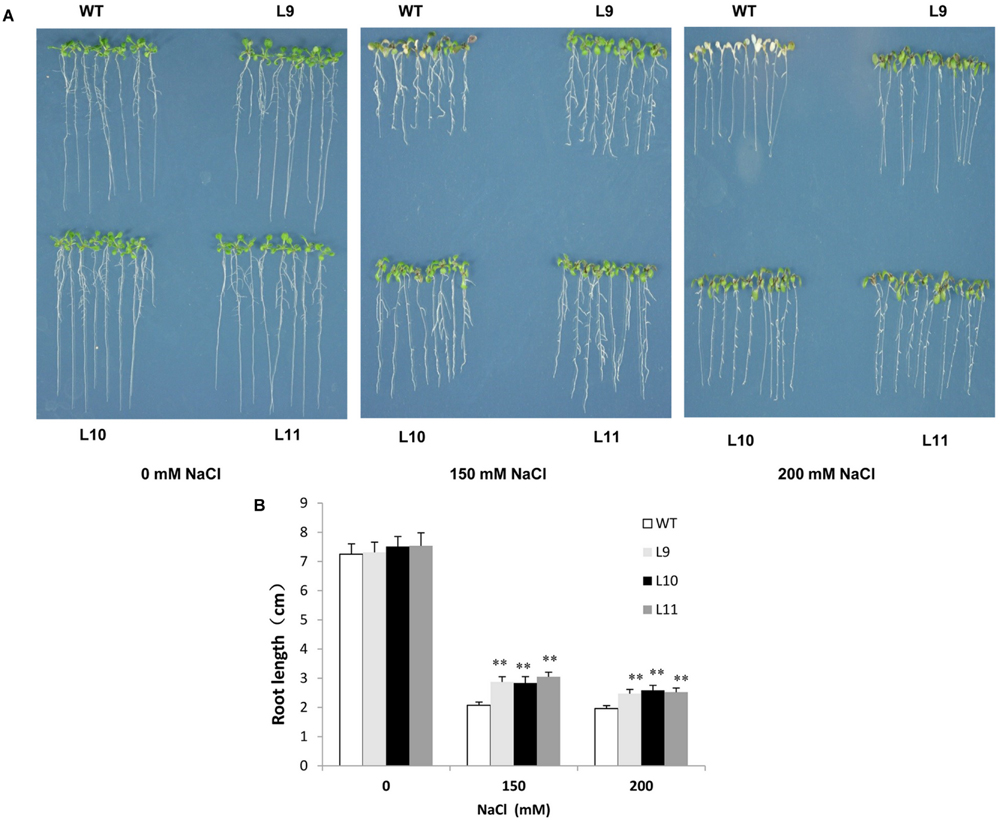
FIGURE 5. Analysis of the enhanced salt tolerance in TaNAC47-overexpressing Arabidopsis seedling lines. (A) Phenotype of WT and transgenic plants on MS with 0, 150, and 200 mM NaCl. (B) Root lengths of plants in (A). The data represent the means from three experiments. The bars show the SD. Significant differences between the transgenic and WT lines are indicated as ∗0.01 < P < 0.05 and ∗∗P < 0.01.
For freezing tolerance, 2-week-old plants were exposed to -10 °C for 3 h and then transferred to normal 22°C growing conditions for recovery. As a result, over half of WT plants were dead with a survival rate at approximately 41%. In contrast, the survival rate for transgenic plants was observed at 81–100% (Figures 6A,B). Additionally, the electrolyte leakage was lower in transgenic plants relative to WT plants when they were at the freezing condition (Figure 6C). Transgenic plants further produced remarkably high levels of proline and soluble sugars under freezing condition compared to WT plants (Figures 6D,E).
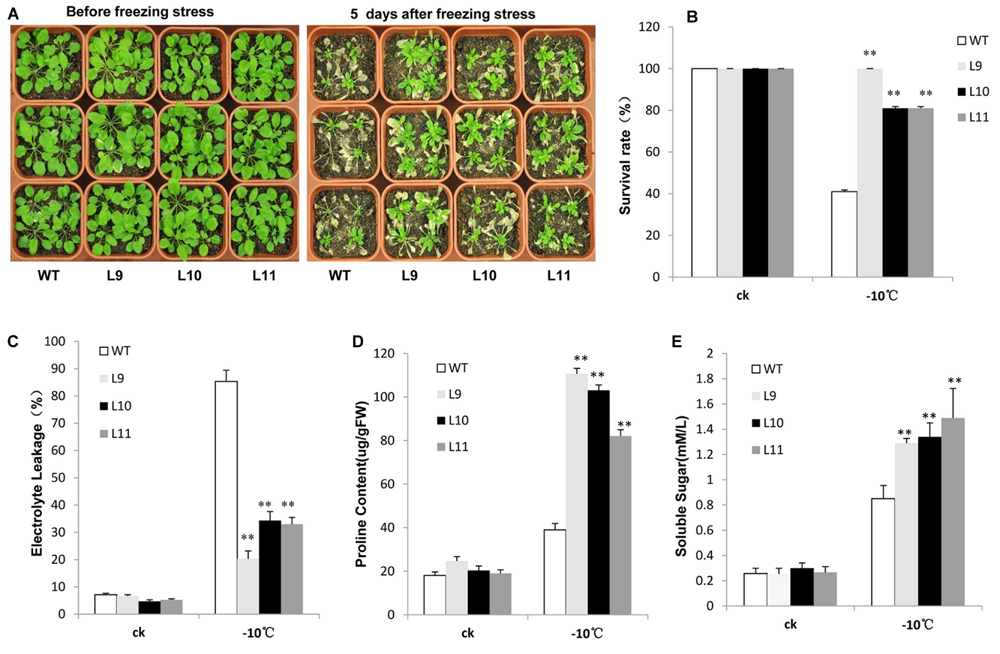
FIGURE 6. Analysis of the enhanced freezing tolerance in TaNAC47-overexpressing Arabidopsis seedling lines. (A) Phenotype of WT and transgenic plants before and after freezing treatments. (B) Survival rate after freezing. (C) Relative electrolyte leakage. (D) Proline content. (E) Soluble sugar content. The data represent the means from three experiments. The bars show the SD. Significant differences between the transgenic and WT lines are indicated as ∗0.01 < P < 0.05 and ∗∗P < 0.01.
The drought tolerance study was evaluated in soils. Soon after irrigation was suspended, no discernible developmental differences were observed between transgenic and WT plants. However, after withholding water for 35 days, WT plants showed visible symptoms of drought-induced damage, such as drying, wilting, and even death. In contrast, some transgenic plants remained green with expanded leaves. Further analyses showed that after re-watering the survival rate was significantly different between transgenic and WT plants. Few WT plants survived, whereas about 10–51% of transgenic plants continued to grow (Figures 7A,B). When plants were grown under drought stress condition, the electrolyte leakage was lower in transgenic plants than in WT plants (Figure 7C). Transgenic plants further showed remarkably higher levels of proline and soluble sugars relative to those found in WT plants (Figures 7D,E). The water-loss rates were also lower in transgenic plants than in WT plants (Figure 7F).
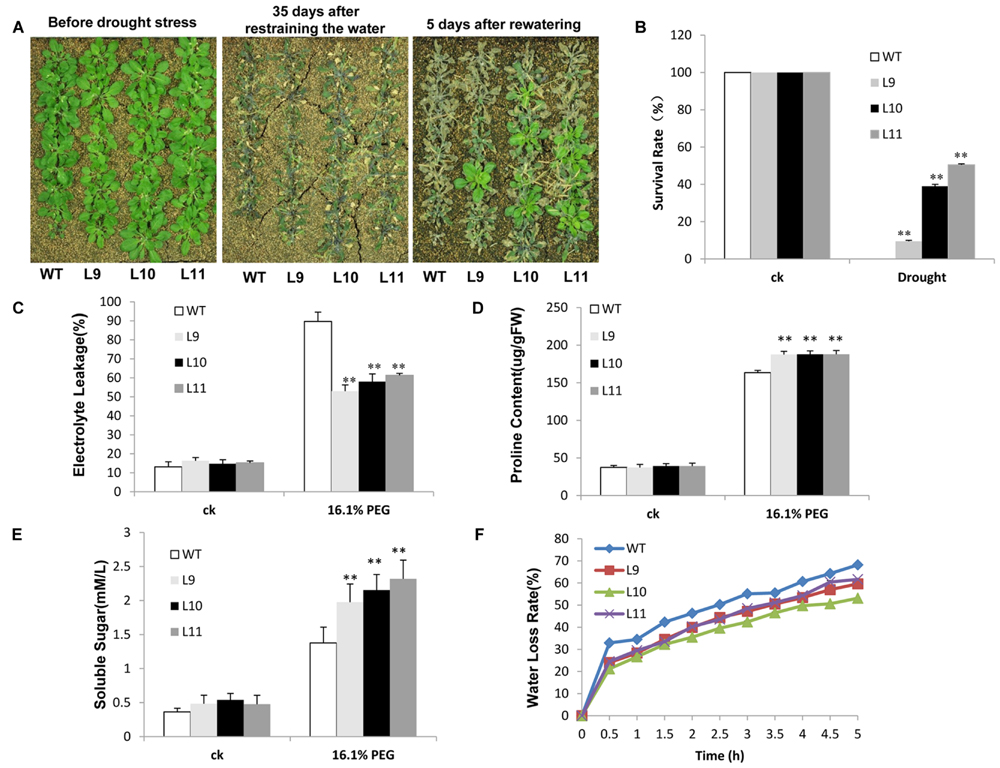
FIGURE 7. Analysis of the enhanced drought tolerance in TaNAC47-overexpressing Arabidopsis seedling lines. (A) Phenotype of WT and transgenic plants before and after drought treatments. (B) Survival rate after drought treatment. (C) Relative electrolyte leakage. (D) Proline content. (E) Soluble sugar content. (F) Water loss rate of the detached leaves. The data represent the means from three experiments. The bars show the SD. Significant differences between the transgenic and WT lines are indicated as ∗0.01 < P < 0.05 and ∗∗P < 0.01.
The ABA sensitivity in transgenic plants was assessed. In the absence of ABA, no differences in root length were found between transgenic and WT plants under normal growing conditions. However, the root growth was significantly inhibited in transgenic plants, but not in WT plants in the presence of 10 μM ABA (Figure 8). These results suggested that overexpression of the TaNAC47 gene led to increased sensitivity to ABA, which resulted in retarded growth of transgenic plants.
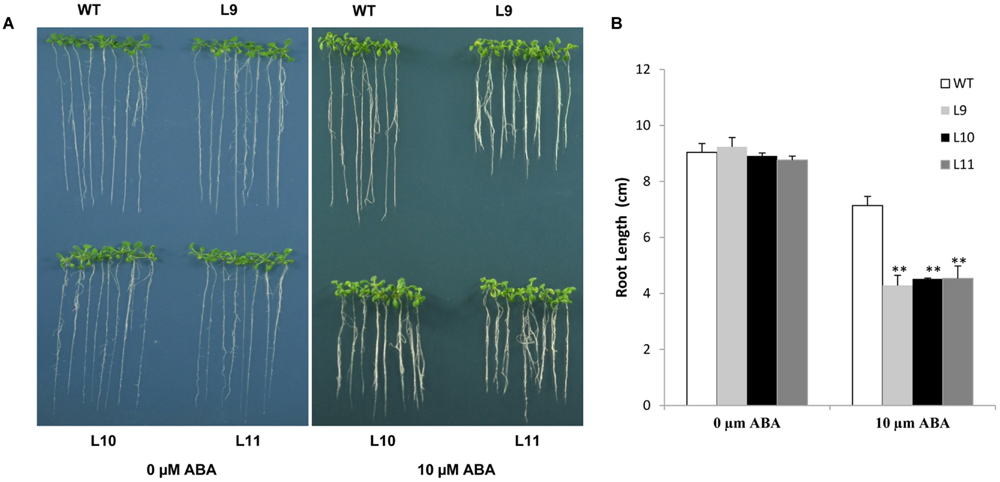
FIGURE 8. Responses of TaNAC47-overexpressing transgenic plants to ABA. (A) Phenotype of the transgenic and WT plants on MS with 0 and 10 μM ABA. (B) Root lengths of plants on MS with 10 μM ABA. The data represent the means from three experiments. The bars show the SD. Significant differences between the transgenic and WT lines are indicated as ∗0.01 < P < 0.05 and ∗∗P < 0.01.
Altered Expression of Stress-Responsive Genes in Transgenic TaNAC47 Plants
The TaNAC47 transgenic plants exhibited an improved tolerance to freezing, drought and salt stresses. Next, we examined whether expressions of any other genes involving stress response were also altered in these transgenic plants. When the plants were stressed from the salt treatment, the expression levels of genes AtRD29A, AtRD29B, AtCOR47, AtRD20, AtGSTF6, and AtP5CS1 were increased in TaNAC47 transgenic plants compared to WT plants (Figure 9). We searched the Arabidopsis genome database to acquire the promoter regions of these up-regulated genes. Results showed that these genes also contained the NAC core motif CGT[G/A] within their promoter regions (Jiang G et al., 2014), implying that TaNAC47 may confer stress tolerance through regulating various stress-responsive genes.
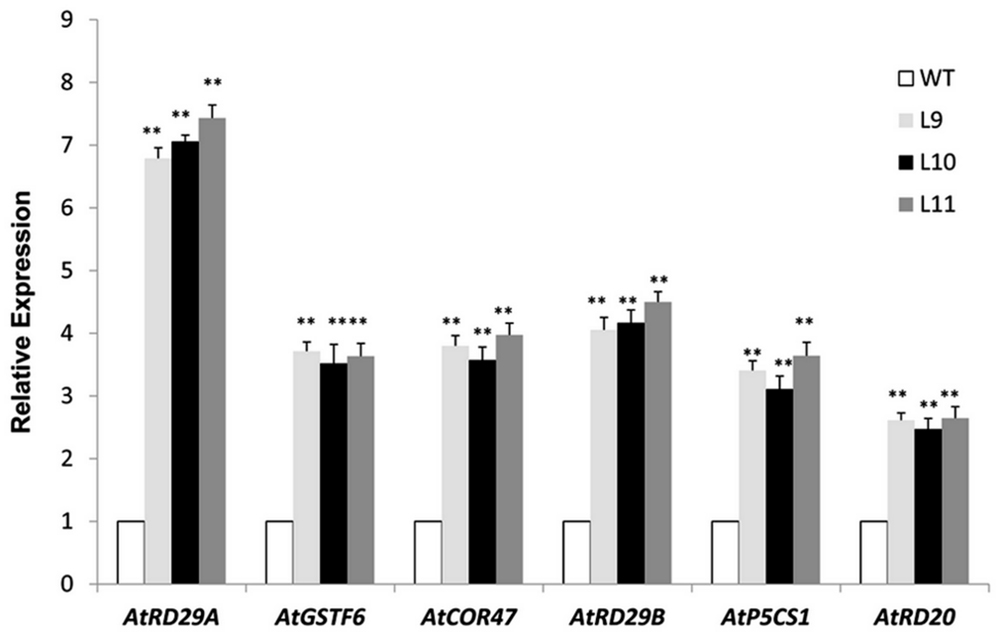
FIGURE 9. Expression levels of the stress-associated genes under salt stress. Gene-specific primers were used to detect the relative transcript levels of the stress-related genes. The data represent the means from three experiments. The bars show the SD. Significant differences between the transgenic and WT lines are indicated as ∗0.01 < P < 0.05 and ∗∗P < 0.01.
Discussion
The NAC superfamily is one of the largest TF families found only in plants (Wang N et al., 2013). We characterized wheat TaNAC47 gene, a novel stress-related member of the NAC gene family in wheat. High protein sequence identity was found between TaNAC47 and another reported stress responsive NAC protein TaNAC67. Although they shared high sequence identity, these two genes showed several intrinsic differences. First is the gene structure difference, no intron was detected in the TaNAC47 gene, while the TaNAC67 contained one intron and two exons. Secondly, a significant difference was observed in their expression levels and response times (Mao et al., 2014). Finally, although overexpression of the two genes both enhanced the tolerance to cold, drought and salt stresses in transgenic plants, only the seedlings overexpressing the TaNAC47 gene increased the sensitivity to ABA (Figure 8). The promoter region of TaNAC47 contained not only ABRE but also A-box, C-box and W-box elements, which were known to participate in abscisic acid responsiveness (Supplementary Table S1), the presence of these cis-elements may explain why TaNAC47 was induced more quickly in response to ABA treatment compared with TaNAC67.
We found tolerances to salt, cold, and drought stresses were likely conferred by overexpressing TaNAC47 in transgenic plants. This was supported by the results from stress-induced expression profiles of the TaNAC47 gene. There is a strong correlation between stress induction of the gene and stress tolerance expressed in the corresponding transgenic plants based on the physiological and molecular changes in the plants. At the physiological and biochemical levels, soluble sugars, and proline contents in plants overexpressing TaNAC47 have increased after drought and cold treatments. This agreed with previous report showing the increase in proline under stress in plants was associated with stress tolerance (Wang C et al., 2013). Furthermore, proline contributed to the adjustment of osmotic balance and induced the expression of stress-related genes (Ashraf and Foolad, 2007). The enhancement in soluble sugar content was thought to relate to protecting cellular functions, such as maintaining the structure of cellular components or acting as signal molecules (Seki et al., 2007). In addition to these physiological changes, the rate of leaf water-loss and the survival rate were both used as typical physiological indices to investigate plant resistance. Plants showing higher survival rates and less water-loss had higher tolerance and were more resistant to stresses. In this study, the leaf water-loss and the survival rate of the TaNAC47 transgenic plants were consistent with previous reports from the transcription factors OsNAC52 and RhNAC3 (Gao et al., 2010; Jiang X et al., 2014). The changes in the physiological indices in TaNAC47 transgenic plants likely resulted in enhancing tolerance to adverse conditions at the physiological level.
At gene transcription level, transgenic plants overexpressing TaNAC47 exhibited up-regulation of various other stress responsive genes, including AtRD29A, AtRD29B, AtCOR47, AtRD20, AtGSTF6, and AtP5CS1 (Figure 9). Putative NAC-binding cis-elements were found in the promoter sequences of these six genes, suggesting that these genes might be transcriptionally regulated directly by TaNAC47 (Jiang G et al., 2014). Furthermore, the RhNAC3 and AhNAC2 transcription factors were shown to enhance the expression of these genes and bind to the putative NAC recognition site of the AtRD29A, AtCOR47 and AtRD20 genes based on EMSA (Liu et al., 2011; Jiang G et al., 2014). The P5CS1 gene was involved in the proline biosynthetic pathway and was induced under stress conditions (Ashraf and Foolad, 2007), thereby contributing to the accumulation of proline contents in transgenic plants and making plants more resistant to stress. GSTF6 was found to play vital roles in plant stress tolerance and detoxification (Lan et al., 2013). Most of the up-regulated genes in TaNAC47 transgenic plants were likely involved in the ABA-dependent signaling pathway. We showed overexpression of TaNAC47 resulted in enhanced ABA sensitivity, which was consistent with previous studies on the RhNAC3, SNAC2 and OsNAC52 transcription factors from other species (Hu et al., 2008; Gao et al., 2010). ABRE is regarded as the major type of cis-element involved in the ABA signaling pathway. Previous studies on the interaction of other NAC transcription factors with ABRE were not available. In this study, we demonstrated the binding of the TaNAC47 protein to ABRE based on a yeast one-hybrid assay. Thus, our results strongly implied that TaNAC47 may participate in the ABA-dependent signaling pathway.
Conclusion
The functions of a novel wheat TaNAC47 gene were evaluated in this study overexpression of TaNAC47 in Arabidopsis, in general, enhanced the tolerance of transgenic plants to drought, freezing and salt stresses, and led to increased ABA sensitivity. Furthermore, an interaction between TaNAC47 encoded protein and ABRE cis-element was also revealed. Our data indicated that TaNAC47 regulates plant adaptation to various abiotic stresses and is a potential candidate gene to improve stress tolerance in crops.
Author Contributions
LNZ, LCZ, CX and GYZ performed most of the experiments. LNZ, LCZ, JZJ and XYK designed the experiments and wrote the paper. All authors read and approved the final manuscript.
Funding
This work was supported by the National Transgenic Research Project [2014ZX0800918B] and the National 863 Project [2011AA100104, 2012AA10A309].
Conflict of Interest Statement
The authors declare that the research was conducted in the absence of any commercial or financial relationships that could be construed as a potential conflict of interest.
Acknowledgments
We are thankful to Prof. Yongfu Fu and Prof. Jun Zhao from CAAS for kindly providing plant expression vector pEarleyGate 100 and the bait plasmid. We are grateful to Yongqiang Gu and Shiaoman Chao from USDA-ARS for their helpful comments on the manuscript and to Lei Pan for his kind experimental help.
Supplementary Material
The Supplementary Material for this article can be found online at: http://journal.frontiersin.org/article/10.3389/fpls.2015.01174
Footnotes
- ^http://expasy.org/tools/protparam.html.
- ^http://www.expasy.ch/tools/scanprosite/.
- ^http://www.dna.affrc.go.jp/PLACE/.
References
Aida, M., Ishida, T., Fukaki, H., Fujisawa, H., and Tasaka, M. (1997). Genes involved in organ separation in Arabidopsis: an analysis of the cup-shaped cotyledon mutant. Plant Cell 9, 841–857. doi: 10.1105/tpc.9.6.841
Ashraf, M., and Foolad, M. (2007). Roles of glycine betaine and proline in improving plant abiotic stress resistance. Environ. Exp. Bot. 59, 206–216. doi: 10.1016/j.envexpbot.2005.12.006
Cao, W., Liu, J., He, X., Mu, R., Zhou, H., Chen, S., et al. (2007). Modulation of ethylene responses affects plant salt-stress responses. Plant Physiol. 143, 707–719. doi: 10.1104/pp.106.094292
Chen, X., Wang, Y., Lv, B., Li, J., Luo, L., Lu, S., et al. (2014). The NAC family transcription factor OsNAP confers abiotic stress response through the ABA pathway. Plant Cell Physiol. 55, 604–619. doi: 10.1093/pcp/pct204
Clough, S., and Bent, A. (1998). Floral dip: a simplified method for Agrobacterium-mediated transformation of Arabidopsis thaliana. Plant J. 16, 735–743. doi: 10.1046/j.1365-313x.1998.00343.x
Delessert, C., Kazan, K., Wilson, I., Van Der Stracten, D., Manners, J., Dennis, E., et al. (2005). The transcription factor ATAF2 represses the expression of pathogenesis-related genes in Arabidopsis. Plant J. 43, 745–757. doi: 10.1111/j.1365-313X.2005.02488.x
Ernst, H., Olsen, A., Larsen, S., and Leggio, L. (2004). Structure of the conserved domain of ANAC, a member of the NAC family of transcription factors. EMBO Rep. 5, 297–303. doi: 10.1038/sj.embor.7400093
Gao, F., Xiong, A., Peng, R., Jin, X., Xu, J., Zhu, B., et al. (2010). OsNAC52, a rice NAC transcription factor, potentially responds to ABA and confers drought tolerance in transgenic plants. Plant Cell Tiss. Organ Cult. 100, 255–262. doi: 10.1007/s11240-009-9640-9
Guo, Y., and Gan, S. (2006). AtNAP, a NAC family transcription factor, has an important role in leaf senescence. Plant J. 46, 601–612. doi: 10.1111/j.1365-313X.2006.02723.x
He, X., Mu, R., Cao, W., Zhang, Z., Zhang, J., and Chen, S. (2005). AtNAC2, a transcription factor downstream of ethylene and auxin signaling pathways, is involved in salt stress response and lateral root development. Plant J. 44, 903–916. doi: 10.1111/j.1365-313X.2005.02575.x
Hendelman, A., Stav, R., Zemach, H., and Arazi, T. (2013). The tomato NAC transcription factor SINAM2 is involved in flower-boundary morphogenesis. J. Exp. Bot. 64, 5497–5507. doi: 10.1093/jxb/ert324
Hu, H., You, J., Fang, Y., Zhu, X., Qi, Z., and Xiong, L. (2008). Characterization of transcription factor gene SNAC2 conferring cold and salt tolerance in rice. Plant Mol. Biol. 67, 169–181. doi: 10.1007/s11103-008-9309-5
Jeong, J., Kim, Y., Baek, K., Jung, H., Ha, S., Choi, Y., et al. (2010). Root-specific expression of OsNAC10 improves drought tolerance and grain yield in rice under field drought conditions. Plant Physiol. 153, 185–197. doi: 10.1104/pp.110.154773
Jia, J., Zhao, S., Kong, X., Li, Y., Zhao, G., He, W., et al. (2013). Aegilops tauschii draft genome sequence reveals a gene repertoire for wheat adaptation. Nature 496, 91–95. doi: 10.1038/nature12028
Jiang, G., Jiang, X., Lü, P., Liu, J., Gao, J., and Zhang, C. (2014). The rose (Rosa hybrida) NAC transcription factor 3 gene, RhNAC3, involved in ABA signaling pathway both in rose and Arabidopsis. PLoS ONE 9:e109415. doi: 10.1371/journal.pone.0109415
Jiang, X., Zhang, C., Lü, P., Jiang, G., Liu, X., Dai, F., et al. (2014). RhNAC3, a stress-associated NAC transcription factor, has a role in dehydration tolerance through regulating osmotic stress-related genes in rose petals. Plant Biotechnol. J. 12, 38–48. doi: 10.1111/pbi.12114
Kato, H., Motomura, T., Komeda, Y., Saito, T., and Kato, A. (2010). Over expression of the NAC transcription factor family gene ANAC036 results in a dwarf phenotype in Arabidopsis thaliana. J. Plant Physiol. 167, 571–577. doi: 10.1016/j.jplph.2009.11.004
Kim, H., Park, B., Yoo, J., Jung, M., Lee, S., Han, H., et al. (2007). Identification of a calmodulin-binding NAC protein as a transcriptional repressor in Arabidopsis. J. Biol. Chem. 282, 36292–36302. doi: 10.1074/jbc.M705217200
Kim, Y., Kim, S., Park, J., Park, H., Lim, M., and Chua, N. (2006). A membrane-bound NAC transcription factor regulates cell division in Arabidopsis. Plant Cell 18, 3132–3144. doi: 10.1105/tpc.106.043018
Lan, T., Wang, X., and Zeng, Q. (2013). Structural and functional evolution of positively selected sites in pine glutathione S-transferase enzyme family. J. Biol. Chem. 288, 24441–24451. doi: 10.1074/jbc.M113.456863
Lehner, A., Corbineau, F., and Bailly, C. (2006). Changes in lipid status and glass properties in cotyledons of developing sunflower seeds. Plant Cell Physiol. 47, 818–828. doi: 10.1093/pcp/pcj053
Liu, X., Hong, L., Li, X., Yao, Y., Hu, B., and Li, N. (2011). Improved drought and salt tolerance in transgenic Arabidopsis overexpressing NAC transcription factor from Arachis hypogaes. Biosci. Biotechnol. Biochem. 75, 443–450. doi: 10.1271/bbb.100614
Mao, X., Chen, S., Li, A., Zhai, C., and Jing, R. (2014). Novel NAC transcription factor TaNAC67 confers enhanced multi-abiotic stress tolerances in Arabidopsis. PLoS ONE 9:e84359. doi: 10.1371/journal.pone.0084359
Mao, X., Zhang, H., Qian, X., Li, A., Zhao, G., and Jing, R. (2012). TaNAC2, a NAC-type wheat transcription factor conferring enhanced multiple abiotic stress tolerances in Arabidopsis. J. Exp. Bot. 63, 2933–2946. doi: 10.1093/jxb/err462
Mitsuda, N., Iwase, A., Yamamoto, H., Yoshida, M., Seki, M., Shinozaki, K., et al. (2007). NAC transcription factors, NST1 and NST3, are key regulators of the formation of secondary walls in woody tissues of Arabidopsis. Plant Cell 19, 270–280. doi: 10.1105/tpc.106.047043
Nakashima, K., Takasaki, H., Mizoi, J., Shinozaki, K., and Yamaguchi-Shinozaki, K. (2012). NAC transcription factors in plant abiotic stress responses. Biochim. Biophys. Acta 1819, 97–103. doi: 10.1016/j.bbagrm.2011.10.005
Nakashima, K., Tran, L., Nguyen, S., Fujita, M., Maruyama, K., Todaka, D., et al. (2007). Functional analysis of a NAC-type transcription factor OsNAC6 involved in abiotic and biotic stress-responsive gene expression in rice. Plant J. 51, 617–630. doi: 10.1111/j.1365-313X.2007.03168.x
Olsen, A. N., Ernst, H., Leggio, L., and Skriver, K. (2005). NAC transcription factors: structurally distinct, functionally diverse. Trends Plant Sci. 10, 79–87. doi: 10.1016/j.tplants.2004.12.010
Park, J., Kim, Y., Kim, S., Jung, J., Woo, J., and Park, C. (2011). Integration of auxin and salt signals by the NAC transcription factor NTM2 during seed germination in Arabidopsis. Plant Physiol. 156, 537–549. doi: 10.1104/pp.111.177071
Puranik, S., Bahadur, R., Srivastava, P., and Prasad, M. (2011). Molecular cloning and characterization of a membrane associated NAC family gene, SiNAC from foxtail millet [Setaria italica (L.) P. Beauv]. Mol. Biotechnol. 49, 138–150. doi: 10.1007/s12033-011-9385-7
Quach, T., Tran, L., Valliyodan, B., Nguyen, H., Kumar, R., Neelakandan, A., et al. (2014). Functional analysis of water stress-responsive soybean GmNAC003 and GmNAC004 transcription factors in lateral root development in Arabidopsis. PLoS ONE 9:e84886. doi: 10.1371/journal.pone.0084886
Redillas, M., Jeong, J., Kim, Y., Jung, H., Bang, S., Choi, Y., et al. (2012). The overexpression of OsNAC9 alters the root architecture of rice plants enhancing drought resistance and grain yield under field conditions. Plant Biotechnol. J. 10, 792–805. doi: 10.1111/j.1467-7652.2012.00697.x
Saad, A., Li, X., Li, H., Huang, T., Gao, C., Guo, M., et al. (2013). A rice stress-responsive NAC gene enhances tolerance of transgenic wheat to drought and salt stresses. Plant Sci. 203, 33–40. doi: 10.1016/j.plantsci.2012.12.016
Saga, H., Ogawa, T., Kai, K., Suzuki, H., Ogata, Y., Sakurai, N., et al. (2012). Identification and characterization of ANAC042, a transcription factor family gene involved in the regulation of camalexin biosynthesis in Arabidopsis. Mol. Plant Microbe Interact. 25, 684–696. doi: 10.1094/MPMI-09-11-0244
Seki, M., Umezawa, T., Urano, K., and Shinozaki, K. (2007). Regulatory metabolic networks in drought stress responses. Curr. Opin. Plant Biol. 10, 296–302. doi: 10.1016/j.pbi.2007.04.014
Shah, S., Pang, C., Fan, S., Song, M., Arain, S., and Yu, S. (2013). Isolation and expression profiling of GhNAC transcription factor genes in cotton (Gossypium hirsutum L.) during leaf senescence and in response to stresses. Gene 531, 220–234. doi: 10.1016/j.gene.2013.09.007
Sheludko, Y. V., Sindarovska, Y. R., Gerasymenko, I. M., Bannikova, M. A., and Kuchuk, N. V. (2007). Comparison of several Nicotiana species as hosts for high-scale Agrobacterium-mediated transient expression. Biotechnol. Bioeng. 96, 608–614. doi: 10.1002/bit.21075
Souer, E., Houwelingen, A., Kloos, D., Mol, J., and Koes, R. (1996). The no apical meristem gene of Petunia is required for pattern formation in embryos and flowers and is expressed at meristem and primordial boundaries. Cell 85, 159–170. doi: 10.1016/S0092-8674(00)81093-4
Takasaki, H., Maruyama, K., Kidokoro, S., Ito, Y., Fujita, Y., Shinozaki, K., et al. (2010). The abiotic stress responsive NAC-type transcription factor OsNAC5 regulates stress inducible genes and stress tolerance in rice. Mol. Genet. Genomics 284, 173–183. doi: 10.1007/s00438-010-0557-0
Tang, Y., Liua, M., Gao, S., Zhang, Z., Zhao, X., Zhao, C., et al. (2012). Molecular characterization of novel TaNAC genes in wheat and overexpression of TaNAC2a confers drought tolerance in tobacco. Physiol. Plant 144, 210–224. doi: 10.1111/j.1399-3054.2011.01539.x
Tran, L., Nishiyama, R., Yamaguchi-Shinozaki, K., and Shinozaki, K. (2010). Potential utilization of NAC transcription factors to enhance abiotic stress tolerance in plants by biotechnological approach. GM Crops 1, 32–39. doi: 10.4161/gmcr.1.1.10569
Voitsik, A., Muench, S., Deising, H., and Voll, L. (2013). Two recently duplicated maize NAC transcription factor paralogs are induced in response to colletotrichum graminicola infection. BMC Plant Biol. 13:85. doi: 10.1186/1471-2229-13-85
Wang, C., Deng, P., Chen, L., Wang, X., Ma, H., and Hu, W. (2013). A wheat WRKY transcription factor TaWRKY10 confers tolerance to multiple abiotic stresses in transgenic tobacco. PLoS ONE 8:e65120. doi: 10.1371/journal.pone.0065120
Wang, N., Zheng, Y., Xin, H., Fang, L., and Li, S. (2013). Comprehensive analysis of NAC domain transcription factor gene family in Vitis vinifera. Plant Cell Rep. 32, 61–75. doi: 10.1007/s00299-012-1340-y
Xia, N., Zhang, G., Liu, X., Deng, L., Cai, C., Zhang, Y., et al. (2010a). Characterization of a novel wheat NAC transcription factor gene involved in defense response against stripe rust pathogen infection and abiotic stresses. Mol. Biol. Rep. 37, 3703–3712. doi: 10.1007/s11033-010-0023-4
Xia, N., Zhang, G., Sun, Y., Zhu, L., Xu, L., Chen, X., et al. (2010b). TaNAC8, a novel NAC transcription factor gene in wheat, responds to stripe rust pathogen infection and abiotic stresses. Physiol. Mol. Plant Pathol. 74, 394–402. doi: 10.1016/j.pmpp.2010.06.005
Xue, G., Way, H., Richardson, T., Drenth, J., Joyce, P., and Mcintyre, C. (2011). Overexpression of TaNAC69 leads to enhanced transcript levels of stress up-regulated genes and dehydration tolerance in bread wheat. Mol. Plant 4, 697–712. doi: 10.1093/mp/ssr013
Yamaguchi, M., Ohtani, M., Mitsuda, N., Kubo, M., Ohme-Takagi, M., Fukuda, H., et al. (2010). VND-INTERACTING2, a NAC domain transcription factor, negatively regulates xylem vessel formation in Arabidopsis. Plant Cell 22, 1249–1263. doi: 10.1105/tpc.108.064048
Yang, S., Seo, P., Yoon, H., and Park, C. (2011). The Arabidopsis NAC transcription factor VNI2 integrates abscisic acid signals into leaf senescence via the COR/RD genes. Plant Cell 23, 2155–2168. doi: 10.1105/tpc.111.084913
Yokotani, N., Tsuchida, M., Ichikawa, H., Mitsuda, N., Ohme, T., Kaku, H., et al. (2014). OsNAC111, a blast disease-responsive transcription factor in rice, positively regulates the expression of defense-related genes. Mol. Plant Microbe Interact. 27, 1027–1034. doi: 10.1094/MPMI-03-14-0065-R9.007
Zhang, L. C., Zhao, G. Y., Xia, C., Jia, J. Z., Liu, X., and Kong, X. Y. (2012). Overexpression of a wheat MYB transcription factor gene, TaMYB56-B, enhances tolerances to freezing and salt stresses in transgenic Arabidopsis. Gene 505, 100–107. doi: 10.1016/j.gene.2012.05.033
Zhao, Q., Gallego, G., Wang, H., Zeng, Y., Ding, S., Chen, F., et al. (2010). An NAC transcription factor orchestrates multiple features of cell wall development in Medicago truncatula. Plant J. 63, 100–114. doi: 10.1111/j.1365-313X.2010.04223.x
Zhao, Y., Sun, J., Xu, P., Zhang, R., and Li, L. (2014). Intron-mediated alternative splicing of WOOD-ASSOCIATED NAC TRANSCRIPTION FACTOR1B regulates cell wall thickening during fiber development in Populus species. Plant Physiol. 164, 765–776. doi: 10.1104/pp.113.231134
Keywords: NAC transcription factor, abiotic stress, ABRE, wheat, ABA
Citation: Zhang L, Zhang L, Xia C, Zhao G, Jia J and Kong X (2016) The Novel Wheat Transcription Factor TaNAC47 Enhances Multiple Abiotic Stress Tolerances in Transgenic Plants. Front. Plant Sci. 6:1174. doi: 10.3389/fpls.2015.01174
Received: 29 September 2015; Accepted: 07 December 2015;
Published: 18 January 2016.
Edited by:
Irene Murgia, Università degli Studi di Milano, ItalyReviewed by:
Ravi Valluru, International Maize and Wheat Improvement Center, MexicoFelipe Klein Ricachenevsky, Universidade Federal do Rio Grande do Sul, Brazil
Copyright © 2016 Zhang, Zhang, Xia, Zhao, Jia and Kong. This is an open-access article distributed under the terms of the Creative Commons Attribution License (CC BY). The use, distribution or reproduction in other forums is permitted, provided the original author(s) or licensor are credited and that the original publication in this journal is cited, in accordance with accepted academic practice. No use, distribution or reproduction is permitted which does not comply with these terms.
*Correspondence: Xiuying Kong, a29uZ3hpdXlpbmdAY2Fhcy5jbg==
†These authors have contributed equally to this work.