- 1Laboratory of Cell Biology, Institute of Experimental Botany, Academy of Sciences of Czech Republic, Prague, Czech Republic
- 2Laboratory of Cell Morphogenesis, Department of Experimental Plant Biology, Faculty of Science, Charles University in Prague, Prague, Czech Republic
Even though resistance (R) genes are among the most studied components of the plant immunity, there remain still a lot of aspects to be explained about the regulation of their function. Many gain-of-function mutants of R genes and loss-of-function of their regulators often demonstrate up-regulated defense responses in combination with dwarf stature and/or spontaneous leaf lesions formation. For most of these mutants, phenotypes are a consequence of an ectopic activation of R genes. Based on the compilation and comparison of published results in this field, we have concluded that the constitutively activated defense phenotypes recurrently arise by disruption of tight, constitutive and multilevel negative control of some of R proteins that might involve also their targeting to the autophagy pathway. This mode of R protein regulation is supported also by protein–protein interactions listed in available databases, as well as in silico search for autophagy machinery interacting motifs. The suggested model could resolve some explanatory discrepancies found in the studies of the immunity responses of autophagy mutants.
Introduction
There are several approaches how to study and classify the plant immunity related events, and the most widespread is division of the plant immunity into two modes – a pathogen-associated molecular patterns (PAMPs) triggered immunity (PTI), which is triggered usually by recognition of structural components of pathogen on the surface of the host cell, and effector triggered immunity (ETI; Jones and Dangl, 2006). These two defense modes employ basically the same means, but PTI is more general and mild, while ETI is much stronger and more efficient. ETI is triggered by the direct or indirect interaction between a specific disease resistance (R) protein and a corresponding avirulence (Avr) protein of pathogen and is accompanied by a number of changes within the plant – production of reactive oxygen species (ROS) by an oxidative burst, accumulation of the salicylic acid (SA), and the transcriptional activation of genes involved in defense response, that lead to a possible final stage – localized programmed cell death called the hypersensitive response (HR; Pontier et al., 1998; review in McDowell and Woffenden, 2003; Vlot et al., 2008).
Disease resistance (R) genes are central components of the plant immune response. All R proteins contain at least some of basic motifs – either Toll/interleukin-1 receptor (TIR) or coiled-coil (CC) structure on the N terminal part, nucleotide-binding site (NBS), leucine-rich repeat LRR), protein kinase and transmembrane domains (review by Martin, 1999; Liu et al., 2007). There are 145 putative genes encoding a product with a TIR domain and 51 with CC domain predicted in the Arabidopsis thaliana Col-0 genome (Meyers et al., 2003; Jacob et al., 2013). Majority encode proteins with TIR, NBS, and LRR domains, making the TNL group; some genes encode proteins with TIR and NBS domains but no LRR domain (TN genes) and some encode proteins with a TIR domain only (TX genes; Meyers et al., 2003; Nandety et al., 2013). Besides CC-NBS-LRR containing proteins which make CNL group, there are also four proteins that have NBS motifs similar to CNLs, but lack a CC motif (Meyers et al., 2003).
There are several important molecules involved in signaling downstream the successful R-Avr recognition – ENHANCED DISEASE SENSITIVITY 1 (EDS1), PHYTOALEXIN DEFICIENT 4 (PAD4), NON-RACE SPECIFIC DISEASE RESISTANCE 1 (NDR1) and SENESCENCE ASSOCIATED GENE 101 (SAG101), which are essential for the accomplishment of HR and for the accumulation of the SA. EDS1, PAD4 and SAG101 are involved in transferring signals mainly from TNL proteins, while CNL pathway mostly relies on signaling through NDR1 (Century et al., 1997; Feys et al., 2001; He and Gan, 2002; Wagner et al., 2013).
In Arabidopsis mutants in genes coding for R and R-associated proteins, along with defense related deviations, two other most frequent phenotypes are a dwarf stature and a spontaneous HR lesion formation; many times present even simultaneously (Table 1). Rarely, a lethal phenotype occurs as well, even though no developmental function for these genes has been found so far. We could notice that for most of the R genes mutants, described phenotypes are a consequence of their activation, in some cases even a gain of function mutations (GOF). Based on the comparison of different studies of plant immunity, our hypothesis aims to suggest a model in which the hyper immune phenotypes arise as a result of disruption of tight, multistep and constitutive negative control of R proteins that possibly involves also their inactivation by the autophagy pathway.
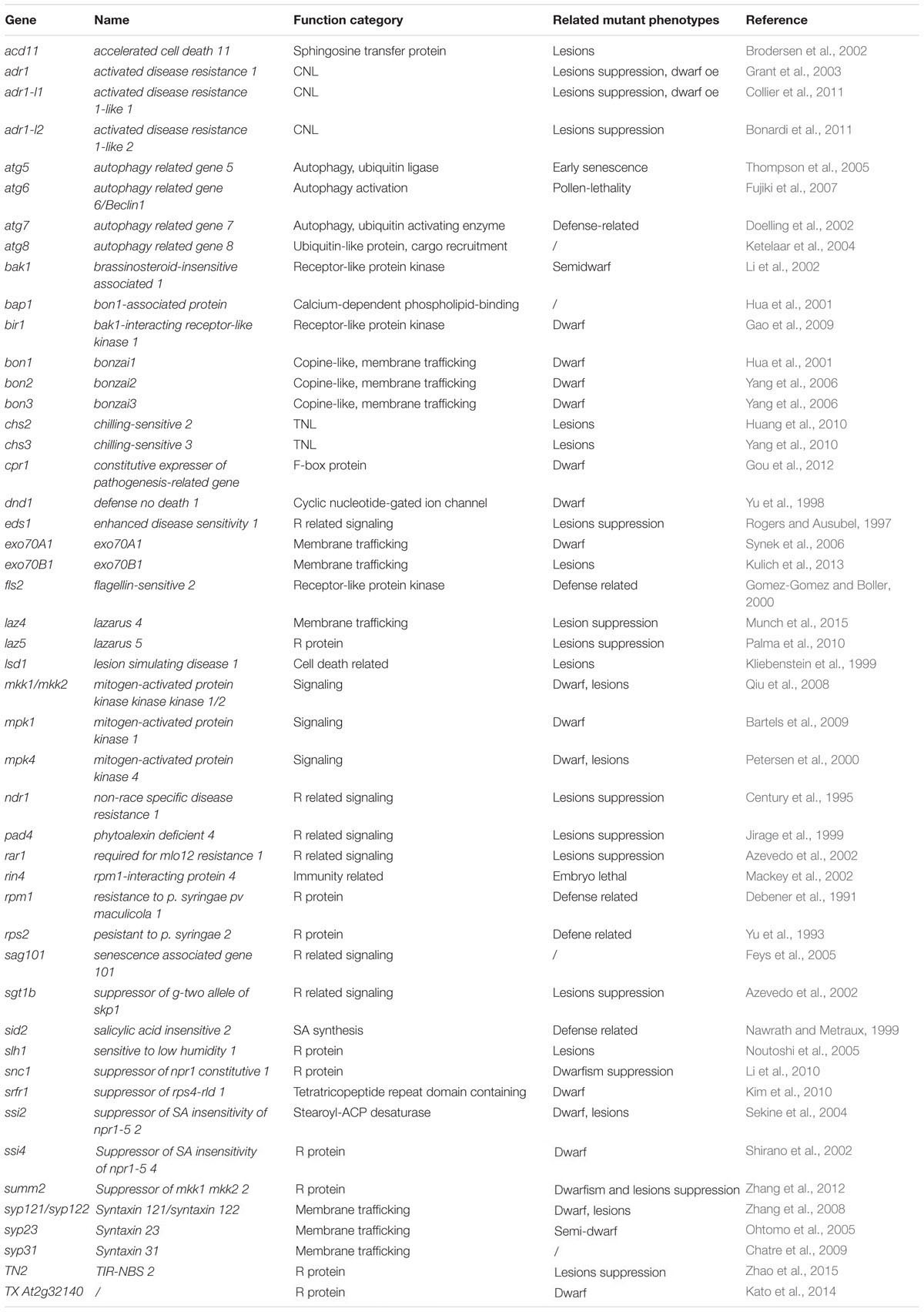
TABLE 1. List of Arabidopsis mutants related to R proteins hyper activity causing dwarf and lesion mimic phenotypes.
Of Dwarfs and Lesions
It was shown that mutants with over activated R protein dependent defense response develop mostly two phenotypes – dwarfism and/or necrotic leaf lesions (reviewed e.g., in Lorrain et al., 2003 and Janda and Ruelland, 2014). For instance, in plants overexpressing a CNL gene ACTIVATED DISEASE RESISTANCE 1 (ADR1), a constitutive defense response and a dwarf phenotype were found (Grant et al., 2003). A TNL protein SUPPRESSOR OF NPR1 CONSTITUTIVE 1 (SNC1) was found to be overactive in the bonzai1-1 (bon1-1) mutant which also shows a constitutive defense response and reduced plant size (Yang and Hua, 2004). Along with bon1, several other autoimmune dwarf mutations were found to be suppressed by mutation of SNC1 locus; namely in BON1-ASSOCIATED PROTEIN (bap1), BAK1-INTERACTING RECEPTOR-LIKE KINASE 1 (bir1), SUPPRESSOR OF RPS4-RLD 1 (srfr1), CONSTITUTIVE EXPRESSER OF PATHOGENESIS-RELATED GENE (cpr1) and MITOGEN-ACTIVATED PROTEIN KINASE 1 (mpk1; review in Gou and Hua, 2012). Plants overexpressing a TIR-X gene At2g32140 show also dwarf phenotype and activated expression of defense-related genes (Kato et al., 2014). This phenotype was dependent on EDS1, PAD4, and partially dependent on SALICYLIC ACID INDUCTION DEFICIENT 2 (SID2).
HR-like spontaneous leaf necrotic lesions were found to be even more frequently associated with the mutations in R genes and constitutively activated immunity. For instance, a GOF mutant in TNL RPP4 locus called chilling sensitive 2 (chs2) shows lesions in the low temperature conditions (Huang et al., 2010). GOF Arabidopsis mutant in the other CHS gene, chs3-1, which encodes an unconventional disease resistance (R) protein belonging to the TIR-NB-LRR class with a zinc-binding LIM domain (Lin-11, Isl-1 and Mec-3 domains) at the carboxyl terminus, shows arrested growth, chlorosis and constitutively activated defence responses at 16°C (Yang et al., 2010). A mutant in TNL gene ssi4 develops chlorotic lesions which can be suppressed by high humidity (Shirano et al., 2002; Zhou et al., 2004). In addition, there are several examples of mutants with spontaneous lesions induction which are suppressed by mutations in loci encoding R proteins of CNL type – ACTIVATED DISEASE RESISTANCE 1 – adr1, adr1-l1 and adr1-l2 suppress LESION SIMULATING DISEASE 1 (lsd1) by down regulating SA signaling (Bonardi et al., 2011; Roberts et al., 2013). Likewise, when a putative TNL encoded by LAZARUS 5 (LAZ5) gene is mutated, accelerated cell death 11 (acd11) lesion phenotype can be suppressed (Palma et al., 2010). It was also shown, that in the absence of the copine-like proteins BON1 and BON3 function, several R-like genes of the TNL/TN type were found to trigger lesion cell death (LCD; Li et al., 2009). Mutation in SUPPRESSOR OF MKK1 MKK2 2 (summ2) which encodes putative NB-LRR, suppresses lesions formation and dwarfism of mutants of MAP kinase pathway mkk1/mkk2 and mpk4 (Kong et al., 2012).
There are genes coding for other defense related components that when mutated trigger the same constitutive immunity activation and dwarf or/and lesion mimic phenotypes – e.g., CONSTITUTIVE EXPRESSOR OF PATHOGENESIS-RELATED GENE 1 (CPR1), SUPPRESSOR OF SALICYLIC ACID INSENSITIVITY OF NPR1-5 2 (SSI2), DEFENSE NO DEATH 1 (DND1), TYPE III PHOSPHATIDYLINOSITOL-4-KINASES β1β2 (PI4KIIIβ1β2) (Bowling et al., 1994; Yu et al., 1998; Zhang et al., 2003; Sekine et al., 2004; Gou et al., 2012; Sasek et al., 2014). As a regular aspect of these mutants’ phenotype deviations, hyper accumulation of SA was observed.
Lethality of the Hub
Overactive immunity can disturb plant growth and fitness, and in an extreme case, this can be deleterious. Unexpectedly, an embryo lethal phenotype was found for LOF mutation of a defense related gene RPM1-INTERACTING PROTEIN 4 (RIN4). Being evolutionarily conserved protein in plants, RIN4 is targeted to the plasma membrane by C-terminal acylation, and is required for the activation of a CNL RESISTANCE TO PSEUDOMONAS SYRINGAE PV. MACULICOLA 1 (RPM1; Kim et al., 2005; Takemoto and Jones, 2005). RIN4 is phosphorylated upon infection with P. syringae expressing either AvrB or AvrRpm1 (Mackey et al., 2002). RIN4 is also involved in the activation of another CNL type R protein RESISTANCE TO P. SYRINGAE 2 (RPS2) by putative Cys protease AvrRpt2 of P. syringae, which causes posttranscriptional cleavage and disappearance of RIN4 and this is required for full RPS2 activation (Axtell and Staskawicz, 2003; Mackey et al., 2003). Interestingly, in coimmunoprecipitation experiments, RIN4 was found to associate with RPM1, RPS2 as well as with pathogen recognition receptor (PRR) FLAGELLIN-SENSITIVE 2 (FLS2), creating thus a physical link between PTI and ETI (Qi et al., 2011). The rin4 null mutation lethality is rescued in a rin4rps2 double mutant, indicating that RIN4 negatively regulates inappropriate activation of RPS2 (Mackey et al., 2003). In addition, fragments of RIN4, including those produced by AvrRpt2, each containing a nitrate-induced (NOI) domain specific for plants, suppress PTI, also in the rpm1/rps2/rin4 mutant background, and activate a cell death response in the wild type (Afzal et al., 2011).
Membrane Trafficking and the R Proteins-Dependent Immunity
Surprisingly, several basic regulators expected to function in the endomembrane trafficking and membrane fusion events, such as SNARE and exocyst proteins, might be also connected to the regulation of activity of R proteins. For instance, the dwarf and lesion-mimic double mutant of plasma membrane syntaxins SYP121 and SYP122 constitutively expresses the SA signaling pathway- as well as other known pathogen-responsive genes (Zhang et al., 2008). The same study shows that based on the suppressor mutant analysis of syp121 syp122, PAD4 is of key importance for the lesion development. Mutant alleles of signaling mediators of both TNL and CNL-type resistances EDS1, NDR1, REQUIRED FOR MLO12 RESISTANCE 1 (RAR1) and SUPPRESSOR OF G-TWO ALLELE OF SKP1 (SGT1b) partially rescued the lesion-mimic phenotype. Interestingly, the double mutant was crossed to the autophagy atg7 mutant, however, as there was no effect of this mutation on the appearance of lesions, authors concluded that the autophagy does not play a role in this process (Azevedo et al., 2002; Zhang et al., 2008).
Recently, exo70B1 loss-of-function mutant was found to develop spontaneous leaf lesions, over-express defense responses genes and show enhanced resistance to fungal, oomycete and bacterial pathogens (Kulich et al., 2013; Stegmann et al., 2013). Unexpectedly, its function is not related to the secretion of secretory vesicles to the plasma membrane; instead, EXO70B1 positive compartments were found to end in the central vacuole and to co-localize with autophagosomal marker ATG8f. In a screen for mutants that suppress exo70B1 phenotype, nine alleles of TIR-NBS2 (TN2) were identified, suggesting that loss-of-function of EXO70B1 leads to activation of this TN protein (Zhao et al., 2015). It was also shown that TN2 interacts with EXO70B1 in yeast and in planta. However, it is not known whether TN2 directly monitors EXO70B1 integrity (as proposed by Zhao et al., 2015) or whether EXO70B1 is only required for autophagic transport to the vacuole and subsequent degradation of TN2. EXO70B1-mediated autophagy-related transport to the vacuole might be participating in TN2 degradation. Both scenarios would explain the observed phenotype.
Additionally, recent work confirmed the importance of membrane trafficking in the plant cell death lesion suppression – lazarus 4 (laz4) was found to be mutated in one of three VACUOLAR PROTEIN SORTING 35 (VPS35) genes which code for a subunit of the retromer complex functioning in endosomal protein sorting and vacuolar trafficking – esp. of retrograde retrieval of vacuolar sorting receptors. These results also showed that the retromer deficiency impairs endosomal sorting of immune components and targeting of vacuolar cargo (Munch et al., 2015).
Interestingly, the endosomal compartment may be as well the site of R-Avr proteins interaction – potato R3A and Phytophtora infestans effector AVR3a interact and relocalize from the cytoplasm to endocytotic compartment from where they turn on HR signaling (Engelhardt et al., 2012).
Even though it was not described for plants so far, we can expect that the both endosomes and autophagy related membrane trafficking will provide pathogens an opportunity to manipulate both for the purposes of more successful infection. Such an example was recently described for human epithelium-Salmonella interaction – at early stages of S. typhimurium infection, autophagy is used to seal endosomal membranes damaged by Salmonella secretion system during host cell invasion, but later it is also necessary for the further progression of Salmonella infection (Kreibich et al., 2015).
From Autophagy to Immunity
Autophagy is a bulk degradation by which cell/organism recycles nutrients, deals with stress, clears off dysfunctional organelles, aggregates etc. (Levine and Klionsky, 2004). Several types of autophagy have been reported, including macroautophagy, which is present in many organisms including fungi, animals and plants. This process relies on the concerted action of autophagy-related (ATG) genes encoded proteins to form first phagophore, to promote phagophore enclosure into autophagosome, and to deliver autophagosomes to the vacuole or lysosomes to release the autophagic bodies for eventual breakdown (Li and Vierstra, 2012; Reggiori and Klionsky, 2013).
When Arabidopsis mutants are disrupted in ATG genes represented by single loci, they grow normally under non-stress conditions, but are hypersensitive to nitrogen and carbon starvation (Doelling et al., 2002; Hanaoka et al., 2002; Yoshimoto et al., 2004; Thompson et al., 2005). However, unlike other non-plant organisms, Arabidopsis has nine ATG8 and two ATG12 gene isoforms, which makes the study of their role more difficult and suggests that the autophagic process in plants is more complicated than in other organisms. Some of its complexity is reflected in the role of autophagy in plant immunity.
The importance of autophagy in the plant immunity was first demonstrated in Liu et al. (2005) – it was found that the autophagy was required to restrict the spread of plant HR cell death. The activation of hypersensitive cell death via the R gene RPM1 upon infection with bacteria also led to cell death beyond the borders of the infection site in plants silenced for atg6/Beclin1 (Patel and Dinesh-Kumar, 2008). It was concluded that autophagy prevents unrestricted HR cell death and that functions as a pro-survival pathway in plant–pathogen interactions. All of these observations and conclusions were based on experimenting with older Arabidopsis plants and on tissues surrounding the actual infection sites, a few days after local infection.
However, a pro-death function of autophagy during HR cell death was reported as well (Hofius et al., 2009). Autophagy was found to be triggered by some, but not all types of R proteins in the infected tissue and its surroundings. HR cell death triggered by R proteins RPS4, RPP1 and RPM1 was significantly suppressed in atg (autophagy) mutants; especially the first two of them which signal through EDS1 signaling component. In this case, cell death was monitored in the actual infection site, in the range of hours after inoculation (Hofius et al., 2009).
Yoshimoto et al. (2009), found no deviations in RPM1-triggered cell death beyond the initial infection site in younger atg mutants. However, in older atg mutants such as atg5, they observed lesions in non-infected tissues 6–9 days after infection. Interestingly, these effects were suppressed by removal of the SA and by mutations in SA signaling hub “non-expressor of PR genes” – NPR1. The authors proposed that autophagy negatively regulates the cell death by controlling NPR1-dependent SA signaling. In contrast to younger leaves, older atg mutant leaves contain higher levels of toxic metabolites, disrupted organelles and oxidized proteins which contribute to the cell death spread (Yoshimoto et al., 2009). This could be as well explained as a combination of effects of different sets of genes involved in the adult plant resistance and ineffective autophagy (Carviel et al., 2009). Scientists tried to explain and integrate these conflicting results obtained from studies on HR lesions of atg mutants. Zhou et al. (2014) propose that autophagy suppresses SA and ROS signaling amplification loop that leads to cell death, while in the resistance to necrotrophic pathogens it promotes JA signaling. Consistently, a recent hypothesis suggests that SA is not only an autophagy inducer, but also a cargo for autophagy-related ER to vacuole membrane transport and catabolism (Kulich and Zarsky, 2014). Recently, a model was worked out in which the autophagy is both initiator and executioner of cell death and is placed downstream of the R protein activation, and supposed to help the cell to deal with the ER stress provoked by a heavy load with pathogenesis related proteins (PRs, review in Minina et al., 2014).
Conclusion and Perspectives
Here we show that most of the observed defects in Arabidopsis R protein regulator mutants are a direct or indirect consequence of non-pathogen related ectopic R protein activation. It thus seems conceivable that the plant constantly down regulates R protein function, and when this constitutive negative regulation is disturbed, the R proteins are activated and spontaneously signal the non-existent pathogen attack. Based on the example of rin4 mutant lethality we could speculate, that, similarly to other organisms, the proper function of the negative control might be set already in the earliest stages of development. The plant innate immunity has to be kept as low as possible when it is not necessary in order to prevent high energy costs of defense, and yet in the state of alertness which will allow its fast, in fact instantaneous, activation. We believe that the best way to achieve this is to keep these components (i.e., in our case R genes) transcribed and translated on a sufficient basic level, but to keep their function tightly under negative control which will prevent undesired overactive autoimmunity. How could be this achieved? There are many examples of negative controls involved at various stages of defense that include ubiquitination and proteolysis, phosphorylation of proteins, as well as redox dependent changes in protein multimerization and localization (e.g., Trujillo et al., 2008; Anderson et al., 2011; Vogelmann et al., 2012). We suggest that one of the mechanisms to achieve this is also targeting of defense machinery components – here especially R proteins – to the autophagy pathway for degradation (Figure 1). Once the R protein is recruited by autophagy machinery into the autophagosome, it might share the destiny of other autophagic cargos – transport to the vacuole and degradation. We speculate that along with proteins the autophagy related degradation process might destroy also other molecules including signaling relevant molecules as ROS or SA. After the interaction of R protein with its counterpart Avr, R protein is protected against this autophagy-dependent degradation and can interact with downstream components and trigger ETI. This model may be valid also for indirect R-Avr interactions; e.g., the proposed R protein guard function (reviewed e. g. in Spoel and Dong, 2012) could be based on the avoidance of this negative regulation after the recognition of the changed status of the guardee. It should be stressed that we certainly expect other ways of regulations of R proteins to exist, such as a switch from inactive to active state of R protein upon Avr recognition, as well as other ways of negative regulation.
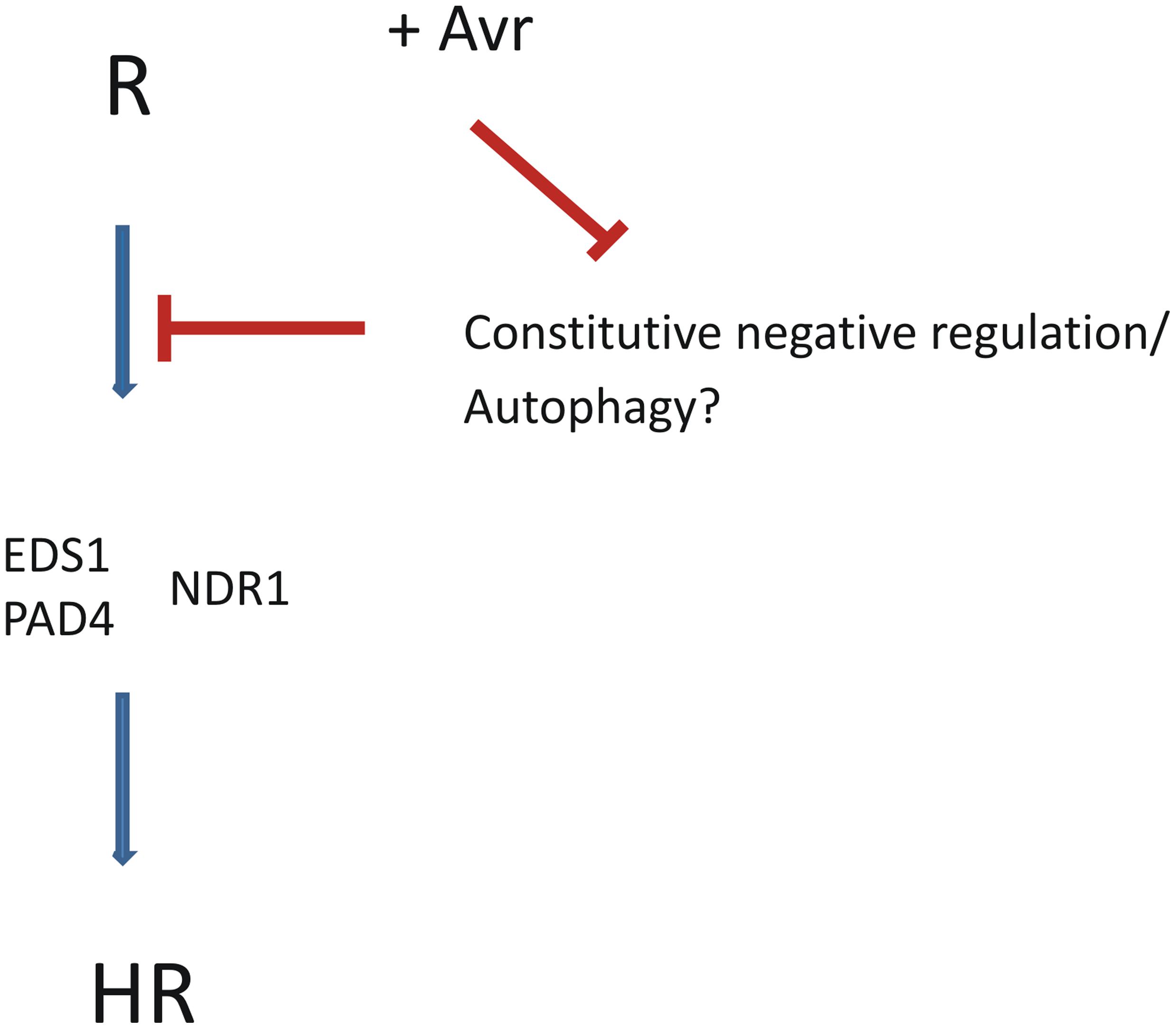
FIGURE 1. The plant constitutively negatively regulates R protein function. The interaction R-Avr serves mainly to release this negative regulation. The important component of negative regulation is autophagy; when it is non-efficient, R proteins spontaneously over activate defense responses.
Based on our hypothesis one would expect that autophagy mutants should copy the phenotype of exo70B1 mutant, having at least some R proteins constitutively activated. While some of the mutants in the autophagy pathway indeed show similar phenotypic deviations (e.g., early senescence and yellowing, sensitivity to starvation, as well as SA hyperaccumulation in atg2 and atg5 mutants; Yoshimoto et al., 2009; Wang et al., 2011), others seem to display only early senescence phenotype and cell death phenotypes only after starvation induction (like atg7 mutant). It also seems that some subunits of autophagy machinery might be more important for the negative regulation of the immunity, while others, e.g., ATG7 and ATG9, in the execution of HR (Hofius et al., 2009; Minina et al., 2014). It should be, however, noted that autophagy proteins (and EXO70B1) have been also implicated in diverse cellular processes independently of their roles in autophagy.
We also expect that, pathogen effectors might have evolved to manipulate and hijack this negative regulation and worsen the plant defense – recently, a Phytophtora infestans effector PexRD54 has been shown to outcompete the autophagy cargo receptor Joka and enhance virulence of this pathogen. Interestingly, PexRD54 does this probably through the activation of selective autophagy. Joka could participate in the removal of plant or pathogen molecules that negatively affect host defenses. As authors of the study speculate, PexRD54 would thus counteract the positive role of Joka2-mediated selective autophagy in pathogen defense. An alternative, but not exclusive explanation based on our hypothesis would be that PexRD54 at the same time stimulates the selective autophagy of R proteins capable of detecting it and thus promotes pathogen virulence (Dagdas et al., 2016). Already the report of Engelhardt et al. (2012) demonstrated the capability of cytoplasmic R protein to be recruited to endomembranes, but not for degradation, rather for the purpose of activation. However, this is not exclusive with our model – the interaction of R3A and Avr3A might release the negative regulation of R3A and switch on the HR. This interaction is obviously indirect and requires an intermediate connected to ARA6/ARA7 marked endosomes. It is possible that this activation evolved from the mechanisms of negative regulation. More information on R3A and Avr3A interactors could help to solve this ambivalent situation.
We found an indirect support for our hypothesis in the autopagy-related events described for mammalian cells – it is known from experiments performed on HeLa cells that endocytosed plasma membrane contributes to ATG12–ATG5-ATG16L1-positive/ATG8-negative phagophore precursor vesicles by both clathrin-dependent and -independent routes (Moreau and Rubinsztein, 2012). The subsequent maturation of these small phagophore precursors into phagophores (ATG12–ATG5-ATG16L1-positive/ATG8-positive) is assisted by SNARE-mediated homotypic fusion that increases their size. Additionally, Arabidopsis BON1/2/3 belong to copine proteins, a family of ubiquitous Ca(2+)-dependent, phospholipid-binding proteins that are known to be involved in animal membrane trafficking events (Tomsig and Creutz, 2002), and in Dictyostelium localize to plasma membrane, contractile vacuoles, organelles of the endolysosomal pathway, and phagosomes (Damer et al., 2005). Therefore, besides confirmed role of EXO70B1 in autophagy and regulation of TN2 activity, very probably SNARE and BON proteins could implement similar role in autophagy-related membrane targeting and membrane fusion events leading to the negative control of R proteins.
We found further support for this hypothesis in the connection between assumed autophagy regulating proteins and R proteins, as well as other key molecules of the both PTI and ETI immune response, in the web of protein-protein interactions that are available in Biogrid and PPIN databases (Stark et al., 2006; Mukhtar et al., 2011; Figure 2; Table 1). The components of PAMP-sensing complexes interact with RIN4, which further interacts with R proteins. Mainly through mediating kinase BAK1, they are connected and interact as well with BON1, BON2 and BAP. RIN4 interacts with R proteins as well as with EXO70B1 (Afzal et al., 2013). Besides its capability to interact with other exocyst and SNARE proteins, EXO70B1, together with 20 other paralogs of Arabidopsis EXO70 exocyst subunits, possess ATG8 interacting motives, which indicates that the autophagy machinery and exocyst complex functions are multiply connected (Cvrčková and Zárský, 2013; Tzfadia and Galili, 2013; Sabol et al., in preparation). Thus, in the vicinity of plasma membrane, and depending on membrane trafficking which involves SNARE, exocyst and autophagy complex proteins, a tight control of R protein activation allows the immunity to be kept low but in a constant alert.
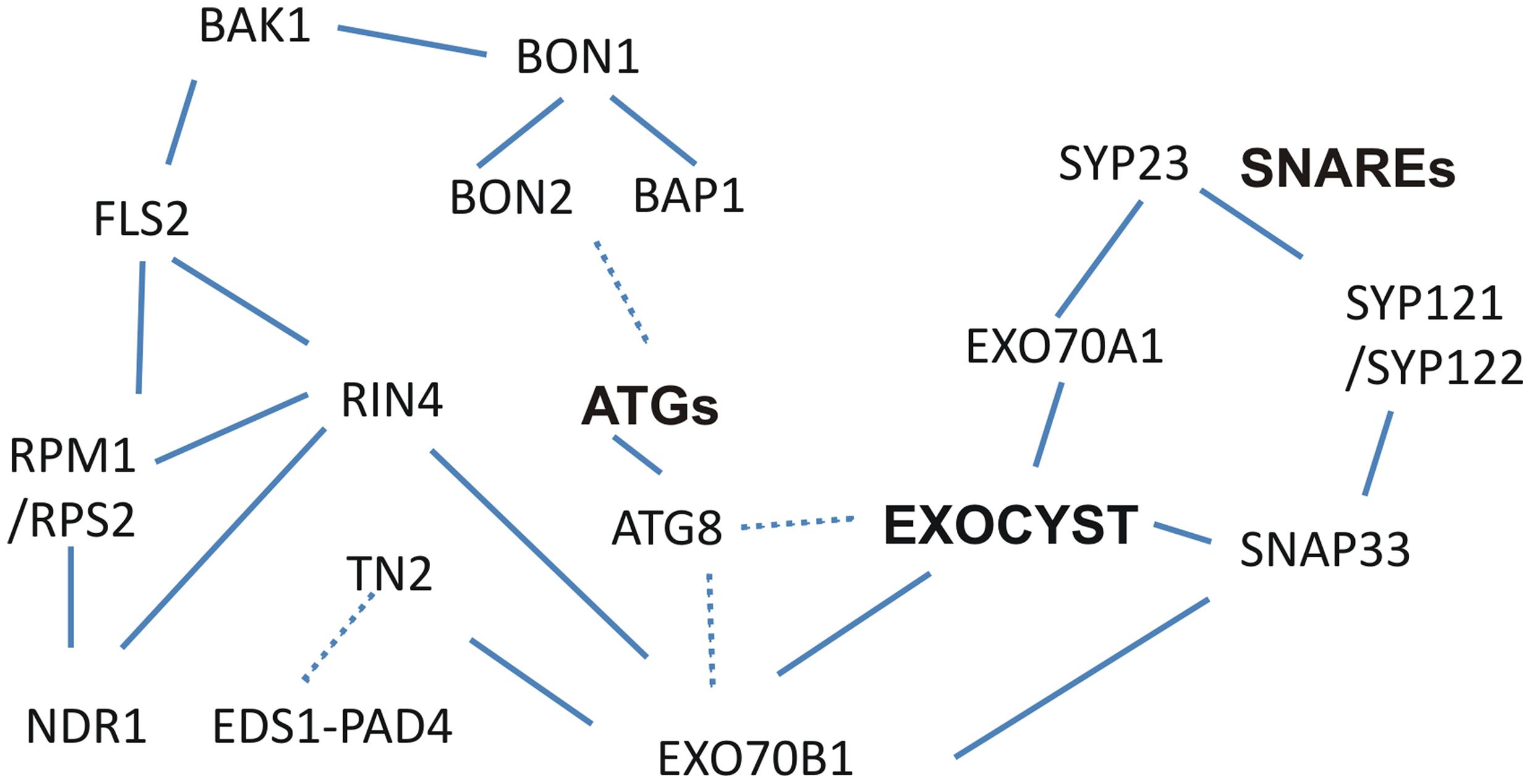
FIGURE 2. Interactions of PTI, ETI and ATG related proteins with SNAREs and exocyst. The RIN4 interacts with PTI receptor FLS2 and associated proteins, which further interact with BON1, BON2 and BAP. Additionally, RIN4 and related R-proteins may be in the same complex. R proteins and autophagy functions are connected by exocyst and SNARE interactions. (Experimentally confirmed interactions are visualized with lines, non-confirmed with dashed lines).
Recently, a role for EXO70F3 of Oryza sativa in immunity against Magnaporthe oryzae was found – OsEXO70F3 appears to play a crucial role in immunity triggered by Pii, suggesting a role for this EXO70 paralog as a decoy or helper in Pii/Avr-Pii interaction (Fujisaki et al., 2015). It may be true that pathogen effectors target these and other exocyst subunits in order to suppress defense, however, we don’t consider it to be mutually exclusive with our hypothesis.
Our model could help to better understand and reconcile conflicting aspects of autophagy in the plant immunity (Teh and Hofius, 2014): in the infection sites, R-Avr recognition prevents R protein targeting to inactivation/destruction pathway and triggers the ETI, and with the increased distance from the infection site, declining concentration of Avr protein allows the autophagy to overtake again a control over R protein. In atg mutants, the existing constitutive immunity activation results in spontaneous HR lesions formation; but after the pathogen attack, in the case of younger leaves, in addition to R protein deregulation, R is further activated by Avr recognition, which makes cells more resistant and lesions smaller. Or, under conditions with additional stresses, as in the case also of older leaves, because of coincidence between consequences of ineffective autophagy of atg mutants and Avr-enhanced over activation of R proteins, less Avr is needed for HR threshold to be crossed and lesions spread farther.
Our model’s aim is to focus on one aspect only – a possibility of a negative regulation of some NLRs/innate immunity related proteins by autophagy in plants. However, there are many difficulties that will have to be overcome in order to confirm its validity. Part of difficulties is coming from the complexity of autophagy machinery and a large number of ATG proteins that have also been implicated in diverse cellular processes independently of their roles in autophagy. Autophagy machinery is also difficult to study separately from other endomembrane compartments, especially by using pharmacological treatments. For instance, wortmannin, which is often used for these purposes, is rather pleiotropic drug – dependent on cell type and concentration it affects different types of phosphoinositide kinases, having thus multiple interference with endomembrane dynamics.
To conclude, plants have mechanisms to downregulate R proteins function, and when they are attacked by an appropriate Avr carrying pathogen, the R proteins are stabilized, activating defense responses. This would also mean that R proteins are capable of immunity activation without Avr and that the interaction R-Avr serves mainly to release R proteins negative regulation. The disturbance of the basic autophagy machinery has pleiotropic effects on many plant functions including development and is influenced by growth conditions, abiotic stresses and senescence, hence it is very difficult to study effects of atg mutants that would concern specifically defense responses. We believe that in the near future, R proteins studies will bring more information on the regulation of their activity including proteins that target them for the suggested autophagy destruction.
Author Contributions
TP did a compilation of data on R-related dwarf and lesion mimic mutants and most of the writing; PS did the RIN4-related data mining and text editing; IK explained the connection to autophagy; JO delt with membrane traficking chapter; VZ did the most of text editing and integrating as well as the finalization of the manuscript.
Funding
This work was supported by The Czech Science Foundation project No. GA15-14886S. The part of the VZ income is covered by Ministry of Education, Youth and Sports project NPU LO1417.
Conflict of Interest Statement
The authors declare that the research was conducted in the absence of any commercial or financial relationships that could be construed as a potential conflict of interest.
The reviewer TOB and handling Editor declared their shared affiliation, and the handling Editor states that the process nevertheless met the standards of a fair and objective review
Acknowledgment
Authors would like to thank Lucie Trdá and Martin Janda for the critical reading of the manuscript.
References
Afzal, A. J., da Cunha, L., and Mackey, D. (2011). Separable fragments and membrane tethering of Arabidopsis RIN4 regulate its suppression of PAMP-triggered immunity. Plant Cell 23, 3798–3811. doi: 10.1105/tpc.111.088708
Afzal, A. J., Kim, J. H., and Mackey, D. (2013). The role of NOI-domain containing proteins in plant immune signaling. BMC Genomics 14:327. doi: 10.1186/1471-2164-14-327
Anderson, J. C., Bartels, S., Gonzalez Besteiro, M. A., Shahollari, B., Ulm, R., and Peck, S. C. (2011). Arabidopsis MAP Kinase Phosphatase 1 (AtMKP1) negatively regulates MPK6-mediated PAMP responses and resistance against bacteria. Plant J. 67, 258–268. doi: 10.1111/j.1365-313X.2011.04588.x
Axtell, M. J., and Staskawicz, B. J. (2003). Initiation of RPS2-specified disease resistance in Arabidopsis is coupled to the AvrRpt2-directed elimination of RIN4. Cell 112, 369–377. doi: 10.1016/S0092-8674(03)00036-9
Azevedo, C., Sadanandom, A., Kitagawa, K., Freialdenhoven, A., Shirasu, K., and Schulze-Lefert, P. (2002). The RAR1 interactor SGT1, an essential component of R gene-triggered disease resistance. Science 295, 2073–2076. doi: 10.1126/science.1067554
Bartels, S., Anderson, J. C., Gonzalez Besteiro, M. A., Carreri, A., Hirt, H., Buchala, A., et al. (2009). MAP kinase phosphatase1 and protein tyrosine phosphatase1 are repressors of salicylic acid synthesis and SNC1-mediated responses in Arabidopsis. Plant Cell 21, 2884–2897. doi: 10.1105/tpc.109.067678
Bonardi, V., Tang, S., Stallmann, A., Roberts, M., Cherkis, K., and Dangl, J. L. (2011). Expanded functions for a family of plant intracellular immune receptors beyond specific recognition of pathogen effectors. Proc. Natl. Acad. Sci. U.S.A. 108, 16463–16468. doi: 10.1073/pnas.1113726108
Bowling, S. A., Guo, A., Cao, H., Gordon, A. S., Klessig, D. F., and Dong, X. (1994). A mutation in Arabidopsis that leads to constitutive expression of systemic acquired resistance. Plant Cell 6, 1845–1857. doi: 10.1105/tpc.6.12.1845
Brodersen, P., Petersen, M., Pike, H. M., Olszak, B., Skov, S., Odum, N., et al. (2002). Knockout of Arabidopsis accelerated-cell-death11 encoding a sphingosine transfer protein causes activation of programmed cell death and defense. Genes Dev. 16, 490–502. doi: 10.1101/gad.218202
Carviel, J. L., Al-Daoud, F., Neumann, M., Mohammad, A., Provart, N. J., Moeder, W., et al. (2009). Forward and reverse genetics to identify genes involved in the age-related resistance response in Arabidopsis thaliana. Mol. Plant Pathol. 10, 621–634. doi: 10.1111/j.1364-3703.2009.00557.x
Century, K. S., Holub, E. B., and Staskawicz, B. J. (1995). NDR1, a locus of Arabidopsis thaliana that is required for disease resistance to both a bacterial and a fungal pathogen. Proc. Natl. Acad. Sci. U.S.A. 92, 6597–6601. doi: 10.1073/pnas.92.14.6597
Century, K. S., Shapiro, A. D., Repetti, P. P., Dahlbeck, D., Holub, E., and Staskawicz, B. J. (1997). NDR1, a pathogen-induced component required for Arabidopsis disease resistance. Science 278, 1963–1965. doi: 10.1126/science.278.5345.1963
Chatre, L., Wattelet-Boyer, V., Melser, S., Maneta-Peyret, L., Brandizzi, F., and Moreau, P. (2009). A novel di-acidic motif facilitates ER export of the syntaxin SYP31. J. Exp. Bot. 60, 3157–3165. doi: 10.1093/jxb/erp155
Collier, S. M., Hamel, L. P., and Moffett, P. (2011). Cell death mediated by the N-terminal domains of a unique and highly conserved class of NB-LRR protein. Mol. Plant Microbe Interact. 24, 918–931. doi: 10.1094/MPMI-03-11-0050
Cvrčková, F., and Zárský, V. (2013). Old AIMs of the exocyst: evidence for an ancestral association of exocyst subunits with autophagy-associated Atg8 proteins. Plant Signal. Behav. 8, e27099. doi: 10.4161/psb.27099
Dagdas, Y. F., Belhaj, K., Maqbool, A., Chaparro-Garcia, A., Pandey, P., Petre, B., et al. (2016). An effector of the Irish potato famine pathogen antagonizes a host autophagy cargo receptor. Elife 5, e10856. doi: 10.7554/eLife.10856
Damer, C. K., Bayeva, M., Hahn, E. S., Rivera, J., and Socec, C. I. (2005). Copine A, a calcium-dependent membrane-binding protein, transiently localizes to the plasma membrane and intracellular vacuoles in Dictyostelium. BMC Cell Biol. 6:46. doi: 10.1186/1471-2121-6-46
Debener, T., Lehnackers, H., Arnold, M., and Dangl, J. L. (1991). Identification and molecular mapping of a single Arabidopsis thaliana locus determining resistance to a phytopathogenic Pseudomonas syringae isolate. Plant J. 1, 289–302. doi: 10.1046/j.1365-313X.1991.t01-7-00999.x
Doelling, J. H., Walker, J. M., Friedman, E. M., Thompson, A. R., and Vierstra, R. D. (2002). The APG8/12-activating enzyme APG7 is required for proper nutrient recycling and senescence in Arabidopsis thaliana. J. Biol. Chem. 277, 33105–33114. doi: 10.1074/jbc.M204630200
Engelhardt, S., Boevink, P. C., Armstrong, M. R., Ramos, M. B., Hein, I., and Birch, P. R. (2012). Relocalization of late blight resistance protein R3a to endosomal compartments is associated with effector recognition and required for the immune response. Plant Cell 24, 5142–5158. doi: 10.1105/tpc.112.104992
Feys, B. J., Moisan, L. J., Newman, M. A., and Parker, J. E. (2001). Direct interaction between the Arabidopsis disease resistance signaling proteins, EDS1 and PAD4. EMBO J. 20, 5400–5411. doi: 10.1093/emboj/20.19.5400
Feys, B. J., Wiermer, M., Bhat, R. A., Moisan, L. J., Medina-Escobar, N., Neu, C., et al. (2005). Arabidopsis SENESCENCE-ASSOCIATED GENE101 stabilizes and signals within an ENHANCED DISEASE SUSCEPTIBILITY1 complex in plant innate immunity. Plant Cell 17, 2601–2613. doi: 10.1105/tpc.105.033910
Fujiki, Y., Yoshimoto, K., and Ohsumi, Y. (2007). An Arabidopsis homolog of yeast ATG6/VPS30 is essential for pollen germination. Plant Physiol. 143, 1132–1139. doi: 10.1104/pp.106.093864
Fujisaki, K., Abe, Y., Ito, A., Saitoh, H., Yoshida, K., Kanzaki, H., et al. (2015). Rice Exo70 interacts with a fungal effector, AVR-Pii, and is required for AVR-Pii-triggered immunity. Plant J. 83, 875–887. doi: 10.1111/tpj.12934
Gao, M., Wang, X., Wang, D., Xu, F., Ding, X., Zhang, Z., et al. (2009). Regulation of cell death and innate immunity by two receptor-like kinases in Arabidopsis. Cell Host Microbe 6, 34–44. doi: 10.1016/j.chom.2009.05.019
Gomez-Gomez, L., and Boller, T. (2000). FLS2: an LRR receptor-like kinase involved in the perception of the bacterial elicitor flagellin in Arabidopsis. Mol. Cell 5, 1003–1011. doi: 10.1016/S1097-2765(00)80265-8
Gou, M., and Hua, J. (2012). Complex regulation of an R gene SNC1 revealed by auto-immune mutants. Plant Signal. Behav. 7, 213–216. doi: 10.4161/psb.18884
Gou, M., Shi, Z., Zhu, Y., Bao, Z., Wang, G., and Hua, J. (2012). The F-box protein CPR1/CPR30 negatively regulates R protein SNC1 accumulation. Plant J. 69, 411–420. doi: 10.1111/j.1365-313X.2011.04799.x
Grant, J. J., Chini, A., Basu, D., and Loake, G. J. (2003). Targeted activation tagging of the Arabidopsis NBS-LRR gene, ADR1, conveys resistance to virulent pathogens. Mol. Plant Microbe Interact. 16, 669–680. doi: 10.1094/MPMI.2003.16.8.669
Hanaoka, H., Noda, T., Shirano, Y., Kato, T., Hayashi, H., Shibata, D., et al. (2002). Leaf senescence and starvation-induced chlorosis are accelerated by the disruption of an Arabidopsis autophagy gene. Plant Physiol. 129, 1181–1193. doi: 10.1104/pp.011024
He, Y., and Gan, S. (2002). A gene encoding an acyl hydrolase is involved in leaf senescence in Arabidopsis. Plant Cell 14, 805–815. doi: 10.1105/tpc.010422
Hofius, D., Schultz-Larsen, T., Joensen, J., Tsitsigiannis, D. I., Petersen, N. H., Mattsson, O., et al. (2009). Autophagic components contribute to hypersensitive cell death in Arabidopsis. Cell 137, 773–783. doi: 10.1016/j.cell.2009.02.036
Hua, J., Grisafi, P., Cheng, S. H., and Fink, G. R. (2001). Plant growth homeostasis is controlled by the Arabidopsis BON1 and BAP1 genes. Genes Dev. 15, 2263–2272. doi: 10.1101/gad.918101
Huang, X., Li, J., Bao, F., Zhang, X., and Yang, S. (2010). A gain-of-function mutation in the Arabidopsis disease resistance gene RPP4 confers sensitivity to low temperature. Plant Physiol. 154, 796–809. doi: 10.1104/pp.110.157610
Jacob, F., Vernaldi, S., and Maekawa, T. (2013). Evolution and conservation of plant NLR functions. Front. Immunol. 4:297. doi: 10.3389/fimmu.2013.00297
Janda, M., and Ruelland, E. (2014). Magical mystery tour: salicylic acid signaling. Environ. Exp. Bot. 114, 117–128. doi: 10.1016/j.envexpbot.2014.07.003
Jirage, D., Tootle, T. L., Reuber, T. L., Frost, L. N., Feys, B. J., Parker, J. E., et al. (1999). Arabidopsis thaliana PAD4 encodes a lipase-like gene that is important for salicylic acid signaling. Proc. Natl. Acad. Sci. U.S.A. 96, 13583–13588. doi: 10.1073/pnas.96.23.13583
Jones, J. D., and Dangl, J. L. (2006). The plant immune system. Nature 444, 323–329. doi: 10.1038/nature05286
Kato, H., Saito, T., Ito, H., Komeda, Y., and Kato, A. (2014). Overexpression of the TIR-X gene results in a dwarf phenotype and activation of defense-related gene expression in Arabidopsis thaliana. J. Plant Physiol. 171, 382–388. doi: 10.1016/j.jplph.2013.12.002
Ketelaar, T., Voss, C., Dimmock, S. A., Thumm, M., and Hussey, P. J. (2004). Arabidopsis homologues of the autophagy protein Atg8 are a novel family of microtubule binding proteins. FEBS Lett. 567, 302–306. doi: 10.1016/j.febslet.2004.04.088
Kim, H. S., Desveaux, D., Singer, A. U., Patel, P., Sondek, J., and Dangl, J. L. (2005). The Pseudomonas syringae effector AvrRpt2 cleaves its C-terminally acylated target, RIN4, from Arabidopsis membranes to block RPM1 activation. Proc. Natl. Acad. Sci. U.S.A. 102, 6496–6501. doi: 10.1073/pnas.0500792102
Kim, S. H., Gao, F., Bhattacharjee, S., Adiasor, J. A., Nam, J. C., and Gassmann, W. (2010). The Arabidopsis resistance-like gene SNC1 is activated by mutations in SRFR1 and contributes to resistance to the bacterial effector AvrRps4. PLoS Pathog. 6:e1001172. doi: 10.1371/journal.ppat.1001172
Kliebenstein, D. J., Dietrich, R. A., Martin, A. C., Last, R. L., and Dangl, J. L. (1999). LSD1 regulates salicylic acid induction of copper zinc superoxide dismutase in Arabidopsis thaliana. Mol. Plant Microbe Interact. 12, 1022–1026. doi: 10.1094/MPMI.1999.12.11.1022
Kong, Q., Qu, N., Gao, M., Zhang, Z., Ding, X., Yang, F., et al. (2012). The MEKK1-MKK1/MKK2-MPK4 kinase cascade negatively regulates immunity mediated by a mitogen-activated protein kinase kinase kinase in Arabidopsis. Plant Cell 24, 2225–2236. doi: 10.1105/tpc.112.097253
Kreibich, S., Emmenlauer, M., Fredlund, J., Ramo, P., Munz, C., Dehio, C., et al. (2015). Autophagy proteins promote repair of endosomal membranes damaged by the Salmonella type three secretion system 1. Cell Host Microbe 18, 527–537. doi: 10.1016/j.chom.2015.10.015
Kulich, I., Pecenkova, T., Sekeres, J., Smetana, O., Fendrych, M., Foissner, I., et al. (2013). Arabidopsis exocyst subcomplex containing subunit EXO70B1 is involved in autophagy-related transport to the vacuole. Traffic 14, 1155–1165. doi: 10.1111/tra.12101
Kulich, I., and Zarsky, V. (2014). Autophagy-related direct membrane import from ER/cytoplasm into the vacuole or apoplast: a hidden gateway also for secondary metabolites and phytohormones? Int. J. Mol. Sci. 15, 7462–7474. doi: 10.3390/ijms15057462
Levine, B., and Klionsky, D. J. (2004). Development by self-digestion: molecular mechanisms and biological functions of autophagy. Dev. Cell 6, 463–477. doi: 10.1016/S1534-5807(04)00099-1
Li, F., and Vierstra, R. D. (2012). Regulator and substrate: dual roles for the ATG1-ATG13 kinase complex during autophagic recycling in Arabidopsis. Autophagy 8, 982–984. doi: 10.4161/auto.20240
Li, J., Wen, J., Lease, K. A., Doke, J. T., Tax, F. E., and Walker, J. C. (2002). BAK1, an Arabidopsis LRR receptor-like protein kinase, interacts with BRI1 and modulates brassinosteroid signaling. Cell 110, 213–222. doi: 10.1016/S0092-8674(02)00812-7
Li, Y., Pennington, B. O., and Hua, J. (2009). Multiple R-like genes are negatively regulated by BON1 and BON3 in Arabidopsis. Mol. Plant Microbe Interact. 22, 840–848. doi: 10.1094/MPMI-22-7-0840
Li, Y., Tessaro, M. J., Li, X., and Zhang, Y. (2010). Regulation of the expression of plant resistance gene SNC1 by a protein with a conserved BAT2 domain. Plant Physiol. 153, 1425–1434. doi: 10.1104/pp.110.156240
Liu, J., Liu, X., Dai, L., and Wang, G. (2007). Recent progress in elucidating the structure, function and evolution of disease resistance genes in plants. J. Genet. Genomics 34, 765–776. doi: 10.1016/S1673-8527(07)60087-3
Liu, Y., Schiff, M., Czymmek, K., Talloczy, Z., Levine, B., and Dinesh-Kumar, S. P. (2005). Autophagy regulates programmed cell death during the plant innate immune response. Cell 121, 567–577. doi: 10.1016/j.cell.2005.03.007
Lorrain, S., Vailleau, F., Balague, C., and Roby, D. (2003). Lesion mimic mutants: keys for deciphering cell death and defense pathways in plants? Trends Plant Sci. 8, 263–271. doi: 10.1016/S1360-1385(03)00108-0
Mackey, D., Belkhadir, Y., Alonso, J. M., Ecker, J. R., and Dangl, J. L. (2003). Arabidopsis RIN4 is a target of the type III virulence effector AvrRpt2 and modulates RPS2-mediated resistance. Cell 112, 379–389. doi: 10.1016/S0092-8674(03)00040-0
Mackey, D., Holt, B. F. III, Wiig, A., and Dangl, J. L. (2002). RIN4 interacts with Pseudomonas syringae type III effector molecules and is required for RPM1-mediated resistance in Arabidopsis. Cell 108, 743–754. doi: 10.1016/S0092-8674(02)00661-X
Martin, G. B. (1999). Functional analysis of plant disease resistance genes and their downstream effectors. Curr. Opin. Plant Biol. 2, 273–279. doi: 10.1016/S1369-5266(99)80049-1
McDowell, J. M., and Woffenden, B. J. (2003). Plant disease resistance genes: recent insights and potential applications. Trends Biotechnol. 21, 178–183. doi: 10.1016/S0167-7799(03)00053-2
Meyers, B. C., Kozik, A., Griego, A., Kuang, H., and Michelmore, R. W. (2003). Genome-wide analysis of NBS-LRR-encoding genes in Arabidopsis. Plant Cell 15, 809–834. doi: 10.1105/tpc.009308
Minina, E. A., Bozhkov, P. V., and Hofius, D. (2014). Autophagy as initiator or executioner of cell death. Trends Plant Sci. 19, 692–697. doi: 10.1016/j.tplants.2014.07.007
Moreau, K., and Rubinsztein, D. C. (2012). The plasma membrane as a control center for autophagy. Autophagy 8, 861–863. doi: 10.4161/auto.20060
Mukhtar, M. S., Carvunis, A. R., Dreze, M., Epple, P., Steinbrenner, J., Moore, J., et al. (2011). Independently evolved virulence effectors converge onto hubs in a plant immune system network. Science 333, 596–601. doi: 10.1126/science.1203659
Munch, D., Teh, O. K., Malinovsky, F. G., Liu, Q., Vetukuri, R. R., El Kasmi, F., et al. (2015). Retromer contributes to immunity-associated cell death in Arabidopsis. Plant Cell 27, 463–479. doi: 10.1105/tpc.114.132043
Nandety, R. S., Caplan, J. L., Cavanaugh, K., Perroud, B., Wroblewski, T., Michelmore, R. W., et al. (2013). The role of TIR-NBS and TIR-X proteins in plant basal defense responses. Plant Physiol. 162, 1459–1472. doi: 10.1104/pp.113.219162
Nawrath, C., and Metraux, J. P. (1999). Salicylic acid induction-deficient mutants of Arabidopsis express PR-2 and PR-5 and accumulate high levels of camalexin after pathogen inoculation. Plant Cell 11, 1393–1404. doi: 10.2307/3870970
Noutoshi, Y., Ito, T., Seki, M., Nakashita, H., Yoshida, S., Marco, Y., et al. (2005). A single amino acid insertion in the WRKY domain of the Arabidopsis TIR-NBS-LRR-WRKY-type disease resistance protein SLH1 (sensitive to low humidity 1) causes activation of defense responses and hypersensitive cell death. Plant J. 43, 873–888. doi: 10.1111/j.1365-313X.2005.02500.x
Ohtomo, I., Ueda, H., Shimada, T., Nishiyama, C., Komoto, Y., Hara-Nishimura, I., et al. (2005). Identification of an allele of VAM3/SYP22 that confers a semi-dwarf phenotype in Arabidopsis thaliana. Plant Cell Physiol. 46, 1358–1365. doi: 10.1093/pcp/pci146
Palma, K., Thorgrimsen, S., Malinovsky, F. G., Fiil, B. K., Nielsen, H. B., Brodersen, P., et al. (2010). Autoimmunity in Arabidopsis acd11 is mediated by epigenetic regulation of an immune receptor. PLoS Pathog. 6:e1001137. doi: 10.1371/journal.ppat.1001137
Patel, S., and Dinesh-Kumar, S. P. (2008). Arabidopsis ATG6 is required to limit the pathogen-associated cell death response. Autophagy 4, 20–27. doi: 10.4161/auto.5056
Petersen, M., Brodersen, P., Naested, H., Andreasson, E., Lindhart, U., Johansen, B., et al. (2000). Arabidopsis map kinase 4 negatively regulates systemic acquired resistance. Cell 103, 1111–1120. doi: 10.1016/S0092-8674(00)00213-0
Pontier, D., Balague, C., and Roby, D. (1998). The hypersensitive response. A programmed cell death associated with plant resistance. C. R. Acad. Sci. III 321, 721–734. doi: 10.1016/S0764-4469(98)80013-9
Qi, Y., Tsuda, K., Glazebrook, J., and Katagiri, F. (2011). Physical association of pattern-triggered immunity (PTI) and effector-triggered immunity (ETI) immune receptors in Arabidopsis. Mol. Plant Pathol. 12, 702–708. doi: 10.1111/j.1364-3703.2010.00704.x
Qiu, J. L., Zhou, L., Yun, B. W., Nielsen, H. B., Fiil, B. K., Petersen, K., et al. (2008). Arabidopsis mitogen-activated protein kinase kinases MKK1 and MKK2 have overlapping functions in defense signaling mediated by MEKK1, MPK4, and MKS1. Plant Physiol. 148, 212–222. doi: 10.1104/pp.108.120006
Reggiori, F., and Klionsky, D. J. (2013). Autophagic processes in yeast: mechanism, machinery and regulation. Genetics 194, 341–361. doi: 10.1534/genetics.112.149013
Roberts, M., Tang, S., Stallmann, A., Dangl, J. L., and Bonardi, V. (2013). Genetic requirements for signaling from an autoactive plant NB-LRR intracellular innate immune receptor. PLoS Genet. 9:e1003465. doi: 10.1371/journal.pgen.1003465
Rogers, E. E., and Ausubel, F. M. (1997). Arabidopsis enhanced disease susceptibility mutants exhibit enhanced susceptibility to several bacterial pathogens and alterations in PR-1 gene expression. Plant Cell 9, 305–316. doi: 10.1105/tpc.9.3.305
Sasek, V., Janda, M., Delage, E., Puyaubert, J., Guivarc’h, A., Lopez Maseda, E., et al. (2014). Constitutive salicylic acid accumulation in pi4kIIIbeta1beta2 Arabidopsis plants stunts rosette but not root growth. New Phytol. 203, 805–816. doi: 10.1111/nph.12822
Sekine, K. T., Nandi, A., Ishihara, T., Hase, S., Ikegami, M., Shah, J., et al. (2004). Enhanced resistance to Cucumber mosaic virus in the Arabidopsis thaliana ssi2 mutant is mediated via an SA-independent mechanism. Mol. Plant Microbe Interact. 17, 623–632. doi: 10.1094/MPMI.2004.17.6.623
Shirano, Y., Kachroo, P., Shah, J., and Klessig, D. F. (2002). A gain-of-function mutation in an Arabidopsis Toll Interleukin1 receptor-nucleotide binding site-leucine-rich repeat type R gene triggers defense responses and results in enhanced disease resistance. Plant Cell 14, 3149–3162. doi: 10.1105/tpc.005348
Spoel, S. H., and Dong, X. (2012). How do plants achieve immunity? Defence without specialized immune cells. Nat. Rev. Immunol. 12, 89–100. doi: 10.1038/nri3141
Stark, C., Breitkreutz, B. J., Reguly, T., Boucher, L., Breitkreutz, A., and Tyers, M. (2006). BioGRID: a general repository for interaction datasets. Nucleic Acids Res. 34, D535–D539. doi: 10.1093/nar/gkj109
Stegmann, M., Anderson, R. G., Westphal, L., Rosahl, S., McDowell, J. M., and Trujillo, M. (2013). The exocyst subunit Exo70B1 is involved in the immune response of Arabidopsis thaliana to different pathogens and cell death. Plant Signal. Behav. 8, e27421. doi: 10.4161/psb.27421
Synek, L., Schlager, N., Elias, M., Quentin, M., Hauser, M. T., and Zarsky, V. (2006). AtEXO70A1, a member of a family of putative exocyst subunits specifically expanded in land plants, is important for polar growth and plant development. Plant J. 48, 54–72. doi: 10.1111/j.1365-313X.2006.02854.x
Takemoto, D., and Jones, D. A. (2005). Membrane release and destabilization of Arabidopsis RIN4 following cleavage by Pseudomonas syringae AvrRpt2. Mol. Plant Microbe Interact. 18, 1258–1268. doi: 10.1094/MPMI-18-1258
Teh, O. K., and Hofius, D. (2014). Membrane trafficking and autophagy in pathogen-triggered cell death and immunity. J. Exp. Bot. 65, 1297–1312. doi: 10.1093/jxb/ert441
Thompson, A. R., Doelling, J. H., Suttangkakul, A., and Vierstra, R. D. (2005). Autophagic nutrient recycling in Arabidopsis directed by the ATG8 and ATG12 conjugation pathways. Plant Physiol. 138, 2097–2110. doi: 10.1104/pp.105.060673
Tomsig, J. L., and Creutz, C. E. (2002). Copines: a ubiquitous family of Ca(2+)-dependent phospholipid-binding proteins. Cell. Mol. Life Sci. 59, 1467–1477. doi: 10.1007/s00018-002-8522-7
Trujillo, M., Ichimura, K., Casais, C., and Shirasu, K. (2008). Negative regulation of PAMP-triggered immunity by an E3 ubiquitin ligase triplet in Arabidopsis. Curr. Biol. 18, 1396–1401. doi: 10.1016/j.cub.2008.07.085
Tzfadia, O., and Galili, G. (2013). The Arabidopsis exocyst subcomplex subunits involved in a golgi-independent transport into the vacuole possess consensus autophagy-associated atg8 interacting motifs. Plant Signal. Behav. 8, e26732-1–e26732-3. doi: 10.4161/psb.26732
Vlot, A. C., Liu, P. P., Cameron, R. K., Park, S. W., Yang, Y., Kumar, D., et al. (2008). Identification of likely orthologs of tobacco salicylic acid-binding protein 2 and their role in systemic acquired resistance in Arabidopsis thaliana. Plant J. 56, 445–456. doi: 10.1111/j.1365-313X.2008.03618.x
Vogelmann, K., Drechsel, G., Bergler, J., Subert, C., Philippar, K., Soll, J., et al. (2012). Early senescence and cell death in Arabidopsis saul1 mutants involves the PAD4-dependent salicylic acid pathway. Plant Physiol. 159, 1477–1487. doi: 10.1104/pp.112.196220
Wagner, S., Stuttmann, J., Rietz, S., Guerois, R., Brunstein, E., Bautor, J., et al. (2013). Structural basis for signaling by exclusive EDS1 heteromeric complexes with SAG101 or PAD4 in plant innate immunity. Cell Host Microbe 14, 619–630. doi: 10.1016/j.chom.2013.11.006
Wang, Y., Nishimura, M. T., Zhao, T., and Tang, D. (2011). ATG2, an autophagy-related protein, negatively affects powdery mildew resistance and mildew-induced cell death in Arabidopsis. Plant J. 68, 74–87. doi: 10.1111/j.1365-313X.2011.04669.x
Yang, H., Shi, Y., Liu, J., Guo, L., Zhang, X., and Yang, S. (2010). A mutant CHS3 protein with TIR-NB-LRR-LIM domains modulates growth, cell death and freezing tolerance in a temperature-dependent manner in Arabidopsis. Plant J. 63, 283–296. doi: 10.1111/j.1365-313X.2010.04241.x
Yang, S., and Hua, J. (2004). A haplotype-specific Resistance gene regulated by BONZAI1 mediates temperature-dependent growth control in Arabidopsis. Plant Cell 16, 1060–1071. doi: 10.1105/tpc.020479
Yang, S., Yang, H., Grisafi, P., Sanchatjate, S., Fink, G. R., Sun, Q., et al. (2006). The BON/CPN gene family represses cell death and promotes cell growth in Arabidopsis. Plant J. 45, 166–179. doi: 10.1111/j.1365-313X.2005.02585.x
Yoshimoto, K., Hanaoka, H., Sato, S., Kato, T., Tabata, S., Noda, T., et al. (2004). Processing of ATG8s, ubiquitin-like proteins, and their deconjugation by ATG4s are essential for plant autophagy. Plant Cell 16, 2967–2983. doi: 10.1105/tpc.104.025395
Yoshimoto, K., Jikumaru, Y., Kamiya, Y., Kusano, M., Consonni, C., Panstruga, R., et al. (2009). Autophagy negatively regulates cell death by controlling NPR1-dependent salicylic acid signaling during senescence and the innate immune response in Arabidopsis. Plant Cell 21, 2914–2927. doi: 10.1105/tpc.109.068635
Yu, G. L., Katagiri, F., and Ausubel, F. M. (1993). Arabidopsis mutations at the RPS2 locus result in loss of resistance to Pseudomonas syringae strains expressing the avirulence gene avrRpt2. Mol. Plant Microbe Interact. 6, 434–443. doi: 10.1094/MPMI-6-434
Yu, I. C., Parker, J., and Bent, A. F. (1998). Gene-for-gene disease resistance without the hypersensitive response in Arabidopsis dnd1 mutant. Proc. Natl. Acad. Sci. U.S.A. 95, 7819–7824. doi: 10.1073/pnas.95.13.7819
Zhang, Y., Goritschnig, S., Dong, X., and Li, X. (2003). A gain-of-function mutation in a plant disease resistance gene leads to constitutive activation of downstream signal transduction pathways in suppressor of npr1-1, constitutive 1. Plant Cell 15, 2636–2646. doi: 10.1105/tpc.015842
Zhang, Z., Lenk, A., Andersson, M. X., Gjetting, T., Pedersen, C., Nielsen, M. E., et al. (2008). A lesion-mimic syntaxin double mutant in Arabidopsis reveals novel complexity of pathogen defense signaling. Mol. Plant 1, 510–527. doi: 10.1093/mp/ssn011
Zhang, Z., Wu, Y., Gao, M., Zhang, J., Kong, Q., Liu, Y., et al. (2012). Disruption of PAMP-induced MAP kinase cascade by a Pseudomonas syringae effector activates plant immunity mediated by the NB-LRR protein SUMM2. Cell Host Microbe 11, 253–263. doi: 10.1016/j.chom.2012.01.015
Zhao, T., Rui, L., Li, J., Nishimura, M. T., Vogel, J. P., Liu, N., et al. (2015). A truncated NLR protein, TIR-NBS2, is required for activated defense responses in the exo70B1 mutant. PLoS Genet. 11:e1004945. doi: 10.1371/journal.pgen.1004945
Zhou, F., Menke, F. L., Yoshioka, K., Moder, W., Shirano, Y., and Klessig, D. F. (2004). High humidity suppresses ssi4-mediated cell death and disease resistance upstream of MAP kinase activation, H2O2 production and defense gene expression. Plant J. 39, 920–932. doi: 10.1111/j.1365-313X.2004.02180.x
Keywords: resistance, autophagy, R, Avr, ETI, dwarf, lesions, exocyst
Citation: Pečenková T, Sabol P, Kulich I, Ortmannová J and Žárský V (2016) Constitutive Negative Regulation of R Proteins in Arabidopsis also Via Autophagy Related Pathway? Front. Plant Sci. 7:260. doi: 10.3389/fpls.2016.00260
Received: 25 November 2015; Accepted: 18 February 2016;
Published: 04 March 2016.
Edited by:
Pietro Daniele Spanu, Imperial College London, UKReviewed by:
Mario Serrano, Universidad Nacional Autónoma de México, MexicoTolga Osman Bozkurt, Imperial College London, UK
Copyright © 2016 Pečenková, Sabol, Kulich, Ortmannová and Žárský. This is an open-access article distributed under the terms of the Creative Commons Attribution License (CC BY). The use, distribution or reproduction in other forums is permitted, provided the original author(s) or licensor are credited and that the original publication in this journal is cited, in accordance with accepted academic practice. No use, distribution or reproduction is permitted which does not comply with these terms.
*Correspondence: Tamara Pečenková, cGVjZW5rb3ZhQHVlYi5jYXMuY3o=