- Laboratoire de Biochimie et Physiologie Moléculaire des Plantes, Institut de Biologie Intégrative des Plantes “Claude Grignon”, UMR CNRS/INRA/SupAgro/UM, Montpellier, France
Most plants use nitrate (NO3-) as their major nitrogen (N) source. The NO3- uptake capacity of a plant is determined by three interdependent factors that are sensitive to NO3- availability: (i) the functional properties of the transporters in roots that contribute to the acquisition of NO3- from the external medium, (ii) the density of functional transporters at the plasma membrane of root cells, and (iii) the surface and architecture of the root system. The identification of factors that regulate the NO3--sensing systems is important for both fundamental and applied science, because these factors control the capacity of plants to use the available NO3-, a process known as the “nitrate use efficiency.” The molecular component of the transporters involved in uptake and sensing mechanism in Arabidopsis roots are presented and their relative contribution discussed.
Introduction
Nitrate (NO3-) is an essential source of nitrogen for plant development and metabolism. With ammonium (NH4+) and urea (CO(NH2)2), it is the most used fertilizer in agriculture as a source of nitrogen (N) (Kiba and Krapp, 2016). But, beside its role as a nutrient, nitrate is also a signal molecule which is involved in the control of many physiological processes, plant growth and crop yield (Crawford, 1995; Krapp et al., 2014; Vidal et al., 2014). For example, as other nutrients like phosphate or ammonium (Drew et al., 1973; Drew and Saker, 1975), nitrate participates in the regulation of lateral roots development and architecture (Remans et al., 2006a; Walch-Liu et al., 2006b; Krouk et al., 2010a; Ruffel et al., 2011, 2014, 2016), leaf development (Chiu et al., 2004), flowering induction (Castro Marin et al., 2011) and seed dormancy (Alboresi et al., 2005). As a signal molecule, nitrate can induce the expression of a number of genes implicated in nitrate transport and assimilation (Wang et al., 2004; Bi et al., 2007; Krouk et al., 2011; Medici and Krouk, 2014).
Nitrate is often a limited resource and its accessibility is modified in both time and space, therefore plants must adapt nitrate inputs to the needs and availability in the soil. Nitrate can be assimilated in the roots or translocated to aerial organs via the xylem. Nitrate is then reduced to nitrite by nitrate reductase (NR) and further to ammonium by nitrite reductase (NiR) before incorporation in amino acids (Stitt, 1999). Otherwise, nitrate could also be stored, mainly in the vacuoles where concentration could vary from 5 mM to 75 mM (measured in roots of barley seedlings; Miller and Smith, 2008), in roots or shoots for further remobilization when nitrate availability became scarce.
To maximize uptake efficiency in a wide range of external nitrate concentration, plants own transport system with different properties to adjust nitrate uptake capacity (Miller et al., 2007); two types of transport systems known as Low Affinity Transport Systems (LATS) and High Affinity Transport Systems (HATS). The LATS allows transport in high (> 0.5 mM) external nitrate concentration whereas the HATS provide a capacity for nitrate absorption at low (< 0.5 mM) external nitrate concentrations. Within each of these transport systems both constitutive (c) and inducible (i) forms co-exist. Expression of these four kinds of transport systems is essential for an efficient uptake, and expression could be constitutive or inducible function of the external nitrate concentration perceived.
Four families of transporters participate in nitrate uptake, distribution or storage: NITRATE TRANSPORTER 2 (NRT2) transporters (Orsel et al., 2002; Krapp et al., 2014), NITRATE TRANSPORTER 1/PEPTIDE TRANSPORTER FAMILY (NPF) transporters (Léran et al., 2014), CHLORIDE CHANNEL FAMILY (CLC) transporters (Barbier-Brygoo et al., 2011) and SLOW ANION ASSOCIATED CHANNEL HOMOLOG (SLAC/SLAH) (Negi et al., 2008). Because roots constitute the main organ where exchange between plant and its environment take place, this review focuses on transporters identified in Arabidopsis root plasma membrane and contributing to nitrate uptake from the soil (Figure 1). Transporters from the NPF and NRT2 families are involved and interestingly some of these transporters are also involved in nitrate sensing.
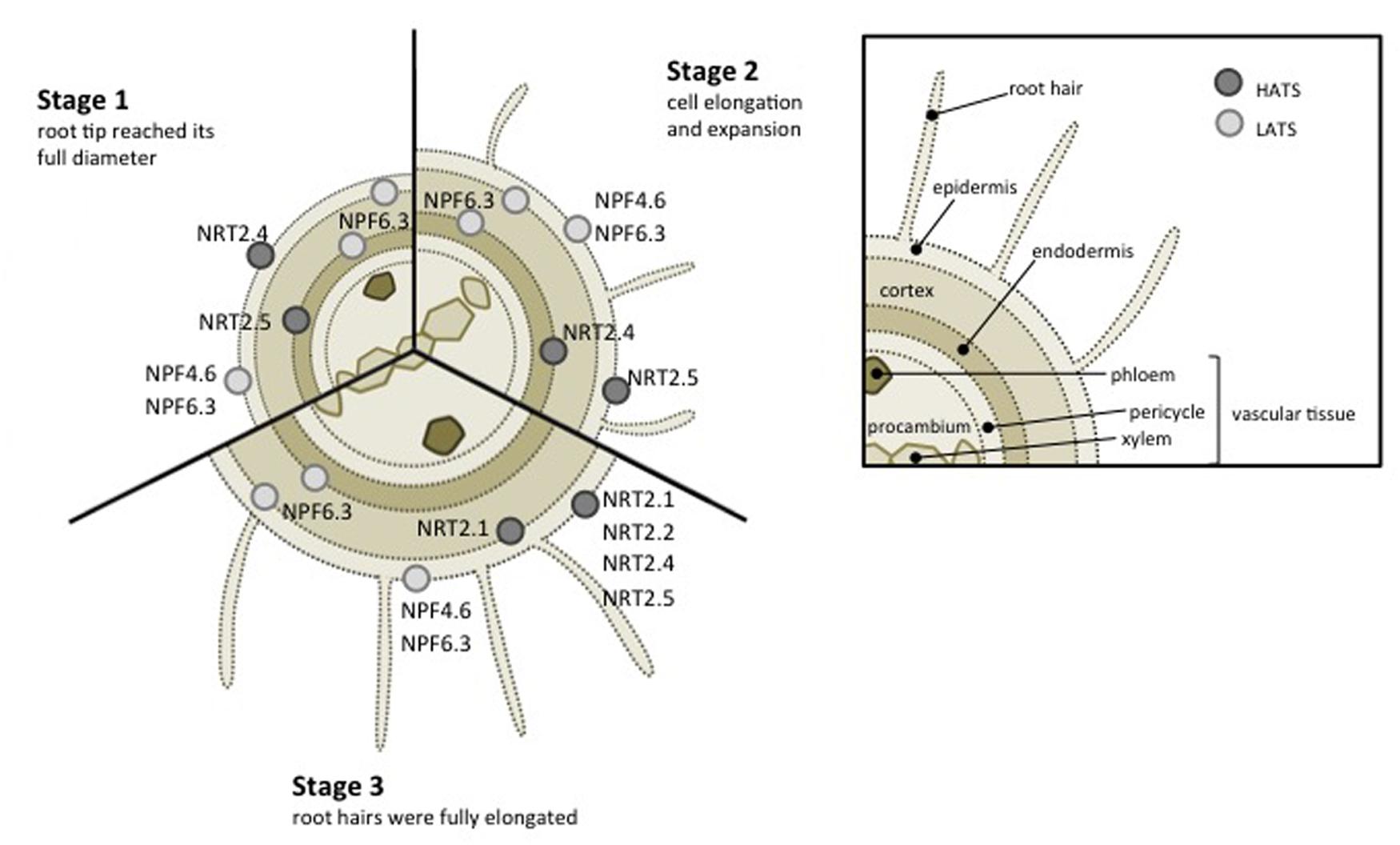
FIGURE 1. Schematic representation of low- (light gray dots) and high- (dark gray dots) affinity nitrate transporters expressed in Arabidopsis root. Three stages of root developement have been represented according to Birnbaum et al. (2003).
High-Affinity Nitrate Transporter: NRT2 Family
Seven members have been described in the NRT2 family in Arabidopsis and characterized as high-affinity nitrate transporters (Krapp et al., 2014). The affinity for nitrate of these transporters is in the range of microM, saturable around 0.2–0.5 milliM. The role in nitrate influx in the root has been demonstrated for four of them: NRT2.1, NRT2.2, NRT2.4, and NRT2.5 (Orsel et al., 2002; Kiba et al., 2012; Lezhneva et al., 2014; Kiba and Krapp, 2016).
NRT2.1 expression is found in the epidermal and cortical cells of mature roots (Figure 1) (Wirth et al., 2007) and constitutes the most studied transporter in NRT2 family. NRT2.1 expression is dependent to nitrate availability and seems to be regulated by different conditions: (i) induced by nitrate (Filleur and Daniel-Vedele, 1999) and as a consequence the representative of the iHATS (inducible high affinity transport system, see Introduction), (ii) repressed in high nitrate or nitrogen concentrations (Crawford, 1995), (iii) briefly expressed in response to nitrate deficiency (Crawford and Glass, 1998; Cerezo et al., 2001). Same regulation was observed in maize (Sorgona et al., 2011). ZmNRT2.1 expression along the maize primary root increases after 4 h of nitrate treatment coordinated with elevation of nitrate uptake rate. These results reflect an important role of NRT2.1 transporter in the regulation of nitrate fluxes in roots. Water status is affected in nrt2.1 mutant, suggesting that NRT2.1 also supports root hydraulic conductivity (Li et al., 2016).
Despite its low expression level, NRT2.2 expression seems to follow the same regulation (i.e., in response to nitrate availability). Moreover, NRT2.1 and NRT2.2 genes are very close in genomic region of chromosome 1 (AGI NRT2.1: At1g08090 and NRT2.2: At1g08100). atnrt2 KO mutants have been obtained for both transporters NRT2.1 and NRT2.2, and are affected in the high affinity nitrate transport activity, but not in the low affinity. However, in this atnrt2 mutant, the deletion of both transporters did not result in the complete disappearance of the nitrate uptake ability in low nitrate concentration, and besides a low affinity transport activity, a residual high affinity nitrate transport response was observed, probably due to the presence of constitutive high affinity nitrate transporters (Cerezo et al., 2001; Miller et al., 2007). In maize, an analysis of several NRT2 genes expression in response to nitrate suggests that ZmNRT2.1 and ZmNRT2.2 are the main genes controlling high-affinity nitrate uptake (Garnett et al., 2013).
Another NRT2 family transporter, NRT2.4, has been identified to participate to nitrate transport in roots, additionally to its contribution to nitrate distribution to shoot. Although a low expression level compared to NRT2.1, NRT2.4 is expressed in the epidermis of the lateral root (Figure 1; also expressed in leaves) and is involved in nitrate uptake at very low nitrate concentration. Interestingly, nitrate perception could control expression of NRT2.4, induced in long-term starvation (Kiba et al., 2012). At low external nitrate concentration (25 μM), nrt2.1/nrt2.2 double mutant is deficient for high affinity nitrate uptake and nitrate transport is severely affected but still occurs. NRT2.4 contribute to maintain a minimal transport activity in this mutant. Kiba et al. (2012) have demonstrated that overexpression of NRT2.4 in the nrt2.1/nrt2.2 double mutant partially restore nitrate uptake activity. However NRT2.4 is not the only one to provide this transport activity because nrt2.1/nrt2.2/nrt2.4 triple mutant keep its ability to transport nitrate (7% relative to WT) at very low concentration (20 μM nitrate), suggesting existence of others high affinity transporters. This role is partly shared by NRT2.5 as quadruple mutant nrt2.1/nrt2.2/nrt2.4/nrt2.5 has a stronger reduced high affinity nitrate transport (3% relative to WT; Lezhneva et al., 2014). Interestingly, added NRT2.4 loss of function to nrt2.1/nrt2.2 double mutant impact much more seedling growth under N deficiency than NRT2.5. Moreover, NRT2.4 and NRT2.5 transcripts increase during N limitation, suggesting significant implication of both in root nitrate uptake (Lezhneva et al., 2014).
Interaction with a small protein NAR2/NRT3 seems to be essential for nitrate uptake of some of the NRT2 (Orsel et al., 2006; Li et al., 2007). Two members constitute NAR2 family in Arabidopsis, but only NAR2.1/NRT3.1 is necessary for nitrate transport activity. Indeed, co-expression in oocytes of NAR2.1/NRT3.1 and all (expect NRT2.7) NRT2 members demonstrate the positive effect of NAR2.1 on NRT2 dependent nitrate uptake (Kotur et al., 2012). Moreover, these NRT2 family members interact with NAR2.1 in yeast two hybrids (Kotur et al., 2012). The effect of NAR2.1/NRT3.1 on NRT2.4 nitrate transport activity in xenopus oocyte is yet fully understood (Kiba et al., 2012). Besides contribution to nitrate transport, NAR2.1 seems to be implicated in NRT2.1 localization or stabilization at the plasma membrane (Wirth et al., 2007). NAR contribution to nitrate transport is also observed in others species, like barley, maize or rice. Measurements of the 15N-nitrate enrichment in oocytes showed that co-injection of HvNRT2.1 and HvNAR2.3 was able to provide significant nitrate uptake (Tong et al., 2005). Lupini et al. (2016) show that ZmNAR2.1 has an important implication in ZmNRT2.1 expression and localization along root axis correlated with nitrate influxes (Lupini et al., 2016). In rice, the expression of the OsNRT2.1, OsNRT2.2, and OsNRT2.3a genes seems to be regulated by OsNAR2.1, itself transcriptionally induced by nitrate (Yan et al., 2011).
Low-Affinity Nitrate Transporter: NPF Family
In higher plants, NPF family includes a large number of genes, divided into eight subfamilies, and able to transport diversified substrates. However, no substrate selectivity correlates with sequence homology, and consequently with each subfamily (Léran et al., 2014). In Arabidopsis, NPF family consists of 53 members mainly characterized as low affinity transporters. Herein, NPF6.3/NRT1.1/CHL1 was firstly identified as low affinity nitrate transporter (Tsay et al., 1993). NPF6.3 is expressed in several root tissues: epidermis, cortex and endodermis (Figure 1; also expressed in young leaf and flower buds) (Huang et al., 1996; Guo et al., 2002; Remans et al., 2006a). Consequently, NPF6.3 allows nitrate uptake from soil (Crawford, 1995; Munos et al., 2004) and is also implicated in nitrate translocation to aerials part (Boursiac et al., 2013; Léran et al., 2013). NPF6.3 reveals also an ability to switch to high-affinity activity in low nitrate conditions (Liu et al., 1999; Liu and Tsay, 2003; Ho et al., 2009). When phosphorylated, Thr 101 confers to NPF6.3 high affinity nitrate transport behavior whereas non-phosphorylated NPF6.3 acts as low-affinity transporter. The protein complex CIPK23-CBL9 (CIPK: CBL-Interacting Protein Kinase, CBL: Calcineurin-B like Protein) is implicated in the dual affinity transition changes thanks to its ability to phosphorylate Thr 101 residue. In low nitrate conditions, CIPK23-CBL9 phosphorylates NPF6.3 Thr 101 residue and promotes NPF6.3 high affinity nitrate transport (Liu and Tsay, 2003; Ho et al., 2009). Other proteins, namely ABI1 and ABI2 belonging to protein phosphatase 2C family from the clade A, contribute to NPF6.3 activity in xenopus oocytes (Léran et al., 2015). In planta experiments demonstrate that only ABI2 has a regulatory role on NPF6.3-dependent nitrate transport. This protein was able to modulate transport activity by preventing phosphorylation of CIPK23-CBL1 complex (Léran et al., 2015).
Functional characterization and regulation of NPF6.3 transporters is still ongoing and many residues seem to be implicated in nitrate transport ability. Recently, crystallization studies of NPF6.3 reveal a crucial role of His 356 residue in nitrate substrate binding and transport. In the presence of nitrate, authors show that NPF6.3 H356A variant loses its nitrate transport capacity compared to wild type NPF6.3 protein (Parker and Newstead, 2014; Sun et al., 2014).
NPF4.6/NRT1.2/AIT1, another nitrate transporter belonging to NPF family is involved in soil nitrate uptake. As for NPF6.3, NPF4.6 is also expressed in root epidermis (Figure 1), however, NPF4.6 display a constitutive expression and activity is restricted to low affinity nitrate transport (Liu et al., 1999). NPF4.6 is also an ABA transporter implicated in seed dormancy and transpiration (Kanno et al., 2012).
NPF2.7/NAXT1 is another member of the NPF family expressed the cortex of mature roots (Figure 1) and implicated in root nitrate uptake in Arabidopsis. However, it seems to be mainly involved in nitrate efflux to the external media and consequently participate in nitrate homeostasis (Segonzac et al., 2007). As for others NPFs (i.e., NPF7.2, NPF7.3, and NPF2.3) expressed in Arabidopsis roots and characterized to date, their role consist mainly to nitrate translocation to xylem tissue for distribution to aerial parts (Lin et al., 2008, Li et al., 2010; Taochy et al., 2015).
Nitrate Sensing by Transporters
Although it constitutes an essential nutrient, nitrate is also known to be a signaling molecule involved in many physiological processes including gene regulation (Wang et al., 2004) and root development (Walch-Liu et al., 2006a). Within the different proteins involved in nitrate sensing (Medici and Krouk, 2014), two transporters described above, namely NRT2.1 and NPF6.3/NRT1.1, are key ones.
NRT2.1
Formation of lateral root is strongly affected by environmental condition and nutrient signals as nitrate are essential to influenced root architecture. Beside its role in high-affinity nitrate transport, NRT2.1 is involved in nitrate-dependent lateral root initiation (LRI). With genetic screen using the repression of LRI in seedlings growing on high sucrose/low nitrogen conditions, a lin1 mutant has been isolated for its ability to initiate a large number of lateral roots (Malamy and Ryan, 2001). Further characterization of lin1 mutant indicates a point mutation in NRT2.1 gene that leads to G119R substitution in the protein sequence is responsible for lin1 phenotype (Little et al., 2005). Loss of function lin1 mutant as well as three others nrt2.1 mutants (nrt2.1-1, nrt2.1-2, and nrt2.1-3) exhibit nitrate-independent increases lateral root formation suggesting that NRT2.1 is implicated in LRI repression under specific conditions (i.e., high sucrose/low nitrate). Nitrate influx in lin1 (as nrt2.1 KO) mutant is significantly reduced compared to WT, indicating that nitrate uptake and/or accumulation could be correlated to inhibition of lateral root primordia (Little et al., 2005; Remans et al., 2006b). However, LRI is not only regulated by direct sensing of the external nitrate concentration and could be influenced by long-term nitrate growth condition. Thus, decrease in nitrate availability [seedling transferred from high (10 mM) to low (0.5 mM) nitrate media] seems to stimulate both lateral root length and LRI in WT plants (Remans et al., 2006b).
These results indicate that NRT2.1 deals with nitrate transporter activity independently from its nitrate sensors activity in the root development control. Nitrate effect on root development not only originates from current nitrate transport but also from nitrate already available from the plant.
NPF6.3/NRT1.1/CHL1
In addition to its nitrate transport activity (Tsay et al., 1993), NPF6.3/NRT1.1 functions as a nitrate sensor and is able to promote physiological response in the control of root system architecture and to modulate the expression level of many gens implicated in nitrate signaling pathway (Wang et al., 2004; Krouk et al., 2009, 2010b; Medici and Krouk, 2014; Medici et al., 2015). The NPF6.3 dependent regulation of NRT2.1 expression has been studied and is modulated according to nitrate concentration. Fast induction of NRT2.1 expression is observed in response to brief exposure to nitrate concentration (Ho et al., 2009; Bouguyon et al., 2015) whereas NRT2.1 is down regulated in long-term high nitrate supply (Munos et al., 2004; Krouk et al., 2006). Another nitrate dependent phenotype, lateral root development, is also NPF6.3/NRT1.1-dependent (Bouguyon et al., 2016). Thus in low nitrate condition, NPF6.3 acts as a repressor of lateral root primordia and it became an activator of root branching in response to nitrate supply (Remans et al., 2006a; Krouk et al., 2010a; Mounier et al., 2014).
The definitive proof of the role of NPF6.3/NRT1.1 in nitrate sensing has been given by the study of chl1-9, NPF6.3/NRT1.1-P492L (Ho et al., 2009). Although a normal level of transcript and protein expression, nitrate transport activity was suppressed in chl1-9 knockout mutant, demonstrating that the Pro 492 residue is essential for nitrate uptake. Interestingly, this mutant is still able to induce NPF6.3/NRT1.1-dependent gene expression measured through NRT2.1 expression level, indicating that PNR is not affected in this mutant. This specific mutation demonstrates that the roles of NPF6.3 in transport and signaling belong to independent regulations (Ho et al., 2009; Bouguyon et al., 2015).
NPF6.3 ability to modulate lateral root development is mediated by its auxin transport capacity (Krouk et al., 2010a; Bouguyon et al., 2015). Correlations have been suggested between NPF6.3 auxin transport ability and nitrate sensing and signaling. Indeed, P492L and T101A point mutations decrease auxin transport capacity and plants expressing these mutants (i.e., chl1-9 and NPF6.3-T101A) are affected in nitrate-dependent lateral root development, suggesting that phosphorylated form of NPF6.3 could be responsible for NPF6.3 dependent regulation of both nitrate and auxin transport and consequently for lateral root development (Bouguyon et al., 2015).
Finally, the NPF6.3/NRT1.1 regulators, the kinase CIPK23 and the phosphatase ABI2, are also involved in nitrate sensing (Ho et al., 2009; Léran et al., 2015): CIPK23 being a negative regulator of NPF6.3/NRT1.1 by phosphorylation, and then a repressor of PNR whereas ABI2 being a positive regulator of the negative regulator complex (CIPK23/CBL1). Recently, the NRG2 (Nitrate Regulatory Gene) gene has been described to impact nitrate signaling via regulation of NPF6.3 in roots (and NPF7.2 in leaves). nrg2 mutant grown in nitrate media supplemented with ammonium shows a lower expression of NPF6.3 expression in roots as well as down regulation of many nitrate responsive genes. Consequently, theses mutants display a defect in nitrate accumulation on roots (Xu et al., 2016).
Conclusion
Two transporters, NRT2.1 and NPF6.3/NRT1.1, as well as their protein partners (NAR2, CBL1, CBL9, CIPK23, ABI2) are involved in nitrate transport and sensing. Although theses nitrate transporters are among the most studied, there are still some missing parts to better interpret the transport regulation and the modulation of root architecture, and as well the adjacent mechanisms responsible for optimization of nitrate use efficiency (NUE; O’Brien et al., 2016). Plant nitrate uptake constitutes an important trait to take into account to improve crop yield and NUE. Indeed in rice, plants overexpressing the high affinity nitrate transporter NRT2.3b have an increased nitrate uptake correlated to an improvement in growth capacity, yield and NUE (Fan et al., 2016), just like OsNRT1.1b also implicated in nitrate transport efficiency and NUE (Fan et al., 2015; Hu et al., 2015). Thus, the capacity of other NPF and NRT2 transporters to sense nitrate will be determined in further experiments and will help us to better understand how plant is able to cope with nitrate heterogeneity. Furthermore, identification of proteins belonging to the NRT2 and NPF6.3/NRT1.1 protein regulatory networks will give new insights in the nitrate sensing capacity of plants. This review deals with plasma membrane transporters, however, vacuolar transporters are involved in nitrate accumulation and consequently impacts on NUE, as observed in rapeseed for BnNRT1.5 (Han et al., 2016). Thus, determination of molecular players and regulatory factors interacting with nitrate transporters, as well as others transporters like SLAH1 through which plants are able to balance between nitrate and chloride loading (Cubero-Font et al., 2016) constitutes a future challenge to better understand how nitrate influences plant growth and development.
Author Contributions
All authors listed, have made substantial, direct and intellectual contribution to the work, and approved it for publication.
Conflict of Interest Statement
The authors declare that the research was conducted in the absence of any commercial or financial relationships that could be construed as a potential conflict of interest.
Acknowledgments
BL is financially supported by the Agence Nationale de la Recherche (ANR-14-CE34-0007-01-HONIT with a post-doctoral fellowship to MN).
References
Alboresi, A., Gestin, C., Leydecker, M. T., Bedu, M., Meyer, C., and Truong, H. N. (2005). Nitrate, a signal relieving seed dormancy in Arabidopsis. Plant Cell Environ. 28, 500–512. doi: 10.1111/j.1365-3040.2005.01292.x
Barbier-Brygoo, H., De Angeli, A., Filleur, S., Frachisse, J. M., Gambale, F., Thomine, S., et al. (2011). Anion channels/transporters in plants: from molecular bases to regulatory networks. Annu. Rev. Plant Biol. 62, 25–51. doi: 10.1146/annurev-arplant-042110-103741
Bi, Y. M., Wang, R. L., Zhu, T., and Rothstein, S. J. (2007). Global transcription profiling reveals differential responses to chronic nitrogen stress and putative nitrogen regulatory components in Arabidopsis. BMC Genomics 8:281. doi: 10.1186/1471-2164-8-281
Birnbaum, K., Shasha, D. E., Wang, J. Y., Jung, J. W., Lambert, G. M., Galbraith, D. W., et al. (2003). A gene expression map of the Arabidopsis root. Science 302, 1956–1960. doi: 10.1126/science.1090022
Bouguyon, E., Brun, F., Meynard, D., Kubes, M., Pervent, M., Léran, S., et al. (2015). Multiple mechanisms of nitrate sensing by Arabidopsis nitrate transceptor NRT1.1. Nature Plants 1:15015. doi: 10.1038/NPLANTS.2015.15
Bouguyon, E., Perrine-Walker, F., Pervent, M., Rochette, J., Cuesta, C., Benkova, E., et al. (2016). Nitrate controls root development through post-transcriptional regulation of the NRT1.1/NPF6.3 transporter/sensor. Plant Physiol. doi: 10.1104/pp.16.01047 [Epub ahead of print].
Boursiac, Y., Léran, S., Corratge-Faillie, C., Gojon, A., Krouk, G., and Lacombe, B. (2013). ABA transport and transporters. Trends Plant Sci. 18, 325–333. doi: 10.1016/j.tplants.2013.01.007
Castro Marin, I., Loef, I., Bartetzko, L., Searle, I., Coupland, G., Stitt, M., et al. (2011). Nitrate regulates floral induction in Arabidopsis, acting independently of light, gibberellin and autonomous pathways. Planta 233, 539–552. doi: 10.1007/s00425-010-1316-5
Cerezo, M., Tillard, P., Filleur, S., Munos, S., Daniel-Vedele, F., and Gojon, A. (2001). Major alterations of the regulation of root NO(3)(-) uptake are associated with the mutation of Nrt2.1 and Nrt2.2 genes in Arabidopsis. Plant Physiol. 127, 262–271. doi: 10.1104/pp.127.1.262
Chiu, C. C., Lin, C. S., Hsia, A. P., Su, R. C., Lin, H. L., and Tsay, Y. F. (2004). Mutation of a nitrate transporter, AtNRT1:4, results in a reduced petiole nitrate content and altered leaf development. Plant Cell Physiol. 45, 1139–1148. doi: 10.1093/pcp/pch143
Crawford, N. M. (1995). Nitrate: nutrient and signal for plant growth. Plant Cell 7, 859–868. doi: 10.1105/tpc.7.7.859
Crawford, N. M., and Glass, A. D. M. (1998). Molecular and physiological aspects of nitrate uptake in plants. Trends Plant Sci. 3, 389–395. doi: 10.1016/S1360-1385(98)01311-9
Cubero-Font, P., Maierhofer, T., Jaslan, J., Rosales, M. A., Espartero, J., Diaz-Rueda, P., et al. (2016). Silent S-Type anion channel subunit SLAH1 gates SLAH3 open for chloride root-to-shoot translocation. Curr. Biol. 26, 2213–2220. doi: 10.1016/j.cub.2016.06.045
Drew, M., Saker, L., and Ashley, T. (1973). Nutrient supply and the growth of the semicla root system in barley. I. The effect of nitrate concentration on the growth of axes and lateral. J. Exp. Bot. 24, 1189–1202. doi: 10.1093/jxb/24.6.1189
Drew, M. C., and Saker, L. R. (1975). Nutrient supply and the growth of the serninal root systern in barley. II. Localized cornpensatory increases in lateral root growth and rates of nitrate uptake when NO, is restricted to only part of the root system. J. Exp. Bot. 26, 79–80. doi: 10.1093/jxb/26.1.79
Fan, X., Feng, H., Tan, Y., Xu, Y., Miao, Q., and Xu, G. (2015). A putative 6-transmembrane nitrate transporter OsNRT1.1b plays a key role in rice under low nitrogen. J. Integr. Plant Biol. 58, 590–599. doi: 10.1111/jipb.12382
Fan, X., Tang, Z., Tan, Y., Zhang, Y., Luo, B., Yang, M., et al. (2016). Overexpression of a pH-sensitive nitrate transporter in rice increases crop yields. Proc. Natl. Acad. Sci. U.S.A. 113, 7118–7123. doi: 10.1073/pnas.1525184113
Filleur, S., and Daniel-Vedele, F. (1999). Expression analysis of a high-affinity nitrate transporter isolated from Arabidopsis thaliana by differential display. Planta 207, 461–469. doi: 10.1007/s004250050505
Garnett, T., Conn, V., Plett, D., Conn, S., Zanghellini, J., Mackenzie, N., et al. (2013). The response of the maize nitrate transport system to nitrogen demand and supply across the lifecycle. New Phytol. 198, 82–94. doi: 10.1111/nph.12166
Guo, F. Q., Wang, R., and Crawford, N. M. (2002). The Arabidopsis dual-affinity nitrate transporter gene AtNRT1.1 (CHL1) is regulated by auxin in both shoots and roots. J. Exp. Bot. 53, 835–844. doi: 10.1093/jexbot/53.370.835
Han, Y. L., Song, H. X., Liao, Q., Yu, Y., Jian, S. F., Lepo, J. E., et al. (2016). Nitrogen use efficiency is mediated by vacuolar nitrate sequestration capacity in roots of Brassica napus. Plant Physiol. 170, 1684–1698. doi: 10.1104/pp.15.01377
Ho, C. H., Lin, S. H., Hu, H. C., and Tsay, Y. F. (2009). CHL1 functions as a nitrate sensor in plants. Cell 138, 1184–1194. doi: 10.1016/j.cell.2009.07.004
Hu, B., Wang, W., Ou, S., Tang, J., Li, H., Che, R., et al. (2015). Variation in NRT1.1B contributes to nitrate-use divergence between rice subspecies. Nat. Genet. 47, 834–838. doi: 10.1038/ng.3337
Huang, N. C., Chiang, C. S., Crawford, N. M., and Tsay, Y. F. (1996). CHL1 encodes a component of the low-affinity nitrate uptake system in Arabidopsis and shows cell type-specific expression in roots. Plant Cell 8, 2183–2191. doi: 10.1105/tpc.8.12.2183
Kanno, Y., Hanada, A., Chiba, Y., Ichikawa, T., Nakazawa, M., Matsui, M., et al. (2012). Identification of an abscisic acid transporter by functional screening using the receptor complex as a sensor. Proc. Natl. Acad. Sci. U.S.A. 109, 9653–9658. doi: 10.1073/pnas.1203567109
Kiba, T., Feria-Bourrellier, A. B., Lafouge, F., Lezhneva, L., Boutet-Mercey, S., Orsel, M., et al. (2012). The Arabidopsis nitrate transporter NRT2.4 plays a double role in roots and shoots of nitrogen-starved plants. Plant Cell 24, 245–258. doi: 10.1105/tpc.111.092221
Kiba, T., and Krapp, A. (2016). Plant nitrogen acquisition under low availability: regulation of uptake and root architecture. Plant Cell Physiol. 57, 707–714. doi: 10.1093/pcp/pcw052
Kotur, Z., Mackenzie, N., Ramesh, S., Tyerman, S. D., Kaiser, B. N., and Glass, A. D. (2012). Nitrate transport capacity of the Arabidopsis thaliana NRT2 family members and their interactions with AtNAR2.1. New Phytol. 194, 724–731. doi: 10.1111/j.1469-8137.2012.04094.x
Krapp, A., David, L. C., Chardin, C., Girin, T., Marmagne, A., Leprince, A. S., et al. (2014). Nitrate transport and signalling in Arabidopsis. J. Exp. Bot. 65, 789–798. doi: 10.1093/jxb/eru001
Krouk, G., Lacombe, B., Bielach, A., Perrine-Walker, F., Malinska, K., Mounier, E., et al. (2010a). Nitrate-regulated auxin transport by NRT1.1 defines a mechanism for nutrient sensing in plants. Dev. Cell 18, 927–937. doi: 10.1016/j.devcel.2010.05.008
Krouk, G., Mirowski, P., LeCun, Y., Shasha, D. E., and Coruzzi, G. M. (2010b). Predictive network modeling of the high-resolution dynamic plant transcriptome in response to nitrate. Genome Biol. 11:R123. doi: 10.1186/gb-2010-11-12-r123
Krouk, G., Ruffel, S., Gutierrez, R. A., Gojon, A., Crawford, N. M., Coruzzi, G. M., et al. (2011). A framework integrating plant growth with hormones and nutrients. Trends Plant Sci. 16, 178–182. doi: 10.1016/j.tplants.2011.02.004
Krouk, G., Tillard, P., and Gojon, A. (2006). Regulation of the high-affinity NO3- uptake system by NRT1.1-mediated NO3- demand signaling in Arabidopsis. Plant Physiol. 142, 1075–1086. doi: 10.1104/pp.106.087510
Krouk, G., Tranchina, D., Lejay, L., Cruikshank, A. A., Shasha, D., Coruzzi, G. M., et al. (2009). A systems approach uncovers restrictions for signal interactions regulating genome-wide responses to nutritional cues in Arabidopsis. PLoS Comput. Biol. 5:e1000326. doi: 10.1371/journal.pcbi.1000326
Léran, S., Edel, K. H., Pervent, M., Hashimoto, K., Corratge-Faillie, C., Offenborn, J. N., et al. (2015). Nitrate sensing and uptake in Arabidopsis are enhanced by ABI2, a phosphatase inactivated by the stress hormone abscisic acid. Sci. Signal. 8:ra43. doi: 10.1126/scisignal.aaa4829
Léran, S., Muños, S., Brachet, C., Tillard, P., Gojon, A., and Lacombe, B. (2013). Arabidopsis NRT1.1 is a bidirectional transporter involved in root-to-shoot nitrate translocation. Mol. Plant 6, 1984–1987. doi: 10.1093/mp/sst068
Léran, S., Varala, K., Boyer, J. C., Chiurazzi, M., Crawford, N., Daniel-Vedele, F., et al. (2014). A unified nomenclature of NITRATE TRANSPORTER 1/PEPTIDE TRANSPORTER family members in plants. Trends Plant Sci. 19, 5–9. doi: 10.1016/j.tplants.2013.08.008
Lezhneva, L., Kiba, T., Feria-Bourrellier, A. B., Lafouge, F., Boutet-Mercey, S., Zoufan, P., et al. (2014). The Arabidopsis nitrate transporter NRT2.5 plays a role in nitrate acquisition and remobilization in nitrogen-starved plants. Plant J. 80, 230–241. doi: 10.1111/tpj.12626
Li, G., Tillard, P., Gojon, A., and Maurel, C. (2016). Dual regulation of root hydraulic conductivity and plasma membrane aquaporins by plant nitrate accumulation and high-affinity nitrate transporter NRT2.1. Plant Cell Physiol. 57, 733–742. doi: 10.1093/pcp/pcw022
Li, J. Y., Fu, Y. L., Pike, S. M., Bao, J., Tian, W., Zhang, Y., et al. (2010). The Arabidopsis nitrate transporter NRT1.8 functions in nitrate removal from the xylem sap and mediates cadmium tolerance. Plant Cell 22, 1633–1646. doi: 10.1105/tpc.110.075242
Li, W., Wang, Y., Okamoto, M., Crawford, N. M., Siddiqi, M. Y., and Glass, A. D. (2007). Dissection of the AtNRT2.1:AtNRT2.2 inducible high-affinity nitrate transporter gene cluster. Plant Physiol. 143, 425–433. doi: 10.1104/pp.106.091223
Lin, S. H., Kuo, H. F., Canivenc, G., Lin, C. S., Lepetit, M., Hsu, P. K., et al. (2008). Mutation of the Arabidopsis NRT1.5 nitrate transporter causes defective root-to-shoot nitrate transport. Plant Cell 20, 2514–2528. doi: 10.1105/tpc.108.060244
Little, D. Y., Rao, H., Oliva, S., Daniel-Vedele, F., Krapp, A., and Malamy, J. E. (2005). The putative high-affinity nitrate transporter NRT2.1 represses lateral root initiation in response to nutritional cues. Proc. Natl. Acad. Sci. U.S.A. 102, 13693–13698. doi: 10.1073/pnas.0504219102
Liu, K. H., Huang, C. Y., and Tsay, Y. F. (1999). CHL1 is a dual-affinity nitrate transporter of Arabidopsis involved in multiple phases of nitrate uptake. Plant Cell 11, 865–874. doi: 10.2307/3870820
Liu, K. H., and Tsay, Y. F. (2003). Switching between the two action modes of the dual-affinity nitrate transporter CHL1 by phosphorylation. EMBO J. 22, 1005–1013. doi: 10.1093/emboj/cdg118
Lupini, A., Mercati, F., Araniti, F., Miller, A. J., Sunseri, F., and Abenavoli, M. R. (2016). NAR2.1/NRT2.1 functional interaction with NO3(-) and H(+) fluxes in high-affinity nitrate transport in maize root regions. Plant Physiol. Biochem. 102, 107–114. doi: 10.1016/j.plaphy.2016.02.022
Malamy, J. E., and Ryan, K. S. (2001). Environmental regulation of lateral root initiation in Arabidopsis. Plant Physiol. 127, 899–909. doi: 10.1104/pp.010406
Medici, A., and Krouk, G. (2014). The primary nitrate response: a multifaceted signalling pathway. J. Exp. Bot. 65, 5567–5576. doi: 10.1093/jxb/eru245
Medici, A., Marshall-Colon, A., Ronzier, E., Szponarski, W., Wang, R., Gojon, A., et al. (2015). AtNIGT1/HRS1 integrates nitrate and phosphate signals at the Arabidopsis root tip. Nat. Commun. 6:6274. doi: 10.1038/ncomms7274
Miller, A. J., Fan, X., Orsel, M., Smith, S. J., and Wells, D. M. (2007). Nitrate transport and signalling. J. Exp. Bot. 58, 2297–2306. doi: 10.1093/jxb/erm066
Miller, A. J., and Smith, S. J. (2008). Cytosolic nitrate ion homeostasis: could it have a role in sensing nitrogen status? Ann. Bot. 101, 485–489. doi: 10.1093/aob/mcm313
Mounier, E., Pervent, M., Ljung, K., Gojon, A., and Nacry, P. (2014). Auxin-mediated nitrate signalling by NRT1.1 participates in the adaptive response of Arabidopsis root architecture to the spatial heterogeneity of nitrate availability. Plant Cell Environ. 37, 162–174. doi: 10.1111/pce.12143
Munos, S., Cazettes, C., Fizames, C., Gaymard, F., Tillard, P., Lepetit, M., et al. (2004). Transcript profiling in the chl1-5 mutant of Arabidopsis reveals a role of the nitrate transporter NRT1.1 in the regulation of another nitrate transporter, NRT2.1. Plant Cell 16, 2433–2447. doi: 10.1105/tpc.104.024380
Negi, J., Matsuda, O., Nagasawa, T., Oba, Y., Takahashi, H., Kawai-Yamada, M., et al. (2008). CO2 regulator SLAC1 and its homologues are essential for anion homeostasis in plant cells. Nature 452, 483–486. doi: 10.1038/nature06720
O’Brien, J. A., Vega, A., Bouguyon, E., Krouk, G., Gojon, A., Coruzzi, G., et al. (2016). Nitrate transport, sensing, and responses in plants. Mol. Plant 9, 837–856. doi: 10.1016/j.molp.2016.05.004
Orsel, M., Chopin, F., Leleu, O., Smith, S. J., Krapp, A., Daniel-Vedele, F., et al. (2006). Characterization of a two-component high-affinity nitrate uptake system in Arabidopsis. Physiology and protein-protein interaction. Plant Physiol. 142, 1304–1317.
Orsel, M., Krapp, A., and Daniel-Vedele, F. (2002). Analysis of the NRT2 nitrate transporter family in Arabidopsis. Structure and gene expression. Plant Physiol. 129, 886–896. doi: 10.1104/pp.005280
Parker, J. L., and Newstead, S. (2014). Molecular basis of nitrate uptake by the plant nitrate transporter NRT1.1. Nature 507, 68–72. doi: 10.1038/nature13116
Remans, T., Nacry, P., Pervent, M., Filleur, S., Diatloff, E., Mounier, E., et al. (2006a). The Arabidopsis NRT1.1 transporter participates in the signaling pathway triggering root colonization of nitrate-rich patches. Proc. Natl. Acad. Sci. U.S.A. 103, 19206–19211. doi: 10.1073/pnas.0605275103
Remans, T., Nacry, P., Pervent, M., Girin, T., Tillard, P., Lepetit, M., et al. (2006b). A central role for the nitrate transporter NRT2.1 in the integrated morphological and physiological responses of the root system to nitrogen limitation in Arabidopsis. Plant Physiol. 140, 909–921. doi: 10.1104/pp.105.075721
Ruffel, S., Gojon, A., and Lejay, L. (2014). Signal interactions in the regulation of root nitrate uptake. J. Exp. Bot. 65, 5509–5517. doi: 10.1093/jxb/eru321
Ruffel, S., Krouk, G., Ristova, D., Shasha, D., Birnbaum, K. D., and Coruzzi, G. M. (2011). Nitrogen economics of root foraging: transitive closure of the nitrate-cytokinin relay and distinct systemic signaling for N supply vs. demand. Proc. Natl. Acad. Sci. U.S.A. 108, 18524–18529. doi: 10.1073/pnas.1108684108
Ruffel, S., Poitout, A., Krouk, G., Coruzzi, G. M., and Lacombe, B. (2016). Long-distance nitrate signaling displays cytokinin dependent and independent branches. J. Integr. Plant Biol. 58, 226–229. doi: 10.1111/jipb.12453
Segonzac, C., Boyer, J. C., Ipotesi, E., Szponarski, W., Tillard, P., Touraine, B., et al. (2007). Nitrate efflux at the root plasma membrane: identification of an Arabidopsis excretion transporter. Plant Cell 19, 3760–3777. doi: 10.1105/tpc.106.048173
Sorgona, A., Lupini, A., Mercati, F., Di Dio, L., Sunseri, F., and Abenavoli, M. R. (2011). Nitrate uptake along the maize primary root: an integrated physiological and molecular approach. Plant Cell Environ. 34, 1127–1140. doi: 10.1111/j.1365-3040.2011.02311.x
Stitt, M. (1999). Nitrate regulation of metabolism and growth. Curr. Opin. Plant Biol. 2, 178–186. doi: 10.1016/S1369-5266(99)80033-8
Sun, J., Bankston, J. R., Payandeh, J., Hinds, T. R., Zagotta, W. N., and Zheng, N. (2014). Crystal structure of the plant dual-affinity nitrate transporter NRT1.1. Nature 507, 73–77. doi: 10.1038/nature13074
Taochy, C., Gaillard, I., Ipotesi, E., Oomen, R., Leonhardt, N., Zimmermann, S., et al. (2015). The Arabidopsis root stele transporter NPF2.3 contributes to nitrate translocation to shoots under salt stress. Plant J. 83, 466–479. doi: 10.1111/tpj.12901
Tong, Y., Zhou, J. J., Li, Z., and Miller, A. J. (2005). A two-component high-affinity nitrate uptake system in barley. Plant J. 41, 442–450. doi: 10.1111/j.1365-313X.2004.02310.x
Tsay, Y. F., Schroeder, J. I., Feldmann, K. A., and Crawford, N. M. (1993). The herbicide sensitivity gene CHL1 of Arabidopsis encodes a nitrate-inducible nitrate transporter. Cell 72, 705–713. doi: 10.1016/0092-8674(93)90399-B
Vidal, E. A., Moyano, T. C., Canales, J., and Gutierrez, R. A. (2014). Nitrogen control of developmental phase transitions in Arabidopsis thaliana. J. Exp. Bot. 65, 5611–5618. doi: 10.1093/jxb/eru326
Walch-Liu, P., Ivanov, I. I., Filleur, S., Gan, Y., Remans, T., and Forde, B. G. (2006a). Nitrogen regulation of root branching. Ann. Bot. 97, 875–881. doi: 10.1093/aob/mcj601
Walch-Liu, P., Liu, L. H., Remans, T., Tester, M., and Forde, B. G. (2006b). Evidence that L-glutamate can act as an exogenous signal to modulate root growth and branching in Arabidopsis thaliana. Plant Cell Physiol. 47, 1045–1057. doi: 10.1093/pcp/pcj075
Wang, R., Tischner, R., Gutierrez, R. A., Hoffman, M., Xing, X., Chen, M., et al. (2004). Genomic analysis of the nitrate response using a nitrate reductase-null mutant of Arabidopsis. Plant Physiol. 136, 2512–2522. doi: 10.1104/pp.104.044610
Wirth, J., Chopin, F., Santoni, V., Viennois, G., Tillard, P., Krapp, A., et al. (2007). Regulation of root nitrate uptake at the NRT2.1 protein level in Arabidopsis thaliana. J. Biol. Chem. 282, 23541–23552. doi: 10.1074/jbc.M700901200
Xu, N., Wang, R., Zhao, L., Zhang, C., Li, Z., Lei, Z., et al. (2016). The Arabidopsis NRG2 protein mediates nitrate signaling and interacts with and regulates key nitrate regulators. Plant Cell 28, 485–504. doi: 10.1105/tpc.15.00567
Keywords: nutrient sensing, transporters, nitrates, development, plants
Citation: Noguero M and Lacombe B (2016) Transporters Involved in Root Nitrate Uptake and Sensing by Arabidopsis. Front. Plant Sci. 7:1391. doi: 10.3389/fpls.2016.01391
Received: 04 June 2016; Accepted: 01 September 2016;
Published: 21 September 2016.
Edited by:
Janin Riedelsberger, University of Talca, ChileReviewed by:
Serge Delrot, University of Bordeaux, FranceZhenhua Zhang, Hunan Agricultural University, China
Copyright © 2016 Noguero and Lacombe. This is an open-access article distributed under the terms of the Creative Commons Attribution License (CC BY). The use, distribution or reproduction in other forums is permitted, provided the original author(s) or licensor are credited and that the original publication in this journal is cited, in accordance with accepted academic practice. No use, distribution or reproduction is permitted which does not comply with these terms.
*Correspondence: Benoît Lacombe, YmVub2l0LmxhY29tYmVAc3VwYWdyby5pbnJhLmZy