- Department of Environmental Science and Engineering, Fudan University, Shanghai, China
The quest for new, promising and indigenous plant growth-promoting rhizobacteria and a deeper understanding of their relationship with plants are important considerations in the improvement of phytoremediation. This study focuses on the screening of plant beneficial Cu/Zn-resistant strains and assessment of their bioremediation potential (metal solubilization/tolerance/biosorption and effects on growth of Brassica napus seedlings) to identify suitable rhizobacteria and examine their roles in microbes-assisted phytoremediation. Sixty Cu/Zn-resistant rhizobacteria were initially isolated from Sonchus oleraceus grown at a multi-metal-polluted site in Shanghai, China. From these strains, 19 isolates that were all resistant to 300 mg⋅L-1 Cu as well as 300 mg⋅L-1 Zn, and could simultaneously grow on Dworkin–Foster salt minimal medium containing 1-aminocyclopropane-1-carboxylic acid were preliminarily selected. Of those 19 isolates, 10 isolates with superior plant growth-promoting properties (indole-3-acetic acid production, siderophore production, and insoluble phosphate solubilization) were secondly chosen and further evaluated to identify those with the highest bioremediation potential and capacity for bioaugmentation. Strain S44, identified as Acinetobacter sp. FQ-44 based on 16S rDNA sequencing, was specifically chosen as the most favorable strain owing to its strong capabilities to (1) promote the growth of rape seedlings (significantly increased root length, shoot length, and fresh weight by 92.60%, 31.00%, and 41.96%, respectively) under gnotobiotic conditions; (2) tolerate up to 1000 mg⋅L-1 Cu and 800 mg⋅L-1 Zn; (3) mobilize the highest concentrations of water-soluble Cu, Zn, Pb, and Fe (16.99, 0.98, 0.08, and 3.03 mg⋅L-1, respectively); and (4) adsorb the greatest quantities of Cu and Zn (7.53 and 6.61 mg⋅g-1 dry cell, respectively). Our findings suggest that Acinetobacter sp. FQ-44 could be exploited for bacteria-assisted phytoextraction. Moreover, the present study provides a comprehensive method for the screening of rhizobacteria for phytoremediation of multi-metal-polluted soils, especially those sewage sludge-amended soils contaminated with Cu/Zn.
Introduction
Heavy metal pollution of soils has become a global environmental concern. Even essential biological trace elements, such as Zn and Cu, can be toxic or lethal to organisms at high concentrations (Ouzounidou, 1995). Unlike organic compounds, heavy metals in soils cannot be mineralized or broken down to less toxic forms (Chen et al., 2014). A large proportion of heavy metals are generally bound to organic and inorganic soil components or exist as insoluble precipitates, and are thus unavailable for root uptake by field-grown plants (Raskin et al., 1994). Therefore, developing appropriate strategies for the remediation of heavy-metal-polluted soils demands urgent attention from the perspectives of environmental conservation and human health (Aboushanab et al., 2006).
Phytoremediation, an emerging, challenging, and solar-driven in situ technology with lower cost and enhanced environmental friendliness in comparison to conventional physicochemical technologies, has received increasing attention from ecological researchers (Kumar et al., 1995). However, this plant-based technique is generally time-consuming, because most hyperaccumulators identified thus far are generally small-biomass and slow-growing (Rajkumar and Freitas, 2008a). Moreover, its efficiency is often limited by the metal bioavailability in soil, plant roots development, and plant tolerance to a particular metal (Pilon-Smits, 2005). Thus, developing alternative strategies that can improve the efficiency of phytoremediation are necessary.
Several researchers have suggested biotechnological approaches and proposed to incorporate plant-associated microorganisms (rhizospheric, endophytic bacteria, and mycorrhizal fungi) into phytoextraction systems (Rajkumar and Freitas, 2008a; Ma et al., 2009a; Sessitsch et al., 2013). In such systems, the plants and rhizosphere are two key factors that make phytoremediation a viable in situ technology. On one hand, the plants to be used for remediation of metal-polluted soils must be qualified with tolerance to at least one metal, high competitiveness, fast growth, and large biomass (Glick, 2010). On the other hand, the rhizosphere, as an important soil-plant interface, provides a complex dynamic microenvironment where root-associated microorganisms form unique communities that have a high potential to detoxify hazardous waste compounds (De Souza et al., 1999; Alford et al., 2010). Moreover, the particular microbial community with high activity and large contact area probably acts as a source of microbial chelates (Kärenlampi et al., 2000). Thus, the microorganism-assisted phytoremediation potential, as well as the mechanisms by which rhizobacteria enhance phytoremediation efficiency, has been attracting increasing research interest lately.
Among the plant-associated microbes, plant growth-promoting rhizobacteria (PGPR) are considered a major component of phytoremediation technology (Glick, 2003). They have capacity of plant growth-promoting (PGP) and improving phytoremediation by various mechanisms, including: fixation of atmospheric nitrogen, utilization of 1-aminocyclopropane-1-carboxylic acid (ACC), production of siderophores and antipathogenic substances, production of plant growth regulators, transformation of nutrient elements (Glick et al., 1999), bacteria-induced metal chelation (Adediran et al., 2015), and synthesis of cysteine-rich peptides (Adediran et al., 2016). Thus, inoculation with metal-resistant PGPR, particularly indigenous PGPR (Kozdrój et al., 2004), can improve the efficiency of heavy metal phytoremediation (Ma et al., 2011; Rajkumar et al., 2012). Therefore, researchers need to isolate and screen competitive and effective PGPR (Paau, 1989) that are well adapted to the conditions of a particular site (Sheng and Xia, 2006). Although PGPR play important roles in phytoremediation strategies, studies on Cu/Zn-resistant PGPR in this area remain very limited (Lucy et al., 2004), particularly field studies. Thus, more laboratory and field studies are needed to advance existing research.
Sonchus oleraceus is a cosmopolitan weed species native to Europe and central Asia (Hutchinson et al., 1984) that grows readily and adapts to diverse environments in many countries (Holm et al., 1977). In China, S. oleraceus is also widely distributed as an annual and roadside pioneer plant. It is one of few species found at disrupted locations, such as oil well sites in oilfields and barren lands (Xiong et al., 1997). Furthermore, S. oleraceus is regarded as the most suitable candidate for the removal of Zn and Cd from soils (Khan et al., 1998).
Despite numerous reports about rhizobacteria-enhanced phytoremediation of heavy metals (Sheng and Xia, 2006; Dell’Amico et al., 2008; Płociniczak et al., 2016), little information is available about effects Cu/Zn-resistant bacteria from the rhizosphere of S. oleraceus on plant growth and heavy metal bioavailability/biosorption in multi-metal-polluted soils. Thus, the quest for novel, beneficial and indigenous rhizobacteria among different plant species grown in multi-metal-polluted environments is very meaningful. In addition, to assess the potential rhizospheric mechanisms underlying the effects on plant growth and uptake and translocation of heavy metals, we explored the biochemical characteristics [production of indole-3-acetic acid (IAA), ACC deaminase (ACCD), and siderophores; and solubilization of inorganic phosphate] of selected bacteria. Furthermore, diverse genera of PGPR could affect plant growth in different ways, because the PGP effect could be plant- and/or PGPR-specific. Thus, our main objectives were to: (1) isolate and preliminarily screen Cu/Zn-resistant and ACCD-containing bacteria from the rhizosphere of S. oleraceus grown in multi-metal-polluted soils; (2) select indigenous PGPR with superior PGP traits that could effectively increase plant biomass under unfavorable conditions; and (3) evaluate the bioremediation potentials of different PGPR (Cu/Zn/Pb/Cd/Fe-solubilization, Cu/Zn-tolerance/biosorption and effects on the growth of rape) to identify more-suitable rhizobacteria and examine the effects of selected bacteria on plant growth and metal uptake/translocation in Brassica napus via sand culture experiments.
Materials and Methods
Sampling, Treatment, and Characterization of Soils and Plants
Soils were randomly sampled from a depth of 0–20 cm in the Jiading Wastewater Disposal Plant (31°22′32″ N, 121°09′57″ E), located at Shanghai, China. The soils used in this study were mixtures of sewage sludge and waste residue, and contaminated with multiple heavy metals. Before the experiments commenced, soil samples pretreatmented were air-dried for 1 month and sieved (4 mm) to remove as many plant materials, soil macrofauna, and stones as possible. The soil subsamples were then passed through a 2-mm stainless steel sieve, and subjected to physicochemical chatracterization according to standard methods (Lu, 1999), some of which are listed in Table 1.
Native in situ S. oleraceus plants were also randomly selected from the same wastewater disposal plant at which the multi-metal-polluted soils were collected. Soon after returning to the laboratory, the rhizospheric soils of S. oleraceus (2 cm radius around the roots) were collected by gently shaking the roots (Wenzel et al., 2003) to remove loosely attached soils and stored in a refrigerator at 4°C until further use.
Isolation and Preliminary Screening of Cu/Zn-Resistant and ACC-Utilizing Rhizobacteria
Rhizobacteria were isolated from S. oleraceus according to the protocol of Jiang et al. (2008). Sixty pure isolates were initially isolated and stored in 30% (v/v) glycerol at –80°C until further analysis (Wei et al., 2009). Viable bacterial populations, including total and resistant bacteria were counted by the plate count method. The CFU/g of fresh soil is presented in Table 1. After isolation, all isolates were further streaked on two Luria–Bertani medium (LB) agar plates containing either Zn or Cu (100 to 500 mg⋅L-1, respectively) and monitored for growth. All plates were incubated in triplicate at 30°C for 48 h.
In order to obtain Cu/Zn-resistant PGPR, 46 isolates that were all simultaneously resistant to 300 mg⋅L-1 Cu and 300 mg⋅L-1 Zn were further tested for their ability to grow on Dworkin–Foster (DF) salt minimal medium containing ACC (denoted ADF) as a sole nitrogen source (Dworkin and Foster, 1958). The DF medium containing (NH4)2SO4 (Rajkumar and Freitas, 2008b) (denoted NDF) and without a nitrogen source were used as controls. We also analyzed the ACCD activity of cell-free extracts analyzed by quantifying the amount of α-ketobutyrate according to a modified method of Honma and Shimomura (1978). After preliminary screening, 19 isolates that were resistant to both 300 mg⋅L-1 Cu and 300 mg⋅L-1 Zn, and simultaneously growing on ADF were selected for further evaluation of PGP parameters (secondary screening).
Evaluation of PGP Properties
Synthesis of IAA by the 19 isolates was quantified as described by Bric et al. (1991), using LB broth supplemented with 0.5 mg⋅mL-1 L-tryptophan. The IAA concentrations were calculated using a calibration curve of pure IAA as the standard (Sigma, USA). Bacterial siderophore production was detected and quantified by the chrome azurol S (CAS) analytical method (Schwyn and Neilands, 1987). According to this assay, the siderophore levels were defined as the A/Ar ratio and a smaller A/Ar ratio indicated higher siderophore output (Sheng et al., 2008). The phosphate-solubilizing ability of the isolates was analyzed in Pikovskaya’s medium (Pikovskaya, 1948) supplemented with 0.5% tricalcium phosphate. The soluble phosphate in the supernatant was quantified by the Mo-blue method (Watanabe and Olsen, 1965). After secondary screening, 10 functional strains with superior PGP traits were selected for further evaluation of bioremediation potential (the third screening).
Evaluation of Bioremediation Potential by Functional Strains
Activation of Soil Metals by Functional Strains
Batch experiments on the effects of the 10 functional isolates on metal mobility in soil were conducted in triplicate 50-mL scaled polypropylene centrifuge tubes according to Chen et al. (2005). Briefly, pure cultures of functional strains were centrifuged at 8000 rpm for 10 min after 20 h of growth, washed twice in phosphate buffer (pH 7.0), and re-suspended in sterile distilled water. One milliliter of each washed bacterial suspension (OD600 = 1.0 ± 0.05) or sterile water (control) was added to the 1 g of autoclaved soils. All tubes were weighed, wrapped in brown paper, and placed on an orbital shaker at 180 rpm and 28°C. After 1 week, the tubes were weighed again to compensate for evaporation. Sterile water (10 mL) was then added to extract water-soluble metals. The soil suspensions were vibrated at 25°C for 2 h and centrifuged at 10,000 rpm for 10 min. The resulting supernatants were filtered through a 0.22-μm membrane filter for determination of pH and water-soluble Cu/Zn/Pb/Cd/Fe. The metal concentrations were determined by inductively coupled plasma-mass spectrometry (ICP-MS, SPECTRO).
Minimum Inhibitory Concentration (MIC) of Functional Strains
To check the extent of resistance, we used the secondly selected isolates to determine the lowest concentration of Cu and Zn that completely inhibited the growth of bacterial strains, termed as the minimum inhibitory concentration (MIC). Isolates were streaked in triplicate on LB agar media supplemented with varying concentrations (600 to 1000 mg⋅L-1) of Cu and Zn, respectively. For each strain and each metal, the lowest concentration that inhibited visible growth at 28°C within 3 days was determined.
Metal Biosorption Analyses
The biosorption of Cu and Zn by bacterial cells was evaluated as described by Hernández et al. (1998) with some modifications. Bacterial cells obtained from the bacterial cultures (grown in LB broth at 28°C, OD600 = 1.0 ± 0.05) were harvested by centrifugation at 8000 rpm for 20 min and washed twice with sterile deionized water. The harvested cells were re-suspended in 150 mg⋅L-1 of Cu or Zn. An uninoculated solution was used as the control. After incubation at room temperature for 10 h, the cells were harvested following centrifugation and the residual metal ions in the supernatant were measured using a flame atomic absorption spectrophotometer (Varian Spectra model AA240FS; USA). The amount of metal absorbed by the bacterial cells was calculated by subtracting the metal concentration in the supernatant from the original concentration.
In vivo Plant Growth Promotion Assay
Growth promotion of the secondly selected isolates was tested according to Patten and Glick (2002) with some modifications. Seeds of B. napus var. Zhongyou-1 were surface-sterilized with a mixture of absolute ethanol and 30% hydrogen peroxide (1:1, v/v) for 20 min, and washed twice with sterile distilled water before being transferred to sterile filter paper in a Petri dish. Seed sterility was monitored by incubating the seeds on LB agar at 30°C and aseptically placed on moistened filter paper. Then 6 mL of each bacterial suspension (OD600 = 0.5 ± 0.02) or sterile distilled water (uninoculated control) was added to glass Petri dishes with two-double filter paper. After incubation of closed Petri dishes for 7 days at 28°C in the dark, the root length, shoot length, fresh weight, and number of seedlings that had sprouted within 3 days were determined. The assay was performed twice with two dishes (10 seeds per dish) for each treatment. After the third screening, among the 10 functional strains, S44 with the highest bioremediation potentials was selected for genetic identification.
Genetic Identification of S44
Genomic DNA of S44 was extracted as per a previously reported protocol (Araújo et al., 2002), and used as a template in 16S rDNA PCR amplification with universal primers 27F (5′-GAGTTTGATCACTGGCTCAG-3′) and 1492R (5′-TACGGCTACCTTGTTACGACTT-3′) (Byers et al., 1998). PCR amplification was performed in a DNA Engine Thermal Cycler (PTC-200, BioRad, USA) under the reaction conditions described by (Branco et al., 2005). The amplified product was purified with a DNA Purification Kit and sequenced at HuaDa Biotechnology Company (Shanghai, China). The partial 16S rDNA sequences obtained were matched with nucleotide sequences in GenBank using the BLAST tool1 Neighbor joining phylogenetic trees were constructed after calculation of a maximum composite likelihood distance matrix using the MEGA 4.0 software (Tamura et al., 2007).
Sand Culture Experiment
Based on the results of the third screening, the Acinetobacter sp. FQ-44 was selected for preliminarily exploring roles of the plant-rhizobacteria partnership in heavy metal remediation. Surface-sterilized seeds of B. napus were pregerminated on sterile filter paper in a Petri dish. After germination (4 days), uniform seedlings were selected and soaked for 2 h in the bacterial culture (OD600 of 1.0 ± 0.05) or sterile water (control). Six seedlings were subsequently transplanted into a plastic pot (top diameter 85 mm, bottom diameter 65 mm, and height 105 mm) containing sterilized vermiculite and saturated with sterile half-strength Hoagland’s nutrient solution (Barac et al., 2004). One week after transplantation, seedlings were thinned to three per pot and subjected to various concentrations of Cu (2, 5, and 10 mg/L). Three replicates were conducted for each treatment. The plantlets were allowed to grow under greenhouse conditions (25 ± 5°C, 16:8 day/night regime). After 45 days, plants were carefully removed from the pots and root surfaces were immersed in 0.01 M EDTA for 30 min, and then rinsed thoroughly with deionized water to remove any surface adsorbed metals. Fresh and dry weights were measured and the concentrations of Cu in roots and shoots were determined using a flame atomic adsorption spectrophotometer. The translocation factor (TF) was calculated as the ratio of metal concentration in the shoots to that in the roots (Liu et al., 2009) and the bioaccumulation factor (BCF) was calculated as the ratio of metal contents in the entire plant to that in the soil (Bu-Olayan and Thomas, 2009).
Statistical Analyses
Results for each treatment were expressed as means ± SD. Significant differences between parameters were tested using the post hoc Fisher’s protected least significant difference (LSD) test after one-way ANOVA. All statistical analyses, including the Pearson’s correlation analysis, were conducted using SPSS 18.0 (SPSS Inc., USA). Unless otherwise indicated, significant level was set at P < 0.05. Graphical analyses were performed on SigmaPlot 11.0 (Jandel Scientific, USA).
Results and Discussion
Isolation and Preliminary Screening of Cu/Zn-Tolerant and ACC-Utilizing Rhizobacteria
Before preliminary screening and identification, 60 cultivable isolates that were simultaneously resistant to 50 mg⋅L-1 of Zn and 50 mg⋅L-1 Cu, were isolated initially from the rhizosphere of S. oleraceus and named S1–S60. These bacterial isolates were autochthonous to the metal-polluted site and were thus more suitable for in situ phytoremediation of the multi-metal-polluted soils. As reported, rhizobacteria isolated from multi-metal-polluted natural environments can be constitutively or adaptively resistant to increasing metal concentrations, as they have adapted to such environments (Nies, 2003).
Soil microbes with generally higher metal resistance are the preferred choice for phytoremediation studies. Our results indicate that most of the isolates tested were resistant to different concentrations of Zn and Cu (Supplementary Table S1). Among all isolates, 34 were simultaneously resistant to 400 mg⋅L-1 Zn and 400 mg⋅L-1 Cu, among which some were even tolerant of 500 mg⋅L-1 Zn or Cu; whereas 46 isolates were able to simultaneously resist 300 mg⋅L-1 Zn and 300 mg⋅L-1 Cu. To obtain more plant beneficial strains, these 46 isolates were selected for further testing of their ACC utilization ability.
Among those 46 isolates, 19 isolates grew significantly better on ADF and NDF than on DF (P < 0.05) (Figure 1). Although these isolates grew well on ADF and NDF, their growth without a nitrogen source was limited (Figure 1). Thus, these 19 rhizobacteria had the potential to utilize ACC as a sole nitrogen source. Moreover, they had the ability to grow on ADF to produce ACCD (Figure 1; Supplementary Table S2), which was supported by earlier observations that ACC-utilizing bacteria could generally produce ACCD. As reported, ACC-utilizing bacteria have been found to facilitate plant growth by producing ACCD that hydrolyzes the ethylene precursor ACC into α-ketobutyrate and ammonia (Glick, 2005) in the presence of salts or heavy metals (Belimov et al., 2005; Zahir et al., 2009). Consequently, these ACC-utilizing isolates could be important for PGPR-mediated phytoremediation.
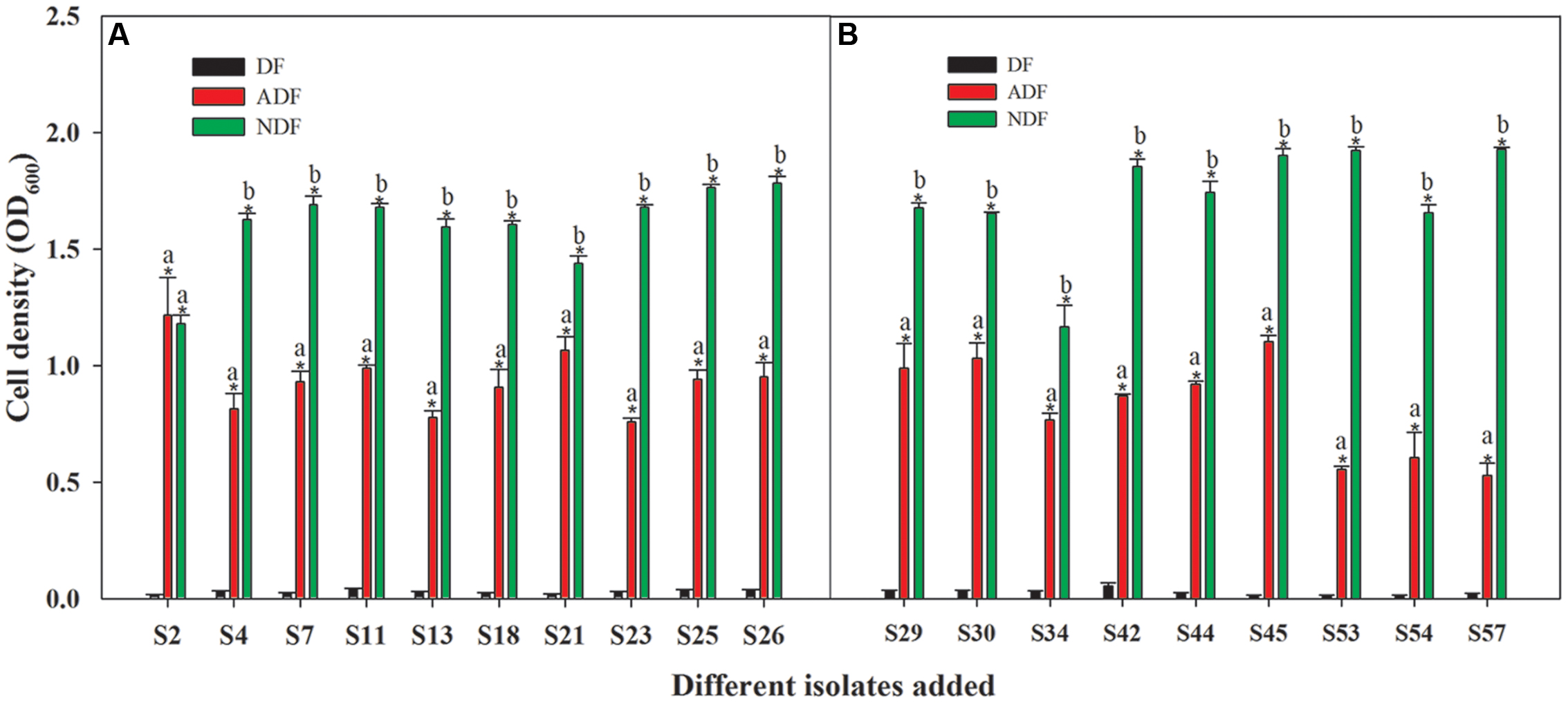
FIGURE 1. Capacity for growth of 19 Cu/Zn-resistant isolates on ADF (A,B). Different small letters denote significant differences between treatments and an asterisk (∗) represents a significantly greater value on ADF and NDF compared to DF (P < 0.05).
Screening of Functional Strains with Superior PGP Ability
Various PGP characteristics could contribute to reduced metal stress and increased growth in their host plants (Ma et al., 2011; Rajkumar et al., 2012). In our study, all 19 ACC-utilizing isolates had inherent abilities of IAA production, siderophore production, and insoluble phosphate solubilization (Supplementary Table S2). Out of 19 isolates, 10 with superior PGP traits were selected for statistical analyses (Table 2), because each had three indices that were all ranked in the top 10.
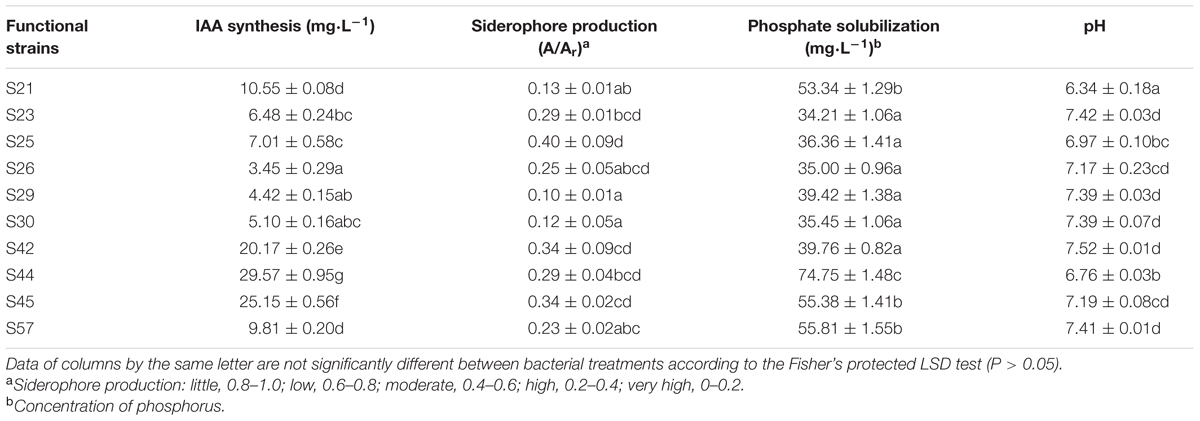
TABLE 2. PGP features of functional strains and pH of solutions in the phosphate solubilization experiment.
As shown in Table 2, S44, the best IAA producer (29.57 mg⋅L-1) in our study, produced significantly more IAA than the other nine strains (P < 0.05). As reported similarly, Enterobacter ludwigii BNM 0357 released about 30 μg IAA mL-1 (Shoebitz et al., 2009). In addition, the IAA production abilities of all 10 isolates might be within a reasonable range for observable PGP effects (Ma et al., 2009b) that might contribute to increased plant biomass. As reported, a low IAA production by PGP bacteria promotes primary root elongation, whereas a high level inhibit primary root growth (Xie et al., 1996). Our rape inoculation experiments also indicated the 10 moderate IAA producers were able to increase root length, which was generally promoted by IAA-producing rhizobacteria (Patten and Glick, 1996). Moreover, Pearson’s correlation analysis also revealed that IAA was significantly positively correlated with the fresh weight of seedlings (r = 0.70, P = 0.02).
Siderophores, another important PGPR-released metabolites, indirectly alleviate heavy metal toxicity by increasing the supply of iron to plants (Burd et al., 2000), thereby facilitating plant growth. In our study, siderophore production was highest in S29 among the 10 isolates, whereas it was lowest in S25 (Table 2). Furthermore, siderophores were responsible for the mobilization of insoluble metals such as Fe (Table 3) and were positively correlated with three growth parameters (Supplementary Table S3), although this was not significant (P > 0.05). Our results concurring with the earlier observations also show that siderophores produced by rhizosphere microorganisms could supply iron to plants via Fe-siderophore complexes under iron-limited conditions (Crowley et al., 1988) and inoculation with a siderophore-producing strain promotes plant growth (Tripathi et al., 2005).
Another crucial PGP mechanism is phosphate solubilization, through which microbes enhance P availability to the host plant and thereby contribute to plant–bacteria interactions and PGP effects in metal-polluted soils (Zaidi et al., 2006). Our findings indicate that phosphate solubilization was positively correlated with all growth parameters (r = 0.45, 0.18, and 0.51 for root length, shoot length, and fresh weight, respectively) (Supplementary Table S3). Moreover, Rajkumar et al. (2009) also reported that phosphate solubilization in the rhizosphere greatly contributes to the PGP effects of bacteria. In addition, the highest phosphate-solubilizing ability was also observed in S44 (74.75 mg⋅L-1), which was significantly higher than other nine isolates (P < 0.05, Table 2).
The foregoing analyses indicate that these isolates were able to facilitate the growth of B. napus probably through these PGP traits. Consequently, the screening of soil bacteria with superior PGP abilities in a multi-metal-polluted environment is one key step in phytoremediation studies.
Final Choice of S44
Effects of Functional Strains on the Mobility of Soil Metals
Besides PGP traits, successful phytoremediation also depends mainly on metal bioavailability in the soil (Shallari et al., 2001). Therefore, to obtain effective metal-mobilizing strains, we further evaluated the ability of 10 isolates to increase water-soluble Cu, Zn, Cd, Pb, and Fe concentrations in soils. As expected, the presence of bacteria resulted in increased concentrations of water-extractable Cu, Zn, Pb, Cd, and Fe in autoclaved soil compared to axenic soil (Figure 2). These results suggest that the 10 Cu/Zn-resistant isolates had metal-solubilizing potential in heavy metal-polluted soil, thereby increasing metal bioavailability. As reported, Soil microorganisms can affect metal mobility and availability via the release of siderophores (Braud et al., 2009) and solubilization of metal phosphates (Aboushanab et al., 2006). Our results also indicate that siderophore and phosphate solubilization were both positively correlated with concentrations of water-soluble Cu, Zn, Pb, Cd, and Fe (Table 3).
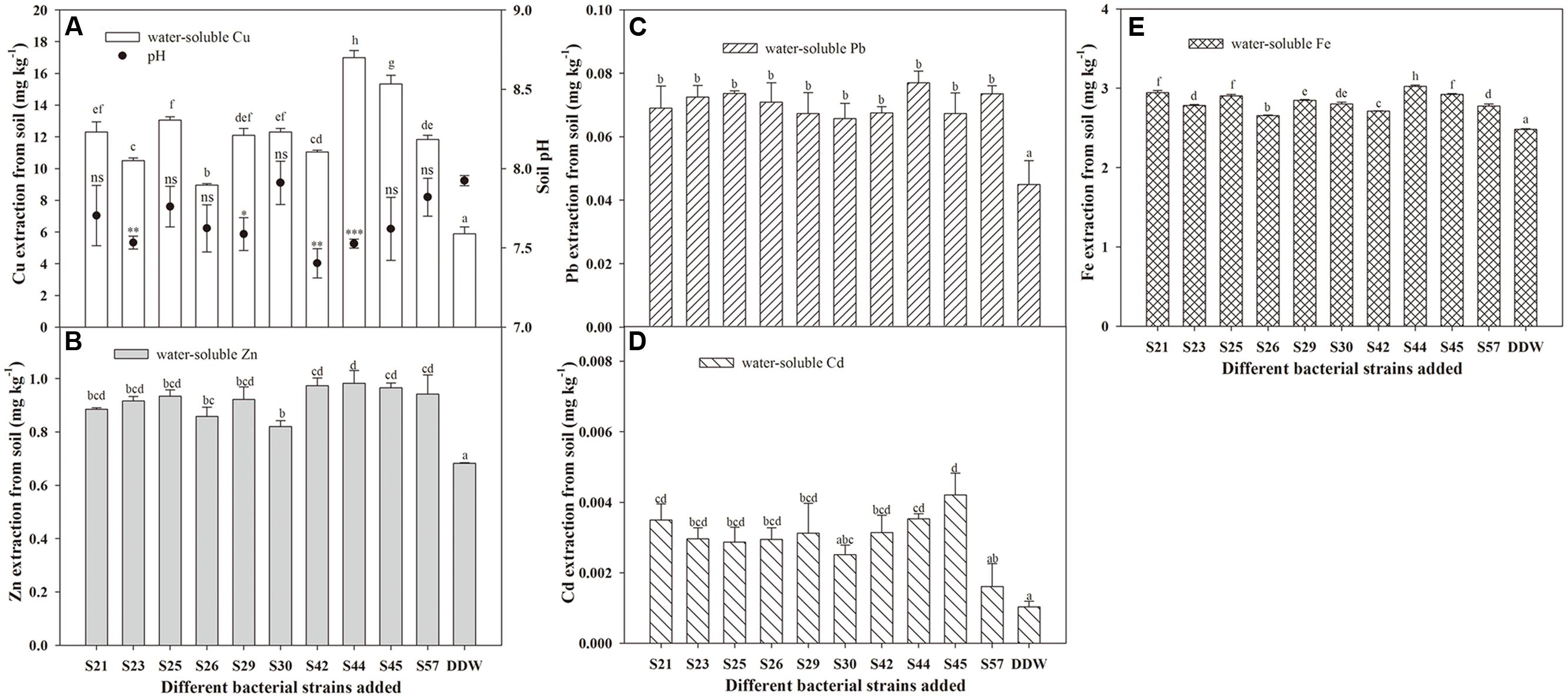
FIGURE 2. Effects of inoculation with 10 isolates on mobilization of Cu (A), Zn (B), Pb (C), Cd (D), and Fe (E) in autoclaved soil. Values are expressed as means ± SE, n = 9. ∗P < 0.05, ∗∗P < 0.01, ∗∗∗P < 0.001; ns, no significant difference. Different letters above the bar indicate significant differences among treatments at the level of P < 0.05 according to the Fisher’s protected LSD test.
Although all 10 isolates had the potential to facilitate the release of non-labile-phase Cu, Zn, Cd, Pb, and Fe from sterile soils, their effects actually differed (Figure 2). For example, the greatest amounts of water-soluble Cu, Zn, Pb, and Fe released in the soil were all found in S44, which were 16.99, 0.98, 0.08, 3.03 mg⋅L-1, respectively, but that of water-soluble Cd was observed in S45. Moreover, inoculation with S44 significantly increased the concentrations of water-soluble Cu, Zn, Pb, Cd, and Fe in soil by 1.88-, 0.44-, 0.71-, 2.50-, and 0.22-fold, respectively, compared to the control. Furthermore, the soil pH following inoculation with S44 dropped significantly compared to the control (P < 0.05; Table 2).
In addition, mobilization characteristics differed among the metals (Figure 2), which could be explained by the physicochemical properties of the various metals, metal-microbe interactions, as well as the unordered competition between metals. However, some isolates, such as S44 and S45, that exhibited high mobilization of one metal, were also remarkably capable of mobilizing other metals.
MIC of Functional Strains
The preliminary resistance results showed that some isolates were able to grow in higher concentrations of all tested metals. Thus, to determine the extent of resistance, we assessed the Cu and Zn MICs of the secondly selected isolates. Our toxicity tests show that S26, S42, S44, S45, and S57 tolerated relatively high levels of Cu and Zn (Table 4). Moreover, among the 10 functional strains, S42, S44, and S45 had the highest Cu (1000 mg⋅L-1) and Zn (800 mg⋅L-1) MICs. This high tolerance of Cu and Zn could be attributed to the fact that these bacteria were isolated from the sewage-amended soils containing high levels of Cu and Zn. However, strain S21 was less tolerant of Cu (600 mg⋅L-1) and Zn (400 mg⋅L-1). In addition, the present results also indicate that Zn was more toxic to the isolates than Cu, which was different from some previous studies (Hassen et al., 1998; Jiang et al., 2008; Guo et al., 2011).
Metal Biosorption Potential of Secondly Selected Isolates
With respect to microbial remediation, it is very important to determine whether selected bacteria have the capacity for metal uptake. Our results indicate that different isolates exhibited different capacities for biosorption of the metal ions tested (Figure 3). Moreover, S44 exhibited the highest potentials to remove Cu (7.53 mg⋅g-1 dry cell) and Zn (6.61 mg⋅g-1 dry cell), and absorbed significantly more Cu and Zn than the other nine isolates (P < 0.05). Thus, application of the effective metal-solubilizing/absorbing S44 would be helpful for improving microbe-assisted phytoremediation. As reported, the biosorption capacity of bacteria plays an important role in reducing metal phytotoxicity by limiting the entry of metal ions into plant cells, and might contribute to enhanced plant growth in metal-contaminated soils (Ma et al., 2011). Furthermore, it should be noted that the biosorption ability for Cu was higher than that for Zn (Figure 3). One possible explanation could be that Cu (0.72 Å) with smaller ionic radius might be more rapidly complexed by bacterial cell wall/membrane compared to Zn (0.88 Å) (Karakagh et al., 2012). Another explanation probably was that Zn was more toxic to these isolates than Cu.
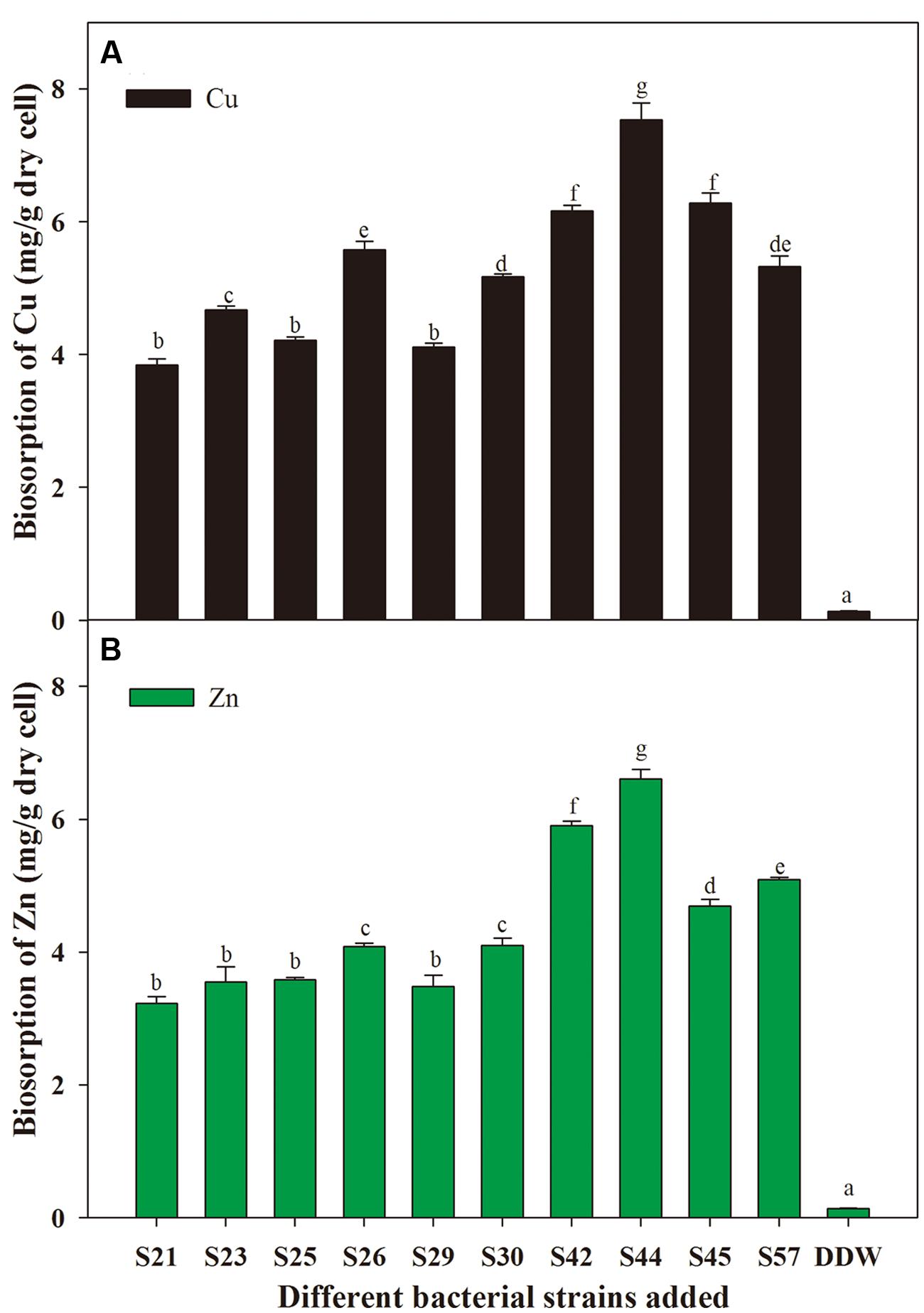
FIGURE 3. Biosorption of Cu (A) and Zn (B) by secondly selected isolates. Different letters above the bar indicate significant differences among treatments at the level of P < 0.05 according to the Fisher’s protected LSD test.
Effects of Functional Strains on Rape Growth
After the 10 representative isolates infecting sterile B. napus L. seeds, seed germination was neither significantly inhibited nor stimulated. For example, seed germination after inoculation with S23 was equal to that of the control (Table 5).
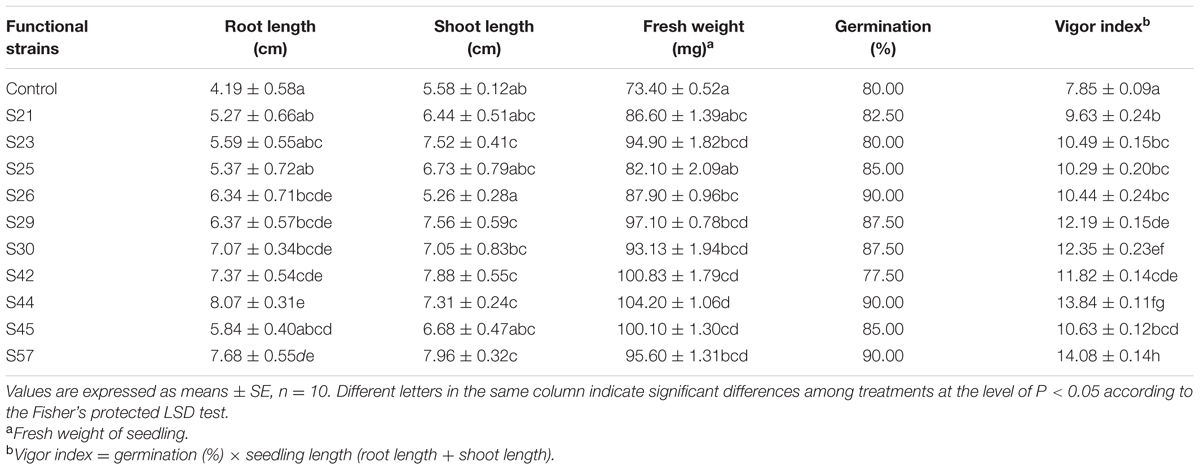
TABLE 5. Growth parameters of Brassica napus L. seedlings in sterile filter paper following infection with 10 isolates.
A deeper understanding of plant-microbe interactions is complicated, but applicable to microbe-assisted phytoreme diation. In our study, seeds inoculated with the various isolates all had longer roots compared to the control (Table 5). Moreover, the most significant increase in root length was observed with S44 (92.60%, P < 0.05). Although the maximum shoot elongation was observed with S57, inoculation with S44 significantly increased shoot length by 31.00% (P < 0.05), compared to the control. Furthermore, the maximum promoting effect on fresh weight was also observed with S44, showing a significant increase by 41.96% (P < 0.05; Table 5). In addition, the highest seed vigor index was observed with S57 followed by S44 and S30, all exhibiting significant effects (P < 0.05). The foregoing results indicate that S44 has higher potential to facilitate the growth of B. napus.
Although the selected isolates showed PGP effects, these responses were not evaluated in the presence of metal stress, which would more effectively demonstrate PGPR-mediated phytoremediation. Of the 10 functional strains, S44 was selected as the most active strain (tolerance of up to 800 mg⋅L-1 Zn and 1000 mg⋅L-1 Cu, adsorption/solubilization of the largest quantities of Cu and Zn, the maximum root length and fresh weight-promoting effects) for molecular identification.
Molecular Identification of Strain S44
S44 was identified as a species of Acinetobacter sp. by 16S rDNA gene sequencing and was named Acinetobacter sp. FQ-44. The highest sequence similarity (99%) and the phylogenetic tree in Figure 4, based on 16S rDNA sequences reveal a relationship between FQ-44 and other relevant bacteria reported. The 16S rDNA sequences (1443 bp) of FQ-44 were deposited in GenBank under accession No. KU206487.
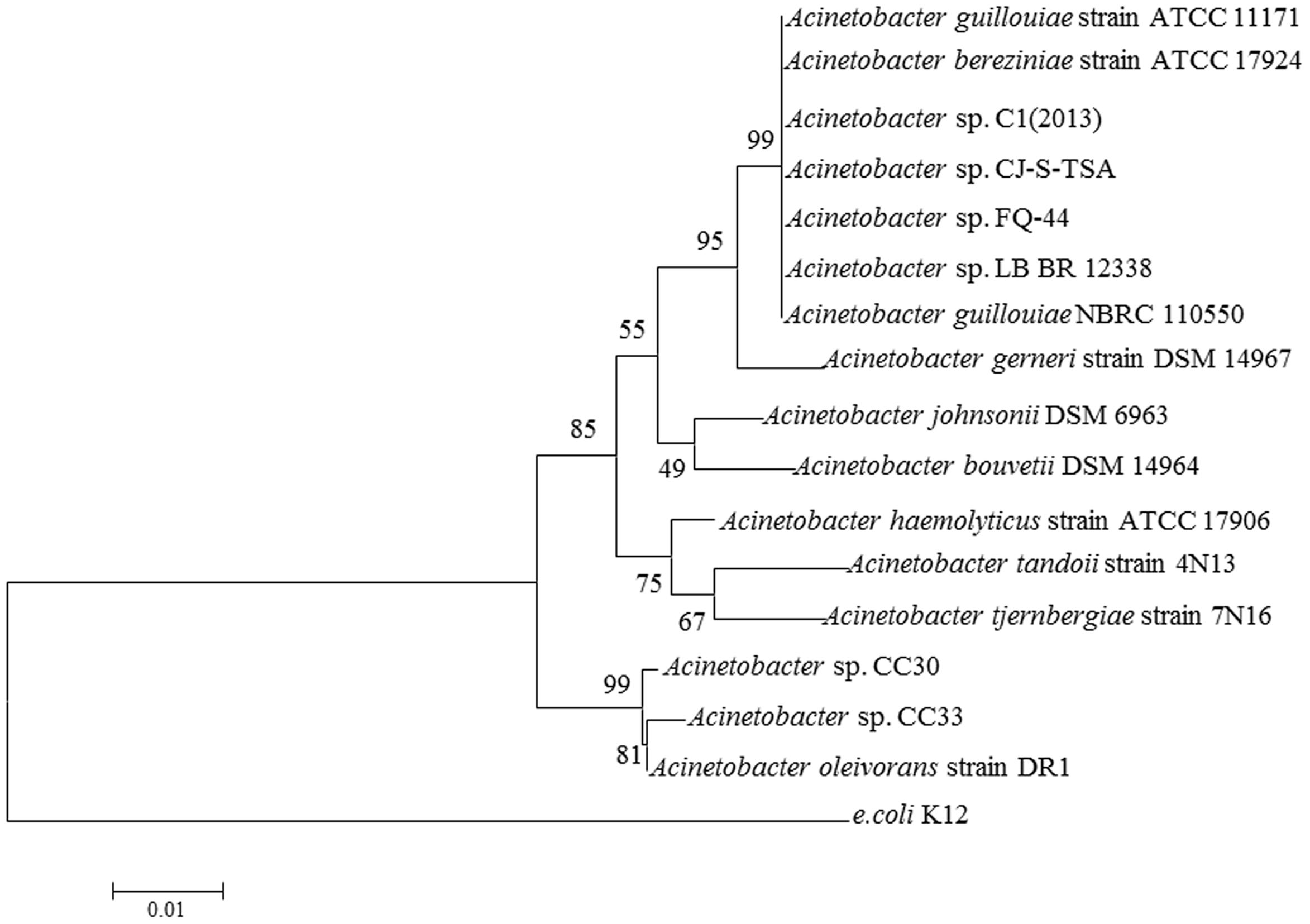
FIGURE 4. Neighbor joining phylogenetic tree analysis of Acinetobacter sp. FQ-44 with closely related strains from GenBank and relevant reports. The scale bar represents 0.01 substitutions per site.
Influence of FQ-44 on Growth and Cu Uptake by B. napus
The plant–bacteria partnership can be applied to increase the phytoremediation efficiency of soil and water contaminated with organic and/or inorganic pollutants (Khan et al., 2015). Therefore, the effects of metal-mobilizing FQ-44 on growth and metal uptake/translocation by B. napus were evaluated. As expected, FQ-44 significantly increased the dry weight of B. napus cultivated in different concentrations of Cu (Figure 5). In general, inoculation with FQ-44 significantly increased plant uptake of Cu (Table 6), which is consistent with significant improvements of BCF of Cu induced by FQ-44. Moreover, FQ-44 also significantly increased the TF of Cu (P < 0.05, Table 6), besides the Cu concentration of 2 mg/L. Yoon et al. (2006) also demonstrated that plants with a greater BCF and TF have the potential for use in heavy metal phytoextraction. The above results suggest that FQ-44 can be used to facilitate the phytoextraction of Cu. Previously, Rojas-Tapias et al. (2012) also reported that Acinetobacter sp. CC30 significantly enhanced Cu uptake by sunflowers. Moreover, Jing et al. (2014) reported that Enterobacter sp. JYX7 and Klebsiella sp. JYX10 significantly improved Zn uptake by B. napus. Recently, Płociniczak et al. (2016) also reported that Brevibacterium casei MH8a colonized white mustard plant tissues and enhanced Cu and Zn phytoextraction.
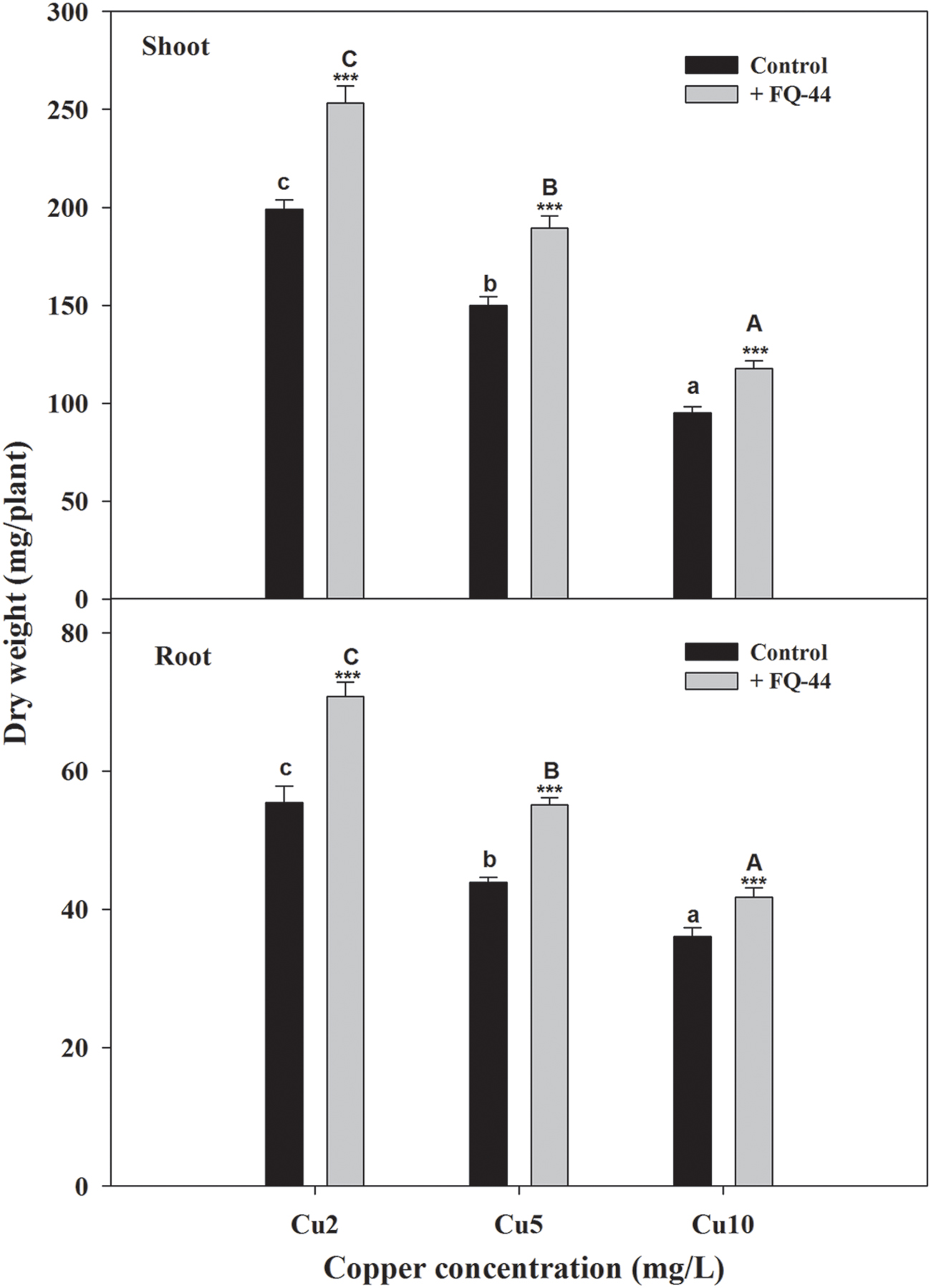
FIGURE 5. Effects of FQ-44 on dry weight of root and shoot in Brassica napus cultivated in different concentrations of Cu. Values are expressed as means ± SE, n = 9, ∗∗∗P < 0.001. Different letters above the bar indicate significant differences within the same microbial treatments (control and inoculation) at the level of P < 0.05, according to the Fisher’s protected LSD test. Cu2, 2 mg/L Cu; Cu5, 5 mg/L Cu; Cu10, 10 mg/L Cu.
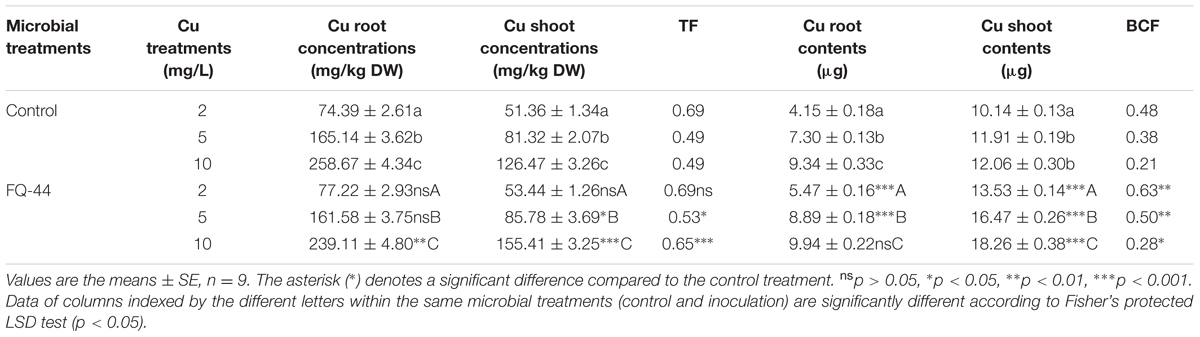
TABLE 6. Effects of FQ-44 on accumulation, uptake, BCF, and TF of Cu in B. napus cultivated in the presence of Cu at various concentrations.
Although FQ-44 showed PGP effects on rape and enhanced phytoextraction of Cu, its colonization and survival properties are crucial features to evaluate its capacity for promoting sustainable plant growth and cope with metal stress in contaminated sites (Ma et al., 2011). Therefore, future studies using pot experiments containing in situ soils are needed to examine the specific effects of selected FQ-44 on the growth of host plants, and to determine whether it has the advantage of rhizosphere colonization.
Conclusion
In the present study, the selection of Cu/Zn-resistant FQ-44 isolated from S. oleraceus was evaluated through three inter-causal screenings. Our results indicate that FQ-44 has potential to facilitate B. napus growth and enhance phytoextraction of Cu by sand culture experiment, which could be attributed to beneficial PGP traits; increased concentrations of water-soluble Cu, Cd, Zn, Pb, and Fe; and tolerance and adsorption of Cu and Zn that effectively improved microbe-assisted phytoremediation. Consequently, these advantages confer bioinoculant properties to FQ-44 that would be helpful for enhancing phytoremediation efficiency of multi-metal-polluted soils, particularly Cu/Zn-contaminated soils. Moreover, the proposed approach to screening in the present study could be useful for the isolation of effective strains and improvement of phytoremediation.
Although FQ-44 possessed PGP traits to facilitate B. napus growth and critical bioremediation potentials, in many cases PGP bacteria failed to induce the desired effects, when applied in a natural environment. Further research will address: (1) the interactions between FQ-44 and host plants; (2) the colonization potential of FQ-44 and mechanisms contributing to increased plant biomass and metal uptake/translocation by pot experiment containing in situ soils; and (3) the roles of FQ-44 in field phytoremediation experiments.
Author Contributions
Conceived and designed the experiments: XW, QF, ZF, and YX. Conducted the work: QF. Analyzed the data: XW, QF, ZF, and YX. Contributed reagents/materials/analysis tools: XW, ZF, YX, and KL, YL. Wrote the manuscript: QF.
Funding
This research was supported by a grant from the National Key Research and Development Program of China (2016YFC0502700), the Major project of Chinese National Social Science (No: 14ZDB140) and the National Science Foundation of China for Yong Scholars (No: KRH1829152).
Conflict of Interest Statement
The authors declare that the research was conducted in the absence of any commercial or financial relationships that could be construed as a potential conflict of interest.
Acknowledgments
We specially thank the reviewers and the editor for providing their constructive comments that greatly improve the manuscript. We also thank GTP and CC for their assistance in the experiment and XYL for help with data analysis. We would like to thank Shanghai Academy of Agricultural Sciences for kindly donating the seeds of B. napus for this experiment.
Supplementary Material
The Supplementary Material for this article can be found online at: http://journal.frontiersin.org/article/10.3389/fpls.2016.01487
Footnotes
References
Aboushanab, R., Angle, J., and Chaney, R. (2006). Bacterial inoculants affecting nickel uptake by Alyssum murale from low, moderate and high Ni soils. Soil Biol. Biochem. 38, 2882–2889. doi: 10.1016/j.soilbio.2006.04.045
Adediran, G. A., Ngwenya, B. T., Mosselmans, J. F. W., and Heal, K. V. (2016). Bacteria-zinc co-localization implicates enhanced synthesis of cysteine-rich peptides in zinc detoxification when Brassica juncea is inoculated with Rhizobium leguminosarum. New Phytol. 209, 280–293. doi: 10.1111/nph.13588
Adediran, G. A., Ngwenya, B. T., Mosselmans, J. F. W., Heal, K. V., and Harvie, B. A. (2015). Mechanisms behind bacteria induced plant growth promotion and Zn accumulation in Brassica juncea. J. Hazard. Mater. 283, 490–499. doi: 10.1016/j.jhazmat.2014.09.064
Alford,É. R., Pilon-Smits, E. A., and Paschke, M. W. (2010). Metallophytes-a view from the rhizosphere. Plant Soil 337, 33–50. doi: 10.1007/s11104-010-0482-3
Araújo, W. L., Marcon, J., Maccheroni, W., van Elsas, J. D., van Vuurde, J. W., and Azevedo, J. L. (2002). Diversity of endophytic bacterial populations and their interaction with Xylella fastidiosa in citrus plants. Appl. Environ. Microbiol. 68, 4906–4914. doi: 10.1128/AEM.68.10.4906-4914.2002
Barac, T., Taghavi, S., Borremans, B., Provoost, A., Oeyen, L., Colpaert, J. V., et al. (2004). Engineered endophytic bacteria improve phytoremediation of water-soluble, volatile, organic pollutants. Nat. Biotechnol. 22, 583–588. doi: 10.1038/nbt960
Belimov, A., Hontzeas, N., Safronova, V., Demchinskaya, S., Piluzza, G., Bullitta, S., et al. (2005). Cadmium-tolerant plant growth-promoting bacteria associated with the roots of Indian mustard (Brassica juncea L. Czern.). Soil Biol. Biochem. 37, 241–250. doi: 10.1016/j.soilbio.2004.07.033
Branco, R., Chung, A. P., Veríssimo, A., and Morais, P. V. (2005). Impact of chromium-contaminated wastewaters on the microbial community of a river. FEMS Microbiol. Ecol. 54, 35–46. doi: 10.1016/j.femsec.2005.02.014
Braud, A., Jézéquel, K., Bazot, S., and Lebeau, T. (2009). Enhanced phytoextraction of an agricultural Cr- and Pb-contaminated soil by bioaugmentation with siderophore-producing bacteria. Chemosphere 74, 280–286. doi: 10.1016/j.chemosphere.2008.09.013
Bric, J. M., Bostock, R. M., and Silverstone, S. E. (1991). Rapid in situ assay for indole acetic acid production by bacteria immobilized on a nitrocellulose membrane. Appl. Environ. Microbiol. 57, 535–538.
Bu-Olayan, A. H., and Thomas, B. V. (2009). Translocation and bioaccumulation of trace metals in desert plants of Kuwait Governorates. Res. J. Environ. Sci. 3, 581–587. doi: 10.3923/rjes.2009.581.587
Burd, G. I., Dixon, D. G., and Glick, B. R. (2000). Plant growth-promoting bacteria that decrease heavy metal toxicity in plants. Can. J. Microbiol. 46, 237–245. doi: 10.1139/w99-143
Byers, H. K., Stackebrandt, E., Hayward, C., and Blackall, L. L. (1998). Molecular investigation of a microbial mat associated with the great artesian basin. FEMS Microbiol. Ecol. 25, 391–403. doi: 10.1111/j.1574-6941.1998.tb00491.x
Chen, L., Luo, S. L., Li, X. J., Wan, Y., Chen, J. L., and Liu, C. B. (2014). Interaction of Cd-hyperaccumulator Solanum nigrum L. and functional endophyte Pseudomonas sp. Lk9 on soil heavy metals uptake. Soil Biol. Biochem. 68, 300–308. doi: 10.1016/j.soilbio.2013.10.021
Chen, Y. X., Wang, Y. P., Lin, Q., and Luo, Y. M. (2005). Effect of copper-tolerant rhizosphere bacteria on mobility of copper in soil and copper accumulation by Elsholtzia splendens. Environ. Int. 31, 861–866. doi: 10.1016/j.envint.2005.05.044
Crowley, D. E., Reid, C. P., and Szaniszlo, P. J. (1988). Utilization of microbial siderophores in iron acquisition by oat. Plant Physiol. 87, 680–685. doi: 10.1104/pp.87.3.680
De Souza, M., Huang, C., Chee, N., and Terry, N. (1999). Rhizosphere bacteria enhance the accumulation of selenium and mercury in wetland plants. Planta 209, 259–263. doi: 10.1007/s004250050630
Dell’Amico, E., Cavalca, L., and Andreoni, V. (2008). Improvement of Brassica napus growth under cadmium stress by cadmium-resistant rhizobacteria. Soil Biol. Biochem. 40, 74–84. doi: 10.1016/j.soilbio.2007.06.024
Dworkin, M., and Foster, J. (1958). Experiments with some microorganisms which utilize ethane and hydrogen. J. Bacteriol. 75, 592–603.
Glick, B. R. (2003). Phytoremediation: synergistic use of plants and bacteria to clean up the environment. Biotechnol. Adv. 21, 383–393. doi: 10.1016/S0734-9750(03)00055-7
Glick, B. R. (2005). Modulation of plant ethylene levels by the bacterial enzyme ACC deaminase. FEMS Microbiol. Lett. 251, 1–7. doi: 10.1016/j.femsle.2005.07.030
Glick, B. R. (2010). Using soil bacteria to facilitate phytoremediation. Biotechnol. Adv. 28, 367–374. doi: 10.1016/j.biotechadv.2010.02.001
Glick, B. R., Patten, C. L., Holguin, G., and Penrose, D. (1999). Biochemical and Genetic Mechanisms Used by Plant Growth Promoting Bacteria. London: Imperial College Press.
Guo, J., Tang, S., Ju, X., Ding, Y., Liao, S., and Song, N. (2011). Effects of inoculation of a plant growth promoting rhizobacterium Burkholderia sp. D54 on plant growth and metal uptake by a hyperaccumulator Sedum alfredii Hance grown on multiple metal contaminated soil. World J. Microbiol. Biotechnol. 27, 2835–2844. doi: 10.1007/s11274-011-0762-y
Hassen, A., Saidi, N., Cherif, M., and Boudabous, A. (1998). Resistance of environmental bacteria to heavy metals. Bioresour. Technol. 64, 7–15. doi: 10.1016/S0960-8524(97)00161-2
Hernández, A., Mellado, R. P., and Martínez, J. L. (1998). Metal accumulation and vanadium-induced multidrug resistance by environmental isolates of Escherichia hermannii and Enterobacter cloacae. Appl. Environ. Microbiol. 64, 4317–4320.
Holm, L., Plunknett, D., Poncho, J., and Herberger, J. (1977). The World’s Worst Weeds: Distribution and Biology. Honolulu, HI: The University Press of Hawaii.
Honma, M., and Shimomura, T. (1978). Metabolism of 1-aminocyclopropane-1-carboxylic acid. Agric. Biol. Chem. 42, 1825–1831. doi: 10.1271/bbb1961.42.1825
Hutchinson, I., Colosi, J., and Lewin, R. A. (1984). The biology of canadian weeds, 63: Sonchus asper (L.) Hill and S. oleraceus L. Can. J. Plant Sci. 64, 731–744. doi: 10.4141/cjps84-100
Jiang, C. Y., Sheng, X. F., Qian, M., and Wang, Q. Y. (2008). Isolation and characterization of a heavy metal-resistant Burkholderia sp. from heavy metal-contaminated paddy field soil and its potential in promoting plant growth and heavy metal accumulation in metal-polluted soil. Chemosphere 72, 157–164. doi: 10.1016/j.chemosphere.2008.02.006
Jing, Y. X., Yan, J. L., He, H. D., Yang, D. J., Xiao, L., Zhong, T., et al. (2014). Characterization of bacteria in the rhizosphere soils of Polygonum pubescens and their potential in promoting growth and Cd, Pb, Zn uptake by Brassica napus. Int. J. Phytoremediation 16, 321–333. doi: 10.1080/15226514.2013.773283
Karakagh, R. M., Chorom, M., Motamedi, H., Kalkhajeh, Y. K., and Oustan, S. (2012). Biosorption of Cd and Ni by inactivated bacteria isolated from agricultural soil treated with sewage sludge. Ecohydrol. Hydrobiol. 12, 191–198. doi: 10.1016/S1642-3593(12)70203-3
Kärenlampi, S., Schat, H., Vangronsveld, J., Verkleij, J., van der Lelie, D., Mergeay, M., et al. (2000). Genetic engineering in the improvement of plants for phytoremediation of metal polluted soils. Environ. Pollut. 107, 225–231. doi: 10.1016/S0269-7491(99)00141-4
Khan, A., Chaudhry, T., Hayes, W., Khoo, C., Hill, L., Fernandez, R., et al. (1998). Physical, chemical and biological characterisation of a steelworks waste site at Port Kembla, NSW, Australia. Water Air Soil Pollut. 104, 389–402. doi: 10.1023/A:1004951530917
Khan, M. U., Sessitsch, A., Harris, M., Fatima, K., Imran, A., Arslan, M., et al. (2015). Cr-resistant rhizo- and endophytic bacteria associated with Prosopis juliflora and their potential as phytoremediation enhancing agents in metal-degraded soils. Front. Plant Sci. 5:755. doi: 10.3389/fpls.2014.00755
Kozdrój, J., Trevors, J., and Van Elsas, J. (2004). Influence of introduced potential biocontrol agents on maize seedling growth and bacterial community structure in the rhizosphere. Soil Biol. Biochem. 36, 1775–1784. doi: 10.1016/j.soilbio.2004.04.034
Kumar, P. N., Dushenkov, V., Motto, H., and Raskin, I. (1995). Phytoextraction: the use of plants to remove heavy metals from soils. Environ. Sci. Technol. 29, 1232–1238. doi: 10.1021/es00005a014
Liu, Z., He, X., Chen, W., Yuan, F., Yan, K., and Tao, D. (2009). Accumulation and tolerance characteristics of cadmium in a potential hyperaccumulator-Lonicera japonica Thunb. J. Hazard. Mater. 169, 170–175. doi: 10.1016/j.jhazmat.2009.03.090
Lu, R. (1999). Analytical Methods for Soils and Agricultural Chemistry. Beijing: China Agricultural Science and Technology Press.
Lucy, M., Reed, E., and Glick, B. R. (2004). Applications of free living plant growth-promoting rhizobacteria. Antonie Van Leeuwenhoek 86, 1–25. doi: 10.1023/B:ANTO.0000024903.10757.6e
Ma, Y., Prasad, M., Rajkumar, M., and Freitas, H. (2011). Plant growth promoting rhizobacteria and endophytes accelerate phytoremediation of metalliferous soils. Biotechnol. Adv. 29, 248–258. doi: 10.1016/j.biotechadv.2010.12.001
Ma, Y., Rajkumar, M., and Freitas, H. (2009a). Improvement of plant growth and nickel uptake by nickel resistant-plant-growth promoting bacteria. J. Hazard. Mater. 166, 1154–1161. doi: 10.1016/j.jhazmat.2008.12.018
Ma, Y., Rajkumar, M., and Freitas, H. (2009b). Isolation and characterization of Ni mobilizing PGPB from serpentine soils and their potential in promoting plant growth and Ni accumulation by Brassica spp. Chemosphere 75, 719–725. doi: 10.1016/j.chemosphere.2009.01.056
Nies, D. H. (2003). Efflux-mediated heavy metal resistance in prokaryotes. FEMS Microbiol. Rev. 27, 313–339. doi: 10.1016/S0168-6445(03)00048-2
Ouzounidou, G. (1995). Cu-ions mediated changes in growth, chlorophyll and other ion contents in a Cu-tolerant Koeleria splendens. Biol. Plant. 37, 71–78. doi: 10.1007/BF02913000
Patten, C. L., and Glick, B. R. (1996). Bacterial biosynthesis of indole-3-acetic acid. Can. J. Microbiol. 42, 207–220. doi: 10.1139/m96-032
Patten, C. L., and Glick, B. R. (2002). Role of Pseudomonas putida indole acetic acid in development of the host plant root system. Appl. Environ. Microbiol. 68, 3795–3801. doi: 10.1128/AEM.68.8.3795-3801.2002
Pikovskaya, R. (1948). Mobilization of phosphorus in soil in connection with vital activity of some microbial species. Mikrobiologiya 17, 362–370.
Pilon-Smits, E. (2005). Phytoremediation. Ann. Rev. Plant Biol. 56, 15–39. doi: 10.1146/annurev.arplant.56.032604.144214
Płociniczak, T., Sinkkonen, A., Romantschuk, M., Sułowicz, S., and Piotrowska-Seget, Z. (2016). Rhizospheric bacterial strain Brevibacterium casei MH8a colonizes plant tissues and enhances Cd, Zn, Cu phytoextraction by white mustard. Front. Plant Sci. 7:101. doi: 10.3389/fpls.2016.00101
Rajkumar, M., Ae, N., and Freitas, H. (2009). Endophytic bacteria and their potential to enhance heavy metal phytoextraction. Chemosphere 77, 153–160. doi: 10.1016/j.chemosphere.2009.06.047
Rajkumar, M., and Freitas, H. (2008a). Effects of inoculation of plant-growth promoting bacteria on Ni uptake by Indian mustard. Bioresour. Technol. 99, 3491–3498. doi: 10.1016/j.biortech.2007.07.046
Rajkumar, M., and Freitas, H. (2008b). Influence of metal resistant-plant growth-promoting bacteria on the growth of Ricinus communis in soil contaminated with heavy metals. Chemosphere 71, 834–842. doi: 10.1016/j.chemosphere.2007.11.038
Rajkumar, M., Sandhya, S., Prasad, M., and Freitas, H. (2012). Perspectives of plant-associated microbes in heavy metal phytoremediation. Biotechnol. Adv. 30, 1562–1574. doi: 10.1016/j.biotechadv.2012.04.011
Raskin, I., Kumar, P. N., Dushenkov, S., and Salt, D. E. (1994). Bioconcentration of heavy metals by plants. Curr. Opin. Biotechnol. 5, 285–290. doi: 10.1016/0958-1669(94)90030-2
Rojas-Tapias, D. F., Bonilla, R. R., and Dussán, J. (2012). Effect of inoculation with plant growth-promoting bacteria on growth and copper uptake by sunflowers. Water Air Soil Pollut. 223, 643–654. doi: 10.1016/j.jhazmat.2011.02.075
Schwyn, B., and Neilands, J. (1987). Universal chemical assay for the detection and determination of siderophores. Anal. Biochem. 160, 47–56. doi: 10.1016/0003-2697(87)90612-9
Sessitsch, A., Kuffner, M., Kidd, P., Vangronsveld, J., Wenzel, W. W., Fallmann, K., et al. (2013). The role of plant-associated bacteria in the mobilization and phytoextraction of trace elements in contaminated soils. Soil Biol. Biochem. 60, 182–194. doi: 10.1016/j.soilbio.2013.01.012
Shallari, S., Echevarria, G., Schwartz, C., and Morel, J. (2001). Availability of nickel in soils for the hyperaccumulator Alyssum murale (Waldst & Kit). South Afr. J. Sci. 97(11/12; PART 2), 568–570.
Sheng, X. F., and Xia, J. J. (2006). Improvement of rape (Brassica napus) plant growth and cadmium uptake by cadmium-resistant bacteria. Chemosphere 64, 1036–1042. doi: 10.1016/j.chemosphere.2006.01.051
Sheng, X. F., Xia, J. J., Jiang, C. Y., He, L. Y., and Qian, M. (2008). Characterization of heavy metal-resistant endophytic bacteria from rape (Brassica napus) roots and their potential in promoting the growth and lead accumulation of rape. Environ. Pollut. 156, 1164–1170. doi: 10.1016/j.envpol.2008.04.007
Shoebitz, M., Ribaudo, C. M., Pardo, M. A., Cantore, M. L., Ciampi, L., and Curá, J. A. (2009). Plant growth promoting properties of a strain of Enterobacter ludwigii isolated from Lolium perenne rhizosphere. Soil Biol. Biochem. 41, 1768–1774. doi: 10.1016/j.soilbio.2007.12.031
Tamura, K., Dudley, J., Nei, M., and Kumar, S. (2007). MEGA4: molecular evolutionary genetics analysis (MEGA) software version 4.0. Mol. Biol. Evol. 24, 1596–1599. doi: 10.1093/molbev/msm092
Tripathi, M., Munot, H. P., Shouche, Y., Meyer, J. M., and Goel, R. (2005). Isolation and functional characterization of siderophore-producing lead-and cadmium-resistant Pseudomonas putida KNP9. Curr. Microbiol. 50, 233–237. doi: 10.1007/s00284-004-4459-4
Watanabe, F., and Olsen, S. (1965). Test of an ascorbic acid method for determining phosphorus in water and NaHCO3 extracts from soil. Soil Sci. Soc. Am. J. 29, 677–678. doi: 10.2136/sssaj1965.03615995002900060025x
Wei, G., Chen, W., Zhu, W., Chen, C., Young, J. P. W., and Bontemps, C. (2009). Invasive Robinia pseudoacacia in China is nodulated by Mesorhizobium and Sinorhizobium species that share similar nodulation genes with native American symbionts. FEMS Microbiol. Ecol. 68, 320–328. doi: 10.1111/j.1574-6941.2009.00673.x
Wenzel, W., Bunkowski, M., Puschenreiter, M., and Horak, O. (2003). Rhizosphere characteristics of indigenously growing nickel hyperaccumulator and excluder plants on serpentine soil. Environ. Pollut. 123, 131–138. doi: 10.1016/S0269-7491(02)00341-X
Xie, H., Pasternak, J., and Glick, B. R. (1996). Isolation and characterization of mutants of the plant growth-promoting rhizobacterium Pseudomonas putida GR12-2 that overproduce indoleacetic acid. Curr. Microbiol. 32, 67–71. doi: 10.1007/s002849900012
Xiong, Z. T., Hu, H. X., Wang, Y. X., Fu, G. H., Tan, Z. Q., and Yan, G. A. (1997). Comparative analyses of soil contaminant levels and plant species diversity at developing and disused oil well sites in Qianjiang oilfield, China. Bull. Environ. Contam. Toxicol. 58, 667–672. doi: 10.1007/s001289900385
Yoon, J., Cao, X., Zhou, Q., and Ma, L. Q. (2006). Accumulation of Pb, Cu, and Zn in native plants growing on a contaminated Florida site. Sci. Total Environ. 368, 456–464. doi: 10.1016/j.scitotenv.2006.01.016
Zahir, Z. A., Ghani, U., Naveed, M., Nadeem, S. M., and Asghar, H. N. (2009). Comparative effectiveness of Pseudomonas and Serratia sp. containing ACC-deaminase for improving growth and yield of wheat (Triticum aestivum L.) under salt-stressed conditions. Arch. Microbiol. 191, 415–424. doi: 10.1007/s00203-009-0466-y
Keywords: Sonchus oleraceus, plant-growth-promoting rhizobacteria, Cu/Zn-resistant, bioremediation, Acinetobacter
Citation: Fang Q, Fan Z, Xie Y, Wang X, Li K and Liu Y (2016) Screening and Evaluation of the Bioremediation Potential of Cu/Zn-Resistant, Autochthonous Acinetobacter sp. FQ-44 from Sonchus oleraceus L. Front. Plant Sci. 7:1487. doi: 10.3389/fpls.2016.01487
Received: 20 April 2016; Accepted: 20 September 2016;
Published: 30 September 2016.
Edited by:
Ying Ma, University of Coimbra, PortugalReviewed by:
Tomasz Płociniczak, University of Silesia in Katowice, PolandGbotemi Adediran, Umeå University, Sweden
Copyright © 2016 Fang, Fan, Xie, Wang, Li and Liu. This is an open-access article distributed under the terms of the Creative Commons Attribution License (CC BY). The use, distribution or reproduction in other forums is permitted, provided the original author(s) or licensor are credited and that the original publication in this journal is cited, in accordance with accepted academic practice. No use, distribution or reproduction is permitted which does not comply with these terms.
*Correspondence: Xiangrong Wang, xrxrwang@fudan.edu.cn