- 1Key Laboratory of Southern Vegetable Crop Genetic Improvement, Ministry of Agriculture, College of Horticulture, Nanjing Agricultural University, Nanjing, China
- 2Suqian Academy of Protected Horticulture, Nanjing Agricultural University, Nanjing, China
With the aim to clarifying the role of the ABA/H2O2 signaling cascade in the regulating the antioxidant capacity of grafted cucumber plants in response to Ca(NO3)2 stress, we investigated the relationship between ABA-mediated H2O2 production and the activities of antioxidant enzymes in the leaves of pumpkin-grafted cucumber seedlings. The results showed that both ABA and H2O2 were detected in pumpkin-grafted cucumber seedlings in response to Ca(NO3)2 treatment within 0.5 h in the leaves and peaked at 3 and 6 h after Ca(NO3)2 treatment, respectively, compared to the levels under control conditions. The activities of superoxide dismutase (SOD), ascorbate peroxidase (APX), and peroxidase (POD) in pumpkin-grafted cucumber leaves gradually increased over time and peaked at 12 h of Ca(NO3)2 stress. Furthermore, in the leaves of pumpkin-grafted cucumber seedlings, the H2O2 generation, the antioxidant enzyme activities and the expression of SOD, POD and cAPX were strongly blocked by an inhibitor of ABA under Ca(NO3)2 stress, but this effect was eliminated by the addition of exogenous ABA. Moreover, the activities and gene expressions of these antioxidant enzymes in pumpkin-grafted leaves were almost inhibited under Ca(NO3)2 stress by pretreatment with ROS scavengers. These results suggest that the pumpkin grafting-induced ABA accumulation mediated H2O2 generation, resulting in the induction of antioxidant defense systems in leaves exposed to Ca(NO3)2 stress in the ABA/H2O2 signaling pathway.
Introduction
Greenhouse cultivation is the most common method of vegetable production worldwide. However, secondary soil salinization in greenhouse soil, which is primarily caused by over-irrigation, intensive farming, lack of rain, and excessive application of nitrogenous fertilizers, restricts the development and productivity of vegetables in China (Blanco and Folegatti, 2002; Liang et al., 2005; Yu et al., 2005; He et al., 2007; Daliakopoulos et al., 2016). Several reports have indicated that the characteristics of greenhouse soil are different from those of coastal and inland saline soil. Its main cation is Ca2+, and its main anion is , which account for 60 and 67–76% of the total cations and anions, respectively (Yuan et al., 2012; Xing et al., 2015). Excessive Ca(NO3)2 not only leads to osmotic stress, inhibits biological nitrogen fixation, and modifies microbial soil biodiversity but also causes the formation of reactive oxygen species (ROS) in plants. ROS can disorder the normal physiological metabolism and then inhibit plant growth and decrease crop yield (Sainju et al., 2001). However, plants have evolved adaptive protection mechanisms, such as antioxidant enzymes to protect themselves against the deleterious effects of ROS.
Cucumber (Cucumis sativus L.) is one of the most economically important vegetables worldwide, grown both in open fields and protected facilities. According to FAO statistics, the world cultivation area of cucumber was 2,115,457 hm2 and the yield was 71,333,414 ton in 2013. In China, these two values were 1,166,690 hm2 and 54,362,750 tons, respectively, making China the country with the largest production of cucumber (FAO, 2013). However, the yield of cucumber in China was only 46.6 tons⋅hm-2, which was far lower than the highest yield (Netherlands, 666.7 ton⋅hm-2), ranking China number 31 in the world. Because of their biological characteristics, cucumber plants are affected by many adverse environmental factors, of which the secondary salinization of greenhouse soil is a major problem, significantly decreasing the yield and quality of cucumber fruits. A common method of adapting plants to environmental stresses is by grafting commercial cultivars onto selected tolerant rootstocks (Lee and Oda, 2002). In horticultural crop production, grafting has already been used for more than 50 years in many regions of the world (Rivard and Louws, 2008). Vegetable seedlings grown under Ca(NO3)2 stress may possess higher contents of osmotic adjustment substances and higher activities of antioxidant enzymes if they are grafted with selected vigorous rootstocks and suffer less oxidative damage, contributing to a higher production and better quality of fruits (Zhang et al., 2008; Xing et al., 2015).
Abscisic acid has a wide range of physiological functions in higher plants, including regulating plant responses to various adverse environmental factors (Sah et al., 2016). ABA is considered a root-derived signaling molecule. It moves within plants, and its transport plays an important role in determining the endogenous hormone concentrations at the site of action (Seo and Koshiba, 2011). A number of studies suggest that ABA might be the chemical substance responsible for root-to-shoot signaling, especially under abiotic stress conditions (Sah et al., 2016). An increasing body of evidence indicates that one mode of ABA action is related to oxidative stress in plant cells. It is well known that ABA can increase the generation of H2O2 (Kwak et al., 2003; Laloi et al., 2004); cause the gene expression of superoxide dismutase (SOD), CAT, and APX, increase the activities of these antioxidant enzymes in plant tissues; and enhance the stress resistance of plants (Guan et al., 2000; Jiang and Zhang, 2003; Park et al., 2004; Saxena et al., 2016).
However, little information exists about whether the increased enzyme activities of antioxidants induced by ABA in an H2O2-dependent way would occur in pumpkin-grafted cucumber seedlings and lead to better plant performance than that in self-grafted cucumber seedlings under Ca(NO3)2 stress. In this study, the ABA and H2O2 contents and antioxidant enzyme activities in the leaves of pumpkin-grafted and self-grafted cucumber seedlings under Ca(NO3)2 stress were examined. In addition, this study investigated whether the increased activities of antioxidant enzymes and their encoded gene (SOD, POD, and cAPX) expression in pumpkin-grafted cucumber leaves under Ca(NO3)2 stress are induced by ABA and whether H2O2 is involved in this induction. The mechanism by which the pumpkin rootstock enhances antioxidant defense for the Ca(NO3)2 stress tolerance of cucumber seedlings is also discussed.
Materials and Methods
Plant Material and Treatments
Cucumber cultivar (Cucumis sativus L. ‘Jinyou No. 3’, obtained from Tianjin Kerun Research Institution) was used as the scion. A salt-tolerant pumpkin ‘Qingzhen 1’ (Cucurbita maxima × Cucurbita moschata, obtained from Qingdao Agriculture Academy of Science) was selected as the rootstock (Xing et al., 2015). Cucumber and pumpkin seeds were surface sterilized with 1% (v/v) sodium hypochlorite, washed thoroughly with distilled water, and then sown in plastic salvers (41 cm × 41 cm × 5 cm) containing quartz sand and incubated in a greenhouse at Nanjing Agriculture University, China. The average day/night temperatures in the greenhouse were at 25–30°C/15–18°C, and the relative humidity was 60–75%. When the scion’s cotyledons were fully expanded and the rootstock’s second true leaves were in the development stage, the insert grafting procedure was performed as described by Lee and Oda (2002). Self-grafted plants were included as the controls. Uniformly sized pumpkin-grafted seedlings were grown hydroponically in plastic containers filled with half-strength Hoagland’s solution (pH 6.5 ± 0.1, EC 2.0–2.2 dS⋅m-1) for the next experiments. The solution was replaced every 3 days and continuously aerated with an air pump at an interval of 20 min to keep the dissolved oxygen level at 8.0 ± 0.2 mg⋅L-1.
After the full development of the third true leaves, the seedlings were treated as follows: 80 mM Ca(NO3)2 was added to the solution for the salt stress treatment. Different exogenous substances such as 10 μM ABA, 1 mM sodium tungstate (T, an ABA synthesis inhibitor), 10 mM 1,2-dihydroxybenzene- 3,5-disulfonic acid (Tiron, a specific scavenger), 100 μM DPI (a specific NADPH oxidase inhibitor) and 5 mM DMTU (a specific H2O2 scavenger) were used in various treatments for 8 h before salt stress treatment. All of the treatments were as follows:
(a) S-G, self-grafted cucumber seedlings grown in Hoagland’s solution;
(b)S-GN, self-grafted cucumber seedlings grown in Hoagland’s solution with 80 mM Ca(NO3)2;
(c)P-G, pumpkin-grafted cucumber seedlings grown in Hoagland’s solution;
(d)P-GN, pumpkin-grafted cucumber seedlings grown in Hoagland’s solution with 80 mM Ca(NO3)2;
(e)P-GN + T, pumpkin-grafted cucumber seedlings pretreated with 1 mM sodium tungstate grown in Hoagland’s solution with 80 mM Ca(NO3)2;
(f)P-GN + T + ABA, pumpkin-grafted cucumber seedlings pretreated with 1 mM sodium tungstate and 10 μM ABA grown in Hoagland’s solution with 80 mM Ca(NO3)2;
(g)P-GN + DPI, pumpkin-grafted cucumber seedlings pretreated with 100 μM DPI grown in Hoagland’s solution with 80 mM Ca(NO3)2;
(h)P-GN + Tiron, pumpkin-grafted cucumber seedlings pretreated with 10 mM Tiron grown in Hoagland’s solution with 80 mM Ca(NO3)2;
(i)P-GN + DMTU, pumpkin-grafted cucumber seedlings pretreated with 5 mM DMTU grown in Hoagland’s solution with 80 mM Ca(NO3)2.
The experiments were arranged in a randomized complete block design with three replicates. Each treatment included three containers with 36 seedlings. The third fully expanded leaves (from the top) were sampled at key time points as indicated below and immediately frozen in liquid nitrogen.
ABA Assay
Fresh leaves were homogenized in an extraction solution containing 80% methanol, 0.05% citric acid and 0.45 mM butylated hydroxytoluene and were then centrifuged at 8,000 × g for 10 min. The samples were dried, and the radioactivity in the pellet was quantified. ABA was assayed using radioimmunoassay as described by Verslues and Bray (2006).
Measurement of the H2O2 Level
The H2O2 content was measured according to the method described by Brennan and Frenkel (1977). The absorbance values via OD at 415 nm were calibrated to a standard graph generated with known concentrations of H2O2.
Measurement of the Antioxidant Enzyme Activity
For extract of antioxidant enzymes, fresh leaves were homogenized with 1.6 mL of 50 mM phosphate buffer (pH 7.8) containing 1 mM EDTA and 2% PVP. The homogenate was centrifuged at 12,000 × g for 20 min at 4°C, and the resulting supernatant was used to assay enzyme activity as follows: the SOD activity was assayed by monitoring the inhibition of the photochemical reduction of NBT following the method of Giannopolitis and Ries (1997). One unit of SOD activity was defined as the amount of enzyme that was required to cause a 50% inhibition of the reduction of NBT as monitored at 560 nm.
The POD activity was measured according to the method of Rao et al. (1996) with slight modification. For this, 5 mL of the extracted enzyme was mixed with 3 mL of the reaction mixture containing 50 mM PBS (pH 7.0) and 20 mM guaiacol. After pre-incubation at 25°C for 5 min, 6 mM H2O2 was added to initiate the reaction. Changes in the absorbance at 470 nm within 2 min were recorded to calculate POD activity. One unit of POD activity was expressed as U.g-1 FW.
A modified method from Aebi (1974) was used to assay the CAT activity. For this, 100 mL of the extraction was added to 3 mL 50 mM PBS buffer (pH 7.0). After incubation, the reaction was started by the addition of 6 mM H2O2. The CAT activity was expressed as U.g-1 FW.
The APX activity was performed as described by Pinhero et al. (1997). The assay was carried out in a reaction mixture consisting of 50 mM PBS (pH7.0), 0.5 mM AsA, 3 mM H2O2 and 100 mL of the extraction. One unit of APX activity was defined as an absorbance change of 0.1 unit min-1, and the APX activity was expressed as U.g-1 FW.
RNA Isolation and Quantitative Real-Time (qRT-PCR) Analysis
Total RNA was extracted from leaves as described in the TRI reagent protocol (Takara Bio Inc). Primers were designed according to cucumber databases1. and NCBI. Gene specificprimers used for real-time quantitative PCR are provided in the following primers: SOD: forward CCTAAACTCTCGTGAATGA and reverse CAGCAGACAAGTATGGATA; POD: forward TTGTAATAATGGCGGCTT and reverse GTGTCATAGAAGGTGGAG; cAPX: forward TGCTTTCATCACCATCAA and reverse TGTTATGTTCTTGTCTTCCT. Actin: forward CCACCAATCTTGTACACATCC and reverse AGACCACCAAGTACTACTGCAC. qRT-PCR was performed on a StepOnePlusTM Real-Time PCR System (Applied Biosystems) using a SYBR® Premix Ex TaqTM II (Tli RNaseH Plus) kit (Takara). The PCR reactions were carried out in triplicate and the thermocycler conditions, 95°C for 30 s, followed by 40 cycles of 95°C for 5 s, 60°C for 30 s, and a final extension of 30 s at 60°C. Relative expression was calculated according to the 2-ΔΔCT method, the relative gene expression level was normalized against actin (the internal standard gene).
Statistical Analysis
All data were statistically analyzed with SAS 13.0 software (SAS Institute, Inc., Cary, NC, USA) using Duncan’s multiple range test at the P < 0.05 level of significance.
Results
Effects of Ca(NO3)2 Stress on the Contents of ABA and H2O2 in the Leaves of Pumpkin-Grafted and Self-Grafted Cucumber Seedlings
Compared to their corresponding controls, the ABA content in the leaves of pumpkin-grafted and self-grafted seedlings significantly increased under 80 mM Ca(NO3)2 stress (Figure 1), whereas the ABA level in the rootstock-grafted leaves was higher than that of the self-grafted leaves under Ca(NO3)2 stress. The ABA content in the leaves of pumpkin-grafted cucumber seedlings increased after 0.5 h of Ca(NO3)2 stress and peaked at 3 h. However, the ABA content in the leaves of the self-grafted cucumber seedlings did not significant changes after the entire duration of Ca(NO3)2 stress.
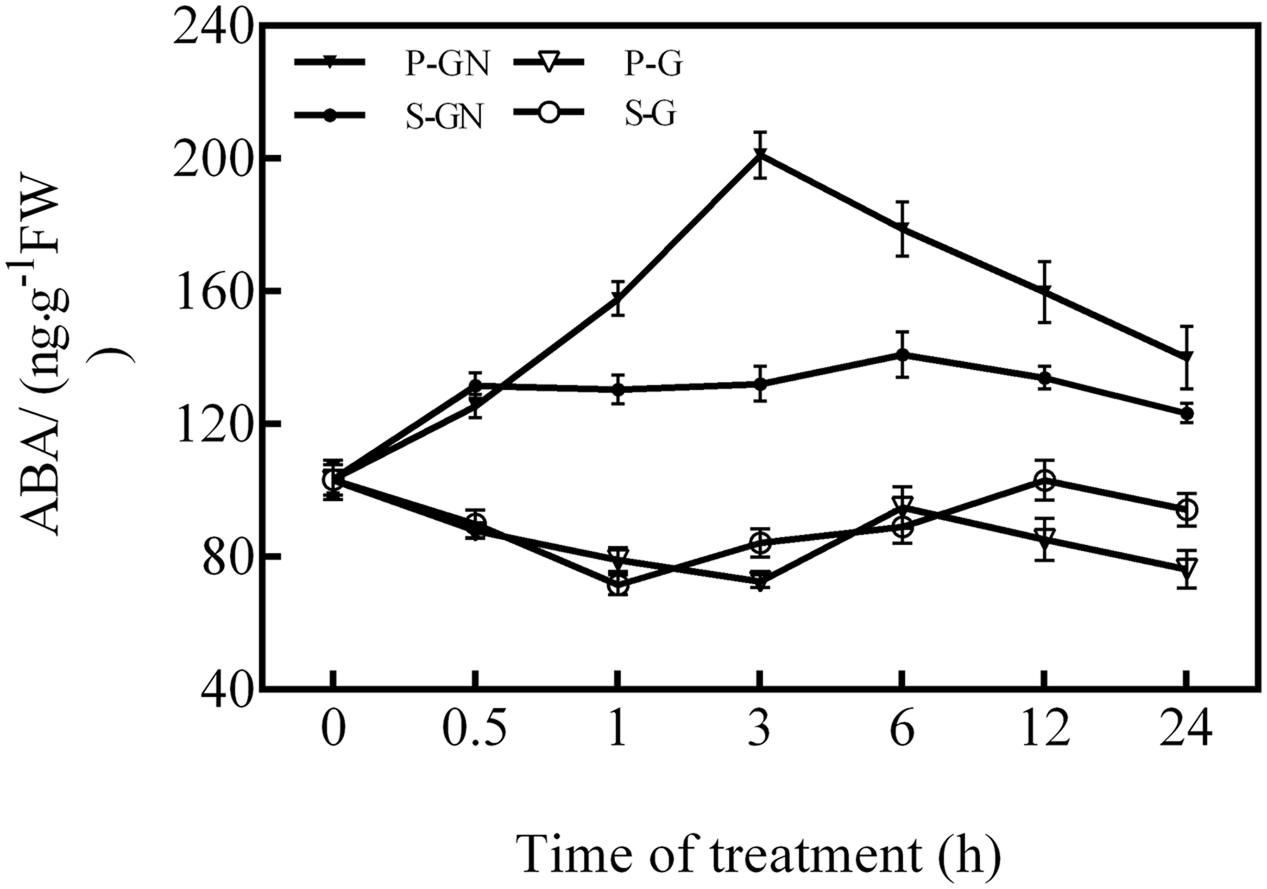
FIGURE 1. Time course of changes in the ABA content in the leaves of self-grafted and pumpkin-grafted cucumber seedlings exposed to 80 mM Ca(NO3)2. Each value is the mean ± SE of six independent experiments (n = 6). P-G, pumpkin-grafted cucumber seedlings grown in Hoagland’s solution; P-GN, pumpkin-grafted cucumber seedlings with 80 mM Ca(NO3)2; S-G, self-grafted cucumber seedlings grown in Hoagland’s solution; S-GN, self-grafted cucumber seedlings with 80 mM Ca(NO3)2.
The H2O2 content in the leaves of pumpkin-grafted and self-grafted cucumber seedlings increased by Ca(NO3)2 stress compared to the corresponding controls (Figure 2). The H2O2 content increased after 0.5 h of Ca(NO3)2 stress and peaked at 6 h, after which it rapidly decreased in the leaves of pumpkin-grafted and self-grafted cucumber seedlings. However, the pumpkin-grafted seedlings had a higher H2O2 level than that of the self-grafted seedlings leaves during the treatment of Ca(NO3)2 stress. Under non-saline conditions, there were no significant differences in the H2O2 level between self-grafted seedlings and pumpkin-grafted cucumber leaves.
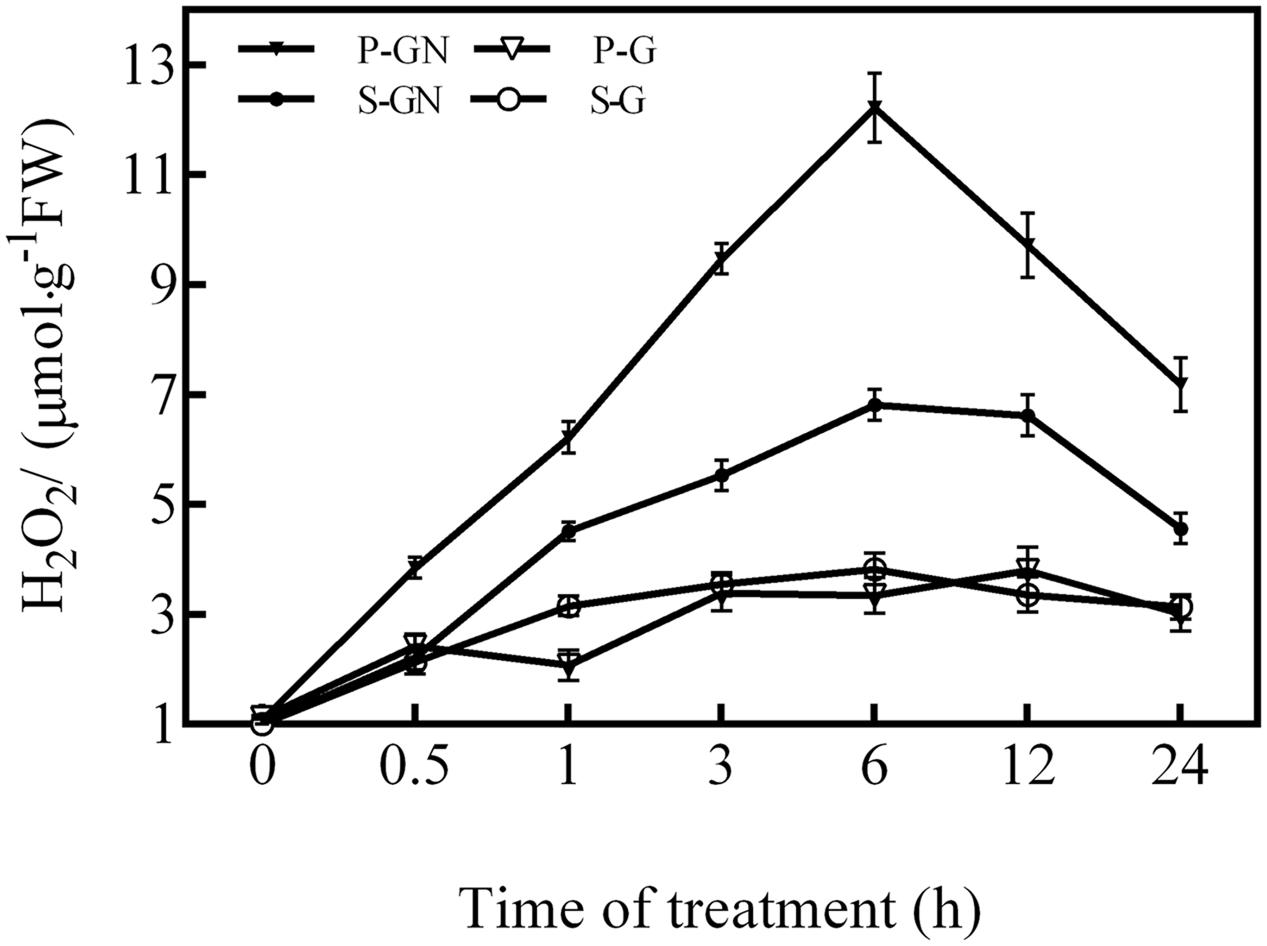
FIGURE 2. Time course of changes in the H2O2 content in the leaves of self-grafted and pumpkin-grafted cucumber seedlings exposed to 80 mM Ca(NO3)2. Each value is the mean ± SE of six independent experiments (n = 6). P-G, pumpkin-grafted cucumber seedlings grown in Hoagland’s solution; P-GN, pumpkin-grafted cucumber seedlings with 80 mM Ca(NO3)2; S-G, self-grafted cucumber seedlings grown in Hoagland’s solution; S-GN, self-grafted cucumber seedlings with 80 mM Ca(NO3)2.
Effects of Ca(NO3)2 Stress on the Activities of Antioxidant Enzymes in the Leaves of Pumpkin-Grafted and Self-Grafted Cucumber Seedlings
Treatment with Ca(NO3)2 stress led to similar changes of in the antioxidant enzyme activities in the leaves of pumpkin-grafted and self-grafted cucumber seedlings, but the changes in the activities of antioxidant enzymes in the rootstock-grafted leaves were more significant and the range was much wider than those of the self-grafted seedlings (Figure 3). Compared to their corresponding controls, the activities of SOD, POD and CAT increased in the leaves of pumpkin-grafted and self-grafted cucumber seedlings under Ca(NO3)2 stress. Moreover, these values of the pumpkin-grafted seedlings were increasingly higher than those of the self-grafted seedlings under Ca(NO3)2 stress. The activities of SOD, POD and APX in the pumpkin-grafted seedlings leaves increased after 6 h of Ca(NO3)2 stress and peaked at 12 h, after which it gradually decreased. The activity of CAT in the leaves increased and peaked at 12 h, then decreased at 24 h, and then increased until 48 h in pumpkin-grafted and self-grafted cucumber seedlings under Ca(NO3)2 stress (Gao et al., 2015). Under non-saline conditions, there was no significant difference in the activities of antioxidant enzymes between pumpkin-grafted and self-grafted seedlings leaves, except for the APX activity at 12 and 36 h.
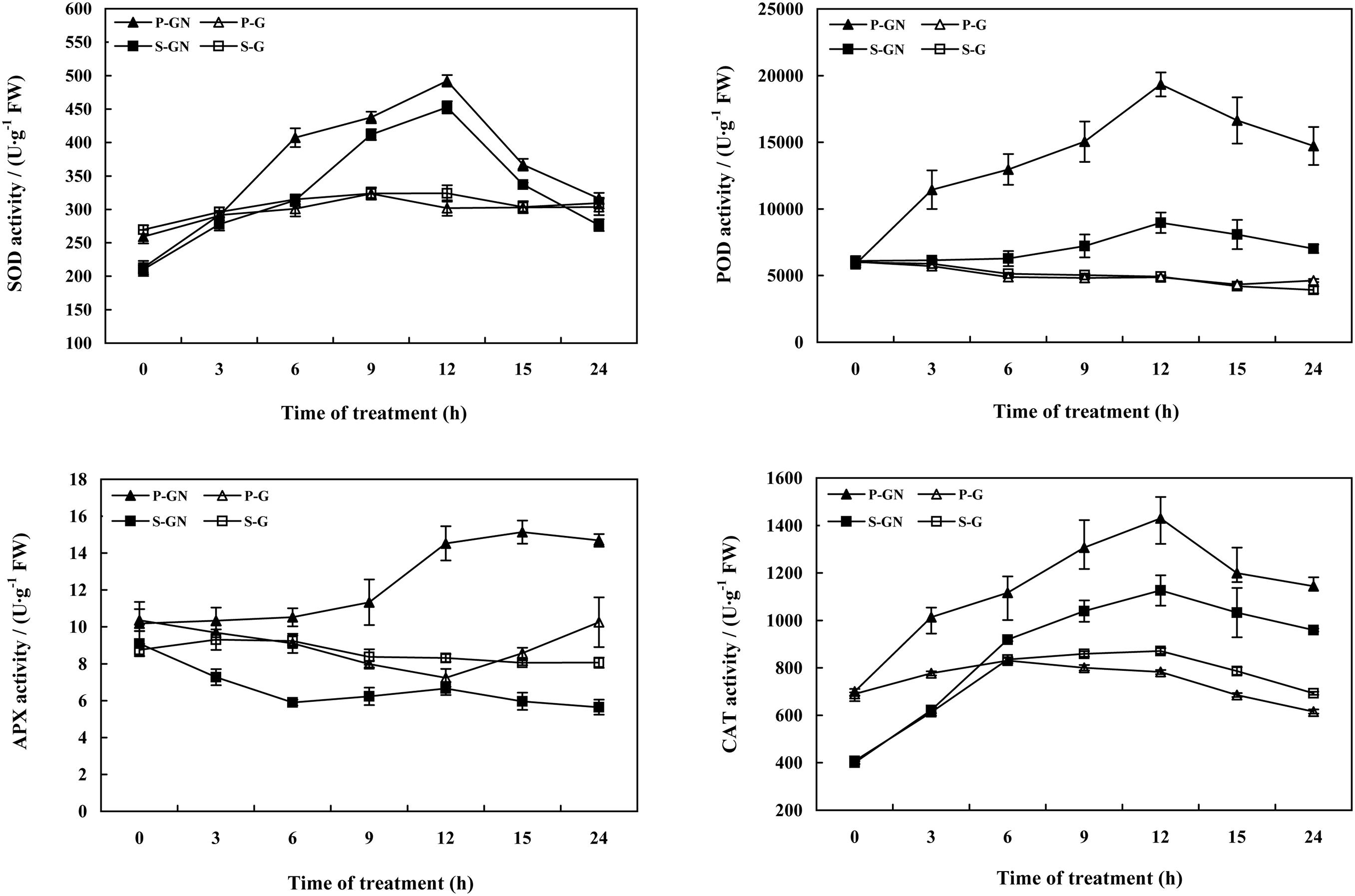
FIGURE 3. Time course of changes in the activities of SOD, POD, CAT, and APX in the leaves of self-grafted and pumpkin-grafted cucumber seedlings exposed to 80 mM Ca(NO3)2. Each value is the mean ± SE of six independent experiments (n = 6). P-G, pumpkin-grafted cucumber seedlings grown in Hoagland’s solution; P-GN, pumpkin-grafted cucumber seedlings with 80 mM Ca(NO3)2; S-G, self-grafted cucumber seedlings grown in Hoagland’s solution; S-GN, self-grafted cucumber seedlings with 80 mM Ca(NO3)2.
ABA-Mediated Accumulation of H2O2 in the Leaves of Pumpkin-Grafted Seedlings under Ca(NO3)2 Stress
Sodium tungstate (T), an ABA synthesis inhibitor, and exogenous ABA were used to determine whether ABA is involved in inducing H2O2 accumulation in pumpkin-grafted cucumber seedlings under Ca(NO3)2 stress. Ca(NO3)2 stress significantly increased the H2O2 content of the pumpkin-grafted seedlings leaves compared to that of the control, but pretreatment with 5 mM sodium tungstate remarkably inhibited the increased H2O2 induced by Ca(NO3)2 stress. However, the application of exogenous 100 μM ABA to Ca(NO3)2 stress alleviated the inhibition of the H2O2 content in the leaves of the pumpkin-grafted seedlings with sodium tungstate (Figure 4).
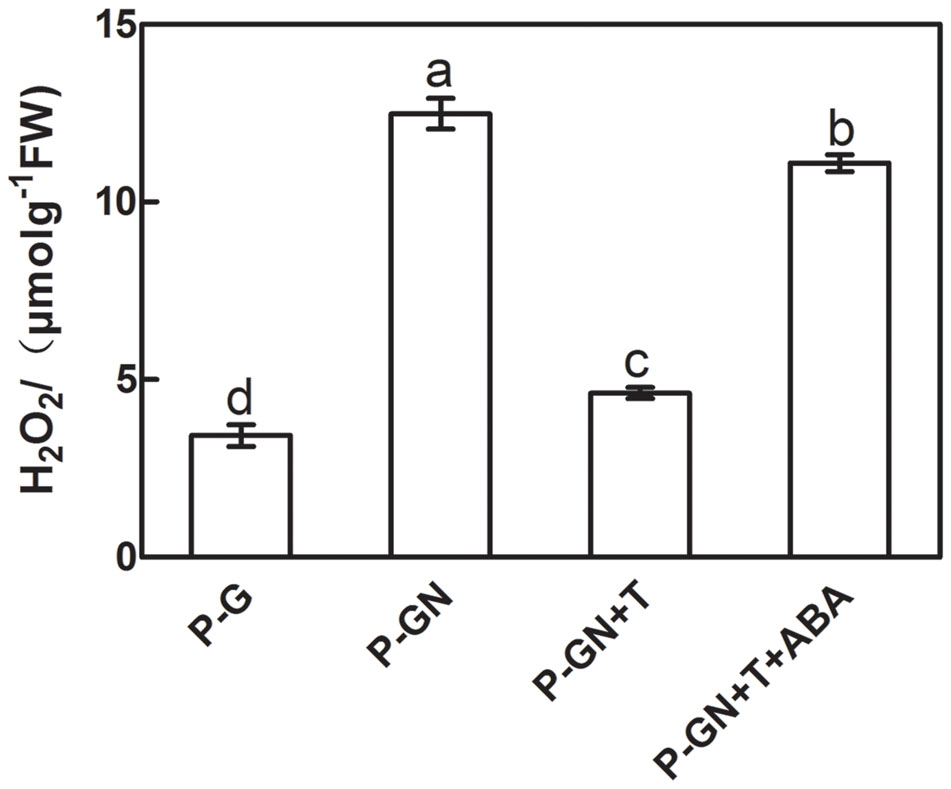
FIGURE 4. Effects of pretreatment with the ABA inhibitor sodium tungstate (T) and exogenous ABA on the content of H2O2 in the leaves of pumpkin-grafted cucumber seedlings exposed to 80 mM Ca(NO3)2 stress. The pumpkin-grafted cucumber seedlings were pretreated with 5 mM T (P-GN + T) and 100 μM exogenous ABA (P-GN + T + ABA) for 8 and 12 h, respectively, and then exposed to 80 mM Ca(NO3)2 for 12 h. Each histogram represents a mean value of three independent experiments, and the vertical bars indicate SE (n = 6). Different letters indicate significant differences at P < 0.05, according to Duncan’s multiple range tests. P-G, pumpkin-grafted cucumber seedlings grown in Hoagland’s solution; P-GN, pumpkin-grafted cucumber seedlings with 80 mM Ca(NO3)2.
ABA Involved in Enhancing Antioxidant Capacity in Pumpkin-Grafted Seedlings Leaves under Ca(NO3)2 Stress
Abscisic acid synthesis inhibitor T was used to determine the effects of ABA on the antioxidant defense of pumpkin-grafted cucumber seedlings under Ca(NO3)2 stress. According to the results in Figure 3, an irregular change in CAT activity was induced by Ca(NO3)2 stress; thus, we only studied the other three enzymes. Pretreatment with sodium tungstate significantly inhibited the increase in the SOD, POD and APX activities in the leaves of the pumpkin-grafted seedlings under Ca(NO3)2 stress (Figure 5). However, exogenous ABA in addition to Ca(NO3)2 stress alleviated the inhibition of the SOD, POD and APX activities of the pumpkin-grafted seedlings in the presence of sodium tungstate. We analyzed the expression profiles of three transcripts that encoded SOD, POD and APX using real-time quantitative RT-PCR to evaluate the correlation between these enzyme activities and their encoded gene expression after 12 h of Ca(NO3)2 stress (Figure 6). The SOD, POD, and cAPX expression patterns were similar to their enzyme activities under different treatment conditions.
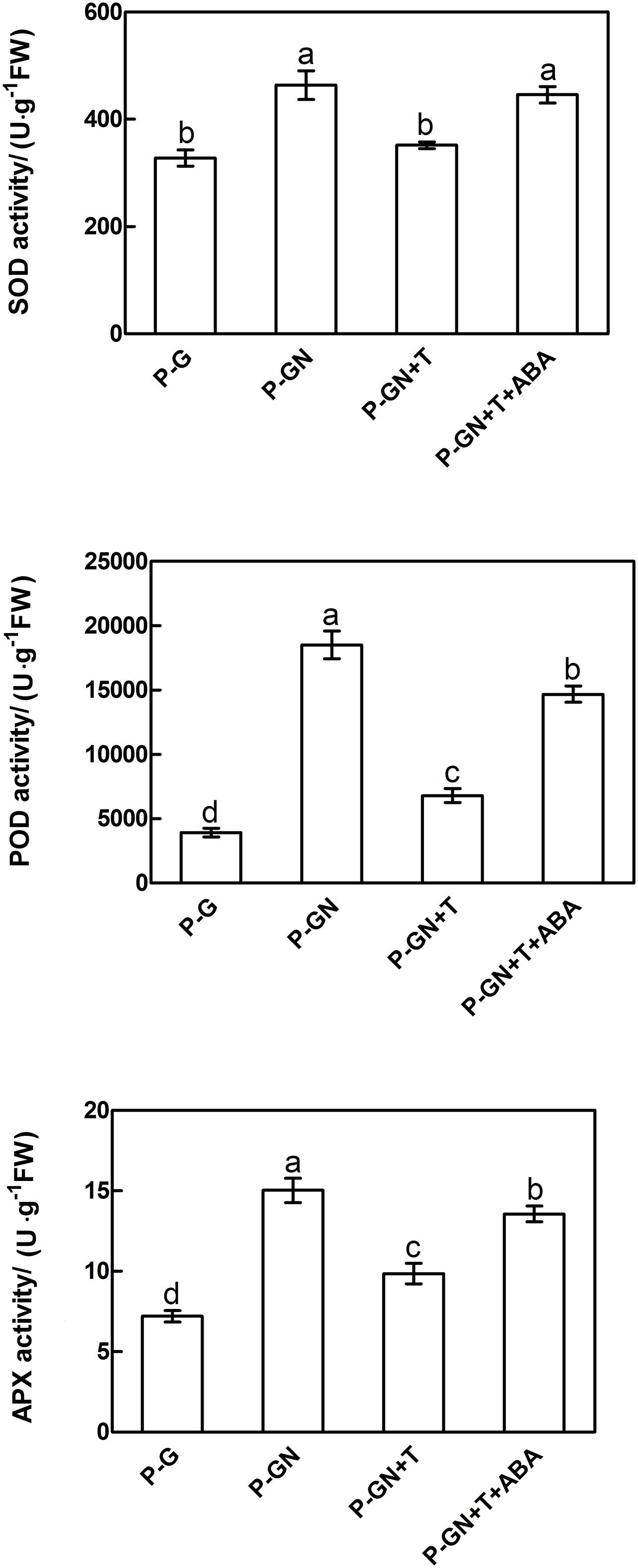
FIGURE 5. Effects of pretreatment with the ABA inhibitor sodium tungstate (T) and exogenous ABA on the activities of SOD, POD and APX in the leaves of pumpkin-grafted cucumber seedlings exposed to mM Ca(NO3)2. The pumpkin-grafted cucumber seedlings were pretreated with 5 mM T (P-GN + T) and 100 μM exogenous ABA (P-GN + T + ABA) for 8 and 12 h, respectively, and then exposed to 80 mM Ca(NO3)2 for 12 h. Each histogram represents the mean value of three independent experiments, and the vertical bars indicate SE (n = 6). Different letters indicate significant differences at P < 0.05, according to Duncan’s multiple range tests. P-G, pumpkin-grafted cucumber seedlings grown in Hoagland’s solution; P-GN, pumpkin-grafted cucumber seedlings with 80 mM Ca(NO3)2.
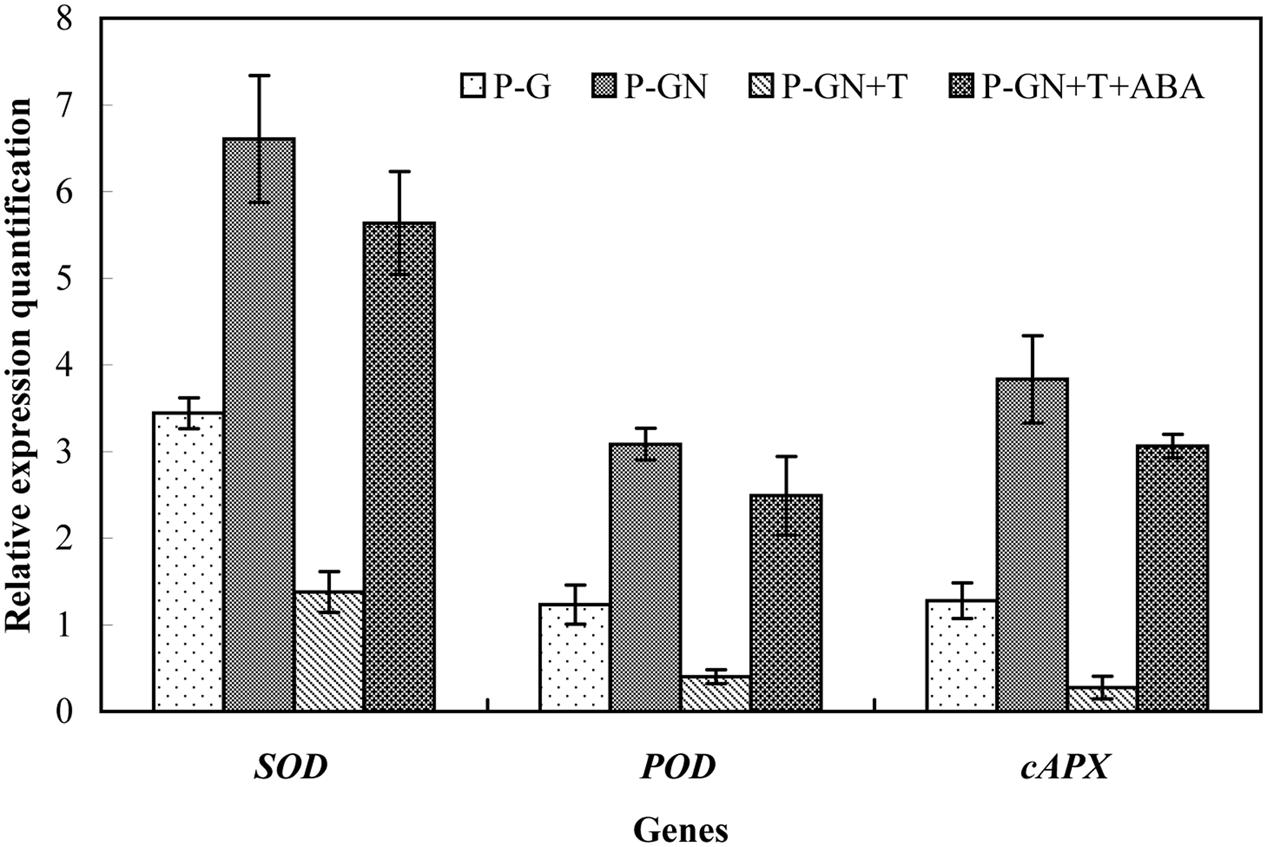
FIGURE 6. Effects of pretreatment with the ABA inhibitor sodium tungstate (T) and exogenous ABA on the expression of SOD, POD, and APX in the leaves of pumpkin-grafted cucumber seedlings exposed to 80 mM Ca(NO3)2. The pumpkin-grafted cucumber seedlings were pretreated with 5 mM T (P-GN + T) and 100 μM exogenous ABA (P-GN + T + ABA) for 8 and 12 h, respectively, and then exposed to 80 mM Ca(NO3)2 for 12 h. Each histogram represents the mean value of three independent experiments, and the vertical bars indicate SE (n = 3). P-G, pumpkin-grafted cucumber seedlings grown in Hoagland’s solution; P-GN, pumpkin-grafted cucumber seedlings with 80 mM Ca(NO3)2.
H2O2 Accumulation Induced Antioxidant Defense in Pumpkin-Grafted Seedlings Leaves under Ca(NO3)2 Stress
Reactive oxygen species are key signaling molecules for stress tolerance in plants. We thus examined the effects of pretreatment with ROS manipulators (DPI, Tiron and DMTU) on the gene expression and activities of antioxidant enzymes in pumpkin-grafted seedling leaves. Ca(NO3)2 stress induced a significant increase in the activities of SOD, POD and APX and their corresponding gene expression in pumpkin-grafted cucumber leaves, and these positive effects were completely prevented by pretreatment with DPI, Tiron and DMTU (Figures 7 and 8). These results indicate that ABA is involved in H2O2-accumulation-mediated antioxidant defense in pumpkin-grafted plants and in the subsequently improved Ca(NO3)2 tolerance of cucumber seedlings.
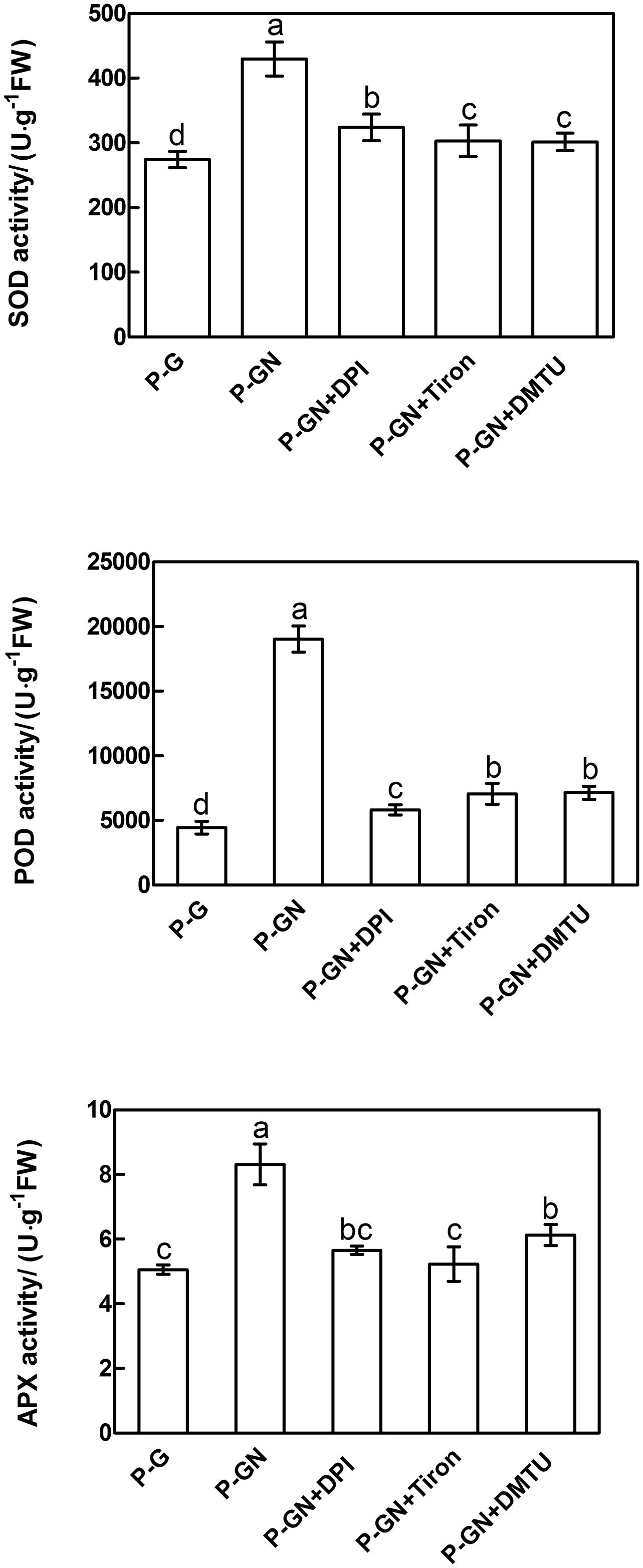
FIGURE 7. Effects of pretreatment with the NADPH oxidase inhibitor diphenyleneiodonium (DPI), the scavenger Tiron and the H2O2 scavenger DMTU on the activities of SOD, POD, and APX in the leaves of pumpkin-grafted cucumber seedlings exposed to 80 mM Ca(NO3)2. The pumpkin-grafted cucumber seedlings were pretreated with 100 μM DPI (P-GN + DPI), 10 mM Tiron (P-GN + Tiron), and 5 mM DMTU (P-GN + DMTU) for 8 h. Each histogram represents the mean value of three independent experiments, and the vertical bars indicate SE (n = 6). Different letters indicate significant differences at P < 0.05, according to Duncan’s multiple range tests. P-G, pumpkin-grafted cucumber seedlings grown in Hoagland’s solution; P-GN, pumpkin-grafted cucumber seedlings with 80 mM Ca(NO3)2.
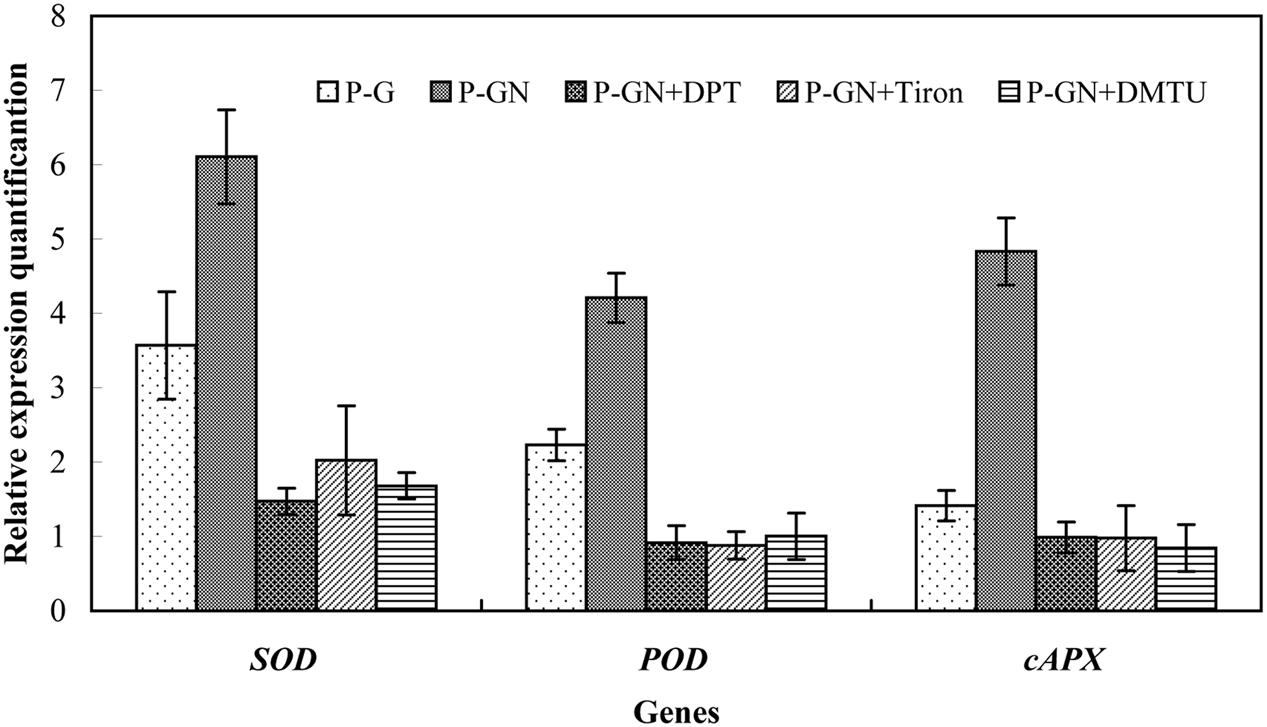
FIGURE 8. Effects of pretreatment with the NADPH oxidase inhibitor diphenyleneiodonium (DPI), the scavenger Tiron and the H2O2 scavenger DMTU on the expression of the SOD, POD, and cAPX in pumpkin-grafted cucumber seedlings leaves exposed to 80 mM Ca(NO3)2. The pumpkin-grafted cucumber seedlings were pretreated with 100 μM DPI (P-GN + DPI), 10 mM Tiron (P-GN + Tiron), and 5 mM DMTU (P-GN + DMTU) for 8 h. Each histogram represents the mean value of three independent experiments, and the vertical bars indicate SE (n = 3). P-G, pumpkin-grafted cucumber seedlings grown in Hoagland’s solution; P-GN, pumpkin-grafted cucumber seedlings with 80 mM Ca(NO3)2.
Discussion
It is well known that grafting with stress-tolerant rootstock can enhance plant tolerance to stress. In our previous studies, the Ca(NO3)2 stress tolerance of cucumber seedlings could be enhanced by grafting with pumpkin from different physiological aspects, including osmotic adjustment ability, nitrogen metabolism, soluble protein expression and antioxidant defense (Wang et al., 2012; Xing et al., 2015), but little information is available about the roles of the ABA and H2O2 signaling pathways in relieving Ca(NO3)2 stress. In this study, we present evidence that grafting-induced ABA accumulation in cucumber leaves triggered H2O2 production, thus enhancing activities of antioxidant enzymes, the expression of their encoding gene and the subsequent salt tolerance in response to Ca(NO3)2 stress.
Abscisic acid is the most important phytohormone that has multiple functions in the developmental processes of plants and enhances plants tolerance to various stresses including salinity, drought, and low temperature (Giraudat et al., 1994; Sah et al., 2016). In this study, the ABA content greatly increased in both self-grafted and rootstock-grafted cucumber leaves during the Ca(NO3)2 treatment (Figure 1). The increased ABA levels by grafting under stressed conditions probably resulted not only from the increased catabolism via the mevalonic acid-independent pathway but also from the translocation of other tissues or organs (Roychoudhury et al., 2013). Moreover, we also observed that the increased ABA content in the leaves of the rootstock-grafted plants was higher than that of the self-grafted plants under Ca(NO3)2 stress. These results may indicate that grafting cucumber seedlings with pumpkin rootstock exhibited a strong ability to resist Ca(NO3)2 stress. It has also been shown that increased endogenous ABA activates a complex signaling network leading to cellular responses to stresses (Ng et al., 2014). Several studies have shown that ABA accumulation induced by abiotic stresses could have a physiological effect on ROS production (Pei et al., 2000; Zhang et al., 2006).
As secondary messengers, ROS mediate variety of physiological functions and defense responses against abiotic stresses in plants. These functions include the regulation of seed germination (Roach et al., 2010), root development (Foreman et al., 2003), photosynthesis (Foyer et al., 2012), senescence (Gao et al., 2016), and adaptive responses to abiotic stresses (Jiang and Zhang, 2002a,b; Saxena et al., 2016). H2O2, a type of ROS, generates rapidly in plants in response to stress conditions (Saxena et al., 2016). However, it is not clear whether there exist similar responses in grafted plants, especially in plants exposed to Ca(NO3)2 stress. In this study, our results showed that Ca(NO3)2 stress increased the ABA and H2O2 contents and the antioxidant defense of pumpkin-grafted cucumber leaves. It is essential for plants to maintain the interaction between ABA and H2O2 to avoid any oxidative stress induced by adverse environmental factors (Saxena et al., 2016). As the time course of the production of ABA (Figure 1) and H2O2 (Figure 2) showed, the peak time of ABA production (3 h) preceded that of H2O2 production (6 h); then, at 12 h of Ca(NO3)2 treatment, the activities of the antioxidant enzymes SOD, POD and APX peaked. It was hypothesized that the antioxidant defense induced by Ca(NO3)2 stress in the leaves of pumpkin-grafted cucumber plants was initiated by ABA and involved H2O2.
To test our hypothesis, subsequent experiments with different inhibitors and scavengers, such as the ABA inhibitor sodium tungstate (T) and the H2O2 scavenger DMTU, were performed. Our results showed that the increased H2O2 content under Ca(NO3)2 stress was blocked in the pumpkin-grafted cucumber leaves that were pretreated with T (Figure 4). However, the decrease in the H2O2 level of the pumpkin-grafted plants with T recovered by the application of exogenous ABA. These results suggest a crosslink between ABA and H2O2 signaling pathways. Previous studies have also clearly demonstrated that the increased H2O2 levels depend on the activation of ABA in pumpkin-grafted cucumber leaves under Ca(NO3)2 stress. Similar result was observed by Guan et al. (2000), who showed that endogenous H2O2 level significantly increased under high concentrations of ABA conditions. In addition, the antioxidant defense of leaves induced by Ca(NO3)2 treatment was significantly inhibited in pumpkin-grafted cucumber plants that were pretreated with T (Figure 5). T blocks the formation of ABA from abscisic aldehyde by impairing abscisic aldehyde oxidase (Hansen and Grossmann, 2000). These results suggest that ABA was required for the increased Ca(NO3)2 stress-induced H2O2 production and antioxidant defense in grafted cucumber plants.
It has been shown that ABA-induced ROS production increases the activities of SOD, CAT, APX and GR in maize (Jiang and Zhang, 2002a,b). Recent studies have determined that ABA and H2O2 induced activation of antioxidant enzymes by using transgenic tobacco plants in combination with their inhibitors or scavengers (Lu et al., 2014). In the present study, pretreatment with several ROS manipulators, such as the NADPH oxidase inhibitor DPI, the scavenger Tiron and the H2O2 scavenger DMTU, almost completely depressed ABA-induced antioxidant defense in the leaves of pumpkin-grafted cucumber plants under Ca(NO3)2 stress (Figure 6). In the leaves of maize seedlings, NADPH oxidase is involved in ABA-induced ROS production (Jiang and Zhang, 2002b). This may induce oxidative damage to plant cells, resulting in disrupted metabolic function and destroyed cellular integrity (Ozden et al., 2009). H2O2 generation induced by NADPH oxidase might be as a reaction cascade that triggers the antioxidant enzyme activities in Arabidopsis thaliana, thereby mitigating the salt stress-induced oxidative damage (Rejeb et al., 2015). According to the review by Forman (2007), H2O2 can be toxic to plants but can also be an important stress signal. H2O2 can be synthesized in response to exogenous ABA. H2O2 mediates, at least in part, ABA responses, including defense mechanisms, stomatal closure and gene expression (Guan et al., 2000; Pei et al., 2000; Saxena et al., 2016). Desikan et al. (2001) provided further evidence of H2O2 as a central signaling mediator of the abiotic stress response in plants by using cDNA microarray technology. Their studies showed that oxidative stress induced the expression of some genes, such as SLN1-SSK1 (a gene encoding a potential hybrid His kinase) and that MAPKKs (MAPK kinases) are up-regulated by H2O2. This evidence suggests that H2O2 was involved in the ABA-induced antioxidant defense in the leaves of pumpkin-grafted seedlings, thus enhancing cucumber tolerance in responses to Ca(NO3)2 stress.
Conclusion
In the present study, our results indicate that the ABA responses of pumpkin-grafted and self-grafted cucumber leaves were differently induced by 80 mM Ca(NO3)2 stress. The accumulation of ABA was involved in the rapid accumulation of H2O2, and the accumulation of H2O2 induced the activities of SOD, POD and APX and the expression of their encoded genes in pumpkin-grafted cucumber leaves (Figure 9). The higher capacity of antioxidant defense in the pumpkin-grafted cucumber plants induced by the ABA signaling pathways presented in our studies may be part of the reason for the better performance of these plants than that of self-grafted cucumber plants under Ca(NO3)2 stress (Supplementary Figure S1). The specific mechanism of ABA-H2O2 signaling requires further investigation to obtain more insight into the root-shoot signaling in rootstock-grafted cucumber plants.
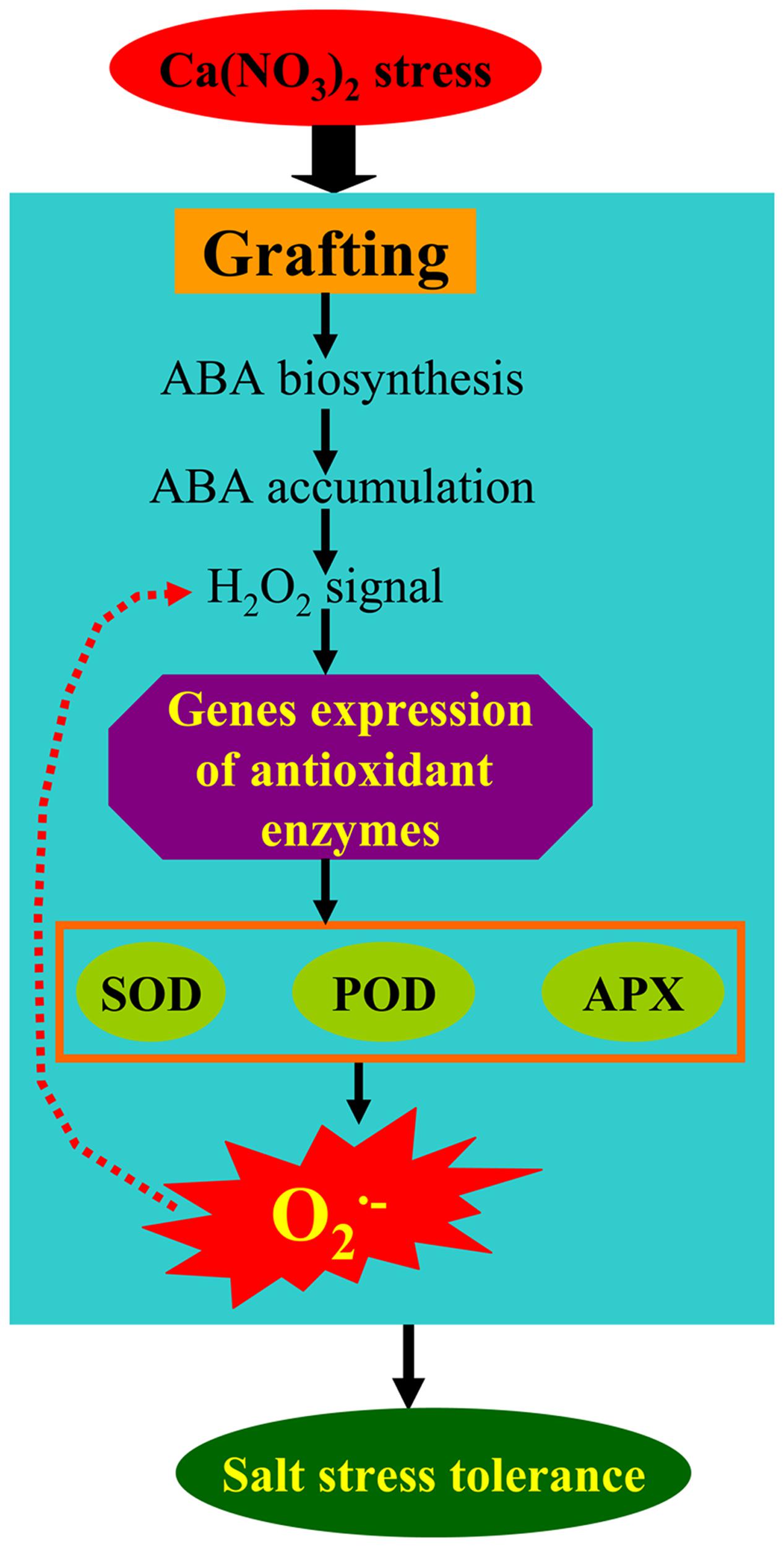
FIGURE 9. A simplified metabolic scheme and signal transduction pathway for ABA and H2O2 in the leaves of pumpkin-grafted cucumber seedlings exposed to 80 mM Ca(NO3)2. The model is based on the results presented here. The red arrow (dotted line) indicates possible metabolic pathways in our experiment.
Author Contributions
SS wrote the main manuscript text. PG, LL and YY prepared all figures and modified this manuscript until submitted. JS performed the experiments. SG designed the research and proposed the research proceeding. All authors reviewed the manuscript.
Funding
This work was financially supported by the Fundamental Research Funds for the Central Universities (6J0745), the National Natural Science Foundation of China (31471869, 31401919 and 31272209), the China Earmarked Fund for Modern Agro-industry Technology Research System (CARS-25-C-03), and the Priority Academic Program Development of Jiangsu Higher Education Institutions (PAPD).
Conflict of Interest Statement
The authors declare that the research was conducted in the absence of any commercial or financial relationships that could be construed as a potential conflict of interest.
Supplementary Material
The Supplementary Material for this article can be found online at: http://journal.frontiersin.org/article/10.3389/fpls.2016.01489
FIGURE S1 | Phenotypic response of self-grafted and pumpkin-grafted cucumber seedlings exposed to 80 mM Ca(NO3)2 for 7 days. P-G, pumpkin-grafted cucumber seedlings grown in Hoagland’s solution; P-GN, pumpkin-grafted cucumber seedlings with 80 mM Ca(NO3)2; S-G, self-grafted cucumber seedlings grown in Hoagland’s solution; S-GN, self-grafted cucumber seedlings with 80 mM Ca(NO3)2.
Abbreviation
ABA, abscisic acid; APX, ascorbate peroxidase; CAT, catalase; DMTU, dimethylthiourea; DPI, diphenyleneiodonium chloride; POD, peroxidase; SOD, superoxide dismutase; Tiron, 1,2-dihydroxybenzene- 3,5-disulphonic acid.
Footnotes
References
Aebi, H. (1974). “Catalase,” in Methods of Enzymatic Analysis, ed. H. U. Bergmeyer (New York, NY: Academic Press), 673–677.
Blanco, F. F., and Folegatti, M. V. (2002). Salt accumulation and distribution in a greenhouse soil as affected by salinity of irrigation water and leaching management. Rev. Bras. Eng. Agríc. E Ambient. 6, 414–419. doi: 10.1590/S1415-43662002000300006
Brennan, T., and Frenkel, C. (1977). Involvement of hydrogen peroxide in the regulation of senescence in pear. Plant Physiol. 59, 411–416. doi: 10.1104/pp.59.3.411
Daliakopoulos, I. N., Pappa, P., Grillakis, M. G., Varouchakis, E. A., and Tsanis, I. K. (2016). Modeling soil salinity in greenhouse cultivations under a changing climate with saltmed: model modification and application in Timpaki. Crete. Soil Sci. 181, 241–251. doi: 10.1097/SS.0000000000000161
Desikan, R., Soheila, A. H., Hancock, J. T., and Neill, S. J. (2001). Regulation of the Arabidopsis transcriptome by oxidative stress. Plant Physiol. 127, 159–172. doi: 10.1104/pp.127.1.159
FAO (2013). Food And Agriculture Organization Of The United Nations Statistics Division. Available at: http://faostat3.fao.org/download/Q/QC/E
Foreman, J., Demidchik, V., Bothwell, J. H. F., Mylona, P., Miedema, H., Torres, M. A., et al. (2003). Reactive oxygen species produced by NADPH oxidase regulate plant cell growth. Nature 422, 442–446. doi: 10.1038/nature01485
Forman, H. J. (2007). Use and abuse of exogenous H2O2 in studies of signal transduction. Free Radic. Biol. Med. 42, 926–932. doi: 10.1016/j.freeradbiomed.2007.01.011
Foyer, C. H., Neukermans, J., Queval, G., Noctor, G., and Harbinson, J. (2012). Photosynthetic control of electron transport and the regulation of gene expression. J. Exp. Bot. 63, 1637–1661. doi: 10.1093/jxb/ers013
Gao, H., Zhang, Z. K., Chai, H. K., Cheng, N., Yang, Y., Wang, D. N., et al. (2016). Melatonin treatment delays postharvest senescence and regulates reactive oxygen species metabolism in peach fruit. Postharvest Biol. Technol. 118, 103–110. doi: 10.1016/j.postharvbio.2016.03.006
Gao, P., Xing, W. W., Li, S. H., Shu, S., Li, H., Li, N., et al. (2015). Effect of pumpkin rootstock on antioxidant enzyme activities and photosynthetic fluorescence characteristics of cucumber under CA(NO3)2 stress. Acta Hortic. 1086, 177–187. doi: 10.17660/ActaHortic.2015.1086.22
Giannopolitis, C. N., and Ries, S. K. (1997). Superoxide dismutase. I. Occurrence in higher plants. Plant Physiol. 59, 309–314.
Giraudat, J., Parcy, F., Bertauche, N., Gosti, F., Leung, J., Morris, P. C., et al. (1994). Current advances in abscisic acid action and signalling. Plant Mol. Biol. 26, 1557–1577. doi: 10.1007/BF00016490
Guan, L. Q., Zhao, J., and Scandalios, J. G. (2000). Cis-elements and trans-factors that regulate expression of the maize Cat1 antioxidant gene in response to ABA and osmotic stress: H2O2 is the likely intermediary signaling molecule for the response. Plant J. 22, 87–95. doi: 10.1046/j.1365-313x.2000.00723.x
Hansen, H., and Grossmann, K. (2000). Auxin-induced ethylene triggers abscisic acid biosynthesis and growth inhibition. Plant Physiol. 124, 1437–1448. doi: 10.1104/pp.124.3.1437
He, F. F., Chen, Q., Jiang, R. F., Chen, X. P., and Zhang, F. S. (2007). Yield and nitrogen balance of greenhouse tomato (Lycopersium esculentum Mill.) with conventional and site-specific nitrogen management in northern China. Nutr. Cycl. Agroecosyst. 77, 1–14. doi: 10.1007/s10705-006-6275-7
Jiang, M. Y., and Zhang, J. H. (2002a). Involvement of plasma membrane NADPH oxidase in abscisic acid and water stress induced antioxidant defense in leaves of maize seedlings. Planta 215, 1022–1030. doi: 10.1007/s00425-002-0829-y
Jiang, M. Y., and Zhang, J. H. (2002b). Water stress-induced abscisic acid accumulation triggers the increased generation of reactive and up-regulates the activities of antioxidant enzymes in maize leaves. J. Exp. Bot. 53, 2401–2410. doi: 10.1093/jxb/erf090
Jiang, M. Y., and Zhang, J. H. (2003). Cross-talk between calcium and reactive oxygen species originated from NADPH oxidase in abscisic acid-induced antioxidant defense in leaves of maize seedlings. Plant Cell Environ. 26, 929–939. doi: 10.1046/j.1365-3040.2003.01025.x
Kwak, J. M., Mori, I. C., Pei, Z. M., Leonhardt, N., Torres, M. A., Dangl, J. L., et al. (2003). NADPH oxidase AtrbohD and AtrbohF genes function in ROS-dependent ABA signaling in Arabidopsis. EMBO J. 22, 2623–2633. doi: 10.1093/emboj/cdg277
Laloi, C., Mestres-Ortega, D., Marco, Y., Meyer, Y., and Reichheld, J. P. (2004). The Arabidopsis cytosolic thioredoxin h5 gene induction by oxidative stress and its w-box-mediated response to pathogen elicitor. Plant Physiol. 134, 1006–1016. doi: 10.1104/pp.103.035782
Lee, J. M., and Oda, M. (2002). Grafting of herbaceous vegetable and ornamental crops. Hortic. Rev. 28, 61–124. doi: 10.1002/9780470650851.ch2
Liang, Y. C., Si, J., Nikolic, M., Peng, Y., Chen, W., and Jiang, Y. (2005). Organic manure stimulates biological activity and barley growth in soil subject to secondary salinization. Soil Biol. Biochem. 37, 1185–1195. doi: 10.1016/j.soilbio.2004.11.017
Lu, S. Y., Zhuo, C. L., Wang, X. H., and Guo, Z. F. (2014). Nitrate reductase (NR)-dependent NO production mediates ABA-and H2O2-induced antioxidant enzymes. Plant Physiol. Biochem. 74, 9–15. doi: 10.1016/j.plaphy.2013.10.030
Ng, L. M., Melcher, K., Teh, B. T., and Xu, H. E. (2014). Abscisic acid perception and signaling: structuralmechanisms and applications. Acta Pharmacol. Sin. 35, 567–584. doi: 10.1038/aps.2014.5
Ozden, M., Demirel, U., and Kahraman, A. (2009). Effects of proline on antioxidant system in leaves of grapevine (Vitis vinifera L.) exposed to oxidative stress by H2O2. Sci. Hortic. 119, 163–168. doi: 10.1016/j.scienta.2008.07.031
Park, S. Y., Ryu, S. H., Jang, I. C., Kwon, S. Y., Kim, J. G., and Kwak, S. S. (2004). Molecular cloning of a cytosolic ascorbate peroxidase cDNA from cell cultures of sweet potato and its expression in response to stress. Mol. Genet. Genomics 271, 339–346. doi: 10.1007/s00438-004-0986-8
Pei, Z. M., Murata, Y., Benning, G., Thomine, S., Klüsener, B., Allen, G. J., et al. (2000). Calcium channels activated by hydrogen peroxide mediate abscisic acid signalling in guard cells. Nature 406, 731–734. doi: 10.1038/35021067
Pinhero, R. G., Rao, M. V., Paliyath, C., Murr, D. P., and Fletcher, R. A. (1997). Changes in activities of antioxidant enzymes and their relationship to genetic and paclobutrazol-induced chilling tolerance of maize seedlings. Plant Physiol. 114, 695–704.
Rao, M. V., Paliyath, G., and Ormrod, D. P. (1996). Ultraviolet B and ozone-induced biochemical changes in antioxidant enzymes of Arabidopsis thaliana. Plant Physiol. 110, 125–136. doi: 10.1104/pp.110.1.125
Rejeb, K. B., Benzarti, M., Debeza, A., Baillyc, C., Savouréb, A., and Abdelly, C. (2015). NADPH oxidase-dependent H2O2 production is required for salt-induced antioxidant defense in Arabidopsis thaliana. J. Plant Physiol. 174, 5–15. doi: 10.1016/j.jplph.2014.08.022
Rivard, C. L., and Louws, F. J. (2008). Grafting to manage soilborne diseases in heirloom tomato production. HortScience 43, 2104–2111.
Roach, T., Beckett, R. P., Minibayeva, F. V., Colville, L., Whitaker, C., Chen, H., et al. (2010). Extracellular superoxide production, viability and redox poise in response to desiccation in recalcitrant Castanea sativa seeds. Plant Cell Environ. 33, 59–75. doi: 10.1111/j.1365-3040.2009.02053.x
Roychoudhury, A., Paul, S., and Basu, S. (2013). Cross-talk between abscisic acid-dependent and abscisic acid-independent pathways during abiotic stress. Plant Cell Rep. 32, 985–1006. doi: 10.1007/s00299-013-1414-5
Sah, S. K., Reddy, K. R., and Li, J. X. (2016). Abscisic acid and abiotic stress tolerance in crop plants. Front. Plant Sci. 7:571. doi: 10.3389/fpls.2016.00571
Sainju, U., Singh, B., and Whitehead, W. (2001). Comparison of the effects of cover crops and nitrogen fertilization on tomato yield, root growth, and soil properties. Sci. Hortic. 91, 201–214. doi: 10.1016/S0304-4238(01)00264-3
Saxena, I., Srikanth, S., and Chen, Z. (2016). Cross talk between H2O2 and interacting signal molecules under plant stress response. Front. Plant Sci. 7:570. doi: 10.3389/fpls.2016.00570
Seo, M., and Koshiba, T. (2011). Transport of ABA from the site of biosynthesis to the site of action. J. Plant Res. 124, 501–507. doi: 10.1007/s10265-011-0411-4
Verslues, P. E., and Bray, E. A. (2006). Role of abscisic acid (ABA) and Arabidopsis thaliana ABA-insensitive loci in low water potential-induced ABA and proline accumulation. J. Exp. Bot. 57, 201–212. doi: 10.1093/jxb/erj026
Wang, L. P., Sun, J., Guo, S. R., Tian, J., Yang, Y. J. (2012). Effects of graft with pumpkin rootstock on nitrogen metabolism and protein expression in the cucumber seedlings under iso-osmotic Ca(NO3)2 or NaCl stress. Plant Nutr. Fertilizer Sci. 18, 689–698.
Xing, W. W., Li, L., Gao, P., Li, H., Shao, Q. S., Shu, S., et al. (2015). Effects of grafting with pumpkin rootstock on carbohydrate metabolism in cucumber seedlings under Ca(NO3)2 stress. Plant Physiol. Biochem. 87, 124–132. doi: 10.1016/j.plaphy.2014.12.011
Yu, H. Y., Li, T. X., and Zhou, J. M. (2005). Secondary salinization of greenhouse soil and its effects on soil properties. Soils 37, 581–586. doi: 10.3321/j.issn:0253-9829.2005.06.002
Yuan, L. Y., Yuan, Y. H., Du, J., Sun, J., and Guo, S. R. (2012). Effects of 24-epibrassinolide on nitrogen metabolism in cucumber seedlings under Ca(NO3)2 stress. Plant Physiol. Biochem. 61, 29–35. doi: 10.1016/j.plaphy.2012.09.004
Zhang, A. Y., Jiang, M. Y., Zhang, J. H., Tan, M. P., and Hu, X. L. (2006). Mitogen-activated protein kinase is involved in abscisic acid-induced antioxidant defense and acts downstream of reactive oxygen species production in leaves of maize plants. Plant Physiol. 141, 475–487. doi: 10.1104/pp.105.075416
Keywords: abscisic acid, antioxidant enzymes, Ca(NO3)2 stress, cucumber, grafting, hydrogen peroxide
Citation: Shu S, Gao P, Li L, Yuan Y, Sun J and Guo S (2016) Abscisic Acid-Induced H2O2 Accumulation Enhances Antioxidant Capacity in Pumpkin-Grafted Cucumber Leaves under Ca(NO3)2 Stress. Front. Plant Sci. 7:1489. doi: 10.3389/fpls.2016.01489
Received: 31 May 2016; Accepted: 20 September 2016;
Published: 30 September 2016.
Edited by:
Narendra Tuteja, International Centre for Genetic Engineering and Biotechnology, IndiaReviewed by:
Andrea Chini, Spanish National Research Council, SpainHarikesh Bahadur Singh, Banaras Hindu University, India
Copyright © 2016 Shu, Gao, Li, Yuan, Sun and Guo. This is an open-access article distributed under the terms of the Creative Commons Attribution License (CC BY). The use, distribution or reproduction in other forums is permitted, provided the original author(s) or licensor are credited and that the original publication in this journal is cited, in accordance with accepted academic practice. No use, distribution or reproduction is permitted which does not comply with these terms.
*Correspondence: Shirong Guo, c3JndW9AbmphdS5lZHUuY24=