- 1State Key Laboratory of Ecological Pest Control for Fujian and Taiwan Crops, Fujian Agriculture and Forestry University, Fuzhou, China
- 2Plant Protection College, Fujian Agriculture and Forestry University, Fuzhou, China
- 3Key Laboratory of Biopesticide and Chemical Biology, Ministry of Education, Fuzhou, China
- 4Key Laboratory of Integrated Pest Management for Fujian-Taiwan Crops, Ministry of Agriculture, China, Fuzhou, China
- 5Institute of Applied Ecology, Fujian Agriculture and Forestry University, Fuzhou, China
Transmission of plant pathogens through insect vectors is a complex biological process involving interactions between the host plants, insects, and pathogens. Simultaneous impact of the insect damage and pathogenic bacteria in infected host plants induce volatiles that modify not only the behavior of its insect vector but also of their natural enemies, such as parasitoid wasps. Therefore, it is essential to understand how insects such as the predator ladybird beetle responds to volatiles emitted from a host plant and how the disease transmission alters the interactions between predators, vector, pathogens, and plants. In this study, we investigated the response of Propylaea japonica to volatiles from citrus plants damaged by Diaphorina citri and Candidatus Liberibacter asiaticus through olfactometer bioassays. Synthetic chemical blends were also used to determine the active compounds in the plant volatile. The results showed that volatiles emitted by healthy plants attracted more P. japonica than other treatments, due to the presence of high quantities of D-limonene and beta-ocimene, and the lack of methyl salicylate. When using synthetic chemicals in the olfactory tests, we found that D-limonene attracted P. japonica while methyl salicylate repelled the predator. However, beta-ocimene attracted the insects at lower concentrations but repelled them at higher concentrations. These results indicate that P. japonica could not efficiently search for its host by using volatile cues emitted from psyllids- and Las bacteria-infected citrus plants.
Introduction
Plants are constantly damaged by sap-feeding insects that not only create physical damage while probing but also transmit pathogens that may cause serious disease in plants. Insect-borne pathogens can induce changes in the traits of their primary hosts as well as their vectors that eventually affect the frequency and nature of interactions between hosts and vectors (Eigenbrode et al., 2002; Purcell, 2003). Simultaneous damage by insects as well as pathogenic bacteria induces infested host plants to secrete volatiles that modify not only the behavior of its insect vector but also their natural enemies.
Infected plants have been reported to attract insects infested with pathogens more than a healthy plant (Mann et al., 2009). For example, cucumber mosaic virus (CMV) infected plants emit some chemicals that can manipulate the behavior (increase the attractiveness) of the vector aphids, Myzus persicae (Sulzer) and Aphis gossypii Glover (Hemiptera: Aphididae) (Mauck et al., 2010). In another study, the phytopathogenic bacterium, Candidatus Liberibacter asiaticus (Rhizobiales: Rhizobiaceae) (Las) infected citrus was found to alter the release of specific headspace volatiles and attract more Diaphorina citri Kuwayama (Hemiptera: Psyllidae) vectors (Mann et al., 2012). In addition, Las has the ability to manipulate the propensity of psyllids movement and consequently satisfy the vector to promote their spread (Martini et al., 2015). However, de Oliveira et al. (2014) found that the bird cherry-oat aphid, Rhopalosiphum padi (Linnaeus) (Hemiptera: Aphididae), which is a vector of cereal yellow dwarf virus (CYDV), a pathogen of wheat, became more vulnerable to attacks by the parasitoid wasp, Aphidius colemani Viereck (Hymenoptera: Braconidae) when carrying CYDV. This indicates that plant pathogens alter the metabolites of infected host plants (Rogers and Bates, 2007; Mauck et al., 2010; Van Den Abbeele et al., 2010; Davis et al., 2012; Mann et al., 2012).
Studies have shown that parasitoids are more sensitive to volatiles emitted by infected plants. To attract more insect vectors, Las induces citrus plants to emit more methyl salicylate (Mann et al., 2012). However, simultaneous attack of citrus plants by Las and psyllids, change both the profile and quantity of volatiles secreted (Hijaz and Killiny, 2016). Interestingly, methyl salicylate, produced by Las-infested host plants, is not only used by the bacterial pathogen to manipulate the behavior of its insect vector to promote its own proliferation, but also used by an effective ectoparasitoid of D. citri, Tamarixia radiata (Waterston) (Hymenoptera: Eulophidae), as a cue to trace its host insect (Martini et al., 2014).
As a consequence, plant pathogens not only regulate the metabolism of host plants, but also influence their vectors, while parasitoids become more sensitive to new metabolites. Polyphagous insect predators are also attracted by herbivore-induced-plant volatiles (Ninkovic et al., 2001; Gencer et al., 2009). It is known that parasitoids are more sensitive to and are attracted by the induced volatiles than their predators (Li et al., 2014). However, little is known about the mechanism that regulates this difference. Therefore, in this study, we used ladybird beetles as models to address the question how ladybird beetles respond to volatiles emitted from host plants that are damaged by insect vectors as well as are infected by plant pathogens.
Las is a phytopathogenic bacterium that is spread by an insect vector, the Asian citrus psyllid, D. citri. It is a gram-negative, fastidious, phloem-limited bacterium that causes huanglongbing (HLB) disease in citrus (Roossinck, 2011). Ladybird beetles are known as one of the polyphagous insect predators of D. citri in citrus orchards (Michaud et al., 2004). In this study, we investigated the response of a common insect vector Propylaea japonica (Thunberg) (Coleoptera: Coccinellidae), in the crop systems of China, to volatiles from citrus damaged by both D. citri and Las bacteria. Synthetic chemicals were also used to determine the active compounds in plant volatile blends that affect P. japonica.
Materials and Methods
Plants
Sour orange (Citrus aurantium L.) seeds were cultured in an insect-proof greenhouse at 28°C, 40% RH and L16: D8 for 4 months. The 4-month-old seedlings were infested by grafting them with four pieces of bud wood sticks from a PCR-positive HLB source. The infection was determined and confirmed using PCR as described by Tatineni et al. (2008). Infected (4 month post inoculation) and uninfected 8-month-old citrus plants were used in this study.
Insect Colonies
Propylaea japonica adults and larvae were collected from the fields of Fuzhou, Fujian, China (26.08 N, 119.28 W) in 2014, and reared on D. citri that were maintained on Murraya paniculata L. (Jack). Five-day-old adult P. japonica, which emerged from the established colony (third generation) were selected for experiments. Diaphorina citri used for rearing the ladybird beetles and for the experiments were obtained from a colony established in 2014 from field populations in Ganzhou, Jiangxi, China (25.85 N, 114.92 W); and 2- to 3-day-old adults were selected for the tests. This D. citri culture was maintained on uninfected sour orange plants in a clean 50 cm × 50 cm × 50 cm netted cage in an insect-proof greenhouse maintained at 28°C, 40% RH, and L16: D8. Psyllids reared on the infested citrus plants were sampled to assess Las bacteria infection by PCR, and the results were positive.
Olfactometer Assays of Propylaea japonica
The response of P. japonica to odor sources was studied in two-choice tests with a closed system Y-tube olfactometer, using the method described by Gencer et al. (2009) with a slight modification. Five treatment combinations were used to test the attraction of the chemicals to P. japonica with an olfactometer: (1) healthy citrus (HC) vs. Las-infected citrus (LC); (2) healthy citrus infested with psyllids D. citri (HCfP) vs. Las-infected citrus infested with psyllids (LCfP); (3) HC vs. HCfP; (4) LC vs. LCfP; and (5) psyllids only vs. fresh air only. For the feeding damage treatments or citrus infestation with the psyllids, 50 adult male and 50 adult female psyllids were placed on plants for 24 h before assays (Mann et al., 2012; Martini et al., 2014). This duration of feeding damage has been proven sufficient to release herbivore-induced volatiles from citrus plants (Mann et al., 2012). Plant samples were placed in 35 cm tall × 25 cm wide dome-shaped volatile collection chambers as described by Lin et al. (2016). For the olfactometer experiment, purified air at the rate of 300 ml min-1 was blown into the chambers from the inlet, and then along with headspace and flowed out of the outlet, then went into the arm of the Y-tube (Figure 1). A cotton ball was placed at the end of each arm of the Y-tube to block the released ladybird beetle. Adult P. japonica were released individually at the entry of the Y-tube and were allowed 300 s to exhibit a behavioral response (Martini et al., 2015). Fifty adult P. japonica (male:female ratio 1:1) were individually released into the Y-tubes for each of the five treatments as defined above. Y-tubes were cleaned with hot water (above 60°C) after every set of five insects was tested. Different sets of plant treatments were used to test 50 adult P. japonica, and the selected adult ladybird beetles were starved for 24 h before testing. In total, there were five replicates in this experiment.
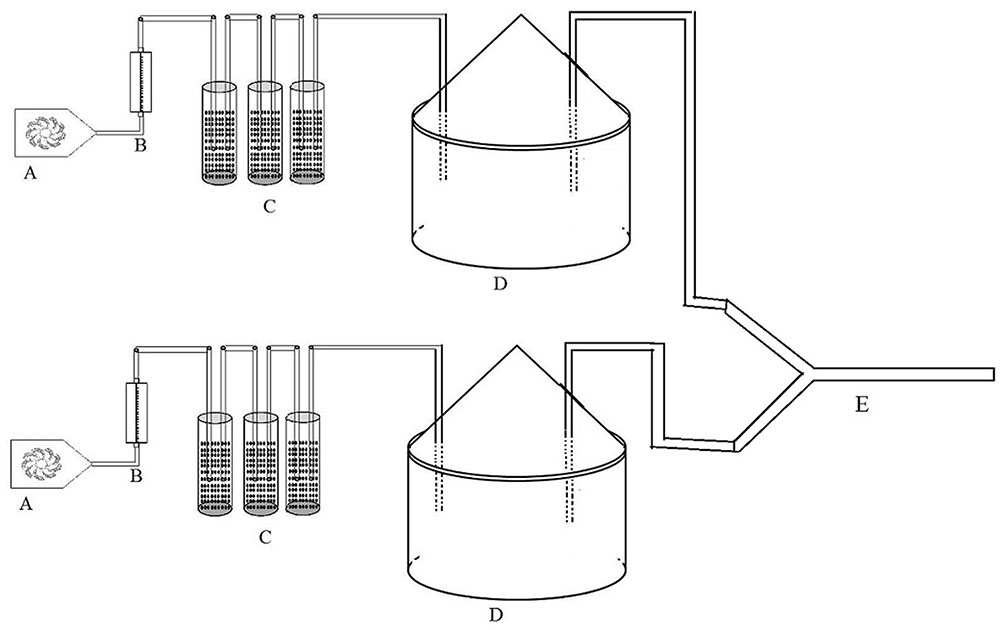
FIGURE 1. Schematic diagram of headspace collecting system. (A) pump, (B) flow meter, (C) air-purify cup, (D) glass jar, and (E) Y-tube.
Headspace Collection and Volatiles Analysis
Headspace was used to collect volatiles for 24 h with a collecting system described by Pineda et al. (2013). Four treatments were used as described above; HC, LC, HCfP, and LCfP. For the feeding damage treatments, 50 adult male and 50 adult female psyllids were placed on plants for 24 h prior to the bioassays (Mann et al., 2012; Martini et al., 2014). All the treatment plants were placed in clean glass jars inside the collecting chamber as mentioned above. Fresh air was blown into the jar at the rate of 300 ml min-1 and flowed out of the headspace through a glass tube filled with absorbent, 200 mg Tenax TA (60/80 mesh; Grace-Alltech, Deerfield, MA, USA). After 24 h collection, the herbivore-induced volatiles, Las-induced volatiles and herbivore-Las-induced volatiles (HBIPVs), which were trapped by Tenax TA, were immediately eluted into 1 ml methenyl trichloride and kept at -20°C until analysis.
The herbivore-induced volatiles, Las-induced volatiles and HBIPVs were analyzed with a gas chromatograph (Agilent Technologies 7890B GC System)-mass spectrometer (Agilent Technologies 5977A MSD) (GC-MS) aligned with HP-5 column (30 mm × 0.25 mm, i.d., 1.0 μm film thickness, Agilent). The GC-MS oven temperature was programmed from 40°C (5-min hold) to 280°C at the rate of 10°C min-1. The column effluent was ionized by electron impact ionization at 70 eV. Mass spectra were acquired by scanning from 35 to 350 m/z with a scan rate of 5.38 scans s-1.
Compounds were identified by using the deconvolution software AMDIS (version 2.64, NIST, USA) in combination with NIST 05 and Wiley seventh edition spectral libraries and by comparing their retention indices with those from the literature. Additional experiments were conducted prior to the bioassays and the results are compiled in the Supplementary documents (Supplementary Experiments 1, 2, and 3 dataset).
Propylaea japonica Response to Synthetic HBIPVs Source
As shown in Supplementary Table 3, D-limonene, methyl salicylate and beta-ocimene volatile compounds collected from the headspace have a significant correlation with the olfactory response of P. japonica and therefore were considered for single and blends bioassays. For the experiments, the synthetic version of the three chemicals mentioned above (methyl salicylate, beta-ocimene, and D-limonene) were purchased from Macklin Biochemical Co. Ltd. (Shanghai, China) with approximately 97–99% purity.
For testing single dosage, D-limonene was dissolved in triethyl citrate (TEC) to 50, 25, 17, 10, 1, and 0.1 nmol ml-1; methyl salicylate was dissolved in TEC to 0.03, 0.06, 0.15, 0.18, 0.32, and 0.64 nmol ml-1; and beta-ocimene was dissolved in TEC to 0.04, 0.08, 0.20, 0.45, 1.00, and 2.00 nmol ml-1 concentrations. The range of dilutions used for the bioassays was based on the Supplementary Experiment 2 mentioned above (Supplementary Tables 4 and 5). However, for testing blends, these three chemical compounds were mixed in TEC at different concentrations as follows: (1) blend HC: D-limonene 24.75 nmol ml-1 and beta-ocimene 1.00 nmol ml-1; (2) blend LC: D-limonene 17.32 nmol ml-1, methyl salicylate 0.06 nmol ml-1 and beta-ocimene 0.45 nmol ml-1; (3) blend HCfP: D-limonene 10.13 nmol ml-1, methyl salicylate 0.15 nmol ml-1, and beta-ocimene 0.20 nmol ml-1; and (4) blend LCfP: D-limonene 1.06 nmol ml-1, methyl salicylate 0.18 nmol ml-1, and beta-ocimene 0.08 nmol ml-1 (Supplementary Table 5).
Then, 1 ml of each suspension solution was applied to a small cotton roll and placed in the collecting chamber. Purified air at the rate of 300 ml min-1 was pumped/blown into chambers from the inlet, and then along with volatile and flowed out from the outlet, through the arm of the Y-tube. At the same time, fresh air was forced/pumped directly into the other arm of the Y-tube with a pump at the rate of 300 ml min-1 (Figure 1). Adult P. japonica were released individually at the entry of the Y-tube and allowed 300 s to exhibit a behavioral response as described above (Martini et al., 2015). Y-tubes were cleaned with hot water (above 60°C) after every set of five insects was tested. Different sets of blends (blend HC, blend LC, blend HCfP, and blend LCfP) and single dosages as defined above were used to test 50 adult P. japonica, and selected adult ladybird beetles were starved for 24 h prior to the bioassay test, and the experiment was conducted five times.
Data Analysis
For the Y-tube olfactometer assays, we performed chi-squared tests on the mean values of five replicates. In the Supplementary Experiment, we performed Pearson Correlation to determine the correlation between the olfactory responses of P. japonica to citrus volatile blends. We used SPSS 21.0 for overall statistical analyses.
Results
Olfactometer Assays of P. japonica
The results obtained from the olfactometer assays that tested the behavior of P. japonica to the different volatiles emitted from damaged citrus plants are shown in Figure 2 with different effects on the predator. HC treatment was more attractive to P. japonica than treatments LC (χ2 = 12.89, df = 4, P = 0.024) and HCfP (χ2 = 11.63, df = 4, P = 0.040). Conversely, treatment LCfP was less attractive to P. japonica than treatments LC (χ2 = 9.85, df = 4, P = 0.043) and HCfP (χ2 = 17.31, df = 4, P = 0.004) (Figure 2).
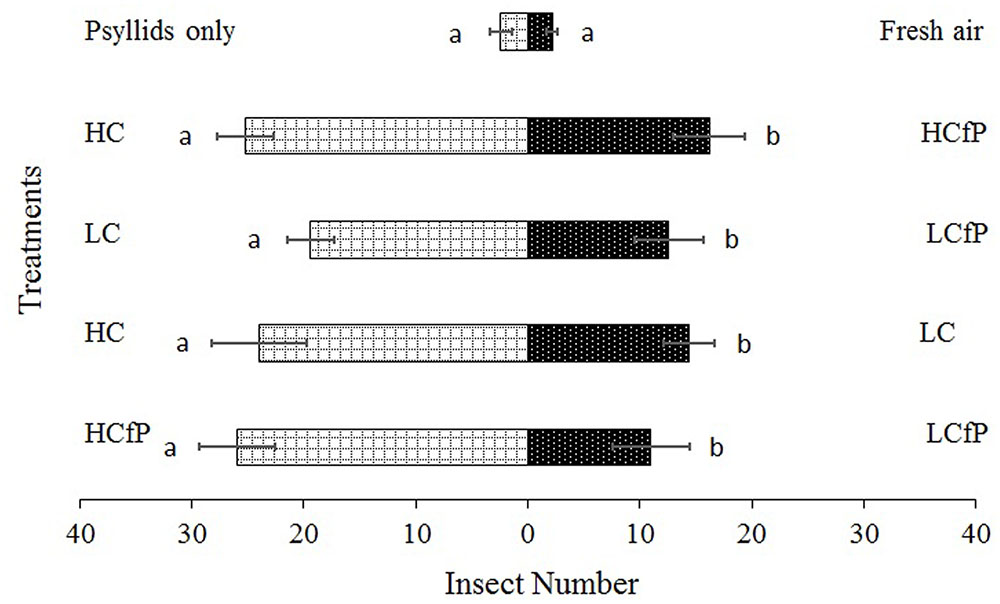
FIGURE 2. Olfactometer assays of Propylaea japonica with volatiles emitted from treated citrus plants. Bars indicate mean ± SE; means within rows followed by different alphabets indicate significant differences at P < 0.05. HC, healthy citrus; LC, Las-infected citrus; HCfP, healthy citrus infested with psyllids; LCfP, Las-infected citrus infested with psyllids.
Headspace Collected Volatiles and Analysis
The various volatiles collected from the headspace system from the damaged and infested citrus plants were detected and quantified by GC-MS. Three major chemical compounds (D-limonene, methyl salicylate, and beta-Ocimene) were identified as shown in Table 1. All three compounds were found in the various treatments in variable quantities, except methyl salicylate, which was absent in the HC treatment (Table 1). There was significant difference (F2, 2 = 65.01, P = 0.002) in the emitted quantity of D-limonene between the treatments. The amounts of D-limonene in treatments HC (13.45 ± 0.99) and LC (10 ± 0.62) did not vary but were considerably higher than in treatments HCfP (6.26 ± 0.73) and LCfP (0.70 ± 0.03) (Table 1). Similarly, there was significant difference (F2, 2 = 165.86, P = 0.0001) between the treatments in the quantities of methyl salicylate produced, which was higher in LCfP (0.097 ± 0.006) compared to HCfP (0.055 ± 0.001) and LC (0.028 ± 0.001). In addition, significantly high amounts of beta-ocimene was obtained in HC (0.62 ± 0.04) compared to other treatments (F2, 2 = 58.84, P = 0.002); while the lowest quantity was emitted in LCfP (0.051 ± 0.037) (Table 1).
Propylaea japonica Responses to Synthetic Compounds
Three compounds, D-limonene, methyl salicylate and beta-ocimene, among the emitted volatiles correlated significantly with the olfactory responses of P. japonica, while the remaining were not (Supplementary Table 3). Therefore, D-limonene, beta-ocimene and methyl salicylate were chosen for the bioassays to further study the responses of P. japonica.
Single dosages and blends of D-limonene, methyl salicylate, and beta-ocimene were used as odor sources for the olfactometer assays. The responses of P. japonica to the three synthetic compounds are shown in Figures 3 and 4.
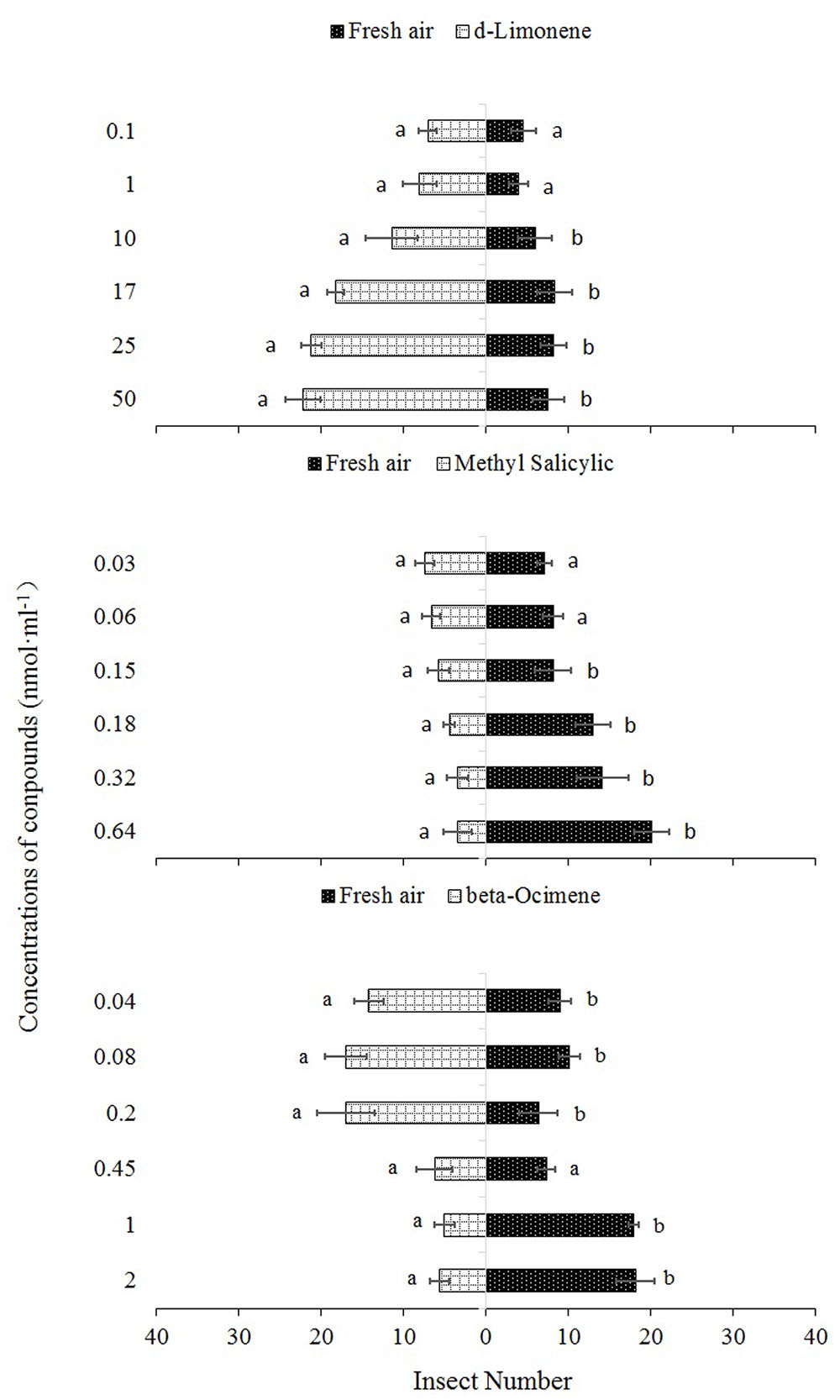
FIGURE 3. Propylaea japonica responses to three synthetic chemical compounds in the olfactometer. Bars indicate mean ±SE; means within rows followed by different alphabets indicate significant differences at P < 0.05. HC, healthy citrus; LC, Las-infected citrus; HCfP, healthy citrus infested with psyllids; LCfP, Las-infected citrus infested with psyllids.
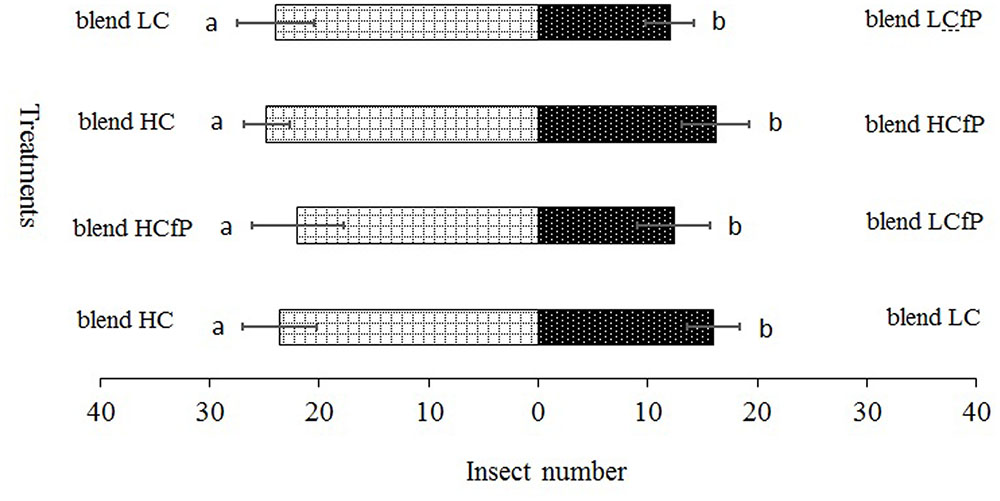
FIGURE 4. Propylaea japonica responses to the three different chemical compound blends in olfactometer. (1) blend HC: D-limonene 24.75 nmol ml-1 and beta 1.00 nmol ml-1; (2) blend LC: D-limonene 17.32 nmol ml-1, methyl salicylate 0.06 nmol ml-1, and beta-ocimene 0.45 nmol ml-1; (3) blend HCfP: D-limonene 10.13 nmol ml-1, methyl salicylate 0.15 nmol ml-1, and beta-ocimene 0.20 nmol ml-1; (4) blend LCfP: D-limonene 1.06 nmol ml-1, methyl salicylate 0.18 nmol ml-1, and beta-ocimene 0.08 nmol ml-1. Bars indicate mean ±SE; means within rows followed by different alphabets indicate significant differences at P < 0.05.
In the single dosage treatments, D-limonene concentrations of 50, 25, 17, and 10 nmol ml-1 were significantly more attractive to P. japonica than fresh air (χ2 = 12.67, df = 4, P = 0.013; χ2 = 11.05, df = 4, P = 0.026; χ2 = 9.85, df = 4, P = 0.043; χ2 = 9.73, df = 4, P = 0.045, respectively). However, no significant differences were observed between fresh air and concentrations 0.1 nmol ml-1 (χ2 = 0.99, df = 4, P = 0.912) and 1 nmol ml-1 (χ2 = 1.00, df = 4, P = 0.910) (Figure 3). Similarly, in the single treatment of methyl salicylate, concentrations 0.64, 0.32, 0.18, and 0.15 nmol ml-1 had significantly high repellent effects on P. japonica compared to fresh air (χ2 = 10.45, df = 4, P = 0.033; χ2 = 10.45, df = 4, P = 0.033; χ2 = 9.95, df = 4, P = 0.041; χ2 = 11.35, df = 4, P = 0.023, respectively) while no significant differences were observed between fresh air and 0.03 nmol ml-1 (χ2 = 5.00, df = 4, P = 0.288) and 0.06 nmol ml-1 (χ2 = 2.51, df = 4, P = 0.644) concentrations (Figure 3). In the single treatment of beta-ocimene, concentrations 0.04, 0.08, and 0.2 nmol ml-1 showed significantly high attractive effect (χ2 = 11.78, df = 4, P = 0.019; χ2 = 9.69, df = 4, P = 0.046; χ2 = 11.53, df = 4, P = 0.021, respectively) to P. japonica, while 1.0 nmol ml-1 (χ2 = 13.91, df = 4, P = 0.008) and 2.0 nmol ml-1 (χ2 = 10.29, df = 4, P = 0.036) were significantly repellent to the predator compared to fresh air and the three remaining concentrations (0.04, 0.08, and 0.2 nmol ml-1) (Figure 3). However, there was no significant difference (χ2 = 1.51, df = 4, P = 0.825) between 0.45 nmol ml-1 and fresh air (Figure 3).
For the blend treatments, the results showed that the HC blend was more attractive to P. japonica than blends LC (χ2 = 12.20, df = 4, P = 0.016) and HCfP (χ2 = 10.06, df = 4, P = 0.039). Conversely, LCfP was less attractive to P. japonica than LC (χ2 = 11.29, df = 4, P = 0.024) and HCfP (χ2 = 13.44, df = 4, P = 0.009) (Figure 4).
Discussion
Plants effectively use their secondary metabolites to protect themselves against herbivores and pathogens (Francis et al., 2004; Zhu and Park, 2005; Boone et al., 2008; Halitschke et al., 2008; Unsicker et al., 2015). At the same time, natural enemies use these induced compounds to seek their hosts. When insect vectors feed on pathogen infected plants, the defense mechanisms of the natural enemies, plants as well as the vectors are disturbed, due to changes in the synthesis of plant volatiles (Francis et al., 2004; Sullivan, 2005; Boone et al., 2008; Unsicker et al., 2015). In this study, we found that D. citri-infected citrus emitted less D-limonene and beta-ocimene, compared to citrus damaged by the vector insect as well as infected by Las; these plants produced high quantities of methyl salicylate. This finding suggested that the plant pathogen, Las, interacts with its vector D. citri and regulates volatiles emission from the host plant.
In the first olfactometer test using citrus with multiple treatments, we found that volatiles emitted from HC plants attracted the most P. japonica, while volatiles from citrus damaged by the vector as well as Las were less attractive to the vector. This result is inconsistent with previous findings from the olfactometer tests conducted in wasps (Gouinguene et al., 2003; D’Alessandro and Turlings, 2005; Dannon et al., 2010; Xu et al., 2014; Mutyambai et al., 2015; Takemoto and Takabayashi, 2015). It suggests that HC plants can produce volatiles that may attractant P. japonica, but these volatiles may be down-regulated by Las and psyllids through volatile profiles modification. In addition, our results also indicated that, the two pests (Las bacteria Candidatus Liberibacter asiaticus and D. citri) may induce citrus host plants to emit repellent chemicals for P. japonica. Simultaneous insect damage and pathogenic bacterial infection of citrus host plants have been reported to induce volatiles that modify not only the behavior of its insect vector (Mann et al., 2012) but also their natural enemies (Li et al., 2014). A number of studies have demonstrated that HIPVs emitted by infected plants attract more obligatory parasites or wasps (Francis et al., 2004; Sullivan, 2005; Zhu and Park, 2005; Boone et al., 2008; Halitschke et al., 2008; Zhang et al., 2009; Unsicker et al., 2015). The results of this study showed that infection of plants with pathogens through insect vectors may alter the balance and amounts of volatiles emitted by the infected plants thus reducing their attraction to a facultative predator, P. japonica.
Among the major compounds emitted from treated citrus plants, D-limonene, methyl salicylate and beta-ocimene had significant correlation with the olfactory responses of P. japonica, while others did not (Supplementary Table 3). In the bioassay responses of P. japonica, we found that synthetic D-limonene was attractive to P. japonica while synthetic methyl salicylate repelled it. Besides, synthetic beta-ocimene had dual effects by attracting the predator at lower concentrations and repelling it at high concentrations, regardless of whether the citrus plants were damaged by psyllids and/or Las. These three synthetic compounds were therefore found to have a significant impact on P. japonica behavior particularly in the search for prey on infested citrus plants. This result suggests that D-limonene and beta-ocimene may act as attractive cues, while methyl salicylate could serve as a repellent signal for P. japonica. D-limonene is one of most significant emitted volatiles of citrus and was previously identified to attract predatory beetles (Wheeler et al., 2002; Francis et al., 2004; Himanen et al., 2005; Zhu and Park, 2005; Heil and Silva Bueno, 2007; Wei et al., 2007; Boone et al., 2008; Halitschke et al., 2008; Zhang et al., 2009; Hare and Sun, 2011; Unsicker et al., 2015). Contrary to this, some studies also demonstrated that methyl salicylate could be used as an attractant cue for some beetle species (Francis et al., 2004; Boone et al., 2008; Halitschke et al., 2008; Zhang et al., 2009; Unsicker et al., 2015). Variations in the emitted quantities of these three major compounds may therefore explain the reduction in the attractiveness of Las-infected and/or psyllids-infested citrus host plants to P. japonica, compared to healthy plants. By comparing the three synthetic chemicals, D-limonene, beta-ocimene and methyl salicylate, we found that D-limonene is more attractive to P. japonica at concentrations 1nmol or less. On the contrary, methyl salicylate repelled the beetle, and the effect was more robust at higher concentrations. This suggests that the reduction in P. japonica attraction in treated citrus plants could have been caused by D-limonene (decreasing) and methyl salicylate (increasing). Interestingly, beta-ocimene showed both attractive and repellent activity at different concentrations and consequently, could be used together with D-limonene to increase P. japonica attraction in the management of D. citri.
It is interesting that psyllids induced volatiles from LC were less attractive to P. japonica than volatiles emitted from a HC plant since it is know that many insect infested host plants emit HIPVs to attract natural enemies, especially parasitoid wasps. One reason in the reduction of P. japonica prey performance might be due to changes in the physical constitution of psyllids; i.e., modifications caused by Las bacteria might not support the feeding and development of P. japonica. Since P. japonica is generalist ladybird beetle (Halitschke et al., 2008), it may exercise choice feeding on other prey and not feed on infected psyllids.
It seems that the vector and Las developed a strategy through long-term evolution to deceive infested citrus plants into repelling the ladybird beetles. This could be a mechanism by which herbivores fight their predators through avoidance behavior. It therefore suggests that the ladybird beetle, P. japonica, might not be a good biological candidate to be used to control vectors in some situations. On the other hand, some studies have shown that the volatiles from different cultivars, and the emission from resistant cultivar were more attractive to natural enemies than those from susceptible cultivars (Krips et al., 1996; Michereff et al., 2011). This may be due to the variation in volatile profiles therefore the combination of predator/prey/pathogens in different plant genetic backgrounds needs to be studied. Further studies are also warranted to understand why P. japonica reacts differently to the three emitted compounds. These findings may promote the application of volatiles and specifically D-limonene in biocontrol strategies.
Author Contributions
Conceived and designed the experiments: YL, SL, and LW. Performed the experiments: YL and MH. Analyzed the data: YL, MH, and KA. Contributed reagents/materials/analysis tools: YL, SL, and KA. Wrote the paper: YL, MH, KA, and LW.
Funding
This project is funded by National Natural Science Foundation of China (31371998), Key Projects of Science and Technology of Fujian Province (2016N0005) and a Research Fund for the Doctoral Program of Higher Education of China (20123515110003).
Conflict of Interest Statement
The authors declare that the research was conducted in the absence of any commercial or financial relationships that could be construed as a potential conflict of interest.
Acknowledgments
The authors are grateful to Prof. Minsheng You (Institute of Applied Ecology, FAFU, China) for his kind cooperation. This project was funded by the National Natural Science Foundation of China (31371998) and Key projects of Science and Technology of Fujian Province (2016N0005). The authors also thank LetPub (www.letpub.com) for its linguistic assistance during the preparation of this manuscript.
Supplementary Material
The Supplementary Material for this article can be found online at: http://journal.frontiersin.org/article/10.3389/fpls.2016.01969/full#supplementary-material
References
Boone, C. K., Six, D. L., Zheng, Y., and Raffa, K. F. (2008). Parasitoids and dipteran predators exploit volatiles from microbial symbionts to locate bark beetles. Environ. Entomol. 37, 150–161. doi: 10.1093/ee/37.1.150
D’Alessandro, M., and Turlings, T. C. (2005). In situ modification of herbivore-induced plant odors: a novel approach to study the attractiveness of volatile organic compounds to parasitic wasps. Chem. Senses 30, 739–753. doi: 10.1093/chemse/bji066
Dannon, E. A., Tamo, M., Van Huis, A., and Dicke, M. (2010). Effects of volatiles from Maruca vitrata larvae and caterpillar-infested flowers of their host plant Vigna unguiculata on the foraging behavior of the parasitoid Apanteles taragamae. J. Chem. Ecol. 36, 1083–1091. doi: 10.1007/s10886-010-9859-2
Davis, T. S., Horton, D. R., Munyaneza, J. E., and Landolt, P. J. (2012). Experimental infection of plants with an herbivore-associated bacterial endosymbiont influences herbivore host selection behavior. PLoS ONE 7:e49330. doi: 10.1371/journal.pone.0049330
de Oliveira, C. F., Long, E. Y., and Finke, D. L. (2014). A negative effect of a pathogen on its vector? A plant pathogen increases the vulnerability of its vector to attack by natural enemies. Oecologia 174, 1169–1177. doi: 10.1007/s00442-013-2854-x
Eigenbrode, S. D., Ding, H., Shiel, P., and Berger, P. H. (2002). Volatiles from potato plants infected with potato leafroll virus attract and arrest the virus vector, Myzus persicae (Homoptera: Aphididae). Proc. R. Soc. B Biol. Sci. 269, 455–460. doi: 10.1098/rspb.2001.1909
Francis, F., Lognay, G., and Haubruge, E. (2004). Olfactory responses to aphid and host plant volatile releases: (E)-beta-farnesene an effective kairomone for the predator Adalia bipunctata. J. Chem. Ecol. 30, 741–755. doi: 10.1023/B:JOEC.0000028429.13413.a2
Gencer, N. S., Kumral, N. A., Sivritepe, H. O., Seidi, M., Susurluk, H., and Senturk, B. (2009). Olfactory response of the ladybird beetle Stethorus gilvifrons to two preys and herbivore-induced plant volatiles. Phytoparasitica 37, 217–224. doi: 10.1007/s12600-009-0032-9
Gouinguene, S., Alborn, H., and Turling, T. C. (2003). Induction of volatile emissions in maize by different larval instars of Spodoptera littoralis. J. Chem. Ecol. 29, 145–162. doi: 10.1023/A:1021984715420
Halitschke, R., Stenberg, J. A., Kessler, D., Kessler, A., and Baldwin, I. T. (2008). Shared signals -‘alarm calls’ from plants increase apparency to herbivores and their enemies in nature. Ecol. Lett. 11, 24–34. doi: 10.1111/j.1461-0248.2007.01123.x
Hare, J. D., and Sun, J. J. (2011). Production of herbivore-induced plant volatiles is constrained seasonally in the field but predation on herbivores is not. J. Chem. Ecol. 37, 430–442. doi: 10.1007/s10886-011-9944-1
Heil, M., and Silva Bueno, J. C. (2007). Within-plant signaling by volatiles leads to induction and priming of an indirect plant defense in nature. Proc. Natl. Acad. Sci. U.S.A. 104, 5467–5472. doi: 10.1073/pnas.0610266104
Hijaz, F., and Killiny, N. (2016). Citrus leaf volatiles response to ‘Candidatus Liberibacter asiaticus’ and to its insect vector Asian citrus psyllid. J. Citrus Pathol. 1, 222.
Himanen, S., Vuorinen, T., Tuovinen, T., and Holopainen, J. K. (2005). Effects of cyclamen mite (Phytonemus pallidus) and leaf beetle (Galerucella tenella) damage on volatile emission from strawberry (Fragaria x ananassa Duch.) plants and orientation of predatory mites (Neoseiulus cucumeris, N. californicus, and Euseius finlandicus). J. Agric. Food Chem. 53, 8624–8630. doi: 10.1021/jf050676j
Krips, O. E., Willems, P. E. L., Dicke, M., and Posthumus, M. A. (1996). “How a tritrophic interaction varies with plant cultivar: hostplant resistance, predator population increase and herbivore-induced synomones,” in Proceedings of the International Society of Chemical Ecology 13th Annual Meeting: Book of Abstracts, Conference Information, Prague, Vol. 16, 335–339.
Li, S. J., Ren, S. L., Xue, X., Ren, S. X., Cuthbertson, A. G., Dam, N. M. V., et al. (2014). Efficiency of plant induced volatiles in attracting Encarsia formosa and Serangium japonicum, two dominant natural enemies of whitefly Bemisia tabaci in China. Pest Manag. Sci. 70, 1604–1610. doi: 10.1002/ps.3749
Lin, Y., Mubasher, H., Bruce, A. P., Muhammad, Q., Fang, D., and Wang, L. (2016). Volatiles from plants induced by multiple aphid attacks promote conidial performance of Lecanicillium lecanii. PLoS ONE 11:e0151844. doi: 10.1371/journal.pone.0151844
Mann, R. S., Ali, J. G., Hermann, S. L., Tiwari, S., Pelzstelinski, K. S., Alborn, H. T., et al. (2012). Induced release of a plant-defense volatile ‘deceptively’ attracts insect vectors to plants infected with a bacterial pathogen. PLoS Pathog. 8:e1002610. doi: 10.1371/journal.ppat.1002610
Mann, R. S., Sidhu, J. S., and Butter, N. S. (2009). Settling preference of the whitefly Bemisia tabaci (Hemiptera: Aleyrodidae) on healthy versus cotton leaf curl virus-infected cotton plants. Int. J. Trop. Insect Sci. 29, 57–61. doi: 10.1017/S1742758409990142
Martini, X., Hoffmann, M., Coy, M. R., Stelinski, L. L., and Pelz-Stelinski, K. S. (2015). Infection of an insect vector with a bacterial plant pathogen increases its propensity for dispersal. PLoS ONE 10:e0129373. doi: 10.1371/journal.pone.0129373
Martini, X., Pelzstelinski, K. S., and Stelinski, L. L. (2014). Plant pathogen-induced volatiles attract parasitoids to increase parasitism of an insect vector. Front. Ecol. Evol. 2:8. doi: 10.3389/fevo.2014.00008
Mauck, K. E., De Moraes, C. M., and Mescher, M. C. (2010). Deceptive chemical signals induced by a plant virus attract insect vectors to inferior hosts. Proc. Natl. Acad. Sci. U.S.A. 107, 3600–3605. doi: 10.1073/pnas.0907191107
Michaud, J. P., Olsen, L. E., and Olsen, L. E. (2004). Suitability of Asian citrus psyllid, Diaphorina citri, as prey for ladybeetles. Biocontrol 49, 417–431.
Michereff, M. F. F., Laumann, R. A., Borges, M., Michereff-Filho, M., Diniz, I. R., Neto, A. L. F., et al. (2011). Volatiles mediating a plant-herbivore-natural enemy interaction in resistant and susceptible soybean cultivars. J. Chem. Ecol. 37, 273–285. doi: 10.1007/s10886-011-9917-4
Mutyambai, D. M., Bruce, T. J., Midega, C. A., Woodcock, C. M., Caulfield, J. C., Van Den Berg, J., et al. (2015). Responses of parasitoids to volatiles induced by Chilo partellus oviposition on teosinte, a wild ancestor of maize. J. Chem. Ecol. 41, 323–329. doi: 10.1007/s10886-015-0570-1
Ninkovic, V., Abassi, S. A., and Pettersson, J. (2001). The influence of aphid-induced plant volatiles on ladybird beetle searching behavior. Biol. Control 21, 191–195. doi: 10.1006/bcon.2001.0935
Pineda, A., Soler, R., Weldegergis, B. T., Shimwela, M. M., Jj, V. A. N. L., and Dicke, M. (2013). Non-pathogenic rhizobacteria interfere with the attraction of parasitoids to aphid-induced plant volatiles via jasmonic acid signalling. Plant Cell Environ. 36, 393–404. doi: 10.1111/j.1365-3040.2012.02581.x
Purcell, A. H. (2003). Insect vector relationships with procaryotic plant pathogens. Annu. Rev. Phytopathol. 20, 397–417. doi: 10.1146/annurev.py.20.090182.002145
Rogers, M. E., and Bates, P. A. (2007). Leishmania manipulation of sand fly feeding behavior results in enhanced transmission. PLoS Pathog. 3:e91. doi: 10.1371/journal.ppat.0030091
Roossinck, M. J. (2011). The good viruses: viral mutualistic symbioses. Nat. Rev. Microbiol. 9, 99–108. doi: 10.1038/nrmicro2491
Sullivan, B. T. (2005). Electrophysiological and behavioral responses of Dendroctonus frontalis (Coleoptera: Curculionidae) to volatiles isolated from conspecifics. J. Econ. Entomol. 98, 2067–2078. doi: 10.1603/0022-0493-98.6.2067
Takemoto, H., and Takabayashi, J. (2015). Parasitic wasps Aphidius ervi are more attracted to a blend of host-induced plant volatiles than to the independent compounds. J. Chem. Ecol. 41, 801–807. doi: 10.1007/s10886-015-0615-5
Tatineni, S., Sagaram, U. S., Gowda, S., Robertson, C. J., Dawson, W. O., Iwanami, T., et al. (2008). In planta distribution of ‘Candidatus Liberibacter asiaticus’ as revealed by polymerase chain reaction (PCR) and real-time PCR. Phytopathology 98, 592–599. doi: 10.1094/PHYTO-98-5-0592
Unsicker, S. B., Gershenzon, J., and Kollner, T. G. (2015). Beetle feeding induces a different volatile emission pattern from black poplar foliage than caterpillar herbivory. Plant Signal. Behav. 10:e987522. doi: 10.4161/15592324.2014.987522
Van Den Abbeele, J., Caljon, G., De Ridder, K., De Baetselier, P., and Coosemans, M. (2010). Trypanosoma brucei modifies the tsetse salivary composition, altering the fly feeding behavior that favors parasite transmission. PLoS Pathog. 6:e1000926. doi: 10.1371/journal.ppat.1000926
Wei, J. R., Yang, Z. Q., Dai, J. Q., and Du, J. W. (2007). [Tritrophic system of tree-trunkborer-insect natural enemy association]. Ying Yong Sheng Tai Xue Bao 18, 1125–1131.
Wheeler, G. S., Massey, L. M., and Southwell, I. A. (2002). Antipredator defense of biological control agent Oxyops vitiosa is mediated by plant volatiles sequestered from the host plant Melaleuca quinquenervia. J. Chem. Ecol. 28, 297–315. doi: 10.1023/A:1017982007812
Xu, H., He, X., Zheng, X., Yang, Y., Tian, J., and Lu, Z. (2014). Infection of rice plants by rice black streaked dwarf virus improves an egg parasitoid, Anagrus nilaparvatae (Hymenoptera: Mymaridae), of rice planthoppers. Environ. Entomol. 43, 1235–1239. doi: 10.1603/EN14044
Zhang, Y., Xie, Y., Xue, J., Peng, G., and Wang, X. (2009). Effect of volatile emissions, especially alpha-pinene, from persimmon trees infested by Japanese wax scales or treated with methyl jasmonate on recruitment of ladybeetle predators. Environ. Entomol. 38, 1439–1445. doi: 10.1603/022.038.0512
Keywords: ladybird beetle, volatiles, huanglongbing disease, insect vector, D-limonene, methyl salicylate
Citation: Lin Y, Lin S, Akutse KS, Hussain M and Wang L (2016) Diaphorina citri Induces Huanglongbing-Infected Citrus Plant Volatiles to Repel and Reduce the Performance of Propylaea japonica. Front. Plant Sci. 7:1969. doi: 10.3389/fpls.2016.01969
Received: 02 September 2016; Accepted: 12 December 2016;
Published: 26 December 2016.
Edited by:
Anton Hartmann, Helmholtz Zentrum München, GermanyReviewed by:
Bryan Bailey, United States Department of Agriculture, USAYi Li, Peking University, China
Copyright © 2016 Lin, Lin, Akutse, Hussain and Wang. This is an open-access article distributed under the terms of the Creative Commons Attribution License (CC BY). The use, distribution or reproduction in other forums is permitted, provided the original author(s) or licensor are credited and that the original publication in this journal is cited, in accordance with accepted academic practice. No use, distribution or reproduction is permitted which does not comply with these terms.
*Correspondence: Liande Wang, bGlhbmRlX3dhbmdAMTI2LmNvbQ==
†These authors have contributed equally to this work.