- Institute of Biochemical Plant Pathology, Helmholtz Zentrum München, German Research Center for Environmental Health, Neuherberg, Germany
Introduction
Physiological functions of nitric oxide (NO) in plants are often investigated by using chemical NO donors (Feelisch, 1998; Floryszak-Wieczorek et al., 2006) or fumigation of plants with NO gas (Huang et al., 2004; Palmieri et al., 2008; Vitor et al., 2013; Frungillo et al., 2014; Kasten et al., 2016; Krasuska et al., 2016; León et al., 2016; Melo et al., 2016). Treatment with gaseous NO has the advantage of being non-invasive and time- as well as cost effective. However, NO can react with air-oxygen resulting in the rise of toxic nitrogen dioxide (NO2; Groß et al., 2013; Heinrich et al., 2013). In human medicine NO2 formation is a well-established risk factor during NO inhalation as a cure against pulmonary diseases (Schedin et al., 1999; Sokol et al., 1999). The damaging effect of NO2 on plants has been frequently demonstrated (Wellburn, 1990; Xu et al., 2010; Liu et al., 2015; Kasten et al., 2016). In this current opinion article fumigation approaches were critically re-evaluated with a special focus on contaminations of NO-enriched air with NO2. Potential artifacts and data misinterpretation due to unintended co-treatment of plants with both gases are highlighted.
NO Fumigation
The chemistry of NO donors is rather complex. For instance, the commonly used NO donors sodium nitroprusside, S-nitroso-N-acetyl-penicillin, and S-nitrosoglutathione do not only release various NO derivatives but also cyanide ions, N-acetyl-penicillin, and oxidized glutathione, respectively (Feelisch, 1998). All of these compounds could evoke specific responses in plant cells and therefore have to be evaluated carefully by appropriate control treatments.
By contrast, NO fumigation loads the leaf mainly with NO and nitrite that also emerge under natural conditions e.g., during stress signaling (Ignarro et al., 1993; Groß et al., 2013). In the aqueous environment of a cell both nitrogen oxides are in equilibrium, with nitrous acid as an unstable intermediate. Nitrite is either converted to NO or is efficiently scavenged by the enzyme nitrite reductase. Accordingly, fumigation with 30 parts per million (ppm) NO for 1 h did not induce nitrite accumulation in leaves of Arabidopsis (Arabidopsis thaliana; Kasten et al., 2016). Gaseous NO enters leaves via the stomata although NO as a lipophilic molecule can also penetrate the cuticle to a certain extent (Wellburn, 1990). This facilitates the non-invasive treatment of many plants in parallel such as in the course of a mutant screen (Kasten et al., 2016). Another advantage of gaseous NO is the possibility of its continuous application over long time periods e.g., during pathogen infection (Vitor et al., 2013). In any case, it is necessary to determine the plants stomatal conductance under the prevailing experimental conditions. Hereby, an equal uptake of NO by the plants is ensured, especially when different mutant lines are compared (Kasten et al., 2016).
Fumigations of plants with high concentrations of NO are usually done either in closed- or flow-through chambers. A very basic set-up for a closed system would consist of a plant placed in a sealed container filled with (NO-free) air. Dependent on the volume of the headspace, an appropriate dose of NO (usually formulated in N2) would be injected into the chamber to adjust the desired NO concentration (Huang et al., 2004; Palmieri et al., 2008; León et al., 2016). Alternatively, a NO-releasing nitrite/hydrogen chloride solution could be placed in the container alongside the plants (Krasuska et al., 2016).
An advanced flow-through system for fumigation with NO is shown in Figure 1A (Kasten et al., 2016). Plants are placed into an air-tight fumigation chamber which is set to appropriate illumination and temperature conditions. A constant and manipulable air flow is realized by adjustable inlet and outlet air flows. Here, the air withdrawal (outlet air flow) from the chamber should exceed the air intake (inlet air flow) to cause slight negative pressure. By regulating the inlet and outlet air flow, the flow rate within the chamber can be manipulated. The air is charged with NO (here 15% NO in N2) upstream of the fumigation chamber at a distance that ensures proper mixing of the gases. NO volumes introduced to the system per time unit (e.g., ml/min) are regulated by a mass flow controller. The final NO concentration within the chamber is monitored by branching off the outlet flow to an AC32M NO analyzer (Environment S.A.) when it exits the chamber. This device is able to determine the amount (ppm) of NO, NO2, and the sum of both (NOx) within an air sample.
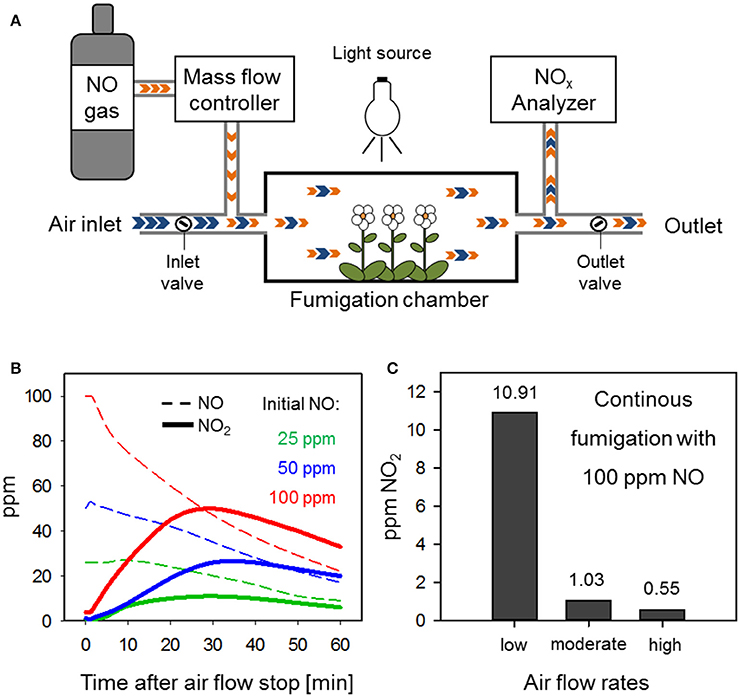
Figure 1. An adequate set-up of a flow-through system for NO fumigation prevents NO2 formation. (A) Schematic diagram of an advanced flow-through system for NO fumigation. Blue arrows, air; orange arrows, NO. (B) NO2 accumulation in a fumigation chamber with disrupted air flow. Different initial NO concentrations (25 ppm = green, 50 ppm = blue, 100 ppm = red) where adjusted within the chamber before stopping the air flow (t = 0 min) by shutting the inlet and outlet valves. Changes in NO (dotted lines) and NO2 (solid lines) were measured over 60 min via an NOx Analyzer. (C) NO2 formation in a flow-through system is dependent on air flow rates. The NO concentration within the chamber was set to 100 ppm NO under low, moderate, or high air flow rate conditions and NO2 accumulation was measured 30 min after the flow-through system was equilibrated.
NO2 Formation during NO Fumigation
A risk of fumigations with high levels of NO is the concomitant emergence of NO2. Autoxidation of NO in the presence of molecular oxygen (according to the formula 2NO + O2 → 2NO2) exhibits a second-order dependence on NO concentration and, therefore, is a slow reaction at low but a rapid reaction at high NO levels (Schedin et al., 1999; Sokol et al., 1999; Heinrich et al., 2013). Disruption of the air flow through the NO fumigation chamber shown in Figure 1A by closing the inlet and outlet valves caused the accumulation of NO2 within a few minutes (Figure 1B). At a starting concentration of 100 ppm NO the level of NO2 reached 15 ppm at 5 min and 27 ppm at 10 min after stopping the flow. Initial 25 ppm NO resulted in the formation of 2 and 7 ppm NO2 at 5 and 10 min after chamber closure (Figure 1B). In comparison, other researchers exposed Arabidopsis to 300 ppm NO for 5 min (León et al., 2016) or even 1250 ppm NO for 10 min (Huang et al., 2004; Palmieri et al., 2008) in closed containers. Under such conditions a significant build-up of NO2 can be expected.
In flow-through systems the air/O2/NO mixture is continuously exchanged which limits the reaction time for autoxidation of NO. Hence, it depends on the flow rate how much NO2 is formed in the system. The relationship between flow rate and NO2 formation is illustrated in Figure 1C. Here, a constant level of 100 ppm NO was accompanied by 1.03 ppm NO2 at moderate, 10.9 ppm NO2 at low but only 0.55 ppm NO2 at high air flux rates. The aforementioned NO2 levels were already formed 5 min after the start of fumigation and remained stable over the next 30 min (data not shown). Baseline NO2 levels of 0.05 ppm were measured in air that was not charged with NO (data not shown). These results suggest that previously applied NO concentrations between 50 and 150 ppm (Frungillo et al., 2014; Melo et al., 2016) could readily react with air-O2 leading to the formation of contaminating NO2 in the upper parts per billion (ppb) to low ppm range dependent on air flow. As compared to closed chamber systems, the rate of NO2 formation is rather low in flow-through systems. However, long-term exposure to ppb levels of NO2 can still have a profound impact on plants as discussed in the next chapter.
It is worth mentioning that long-term storage of commercial NO gas in pressurized cylinders can cause a substantial accumulation of NO2 due to NO conversion under high pressure to NO2 and N2O (Tsukahara et al., 2002). Such findings emphasize again the need for careful monitoring of NO2 during NO fumigations.
NO2 and NO Induce Distinct but Overlapping Responses
Recently, a highly controlled fumigation system was employed for comparing responses of Arabidopsis to ppm levels of NO and NO2 (Kasten et al., 2016). The nitrite content was strongly increased after NO2 but decreased after NO exposure. Fumigation for 1 h with 20 or 30 ppm NO2 triggered rapid lesion formation that was dependent on NO and hydrogen peroxide (Kasten et al., 2016). By contrast, neither 30 ppm NO for 1 h (Kasten et al., 2016) nor 60 ppm NO for 12 h (Vitor et al., 2013; Frungillo et al., 2014) or even 50–150 ppm NO for up to 72 h (Melo et al., 2016) led to any visible leaf damage in Arabidopsis and tomato (Solanum lycopersicum). Collectively, these results demonstrate that NO2 and NO have distinct chemistry and toxicity within the leaf.
Exposure of Arabidopsis plants to 300 ppm NO for 10 min in a closed chamber resulted in cell death, protein tyrosine nitration, oxylipin accumulation, and ascorbate depletion (León et al., 2016). Importantly, all of these effects were also observed after treatment with 30 ppm NO2 but not 30 ppm NO in a flow-through fumigation system (Kasten et al., 2016). Moreover, gaseous NO administered in a sealed vessel regulated a disparate set of genes than the NO donor NOR3 (NO-releasing agent-3; Palmieri et al., 2008). This further supports the assumption that at least some of the observed plant responses to NO fumigation in closed chamber systems were actually induced by the unnoticed rise of NO2 under such conditions.
Long-term fumigation of plants with NO2 can induce growth and leaf greening at ppb levels (Srivastava et al., 1994; Takahashi et al., 2014) or antioxidant defense, severe stress responses, and leaf damage at low ppm levels (Xu et al., 2010; Liu et al., 2015) dependent on the sensitivity of the plant species investigated. In this regard, it would be of interest if some of these plant responses that were also reported after long-term fumigations with NO were actually mediated by the concomitant formation of NO2. For instance, fumigation for 24–72 h with 50–150 ppm NO triggered the biosynthesis of chlorophyll and carotenoids in greening tomato seedlings (Melo et al., 2016). Previous work revealed an elevated chlorophyll and total nitrogen content in bean (Phaseolus vulgaris) grown for 5 days in an atmosphere containing 0.3 ppm NO2 (Srivastava et al., 1994). Other researchers found that carotenoid antioxidants efficiently scavenge NO2 in vitro and in vivo in human leucocytes (Cooney et al., 1994; Böhm et al., 1995). Thus, it seems feasible that chlorophyll and carotenoid biosynthesis are activated by NO2. The involvement of NO in this process remains to be elusive due to a lack of convincing evidence.
Conclusions
High (ppm) levels of NO efficiently react with air-O2 to give NO2. This must be considered when planning treatments of plants with gaseous NO. Particularly in closed chamber systems without air flow, NO2 strongly accumulates within a few minutes. Even in advanced flow-through systems high NO levels are often accompanied by NO2 concentrations known to trigger stress responses in plants. Actually, in many published studies it is inconclusive if NO2 rather than NO was the bioactive compound within the applied gas mixture. Therefore, the central message of the current opinion paper is a strong recommendation to monitor NO2 during NO fumigation. This would improve the interpretation and reproduction of published results from NO fumigation experiments. If NO and NO2 cannot be determined, the respective treatments should be referred to as “NOx fumigation,” and NO2 should be discussed as a potential inducer of the observed plant responses.
Author Contributions
DK and FG did the fumigation experiments. DK, JD, and FG wrote the manuscript.
Conflict of Interest Statement
The authors declare that the research was conducted in the absence of any commercial or financial relationships that could be construed as a potential conflict of interest.
References
Böhm, F., Tinkler, J. H., and Truscott, T. G. (1995). Carotenoids protect against cell membrane damage by the nitrogen dioxide radical. Nat. Med. 1, 98–99. doi: 10.1038/nm0295-98
Cooney, R. V., Harwood, P. J., Custer, L. J., and Franke, A. A. (1994). Light-mediated conversion of nitrogen dioxide to nitric oxide by carotenoids. Environ. Health Perspect. 102, 460–462. doi: 10.1289/ehp.94102460
Feelisch, M. (1998). The use of nitric oxide donors in pharmacological studies. Naunyn. Schmiedebergs. Arch. Pharmacol. 358, 113–122. doi: 10.1007/PL00005231
Floryszak-Wieczorek, J., Milczarek, G., Arasimowicz, M., and Ciszewski, A. (2006). Do nitric oxide donors mimic endogenous NO-related response in plants? Planta 224, 1363–1372. doi: 10.1007/s00425-006-0321-1
Frungillo, L., Skelly, M. J., Loake, G. J., Spoel, S. H., and Salgado, I. (2014). S-nitrosothiols regulate nitric oxide production and storage in plants through the nitrogen assimilation pathway. Nat. Commun. 5, 5401. doi: 10.1038/ncomms6401
Groß, F., Durner, J., and Gaupels, F. (2013). Nitric oxide, antioxidants and prooxidants in plant defence responses. Front. Plant Sci. 4:419. doi: 10.3389/fpls.2013.00419
Heinrich, T. A., da Silva, R. S., Miranda, K. M., Switzer, C. H., Wink, D. A., and Fukuto, J. M. (2013). Biological nitric oxide signalling: chemistry and terminology. Br. J. Pharmacol. 169, 1417–1429. doi: 10.1111/bph.12217
Huang, X., Stettmaier, K., Michel, C., Hutzler, P., Mueller, M. J., and Durner, J. (2004). Nitric oxide is induced by wounding and influences jasmonic acid signaling in Arabidopsis thaliana. Planta 218, 938–946. doi: 10.1007/s00425-003-1178-1
Ignarro, L. J., Fukuto, J. M., Griscavage, J. M., Rogers, N. E., and Byrns, R. E. (1993). Oxidation of nitric oxide in aqueous solution to nitrite but not nitrate: comparison with enzymatically formed nitric oxide from L-arginine. Proc. Natl. Acad. Sci. U.S.A. 90, 8103–8107. doi: 10.1073/pnas.90.17.8103
Kasten, D., Mithöfer, A., Georgii, E., Lang, H., Durner, J., and Gaupels, F. (2016). Nitrite is the driver, phytohormones are modulators while NO and H2O2 act as promoters of NO2-induced cell death. J. Exp. Bot. 67, 6337–6349. doi: 10.1093/jxb/erw401
Krasuska, U., Ciacka, K., and Gniazdowska, A. (2016). Nitric oxide-polyamines cross-talk during dormancy release and germination of apple embryos. Nitric Oxide. doi: 10.1016/j.niox.2016.11.003. [Epub ahead of print].
León, J., Costa, Á., and Castillo, M.-C. (2016). Nitric oxide triggers a transient metabolic reprogramming in Arabidopsis. Sci. Rep. 6:37945. doi: 10.1038/srep37945
Liu, X., Hou, F., Li, G., Sang, N., Liu, X., Hou, F., et al. (2015). Effects of nitrogen dioxide and its acid mist on reactive oxygen species production and antioxidant enzyme activity in Arabidopsis plants. J. Environ. Sci. 34, 93–99. doi: 10.1016/j.jes.2015.03.011
Melo, N. K. G., Bianchetti, R. E., Lira, B. S., Oliveira, P. M. R., Zuccarelli, R., Dias, D. L. O., et al. (2016). Nitric oxide, ethylene, and auxin cross talk mediates greening and plastid development in deetiolating tomato seedlings. Plant Physiol. 170, 2278–2294. doi: 10.1104/pp.16.00023
Palmieri, M. C., Sell, S., Huang, X., Scherf, M., Werner, T., Durner, J., et al. (2008). Nitric oxide-responsive genes and promoters in Arabidopsis thaliana: a bioinformatics approach. J. Exp. Bot. 59, 177–186. doi: 10.1093/jxb/erm345
Schedin, U., Frostell, C. G., and Gustafsson, L. E. (1999). Formation of nitrogen dioxide from nitric oxide and their measurement in clinically relevant circumstances. Br. J. Anaesth. 82, 182–192. doi: 10.1093/bja/82.2.182
Sokol, G. M., Van Meurs, K. P., Wright, L. L., Rivera, O., Thorn, W. J., Chu, P. M., et al. (1999). Nitrogen dioxide formation during inhaled nitric oxide therapy. Clin. Chem. 45, 382–387.
Srivastava, H. S., Ormrod, D. P., and Hale, B. A. (1994). Responses of greening bean seedling leaves to nitrogen dioxide and nutrient nitrate supply. Environ. Pollut. 86, 2–7. doi: 10.1016/0269-7491(94)90007-8
Takahashi, M., Furuhashi, T., Ishikawa, N., Horiguchi, G., Sakamoto, A., Tsukaya, H., et al. (2014). Nitrogen dioxide regulates organ growth by controlling cell proliferation and enlargement in Arabidopsis. New Phytol. 201, 1304–1315. doi: 10.1111/nph.12609
Tsukahara, H., Ishida, T., and Mayumi, M. (2002). Gas-phase oxidation and disproportionation of nitric oxide. Methods Enzymol. 359, 168–179. doi: 10.1016/S0076-6879(02)59181-3
Vitor, S. C., Duarte, G. T., Saviani, E. E., Vincentz, M. G. A., Oliveira, H. C., and Salgado, I. (2013). Nitrate reductase is required for the transcriptional modulation and bactericidal activity of nitric oxide during the defense response of Arabidopsis thaliana against Pseudomonas syringae. Planta 238, 475–486. doi: 10.1007/s00425-013-1906-0
Wellburn, A. R. (1990). Why are atmospheric oxides of nitrogen usually phytotoxic and not alternative fertilizers? New Phytol. 115, 395–429. doi: 10.1111/j.1469-8137.1990.tb00467.x
Keywords: nitric oxide, nitrogen dioxide, gas, fumigation, response
Citation: Kasten D, Durner J and Gaupels F (2017) Gas Alert: The NO2 Pitfall during NO Fumigation of Plants. Front. Plant Sci. 8:85. doi: 10.3389/fpls.2017.00085
Received: 19 December 2016; Accepted: 16 January 2017;
Published: 31 January 2017.
Edited by:
John Hancock, University of the West of England, UKReviewed by:
Marek Petrivalsky, Palacký University Olomouc, CzechiaDavid Wendehenne, University of Burgundy, France
Copyright © 2017 Kasten, Durner and Gaupels. This is an open-access article distributed under the terms of the Creative Commons Attribution License (CC BY). The use, distribution or reproduction in other forums is permitted, provided the original author(s) or licensor are credited and that the original publication in this journal is cited, in accordance with accepted academic practice. No use, distribution or reproduction is permitted which does not comply with these terms.
*Correspondence: Frank Gaupels, ZnJhbmsuZ2F1cGVsc0BoZWxtaG9sdHotbXVlbmNoZW4uZGU=