- 1Key Laboratory of Mountain Surface Processes and Ecological Regulation, Institute of Mountain Hazards and Environment, Chinese Academy of Sciences, Chengdu, China
- 2University of Chinese Academy of Sciences, Beijing, China
- 3Institute of Evolution and the Department of Evolutionary and Environmental Biology, University of Haifa, Haifa, Israel
With increasing altitude, solar UV-B radiation is enhanced. Based on the phenomenon of male-biased sex ratio of Populus cathayana Rehder in high altitude alpine area, we hypothesized that males have a faster and more sophisticated responsive mechanism to high UV-B radiation than that of females. Our previous studies have shown sexually different responses to high UV-B radiation were existed in P. cathayana at the morphological, physiological, and transcriptomic levels. However, the responses at the proteomic level remain unclear. In this study, an isobaric tag for relative and absolute quantification (iTRAQ)-based quantitative proteome analysis was performed in P. cathayana females and males. A total of 2,405 proteins were identified, with 331 proteins defined as differentially expressed proteins (DEPs). Among of these, 79 and 138 DEPs were decreased and 47 and 107 DEPs were increased under high solar UV-B radiation in females and males, respectively. A bioinformatics analysis categorized the common responsive proteins in the sexes as related to carbohydrate and energy metabolism, translation/transcription/post-transcriptional modification, photosynthesis, and redox reactions. The responsive proteins that showed differences in sex were mainly those involved in amino acid metabolism, stress response, and translation/transcription/post-transcriptional modification. This study provides proteomic profiles that poplars responding to solar UV-B radiation, and it also provides new insights into differentially sex-related responses to UV-B radiation.
Introduction
Natural levels of ultraviolet-B (UV-B: 280–320 nm) radiation act as an environmental regulatory factor in plants and control gene expression, growth and development (Jenkins, 2009; Hideg et al., 2013; Czégény et al., 2016). In alpine areas, with increasing altitude, UV-B radiation is enhanced. High doses of UV-B radiation negatively affect cellular/subcellular and macromolecular structures in vegetative plant tissues, including DNA, RNA, and proteins, modulate metabolites and reduce photosynthesis, biomass, and seed production (Jansen et al., 1998; Ries et al., 2000). Thus, plants must sense natural UV-B intensity and quickly respond to high levels of UV-B radiation as an acclimation process (Jenkins, 2009). A number of studies have reported that the responsive and adaptive mechanisms of plants to high altitude with high UV-B radiation differ among species, populations and genotypes (Ren et al., 2006, 2007; Wang et al., 2016). However, few studies have focused on the sexual differences in the responses.
In nature, the sex ratio of Populus is consistent with a 1:1 equilibrium in low-altitude habitats (1,600–2,000 m). However, at high altitude (2,000–2,600 m), there is a significant male-biased sex ratio (Wang et al., 2011; Lei et al., 2017). Therefore, we hypothesized that males are more resistant and have a more sophisticated response to high UV-B radiation than that of females, thus leading to a male-biased sex ratio at high altitude. To test this hypothesis, based on previous work, we exposed Populus cathayana Rehder to different UV-B radiation intensities and showed differences in the sexual responses to high UV-B radiation (Xu et al., 2010; Feng et al., 2014; Jiang et al., 2015). At the physiological level, males appear to be more resistant to high UV-B radiation. Observations of the organelle ultrastructure, photosynthetic rate and biomass accumulation indicated that these parameters were less affected by increased UV-B intensity in males than in females (Xu et al., 2010; Feng et al., 2014), and UV-B exposure enhanced bud break in male clones (Stromme et al., 2015). A comparative transcriptome analysis indicated that in P. cathayana males, sex-related transcriptional reprogramming occurred in certain important metabolic processes, and showed that sex-biased gene regulation under solar UV-B radiation was more responsive in males than in females (Jiang et al., 2015). At the metabolic level, UV-B radiation has been reported to mediate metabolic rearrangement in gray poplars (P. canescens syn.; Kaling et al., 2015), and increased UV radiation has been shown to promote the emission of more volatile organic compounds in females of European aspen (P. tremula L.) than in male plants (Randriamanana et al., 2015; Maja et al., 2016). However, sexual differences in the response to UV-B radiation at the proteomic level have not been reported. Because of the complicated and sophisticated modification and regulation of gene expression processes in plant cells, UV-B radiation may promote different patterns of change in the pathways at the mRNA, protein and metabolite levels. Therefore, determining how plants respond to high UV-B radiation at the leaf proteomic level will provide new insights into UV-B responsive processes and ultimately increase our understanding of the sex-ratio variation in high-altitude alpine areas. In this study, an iTRAQ-based quantitative proteome analysis was performed. The objectives were to (1) understand how UV-B radiation triggers overall proteomic changes and (2) assess whether males are more responsive than females to high UV-B radiation.
Materials and Methods
Plant Materials and Experimental Design
The material treatment and experimental design were as described by Jiang et al. (2015). Briefly, male and female cuttings were produced from F1 individuals derived from a controlled cross between two P. cathayana genotypes with divergent phenotypes. Sixty male and female cuttings were planted in 10 L plastic pots (one plant per pot) filled with 8 kg of homogenized soil and 8 g of slow-release fertilizer (13% N, 10% P, and 14% K) in a greenhouse located in the Wanglang National Nature Reserve (32°98′N and 104°08′E, Supplementary Figure 1). The altitude, mean annual rainfall, annual temperature, and annual summer temperature in the area are 2,600 m, 801 mm, 2.9 and 12.7°C, respectively.
Two UV-B radiation intensities were used: 2.1 and 8.5 kJ m−2 day−1 of average daily biologically effective UV-B (UV-BBE) radiation. In the low solar UV-B radiation treatment (2.1 kJ m−2 day−1), a polyester film (0.13 mm, Shanghai HiTeC Plastics Co. Ltd., Shanghai, China) was used to selectively exclude solar transmission, including UV-B and UV-C radiation. In the high solar UV-B radiation treatment (8.5 kJ m−2 day−1), 0.13 mm cellulose diacetate film (Qingzhou Yi-Run Agricultural Film Factory, Qingzhou, Shandong, China) was employed, which allowed the transmission of both UV-A and UV-B radiation (wavelength ≥290 nm). The spectral irradiance was weighted using the generalized plant response function normalized at 300 nm to obtain the UV-BBE radiation (Caldwell, 1971). The polyester and cellulose diacetate films were both replaced weekly. The spectral irradiance of the solar radiation at the plant level was determined using a USB2000 Fiber Optic Spectrometer (Ocean Optics, Inc., Dunedin, New Zealand) with a CC-3-UV Cosine Corrector. Prior to the measurements, the spectrometer was calibrated using a DH2000-CAL Radiometric Calibrated Deuterium Tungsten Source (210–1,050 nm, OceanOptics, Inc., Minneola, Florida, US). Figure 1 shows the high and low solar UV-B radiation intensities from June to October 2012.
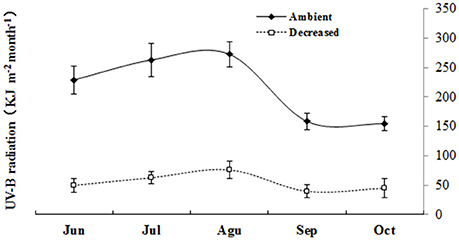
Figure 1. Monthly UV-B radiation accumulation of the two different UV-B radiation intensities in the experimental site during June to October.
The experimental layout was completely randomized with two factors: sex and UV-B radiation. Therefore, there were four treatments: (1) males exposed to low solar UV-B radiation (MC), (2) females exposed to low solar UV-B radiation (FC), (3) males exposed to high solar UV-B radiation (MU), and (4) females exposed to high solar UV-B radiation (FU). The duration of the experiments was 100 days (from 1 June to 10 August).
Gas Exchange and Chlorophyll Fluorescence Measurements
Five cuttings of the 4th fully expanded leaves from each sex per treatment were randomly selected for the gas exchange and chlorophyll fluorescence measurements. The net photosynthesis rate (Pn), stomatal conductance (gs), intercellular CO2concentration (Ci), and transpiration rate (E) were measured using a LI-COR 6400 portable photosynthesis measuring system (LI-COR, Lincoln, NE, USA) between the hours of 08:30 and 11:30. Prior to conducting the measurements, the samples were illuminated with saturated PPFD provided by the LED light source of the equipment for 10 min to achieve full photosynthetic induction. The saturated photosynthetic photon flux density (PPFD) was determined by preliminary experiments, and a carbon dioxide gas cylinder (LI-COR, Lincoln, NE, USA) was used to provide constant and stable CO2 during the measurements. A standard LI-COR leaf chamber (2 × 3 cm) was used, and the optimal parameters were as follows: leaf temperature of 25°C, leaf-air vapor pressure deficit of 1.5 ± 0.5 kPa, CO2 concentration of 400 ± 5 μmol mol−1, and photosynthetic photon flux density of 1,400 mmol m−2 s−1.
Chlorophyll fluorescence was measured using a PAM chlorophyll fluorometer (PAM 2100, Walz, Effeltrich, Germany). The leaf samples were placed in the dark for 30 min using an aluminum foil cover, followed by measurement of the minimal fluorescence (Fo) and the maximal fluorescence (Fm). Then, the leaves were illuminated with actinic light at an intensity of 250 μmol m−2 s−1. The actinic light was removed and the minimal fluorescence () and the maximal fluorescence () were measured by illuminating the leaves with far-red light for 3 s. Finally, a saturating white light with a pulse of 8,000 μmol m−2 s−1 was applied for 0.8 s to measure the Fm and values. The measurements were conducted from 07:30 to 09:00. The chlorophyll fluorescence kinetics parameters (Fv/Fm, maximum efficiency of PSII; Yield, the effective quantum yield of PSII; qP, photochemical quenching coefficient; qN, non-photochemical quenching coefficient) were measured and calculated as described by Vankooten and Snel (1990).
Leaf Transmission Electron Microscopy
For transmission electron microscopy analysis, small leaf sections (2 mm in length; avoiding the midrib) were selected from the middle position of the leaves. Five biological replicates were used. Leaf sections were fixed in 2.5% (v/v) glutaralpentanedial in 0.2 M phosphate buffered saline (pH 7.0) for 3 h at 22°C and post-fixed in 2% osmium tetroxide (OsO4) for 2 h. Then, the leaves were sequentially dehydrated in 30, 50, 70, and 90% acetone, respectively, and embedded in Epon 812 for ~2 h. Ultra-thin sections (80 nm) were sliced, stained with uranyl acetate and lead citrate, and mounted on copper grids for viewing in a H-600IV TEM (Hitachi, Tokyo, Japan) at an accelerating voltage of 60.0 kV.
Hydrolyzed Amino Acid Compound Measurements
Powdered dried leaves (0.1 g) were transferred into a 20-ml hydrolysis tube with 10 ml of 6 mol l−1 hydrochloric acid. The hydrolysis tube was sealed under vacuum and transferred to a constant-temperature drier at 110 ± 2°C for 22 h. After the tubes were removed from the dryer and cooled, the hydrolysis liquid was filtered, transferred into a 50-ml volumetric flask, and diluted with deionized water to scale. Then, 1 ml of the diluted hydrolysis liquid was withdrawn and dried in a vacuum drier at 40–50°C. The residue was dissolved in 1 ml of sodium citrate-hydrochloric acid buffer solution (pH 2.2) for analysis. The concentration and composition of amino acids were determined by an L-8800 automatic amino acid analyzer (Hitachi, Tokyo, Japan).
Protein Extraction, Digestion, and iTRAQ Labeling
Total proteins were extracted from three biological replicates (fresh leaves from tree cuttings) in each treatment using acetone methods as previously described (Zhang S. et al., 2016). The leaves were ground to a fine powder and suspended in 0.5 M triethylammonium bicarbonate (TEAB) buffer with 1 mM phenylmethyl sulfonyl fluoride and 0.1% SDS (w/v), and the samples were then sonicated for 5 min and centrifuged at 25,000 g for 20 min. The supernatant was transferred to another tube, 0.5 M TEAB buffer was added to the pellet to repeat the protein extraction, and the sample was centrifuged at 25,000 g for 20 min. The proteins in the combined supernatant were reduced (10 mM DTT, 56°C for 60 min), alkylated (55 mM iodoacetamide, dark room temperature for 45 min), precipitated by pre-cooled acetone at −20°C for 2 h, and then centrifuged at 25,000 g for 20 min. The pellet was washed twice with acetone, and the final pellet was dissolved in 0.5 M TEAB buffer with 0.1% SDS, sonicated for 15 min, and centrifuged at 25,000 g for 20 min. The supernatant was used for liquid digestion, and the protein concentration was determined using the Bradford assay.
The processed protein (100 μg) was removed from each sample solution and digested with Trypsin Gold (Promega, Madison, WI, USA) at a protein: trypsin ratio of 20:1 at 37°C for 12 h. After trypsin digestion, the peptides were dried by vacuum centrifugation, followed by reconstitution in 0.5 M TEAB and processing according to the manufacturer's protocol for 8-plex iTRAQ (Applied Biosystems). In this work, three biological replicates from each treatment and sex were analyzed. Thus, 12 samples were divided into two sets for iTRAQ. Males exposed to high (MU) and low (MC) solar UV-B radiation were in set 1, and females exposed to high (FU) and low (FC) solar UV-B radiation were in set 2. The MC1 samples were labeled in both sets as the control group. The detailed labeling conditions are listed in Table 1.
Separation of Peptides by Strong Cation Exchange (SCX) and ESI Mass Spectrometric Analysis
The labeled samples were fractionated using a LC-20AB high-performance liquid chromatography (HPLC) system (Shimadzu, Kyoto, Japan) and a 4.6 × 250 mm Ultremex strong cation exchange (SCX) column (Phenomenex, Torrance, CA, USA). After reconstitution of the labeled peptide mixtures with 4 ml of buffer A [10 mM NaH2PO4 in 25% acetonitrile (CAN, v/v), pH 2.6], SCX separation was performed at a flow rate of 1 ml min−1 using elution buffer A for 10 min, followed by a linear gradient of 5–60% buffer B (25 mM NaH2PO4, 1 M KCl in 25% ACN, pH 2.7) for 20 min and 100% buffer B for 2 min. The column was equilibrated with buffer A for 10 min prior to the next injection. The eluted fractions were monitored by measuring the absorbance at 214 nm, desalted with a Strata X C18 column (Phenomenex, Torrance, CA, USA), and vacuum dried.
Each fraction was resuspended in buffer A [5% ACN, 0.1% FA (v/v)] and centrifuged at 20,000 g for 10 min. The final concentration of peptide was ~0.5 μg μl−1. On average, 10 μl supernatant was loaded on a LC-20 AD nanoHPLC (Shimadzu, Kyoto, Japan) by an auto sampler onto a 2 cm C18 trap column. The peptides were eluted onto a 10-cm analytical C18 column (inner diameter 75 μm) packed in-house. The samples were loaded at 8 μl min−1 for 4 min. Then, a 35-min gradient was run at 300 nl min−1 from 2 to 35% B (95% ACN, 0.1% FA), followed by a 5-min linear gradient to 60% (v/v), a 2-min linear gradient to 80%, maintenance at 80% B for 4 min, and 5% for 1 min.
MS analysis was performed using a Triple TOF 5600 System (AB SCIEX, Concord, ON) fitted with a Nanospray III source (AB SCIEX, Concord, ON) and a pulled quartz tip as the emitter (New Objectives, Woburn, MA). The data were acquired using an ion spray voltage of 2.5 kV, curtain gas of 30 psi, nebulizer gas of 15 psi, and an interface heater temperature of 150°C. The MS was operated with an RP ≥30,000 FWHM for the TOF MS scans. For the IDA, survey scans were acquired in 250 ms, and as many as 30 product ion scans were collected if a threshold of 120 counts per second (counts s−1) was exceeded at a 2+ to 5+ charge state. The total cycle time was fixed to 3.3 s. The Q2 transmission window was 100 Da for 100%. Four time bins were summed for each scan at a pulse frequency value of 11 kHz via monitoring of the 40 GHz multichannel TDC detector with a four-anode channel detection ion. A sweeping collision energy setting of 35 ± 5 eV coupled with iTRAQ-adjusted rolling collision energy was applied to all pre-cursor ions for collision-induced dissociation. In this experiment, full MS scans were acquired in a mass range of m/z 350 to 1,500 in a scan time of 250 ms. Fragment ion spectra were acquired in the mass range of m/z 100–2,000 and excluded for further fragmentation over 15 s.
Protein Identification and Quantification
The raw data files were converted into MGF files and then searched against a local poplar database (ftp://ftp.jgi-psf.org/pub/compgen/phytozome/v9.0/Ptrichocarpa/annotation/) using the Mascot server (version 2.3.02, Matrix Science, Boston, MA). The data were downloaded from Populus trichocarpa V 3.0 (73013 protein-coding transcripts; https://phytozome.jgi.doe.gov/pz/portal.html#!info?alias=Org_Ptrichocarpa). The Mascot search settings were as follows: one missed cleavage site by trypsin was allowed with a fixed modification of carbamidomethyl (C), iTRAQ8plex (N-term) and iTRAQ8plex (K) and variable modifications of Gln- > pyro-Glu (N-term Q), oxidation (M), and iTRAQ8plex (Y). The fragment mass tolerance was ±0.1 Da, and the peptide mass tolerance was ±0.05 Da. The max missed cleavages value was 1. The specified false discovery rate (FDR) was 1% when automatic searching using software of pFind 2.0 (Institute of Computing Technology, Chinese Academy of Sciences, Beijing, China). The detailed information of pFind can be referenced to Wang et al. (2007) and Li et al. (2005). For protein identification, at least 2 unique peptides identified were considered. For proteins that were not identified in the local poplar database, SwissProt database (August, 2016, including 553,474 sequences) was used for re-searching using the same parameter settings. The ratio of treatments to controls over ±1.5 and P ≤ 0.05 (t-test) were considered to indicate the differentially expressed proteins (DEPs). The proteins identified within a family were grouped in the Mascot protein family summary. The sample labeled 113 (MC1) was used as the reference (the control group) based on the weighted average of the intensity of report ions in each identified peptide. The final ratios of protein were then normalized by the median average protein ratio for the mixes of different labeled samples. This normalization corrects the systematic error. For each sex, only DEPs identified in all biological replicates (including the three controls and three UV-B treatments) were further used in the analysis of sexually differential expression. DEPs that were detected in both sexes were defined as commonly changed DEPs, and those detected in only one gender were defined as sexual DEPs. All of the DEPs were functionally categorized using Blast2go, a web-based bioinformatics tool that groups proteins based on their GO annotations. According to the molecular functions listed on the UniProt and Gene Ontology websites, the DEPs were classified into different functional categories in both sexes.
Statistical Analysis
For the physiological parameters, the effects of UV-B radiation, sex and their interaction were analyzed by an analysis of variance (ANOVA) using a randomized complete-block design in SPSS 16.0 (SPSS, Chicago, IL, USA). Prior to the analysis, the data were checked for normality and homogeneity of variance. Post-hoc comparisons were tested using Tukey's test at a significance level of P < 0.05. The mean values and standard errors were determined for each variable. For protein identification, at least 2 unique peptides identified were considered. The ratio of treatments to controls over ±1.5 and P ≤ 0.05 were considered to indicate the DEPs.
Results
Gas Exchange and Chlorophyll Fluorescence Parameter Changes in Both Sexes
Overall, compared with the low solar UV-B radiation treatment, the high solar UV-B radiation significantly reduced the Pn, gs, Ci, E, qP, and chlorophyll fluorescence Yield values in females but not in males. For all parameters, the interactive effects of sex and UV-B were significant (Table 2). Additionally, the gs, Ci, E, qP, and Yield values showed significant sexual differences under the high UV-B radiation treatment but not under the low UV-B radiation treatment.
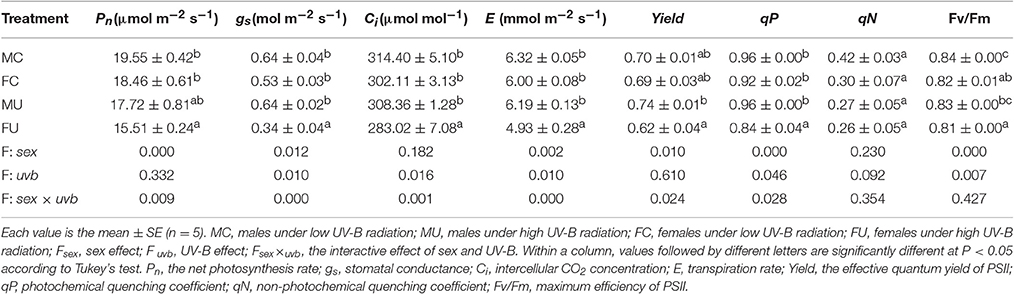
Table 2. Gas exchange and chlorophyll fluorescence parameters in female and male P. cathayana cuttings as affected by solar UV-B radiation.
Ultrastructural Morphological Changes in Both Sexes
As shown in Figure 2, under low UV-B radiation, the chloroplasts of both sexes had a typical ellipsoidal shape and typical structure, and 15–30 well-arranged thylakoids (on average 20) were included in each granum (Figures 2A,C). Under high UV-B radiation, the number of starch grains was lower (Figures 2B,D) than that in the individuals under the low UV-B conditions, and additional plastoglobuli were present in the chloroplasts. The accumulation of plastoglobuli is observed as lipid droplets derived from thylakoid degradation, and it was greater in females (Figure 2D) than in males (Figure 2B). However, the high intensity of UV-B radiation did not cause significant changes in the chloroplast granum number, mitochondria, or cellular membrane in the ultra-structures of the mesophyll cells.
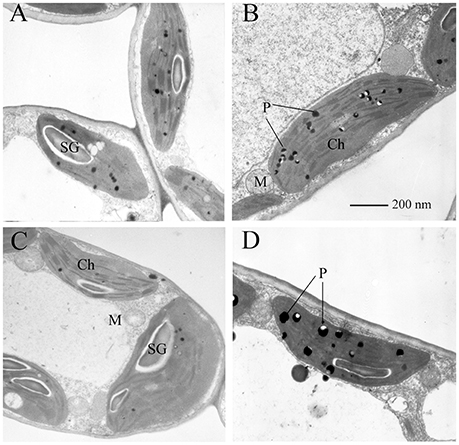
Figure 2. Transmission electron micrographs of the mesophyll cells of P. cathayana males and females exposed to UV-B radiation. (A) males under low UV-B radiation; (B) males under high UV-B radiation; (C) females under low UV-B radiation; (D) females under high UV-B radiation; Ch, chloroplast; M, mitochondrion; P, plastoglobule; SG, starch grain.
Hydrolyzed Amino Acid Compound Changes in Both Sexes
In this study, a total of 17 types of amino acids were detected in both males and females (Table 3). High UV-B radiation significantly decreased the aspartic acid, leucine, arginine, and proline concentrations, whereas cystine exhibited smaller changes in both sexes. Interestingly, the other amino acids were significantly decreased in males but not in females under high UV-B radiation.
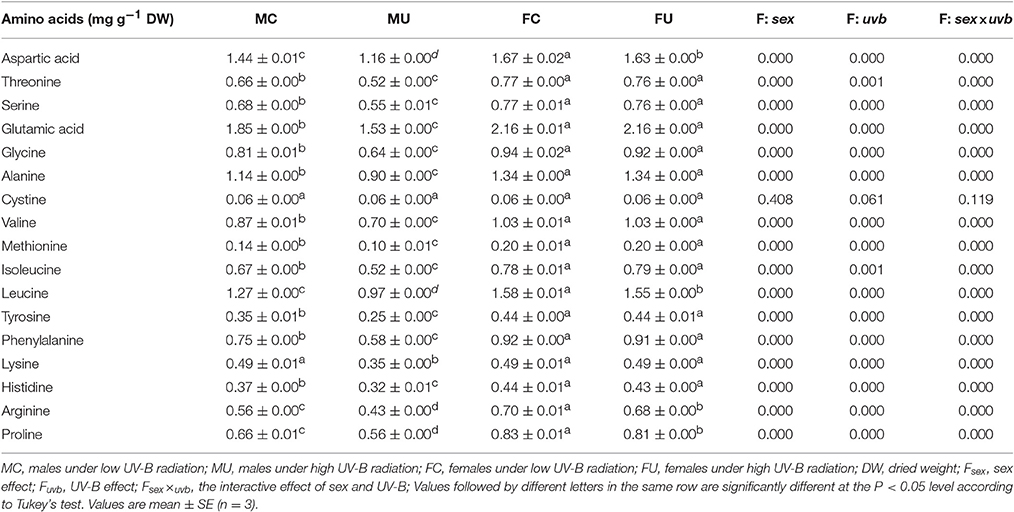
Table 3. The hydrolyzed amino acid concentrations in P. cathayana female and male leaves as affected by solar UV-B radiation.
Protein Expression Profiles in Response to Solar UV-B Radiation in Both Sexes
To investigate the changes in the protein profiles in response to solar UV-B radiation, proteins were extracted from the poplar leaves and analyzed using an iTRAQ-based shotgun proteomics strategy. Using the Mascot search engine, a total of 6,091 peptides from the trypsin-digested proteins were identified; of these, 5,210 peptides were unique. A total of 2,405 proteins were identified. The protein information is listed in Supplementary Table 1. According to the selection criteria of expression abundance ≥1.5 (or ≤ 0.67) and P ≤ 0.05, 331 proteins were defined as DEPs. In females, high UV-B radiation was associated with the decreased abundance of 79 proteins and the increased abundance of 47 proteins (Figure 3 and Supplementary Table 2). In males, high UV-B radiation was associated with the decreased abundance of 138 proteins and the increased abundance of 107 proteins (Figure 3 and Supplementary Table 3). According to the identified protein IDs, decreases and increases were observed in 22 and 15 proteins common in both sexes, respectively (Figure 3 and Table 4).
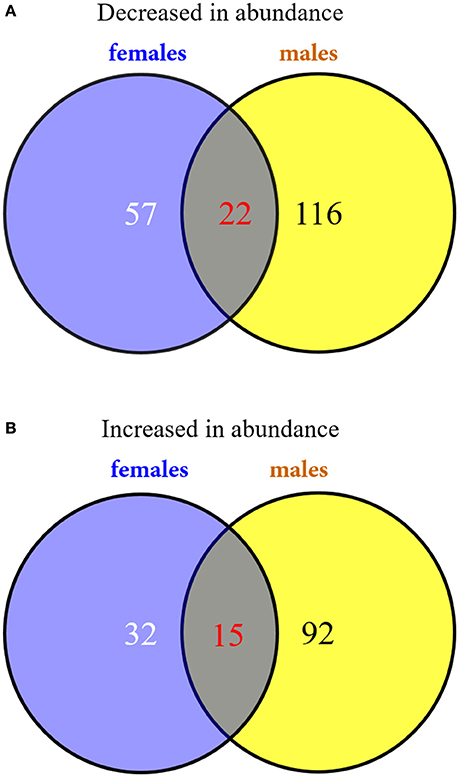
Figure 3. Venn diagrams of the DEPs in P. cathayana males and females under high solar UV-B radiation. (A) the down-regulated DEPs in abundance; (B) the up-regulated DEPs in abundance.
Functional Classification of DEPs
All of the DEPs were functionally categorized using Blast2go, a web-based bioinformatics tool that groups proteins based on their GO annotations. According to the molecular functions listed on the UniProt and Gene Ontology websites, the DEPs were classified into 11 functional categories in both sexes (Figure 4). Among these, the common DEPs in both sexes were involved in carbohydrate and energy metabolism, translation/transcription/post-transcriptional modification, photosynthesis, and redox reactions (Table 4, Supplementary Tables 2, 3 and Figure 5). The sex-specific DEPs were mainly involved in translation/transcription/post-transcriptional modification, amino acid metabolism, and stress responses (Supplementary Tables 2, 3). Additionally, a greater number of DEPs was observed in males than in females in all the categories, particularly in the translation/transcription/post-transcriptional modification proteins.
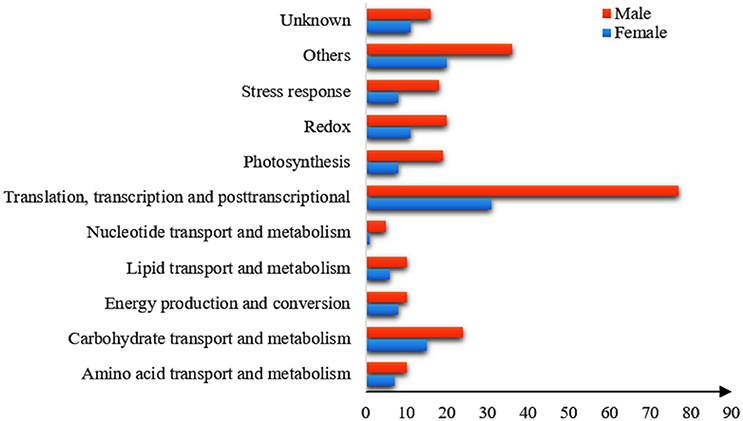
Figure 4. Functional classification of the DEPs in males and females under the high solar UV-B radiation. X-axis presents the number of DEPs. Y-axis presents functional classification of the DEPs.
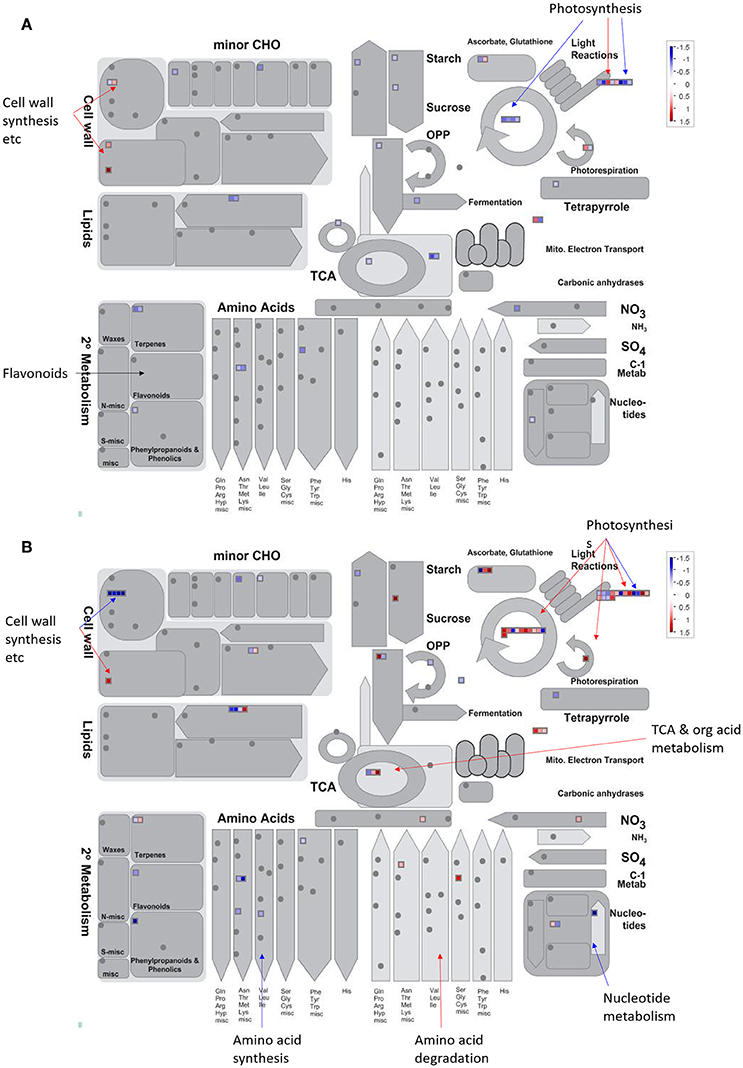
Figure 5. An overview of the DEPs assigned to “metabolism” by MapMan. Changes of proteomic level in (A) males and (B) females under solar UV-B radiation. Data were processed according to the standard protocol of MapMan software. Red indicates DEPs significantly up-regulated whereas blue indicates down-regulated in response to solar UV-B radiation (see color scale).
Discussion
Common Changes between the Sexes in the Proteome in Response to UV-B Radiation
The commonly changed proteins in both sexes under high UV-B radiation were mainly involved in translation/transcription/post-transcriptional modification, carbohydrate and energy metabolism, photosynthesis, and redox reactions (Supplementary Tables 2, 3). Of these, RNA-binding proteins play roles in the transport, stability, and translation of mRNA (Sahi et al., 2007). Several RNA-binding proteins (e.g., glycine-rich RNA-binding protein and glycine-rich RNA-binding protein 2) were up-regulated by UV-B radiation. Glycine-rich RNA-binding protein (GRP) is involved in pre-mRNA splicing, nucleocytoplasmic mRNA transport, mRNA stability and decay, and translation (Dreyfuss et al., 1993; Simpson and Filipowicz, 1996). It plays an important role in the process of regulating gene expression, principally at the post-transcriptional level. Glycine-rich RNA-binding protein 2 may play a role in RNA transcription, which is likely because of its RNA chaperone activity during the stress adaptation process (Kim et al., 2007; Zhang et al., 2012). Numerous peptidyl-prolyl cis-trans isomerases were up-regulated in both sexes, particularly in males under UV-B radiation. The stromal 70 kDa heat shock-related proteins were also increased in abundance in both sexes. Heat shock proteins perform a chaperone function by stabilizing new proteins to ensure correct folding or by aiding the refolding of damaged proteins. The up-regulation of peptidyl-prolyl cis-trans isomerases and stromal 70 kDa heat shock-related proteins may be an adaption to avoiding protein misfolding and promoting the formation of disulfide bonds in nascent proteins under stressed conditions (Marshall and Keegstra, 1992; Schönbrunner and Schmid, 1992). Additionally, in conjunction with rRNA, ribosomal proteins compose the ribosomal subunits involved in the cellular process of translation. The evidence showed that UV-B radiation damaged ribosomes by crosslinking cytosolic and chloroplast ribosomal proteins to RNA. Ribosomal damage that accumulated during the initial stage of UV-B radiation correlated with a progressive decrease in new protein production; however, de novo synthesis of some ribosome proteins increases in the later stage of UV-B radiation (Casati and Walbot, 2004). In our study, a set of cytosolic and chloroplast ribosomal proteins (40S and 60S) was down-regulated in both sexes under UV-B radiation. This decrease may be attributable to ribosome rearrangement. Thus, these changes may enhance the efficiency of protein translation under UV-B stress. Ribosome rearrangement has been reported in many stress studies and is thought to be essential in maintaining efficient translation under stress. In addition, the down-regulation of elongation factors (e.g., elongation factor 1-alpha, beta and Ts, and elongation factor 2) that participate in protein synthesis in the cell cycle and facilitate translational elongation in the ribosome suggests that protein synthesis was hindered under high UV-B radiation. Furthermore, UV-B radiation might change protein phosphorylation levels and accelerate the degradation of misfolded proteins as suggested by the observation that LRR receptor-like serine/threonine-protein kinase At5g45780 was down-regulated and several proteases (e.g., thiol protease aleurain and protease Do-like 8) were up-regulated. The former catalyzes protein phosphorylation at serine/threonine (Gao et al., 2010), and the latter plays a role in proteolysis and leads to the mobilization of nitrogen under stressed conditions. Therefore, the results indicated that UV-B radiation might have a complex effect on gene expression regulation in P. cathayana, and a large variety of changes in gene expression processes, particularly post-transcriptional regulation and post-translational modifications, occurs in poplars. However, further study is needed to test this hypothesis.
Under high UV-B radiation conditions, many biological processes, including ion transport, ROS scavenging, and UV-absorbing compound synthesis, require extra energy. ATP is produced via light-driven photosynthetic reactions in the thylakoid membrane, and it is primarily utilized in carbon fixation reactions (Yin et al., 2010). In this study, ATP synthase subunits were down-regulated in females but up-regulated in males, indicating that more ATP might be produced in males than in females under UV-B radiation. However, several ATP carrier- and exchange-related proteins were decreased in abundance in both sexes, e.g., 3-isopropylmalate dehydrogenase, ATPase and ADP/ATP carrier proteins, suggesting that energy metabolism was hindered by high UV-B radiation in both sexes. However, this result was contrary to other reports that these proteins were up-regulated in plants suffering from short-term UV-B radiation, such as Lonicera japonica and Arabidopsis (Yin et al., 2010; Zhang et al., 2013). One reason might be that these changes in the proteins involved in energy metabolism varied with plant species, stress duration and development. In another study, ATP was produced by light-driven photosynthetic reactions and carbohydrate metabolism. However, in this study, several key enzymes involved in these pathways were down-regulated by UV-B radiation, e.g., PSI, PSII, cytochrome b6, glyceraldehyde-3-phosphate dehydrogenase and glucan endo-1,3-beta-glucosidase. Additionally, the high UV-B treatment lasted for a long time (one growth season), and excessive energy production will lead to accumulation of ROS and plant cell damage. Therefore, the decrease in ATP production may be an adaption to high UV-B conditions in nature, especially under higher intensities.
The photosynthetic system and subcellular organelles are vulnerable to UV-B damage. Previous studies have shown that high UV-B radiation lowers chlorophyll pigment content, damages the photosystem, and distorts chloroplast structure in P. cathayana, thereby leading to a decline in Pn (Ren et al., 2006, 2010; Xu et al., 2010; Feng et al., 2014). The decrease in chlorophyll content also has a very distinct effect on chlorophyll-binding proteins and protein complexes, thus further hindering light capture and delivery (Varsano et al., 2006; Zhang S. et al., 2016). In this study, the abundance of many photosynthetic proteins, especially light-dependent reaction proteins, was changed in both sexes (Figure 5). For example, many chlorophyll a/b binding proteins (CABs) and PSI and PSII reaction center proteins were lower in abundance under solar UV-B radiation, whereas some thylakoid lumen proteins and PsbP domain-containing proteins were increased. CABs are photosynthetic proteins that are typically down-regulated in plants in response to enhanced UV irradiation (Taylor et al., 1996; Zhang et al., 2013). These proteins are responsible for binding to chlorophyll pigment molecules in light-harvesting complexes and transferring excitation energy during photosynthesis (Bassi et al., 1990; Jespersen and Huang, 2015). In plants, the degradation of the photosystem is linked to the remodeling of light-harvesting antenna, e.g., chlorophyll a/b binding proteins (Moseley et al., 2002; Zhang S. et al., 2016). The lower expression of photosystem reaction center proteins implies that UV-B radiation causes electron transport discordance and redox homeostasis imbalance between PSI and PSII (Joshi et al., 2011). The cytochrome b6f complex, which mediates electron transport between PSI and PSII, is also a thylakoid-bound protein that is an essential component of the electron transport chain and serves as the final electron acceptor in the cyclical electron flow pathway of photosynthetic light reactions (Munekaga et al., 2004; Cramer et al., 2006; Zhang Y. et al., 2016). The PsbP and thylakoid lumen proteins were up-regulated in both sexes, indicating that solar UV-B radiation may result in instability of the photosystem (Jiang et al., 2015). PsbP proteins are essential for the regulation and stabilization of PSII (Ifuku et al., 2005). Recent reports have indicated that thylakoid lumen proteins play roles in regulating thylakoid biogenesis and the activity and turnover of photosynthetic protein complexes, especially the PSII and NAD(P)H dehydrogenase-like complexes. However, the function of the majority of luminal proteins in Populus remains unknown (Järvi et al., 2013; Zhang S. et al., 2016). Additionally, the abundance of OEE and Calvin cycle proteins was increased in males only, indicating sexual differences in the response of the photosynthetic system (Figure 5). OEE and Calvin cycle proteins participate in the light-dependent and light-independent reactions of photosynthesis. The up-regulation of these proteins might increase the photosynthetic capacity of males, which could partially explain the higher photosynthetic rate in males than in females under high solar UV-B radiation.
Sex-Specific Changes in the Proteome under UV-B Radiation
Although a common set of proteins was changed in both sexes, there were many of sex-specific DEPs (Figure 5). For example, the 26S proteasome non-ATPase regulatory subunit 1 was up-regulated in females, whereas other proteasomes and their subunits were up-regulated in males (e.g., cysteine proteinase 2 and 3, proteasome subunit beta type-2-A and -6, and subtilisin-like proteases). Calnexin homolog 1 and luminal-binding protein 5, which control the apparatus of incorrectly folded proteins in the endoplasmic reticulum, were down-regulated in males only. The sex-specific changes in these proteins may be attributed to two reasons: (1) sex-specific gene expression and regulation may lead to sex-specific protein abundance and (2) limited proteomic detection technology (e.g., the number of identified peptides per proteins is still low) increases the difficulty of identifying all of the protein profiles in the two sexes, especially for the alkaline proteins and membrane proteins at low abundance.
A considerable number of proteomic profiles indicated that more proteins involved in amino acid metabolism were up-regulated to a greater degree in males than in females under high UV-B radiation (Figure 5). This result is consistent with that of the transcript profiles (Jiang et al., 2015). Alanine aminotransferase 2 and glycine dehydrogenase [decarboxylating] participate in the degradation of L-alanine and glycine, and isoaspartyl peptidase/L-asparaginase 1 are involved in aspartate production. The up-regulation of these proteins indicates that alanine, glycine, and aspartate catabolic processes changed more in males upon UV-B radiation. The changes in hydrolyzed amino acid compound observed in this study also confirmed this phenomenon. Additionally, several lyases, e.g., glycerate dehydrogenase and LL-diaminopimelate aminotransferase, were down-regulated in females, and 3-dehydroquinate synthase, diaminopimelate decarboxylase 2 and N-carbamoyl-L-amino acid hydrolase were down-regulated in males. These lyases catalyze the different chemical reactions that utilize and produce special amino acids. Glycerate dehydrogenase participates in glycine, serine, and threonine metabolism and glyoxylate and dicarboxylate metabolism, and their down-regulation only in females indicates that these metabolic processes are regulated more stringently in females than in males. Our results provide further evidence that the female strategy of gene regulation differs from that of males at the proteomic level.
For the females, except for 2 proteins that changed in common in both sexes, most of the stress responsive proteins were down-regulated. For example, 12-oxophytodienoate reductase 3 catalyzes the biosynthesis of jasmonic acid (JA), which is important as a gene regulator for development and defense (Schaller et al., 2000; Tani et al., 2008). 1-aminocyclopropane-1-carboxylate oxidase 3 (ACO3) is an essential gene during plant senescence and development and may play a role in ethylene synthesis (Hunter et al., 1999). The auto-inhibited plasma membrane P-type H+ ATPases were activated by 14-3-3 proteins (Jahn et al., 2002). Additionally, actin-7, phosphate carrier protein and protein canopy-1 were down-regulated in females, and several proteins were also down-regulated in males including allene oxide cyclase 3 (AOC3), probable linoleate 9S-lipoxygenase 5 (LOX1.5) and two protein aspartic proteases in guard cell 1 (ASPG1). AOC3 is involved in the production of 12-oxo-phytodienoic acid (OPDA), a pre-cursor of JA. ASPG1 play essential roles in restricting bacterial growth, plant defense and drought avoidance (Willmann et al., 2011; Yao et al., 2012). Plant lipoxygenases may be involved in a number of diverse aspects of plant physiology, including growth and development, pest resistance, senescence, and the wound response (Kolomiets et al., 2000). The down-regulation of these proteins indicates that higher intensity UV-B radiation may induce a sex-specific decrease in the defensive ability of poplars. Interestingly, several stress-related proteins were up-regulated only in males, such as heavy metal-associated isoprenylated plant protein 26 (HIPP26), heme-binding-like protein At3g10130, pathogenesis-related protein 1A (PRP1A), and stable protein 1 (SP1). PRP1A are plant disease-resistance proteins and play roles in plant defense. HIPP26 and At3g10130 are metal-binding proteins and play roles in metal detoxification (Barth et al., 2009; Vanhee et al., 2011). High UV-B radiation resulted in an increase in these stress-response proteins in P. cathayana males but not in females. Thus, these proteins might play roles in enhancing UV-B resistance to a greater extent in P. cathayana males than females.
Our previous parallel studies have shown that at the physiological level, solar UV-B radiation significantly decreases the photosynthetic capacity of P. cathayana, with a greater decrease in females than males (Xu et al., 2010; Feng et al., 2014). At the transcriptome level, solar UV-B radiation induced changes in the expression of a set of genes, and sexual differences were observed. For example, many differentially expressed genes involved in amino acid metabolism are up-regulated in P. cathayana males but down-regulated in females (Jiang et al., 2015). However, a full understanding of poplar regulatory mechanisms under UV-B solar radiation remains to be attained. Sex-related adaption to solar UV-B radiation in P. cathayana is such a sophisticated process that we cannot identify all of the regulating pathways that play dominant roles. For instance, flavonoids were the most frequently reported secondary metabolites responding to UV-B radiation in plants (Bassman, 2004; Schreiner et al., 2012). Our previous transcript study detected two flavonoid genes in P. cathayana males (Jiang et al., 2015). However, we did not identify any protein related to flavonoid metabolism at the proteomic level in this study (Figure 5). Numerous studies have indicated that the correlation between protein abundance and mRNA transcript levels is limited in woody plants (Lippert et al., 2009; Zhang et al., 2010; Dong et al., 2016). It is widely assumed that post-transcriptional regulation and post-translational modification play important roles in translational efficiency, which leads to discordance between mRNA expression and protein abundance. Therefore, to further understand the evolutionary and adaption mechanisms of poplars to UV-B radiation, differences in the translation, post-transcriptional modification, and metabolism responses should be investigated.
Conclusions
Our results showed that P. cathayana females and males under high UV-B radiation exhibited common and sex-specific responses in terms of physiology and proteome dynamics. These common responsive proteins in both sexes were mainly categorized into carbohydrate and energy metabolism, translation/transcription/post-transcriptional modification, photosynthesis and redox reactions. The sexually different responsive proteins were involved in translation/transcription/post-transcriptional modification, amino acid metabolism, and stress responses. P. cathayana males showed a greater scope of protein changes than females under high UV-B radiation, suggesting that males are more sophisticated in their response to UV-B radiation compared with females. Although our study is limited by the low number of replicates (three replicates each treatment), and the results may vary depending on plant growth conditions (e.g., temperature, condensation and available light spectrum), it reveals some dynamic and sex-specific changes in poplars at the proteomic level in response to high UV-B radiation and provides new insights into the mechanism of the male-biased sex ratio at high altitude, thus complementing existing knowledge.
Author Contributions
YunxiangZ was responsible for the analysis of proteomic data and parts of manuscript writing. LF did the field work, and she was also responsible for obtaining proteomic data and analysis of physiological parameters. HJ and YuanbinZ did much of the field work and the measurements. SZ had the initial research idea, was responsible for parts of manuscript writing and acquired the funding for the project which was done in his laboratory.
Funding
This work was supported by the Excellent Young Scientist Program of the National Natural Science Foundation of China (NO. 31322014) and the National Natural Science Foundation of China (No. 31170572 and 31300512).
Conflict of Interest Statement
The authors declare that the research was conducted in the absence of any commercial or financial relationships that could be construed as a potential conflict of interest.
Supplementary Material
The Supplementary Material for this article can be found online at: http://journal.frontiersin.org/article/10.3389/fpls.2017.00320/full#supplementary-material
Supplementary Figure 1. The experimental site and greenhouses used for UV-B filter (A), and the morphology of female plants under low UV-B radiation (B).
Supplementary Table 1. The detailed information of total identified proteins in P. cathayana males and females under UV-B radiation.
Supplementary Table 2. All the identified DEPs in P. cathayana females caused by UV-B radiation.
Supplementary Table 3. All the identified DEPs in P. cathayana males caused by UV-B radiation.
References
Barth, O., Vogt, S., Uhlemann, R., Zschiesche, W., and Humbeck, K. (2009). Stress induced and nuclear localized HIPP26 from Arabidopsis thaliana interacts via its heavy metal associated domain with the drought stress related zinc finger transcription factor ATHB29. Plant Mol. Biol. 69, 213–226. doi: 10.1007/s11103-008-9419-0
Bassi, R., Rigoni, F., and Giacometti, G. M. (1990). Chlorophyll binding-proteins with antenna function in higher-plants and green-algae. Photochem. Photobiol. 52, 1187–1206. doi: 10.1111/j.1751-1097.1990.tb08457.x
Bassman, J. H. (2004). Ecosystem consequences of enhanced solar ultraviolet radiation: secondary plant metabolites as mediators of multiple trophic interactions in terrestrial plant communities. Photochem. Photobiol. 79, 382–398. doi: 10.1562/SI-03-24.1
Caldwell, M. M. (1971). “Solar ultraviolet radiation and growth and development of higher plants,” in Photophysiology, ed A. C. Giese (New York, NY: Academic Press), 131–177.
Casati, P., and Walbot, V. (2004). Crosslinking of ribosomal proteins to RNA in maize ribosomes by UV-B and its effects on translation. Plant Physiol. 136, 3319–3332. doi: 10.1104/pp.104.047043
Cramer, W. A., Zhang, H., Yan, J., Kurisu, G., and Smith, J. L. (2006). Transmembrane traffic in the cytochrome b6f complex. Annu. Rev. Biochem. 75, 769–790. doi: 10.1146/annurev.biochem.75.103004.142756
Czégény, G., Mátai, A., and Hideg, É. (2016). UV-B effects on leaves-Oxidative stress and acclimation in controlled environments. Plant Sci. 248, 57–63. doi: 10.1016/j.plantsci.2016.04.013
Dong, Y. P., Deng, M. J., Zhao, Z. L., and Fan, G. Q. (2016). Quantitative proteomic and transcriptomic study on autotetraploid Paulownia and its diploid parent reveal key metabolic processes associated with Paulownia autotetraploidization. Front. Plant. Sci. 7:892. doi: 10.3389/fpls.2016.00892
Dreyfuss, G., Matunis, M. J., Piñol-Roma, S., and Burd, C. G. (1993). hnRNP proteins and the biogenesis of mRNA. Annu. Rev. Biochem. 62, 289–321. doi: 10.1146/annurev.bi.62.070193.001445
Feng, L., Jiang, H., Zhang, Y., and Zhang, S. (2014). Sexual differences in defensive and protective mechanisms of Populus cathayana exposed to high UV-B radiation and low soil nutrient status. Physiol. Plant. 151, 434–445. doi: 10.1111/ppl.12126
Gao, X., He, K., Yang, H., Yuan, T., Lin, H. H., and Clouse, S. D. (2010). Genome-wide cloning and sequence analysis of leucine-rich repeat receptor-like protein kinase genes in Arabidopsis thaliana. BMC Genet. 11:19. doi: 10.1186/1471-2164-11-19
Hideg, E., Jansen, M. A. K., and Strid, A. (2013). UV-B exposure, ROS, and stress: inseparable companions or loosely linked associates? Trends Plant Sci. 18, 107–115. doi: 10.1016/j.tplants.2012.09.003
Hunter, D. A., Yoo, S. D., Butcher, S. M., and McManus, M. T. (1999). Expression of 1-aminocyclopropane-1-carboxylate oxidase during leaf ontogeny in white clover. Plant Physiol. 120, 131–141. doi: 10.1104/pp.120.1.131
Ifuku, K., Yamamoto, Y., Ono, T. A., Ishihara, S., and Sato, F. (2005). PsbP protein, but not PsbQ protein, is essential for the regulation and stabilization of photosystem II in higher plants. Plant Physiol. 139, 1175–1184. doi: 10.1104/pp.105.068643
Jahn, T. P., Schulz, A., Taipalensuu, J., and Palmgren, M. G. (2002). Post-translational modification of plant plasma membrane H(+)-ATPase as a requirement for functional complementation of a yeast transport mutant. J. Biol. Chem. 277, 6353–6358. doi: 10.1074/jbc.M109637200
Jansen, M. A. K., Gaba, V., and Greenberg, B. M. (1998). Higher plants and UV-B radiation: balancing damage, repair and acclimation. Trends Plant Sci. 3, 131–135. doi: 10.1016/S1360-1385(98)01215-1
Järvi, S., Gollan, P. J., and Aro, E. M. (2013). Understanding the roles of the thylakoid lumen in photosynthesis regulation. Front. Plant Sci. 4:434. doi: 10.3389/fpls.2013.00434
Jenkins, G. I. (2009). Signal transduction in responses to UV-B radiation. Annu. Rev. Plant Biol. 60, 407–431. doi: 10.1146/annurev.arplant.59.032607.092953
Jespersen, D., and Huang, B. (2015). Proteins associated with heat-induced leaf senescence in creeping bentgrass as affected by foliar application of nitrogen, cytokinins, and an ethylene inhibitor. Proteomics 15, 798–812. doi: 10.1002/pmic.201400393
Jiang, H., Zhang, S., Feng, L., Korpelainen, H., and Li, C. (2015). Transcriptional profiling in dioecious plant Populus cathayana reveals potential and sex-related molecular adaptations to solar UV-B radiation. Physiol. Plant. 153, 105–118. doi: 10.1111/ppl.12224
Joshi, P., Gartia, S., Pradhan, M. K., and Biswal (2011). Photosynthetic response of clusterbean chloroplasts to UV-B radiation: energy imbalance and loss in redox homeostasis between Q(A) and Q(B) of photosystem II. Plant Sci. 181, 90–95. doi: 10.1016/j.plantsci.2011.04.001
Kaling, M., Kanawati, B., Ghirardo, A., Albert, A., Winkler, J. B., Heller, W., et al. (2015). UV-B mediated metabolic rearrangements in poplar revealed by non-targeted metabolomics. Plant Cell Environ. 38, 892–904. doi: 10.1111/pce.12348
Kim, J. Y., Park, S. J., Jang, B., Jung, C. H., Ahn, S. J., Goh, C. H., et al. (2007). Functional characterization of a glycine-rich RNA-binding protein 2 in Arabidopsis thaliana under abiotic stress conditions. Plant J. 50, 439–451. doi: 10.1111/j.1365-313X.2007.03057.x
Kolomiets, M. V., Chen, H., Gladon, R. J., Braun, E. J., and Hannapel, D. J. (2000). A leaf lipoxygenase of potato induced specifically by pathogen infection. Plant Physiol. 124, 1121–1130. doi: 10.1104/pp.124.3.1121
Lei, Y., Chen, K., Jiang, H., Yu, L., and Duan, B. (2017). Contrasting responses in the growth and energy utilization properties of sympatric Populus and Salix to different altitudes: implications for sexual dimorphism in Salicaceae. Physiol. Plant. 159, 30–41. doi: 10.1111/ppl.12479
Li, D., Fu, Y., Sun, R., Ling, C. X., Wei, Y., Zhou, H., et al. (2005). pFind: a novel database-searching software system for automated peptide and protein identification via tandem mass spectrometry. Bioinformatics 21, 3049–3050. doi: 10.1093/bioinformatics/bti439
Lippert, D. N., Ralph, S. G., Phillips, M., White, R., Smith, D., Hardie, D., et al. (2009). Quantitative iTRAQ proteome and comparative transcriptome analysis of elicitor-induced Norway spruce (Picea abies) cells reveals elements of calcium signaling in the early conifer defense response. Proteomics 9, 350–367. doi: 10.1002/pmic.200800252
Maja, M. M., Kasurinen, A., Holopainen, T., Julkunen-Tiitto, R., and Holopainen, J. K. (2016). The effect of warming and enhanced ultraviolet radiation on gender-specific emissions of volatile organic compounds from European aspen. Sci. Total Environ. 547, 39–47. doi: 10.1016/j.scitotenv.2015.12.114
Marshall, J. S., and Keegstra, K. (1992). Isolation and characterization of a cDNA clone encoding the major Hsp70 of the pea chloroplastic stroma. Plant Physiol. 100, 1048–1054. doi: 10.1104/pp.100.2.1048
Moseley, J. L., Allinger, T., Herzog, S., Hoerth, P., Wehinger, E., Merchant, S., et al. (2002). Adaptation to Fe-deficiency requires remodeling of the photosynthetic apparatus. EMBO J. 21, 6709–6720. doi: 10.1093/emboj/cdf666
Munekaga, Y., Hashimoto, M., Miyaka, C., Tomizawa, K., Endo, T., Tasaka, M., et al. (2004). Cyclic electron flow around photosystem I is essential for photosynthesis. Nature 429, 579–582. doi: 10.1038/nature02598
Randriamanana, T. R., Lavola, A., and Julkunen-Tiitto, R. (2015). Interactive effects of supplemental UV-B and temperature in European aspen seedlings: implications for growth, leaf traits, phenolic defense and associated organisms. Plant Physiol. Biochem. 93, 84–93. doi: 10.1016/j.plaphy.2015.03.001
Ren, J., Dai, W. R., Xuan, Z. Y., Yao, Y. N., Korpelainen, H., and Li, C. Y. (2007). The effect of drought and enhanced UV-B radiation on the growth and physiological traits of two contrasting poplar species. For. Ecol. Manage. 239, 112–119. doi: 10.1016/j.foreco.2006.11.014
Ren, J., Duan, B., Zhang, X., Korpelainen, H., and Li, C. (2010). Differences in growth and physiological traits of two poplars originating from different altitudes as affected by UV-B radiation and nutrient availability. Physiol. Plant. 138, 278–288. doi: 10.1111/j.1399-3054.2009.01328.x
Ren, J., Yao, Y., Yang, Y., Korpelainen, H., Junttila, O., and Li, C. Y. (2006). Growth and physiological responses to supplemental UV-B radiation of two contrasting poplar species. Tree Physiol. 26, 665–672. doi: 10.1093/treephys/26.5.665
Ries, G., Heller, W., Puchta, H., Sandermann, H., Seidlitz, H. K., and Hohn, B. (2000). Elevated UV-B radiation reduces genome stability in plants. Nature 406, 98–101. doi: 10.1038/35017595
Sahi, C., Agarwal, M., Singh, A., and Grover, A. (2007). Molecular characterization of a novel isoform of rice (Oryza sativa L.) glycine rich-RNA binding protein and evidence for its involvement in high temperature stress response. Plant Sci. 173, 144–155. doi: 10.1016/j.plantsci.2007.04.010
Schaller, F., Biesgen, C., Müssig, C., Altmann, T., and Weiler, E. W. (2000). 12-oxophytodienoate reductase 3 (OPR3) is the isoenzyme involved in jasmonate biosynthesis. Planta 210, 979–984. doi: 10.1007/s004250050706
Schönbrunner, E. R., and Schmid, F. X. (1992). Peptidyl-Prolyl cis-Trans isomerase improves the efficiency of protein disulfide isomerase as a catalyst of protein folding. Proc. Natl. Acad. Sci. U.S.A. 89, 4510–4513. doi: 10.1073/pnas.89.10.4510
Schreiner, M., Mewis, I., Huyskens-Keil, S., Jansen, M. A. K., Zrenner, R., Winkler, J. B., et al. (2012). UV-B-induced secondary plant metabolites - potential benefits for plant and human health. Crit. Rev. Plant Sci. 31, 229–240. doi: 10.1080/07352689.2012.664979
Simpson, G. G., and Filipowicz, W. (1996). Splicing of precursors to mRNA in higher plants: mechanism, regulation and sub-nuclear organisation of the spliceosomal machinery. Plant Mol. Biol. 32, 1–41. doi: 10.1007/BF00039375
StrØmme, C. B., Julkunen-Tiitto, R., Krishna, U., Lavola, A., Olsen, J. E., and Nybakken, L. (2015). UV-B and temperature enhancement affect spring and autumn phenology in Populus tremula. Plant Cell Environ. 38, 867–877. doi: 10.1111/pce.12338
Tani, T., Sobajima, H., Okada, K., Chujo, T., Arimura, S., Tsutsumi, N., et al. (2008). Identification of the OsOPR7 gene encoding 12-oxophytodienoate reductase involved in the biosynthesis of jasmonic acid in rice. Planta 227, 517–526. doi: 10.1007/s00425-007-0635-7
Taylor, R. M., Nikaido, O., Jordan, B. R., Rosamond, J., Bray, C. M., and Tobin, A. K. (1996). Ultraviolet-B-induced DNA lesions and their removal in wheat (Triticum aestivum L) leaves. Plant Cell Environ. 19, 171–181. doi: 10.1111/j.1365-3040.1996.tb00238.x
Vanhee, C., Zapotoczny, G., Masquelier, D., Ghislain, M., and Batoko, H. (2011). The Arabidopsis multistress regulator TSPO is a heme binding membrane protein and a potential scavenger of porphyrins via an autophagy-dependent degradation mechanism. Plant Cell 23, 785–805. doi: 10.1105/tpc.110.081570
Vankooten, O., and Snel, J. F. (1990). The use of chlorophyll fluorescence nomenclature in plant stress physiology. Photosynth. Res. 25, 147–150. doi: 10.1007/BF00033156
Varsano, T., Wolf, S. G., and Pick, U. (2006). A chlorophyll a/b-binding protein homolog that is induced by iron deficiency is associated with enlarged photosystem I units in the eucaryotic alga Dunaliella salina. J. Biol. Chem. 281, 10305–10315. doi: 10.1074/jbc.M511057200
Wang, L. H., Li, D. Q., Fu, Y., Wang, H. P., Zhang, J. F., Yuan, Z. F., et al. (2007). pFind 2.0: a software package for peptide and protein identification via tandem mass spectrometry. Rapid Commun. Mass Spectrom. 21, 2985–2991. doi: 10.1002/rcm.3173
Wang, Q. W., Nagano, S., Ozaki, H., Morinaga, S., Hidema, J., and Hikosaka, K. (2016). Functional differentiation in UV-B-induced DNA damage and growth inhibition between highland and lowland ecotypes of two Arabidopsis species. Environ. Exp. Bot. 131, 110–119. doi: 10.1016/j.envexpbot.2016.07.008
Wang, Z. F., Xu, X., Li, X. F., Yang, P., and Yuan, X. L. (2011). The distribution of male and female Populus cathayana populations along an altitudinal gradient. Acta Ecol. Sin. 31, 7067–7074. (In Chinese with English Abstact).
Willmann, R., Lajunen, H. M., Erbs, G., Newman, M. A., Kolb, D., Tsuda, K., et al. (2011). Arabidopsis lysin-motif proteins LYM1 LYM3 CERK1 mediate bacterial peptidoglycan sensing and immunity to bacterial infection. Proc. Natl. Acad. Sci. U.S.A. 108, 19824–19829. doi: 10.1073/pnas.1112862108
Xu, X., Zhao, H., Zhang, X., Hänninen, H., Korpelainen, H., and Li, C. (2010). Different growth sensitivity to enhanced UV-B radiation between male and female Populus cathayana. Tree Physiol. 30, 1489–1498. doi: 10.1093/treephys/tpq094
Yao, X., Xiong, W., Ye, T., and Wu, Y. (2012). Overexpression of the aspartic protease ASPG1 gene confers drought avoidance in Arabidopsis. J. Exp. Bot. 63, 2579–2593. doi: 10.1093/jxb/err433
Yin, L., Lundin, B., Bertrand, M., Nurmi, M., Solymosi, K., Kangasjärvi, S., et al. (2010). Role of thylakoid ATP/ADP carrier in photoinhibition and photoprotection of photosystem II in Arabidopsis. Plant Physiol. 153, 666–677. doi: 10.1104/pp.110.155804
Zhang, L., Li, X., Zheng, W., Fu, Z., Li, W., Ma, L., et al. (2013). Proteomics analysis of UV-irradiated Lonicera japonica Thunb. with bioactive metabolites enhancement. Proteomics 13, 3508–3522. doi: 10.1002/pmic.201300212
Zhang, S., Chen, F., Peng, S., Ma, W., Korpelainen, H., and Li, C. (2010). Comparative physiological, ultrastructural and proteomic analyses reveal sexual differences in the responses of Populus cathayana under drought stress. Proteomics 10, 2661–2677. doi: 10.1002/pmic.200900650
Zhang, S., Feng, L., Jiang, H., Ma, W., Korpelainen, H., and Li, C. (2012). Biochemical and proteomic analyses reveal that Populus cathayana males and females have different metabolic activities under chilling stress. J. Proteome Res. 11, 5815–5826. doi: 10.1021/pr3005953
Zhang, S., Zhang, Y., Cao, Y., Lei, Y., and Jiang, H. (2016). Quantitative proteomic analysis reveals Populus cathayana females are more sensitive and respond more sophisticatedly to iron deficiency than males. J. Proteome Res. 15, 840–850. doi: 10.1021/acs.jproteome.5b00750
Keywords: dioecious, plant proteomics, UV-B radiation, sexual difference, poplar
Citation: Zhang Y, Feng L, Jiang H, Zhang Y and Zhang S (2017) Different Proteome Profiles between Male and Female Populus cathayana Exposed to UV-B Radiation. Front. Plant Sci. 8:320. doi: 10.3389/fpls.2017.00320
Received: 19 November 2016; Accepted: 22 February 2017;
Published: 07 March 2017.
Edited by:
Nicolas L. Taylor, University of Western Australia, AustraliaReviewed by:
Robert Winkler, Cinvestav-IPN, MexicoMaria Cristina Romero-Rodriguez, Universidad Nacional de Asunción, Paraguay
Copyright © 2017 Zhang, Feng, Jiang, Zhang and Zhang. This is an open-access article distributed under the terms of the Creative Commons Attribution License (CC BY). The use, distribution or reproduction in other forums is permitted, provided the original author(s) or licensor are credited and that the original publication in this journal is cited, in accordance with accepted academic practice. No use, distribution or reproduction is permitted which does not comply with these terms.
*Correspondence: Sheng Zhang, emhhbmdzaGVuZ0BpbWRlLmFjLmNu