- 1Institute for Sustainable Plant Protection, National Research Council, Torino, Italy
- 2Council for Agricultural Research and Economics, Viticulture Research Center, Conegliano, Italy
- 3Department of Biotechnology, University of Verona, Verona, Italy
- 4AGROINNOVA, Centre for Innovation in the Agro-Environmental Sector, University of Torino, Grugliasco, Italy
Rootstocks are among the main factors that influence grape development as well as fruit and wine composition. In this work, rootstock/scion interactions were studied using transcriptomic and metabolic approaches on leaves of the “Gaglioppo” variety, grafted onto 13 different rootstocks growing in the same vineyard. The whole leaf transcriptome of “Gaglioppo” grafted onto five selected rootstocks showed high variability in gene expression. In particular, significant modulation of transcripts linked to primary and secondary metabolism was observed. Interestingly, genes and metabolites involved in defense responses (e.g., stilbenes and defense genes) were strongly activated particularly in the GAG-41B combination, characterized in addition by the down-regulation of abscisic acid (ABA) metabolism. On the contrary, the leaves of “Gaglioppo” grafted onto 1103 Paulsen showed an opposite regulations of those transcripts and metabolites, together with the greater sensitivity to downy mildew in a preliminary in vitro assay. This study carried out an extensive transcriptomic analysis of rootstock effects on scion leaves, helping to unravel this complex interaction, and suggesting an interesting correlation among constitutive stilbenes, ABA compound, and disease susceptibility to a fungal pathogen.
Introduction
Grapevine yield and quality depend on several factors, such as genotype, biotic, and abiotic stresses, agro-technical practices, all interacting with each other and influencing development as well as fruit and wine quality (Marè et al., 2013). The relationship between scion and environment is also mediated by the rootstock, obtained by crossing a large variety of Vitis species, such as Vitis berlandieri, Vitis riparia, and Vitis rupestris. In general, grafting influences vigor, yield, water, nutrient, and hormone metabolism, thereby impacting the function of the whole plant and its responses to stress events. In particular, the long distance transport of mRNAs, proteins, and small RNAs contributes to explain the molecular mechanism for how graft partners interact with each other to produce a successful vine graft. Interestingly, genotypes, and scion/rootstock combinations are among the factors influencing the extent of mRNA exchange across the graft junction, as well as the species of mobile mRNAs and the direction of mRNA movement (Yang et al., 2015; Warschefsky et al., 2016; Pagliarani et al., 2017).
Phylloxera-resistant rootstocks have been required in Europe since the 19th century and represent the most prolonged use of a biological control strategy against a pest. Since the last decade, research on this topic has aimed to develop more efficient and sustainable practices providing resistance to other pests and ameliorating environmental adaptation (Anwar et al., 2002). Besides the physical and chemical mechanisms provided by tolerant rootstocks to limit the pathogen progression, not much is known about the ability of the rootstock to provide increased tolerance against scion diseases also through molecular mechanisms. Apple cultivars susceptible to fire blight disease are usually grafted to resistant rootstocks, and it has been interestingly observed in field a different degree of resistance depending on the rootstock employed (Cline et al., 2001).
The common commercial rootstocks used in viticulture and their phenotypes have been characterized (Tramontini et al., 2013; Corso and Bonghi, 2014). Studies investigating the molecular network of rootstock and scion interactions are still scarce, although recently developed “-omics” tools have partially unraveled complex rootstock/scion interactions. Marguerit et al. (2012), for example, dissected the genetic architecture of scion transpiration control and its acclimation to drought using QTL detection traits, defining the rootstock genetic control of these processes. In another study, Marè et al. (2013) showed the effects of different soil compositions and rootstocks on leaf transcriptomic modulation of Pinot Noir. Similarly, Corso et al. (2015) used a comparative transcriptome approach on drought tolerant and susceptible rootstock genotypes in response to water stress. The authors showed variations in modulation of genes involved in the phenylpropanoid pathway (mainly stilbene and flavonoid genes), suggesting a resveratrol-mediated way to enhance water stress resilience in a tolerant rootstock genotype. Stilbenoids (resveratrol and viniferin in particular) have reactive oxygen species (ROS) scavenging activity and act as a powerful defense system against biotic stresses (e.g., powdery mildew, downy mildew; Dabauza et al., 2015; Houillé et al., 2015). Furthermore, it is well-known that Vitis vinifera represents the best nutritional source of stilbenoid compounds with valuable effects on human health; these compounds have numerous pharmacological activities including anti-fungal, anti-inflammatory and anti-tumor effects (Bai et al., 2010; Zhang et al., 2010; Houillé et al., 2014). Constitutive stilbene production in plants and accumulation in the final product (berries and wines) depends on grape variety, climate, soil, and other factors (Vincenzi et al., 2013). These and other secondary metabolites play a key role in grapevine constitutive and induced defense responses to biotic and/or abiotic stresses. Priming the defense state represents an important issue in grapevine immune system modulation that needs to be elucidated (Chong et al., 2009).
In this work, rootstock/scion interactions were studied using transcriptomic and biochemical approaches on leaves of Gaglioppo variety (Librandi CVT 80 clone), typically cultivated in Southern Italy, grafted onto 13 selected rootstocks grown under same environmental conditions. The goals of this study were therefore to analyze the transcript modulations induced in scion leaves in response to grafting onto different rootstocks, and to evaluate constitutive levels of stress, defense-related genes and metabolites induced by different rootstocks and involved in priming defense responses.
Materials and Methods
Vineyard Description and Experimental Set-Up
The study was carried out during two consecutive seasons (2014 and 2015) in a vineyard located in the South of Italy at the Librandi Estate (Cirò Marina, Calabria region), characterized by clay-loam soil (pH 7.9, organic matter content 1.13%, cation exchange capacity, 30.92 meq/100 g soil) and climate conditions as reported in Supplementary Figure S1. Scions of “Gaglioppo” Librandi CVT 80 clone were grafted onto 13 different rootstocks as described in Table 1. All vines were 3 years old and planted at a density of 7,300 plants ha−1, with a spacing of 1.60 × 0.85 m. Plants were trained to a vertical trellis system with spur pruning; conventional agronomic management was regularly applied in the vineyard. Water was supplied by means of drip irrigation. Drippers were positioned around 50 cm from ground and 10 cm from vine trunk. During the vegetative season, each vine was watered 5 times with 30 liters as follows: one in June, two in July and two in August. Rainfall events were recorded as shown in the Supplementary Figure S1. The 13 graft combinations were located in adjacent blocks in the vineyard, and for each of them, nine plants were considered. For each plant, two fully expanded leaves inserted in the central region of the shoot and of approximately equivalent physiological stage and condition, were collected at the end of August 2014. Leaves from three plants of each combination were pooled to form a biological replicate (2 leaves × 3 plants). Three biological replicates were carried out and immediately frozen in liquid nitrogen. Samples were stored at −80°C until molecular and biochemical analyses. Some agronomic parameters were determined for each vine, i.e., bunch weight and yield at harvest, and vigor measuring the pruning mass at winter time in 2 consecutive years (2014, 2015; see Table 1 reporting the average of the data collected in the 2014 and in the 2015 vintages).
RNA Sequencing Analyses
Total RNA was extracted using the Spectrum™ Plant Total RNA extraction kit (Sigma Aldrich) starting from 100 mg of material, and RNA quantity was checked using a NanoDrop 1000 spectrophotometer (Thermo Fisher Scientific). RNA sample quality was checked on an RNA 6000 Nano Labchip using a Bioanalyzer 1000 (Agilent Technologies, Santa Clara, USA); all samples were RIN ≥ 7. cDNA libraries were prepared using TruSeq RNA Sample Prep Kit v2 (Illumina, San Diego, USA), starting from 2.5 μg of total RNA. Selected mRNAs were sheared for 8 min and finished libraries were amplified using 12 cycles of PCR. Libraries were validated on a DNA 1000 Chip using a Bioanalyzer 1000 (Agilent Technologies, Santa Clara, USA) and then quantified through qPCR using KAPA SYBR FAST Universal qPCR kit (Kapa Biosystems, Wilmington, USA) and an internal standard curve. Finally, a 100 SR sequencing run was performed using TruSeq SBS Kit v3-HS and TruSeq PE Cluster Kit v3-cBot-HS kits on a HiSeq 1000 system (Illumina, San Diego, USA).
Reads Pre-processing, Alignment, and Expression Analysis
Sequenced reads underwent quality filtering prior to expression analysis. Low-quality reads (>50 bases with quality < 7 or >10% undetermined bases) were removed with a custom script, sequencing adapter sequences were clipped using Scythe (https://github.com/vsbuffalo/scythe), and low-quality bases at the 3′ ends of reads were trimmed using a quality threshold of 20 over a 10-base window with Sickle (https://github.com/najoshi/sickle) discarding reads shorter than 20 bp.
Reads were aligned against the PN40024 genome sequence using TopHat v2.0.14 (Trapnell et al., 2012), running Bowtie2 ver.2.2.4 (Siragusa et al., 2013) in very sensitive mode (parameter: –b2-very-sensitive) with IGGP_12x ver. 16 as the reference annotation. Cufflinks ver.2.2.0 (Trapnell et al., 2012) was used to calculate the expression values of all known genes in FPKM (fragments per kilobase of exon per million fragments mapped) using a geometric method for the normalization and setting a False Discovery Rate (FDR) ≤ 5% for the detection of significantly differentially expressed genes among the conditions. Expression values and next-generation sequencing data are available in the Gene Expression Omnibus (GEO) public database with accession number GSE82317. The identification of differentially expressed genes (DEGs) was performed using DESeq software (Zenoni et al., 2010).
Hierarchical clustering (HCL) analysis was applied using Pearson's correlation distance and MeV v4.9 software (http://www.tm4.org/mev.html) using as input log2 transformed FPKM values of genes in the five graft combinations.
All transcripts were annotated against the V1 version of the 12X draft annotation of the grapevine genome (http://genomes.cribi.unipd.it/DATA/). Blast2GO (Conesa et al., 2005) software was used to assign gene ontology (GO) terms, and transcripts were grouped into functional categories based on GO biological processes (GO:0005975, Carbohydrate Metabolism; GO:0044036, Cell Wall Metabolism; GO:0006520, Amino Acid and Derivative Metabolism; GO:0019725, Cellular Homeostasis; GO:0009987, Cellular Processes; GO:0032502, Developmental Process; GO:0090304, DNA/RNA Metabolism; GO:0006091, Energy Pathways; GO:0006629, Lipid Metabolism; GO:0009725, Response to Hormone; GO:0006950, Response to Stress; GO:0019748, Secondary Metabolism; GO:0007165, Signal Transduction; GO:0051090, Transcription Factor Activity Regulation; GO:0006810, Transport). Genes with unknown functions, with “No Hit” annotations and encoding pentatricopeptide (PPR) repeat-containing proteins were also included.
Metabolic pathways were visualized using MapMan software Version 3.6.0RC1 (Thimm et al., 2004; http://mapman.gabipd.org/web/guest/mapman), integrated for grapevine with information from Nimblegen and Affymetrix platforms, as previously reported (Rotter et al., 2009; Dal Santo et al., 2013).
RT-qPCR Analysis of Gene Expression
Total RNA was treated with DNase I (Invitrogen, Thermo Fisher Scientific) in accordance with the manufacturer's instructions. For each biological replicate, first-strand cDNA was synthesized starting from 2 μg of total RNA using the High Capacity cDNA Reverse Transcription kit (Applied Biosystems, Thermo Fisher Scientific) according to the manufacturer's instructions. Gene-specific primers (Supplementary Table S5) were designed using Primer Express® software (v3.0, Applied Biosystems, Thermo Fisher Scientific). Reactions were carried out using Power SYBR® Green PCR Master Mix (Applied Biosystems, Thermo Fisher Scientific) as reported in Gambino et al. (2012). Three technical replicates were run for each biological replicate, and the expression of transcripts was quantified after normalization to two housekeeping genes: ubiquitin (VvUBI) and actin1 (VvACT1). The results were calculated as expression ratios (relative quantity, RQ) to GAG-1103P.
HPLC-MS/MS Analysis of Stilbenes and Abscisic Acid (ABA) Content
Stilbenes and ABA were quantified starting from 200 mg of frozen leaves. Reversed-phase high-performance liquid chromatography analysis was performed on a 1,260 Agilent Technologies system. Chromatographic separation was performed with a Phenomenex (Torrance, CA) Luna C18 column (150 × 2.1 mm, 3 μm particle size), with a C18 SecurityGuard column (4.0 × 3.0 mm ID), operated at room temperature. Elution was carried out using aqueous formic acid (0.1% v/v; mobile phase A) and acetonitrile (mobile phase B). HPLC analysis was performed using a gradient from 20 to 60% of mobile phase B in 15 min, then from 60 to 100% of B in 4 min; after washing for 5 min with solvent B, the column was re-equilibrated. The flow rate was 0.2 mL/min and injection volume was 10 μL. A triple quadrupole mass spectrometer (Varian 310-MS TQ Mass Spectrometer) equipped with an ESI interface operated in negative ion mode was used. The detection of analytes was carried out in multiple reaction monitoring (MRM) mode by monitoring two transitions for each compound. The concentration of analytes was quantified using an external calibration method. Original standards of resveratrol (purity ≥99%), polydatin (purity ≥95%), and viniferin (purity ≥95%), purchased from Sigma-Aldrich, were used to prepare the calibration curves. Similarly, the ABA content was quantified as described by Siciliano et al. (2015).
In vitro Downy Mildew Pathogenicity Test
Leaf disks were cut with a cork borer (9 mm in diameter) from leaves collected in August from each selected scion/rootstock combination. Ten leaf disks were placed with the abaxial surface up on water-saturated filter paper sheets (Whatman®, Sigma-Aldrich) in 90 mm Petri plates. Leaf disks from the same rootstock/scion combination inoculated with sterile water served as control.
The inoculum was prepared by rinsing leaves infected with the AG1 Plasmopara viticola population from a greenhouse-grown susceptible selection of grape cv. Chardonnay with distilled water and adjusting to 5 × 104 sporangia/mL using a hemocytometer. The inoculum was then pipetted onto the abaxial surface of each leaf disk using 30 μL/leaf disk. Petri plates were placed in a growth chamber at 22 ± 1°C under a 16 h photoperiod. The experimental design was a randomized complete block with four replications of six leaf disks each with a total of 18 points of inoculation.
Leaf disks were visually examined 7–10 days after inoculation for sporulation using a scale from 0 to 3: 0 = no symptoms; 1 = >0 to 25%; 2 = >26 to 50%; 3 = >50% of the affected area developed from the drop. Disease severity was estimated on 24 disks leaf/cultivar.
Statistical Analyses
Data management and calculations were performed using a Microsoft Excel spreadsheet. One-way analyses of variance (ANOVA) with treatment as the main factor were performed with the SPSS 23.0 statistical software package (SPSS Inc., Cary, NC, USA). Tukey's HSD-test was applied when ANOVA showed significant differences (P < 0.05). The standard deviation (SD) or the standard error (SE) of all means were calculated.
Results and Discussion
The Rootstock Influences the Agronomic Traits of the Scion
Agronomic, metabolic, and molecular changes induced on V. vinifera cv. Gaglioppo by different rootstocks were investigated in a vineyard located in the Calabria region (Southern Italy), characterized by a Mediterranean climate, as confirmed by the meteorological data collected on the experimental site in the summer 2014 (Supplementary Figure S1). These are the required conditions for the production of “Cirò,” the wine typical of the region derived from “Gaglioppo.”
In terms of agronomic traits, the results collected during the 2014 and 2015 vintages confirmed that the rootstock has a significant influence on yield and vigor of the scion (Table 1). In particular, the GAG-41B combination was characterized by very low agronomical performance in comparison with other rootstocks that induce high vigor and yield in “Gaglioppo” (e.g., 1103 Paulsen, 17–37 Mgt, 775 Paulsen, Gravesac, Rupestris du Lot and SO4). Taking into account those results (Table 1) and considering the genetic origin of rootstocks, that provide a good source of variability, five graft combinations were selected for further transcriptomic analyses by RNA-Seq. In more detail, among the rootstocks originated by the cross V. rupestris × V. berlandieri, we choosed the 1103 Paulsen because is extensively used in all regions characterized by Mediterranean climate for its high vigor and good tolerance to drought (Lovisolo et al., 2016). It could be considered as a reference rootstock for these viticultural regions and according to that, 1103 P was used as reference rootstock in our analyses. Among the crosses V. rupestris × V. berlandieri, we selected also the 17–37 Mgt since is extensively used in the Calabria region. Kober 5BB and 3309C induced medium vigor and yield in “Gaglioppo” (Table 1). In addition, 3309C is the only cross V. riparia × V. rupestris available in our vineyard, and the Kober 5BB is one of the more extensively used rootstock in Italy obtained by crossing of V. riparia × V. berlandieri (Table 1). Differently from any other combination, GAG-41B was characterized by lower agronomical performance and it is the only hybrid rootstock in our selection produced from a V. vinifera (“Chasselas”) parental.
Rootstock Affects the Whole-Transcriptome Modulation in Scion Leaves
RNA-Seq produced 499.5 million fragments, equivalent to 49 Gb of total sequencing data with an average of 33 million fragments per sample (Supplementary Table S1). The sequences were quality filtered and aligned to PN40024 reference genome (IGGP 12X v16 assembly) with a mean success rate of 88%. Out of 29,970 annotated genes, 22,292 (74.4%) were found to be expressed (FPKM ≥ 0.1) and 17,050 were significantly differentially expressed genes (DEGs) in at least one rootstock/scion combination with respect to GAG-1103P, chosen as reference (p-value adjusted with Benjamin-Hochberg ≤ 0.5%; Supplementary Table S2). We decided then to apply a fold-change (FC) cut-off and to analyze only the genes whose expression was |log2FC| ≥ 2. We obtained 2,692 DEGs (Supplementary Table S3), the majority of them up-regulated (65%), meaning that these genes are over-expressed in the four graft combinations with respect to GAG-1103P.
As shown by the Venn diagram in Figure 1A, the GAG-41B vs. GAG-1103P comparison showed the highest number of DEGs (2,526, around 94% of DEGs detected) and, interestingly, 1,845 (68%) were exclusively modulated in GAG-41B. On the contrary, only 260 genes showed different expression levels in GAG-17-37 with respect to the reference GAG-1103P, and 20 (0.7%) were genes exclusively affected in GAG-17-37. These numbers clearly suggest that 41 B Mgt rootstock induced very different responses in “Gaglioppo” at the molecular level with respect to 1103 Paulsen, whereas the scion showed similar transcriptomic changes when grafted onto 17–37 Mgt and 1103 Paulsen.
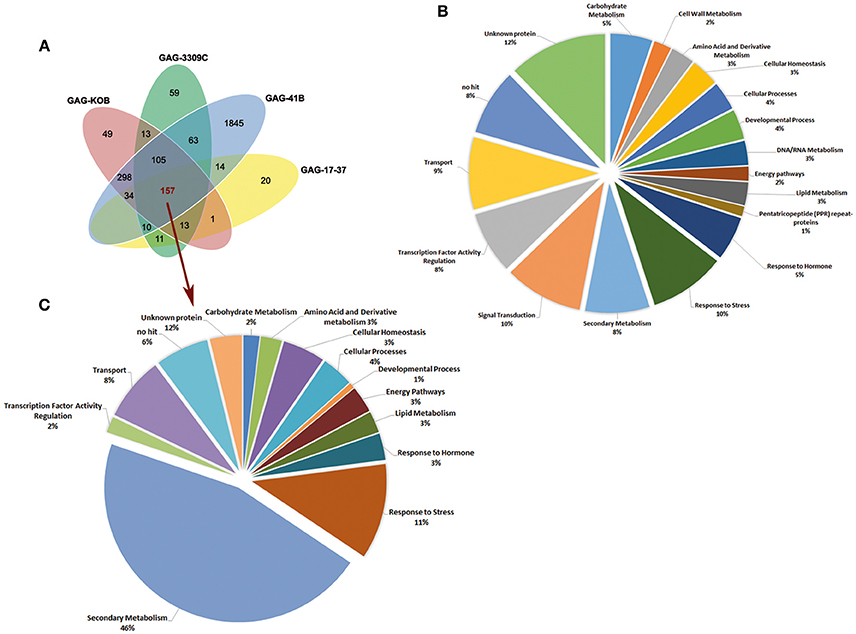
Figure 1. Venn diagrams showing the distribution of the 2,692 DEGs (P < 0.05; |log2FC| ≥ 2) in the graft combinations GAG-KOB, GAG-3309C, GAG-41B, and GAG-17-37, each compared to the reference GAG-1103P (A). Functional category distribution of the 2,692 DEGs (B) and of the 157 genes showing common differential expression in all the graft combinations (C). Transcripts were grouped into the most represented functional categories, based on the GO classification of biological processes.
The 2,692 DEGs analyzed were annotated according to Grimplet et al. (2012), associated with their respective GO terms and then grouped in 18 highly-represented categories, based on GO biological processes (Figure 1B). The analysis of transcript functional categories revealed that the majority of DEGs are involved in stress responses, signal transduction processes, secondary metabolism, transport and transcription factor activity. Considering the 157 DEGs in common to all four graft combinations compared to reference GAG-1103P (Figure 1A), they were almost exclusively up-regulated (97%) and mainly involved in secondary metabolism (46%), with strong up-regulation of genes related to phenylpropanoid biosynthesis (Figure 1C).
A metabolic overview was performed with MapMan software to visualize the metabolic pathways in which DEGs are involved (Supplementary Figure S2A). In the GAG-41B vs. GAG-1103P comparison, the DEGs were distributed among all the metabolic pathways, although mainly concentrated in secondary metabolism (upregulation of terpenes, flavonoids, and phenylpropanoid genes) and in the photosynthesis process, with several up-regulated genes belonging to the two photosystems (Figure 2, Supplementary Figure S2B). Moreover, several DEGs were involved in hormonal responses, cell wall metabolism, and kinase signaling cascades, particularly in the activity of transcription factors such as bZIP, WRKY and MYB; overall, these metabolic changes affect plant responses to abiotic/biotic stresses (Figure 2). On the contrary, considering the comparison GAG-17-37 vs. GAG-1103P, a small number of DEGs were involved in responses to stress and secondary metabolism, while there were no differentially expressed genes involved in photosynthetic pathways (Figure 2, Supplementary Figure S2B).
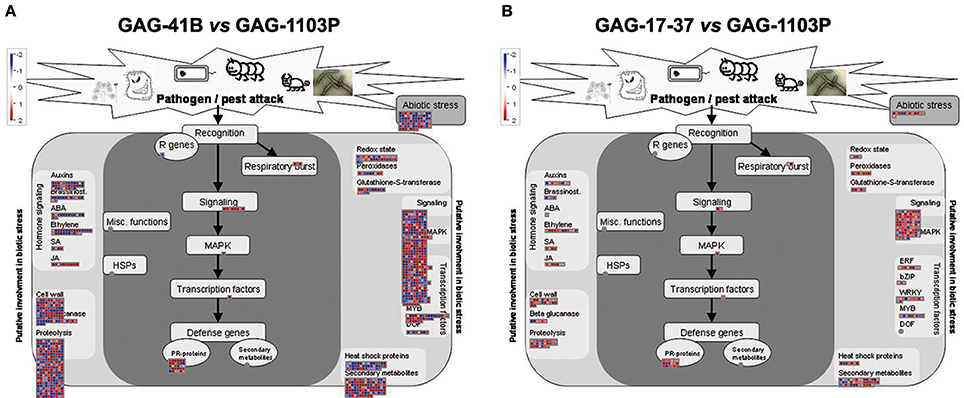
Figure 2. MapMan software output to provide an overview of the effect of 41 B Mgt rootstock (A) and 17–37 Mgt rootstock (B) induced on biotic stress responses and, in particular, on secondary metabolism. Log2 fold changes values referred to GAG-1103P are represented. Up-regulated and down-regulated transcripts are shown in red and blue, respectively.
In order to further analyze the data, a hierarchical clustering (HCL) analysis involving the DEGs with |log2FC| ≥2 was conducted to investigate the relationships of similarity among the five graft combinations. GAG-41B formed a completely separate clade from the others, whereas the remaining rootstock/scion combinations clustered together in a second group, in agreement with the fact that 41 B Mgt rootstock induced the most different transcriptomic changes in “Gaglioppo” (Figure 3A). In addition, the 2,692 DEGs clustered in two major groups (Figure 3B): Cluster 1 is characterized by 1,723 genes up-regulated in GAG-41B, partially up-regulated in GAG-KOB and less expressed in GAG-1103P, whereas Cluster 2 includes the remaining 969 genes down-regulated in GAG-41B with respect to the other combinations (Supplementary Table S4).
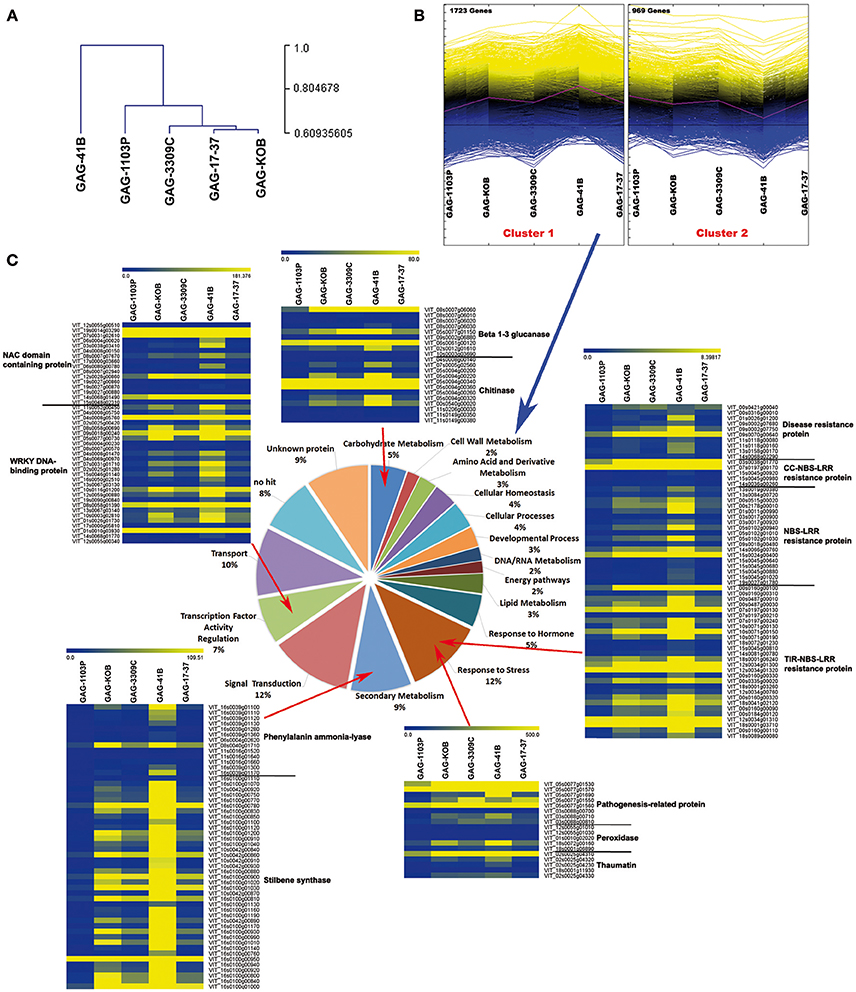
Figure 3. Transcriptomic reprogramming induced in the scion “Gaglioppo” by different rootstocks. Cluster dendrogram of GAG-41B, GAG-1103P, GAG-3309C, GAG-17-37, and GAG-KOB obtained using the average expression value of the three biological replicates (A). Hierarchical clustering analysis of transcripts that were differentially modulated among the five different graft combinations (P < 0.05; |log2FC| ≥ 2) (B). Functional category distribution of the transcripts belonging to Cluster 1, grouped into the most represented functional categories, based on the GO classification of biological processes (C). The heat maps of transcriptional profiles associated with the categories “Carbohydrate metabolism,” “Response to stress,” “Secondary metabolism,” and “Transcription factor activity regulation” were generated with TMeV 4.9 using the average expression value of the three biological replicates.
In Cluster 1, the carbohydrate metabolism category is highly represented by genes such as beta-glucanases, involved in callose degradation, and chitinases. Similarly, other genes related to biotic stress responses such as disease resistance proteins (NBS and NBS-LRR class), pathogenesis-related proteins as well as genes involved in jasmonate metabolism, lipid metabolism, phenylpropanoid metabolism, NAC domain-containing proteins, and WRKY DNA-binding proteins were induced in “Gaglioppo,” in particular by the 41 B Mgt rootstock (Cluster 1; Supplementary Table S4, Figure 3C). Interestingly, genes involved in the ABA metabolism were instead up-regulated in GAG-1103P (Cluster 2; Supplementary Table S4).
A Glimpse on the Defense-Related Responses Induced by the Rootstock
Priming plant defense has obtained increasing attention in the last decade, and several studies have been conducted to enhance multiple stress tolerance by biological, genetic, or chemical methodologies (Savvides et al., 2016). Defense priming is defined as an adaptive, low-cost defensive measure in which the plant is in a persistently primed state of enhanced defense readiness (Martinez-Medina et al., 2016). The priming stimulus leading to this state can be of different nature and studies have revealed the usual presence of defense priming in field conditions (Heil and Silva Bueno, 2007). Our results show how rootstocks are potentially involved in the priming phenomenon, influencing the response of the scion to the environment through different strategies, with the 1103 Paulsen and 41 B Mgt rootstocks representing two opposite examples regarding the regulation of scion metabolism.
Phenylpropanoid Metabolism
Stilbenoids show ROS scavenging activity acting as a powerful defense system against biotic stresses (e.g., powdery mildew, downy mildew; Dabauza et al., 2015; Houillé et al., 2015).
The rootstocks Kober 5BB, 3309 Couderc, 17–37 Mgt, and 41 B Mgt induced the activation of phenylpropanoid metabolism in “Gaglioppo” (Cluster 1) at very high levels in respect to 1103 Paulsen (e.g., through the up-regulation of about 40 genes coding for stilbene synthases). In particular, the 41 B Mgt rootstock induced high activation of these transcripts, with many stilbene synthase genes showing up to 200-fold up-regulation (Figure 3C). We selected two stilbene synthases (VvSTS16-VIT_16s0100g00920 and VvSTS48-VIT_16s0100g01200), members of two different sub-groups of STS (Vannozzi et al., 2012), with different transcription induction in response to stresses. VvSTS48 is a gene induced in particular after downy mildew infection, while VvSTS16 is activated in response to UV-C stress (Vannozzi et al., 2012). We extended the qRT-PCR analyses to all graft combinations. In addition, to validate the RNA-seq data (Supplementary Figure S3), we observed a strong activation of VvSTS16 and VvSTS48 in “Gaglioppo” grafted onto 41 B Mgt and Rupestris du Lot. Conversely, 1103 Paulsen and 140 Ruggeri induced transcription levels up to 100 times lower in comparison to GAG-41B (Figure 4). In addition, GAG-420A, GAG-779P and GAG-SO4 also showed high levels of VvSTS48 in leaves. A possible explanation for this marked activation of stilbene-related genes could be that an active photosynthetic process is usually coupled with active growth of the plant (Corso et al., 2015), whereas in this case, interestingly, despite the up-regulation of photosynthesis—related genes (Supplementary Figure S2B), GAG-41B showed very low productivity and vigor, as already discussed (Table 1). Taking into account that imbalance between light capture and its utilization can result in the production of ROS responsible for the oxidative damage of cellular components (Apel and Hirt, 2004; Kar, 2011), we hypothesize that this imbalance could induce the 41 B Mgt rootstock to up-regulate stilbene-related genes that, with their ROS-scavenging activity, can protect the plant from oxidative damage. Moreover, this indication could be corroborated by the fact that resveratrol is induced by reactive oxygen species such as H2O2 (Wang J. F. et al., 2015). In addition to STSs, other genes involved in the prevention of oxidative stress, such as peroxidases and glutathione S-transferases, were up regulated in particular in GAG-41B (Cluster 1, Supplementary Table S4).
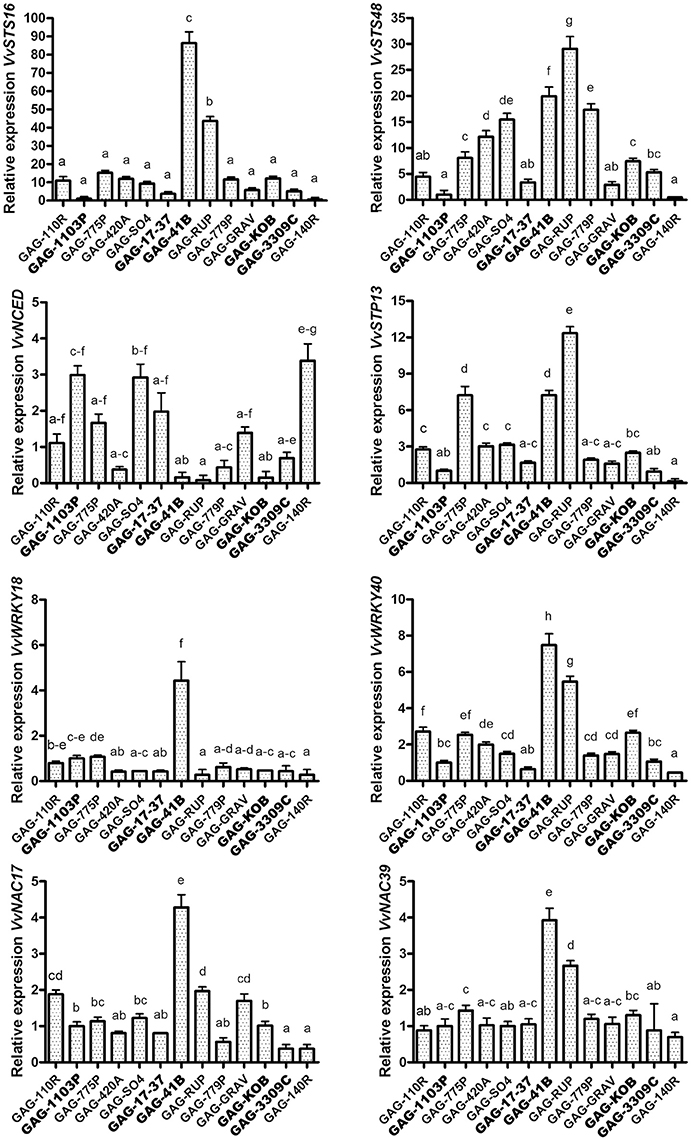
Figure 4. Validation of the RNA sequencing results. Relative expression levels obtained by RT-qPCR analysis of the VvSTS16 (VIT_16s0100g00920), VvSTS48 (VIT_16s0100g01200), VvNCED (VIT_02s0087g00930), VvSTP13 (VIT_05s0020g03140), VvWRKY18 (VIT_04s0008g05760), VvWRKY40 (VIT_09s0018g00240), VvNAC17 (VIT_19s0014g03290) and VvNAC39 (VIT_07s0031g02610) genes tested in the 13 graft combinations. Lower case letters above bars denote significant differences by Tukey's HSD-test (P < 0.05). Data are expressed as mean ± SE (n = 3). The combinations chosen for RNA-seq analyses are indicated in bold font.
The high level of STS transcription reported above in some graft combinations (Figure 4) suggested an increase in stilbene metabolite synthesis and accumulation in leaves. The quantification of resveratrol, viniferin, and piceid contents in leaves was performed in all 13 graft combinations available. Trans–resveratrol (trans-3,5,4′,-trihydroxy-trans-stilbene), its glucoside precursor trans-piceid (resveratrol-3-β-mono-D-glucoside) and the resveratrol dehydrodimer ε-viniferin were chosen since are low molecular weight phenolic compounds that act as antifungal or as phytoalexins common in grape (Bavaresco and Fregoni, 2001; Vincenzi et al., 2013). Trans-piceid was detectable in all samples, with a higher accumulation in GAG-420A and GAG-SO4 (Figure 5A). This last graft combination also showed a higher content of trans-resveratrol, together with GAG-3309C and, in general, trans-resveratrol showed a very similar trend of accumulation to trans-piceid (Figure 5B). Interestingly, not all the graft combinations produced a detectable level of viniferin, a more toxic derivative of trans-resveratrol (Figure 5C). This stilbenoid oligomer was detected only in GAG-110R, GAG-420A, and GAG-SO4.
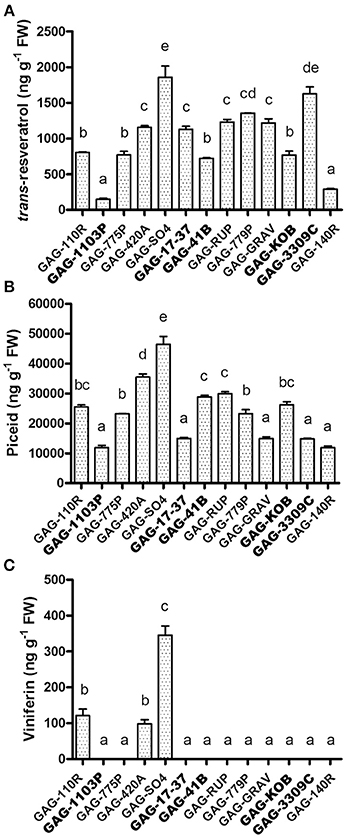
Figure 5. Analysis of stilbenic compounds in scion leaves of 13 graft combinations. Quantification of trans-resveratrol (A), piceid (B) and viniferin (C) assessed in the scion leaves. Lower case letters above bars denote significant differences by Tukey's HSD-test (P < 0.05). Data are expressed as mean ± SD (n = 3). The combinations chosen for RNA-seq analyses are indicated in bold font.
ABA Metabolism
It is well-known that ABA is involved in many plant-stress responses, and although this hormone has been extensively studied in terms of its function in plant drought tolerance, it plays a crucial role also in response to biotic stresses (Cao et al., 2011; Spence and Bais, 2015). ABA modulates the defense against the pathogen through different ways, e.g., interfering with other hormones such as salicylic acid or with ROS accumulation (Asselbergh et al., 2008).
Our transcriptomic results showed an interesting up-regulation of ABA metabolism—related genes peculiar of GAG-1103P. In order to gain information at the metabolite level and to investigate further the rootstock/scion interaction, we performed the quantification of the foliar ABA content in all 13 available graft combinations (Figure 6). Higher ABA levels were measured in GAG-140R and GAG-1103P, confirming what emerged from the RNA sequencing data, whereas the combinations GAG-SO4, GAG-420A, GAG-RUP, GAG-3309C, GAG-110R, and GAG-41B showed a lower foliar ABA content. These results show how the rootstocks induced different ABA level fluctuations in the scion “Gaglioppo.” In addition, we monitored the leaf expression levels of a gene involved in ABA biosynthesis, i.e., 9-cis-epoxycarotenoid dioxygenase (VvNCED, VIT_02s0087g00930; Figure 4). The VvNCED transcript showed a similar trend in respect to the metabolite level, with the exception of some combinations, in particular GAG-SO4. The importance of ABA in root-to-shoot signaling and in the stomatal regulation is well-established (Heilmeier et al., 2007; Lovisolo et al., 2008; Tombesi et al., 2015), and it is well-known that the leaf hormone level is determined not only by biosynthesis but also by ABA transported from the root (Zhang et al., 1987; Lovisolo et al., 2016). This could explain the discrepancies between VvNCED expression and the ABA level in some combinations and underlines the importance of the rootstock influence. Very recent studies have added a further level of complexity to ABA signaling in plants, showing that leaf biosynthesized ABA is transported via phloem to the roots where it controls root architecture and growth by competing with IAA metabolism (Manzi et al., 2015; McAdam et al., 2016). Since we found different VvNCED expression levels in the scion “Gaglioppo,” depending on the graft combination, we can suggest that the rootstock controls leaf ABA levels, not only by providing ABA from the roots via the xylem, but also by directly affecting ABA biosynthesis in the leaf that can be translocated via the phloem to the roots.
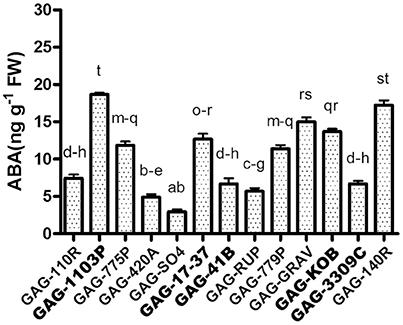
Figure 6. Analysis of endogenous ABA levels. Measurement of the leaves active ABA content in the 13 graft combinations. Lower case letters above bars denote significant differences attested by Tukey's HSD-test (P < 0.05). Data are expressed as mean ± SE (n = 3). The combinations chosen for RNA-seq analyses are indicated in bold font.
Carbohydrate Metabolism
In terms of carbohydrate metabolism, sugars involved in priming plant defense responses against fungal pathogens have been reported for both mono- and dicotyledons. Gòmez-Ariza et al. (2007) demonstrated the sucrose-mediated responsiveness of defense-related genes in rice and a subsequent improved resistance to Magnaporthe oryzae infection. Similarly, Lemonnier et al. (2014) demonstrated in Arabidopsis the key role of sugar transporter 13 (STP13) in intracellular glucose uptake. Here, a high constitutive level of STP13 led to an enhanced capacity to absorb glucose and to increase the basal resistance to Botrytis cinerea by limiting disease severity. Interestingly, VvSTP13, the ortholog of the Arabidopsis sugar transporter protein AtSTP13, was strongly up-regulated, particularly in GAG-41B leaves (Supplementary Table S4). In addition, qRT-PCR analysis of VvSTP13 performed on all 13 graft combinations reported an up-regulation also in GAG-775P and GAG-RUP, whilst GAG-1103P, GAG-3309C, and GAG-140R showed significantly lower activation (Figure 4).
Stress-Responsive Transcripts
A large number of disease resistance protein genes belonging to the nucleotide-binding domain/leucine-rich repeat (NBS-LRR) class and several pathogenesis-related proteins (PRPs) were found to be up-regulated, particularly in GAG-41B, and down-regulated in GAG-1103P (Supplementary Table S4, Figure 3C). NBS-LRR genes have been largely studied and characterized in Arabidopsis as immune receptors against pathogens, and their importance in the innate immune system in plants (Chae et al., 2016) has posed new questions regarding the role(s) of the grapevine rootstock in how the scion perceives molecules released by pathogens; this requires further studies. Taken together, these results suggest the presence of pathogen-responsive priming genes mediated by sugar signaling, as previously reported in rice and tobacco (Murillo et al., 2003; Gòmez-Ariza et al., 2007). In addition, looking at the lipid metabolism category, several lipase and lipoxygenase isoform (LOXs) transcripts were more highly expressed in Cluster 1 (Supplementary Table S4). LOX isoforms encode enzymes that participate in the synthesis of jasmonic acid and have been widely associated with responses to biotic and abiotic factors, suggesting their involvement in priming defense mechanisms (Cervantes-Gámez et al., 2016; Chitarra et al., 2016). In the transcription factor activity regulation category, 27 VvWRKY genes were found to be significantly activated in Cluster 1, while only one was activated in Cluster 2 (Supplementary Table S4, Figure 3C). WRKY proteins are transcription factors that have been found to be involved in the control of a broad range of physiological processes and responses to biotic and abiotic stresses (Wang M. et al., 2015) and are organized in a large superfamily (59 genes) in V. vinifera (Wang et al., 2014). In our transcriptomic data, we found that about 50% of the confirmed VvWRKY genes in grapevine were activated, particularly VvWRKY18 (VIT_04s0008g05760) and VvWRKY40 (VIT_09s0018g00240) which were selected for RT-qPCR experiments involving all graft combinations (Figure 4). High expression of VvWRKY18 was found only in GAG-41B; VvWRKY40 was strongly expressed in GAG-41B and GAG-RUP, whereas it was repressed mainly in GAG-140R with regulation similar to that of VvSTS16 (Figure 4). These genes belong to Group II-a of the phylogenetic tree of WRKY domains in both grapevine and Arabidopsis and are associated with abiotic and biotic stresses, probably in controlling basal defense responses (Wang et al., 2014). Although in the last decade considerable effort has been made in functional studies of WRKYs in physiological and plant defense processes, further studies are needed to clarify these mechanisms in the complex rootstock/scion interaction. VvNAC genes, another transcription factor family playing an important role in the response to stresses, were found to be significantly activated in Cluster 1 (17 genes up-regulated, in particular in GAG-41B Supplementary Table S4, Figure 3C). Overexpression of NAC improves pathogen tolerance in transgenic Arabidopsis (Puranik et al., 2012) and in our experiments we observed a strong up-regulation of VvNAC17—VIT_19s0014g03290 and VvNAC39—VIT_07s0031g02610 (Wang et al., 2013) in GAG-41B and partially in GAG-RUP (Figure 4).
Could Constitutive Stilbenoid and ABA Compounds Trigger Plant Immunity Responses? A Preliminary Indication
It is well-known how Plasmopara viticola and fungal infections in general induce the accumulation of stilbenes as a defense reaction, since these phytoalexins are biologically active compounds with antifungal activities (Jeandet et al., 2002; Chalal et al., 2014). Viniferin is highly toxic and can be considered an important marker for grapevine resistance to downy mildew (Pezet et al., 2004), but very little information is available on the role of constitutive stilbenoids (Duan et al., 2015).
The results discussed above suggest a rootstock-dependent involvement of biotic stress responses. We wondered if this scenario and in particular the constitutive presence of stilbenoid and ABA compounds could influence P. viticola infection. For this purpose, leaf disks from “Gaglioppo” grafted onto different rootstocks were subjected to P. viticola in vitro infection (Figure 7A). Leaf disks were rated as resistant (disease index: 0–10%), moderately susceptible (11 to 30%), and susceptible (>30%). GAG-KOB, GAG-RUP, GAG-SO4, GAG-420A, and GAG-110R leaf disks were resistant to downy mildew and remained fresh and green during the evaluation period, suggesting in three of five combinations that the constitutive presence of viniferin (Figure 5C) could help counteract this pathogen (Figure 7A). The most susceptible combination was GAG-1103P, characterized by low levels of all tested stilbenoids, by the down-regulation of several transcripts involved in defense responses (Cluster 1, Supplementary Table S4) and by higher ABA content at the transcript and metabolite level, while the remaining samples were rated as moderately susceptible. Besides the role of viniferin, a negative correlation between the amount of trans-resveratrol and trans-piceid and the disease index of all samples was observed (Figure 7B), suggesting that graft combinations with a high level of trans-resveratrol and its storage form trans-piceid could be more prepared to respond to pathogen attack. While an inverse relationship between preformed flavonoids and the susceptibility of grapevines to downy mildew has been demonstrated (Agati et al., 2008; Latouche et al., 2013), in this work, we suggest a similar role for the preformed non-oxidized form of stilbenes. Resveratrol and its glycosylated form trans-piceid are produced in susceptible grapevines as response to infection of downy mildew (Pezet et al., 2004; Houillé et al., 2015), with limited antifungal activity when the infection is in progress. Our results suggest how these compounds, probably in association with other defense responses, could play an important role if present in a constitutive form before infection, likely because, as suggested by Pool et al. (1981), the availability and the speed of resveratrol synthesis are positively correlated with the resistance of grapevine varieties to fungal diseases. It is known moreover that pathogens can delay the expression of host genes belonging to the phenylpropanoid pathway during infection (Milcevicova et al., 2010), meaning that a primed state of expression of those genes can help the plant to be more ready to the pathogen attack. Similar indications come from a transcriptomic study on “Gala” apple scion grafted on different rootstocks, showing that differences in scion fire blight susceptibility were associated with high expression of phenylpropanoid and biotic and abiotic stress responses induced by specific rootstocks in uninfected trees (Jensen et al., 2012).
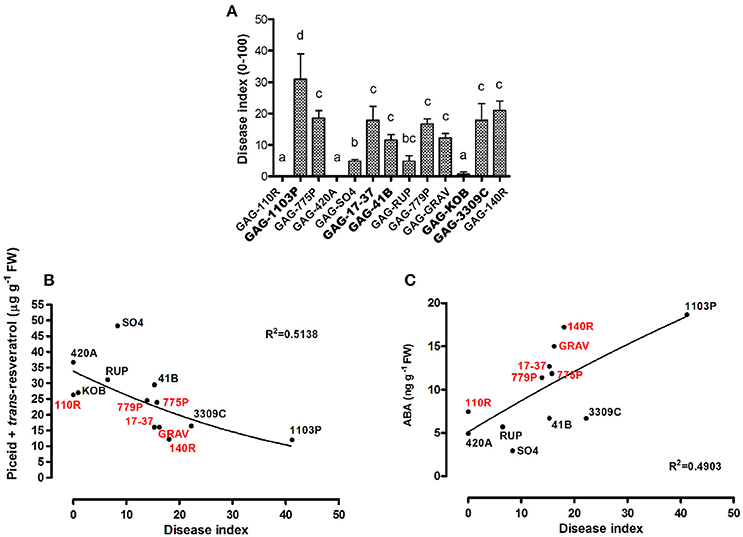
Figure 7. Evaluation of disease resistance of scion leaves of 13 graft combinations against Plasmopara viticola. The Disease Index (DI) is expressed as the percentage of infected leaves. Lower case letters above bars denote significant differences by Tukey's HSD-test (P < 0.05). Data are expressed as mean ± SD (n = 3) (A). The correlations between the disease index and the amount of piceid and trans-resveratrol (p < 0.05) (B) and between the disease index and the ABA content (p < 0.05) (C) related to each graft combination are plotted. Red and black labels are used to underline how graft combinations group according to the negative (B) or positive (C) correlation. The combinations chosen for RNA-seq analyses are indicated in bold font.
On the contrary, an interesting positive correlation between ABA content and disease index was observed in 12 graft combinations out of 13 (Figure 7C). Several studies report a negative role of ABA in plant immunity (reported in Cao et al., 2011) and many specific examples have shown that elevated ABA content in plants is associated with increased susceptibility to various bacterial and fungal pathogens, while decreased ABA content resulted in increased resistance (Spence and Bais, 2015), as observed in our results.
These findings open new perspectives about the growth-defense trade-offs in grapevine also in term of appropriate rootstock selection for viticulturists. It is well-established that growth-defense trade-offs have important consequences in physiological and carbon allocation costs shifting a pool of energy reserves toward growth or defense machineries (Messina et al., 2002; Huot et al., 2014). In general, several studies showed that plants resist against biotic attack producing defense compounds as innate immune responses (e.g., tannins and/or activating phenylpropanoid pathways), often associated with reduced growth (Bandau et al., 2015; Decker et al., 2016) as observed here in GAG-41B graft combination.
To date, the molecular mechanisms involved in plant growth-defense trade-offs need to be elucidated, since studies focused mainly on hormone crosstalk (Huot et al., 2014). Only recently researchers posed more attention to the fine-tuning transcriptional processes behind this phenomenon. For example, Campos et al. (2016) through epistatic gene interactions demonstrated that Arabidopsis growth inhibition in response to a biotic factor is not caused only by allocation of photoassimilates toward defense but by activating a molecular network involving jasmonate signaling. Furthermore, it has been reported that V. vinifera cells grown in vitro respond to methyljasmonate with increase of phytoalexin production, piceid in particular (Krisa et al., 1999). Those results together suggest as jasmonate could play a key role in regulating the signal transduction toward growth or defense. Coherently, our results showed how the 41B rootstock induced higher levels of stilbenoid genes in the scion (Cluster 1; Supplementary Table S4), lower vigor (Table 1) and higher expression of jasmonate synthesis and signaling genes (Cluster 1; Supplementary Table S4), in respect to the other rootstocks considered in the RNA–seq analysis.
In summary, our study showed a transcriptional picture that could elucidate how several rootstocks drive the fitness costs, associated with constitutive defense, modulating stress related genes in scion leaves as well as other transcripts not directly related to the phenotype. Growers for decades selected grapevine rootstocks to achieve an enhanced primed state against biotic or abiotic factors, regulating their growth-defense trade-offs to optimize plant fitness in response to a particular environment.
Conclusions
It is well-known that different rootstocks confer different yield and phenological features to the grapevine scion; however, the results obtained in this work further improve our knowledge of the molecular and metabolic changes involved in this interaction. Following the trade-offs fashion, GAG-41B emerged as a graft combination that responds in a very different way with respect to the others, in particular with respect to GAG-1103P, which showed the opposite behavior in terms of molecular responses; GAG-KOB, GAG-3309C, and GAG17-37 had responses similar to GAG-1103P, although less pronounced. The rootstock 41 B Mgt was able to induce a number of genes involved in early responses to biotic stresses (e.g., VvPRs, VvNBS-LRRs, VvLOXs, VvNACs, VvWRKYs), with particularly high activation of secondary metabolism genes (VvSTSs). For these reasons, “Gaglioppo” grafted onto rootstock 41 B Mgt appears to be constitutively more prepared to respond to pathogen attack, although it is less productive and vigorous, whereas 1103 Paulsen seems to not induce scion responses against biotic stresses. An indication in this direction also emerged from the leaf disk assay regarding in vitro infection with P. viticola, where GAG-41B was rated as moderately susceptible and GAG-1103P was very sensitive to Plasmopara attack.
More in general our results give a first indication about the relationship among constitutive stilbenoids and ABA levels and disease susceptibility to a fungal pathogen. Interestingly stilbenoids and ABA showed an opposite trend in respect to the disease susceptibility, identifying the susceptible graft combination as the one with less stilbenoids and higher ABA level. These results should be further verified under different environmental conditions, at different developmental stages and using other cultivars as scion, in order to further assess the mechanisms of the complex rootstock/scion interaction. The results obtained in the present study, although representing only one time point of the grapevine season, provide an overall picture of the genetic and metabolic modulation inducted by several rootstocks in grapevine. These findings may facilitate the selection of appropriate graft combinations in the field, depending on the environment conditions, and help to identify candidate genes for further functional studies or for cis-genic and genome editing purposes.
Author Contributions
WC and IP performed most of the molecular analysis, analyzed the data and wrote the manuscript; CA and AM performed and elaborated RNA-seq data; PB collaborated to the molecular analysis; DS elaborated agronomical data; GGi performed the pathogenicity test; IS performed the HPLC-MS/MS analysis; MG, FM, and MD critically revised the manuscript; GGa designed the experiments, contributed to data analysis and to the writing; all authors read and approved the manuscript.
Funding
This research was funded by Regione Calabria in the framework of PSR 2007–2013, Misura 124. IP was financed by the Italian Ministry of University and Research, FIR project RBFR13GHC5: “The Epigenomic Plasticity of Grapevine in Genotype per Environment Interactions.”
Conflict of Interest Statement
The authors declare that the research was conducted in the absence of any commercial or financial relationships that could be construed as a potential conflict of interest.
The reviewer JG and handling Editor declared their shared affiliation, and the handling Editor states that the process nevertheless met the standards of a fair and objective review.
Acknowledgments
The authors would like to thank Librandi Estate for carrying out the experiments in its vineyard.
Supplementary Material
The Supplementary Material for this article can be found online at: http://journal.frontiersin.org/article/10.3389/fpls.2017.00654/full#supplementary-material
References
Agati, G., Cerovic, Z. G., Dalla Marta, A., Di Stefano, V., Pinelli, P., Traversi, M. L., et al. (2008). Optically-assessed preformed flavonoids and susceptibility of grapevine to Plasmopara viticola under different light regimes. Funct. Plant Biol. 35, 77–84. doi: 10.1071/FP07178
Anwar, S. A., McKenry, M., and Ramming, D. (2002). A search for more durable grape rootstock resistance to root-knot nematode. Am. J. Enol. Vitic. 53, 19–23.
Apel, K., and Hirt, H. (2004). Reactive oxygen species: metabolism, oxidative stress, and signal transduction. Annu. Rev. Plant Biol. 55, 373–399. doi: 10.1146/annurev.arplant.55.031903.141701
Asselbergh, B., De Vleesschauwer, D., and Höfte, M. (2008). Global switches and fine-tuning-ABA modulates plant pathogen defense. Mol. Plant Microbe Interact. 21, 709–719. doi: 10.1094/MPMI-21-6-0709
Bai, Y., Mao, Q. Q., Qin, J., Zheng, X. Y., Wang, Y. B., Yang, K., et al. (2010). Resveratrol induces apoptosis and cell cycle arrest of human T24 bladder cancer cells in vitro and inhibits tumor growth in vivo. Cancer Sci. 101, 488–493. doi: 10.1111/j.1349-7006.2009.01415.x
Bandau, F., Decker, V. H. G., Gundale, M. J., and Albrectsen, B. R. (2015). Genotypic tannin levels in Populus tremula impact the way nitrogen enrichment affects growth and allocation responses for some traits and not for others. PLoS ONE 10:e0140971. doi: 10.1371/journal.pone.0140971
Bavaresco, L., and Fregoni, M. (2001). “Physiological role and molecular aspects of grapevine stilbenic compounds,” in Molecular Biology and Biotechnology of the Grapevine, ed Roubelakis-Angelakis (Dordrecht: Kluwer Academic Publishers), 153–182.
Campos, M. L., Yoshida, Y., Major, I. T., Ferreira, D. O., Weraduwage, S. M., Froehlich, J. E., et al. (2016). Rewiring of jasmonate and phytochrome B signaling uncouples plant growth-defense tradeoffs. Nat. Commun. 7:12570. doi: 10.1038/ncomms12570
Cao, F. Y., Yoshioka, K., and Desveaux, D. (2011). The roles of ABA in plant–pathogen interactions. J. Plant Res. 124, 489–499. doi: 10.1007/s10265-011-0409-y
Cervantes-Gámez, R. G., Bueno-Ibarra, M. A., Cruz-Mendívil, A., Calderón-Vázquez, C. L., Ramírez-Douriet, C. M., Maldonado-Mendoza, I. E., et al. (2016). Arbuscular mycorrhizal symbiosis-induced expression changes in Solanum lycopersicum leaves revealed by RNA-seq analysis. Plant Mol. Biol. Rep. 34, 89–102. doi: 10.1007/s11105-015-0903-9
Chae, E., Tran, D. T. N., and Weigel, D. (2016). Cooperation and conflict in the plant immune system. PLOS Pathog. 12:e1005452. doi: 10.1371/journal.ppat.1005452
Chalal, M., Klinguer, A., Echairi, A., Meunier, P., Vervandier-Fasseur, D., and Adrian, M. (2014). Antimicrobial activity of resveratrol analogues. Molecules 19, 7679–7688. doi: 10.3390/molecules19067679
Chitarra, W., Pagliarani, C., Maserti, B., Lumini, E., Siciliano, I., Cascone, P., et al. (2016). Insights on the impact of arbuscular mychorrhizal symbiosis on tomato tolerance to water stress. Plant Physiol. 171, 1009–1023. doi: 10.1104/pp.16.00307
Chong, J., Poutaraud, A., and Hugueney, P. (2009). Metabolism and roles of stilbenes in plants. Plant Sci. 177, 143–155. doi: 10.1016/j.plantsci.2009.05.012
Cline, J. A., Hunter, D. M., Bonn, W. G., and Bijl, M. (2001). Resistance of the Vineland series of apple rootstocks to fire blight caused by Erwinia amylovora. J. Am. Pomol. Soc. 55, 218–221
Conesa, A., Götz, S., García-Gómez, J. M., Terol, J., Talón, M., and Robles, M. (2005). Blast2GO: a universal tool for annotation, visualization and analysis in functional genomics research. Bioinformatics 21, 3674–3676. doi: 10.1093/bioinformatics/bti610
Corso, M., and Bonghi, C. (2014). Grapevine rootstock effects on abiotic stress tolerance. Plant Sci. Today 1, 108–113. doi: 10.14719/pst.2014.1.3.64
Corso, M., Vannozzi, A., Maza, E., Vitulo, N., Meggio, F., Pitacco, A., et al. (2015). Comprehensive transcript profiling of two grapevine rootstock genotypes contrasting in drought susceptibility links the phenylpropanoid pathway to enhance tolerance. J. Exp. Bot. 66, 5739–5752. doi: 10.1093/jxb/erv274
Dabauza, M., Velasco, L., Pazos-Navarro, M., Pérez-Benito, E., Hellín, P., Flores, P., et al. (2015). Enhanced resistance to Botrytis cinerea in genetically-modified Vitis vinifera L. plants over-expressing the grapevine stilbene synthase gene. Plant Cell Tissue Organ. Cult. 120, 229–238. doi: 10.1007/s11240-014-0598-x
Dal Santo, S., Tornielli, G. B., Zenoni, S., Fasoli, M., Farina, L., Anesi, A., et al. (2013). The plasticity of the grapevine berry transcriptome. Genome Biol. 14:r54. doi: 10.1186/gb-2013-14-6-r54
Decker, V. H. G., Bandau, F., Gundale, M. J., Cole, C. T., and Albrectsen, B. R. (2016). Aspen phenylpropanoid genes' expression levels correlate with genets' tannin richness and vary both in responses to soil nitrogen and associations with phenolic profiles. Tree Physiol. 37, 1–10. doi: 10.1093/treephys/tpw118
Duan, D., Halter, D., Baltenweck, R., Tisch, C., Troster, V., Kortekamp, A., et al. (2015). Genetic diversity of stilbene metabolism in Vitis sylvestris. J. Exp. Bot. 66, 3242–3257. doi: 10.1093/jxb/erv137
Gambino, G., Cuozzo, D., Fasoli, M., Pagliarani, C., Vitali, M., Boccacci, P., et al. (2012). Co-evolution between Grapevine stem pitting-associated virus and Vitis vinifera L. leads to decreased defence responses and increased transcription of genes related to photosynthesis. J. Exp. Bot. 63, 5919–5933. doi: 10.1093/jxb/ers244
Grimplet, J., Van Hemert, J., Carbonell-Bejerano, P., Díaz-Riquelme, J., Dickerson, J., Fennel, A., et al. (2012). Comparative analysis of grapevine whole-genome gene predictions, functional annotation, categorization and integration of the predicted gene sequences. BMC Res. Notes 5:213. doi: 10.1186/1756-0500-5-213
Gòmez-Ariza, J., Campo, S., Rufat, M., Estopà, M., Messeguer, J., San Segundo, B., et al. (2007). Sucrose-mediated priming of plant defense responses and broad-spectrum disease resistance by overexpression of the maize pathogenesis-related PRms Protein in rice plants. Mol. Plant Microbe Interact. 20, 832–842. doi: 10.1094/MPMI-20-7-0832
Heil, M., and Silva Bueno, J. C. (2007). Within-plant signaling by volatiles leads to induction and priming of an indirect plant defense in nature. Proc. Natl. Acad. Sci. U.S.A. 104, 5467–5472. doi: 10.1073/pnas.0610266104
Heilmeier, H., Schulze, E. D., Jiang, F., and Hartung, W. (2007). General relations of stomatal responses to xylem sap abscisic acid under stress in the rooting zone: a global perspective. Flora 202, 624–636. doi: 10.1016/j.flora.2007.06.002
Houillé, B., Besseau, S., Delanoue, G., Oudin, A., Papon, N., Clastre, M., et al. (2015). Composition and tissue-specific distribution of stilbenoids in grape canes are affected by downy mildew pressure in the vineyard. J. Agric. Food Chem. 63, 8472–8477. doi: 10.1021/acs.jafc.5b02997
Houillé, B., Papon, N., Boudesocque, L., Bourdeaud, E., Besseau, S., Courdavault, V., et al. (2014). Antifungal activity of resveratrol derivatives against Candida species. J. Nat. Prod. 77, 1658–1662. doi: 10.1021/np5002576
Huot, B., Yao, J., Montgomery, B. L., and He, S. Y. (2014). Growth-defense tradeoffs in plants: a balancing act to optimize fitness. Mol. Plant 7, 1267–1287. doi: 10.1093/mp/ssu049
Jeandet, P., Douillet-Breuil, A. C., Bessis, R., Debord, S., Sbaghi, M., and Adrian, M. (2002). Phytoalexins from the Vitaceae: biosynthesis, phytoalexin gene expression in transgenic plants, antifungal activity, and metabolism. J. Agric. Food Chem. 50, 2731–2741. doi: 10.1021/jf011429s
Jensen, P. J., Halbrendt, N., Fazio, G., Makalowska, I., Altman, N., Praul, C., et al. (2012). Rootstock-regulated gene expression patterns associated with fire blight resistance in apple. BMC Genomics 13:9 doi: 10.1186/1471-2164-13-9
Kar, R. K. (2011). Plant responses to water stress: role of reactive oxygen species. Plant Signal. Behav. 6, 1741–1745. doi: 10.4161/psb.6.11.17729
Krisa, S., Larronde, F., Budzinski, H., Descendit, A., Deffieux, G., and Mérillon, J. M. (1999). Stilbene production by Vitis vinifera cell suspension cultures: methyljasmonate induction and 13C biolabeling. J. Nat. Prod. 62, 1688–1690. doi: 10.1021/np990239x
Latouche, G., Bellow, S., Poutaraud, A., Meyer, S., and Cerovic, Z. G. (2013). Influence of constitutive phenolic compounds on the response of grapevine (Vitis vinifera L.) leaves to infection by Plasmopara viticola. Planta 237, 351–361. doi: 10.1007/s00425-012-1776-x
Lemonnier, P., Gaillard, C., Veillet, F., Verbeke, J., Lemoine, R., Coutos-Thévenot, P., et al. (2014). Expression of Arabidopsis sugar transport protein STP13 differentially affects glucose transport activity and basal resistance to Botrytis cinerea. Plant Mol. Biol. 85, 473–484. doi: 10.1007/s11103-014-0198-5
Lovisolo, C., Lavoie-Lamoureux, A., Tramontini, S., and Ferrandino, A. (2016). Grapevine adaptations to water stress: new perspectives about soil/plant interactions. Theor. Exp. Plant Physiol. 28, 53–66. doi: 10.1007/s40626-016-0057-7
Lovisolo, C., Perrone, I., Hartung, W., and Schubert, A. (2008). An abscisic acid-related reduced transpiration promotes gradual embolism repair when grapevines are rehydrated after drought. New Phytol. 180, 642–651. doi: 10.1111/j.1469-8137.2008.02592.x
Manzi, M., Lado, J., Rodrigo, M. J., Zacarías, L., Arbona, V., and Gómez-Cadenas, A. (2015). Root ABA accumulation in long-term water-stressed plants is sustained by hormone transport from aerial organs. Plant Cell Physiol. 56, 2457–2466. doi: 10.1093/pcp/pcv161
Marè, C., Aprile, A., Roncaglia, E., Tocci, E., Corino, L. G., De Bellis, L., et al. (2013). Rootstock and soil induce transcriptome modulation of phenylpropanoid pathway in grape leaves. J. Plant Interact. 8, 334–349. doi: 10.1080/17429145.2012.754958
Marguerit, E., Brendel, O., Lebon, E., Van Leeuwen, C., and Ollat, N. (2012). Rootstock control of scion transpiration and its acclimation to water deficit are controlled by different genes. New Phytol. 194, 416–429. doi: 10.1111/j.1469-8137.2012.04059.x
Martinez-Medina, A., Flors, V., Heil, M., Mauch-Mani, B., Pieterse, C. M. J., Pozo, M. J., et al. (2016). Recognizing plant defense priming. Trends Plant Sci. 21, 818–822. doi: 10.1016/j.tplants.2016.07.009
McAdam, S. A. M., Brodribb, T. J., and Ross, J. J. (2016). Shoot derived abscisic acid promotes root growth. Plant Cell Environ. 39, 652–659. doi: 10.1111/pce.12669
Messina, J. F., Durhan, S. L., Richards, J. H., and McArthur, E. D. (2002). Trade-off between plant growyh and defense? A comparison of sagebrush populations. Oecologia 131, 43–51. doi: 10.1007/s00442-001-0859-3
Milcevicova, R., Gosch, C., Halbwirth, H., Stich, K., Hanke, M. V., Peil, A., et al. (2010). Erwinia amylovora- induced defense mechanisms of two apple species that differ in susceptibility to fire blight. Plant Sci. 179, 60–67. doi: 10.1016/j.plantsci.2010.04.013
Murillo, I., Roca, R., Bortolotti, C., and San Segundo, B. (2003). Engineering photoassimilate partitioning in tobacco plants improves growth and productivity and provides pathogen resistance. Plant J. 36, 330–341. doi: 10.1046/j.1365-313X.2003.01880.x
Pagliarani, C., Vitali, M., Ferrero, M., Vitulo, N., Incarbone, M., Lovisolo, C., et al. (2017). Accumulation of MicroRNAs differentially modulated by drought is affected by grafting in grapevine. Plant Physiol. 173, 2180–2195. doi: 10.1104/pp.16.01119
Pezet, R., Gindro, K., Viret, O., and Spring, J. L. (2004). Glycosilation and oxidative dimerization of resveratrol are respectively associated to sensitivity and resistance of grapevine cultivars to downy mildew. Physiol. Mol. Plant Pathol. 65, 297–303. doi: 10.1016/j.pmpp.2005.03.002
Pool, R. M., Creasy, L. L., and Frackelton, A. S. (1981). Resveratrol and the viniferins, their application to screening for disease resistance in grape breeding programs. Vitis 20, 136–145.
Puranik, S., Sahu, P., Srivastava, P. S., and Prasad, M. (2012). NAC proteins: regulation and role in stress tolerance. Trends Plant Sci. 17, 369–381. doi: 10.1016/j.tplants.2012.02.004
Rotter, A., Camps, C., Lohse, M., Kappel, C., Pilati, S., Hren, M., et al. (2009). Gene expression profiling in susceptible interaction of grapevine with its fungal pathogen Eutypa lata: extending MapMan ontology for grapevine. BMC Plant Biol. 9:104. doi: 10.1186/1471-2229-9-104
Savvides, A., Ali, S., Tester, M., and Fotopoulos, V. (2016). Chemical priming of plants against multiple abiotic stresses: mission possible? Trends Plant Sci. 21, 329–340. doi: 10.1016/j.tplants.2015.11.003
Siciliano, I., Carneiro, G. A., Spadaro, D., Garibaldi, A., and Gullino, M. L. (2015). Jasmonic acid, abscisic acid, and salicylic acid are involved in the phytoalexin responses of rice to Fusarium fujikuroi, a high gibberellin producer pathogen. J. Agr. Food Chem. 63, 8134–8142. doi: 10.1021/acs.jafc.5b03018
Siragusa, E., Weese, D., and Reinert, K. (2013). Fast and accurate read mapping with approximate seeds and multiple backtracking. Nucleic Acids Res. 41:e78. doi: 10.1093/nar/gkt005
Spence, C., and Bais, H. (2015). Role of plant growth regulators as chemical signals in plant–microbe interactions: a double edged sword. Curr. Opin. Plant Biol. 27, 52–58. doi: 10.1016/j.pbi.2015.05.028
Thimm, O., Blasing, O., Gibon, Y., Nagel, A., Meyer, S., Kruger, P., et al. (2004). MAPMAN: a user-driven tool to display genomics data sets onto diagrams of metabolic pathways and other biological processes. Plant J. 37, 914–939. doi: 10.1111/j.1365-313X.2004.02016.x
Tombesi, S., Nardini, A., Frioni, T., Soccolini, M., Zadra, C., Farinelli, D., et al. (2015). Stomatal closure is induced by hydraulic signals and maintained by ABA in drought-stressed grapevine. Sci. Rep. 5:12449. doi: 10.1038/srep12449
Tramontini, S., Vitali, M., Centioni, L., and Schubert, A. (2013). Rootstock control of scion response to water stress in grapevine. Environ. Exp. Bot. 93, 20–26. doi: 10.1016/j.envexpbot.2013.04.001
Trapnell, C., Roberts, A., Goff, L., Pertea, G., Kim, D., Kelley, D. R., et al. (2012). Differential gene and transcript expression analysis of RNA-seq experiments with TopHat and Cufflinks. Nat. Protoc. 7, 562–578. doi: 10.1038/nprot.2012.016
Vannozzi, A., Dry, I. B., Fasoli, M., Zenoni, S., and Lucchin, M. (2012). Genome-wide analysis of the grapevine stilbene synthase multigenic family: genomic organization and expression profiles upon biotic and abiotic stresses. BMC Plant Biol. 12:130 doi: 10.1186/1471-2229-12-130
Vincenzi, S., Tomasi, D., Gaiotti, F., Lovat, L., Giacosa, S., Torchio, F., et al. (2013). Comparative study of the resveratrol content of twenty-one Italian red grape varieties. S. Afr. J. Enol. Vitic. 34, 30–35.
Wang, J. F., Ma, L., Xi, H. F., Wang, L. J., and Li, S. H. (2015). Resveratrol synthesis under natural conditions and after UV-C irradiation in berry skin is associated with berry development stages in ‘Beihong’ (V. vinifera × V. amurensis). Food Chem. 168, 430–438. doi: 10.1016/j.foodchem.2014.07.025
Wang, M., Vannozzi, A., Wang, G., Liang, Y. H., Tornielli, G. B., Zenoni, S., et al. (2014). Genome and transcriptome analysis of the grapevine (Vitis vinifera L.) WRKY gene family. Hortic. Res. 1:16 doi: 10.1038/hortres.2014.16
Wang, M., Vannozzi, A., Wang, G., Zhong, Y., Corso, M., Cavallini, E., et al. (2015). A comprehensive survey of the grapevine VQ gene family and its transcriptional correlation with WRKY proteins. Front. Plant Sci. 6:417 doi: 10.3389/fpls.2015.00417
Wang, N., Zheng, Y., Xin, H., Fang, L., and Li, S. (2013). Comprehensive analysis of NAC domain transcription factor gene family in Vitis vinifera. Plant Cell Rep. 32, 61–75. doi: 10.1007/s00299-012-1340-y
Warschefsky, E., Klein, L. L., Frank, M. H., Chitwood, D. H., Londo, J. P., von Wettberg, E. J. B., et al. (2016). Rootstocks: diversity, domestication, and impacts on shoot phenotypes. Trends Plant Sci. 21, 418–437. doi: 10.1016/j.tplants.2015.11.008
Yang, Y., Mao, L., Jittayasothorn, Y., Kang, Y., Jiao, C., Fei, Z., and Zhong, G. (2015). Messenger RNA exchange between scions and rootstocks in grafted grapevines. BMC Plant Biol. 15:251 doi: 10.1186/s12870-015-0626-y
Zenoni, S., Ferrarini, A., Giacomelli, E., Xumerle, L., Fasoli, M., Malerba, G., et al. (2010). Characterization of transcriptional complexity during berry development in Vitis vinifera using RNA-seq. Plant Physiol. 152, 1785–1795. doi: 10.1104/pp.109.149716
Zhang, F., Liu, J., and Shi, J. S. (2010). Anti-inflammatory activities of resveratrol in the brain: role of resveratrol in microglial activation. Eur. J. Pharmacol. 636, 1–7. doi: 10.1016/j.ejphar.2010.03.043
Keywords: Vitis, abscisic acid, stilbene compounds, transcriptome, pathogens, Plasmopara viticola
Citation: Chitarra W, Perrone I, Avanzato CG, Minio A, Boccacci P, Santini D, Gilardi G, Siciliano I, Gullino ML, Delledonne M, Mannini F and Gambino G (2017) Grapevine Grafting: Scion Transcript Profiling and Defense-Related Metabolites Induced by Rootstocks. Front. Plant Sci. 8:654. doi: 10.3389/fpls.2017.00654
Received: 17 January 2017; Accepted: 10 April 2017;
Published: 27 April 2017.
Edited by:
Michael Wisniewski, Agricultural Research Service (USDA), Appalachian Fruit Research Station, USAReviewed by:
Gennaro Fazio, Agricultural Research Service (USDA), Plant Genetic Resources Unit, USAJessica Marie Guseman, Agricultural Research Service (USDA), USA
Copyright © 2017 Chitarra, Perrone, Avanzato, Minio, Boccacci, Santini, Gilardi, Siciliano, Gullino, Delledonne, Mannini and Gambino. This is an open-access article distributed under the terms of the Creative Commons Attribution License (CC BY). The use, distribution or reproduction in other forums is permitted, provided the original author(s) or licensor are credited and that the original publication in this journal is cited, in accordance with accepted academic practice. No use, distribution or reproduction is permitted which does not comply with these terms.
*Correspondence: Walter Chitarra, d2FsdGVyLmNoaXRhcnJhQGlwc3AuY25yLml0
Irene Perrone, aXJlbmUucGVycm9uZUBpcHNwLmNuci5pdA==
†These authors have contributed equally to this work.