- 1State Key Laboratory of Ecological Pest Control for Fujian and Taiwan Crops, Key Laboratory for Genetics, Breeding and Multiple Utilization of Crops, Fujian Agriculture and Forestry University, Fuzhou, China
- 2College of Life Science, Ningde Normal University, Ningde, China
- 3College of Life Science, Fujian Agriculture and Forestry University, Fuzhou, China
Kenaf (Hibiscus cannabinus L.), an environmental friendly and economic fiber crop, has a certain tolerance to abiotic stresses. Identification of reliable reference genes for transcript normalization of stress responsive genes expression by quantitative real-time PCR (qRT-PCR) is important for exploring the molecular mechanisms of plants response to abiotic stresses. In this study, nine candidate reference genes were cloned, and their expression stabilities were assessed in 132 abiotic stress and hormonal stimuli samples of kenaf using geNorm, NormFinder, and BestKeeper algorithms. Results revealed that HcPP2A (Protein phosphatase 2A) and HcACT7 (Actin 7) were the optimum reference genes across all samples; HcUBC (Ubiquitin-conjugating enzyme like protein) was the worst reference gene for transcript normalization. The reliability of the selected reference genes was further confirmed by evaluating the expression profile of HcWRKY28 gene at different stress durations. This work will benefit future studies on discovery of stress-tolerance genes and stress-signaling pathways in this important fiber crop.
Introduction
Being sessile, plants have to adapt and survive in various unfavorable environmental conditions. Water deficiency, excess salinity and extreme temperatures are major abiotic stresses that can occur at multiple stages of plant development, challenging production, and productivity of crop plants (Bray et al., 2000; Mahajan and Tuteja, 2005). In fact, soil desertification and salinization are rapidly increasing on a global scale, declining average yields for most major crop plants by more than 50% (Bartels and Sunkar, 2005). In response to these stress factors, plants can perceive abiotic stresses, and develop diverse signaling pathways comprising the regulation network of protein-protein reactions, protein-DNA interaction to combat or tolerate stresses. These well-known signals pathways including abscisic acid (ABA), ethylene (ET), jasmonic acid (JA), and salicylic acid (SA) pathways, which play vital roles in the plant developmental regulation process, and abiotic or biotic stresses alleviation (Mahajan and Tuteja, 2005; Bari and Jones, 2009). Therefore, elucidating the molecular mechanism of plant tolerance to adverse conditions is of fundamental importance to crop improvement.
Kenaf (Hibiscus cannabinus L.), belonging to the Malvaceae family, is an industrial crop holding high cellulosic fiber content, predominantly grown in Asia and Africa (Ayadi et al., 2011; Niu et al., 2015a). Kenaf is an appealing fiber source for the paper manufacture, owing to its environmentally friendly nature, such as biodegradability, renewability, and low energy consumption (Bhardwaj et al., 2005; Villar et al., 2009; Niu et al., 2015a). It has also a great potential for utilization in oil absorption, animal feed, and value-added industrial products (Ayadi et al., 2011; Niu et al., 2015a). Moreover, it is reported that kenaf could survive in areas with deficient water and well adapt to diverse adverse stresses, such as high salinity, drought and extreme temperature (Curtis and Läuchli, 1985; Francois et al., 1992; Banuelos et al., 2002). Thus, it is of fundamental importance to explicate the mechanism of fiber development and stress adaptation of Hibiscus cannabinus. However, limited genome sequence information is available, which greatly hinders kenaf functional gene discovery, expression profiling, and functional analysis, ultimately resulting in a slow improving advancement of kenaf fibers. Based on gene expression analysis, several stress response genes were recently identified and validated, which provides us a better reference to study the gene functions (Chuaqui et al., 2002; Zhu, 2002; Rasmussen et al., 2013). In this respect, qRT-PCR, a widely used high-throughout technique, was applied for validating microarray results and transcriptional expression of target genes, due to its high specificity, sensitivity and reproducibility (Bustin, 2002). To obtain an accurate expression data, one or more reference genes are introduced as normalization factors to lessen the variance. However, it is reported that unstable reference gene(s) used as normalization factor can lead to false inferences or misinterpretations of quantification results (Gutierrez et al., 2008; Guenin et al., 2009). Therefore, the stability of reference gene(s) should be systematically evaluated across different varieties, tissue samples, experimental treatments, and developmental stages prior an application in qRT-PCR analysis (Bustin, 2002; Guenin et al., 2009).
Currently, several studies have been performed in various fiber crops for selecting the stable reference genes across different tissue samples, developmental stages and abiotic/biotic stress conditions (Artico et al., 2010; Huis et al., 2010; Niu et al., 2015a,b). However, there is no systematic study performed in any fiber crop for reliable reference gene(s) selection under different hormonal stimuli and abiotic stresses, which limits further the functional gene studies and transcriptomic analysis.
Taken the need for normalization analysis into consideration, the present study was performed to identify and evaluate 9 candidate reference genes: 18S rRNA (18S ribosomal RNA), ACT7 (Actin 7), UBC (Ubiquitin-conjugating enzyme like protein), PTB (Polypyrimidine tract-binding protein), PP2A (Protein phosphatase 2A), TUB8 (Beta-tubulin 8), MZA (Clathrin adaptor complexes medium subunit family protein), RAN (Ras-related small GTP-binding protein), and EF1α (Elongation factor 1-alpha) in kenaf exposed to different abiotic stresses (salinity, drought and cold) and hormonal stimuli (ABA, ET, JA, and SA) at various stress durations. The expression stability of candidate reference genes was assessed by three statistical algorithms, namely, geNorm (Vandesompele et al., 2002), NormFinder (Andersen et al., 2004), and BestKeeper (Pfaffl et al., 2004). Furthermore, the HcWRKY28 gene of H. cannabinus was used to validate the reliability of the selected reference genes under different conditions.
Materials and Methods
Plant Materials and Treatments
Three kenaf varieties, Fuhong992, Fuhong952, and GV42, were used for stress treatments. Seeds were germinated on filter paper saturated with water, and seedlings were grown in nutritional soil in an artificial climate chamber (28°C, 16/8 h light/darkness; light intensity 300 μmol·m−2·s−1; relative humidity 50 ± 10%). For each variety, three 5-leaf-stage seedlings from the same experimental treatments were collected for 3 replicates. For the salinity and drought treatments, the details refer to the method of Niu et al. (2016). Cold treatment was performed by exposing seedlings to 4°C in light and harvested at the same time points. For the hormone treatments, leaves were sprayed with 0.3 mM abscisic acid (ABA treatment), 5.0 mM ethylene (ET treatment), 0.1 mM jasmonic acid (JA treatment), and 0.2 mM salicylic acid (SA treatment) and then harvested at the same time points above. All samples were harvested from three biological replicates in different pots, giving a total of 132 samples comprised of 57 abiotic and 75 hormone treatment samples for each variety. All samples were frozen in liquid nitrogen and stored at −80°C.
Total RNA Isolation and cDNA Synthesis
The OMEGA isolation kit (R6827-01, Omega Bio-tech, USA) was used for total RNA isolation. The concentration and purity of each RNA sample was estimated by a NanoDrop ND 2000 spectrophotometer (NanoDrop, Thermo Scientific). RNAs with an OD260/OD280 value between 1.9 and 2.1, and OD260/OD230 > 2.0 were used for further analyses. The first-strand cDNA was synthesized using the PrimeScript® RT reagent kit (TaKaRa, Japan) and stored at –20°C for further use.
Primer Design and PCR Products Verification
Nine candidate reference genes, 18S rRNA, ACT7, UBC, PTB, PP2A, TUB8, MZA, RAN, and EF1α, were screened from the Arabidopsis database. The potential homologs were identified by querying from the kenaf expressed sequence tags (ESTs) database and the kenaf transcriptome database (SRP060459) (Zhang et al., 2015). Based on these potential reference gene sequences, primers were designed for detection and cloning of reference genes using Primer v6. The detailed information of primers, such as GC content, melting temperatures, primer lengths, and amplicon lengths, were presented in Table 1. Cloning information was provided in Table S1. The specificity of PCR products were confirmed by agarose gel electrophoresis and sequencing.
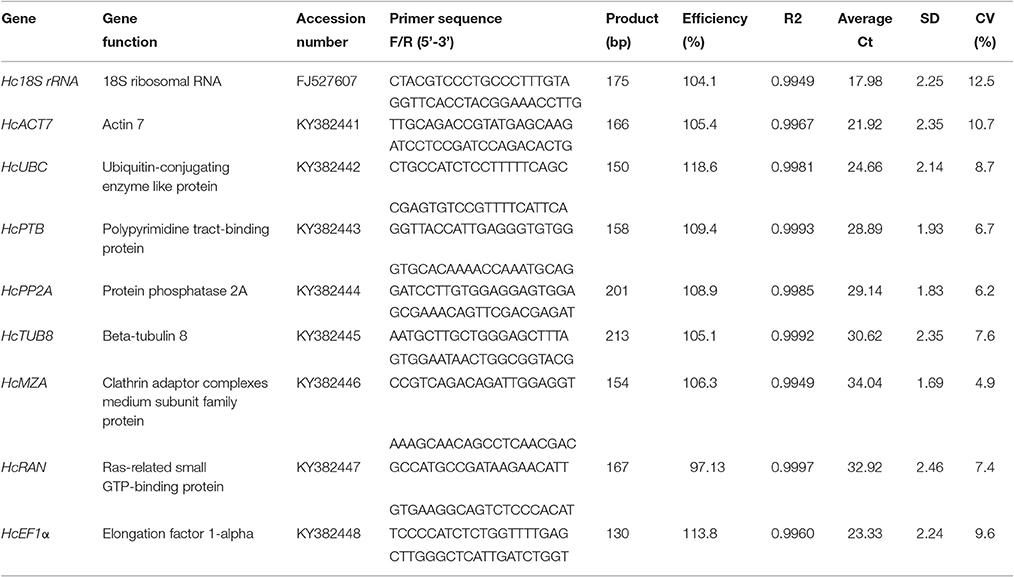
Table 1. Characteristics of nine candidate reference genes in H. cannabinus and parameters derived from qRT-PCR analysis.
qRT-PCR Assay
qRT-PCR was performed in 96-well plates by using a Applied Biosystems 7500 Real-Time PCR System (Applied Biosystems, USA). cDNA was amplified by using SYBR® Premix Ex Taq (Tli RNaseH Plus, TaKaRa, Japan), and a final volume of 20 μl reaction contains 10 μl 2 × SYBR Premix Ex Taq, 2 μl cDNA template, 0.4 μl each amplification primer, 0.4 μl ROX Reference Dye II, and 6.8 μl dH2O. Thermal cycling was performed with an initial step of 95°C for 30 s, 40 cycles at 95°C for 5 s, and 60°C for 34 s. The final melting curve was obtained from 60° to 95°C to verify primer specificity. All assays were carried out in three technical and biological replicates with template-free negative controls being performed in parallel. The amplification efficiency (E) and correlation coefficient (R2) were determined by the standard curves, based on the 10-fold cDNA diluted series.
Data Analysis
Three available statistical algorithms, geNorm, NormFinder, and BestKeeper were used for evaluating the expression stability of each candidate gene. All analyses were conducted following the operation instructions. The raw Ct values of all reference genes were transformed into the required data format. The relative quantities were calculated according to the formula: 2−ΔCt, where ΔCt = corresponding Ct—minimum Ct. A comprehensive ranking of reference genes was generated as suggested by Niu et al. (2015a,b). Statistical analysis of gene expression data were performed using the software SPSS 22.0 (SPSS Inc., USA).
Results
Cloning and Detection of Candidate Reference Genes
On the basis of the homology analysis with Arabidopsis genes, 9 candidate reference genes (Hc18S rRNA, HcACT7, HcUBC, HcPTB, HcPP2A, HcTUB8, HcMZA, HcRAN, and HcEF1α) were identified from the H. cannabinus transcriptome (SRP060459). The full-length cDNA sequences of these genes were cloned from H. cannabinus variety Fuhong992, and the sequence information was deposited in GenBank, and the accession numbers were showed in Table 1. Subsequently, specific primers were designed, and the specificity of the primers was confirmed on the basis of the amplification efficiency and specificity: (a) all primers were amplified with a single band after 1.5% agarose gel electrophoresis (Figure S1); (b) a single peak in the melting curve analysis; and (c) the primers amplification efficiency (E) ranged from 97.13 to 118.6%, and the correlation coefficients (R2) of the standard curve varied from 0.9949 to 0.9997 (Table 1 and Figure S2).
Reference Gene Expression Profiles
Transcript abundances of 9 candidate reference genes were assessed by qRT-PCR in 396 samples, and the Ct values were examined under three groups including abiotic stresses, hormonal stimuli, and all samples. Lower Ct values represent higher expression abundance, and higher Ct values represent lower expression abundance. As shown in Figure 1 and Table 1, the mean Ct values ranged from 17.98 to 34.04, with SD varied from 1.69 to 2.46 and CV from 4.9 to 12.5% among these 9 candidate genes. These data means that Hc18S rRNA expressed abundantly (Ct < 18); HcACT7, HcUBC, and HcEF1α were moderately expressed genes (21 < Ct < 24); HcPTB, HcPP2A, and HcTUB8 showed slightly lower expression levels (28 < Ct < 31); and HcRAN and HcMZA showed the lowest expression levels with Ct values as high as 32 and 34 cycles, respectively. Additionally, HcMZA showed the least variable gene (CV = 4.9%), followed by HcPP2A (6.2%), and Hc18S rRNA showed the most variability (12.5%) among the 9 candidate genes. Based on CV values, the stability ranking of all reference genes was as follows: HcMZA > HcPP2A > HcPTB > HcRAN > HcTUB8 > HcUBC > HcEF1α > HcACT7 > Hc18S rRNA (Table 1). In Brief, these data indicate that transcription levels of reference genes are unstable under different experimental conditions.
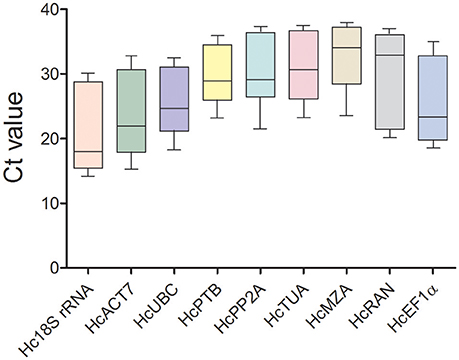
Figure 1. Ct values of 9 candidate reference genes in all experimental samples. The box indicates 25th and 75th percentiles. Lines across the box depict the median value. The whiskers represent the 5th and 95th percentiles.
Reference Genes Stability Analysis by geNorm
The geNorm statistical algorithm was used for evaluating expression stability of the 9 candidate references. This algorithm determines the gene expression stability (M) based on the average pairwise variation of all tested genes (Vandesompele et al., 2002; Liu et al., 2016). The lower M means the gene expresses more stable. For kenaf variety Fuhong992, the candidate genes in each experimental group showed much lower M values than the threshold value of 1.5. Therefore, HcPP2A and HcPTB (M = 0.08) were ranked as the most stable, HcUBC (M = 0.19) was the least gene in the abiotic stress group. Under the hormonal stimuli conditions, HcACT7 and Hc18S rRNA (M = 0.10), followed by HcTUB8 (M = 0.11), were identified as the most stable genes, HcUBC (M = 0.26) showed the worst stability. When all samples was considered, Hc18S rRNA and HcACT7 (M = 0.09) featured the most stable genes whereas HcEF1α (M = 0.21) and HcUBC (M = 0.25) were the least genes (Table 2). For kenaf varieties Fuhong952, HcPTB and HcEF1α (M = 0.03) were listed as the best genes, HcUBC and HcMZA were the worst in the abiotic stress group. Under the hormonal stimuli conditions, HcACT7 and HcTUB8 (M = 0.02), followed by HcPP2A (M = 0.04), were identified as the most stable genes, while HcUBC (M = 0.13) gene showed the worst stability. As to total samples, HcPP2A and Hc18S rRNA (M = 0.07) featured the most stable genes whereas HcTUB8 (M = 0.21) was the least (Table S2). For kenaf varieties GV42, HcPP2A and HcACT7 expressed stably, HcUBC and HcEF1α expressed unstably under abiotic stresses, hormonal stimuli, and total samples. (Table S3).
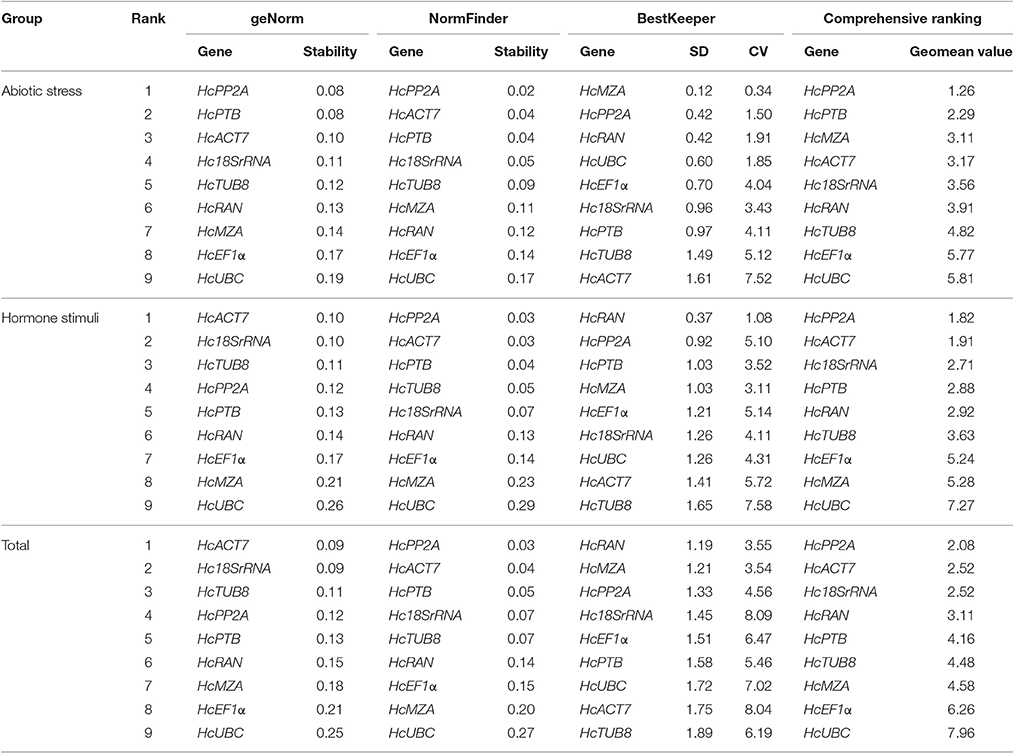
Table 2. geNorm, NormFinder, BestKeeper and Comprehensive ranking analyzed the gene expression stability in kenaf.
The optimal number of reference genes was also determined by geNorm algorithm based on the pairwise variation (Vn) between normalization factors (NFn). The threshold value (Vn/Vn+1 = 0.15) indicates that the number of reference genes less than or equal to the value of n is sufficient to use as reference gene. As depicted in Figure 2, the pairwise variation value V2/V3 of all experimental samples was less than 0.15, suggesting that two reference genes should be sufficient for normalization under these conditions.
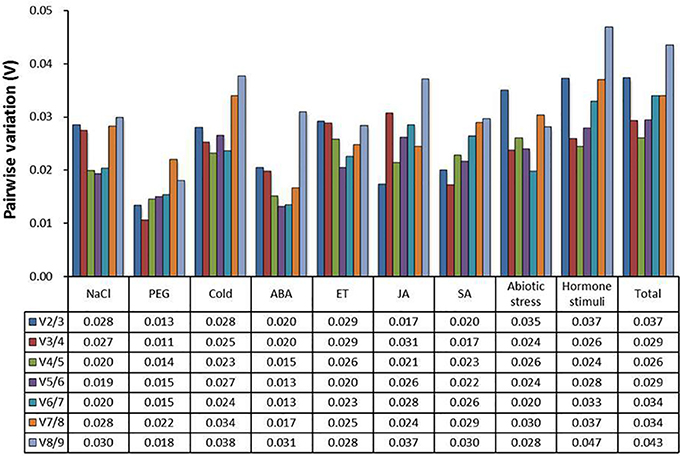
Figure 2. Pairwise variation (V) of candidate reference genes calculated by geNorm. Pairwise variation (Vn/Vn+1) was calculated between normalization factors NFn and NFn+1 to determine the optimal number of reference genes. “Abiotic stress” includes NaCl, PEG, and Cold. “Hormone stimuli” includes ABA, ET, JA, and SA. “Total” represents all samples.
Reference Genes Stability Analysis by NormFinder
The NormFinder approach determines the stability of candidate reference genes based on inter- and intra-group variations in expression across different sample groups (Andersen et al., 2004). Similar to the geNorm analysis, the lower M value means the gene expressed more stable. We obtained the similar results through the NormFinder analysis, which predicted HcPP2A, HcACT7, and HcPTB to be the three stable genes for abiotic stress group, hormonal stimuli group, and the total samples group in Fuhong992 and GV42 (Table 2; Table S3). For kenaf varieties Fuhong952, HcPP2A, Hc18S rRNA, HcPTB, HcACT7 were the most stable reference genes under different conditions (Table S2). Overall, HcUBC and HcMZA were ranked as the worst reference gene, which was consistently found in the three subsets.
Reference Genes Stability Analysis by BestKeeper
The BestKeeper program was applied for evaluating the candidate references stability based on CV and SD of Ct values (Pfaffl et al., 2004). The lower CV ± SD value, the more stable expressed. The analysis results of BestKeeper were also listed in Table 2. For kenaf variety Fuhong992, HcMZA (CV ± SD = 0.34 ± 0.12) and HcPP2A (CV ± SD = 1.50 ± 0.42) showed remarkably stable expression in the abiotic stress group. HcRAN (1.08 ± 0.37) and HcPP2A (5.10 ± 0.92) were identified as the two best reference genes in the different hormonal stimuli group. When all samples were analyzed together, the two reference genes, HcRAN (3.35 ± 1.19) and HcMZA (3.54 ± 1.21), showed the highest expression stabilities in the total group (Table 2). For kenaf variety Fuhong952, HcRAN (0.71 ± 0.24) and HcEF1α (1.45 ± 0.29) showed stable expression in the abiotic stress group. HcRAN (0.32 ± 0.10) and HcPP2A (2.36 ± 0.65) were identified as the two best reference genes in hormonal stimuli group. HcPTB (2.35 ± 0.64) and HcPP2A (2.37 ± 0.66) showed the highest expression stabilities in the total group (Table S2). For kenaf variety GV42, HcRAN and HcTUB8 were the two best reference genes in abiotic stress group, hormonal stimuli group, and total group (Table S3). All these results were inconsistent with those obtained from the geNorm and NormFinder analyses.
Comprehensive Stability Analysis
To develop a consistent result, a comprehensive ranking of reference genes was obtained according to the method described by Niu et al. (2015b). The results were shown in Table 2, Tables S2, S3 for Fuhong992, Fuhong952, and GV42, respectively. Based on geNorm algorithm, the best combination of reference gene in each group was further obtained. As shown in Table 3, HcPP2A and HcPTB were the most stable references under abiotic stress condition; HcPP2A and HcACT7 in the different hormonal stimuli and total samples groups for kenaf variety Fuhong992. For Fuhong952, HcRAN, HcPP2A, and HcPTB were listed as the most stable genes in the abiotic stress group; HcPP2A and HcACT7 in the different hormonal stimuli group; HcPP2A and HcPTB, followed by Hc18S rRNA in the total samples group. For GV42 variety, HcPP2A and HcACT7 showed the optimal performance in the abiotic stress group, hormonal stimuli group, and the total samples group (Table 3).
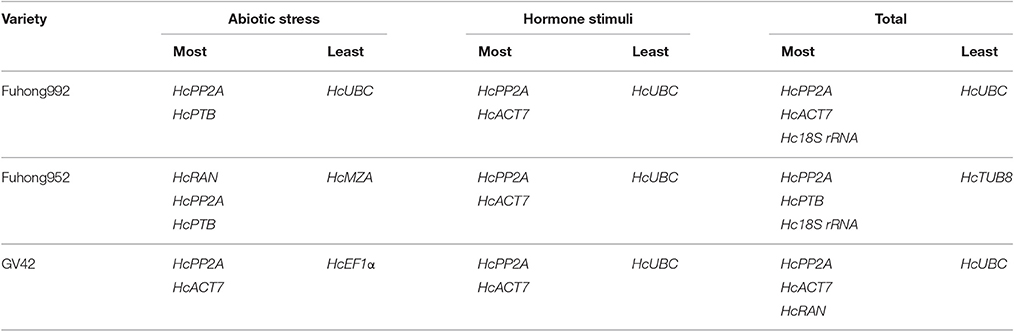
Table 3. Suitable reference genes ranked by geNorm and comprehensive analysis in different kenaf varieties.
Validation of the Selected Reference Genes
To confirm the reliability of the selected references, the relative expression level of HcWRKY28 was normalized using the most stable and the least stable reference genes by qRT-PCR analysis in kenaf variety Fuhong992. As shown in Figure 3, the relative transcript abundance of HcWRKY28 under different abiotic stress or hormonal stimuli was found to be impartial when HcPP2A, HcPTB, and HcACT7 or their combinations (HcPP2A and HcPTB or HcACT7) were used as normalization factors. Using references HcPP2A, HcPTB, or combination of HcPP2A and HcPTB, the HcWRKY28 gene was significantly up-regulated under salinity and cold stress at 8 h, and was down-regulated under drought stress at 6, 8, and 12 h (Figure 3A). Correspondingly, for different hormonal stimuli, the HcWRKY28 showed relative up-regulation (JA and SA stimuli) or down-regulation (ABA and ET stimuli) at each time point when HcPP2A, HcACT7 or their combination were used for transcript normalization (Figure 3B). However, when the worst reference gene HcUBC used as an internal control, the expression pattern of HcWRKY28 showed strong fluctuations, and the gene expression change level was 5-fold higher than that by the most stable genes under different abiotic stress and hormonal stimuli (Figure 3).
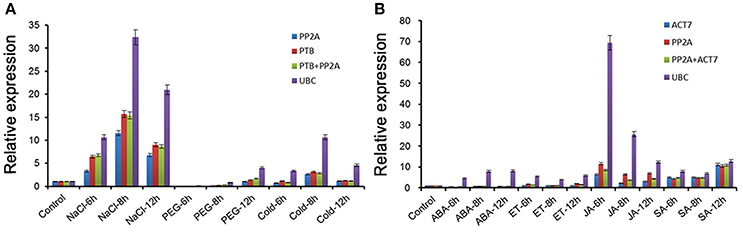
Figure 3. Relative expression of HcWRKY28 using the selected reference genes. Results were normalized using the selected stable references (singly or in combination) and the unstable reference gene in sample sets across (A) abiotic stress-, and (B) hormonal stimuli-treated samples at 0, 6, 8, 12 h. Bar indicates the standard error (±SE) evaluated from three biological replicates.
Discussion
Agricultural productivity worldwide is challenged by the unfavorable environmental factors, such as drought, salinity and extreme temperatures due to their high intensity of impact and wide distribution (Bartels and Sunkar, 2005; Mittler, 2006). Being sessile, plants have to develop a series of complicated mechanisms to resist and/or adapt to adverse conditions. At the molecular level, transcriptomics and functional genomics approaches have been implemented for understanding the defense mechanisms of plant response to unfavorable environmental conditions. qRT-PCR has become the most powerful method for detection of transcriptomics data and study the molecular mechanisms of plant stress responses. However, accurate gene expression data by qRT-PCR analysis relies on the stable normalization factors. Using a stable reference gene as the normalization factor will ensure the qRT-PCR data to be reliable for target genes. On the contrary, the use of an inappropriate internal reference gene will result in deviation.
To avoid bias introduced, nine commonly used reference genes were cloned from kenaf for evaluation of their expression stability under different stress conditions in the present study. As expected, the distinct stability rankings were generated among the three groups, owing to the different statistical algorithms. For instance, HcPP2A and HcPTB were ranked as the most stable references in abiotic stress group by geNorm algorithm; HcPP2A and HcPTB/HcACT7 by NormFinder; while HcMZA and HcPP2A/HcRAN by BestKeeper. The similar inconsistence was also observed in hormonal stimuli and total groups. To obtain an accordant result, the stability rankings ranked by three algorithms were integrated, generating a comprehensive ranking. It was recommended that HcPP2A and HcACT7 were the top two most stable reference genes in all samples groups. HcPP2A also paired with either HcPTB or HcACT7 as the most stable gene by geNorm algorithm across the abiotic stress samples, hormonal stimuli or total samples, respectively. Taken together, our results further confirmed several previous studies, where PP2A was also selected as the best normalization factor. For example, PP2A expressed stably in Pennisetum glaucum under different abiotic stresses and hormonal stimuli (Saha and Blumwald, 2014; Shivhare and Lata, 2016), Caragana intermedia under osmotic and heat stress (Zhu et al., 2013), Agrostis stolonifera under different abiotic stresses (Chen et al., 2015), Brassica napus under drought, salinity and JA stresses (Wang et al., 2014b), Cynodon dactylon under different abiotic stresses (Chen et al., 2014). ACT7, one of the best reference genes in this study, was also stably expressed under different abiotic stresses and hormonal stimuli of Daucus carota (Tian et al., 2015), JA and ABA stresses of Brassica napus (Wang et al., 2014b), drought stress of Populus euphratica (Wang et al., 2014a), and Agrostis stolonifera (Chen et al., 2015). Another stable gene is PTB, a novel reference gene identified in A. thaliana, has been identified as one of the best reference for Gossypium hirsutum (Artico et al., 2010) under abiotic stress. Hc18S rRNA, HcMZA, HcRAN, and HcTUB8 were regarded as unsuitable genes due to their expression variability, thereby rendering them inappropriate to be reference genes. However, good performance of Hc18SrRNA under salinity and drought stress conditions was reported in another study of kenaf (Niu et al., 2015a). The possible reason might be that the cold stress samples added and merged into a new stress combination, and then analyzed by different statistical algorithms, finally generating different results. The MZA gene showed high stability in G. hirsutum (Artico et al., 2010), Phyllostachys edulis (Fan et al., 2013), and Solanum lycopersicon (Exposito-Rodriguez et al., 2008); but low stability in our study though BestKeeper algorithm ranked it 1st position in abiotic stress samples. RAN was also the optimal performer in Cucumis melo (Kong et al., 2014) treated with growth regulators but was variable under different conditions in the present study. EF1α was previously reported as the most stably expressed reference gene in many species, for example, Glycine max under drought and salinity stresses (Saraiva et al., 2014), Solanum tuberosum under abiotic stresses (Nicot et al., 2005), Saccharum spp. under drought and salinity stresses (Guo et al., 2014), Pennisetum glaucum under individual and multiple abiotic stresses (Shivhare and Lata, 2016). However, HcEF1α was recognized as the least stable gene by the three algorithms, and UBC, a commonly used reference gene, was also consistently ranked as the worst gene by geNorm and NormFinder across all samples groups in this study.
To validate the availability of the selected references, the expression levels of HcWRKY28 gene were normalized by using the selected reference genes. The HcWRKY28 showed consistent amplification profiles when the two most stable genes were used as internal controls either singly or in combination for both abiotic stresses and hormonal stimuli. While severe disparities occurred when the least stable gene HcUBC was used for normalization. These results indicated that HcPP2A singly or in combination with HcACT7 or HcPTB are suitable reference gene(s) for transcript normalization under different abiotic stresses, hormonal stimuli and different kenaf varieties. It is without any doubt that the uncharacterized reference genes may also be the better candidates for transcript normalization in kenaf under different conditions. To our knowledge, the present study firstly provides a systematic evaluation on reliability of reference genes used for qRT-PCR normalization in any fiber crop under various abiotic stresses and hormonal stimulus at different stress durations. Therefore, this work will facilitate the accurate qRT-PCR gene expression studies on cross-talking mechanisms between abiotic stresses and hormone signaling pathway in kenaf.
Author Contributions
XN and JQ initiated and designed the research. XN, MC, XH, and HC performed the experiments. XN, AT, and JX analyzed the data. XN, MC, and HC prepared reagents/materials. XN wrote the paper. XN and JQ revised paper.
Conflict of Interest Statement
The authors declare that the research was conducted in the absence of any commercial or financial relationships that could be construed as a potential conflict of interest. The reviewer YQ declared a shared affiliation, though no other collaboration, with several of the authors XN, XH, HC, AT, JX, JQ to the handling Editor, who ensured that the process nevertheless met the standards of a fair and objective review.
Acknowledgments
The research was supported by the National bast fiber germplasm resources project of China (K47NI201A), 948 project of the Agricultural Department of China (2013-Z70), National bast fiber research system of China (CARS-19-E06) and Weng Hongwu Academic Innovation Research Fund of Peking University.
Supplementary Material
The Supplementary Material for this article can be found online at: http://journal.frontiersin.org/article/10.3389/fpls.2017.00771/full#supplementary-material
References
Andersen, C. L., Jensen, J. L., and Ørntoft, T. F. (2004). Normalization of real-time quantitative reverse transcription-PCR data: a model-based variance estimation approach to identify genes suited for normalization, applied to bladder and colon cancer data sets. Cancer Res. 64, 5245–5250. doi: 10.1158/0008-5472.CAN-04-0496
Artico, S., Nardeli, S. M., Brilhante, O., Grossi-De-Sa, M. F., and Alves-Ferreira, M. (2010). Identification and evaluation of new reference genes in Gossypium hirsutum for accurate normalization of real-time quantitative RT-PCR data. BMC Plant Biol. 10:49. doi: 10.1186/1471-2229-10-49
Ayadi, R., Hamrouni, L., Hanana, M., Bouzid, S., Trifi, M., and Khouja, M. L. (2011). In vitro propagation and regeneration of an industrial plant kenaf (Hibiscus cannabinus L.). Ind. Crops Prod. 33, 474–480. doi: 10.1016/j.indcrop.2010.10.025
Banuelos, G. S., Bryla, D. R., and Cook, C. G. (2002). Vegetative production of kenaf and canola under irrigation in central California. Ind. Crops Prod. 15, 237–245. doi: 10.1016/S0926-6690(01)00119-4
Bari, R., and Jones, J. D. (2009). Role of plant hormones in plant defence responses. Plant Mol. Biol. 69, 473–488. doi: 10.1007/s11103-008-9435-0
Bartels, D., and Sunkar, R. (2005). Drought and salt tolerance in plants. Crit. Rev. Plant Sci. 24, 23–58. doi: 10.1080/07352680590910410
Bhardwaj, H. L., Webber, C. L., and Sakamoto, G. S. (2005). Cultivation of kenaf and sunn hemp in the mid-Atlantic United States. Ind. Crops Prod. 22, 151–155. doi: 10.1016/j.indcrop.2004.08.002
Bray, E. A., Bailey-Serres, J., and Weretilnyk, E. (2000). Responses to abiotic stresses. Biochem. Mol. Biol. Plants 1158:e1203.
Bustin, S. A. (2002). Quantification of mRNA using real-time reverse transcription PCR (RT-PCR): trends and problems. J. Mol. Endocrinol. 29, 23–39. doi: 10.1677/jme.0.0290023
Chen, Y., Hu, B., Tan, Z., Liu, J., Yang, Z., Li, Z., et al. (2015). Selection of reference genes for quantitative real-time PCR normalization in creeping bentgrass involved in four abiotic stresses. Plant Cell Rep. 34, 1825–1834. doi: 10.1007/s00299-015-1830-9
Chen, Y., Tan, Z., Hu, B., Yang, Z., Xu, B., Zhuang, L., et al. (2014). Selection and validation of reference genes for target gene analysis with quantitative RT-PCR in leaves and roots of bermudagrass under four different abiotic stresses. Physiol. Plant 155, 138–148. doi: 10.1111/ppl.12302
Chuaqui, R. F., Bonner, R. F., Best, C. J., Gillespie, J. W., Flaig, M. J., Hewitt, S. M., et al. (2002). Post-analysis follow-up and validation of microarray experiments. Nat. Genet. 32, 509–514. doi: 10.1038/ng1034
Curtis, P. S., and Läuchli, A. (1985). Responses of kenaf to salt stress: germination and vegetative growth. Crop Sci. 25, 944–949. doi: 10.2135/cropsci1985.0011183X002500060011x
Expósito-Rodríguez, M., Borges, A. A., Borges-Pérez, A., and Pérez, J. A. (2008). Selection of internal control genes for quantitative real-time RT-PCR studies during tomato development process. BMC Plant Biol. 8:131. doi: 10.1186/1471-2229-8-131
Fan, C., Ma, J., Guo, Q., Li, X., Wang, H., and Lu, M. (2013). Selection of reference genes for quantitative real-time PCR in bamboo (Phyllostachys edulis). PLoS ONE 8:e56573. doi: 10.1371/journal.pone.0056573
Francois, L. E., Donovan, T. J., and Maas, E. V. (1992). Yield, vegetative growth, and fiber length of kenaf grown on saline soil. Agron. J. 84, 592–598. doi: 10.2134/agronj1992.00021962008400040010x
Guénin, S., Mauriat, M., Pelloux, J., Van Wuytswinkel, O., Bellini, C., and Gutierrez, L. (2009). Normalization of qRT-PCR data: the necessity of adopting a systematic, experimental conditions-specific, validation of references. J. Exp. Bot. 60, 487–493. doi: 10.1093/jxb/ern305
Guo, J., Ling, H., Wu, Q., Xu, L., and Que, Y. (2014). The choice of reference genes for assessing gene expression in sugarcane under salinity and drought stresses. Sci. Rep. 4:7042. doi: 10.1038/srep07042
Gutierrez, L., Mauriat, M., Guénin, S., Pelloux, J., Lefebvre, J. F., Louvet, R., et al. (2008). The lack of a systematic validation of reference genes: a serious pitfall undervalued in reverse transcription-polymerase chain reaction (RT-PCR) analysis in plants. Plant Biotechnol. J. 6, 609–618. doi: 10.1111/j.1467-7652.2008.00346.x
Huis, R., Hawkins, S., and Neutelings, G. (2010). Selection of reference genes for quantitative gene expression normalization in flax (Linum usitatissimum L.). BMC Plant Biol. 10:71. doi: 10.1186/1471-2229-10-71
Kong, Q., Yuan, J., Niu, P., Xie, J., Jiang, W., Huang, Y., et al. (2014). Screening suitable reference genes for normalization in reverse transcription quantitative real-time PCR analysis in melon. PLoS ONE 9:e87197. doi: 10.1371/journal.pone.0087197
Liu, Q., Wei, C., Zhang, M.-F., and Jia, G.-X. (2016). Evaluation of putative reference genes for quantitative real-time PCR normalization in Lilium regale during development and under stress. PeerJ 4:e1837. doi: 10.7717/peerj.1837
Mahajan, S., and Tuteja, N. (2005). Cold, salinity and drought stresses: an overview. Arch. Biochem. Biophys. 444, 139–158. doi: 10.1016/j.abb.2005.10.018
Mittler, R. (2006). Abiotic stress, the field environment and stress combination. Trends Plant Sci. 11, 15–19. doi: 10.1016/j.tplants.2005.11.002
Nicot, N., Hausman, J. F., Hoffmann, L., and Evers, D. (2005). Housekeeping gene selection for real-time RT-PCR normalization in potato during biotic and abiotic stress. J. Exp. Bot. 56, 2907–2914. doi: 10.1093/jxb/eri285
Niu, X., Chen, M., Tao, A., and Qi, J. (2016). Salinity and drought treatment assays in kenaf (Hibiscus cannabinus L.). Bio-protocol 6:e1918. doi: 10.21769/bioprotoc.1918
Niu, X., Qi, J., Chen, M., Zhang, G., Tao, A., Fang, P., et al. (2015a). Reference genes selection for transcript normalization in kenaf (Hibiscus cannabinus L.) under salinity and drought stress. PeerJ 3:e1347. doi: 10.7717/peerj.1347
Niu, X., Qi, J., Zhang, G., Xu, J., Tao, A., Fang, P., et al. (2015b). Selection of reliable reference genes for quantitative real-time PCR gene expression analysis in Jute (Corchorus capsularis) under stress treatments. Front. Plant Sci. 6:848. doi: 10.3389/fpls.2015.00848
Pfaffl, M. W., Tichopad, A., Prgomet, C., and Neuvians, T. P. (2004). Determination of stable housekeeping genes, differentially regulated target genes and sample integrity: BestKeeper–Excel-based tool using pair-wise correlations. Biotechnol. Lett. 26, 509–515. doi: 10.1023/B:BILE.0000019559.84305.47
Rasmussen, S., Barah, P., Suarez-Rodriguez, M. C., Bressendorff, S., Friis, P., Costantino, P., et al. (2013). Transcriptome responses to combinations of stresses in Arabidopsis. Plant Physiol. 161, 1783–1794. doi: 10.1104/pp.112.210773
Saha, P., and Blumwald, E. (2014). Assessing reference genes for accurate transcript normalization using quantitative real-time PCR in pearl millet [Pennisetum glaucum (L.) R. Br]. PLoS ONE 9:e106308. doi: 10.1371/journal.pone.0106308
Saraiva, K. D., Fernandes De Melo, D., Morais, V. D., Vasconcelos, I. M., and Costa, J. H. (2014). Selection of suitable soybean EF1alpha genes as internal controls for real-time PCR analyses of tissues during plant development and under stress conditions. Plant Cell Rep. 33, 1453–1465. doi: 10.1007/s00299-014-1628-1
Shivhare, R., and Lata, C. (2016). Selection of suitable reference genes for assessing gene expression in pearl millet under different abiotic stresses and their combinations. Sci. Rep. 6:23036. doi: 10.1038/srep23036
Tian, C., Jiang, Q., Wang, F., Wang, G. L., Xu, Z. S., and Xiong, A. S. (2015). Selection of suitable reference genes for qPCR normalization under abiotic stresses and hormone stimuli in carrot leaves. PLoS ONE 10:e0117569. doi: 10.1371/journal.pone.0117569
Vandesompele, J., De Preter, K., Pattyn, F., Poppe, B., Van Roy, N., De Paepe, A., et al. (2002). Accurate normalization of real-time quantitative RT-PCR data by geometric averaging of multiple internal control genes. Genome Biol. 3:RESEARCH0034. doi: 10.1186/gb-2002-3-7-research0034
Villar, J. C., Revilla, E., Gómez, N., Carbajo, J. M., and Simón, J. L. (2009). Improving the use of kenaf for kraft pulping by using mixtures of bast and core fibers. Ind. Crops Prod. 29, 301–307. doi: 10.1016/j.indcrop.2008.06.002
Wang, H. L., Chen, J., Tian, Q., Wang, S., Xia, X., and Yin, W. (2014a). Identification and validation of reference genes for Populus euphratica gene expression analysis during abiotic stresses by quantitative real-time PCR. Physiol. Plant. 152, 529–545. doi: 10.1111/ppl.12206
Wang, Z., Chen, Y., Fang, H., Shi, H., Chen, K., Zhang, Z., et al. (2014b). Selection of reference genes for quantitative reverse-transcription polymerase chain reaction normalization in Brassica napus under various stress conditions. Mol. Genet. Genomics 289, 1023–1035. doi: 10.1007/s00438-014-0853-1
Zhang, L., Wan, X., Xu, J., Lin, L., and Qi, J. (2015). De novo assembly of kenaf (Hibiscus cannabinus) transcriptome using Illumina sequencing for gene discovery and marker identification. Mol. Breed. 35:192. doi: 10.1007/s11032-015-0388-0
Zhu, J. K. (2002). Salt and drought stress signal transduction in plants. Annu. Rev. Plant Biol. 53, 247–273. doi: 10.1146/annurev.arplant.53.091401.143329
Keywords: reference gene, kenaf (Hibiscus cannabinus L.), abiotic stress, hormonal stimulus, gene expression
Citation: Niu X, Chen M, Huang X, Chen H, Tao A, Xu J and Qi J (2017) Reference Gene Selection for qRT-PCR Normalization Analysis in kenaf (Hibiscus cannabinus L.) under Abiotic Stress and Hormonal Stimuli. Front. Plant Sci. 8:771. doi: 10.3389/fpls.2017.00771
Received: 21 September 2016; Accepted: 25 April 2017;
Published: 12 May 2017.
Edited by:
Roger Deal, Emory University, USAReviewed by:
M. Teresa Sanchez-Ballesta, Instituto de Ciencia y Tecnología de Alimentos y Nutrición (ICTAN-CSIC), SpainYuan Qin, Fujian Agriculture and Forestry University, China
Copyright © 2017 Niu, Chen, Huang, Chen, Tao, Xu and Qi. This is an open-access article distributed under the terms of the Creative Commons Attribution License (CC BY). The use, distribution or reproduction in other forums is permitted, provided the original author(s) or licensor are credited and that the original publication in this journal is cited, in accordance with accepted academic practice. No use, distribution or reproduction is permitted which does not comply with these terms.
*Correspondence: Xiaoping Niu, eHBuaXUwNjEzQDEyNi5jb20=
Jianmin Qi, cWlqbTg2M0AxNjMuY29t