- 1Key Laboratory of Vegetation Restoration and Management of Degraded Ecosystems, South China Botanical Garden, Chinese Academy of Sciences, Guangzhou, China
- 2Guangdong Provincial Key Laboratory of Applied Botany, South China Botanical Garden, Chinese Academy of Sciences, Guangzhou, China
- 3College of Life Sciences, University of Chinese Academy of Sciences, Beijing, China
It is well-known that drought has considerable effects on plant traits from leaf to ecosystem scales; however, little is known about the relative contributions of various traits within or between tree species in determining the plant’s sensitivity or the tolerance to drought under field conditions. We conducted a field throughfall exclusion experiment to simulate short-term drought (∼67% throughfall exclusion during the dry season from October to March) and prolonged drought (∼67% throughfall exclusion prolonging the dry season from October to May) and to understand the effects of drought on two dominant tree species (Michelia macclurei and Schima superba) in subtropical forests of southern China. The morphological, physiological, and nutritional responses of the two species to the two types of drought were determined. There were significantly different morphological (leaf max length, max width, leaf mass per area), physiological (leaf proline) and nutritional (P, S, N, K, Ca, Mg) responses by M. macclurei and S. superba to prolonged drought. Comparison between the drought treatments for each species indicated that the trees responded species–specifically to the short-term and prolonged drought, with S. superba exhibiting larger plasticity and higher adaption than M. macclurei. M. macclurei responded more sensitively to prolonged drought in terms of morphology, proline content, and nutritional traits and to short-term drought with regard to soluble sugars content. The differential species-specific responses to drought will allow us to estimate the changes in dominant trees in subtropical forests of China that have experienced a decade’s worth of annual seasonal drought.
Introduction
Both global climate models (Fischer et al., 2013; Singh et al., 2013) and observed precipitation trends (Marvel and Bonfils, 2013) have revealed that climate change is intensifying the hydrologic cycle and is expected to increase the variation in precipitation regimes worldwide. The variation in precipitation patterns at both regional and global scales will occur with great spatio–temporal variability and complexity (Beier et al., 2012). At a global scale, the amount of rainfall is predicted to increase at mid– and high–latitudes and to decrease at low latitudes, with notable changes in seasonal intensity and frequency (Greve et al., 2014). Regionally, e.g., in south China, it will be drier in the dry season and wetter in the wet season with ongoing climate change (Zhou et al., 2013). Accordingly, the dry season has lasted longer and the wet season has had more intensive rainstorms in the last 10 years, despite the total annual precipitation amount not changing significantly, in some regions of south China (Zhou et al., 2011).
Changes in precipitation regimes have considerable ecological and evolutionary impacts on terrestrial ecosystems (Pratt and Mooney, 2013; Moran et al., 2014; Zeppel et al., 2014; Knapp et al., 2015). Physiological properties of plant species (Coe and Sparks, 2014); encroachment of woody plants (Kulmatiski and Beard, 2013); tree mortality (Anderegg et al., 2015); forest structure (Hoeppner and Dukes, 2012); evapotranspiration dynamics (Raz-Yaseef et al., 2012); plant community composition (Cleland et al., 2013); biomass and net primary productivity (Hovenden et al., 2014; Wilcox et al., 2015); litterfall (Travers and Eldridge, 2013); the availability, uptake, transport, and accumulation of plant nutrients (including N, P, S, K, Ca, and Mg) (Rouphael et al., 2012); soil respiration (Thomey et al., 2011); and soil microbial communities (Zeglin et al., 2013), have been well documented to be affected by the rainfall patterns.
Plant functional traits, e.g., morphological, bio-physiological, and nutritional traits of leaves and roots—can individually or in combination, indicate the responses of plants to environmental changes (Wright et al., 2005; Meng et al., 2009; Pratt and Mooney, 2013), and further provide insights into the adaptation strategies and survival abilities of plants (Picotte et al., 2009). Droughts have considerable effects on plant functional traits from leaf to ecosystem scales (Moreno–Gutiérrez et al., 2012; Flanagan and Farquhar, 2014). However, the drought responses of dominant species in tropical and subtropical forests to drought remains relatively poorly understood (Beier et al., 2012), since knowledge of direct and indirect effects of droughts has usually been derived from studies of pot experiments with seedlings, whose results are generally inconsistent with field manipulations of mature trees (Liu et al., 2011). Pot experiments may have hindered the understanding of drought responses among species. Throughfall exclusion experiments (TEE) and throughfall displacement experiments (TDE) in forest ecosystems (Borken et al., 2006; Nepstad et al., 2007; Gaul et al., 2008; da Costa et al., 2010; Limousin et al., 2010) provided better methods of determining whether the morphological, physiological and nutritional changes occur simultaneously or consistently between species or in response to different types of drought.
Michelia macclurei and Schima superba are two dominant angiosperm species in subtropical evergreen broadleaf forests in southern China. Both the species are evergreen tall arbors with important ecological and commercial traits. However, M. macclurei is distinguished from S. superba by its rooting systems. M. macclurei is characterized by shallow lateral roots (Liao et al., 1995) and large proportion of fine roots in the total roots biomass (Li et al., 1993), whereas S. superba has deep tap roots and a lower proportion of fine roots (Zheng, 2006). The species’ distinct root systems facilitates the study of the potential differences in the species’ responses to two types of seasonal drought (short-term and prolonged drought).
In this study, we designed a TEE experiment in a subtropical evergreen broadleaf forest, the most common forest type and the largest carbon sink in China (Piao et al., 2009), to investigate the morphological, physiological, and nutritional responses of M. and S. superba to different seasonal droughts. We hypothesized that there would be differences between the two species in their responses to the different types of droughts. Our particular interest was whether similar adaptive strategies would emerge, based on the variation in morphological, physiological, and nutritional traits. We expected to find evidence of differential responses to drought, since consistent differences in responses to drought among tree species would likely indicate compositional shifts in forests (da Costa et al., 2010). This type of research will help to estimate the possible changes that may have occurred in the dominant trees in subtropical forests of China as a result of seasonal drought that have occurred over the last decade (Zhou et al., 2013) and also to future drought.
Materials and Methods
Experimental Site
The experimental site is located 80 km southwest of Guangzhou (southern China) in the Heshan National Field Research Station Forest Ecosystem (112°54′E, 22°41′N, 80 m a.s.l.). The area has a subtropical climate with a mean annual temperature of 21.7°C, with the coldest month being January (13.1°C) and the hottest month, July (28.7°C). The mean annual precipitation is 1700 mm with a range of 1060–2060 mm recorded during the past 4 decades. The dry season, lasting from October to March, is distinct from the wet season, which lasts from April to September and is when the majority of the rainfall occurs. In the mid-1980s, researchers constructed an experimental plantation forest mixed with native broadleaf species at this station. After 30 years of succession, the plantation is currently characterized as secondary evergreen broadleaf forest dominated by S. superba and M. macclurei in the canopy layer; by Ilex asprella, Psychotria asiatica, and Melicope pteleifolia in the shrub layer; and by Lophatherum gracile and Adiantum flabellulatum in the herb layer. The mean canopy height and the diameter at breast height of this forest are approximately 14 m and 22 cm, respectively. The density of the trees was 1660 stem⋅ha-1 in 2012 (Jiao and Shen, 2014). The soil is laterite developed from sandshale. The average slope of this forest is approximately 15°.
Experimental Design
Based on the decreasing trend of rainfall in the dry season in this region (Zhou et al., 2011), we designed a TEE in this secondary evergreen broadleaf forest. Throughfall exclusion was achieved by using a system of plastic panels and plastic-lined guttering installed at a height of 1.5 m above the ground (Fisher et al., 2007). Eight 12 m × 12 m plots (at least 2 m away from each other) randomly distributed in the forest were subjected to one of two treatments reflecting two types of seasonal drought via throughfall exclusion: (1) drier dry season (DD) treatment, a relatively short-term drought and (2) extended dry season (ED) treatment, a prolonged drought. Another four plots were not subjected to drought treatment, reflecting the influence of trenching control (TC).
Briefly, in the DD treatment, in the dry season (from October to March), net input throughfall was reduced by ∼67% compared with the TC treatment. In the ED treatment, ∼67% of net input throughfall was again excluded, and the exclusion was continued until May using the same system; this treatment simulated a prolonged dry season from October to May. In both the DD and ED treatments, trenches (60–80 cm deep to bedrock) were dug and PVC panels were embedded around the perimeters of the plots to reduce the lateral inflow of water through the soil matrix and surface runoff from outside the plots. In the TC treatment, no throughfall exclusion facility was constructed, but trenches were used as a control for the DD and ED treatments. The effect of rainfall exclusion could be detected by comparing the DD and ED treatments to the TC treatment.
Field Sampling
Prior to the drought treatment, the plots were inventoried and trees selected for sampling were tabbed with red plastic tape. The total number of sample S. superba was 37 (DD 12, ED 12, TC 13), and of M. macclurei, 31 (DD 11, ED 10, TC 10). To diminish the influence of season on the morphological, physiological, and nutritional traits of the two species, the trees were sampled at different times according to the treatments: (1) background sampling (September, 2013) for all treatments, (2) the end of the DD in comparison with the TC treatment (March, 2014), (3) the end of the ED in comparison with the TC treatments (May, 2014). At each sampling, at least 20 mature leaves were collected from the canopy of each tabbed trees in each treatment. To minimize the influence of leaf age on the traits, the leaves were sampled from among those developed in the previous growing season (April–June, 2013). Approximately 10 g (fresh weight) fine roots were collected from below the surface soils of each tabbed tree. The leaves and the fine roots were stored in ice bags, and immediately carried to the laboratory for analysis.
Laboratory Analysis
Leaf Morphology
The sampled leaves were divided into two groups: one for morphological and nutritional measurement, the other for measurement of chlorophyll and other physiological traits. The maximum length (L, mm), maximum width (W, mm), and area (A, mm2) of each leaf were determined with a portable area meter (Li-3000C, Li-Cor Bioscience, UAS). The leaves were then oven-dried at 60°C for at least 48 h and weighed for dry mass. The leaf mass per area (LMA, g⋅mm-2) and the ratios of maximum length to maximum width (L/W) were calculated.
Leaf Physiological Traits
Frozen leaves (approximately 0.2 g each) from individual trees were cut and dipped into 5 mL 80% acetone to determine chlorophyll a and chlorophyll b (Chla and Chlb, respectively) contents. The contents of Chla and Chlb (ug⋅mg-1, fresh weight) were determined by the absorption at 663 and 645 nm, respectively, using a spectrophotometer (Unico, Shanghai, China). Chla and Chlb were summed to arrive at the total chlorophyll (ChlTotal).
The contents of soluble sugar in the leaves and fine roots of individual trees were determined according to the methods described in Kuang et al. (2012). Briefly, approximately 50 mg of the oven-dried leaf or fine root powder was extracted with 80% ethanol (v/v) at 85°C for 1 h. The solution was then centrifuged at 12,000 r for 10 min. The resulting supernatants were then combined, treated with activated charcoal, and evaporated to dryness in a vacuum evaporator. The residues were then re-dissolved in distilled water, then subjected to soluble sugar analysis using the anthrone–sulfuric acid method.
Free proline was extracted and determined as described by Hamid et al. (2003). Leaf and fine root samples were extracted with 3% sulphosalicylic acid. Extracts were mixed with 2 mL glacial acetic acid and 2 mL acid ninhydrin and maintained for 1 h at 100°C. Cold toluene was then added and the samples were shaken. Absorbance was read at 520 nm. The amount of proline was determined from a standard curve.
Nutritional Analysis
The powdered leaves were digested with concentrated nitric and perchloric acid (8:1, v/v) in a microwave system (Multiwave 3000, Anton Paar, Austria). The analytical procedure was performed using the method established by Dong (1996). After digestion, the concentrations (mg⋅kg-1) of the nutrients potassium (K), calcium (Ca), magnesium (Mg), and phosphorus (P) were determined using an inductively coupled plasma atomic emission spectrometer (ICP–OES, Optima 2000, USA). The content of nitrogen (N) was measured using an elemental analyzer (Flash EA 1112 Series, Thermo Finnigan, Milan, Italy). The content of sulfur (S) was determined using a sulfur analyzer (LTDL–9, China). The reliabilities of the analytical procedure were tested with both blanks and national standard reference materials (GSV–3).
Statistical Analysis
A linear mixed effects regression was performed by using Rstudio software (v0.98.9531; R package, lme4), where the species and the treatment were considered as fixed factors, and individuals nested in plots as random factors, to analyze the effects of drought treatment, the species, and their interactions on the morphological, physiological, and nutritional traits. Mean comparison was performed using ANOVAs between the drought and TC treatments to test whether the drought had significantly influenced the leaf (fine roots) traits. Effects with probabilities of p < 0.05 were considered to be significant. The data are shown as the mean ± standard deviation (SD).
Results
Leaf Traits
Prior to the throughfall exclusion, there were no significant differences in the leaf traits (L, W, L/W, and LMA) among the treatments in either S. superba or M. macclurei (Figure 1). For M. macclurei, the prolonged drought (ED) significantly influenced the leaf traits, i.e., it significantly increased L and L/W and decreased W and LMA in comparison to the TC treatment. However, the short-term drought (DD) had no significant effects on the leaf traits of M. macclurei. For S. superba, the two types of drought had no significant effects on morphological traits except that the ED treatment notably decreased the leaf width. However, there were significant differences between the two species in the L and W response to the ED treatment. Leaf mass area responded significantly differently to both ED and DD treatments due to the interaction of species and treatment (p < 0.05, Table 1).
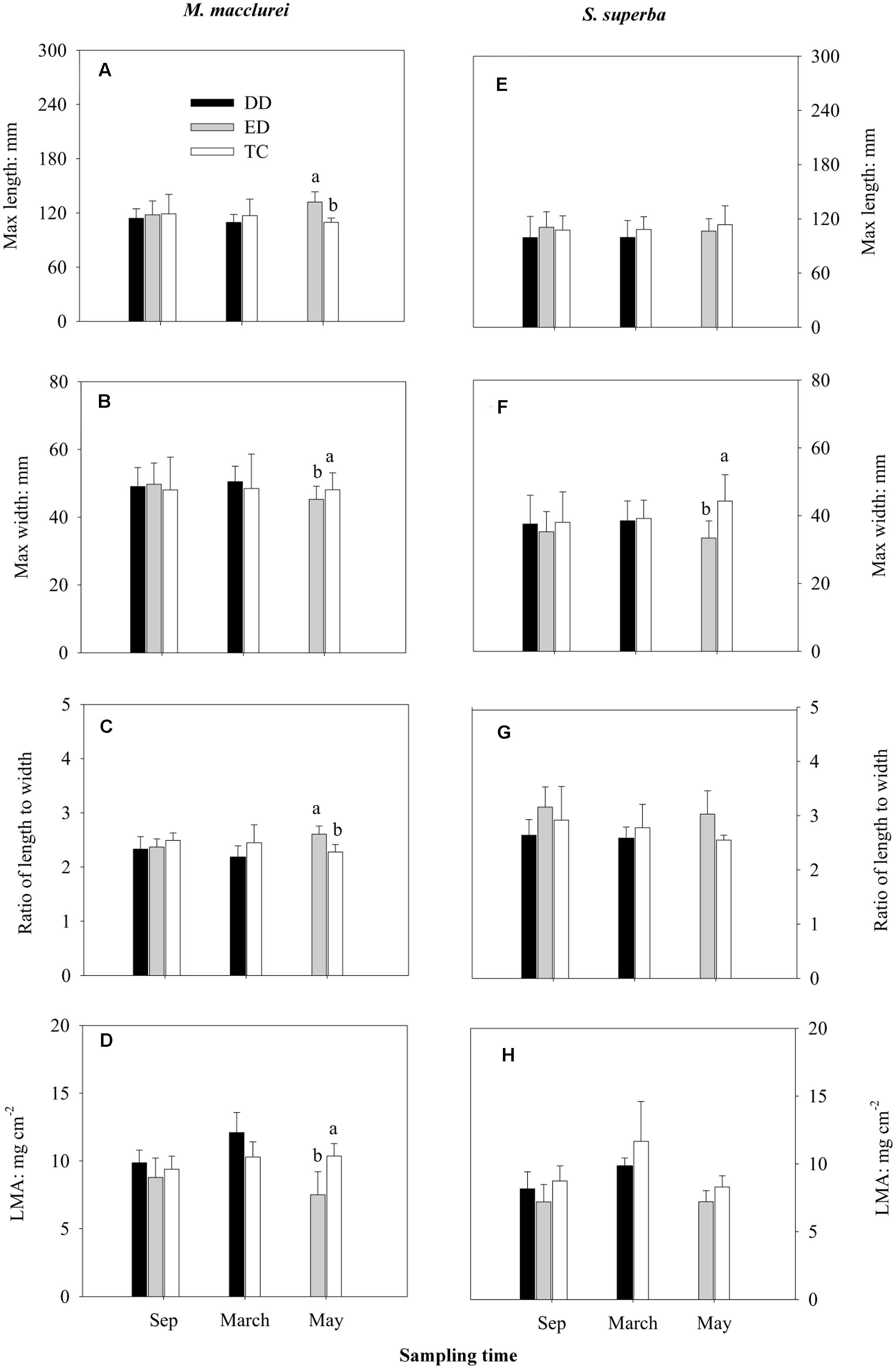
FIGURE 1. Effects of drier dry season drought (DD), prolonged drought (ED), and trenching control (TC) on the leaf max length (A,E), max width (B,F), the ratios of max length to max width (C,G), and the leaf mass per area (LMA; D,H) of M. macclurei (left column) and S. superba (right column). Data are shown as the mean and one standard deviation. Different letters show the significant differences among the treatments (p < 0.05).
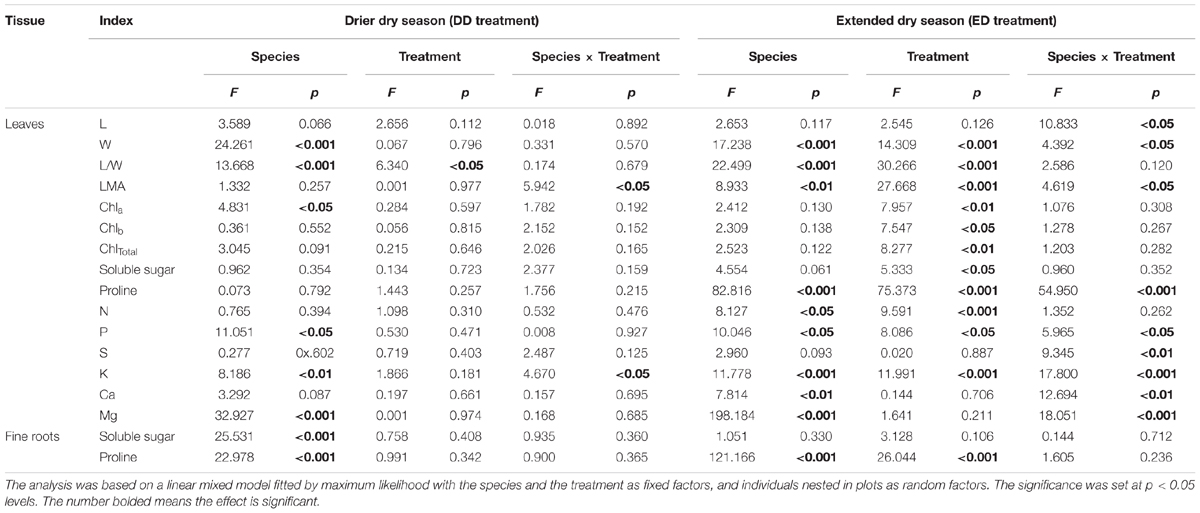
TABLE 1. Effect of drought treatment, species, and their interactions on the morphological, physiological, and nutritional traits.
Physiological Traits
Leaf Chlorophyll
Similar to the leaf morphological traits, leaf chlorophyll contents showed no significant differences among the treatments prior to the drought (Sep 2013). Unexpectedly, neither the DD (March 2014) nor the ED (May 2014) treatments significantly influenced the contents of Chla, Chlb, or ChlTotal in the leaves of either species, although Chla, Chlb, and ChlTotal showed decreasing tendencies in DD treatment and increasing tendencies in ED treatment for M. macclurei and increasing tendencies in both DD and ED treatments for S. superba. Trenching had little effect on leaf chlorophyll contents (Figure 2). The species and treatment interaction was not a significant factor in the response of leaf chlorophyll to drought (p = 0.192, 0.152, 0.165, respectively, in DD treatment, and p = 0.308, 0.267, 0.282, respectively, in ED treatment, Table 1).
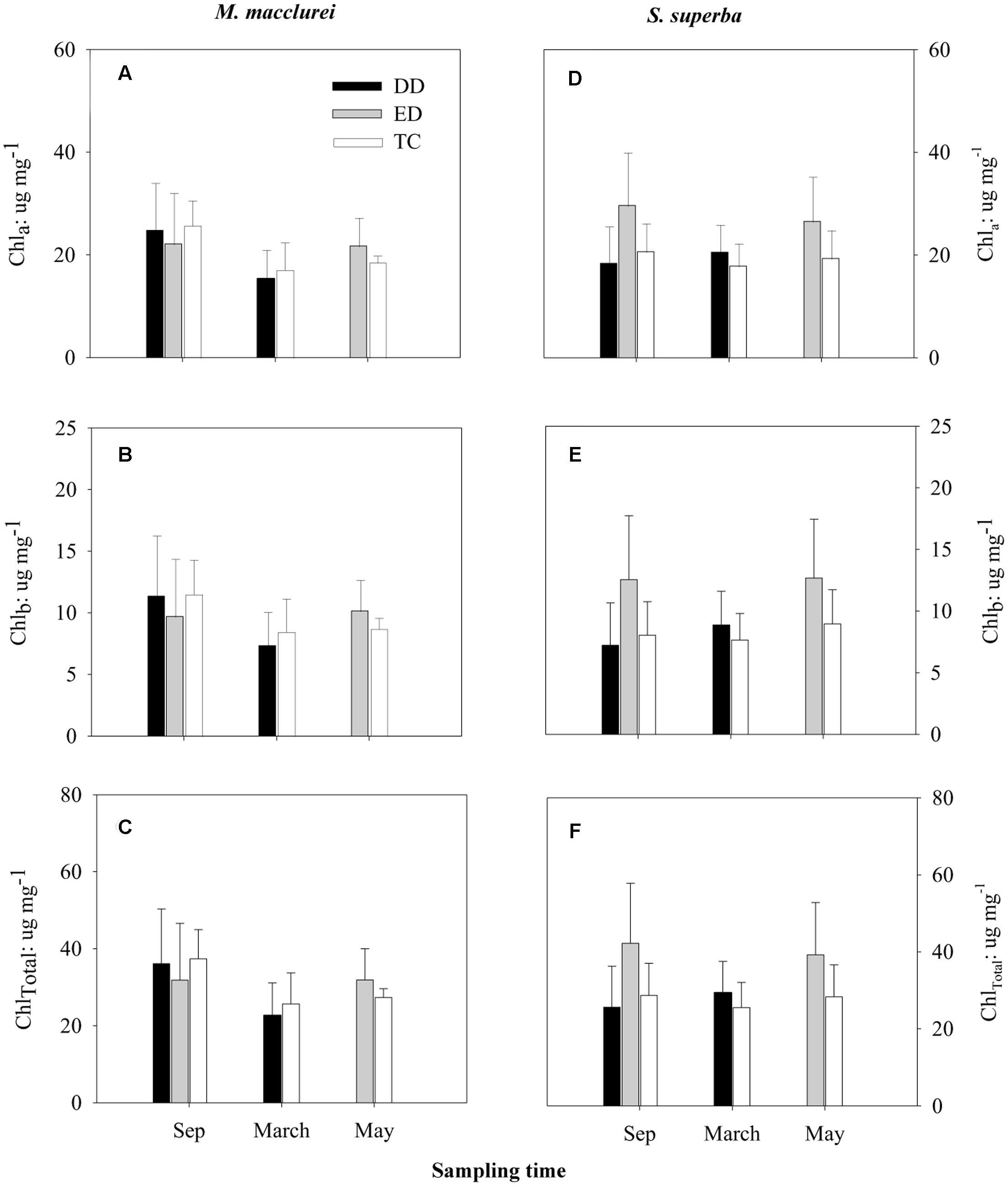
FIGURE 2. Responses of leaf chlorophyll a (Chla; A,D), chlorophyll b (Chlb; B,E), and total chlorophyll (ChlTotal; C,F) to the drier dry season drought (DD), prolonged drought (ED), and trenching control (TC) of M. macclurei (left column) and S. superba (right column). Data are shown as the mean and one standard deviation.
Soluble Sugars and Proline
Prior to the drought (September, 2013), the osmotic solutes (soluble sugars and proline) in both the leaves and fine roots of M. macclurei and S. superba did not differ significantly among the treatments. The DD treatment significantly increased the soluble sugar content in the leaves and fine roots of both M. macclurei (Figures 3A,B) and S. superba (Figure 3F). Meanwhile, the DD treatment caused a remarkable increase in proline content in the fine roots of M. macclurei (Figure 3D). The proline content in the leaves of both M. macclurei and S. superba did not seem to be influenced by the short-term drought (Figures 3C,G). The ED treatment did not notably influence the soluble sugars in the leaves and fine roots of the two species (Figures 3A,B,E,F). It did cause significantly higher proline content in the leaves and fine roots of both M. macclurei and S. superba (Figures 3C,D,G,H). Unexpectedly, only the proline content in the leaves responded significantly differently between the species to the extended drought (p < 0.001, Table 1).
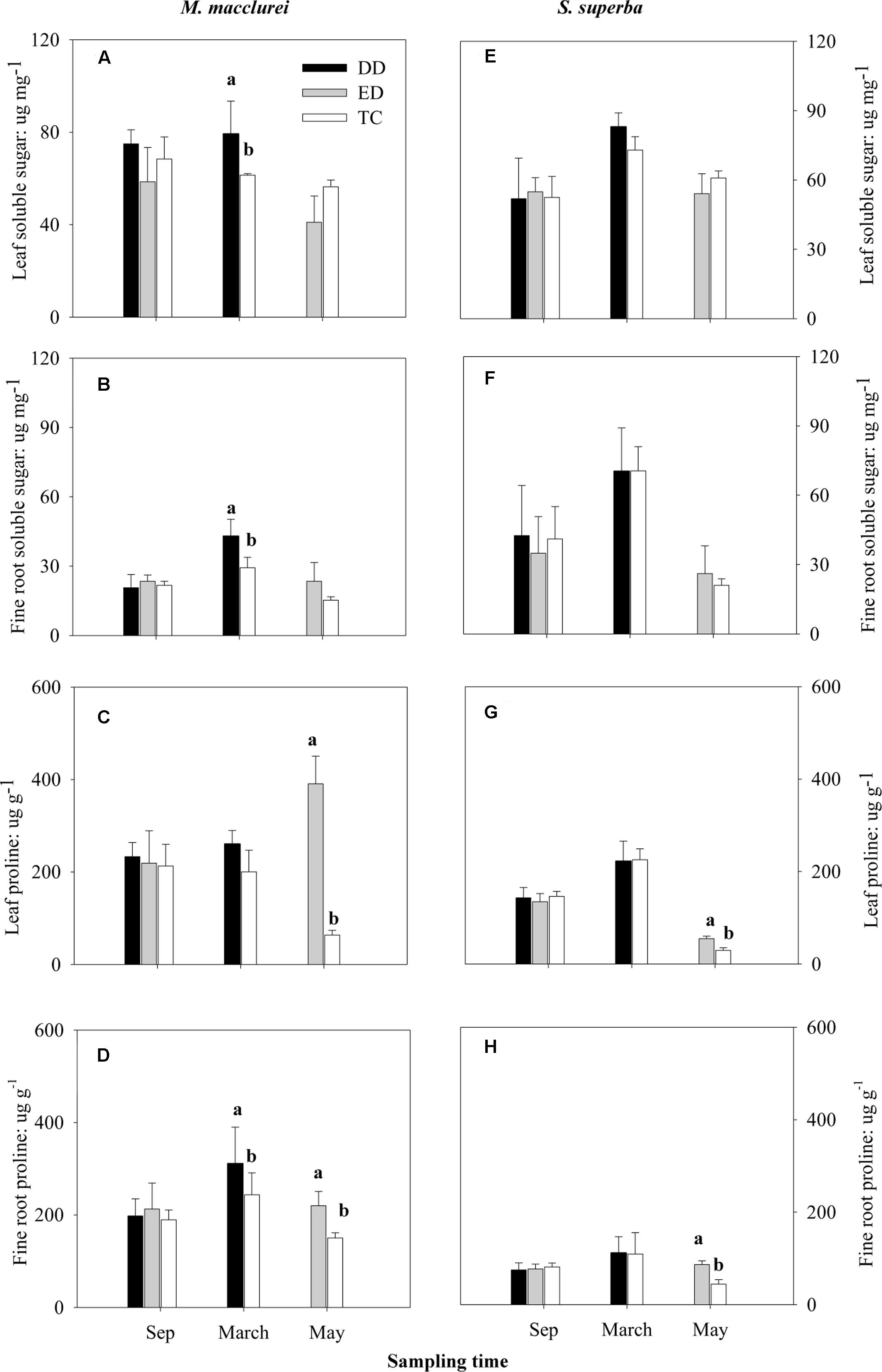
FIGURE 3. Responses of soluble sugar and proline in (A,C,E,G) leaves and (B,D,F,H) fine roots of M. macclurei (left column) and S. superba (right column) to the drier dry season drought (DD), prolonged drought (ED), and trenching control (TC) treatments. Data are shown as the mean and one standard deviation. Different letters denote a significant difference among the treatments (p < 0.05).
Nutritional Traits
None of the nutrients (N, P, S, K, Ca, and Mg) in the leaves were significantly different among treatments prior to the drought treatments but responded to the drought differently in each species (Figure 4). For M. macclurei, N and K content increased and P, S, Ca, and Mg content decrease significantly in response to the prolonged drought. The short-term drought (DD) treatment and the TC had no notable influence on any of the studied nutrients. For S. superba, the nutrients in the leaves were not influenced by either the short-term or the prolonged drought, except for N, which increased significantly in the prolonged drought (Figures 4G–L). Interestingly, leaf nutrients except for N responded to the extended drought significantly differently in the two species, while in the short-term drought leaf nutrients except for N had no significantly different responses between the species (Table 1).
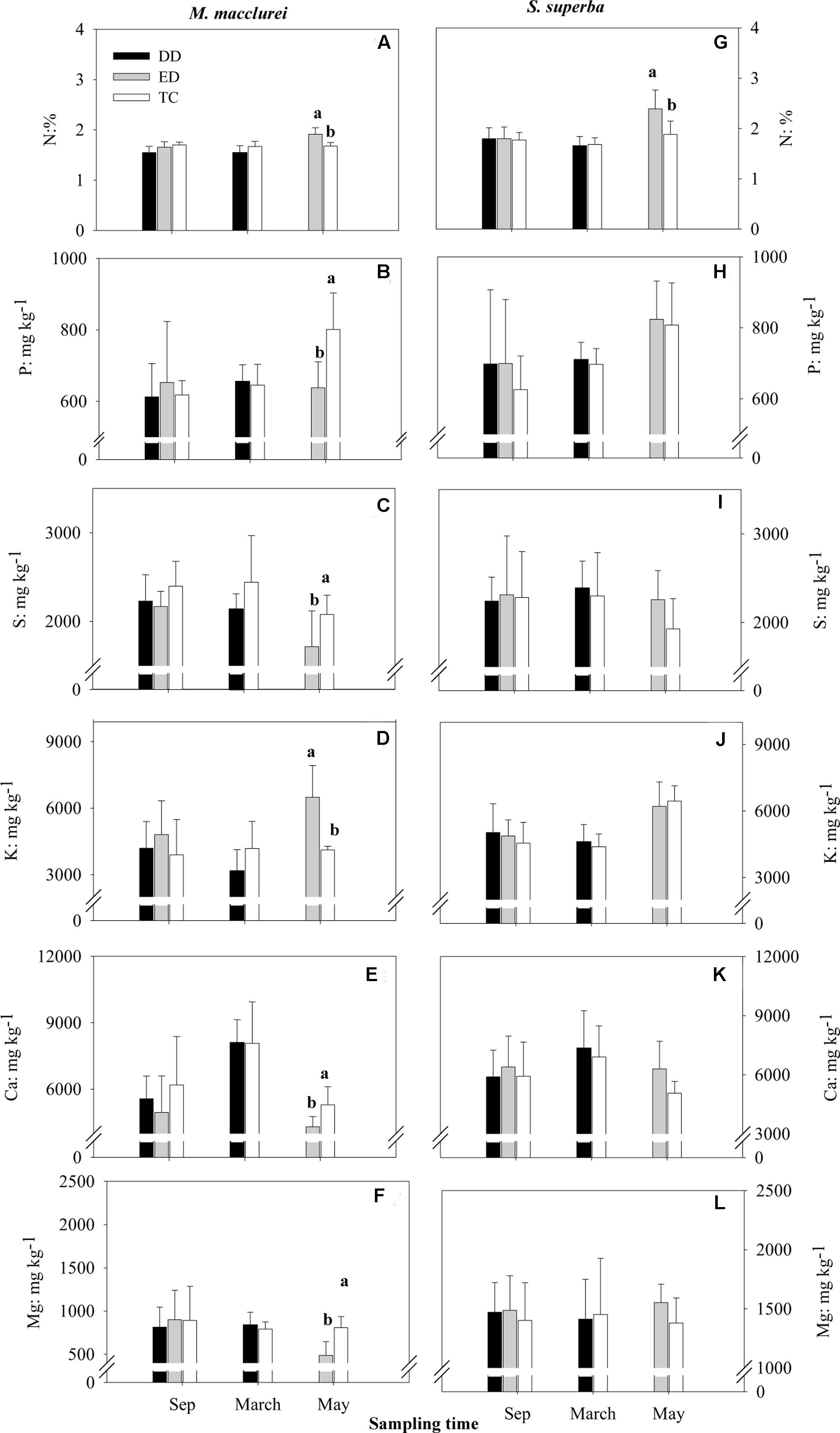
FIGURE 4. Concentrations of N (A,G), P (B,H), S (C,I), K (D,J), Ca (E,K), and Mg (F,L) in the leaves of M. macclurei (left column) and S. superba (right column) responding to the drier dry season drought (DD), prolonged drought (ED), and trenching control (TC) treatments. Different letters denote significant differences among the drought treatments (p < 0.05).
Discussion
The two species had significantly different responses to the prolonged drought in leaf L, W, and LMA (p < 0.05, Table 1), but in the short-term drought, only LMA responded differently (p < 0.05, Table 1). The responses of leaf morphologies to the two types of drought were species-specific, with M. macclurei obviously showing more sensitivity to prolonged drought than short-term drought and with S. superba showing more plasticity to drought in leaf morphologies (Figure 1). Under prolonged drought conditions, M. macclurei attempted to increase the leaf L, decrease the leaf W (Figures 1A,B), and narrow the leaf mass per area to adapt to the drought (Figures 1C,D). This type of adjustment can enhance the water–use efficiency (Knight and Ackerly, 2003) and reduce water loss via the smaller leaf area (Rozendaal et al., 2006). The significantly increased L/W of M. macclurei (Figure 1C) implied its higher sensitivity to prolonged drought in comparison to S. superba. Since the increased L/W can enlarge the leaf boundary layer, it can reduce respiration and retain leaf lipids for use in physiological activities (Han et al., 2014). Generally, plants with higher drought tolerance have longer and narrower leaves and larger LMA (Niinemets, 2001; Liu et al., 2011). The significantly different leaf morphologies in the prolonged drought treatment implied that M. macclurei is more sensitive to drought than S. superba, which showed higher plasticity to the significant changes in soil water availability. The preferential variation in leaf morphology may be one of the response mechanisms of M. macclurei, along with its large amount of shallow fine roots in comparison to S. supeerba (Li et al., 1993; Liao et al., 1995; Zheng, 2006). However, since mature trees respond more slowly to the changes in soil water than do woody seedlings (Liu et al., 2011), it is important to have longer-term monitoring data to gather considerably more evidence before drawing this conclusion.
Leaf chlorophyll can quickly respond to abiotic stress such as drought (Sapeta et al., 2013). However, the response of leaf chlorophyll did not significantly differ between M. macclurei and S. superba neither in the short drought (p = 0.192, 0.152, and 0.165, for Chla, Chlb, and ChlTotal, respectively; Table 1) nor the prolonged drought (p = 0.308, 0.267, and 0.282; Table 1). The insignificant differences may be ascribed to the drought intensity, drought duration, tree species, or other factors. Longer or more intense drought treatments may give further insight into the species’ chlorophyll responses. After all, the drought in this study was a rainfall reduction to one-third of normal, for one more month than the natural dry season.
Osmoregulation via accumulation of osmoregulators is recognized as one of the most important tolerance mechanisms that plants develop to adapt to a water deficit (Hoekstra and Buitink, 2001). The results in the present study showed that the responses of leaf proline under prolonged drought significantly differed between the species (p < 0.001, Table 1), implying a species-specificity in osmotic adjustment. Proline, as the best-known osmoregulator in plants (Ben Ahmed et al., 2009; Hessini et al., 2009; Dobrá et al., 2011), plays a key role in regulating redoxpotential (Shao et al., 2006), scavenging the hydroxyl radical and acting as a cytosolic osmoticum (Kishore et al., 2005), and is thought to protect plant cells by stabilizing membranes (Szabados and Savouré, 2010). The prolonged drought caused M. macclurei (more than 6 times the TC, Figure 3C) and S. superba (∼2 times the TC, Figure 3G) to significantly accumulate leaf proline. The result implied that both M. macclurei and S. superba could actively adjust proline in leaves in response to extended drought, which may be an adaptive strategy to protect it against drought stress and increase drought stress tolerance (Cvikrová, 2013; Königshofer and Löppert, 2015).
Furthermore, we found the short-term drought significantly increased the accumulation of soluble sugars in both leaves and fine roots of M. macclurei (Figures 3A,B) rather than of S. superba (Figures 3E,F). Soluble sugars, the main contributors to osmotic adjustment under drought stress (Hessini et al., 2009; Hummel et al., 2010), are important in regulating plant metabolism and development (Wingler and Roitsch, 2008; Bolouri-Moghaddam et al., 2010). The significant accumulations of soluble sugars implied that M. macclurei could adaptively respond to short-term drought, which may be a biochemical mechanism helping it to acclimate to drought (Sanders and Arndt, 2012), improve drought tolerance (Lee et al., 2008; Regier et al., 2009), or enhance resistance to drought (Elsheery and Cao, 2008).
Interestingly, M. macclurei had remarkable accumulation of proline in fine roots under both types of drought conditions (Figure 3D), while S. superba had higher proline accumulation only during prolonged drought (Figure 3H), further implying that the osmotic responses to drought could be different between species. For M. macclurei, the remarkable accumulation of proline in fine roots was relatively higher compared to that in the leaves in the short-term drought (Figures 3C,D) indicating that fine roots have a more sensitive osmotic response to drought than can leaves. For S. superba, proline was more sensitive to prolonged drought than the soluble sugars were. The significantly higher accumulation of osmotic solutes in the fine roots of M. macclurei suggested this species had a higher sensitivity to osmotic adjustment, indicating that it could maintain water absorption more efficiently under drought conditions than S. superba.
The significantly different responses of P, S, K, Ca, and Mg (p < 0.05, 0.01, 0.001, 0.01, and 0.001, respectively) to prolonged drought and of K (p < 0.05) to short-term drought (Table 1) between the two species was similar to those of other species (Hessini et al., 2009; Hummel et al., 2010). The significant increase in N content during the prolonged drought in the leaves of both species (Figures 4A,G) could be explained by the increase in nitrate content (Kameli and Lösel, 1995) as a result of rapid decline in nitrate reductase activity (Sivaramakrishnan et al., 1988), presumably due to the dieback of the microbial biomass that is induced by drought (Borken and Matzner, 2009). The significantly lower P, S, Ca and Mg content in the leaves of M. macclurei during the prolonged drought relative to the other treatments indicated that M. macclurei can more easily respond to prolonged rather than short-term drought, since the transpiration rate is the primary determinant of nutrients distribution within plants (de Freitas et al., 2011). The unexpectedly significantly increased K content in the leaves of M. macclurei under the ED treatment may have been remobilized from older tissues, which is one mechanism for providing sufficient K to the younger tissues within a plant. It has been reported that the K concentrations may increase even if the drought was of short duration and low intensity (McWilliams, 2003), since the K ion in plant tissues can act as one of the main contributors to osmotic adjustment during drought (Hessini et al., 2009; Hummel et al., 2010).
For S. superba, however, the nutrients (except for N) did not vary significantly under the two drought treatments, implying that S. superba may be more plastic and have higher adaption to drought relative to M. macclurei. However, longer-duration and higher-intensity droughts may provide more evidence for assessing the adaptation of plants to drought.
Results from this study provide new insights into the differentiation of subtropical tree species’ responses to two types of drought via adjusting morphological, physiological and nutritional traits. The species-specificity of responses between the trees should be taken into consideration in the development of some models, e.g., global impact models (Fischer et al., 2013; Singh et al., 2013), forest ecosystem water-use efficiency process-based model (Huang et al., 2016), assessing the forest responses to seasonal drought or in the management of tree/forest practice under drought conditions. The inconsistency of species’ responses can help facilitate the future design of through-fall exclusion experiments enabling common and comparable treatments to be imposed in disparate species. This facilitation will allow experiments to more realistically simulate precipitation regimes and more accurately assess the ecological consequences.
Conclusion
There are significantly different morphological (leaf max length, leaf max width, LMA), physiological (leaf proline) and nutritional (P, S, K, K, Ca, Mg) responses to throughfall–exclusion–induced prolonged drought between M. macclurei and S. superba. Under both short-term and prolonged drought conditions, S. superba exhibited larger plasticity and higher adaption than did M. macclurei, based on the variation in morphological, biochemical, osmotic, and nutritional traits in the trees during the drought treatments. M. macclurei responded more sensitively to the prolonged drought with respect to morphology, proline content, and nutritional traits and to the short-term drought with respect to soluble sugars content than did S. superba. The differential species-specific responses to drought will allow us to estimate the possible changes in dominant trees in subtropical forests of China which have experienced a decade’s worth of annual seasonal drought.
Author Contributions
WS and YK conceived and designed the experiments. YX and LZ performed the experiments. YX, YK, and EH analyzed the data. YK wrote the manuscript; other authors provided editorial advice.
Funding
This research was financially supported by the National Natural Science Foundation of China (Nos. 31130011, 41471443, 31290222, and 31425005).
Conflict of Interest Statement
The authors declare that the research was conducted in the absence of any commercial or financial relationships that could be construed as a potential conflict of interest.
The reviewer SR and handling Editor declared their shared affiliation, and the handling Editor states that the process met the standards of a fair and objective review.
Acknowledgment
We are thankful to Heshan National Field Research Station of Forest Ecosystem for technological support and for field sampling convenience.
Footnotes
References
Anderegg, W. R. L., Flint, A., Huang, C., Flint, L., Berry, J. A., Davis, F. W., et al. (2015). Tree mortality predicted from drought-induced vascular damage. Nat. Geosci. 8, 367–371. doi: 10.1038/ngeo2400
Beier, C., Beierkuhnlein, C., Wohlgemuth, T., Penuelas, J., Emmett, B., Körner, C., et al. (2012). Precipitation manipulation experiments: challenges and recommendations for the future. Ecol. Lett. 15, 899–911. doi: 10.1111/j.1461-0248.2012.01793.x
Ben Ahmed, C., Ben Rouina, B., Sensoy, S., Boukhris, M., and Ben Abdallah, F. (2009). Changes in gas exchange, proline accumulation and antioxidative enzyme activities in three olive cultivars under contrasting water availability regimes. Environ. Exp. Bot. 67, 345–352. doi: 10.1016/j.envexpbot.2009.07.006
Bolouri-Moghaddam, M. R., Le Roy, K., Xiang, L., Rolland, F., and Van den Ende, W. (2010). Sugar signaling and antioxidant network connections in plant cells. FEBS J. 277, 2022–2037. doi: 10.1111/j.1742-4658.2010.07633.x
Borken, W., and Matzner, E. (2009). Reappraisal of drying and wetting effects on C and N mineralization and fluxes in soils. Glob. Chang. Biol. 15, 808–824. doi: 10.1111/j.1365-2486.2008.01681.x
Borken, W., Savage, K., Davidson, E. A., and Trumbore, S. E. (2006). Effects of experimental drought on soil respiration and radiocarbon efflux from a temperate forest soil. Glob. Chang. Biol. 12, 177–193. doi: 10.1111/j.1365-2486.2005.001058.x
Cleland, E. E., Collins, S. L., Dickson, T. L., Farrer, E. C., Gross, K. L., Gherardi, L. A., et al. (2013). Sensitivity of grassland plant community composition to spatial vs. temporal variation in precipitation. Ecology 94, 1687–1696. doi: 10.1890/12-1006.1
Coe, K. K., and Sparks, J. P. (2014). Physiology-based prognostic modeling of the influence of changes in precipitation on a keystone dryland plant species. Oecologia 176, 933–942. doi: 10.1007/s00442-014-3067-7
Cvikrová, M., Gemperlová, L., Martincová, O., and Vanková, R. (2013). Effect of drought and combined drought and heat stress on polyamine metabolism in proline-over-producing tobacco plants. Plant Physiol. Biochem. 73, 7–15. doi: 10.1016/j.plaphy.2013.08.005
da Costa, A. C. L., Galbraith, D., Almeida, S., Portela, B. T. T., da Costa, M., Junior, J. A. S., et al. (2010). Effect of 7 yr of experimental drought on vegetation dynamics and biomass storage of an eastern Amazonian rainforest. New Phytol. 187, 579–591. doi: 10.1111/j.1469-8137.2010.03309.x
de Freitas, S. T., Shackel, K. A., and Mitcham, E. J. (2011). Abscisic acid triggers whole–plant and fruit specific mechanisms to increase fruit calcium uptake and prevent blossom end rot development in tomato fruit. J. Exp. Bot. 62, 2645–2656. doi: 10.1093/jxb/erq430
Dobrá, J., Vanková, R., Havlová, M., Burman, A. J., Libus, J., and Storchová, H. (2011). Tobacco leaves and roots differ in the expression of proline metabolism-related genes in the course of drought stress and subsequent recovery. J. Plant Physiol. 168, 1588–1597. doi: 10.1016/j.jplph.2011.02.009
Dong, M. (1996). Survey, Observation and Analysis of Terrestrial Biocommunities, 1st Edn. Beijing: Standards Press of China.
Elsheery, N. I., and Cao, K. F. (2008). Gas exchange, chlorophyll fluorescence, and osmotic adjustment in two mango cultivars under drought stress. Acta Physiol. Plant. 30, 769–777. doi: 10.1007/s11738-008-0179-x
Fischer, E. M., Beyerle, U., and Knutti, R. (2013). Robust spatially aggregated projections of climate extremes. Nat. Clim. Change 3, 1033–1038. doi: 10.1038/nclimate2051
Fisher, R. A., Williams, M., Lola Da Costa, A. L., Malhi, Y., Da Costa, R. F., Almeida, S., et al. (2007). The response of an eastern Amazonian rain forest to drought stress: results and modelling analyses from a throughfall exclusion experiment. Glob. Change Biol. 13, 2361–2378. doi: 10.1111/j.1365-2486.2007.01417.x
Flanagan, L. B., and Farquhar, G. D. (2014). Variation in the carbon and oxygen isotope composition of plant biomass and its relationship to water use efficiency at the leaf– and ecosystem–scales in a northern Great Plains grassland. Plant Cell Environ. 37, 425–438. doi: 10.1111/pce.12165
Gaul, D., Hertel, D., Borken, W., Matzner, E., and Leuschner, C. (2008). Effects of experimental drought on the fine root system of mature Norway spruce. For. Ecol. Manage. 256, 1151–1159. doi: 10.1016/j.foreco.2008.06.016
Greve, P., Orlowsky, B., Mueller, B., Sheffield, J., Reichstein, M., and Seneviratne, S. I. (2014). Global assessment of trends in wetting and drying over land. Nat. Geosci. 7, 716–721. doi: 10.1038/ngeo2247
Hamid, H. A., Khaedr, A., Mohammad, A. A., Amal, A. A., Quick, P. W., and Abogadallah, M. (2003). Proline induces the expression of salt–stress–responsive proteins and may improve the adoption of Pancratium maritimum L. to salt stress. J. Exp. Bot. 54, 2553–2562.
Han, W., Liu, C., Fan, Y. W., Zhao, N., Ye, S. Y., Yin, W. L., et al. (2014). Responses of leaf morphological traits for broadleaved woody plants along the altitudinal gradient of Changbai Mountain, northeastern China. J. Beijing For. Univ. 36, 47–53.
Hessini, K., Martínez, J. P., Gandour, M., Albouchi, A., Soltani, A., and Abdelly, C. (2009). Effect of water stress on growth, osmotic adjustment, cell wall elasticity and water–use efficiency in Spartina alterniflora. Environ. Exp. Bot. 67, 312–319. doi: 10.1016/j.envexpbot.2009.06.010
Hoekstra, F. A., and Buitink, J. (2001). Mechanisms of plant desiccation tolerance. Trends Plant Sci. 8, 431–438. doi: 10.1016/S1360-1385(01)02052-0
Hoeppner, S. S., and Dukes, J. S. (2012). Interactive responses of old–field plant growth and composition to warming and precipitation. Glob. Change Biol. 18, 1754–1768. doi: 10.1111/j.1365-2486.2011.02626.x
Hovenden, M. J., Newton, P. C. D., and Wills, K. E. (2014). Seasonal not annual rainfall determines grassland biomass response to carbon dioxide. Nature 511, 583–586. doi: 10.1038/nature13281
Huang, M. T., Piao, S. R., Zeng, Z. Z., Peng, S. S., Ciais, P., Cheng, L., et al. (2016). Seasonal responses of terrestrial ecosystem water-use efficiency to climate change. Glob. Change Biol. 22, 2165–2177. doi: 10.1111/gcb.13180
Hummel, I., Pantin, F., Sulpice, R., Piques, M., Rolland, G., Dauzat, M., et al. (2010). Arabidopsis plants acclimate to water deficit at low cost through changes of carbon usage: an integrated perspective using growth, metabolite, enzyme, and gene expression analysis. Plant Physiol. 154, 357–372. doi: 10.1104/pp.110.157008
Jiao, M., and Shen, W. J. (2014). Effects of seasonal precipitation variation on litter–fall in lower subtropical evergreen broad–leaved forest. J. Trop. Subtrop. Bot. 22, 323–331.
Kameli, A., and Lösel, D. M. (1995). Contribution of carbohydrates and other solutes to osmotic adjustment in wheat leaves under water stress. J. Plant Physiol. 145, 363–366. doi: 10.1016/S0176-1617(11)81903-6
Kishore, G. K., Pande, S., and Podile, A. R. (2005). Biological control of late leaf spot of peanut (Arachis hypogaea L.) with chitinolytic bacteria. Phytopathology 95, 1157–1165. doi: 10.1094/PHYTO-95-1157
Knapp, A. K., Carroll, C. J. W., Denton, E. M., La Pierre, K. J., Collins, S. L., and Smith, M. D. (2015). Differential sensitivity to regional-scale drought in six central U.S. grasslands. Oecologia 177, 949–957. doi: 10.1007/s00442-015-3233-6
Knight, C. A., and Ackerly, D. D. (2003). Evolution and plasticity of photosynthetic thermal tolerance, specific leaf area and leaf size: congeneric species from desert and coastal environments. New Phytol. 160, 337–347. doi: 10.1046/j.1469-8137.2003.00880.x
Königshofer, H., and Löppert, H. G. (2015). Regulation of invertase activity in different root zones of wheat (Triticum aestivum L.) seedlings in the course of osmotic adjustment under water deficit conditions. J. Plant Physiol. 183, 130–137. doi: 10.1016/j.jplph.2015.06.005
Kuang, Y. W., Xi, D., Li, J., Zhu, X. M., and Zhang, L. L. (2012). Traffic pollution influence leaf biochemistries of Broussonetia papyrifera. Open J. For. 2, 71–76. doi: 10.4236/ojf.2012.22010
Kulmatiski, A., and Beard, K. H. (2013). Woody plant encroachment facilitated by increased precipitation intensity. Nat. Clim. Change 3, 833–837. doi: 10.1038/nclimate1904
Lee, B. R., Jin, Y. L., Jung, W. J., Avice, J. C., Morvan–Bertrand, A., Ourry, A., et al. (2008). Water–deficit accumulates sugars by starch degradation – not by de novo synthesis – in white clover leaves (Trifolium repens). Physiol. Plant 134, 403–411. doi: 10.1111/j.1399-3054.2008.01156.x
Li, Z. W., Yang, Y. S., Wu, Z. X., and Lai, S. Z. (1993). Root system of mixed stand of Chinese Fir and Homana. Chi. J. Ecol. 12, 20–24.
Liao, L. P., Chen, C. Y., Zhang, J. W., and Gao, H. (1995). Turnover of fine roots in pure and mixed Cunninghamica lanceolata and Michela macclurei. Chi. J. Appl. Ecol. 6, 7–10.
Limousin, J. M., Misson, L., Lavoir, A. V., Martin, N. K., and Rembal, S. (2010). Do photosynthetic limitations of evergreen Quercus ilex leaves change with long–term increased drought severity? Plant Cell Environ. 33, 863–875. doi: 10.1111/j.1365-3040.2009.02112.x
Liu, C., Liu, Y., Guo, K., Fan, D., Li, G., Zheng, Y., et al. (2011). Effect of drought on pigments, osmotic adjustment and antioxidant enzymes in six woody plant species in karst habitats of southwestern China. Environ. Exp. Bot. 71, 174–183. doi: 10.1016/j.envexpbot.2010.11.012
Marvel, K., and Bonfils, C. (2013). Identifying external influences on global precipitation. Proc. Natl. Acad. Sci. U.S.A. 110, 19301–19306. doi: 10.1073/pnas.1314382110
McWilliams, D. (2003). Drought Strategies for Cotton, Cooperative Extension Service Circular 582, College of Agriculture and Home Economics. Las Cruces, NM: New Mexico State University.
Meng, T. T., Ni, J., and Harrison, S. P. (2009). Plant morphometric traits and climate gradients in northern China: a meta–analysis using quadrat and flora data. Ann. Bot. 104, 1217–1229. doi: 10.1093/aob/mcp230
Moran, M. S., Ponce-Campos, G. E., Huete, A., McClaran, M. P., Zhang, Y. G., Hamerlynck, E. P., et al. (2014). Functional response of U.S. grasslands to the early 21 st-century drought. Ecology 95, 2121–2133. doi: 10.1890/13-1687.1
Moreno–Gutiérrez, C., Battipaglia, G., Cherubini, P., Saurer, M., Nicolás, E., Contreras, S., et al. (2012). Stand structure modulates the long–term vulnerability of Pinus halepensis to climatic drought in a semiarid Mediterranean ecosystem. Plant Cell Environ. 35, 1026–1039. doi: 10.1111/j.1365-3040.2011.02469.x
Nepstad, D. C., Tohver, I. M., Ray, D., Moutinho, P., and Cardinot, G. (2007). Mortality of large trees and lianas following experimental drought in an Amazon forest. Ecology 88, 2259–2269. doi: 10.1890/06-1046.1
Niinemets, U. (2001). Global–scale climatic controls of leaf dry mass per area, density, and thickness in trees and shrubs. Ecology 82, 453–469. doi: 10.1890/0012-9658(2001)082[0453:GSCCOL]2.0.CO;2
Piao, S. L., Fang, J. Y., Ciais, P., Peylin, P., Huang, Y., Sitch, S., et al. (2009). The carbon balance of terrestrial ecosystems in China. Nature 458, 1009–1013. doi: 10.1038/nature07944
Picotte, J. J., Rhode, J. M., and Cruzan, M. B. (2009). Leaf morphological responses to variation in water availability for plants in the Piriqueta caroliniana complex. Plant Ecol. 200, 267–275. doi: 10.1007/s11258-008-9451-9
Pratt, J. D., and Mooney, K. A. (2013). Variation in Artemisia californica functional traits and plasticity along a steep environmental cline: implications for plant response to predicted climate change. Glob. Change Biol. 19, 2454–2466. doi: 10.1111/gcb.12199
Raz-Yaseef, N., Yakir, D., Schiller, G., and Cohen, S. (2012). Dynamics of evapotranspiration partitioning in a semi-arid forest as affected by temporal rainfall patterns. Agric. For. Meteorol. 157, 77–85. doi: 10.1016/j.agrformet.2012.01.015
Regier, N., Streb, S., Cocozza, C., Schaub, M., Cherubini, P., Zeeman, S. C., et al. (2009). Drought tolerance of two black poplar (Populus nigra L.) clones: contribution of carbohydrates and oxidative stress defence. Plant Cell Environ. 32, 1724–1736. doi: 10.1111/j.1365-3040.2009.02030.x
Rouphael, Y., Cardarelli, M., Schwarz, D., Franken, P., and Colla, G. (2012). “Effects of drought on nutrient uptake and assimilation in vegetable crops,” in Plant Responses to Drought Stres: From Morphological to Molecular Features, ed. R. Aroca (New York, NY: Springer), 171–195.
Rozendaal, D. M. A., Hurtado, V. H., and Poorter, L. (2006). Plasticity in leaf traits of 38 tropical tree species in response to light; relationships with light demand and adult stature. Funct. Ecol. 20, 207–216. doi: 10.1111/j.1365-2435.2006.01105.x
Sanders, G. J., and Arndt, S. K. (2012). “Osmotic adjustment under drought conditions,” in Plant Responses to Drought Stress: from Morphological to Molecular Features, ed. R. Aroca (New York, NY: Springer), 199–229. doi: 10.1007/978-3-642-32653-0_8
Sapeta, H., Costa, J. M., Lourenço, T., Maroco, J., van der Linde, P., and Oliveira, M. M. (2013). Drought stress response in Jatropha curcas: growth and physiology. Environ. Exp. Bot. 85, 76–84. doi: 10.1016/j.envexpbot.2012.08.012
Shao, H. B., Chen, X. Y., Chu, L. Y., Zhao, X. N., Wu, G., Yuan, Y. B., et al. (2006). Investigation on the relationship of proline with wheat anti-drought under soil water deficits. Colloids Surf. B Biointer. 53, 113–119. doi: 10.1016/j.colsurfb.2006.08.008
Singh, D., Tsiang, M., Rajaratnam, B., and Diffenbaugh, N. S. (2013). Precipitation extremes over the continental United States in a transient, high-resolution, ensemble climate model experiment. J. Geophys. Res. Atmos. 118, 7063–7086. doi: 10.1029/2011JD016458
Sivaramakrishnan, S., Patell, V. Z., Flower, D. J., and Peacock, J. M. (1988). Proline accumulation and nitrate reductase activity in contrasting sorghum lines during mild–season drought stress. Physiol. Plant. 74, 418–426. doi: 10.1111/j.1399-3054.1988.tb01997.x
Szabados, L., and Savouré, A. (2010). Proline: a multifunctional amino acid. Trends Plant Sci. 15, 89–97. doi: 10.1016/j.tplants.2009.11.009
Thomey, M. L., Collins, S. L., Vargas, R., Johnson, J. E., Brown, R. F., Natvig, D. O., et al. (2011). Effect of precipitation variability on net primary production and soil respiration in a Chihuahuan Desert grassland. Glob. Change Biol. 17, 1505–1515. doi: 10.1111/j.1365-2486.2010.02363.x
Travers, S. K., and Eldridge, D. J. (2013). Increased rainfall frequency triggers an increase in litter fall rates of reproductive structures in an arid eucalypt woodland. Austral Ecol. 38, 820–830. doi: 10.1111/aec.12055
Wilcox, K. R., Fischer, J. C. V., Muscha, J. M., Petersen, M. K., and Knapp, A. K. (2015). Contrasting above- and belowground sensitivity of three Great Plains grasslands to altered rainfall regimes. Glob. Change Biol. 21, 335–344. doi: 10.1111/gcb.12673
Wingler, A., and Roitsch, T. (2008). Metabolic regulation of leaf senescence: interactions of sugar signalling with biotic and abiotic stress responses. Plant Biol. 10, 50–62. doi: 10.1111/j.1438-8677.2008.00086.x
Wright, I. J., Reich, P. B., Cornelissen, J. H., Falster, D. S., Garmier, E., Hikosaka, K., et al. (2005). Assessing the generality of global leaf trait relationships. New Phytol. 166, 485–496. doi: 10.1111/j.1469-8137.2005.01349.x
Zeglin, L. H., Bottomley, P. J., Jumpponen, A., Rice, C. W., Arango, M., Lindsley, A., et al. (2013). Altered precipitation regime affects the function and composition of soil microbial communities on multiple time scales. Ecology 94, 2334–2345. doi: 10.1890/12-2018.1
Zeppel, M. J. B., Wilks, J. V., and Lewis, J. D. (2014). Impacts of extreme precipitation and seasonal changes in precipitation on plants. Biogeosciences 11, 3083–3093. doi: 10.1007/s00442-011-2021-1
Zheng, F. X. (2006). A Preliminary Study of Aluminum Toxicity on Four Woody Plants in Acid Rain Area of South China. Ph.D. thesis, Graduate University of the Academy of Science, Beijing.
Zhou, G. Y., Peng, C. H., Li, Y. L., Liu, S. Z., Zhang, Q. M., Tang, X. L., et al. (2013). A climate change–induced threat to the ecological resilience of a subtropical monsoon evergreen broad–leaved forest in Southern China. Glob. Change Biol. 19, 1197–1210. doi: 10.1111/gcb.12128
Keywords: functional traits, morphological traits, nutritional traits, subtropical forest, throughfall exclusion, precipitation change
Citation: Kuang YW, Xu YM, Zhang LL, Hou EQ and Shen WJ (2017) Dominant Trees in a Subtropical Forest Respond to Drought Mainly via Adjusting Tissue Soluble Sugar and Proline Content. Front. Plant Sci. 8:802. doi: 10.3389/fpls.2017.00802
Received: 24 January 2017; Accepted: 28 April 2017;
Published: 15 May 2017.
Edited by:
Sebastian Leuzinger, Auckland University of Technology, New ZealandReviewed by:
Qi Deng, Tennessee State University, USAMireia Gomez Gallego, Scion, New Zealand
Sridevi Ravi, Auckland University of Technology, New Zealand
Copyright © 2017 Kuang, Xu, Zhang, Hou and Shen. This is an open-access article distributed under the terms of the Creative Commons Attribution License (CC BY). The use, distribution or reproduction in other forums is permitted, provided the original author(s) or licensor are credited and that the original publication in this journal is cited, in accordance with accepted academic practice. No use, distribution or reproduction is permitted which does not comply with these terms.
*Correspondence: Weijun Shen, c2hlbndlaWpAc2NiZy5hYy5jbg==