- 1College of Plant Protection, Nanjing Agricultural University, Nanjing, China
- 2Key Laboratory of Integrated Management of Crop Diseases and Pests – Nanjing Agricultural University, Ministry of Education, Nanjing, China
- 3Australian Herbicide Resistance Initiative (AHRI), School of Agriculture & Environment, University of Western Australia, Perth, WA, Australia
- 4Biotechnology Research Center, Hunan Academy of Agricultural Sciences, Changsha, China
Herbicide resistance can be either target-site or non-target-site based. The molecular mechanisms underlying non-target-site resistance (NTSR) are poorly understood, especially at the level of gene expression regulation. MicroRNAs (miRNAs) represent key post-transcriptional regulators of eukaryotic gene expression and play important roles in stress responses. In this study, the miR397 gene from Beckmannia syzigachne (referred to as bsy-miR397) was functionally characterized to determine its role in regulating fenoxaprop-P-ethyl resistance. We showed that (1) bsy-miR397 transcript level is constitutively higher in resistant than in sensitive B. syzigachne plants, whereas bsy-Laccase expression and activity show the opposite trend, and (2) bsy-miR397 suppresses the expression of bsy-Laccase in tobacco, indicating that it negatively regulates bsy-Laccase at the transcriptional level. We found evidences that miR397/laccase regulation might be involved in fenoxaprop-P-ethyl NTSR. First, the rice transgenic line overexpressing OXmiR397 showed improved fenoxaprop-P-ethyl tolerance. Second, following activation of bsy-Laccase gene expression by CuSO4 treatment, fenoxaprop resistance in B. syzigachne tended to decrease. Therefore, we suggest that bsy-miR397 might play a role in fenoxaprop-P-ethyl NTSR in B. syzigachne by down-regulating laccase expression, potentially leading to the enhanced expression of three oxidases/peroxidases genes to introduce an active moiety into herbicide molecules in Phase-2 metabolism. Bsy-miR397, bsy-Laccase, and other regulatory components might form a regulatory network to detoxify fenoxaprop-P-ethyl in B. syzigachne, supported by the differential expression of transcription factors and oxidases/peroxidases in the rice transgenic line overexpressing OXmiR397. This implies how down-regulation of a gene (laccase) can enhance NTSR. Our findings shed light on the daunting task of understanding and managing complex NTSR in weedy plant species.
Introduction
Herbicide resistance can be achieved by target-site and non-target-site based mechanisms (Powles and Yu, 2010). Target-site resistance (TSR) can be easily investigated by detecting mutation or expression of the target molecule. Non-target-site resistance (NTSR) refers to any mechanisms not belonging to TSR (Délye, 2013). NTSR is common among weed species and leads to the formation of unpredictable resistant phenotypes (Délye, 2013; Yu and Powles, 2014). NTSR to herbicides encompasses a range of diverse mechanisms, including reduced herbicide penetration and translocation, enhanced herbicide metabolism, and protection against the collateral damage of herbicide action, and regulatory pathways (Délye, 2013). NTSR can be mediated via several mechanisms (Délye, 2013; Yu and Powles, 2014), and often under polygenic control (Busi et al., 2013; Gaines et al., 2014; Duhoux et al., 2015; Gardin et al., 2015). Nevertheless, most recent studies have focused on metabolic resistance (Gaines et al., 2014; Duhoux et al., 2015; Gardin et al., 2015; Pan et al., 2016), and very few of them have investigated the molecular mechanisms underlying NTSR.
Plants are subjected to biotic and abiotic stresses in their environment and develop adaptation by evolving complex networks of stress detection and signaling and response pathways that trigger both general and specific responses along with adjustment of the response (Vaahtera and Brosché, 2011). Herbicides represent a powerful abiotic stress and are expected to trigger some of these pathways. Therefore, NTSR is assumed to be a part of weed stress response (Délye, 2013), and some of the herbicide-induced stress responses could be involved in NTSR. The regulation of plant response to stress involves multiple factors; NTSR is achieved by not only activating protection reactions but also via modifying regulators such as microRNAs (miRNAs) (Sunkar et al., 2012; Délye, 2013). As NTSR poses a high risk to crop production (Délye, 2013; Yu and Powles, 2014), determining the possible role of miRNAs in regulating herbicide response/resistance is necessary.
MicroRNAs are non-coding RNAs that are approximately 20–24 nucleotides long and are key posttranscriptional regulators of eukaryotic gene expression (Llave et al., 2002). They down-regulate the expression of target genes primarily by inhibiting or repressing their translation and are known to play important roles in adaptive responses to abiotic stresses (Khraiwesh et al., 2012). The first evidence of such roles for miRNA was found in Arabidopsis thaliana subjected to abiotic stress conditions, showing miRNAs different from those found in A. thaliana grown under normal conditions (Jones-Rhoades and Bartel, 2004; Sunkar and Zhu, 2004). To date, many miRNAs have been identified, and some of them have been experimentally confirmed to be related to various abiotic stress responses.
American sloughgrass (Beckmannia syzigachne Steud.) is an annual winter grass widely distributed in China. Since the 1990s, fenoxaprop-P-ethyl has been extensively and continuously utilized to control grass weeds, including B. syzigachne. Consequently, many B. syzigachne populations have evolved resistance to fenoxaprop-P-ethyl, thereby rendering them as hard-to-control weeds (Pan et al., 2015b). In our previous studies, three fenoxaprop-P-ethyl-resistant B. syzigachne populations involving both TSR and NTSR were identified (Pan et al., 2015b), and our RNA-seq analysis detected structural changes and alterations in the transcriptional regulation of several genes encoding metabolizing enzymes (Pan et al., 2016). The RNA-seq analysis also showed that laccase gene expression was down-regulated in the three fenoxaprop-P-ethyl-resistant B. syzigachne populations. However, we found no difference in laccase gene expression in several B. syzigachne populations exhibiting only TSR-based fenoxaprop-P-ethyl-resistance, compared to a susceptible population. All these results indicate that alterations in laccase gene transcriptional regulation might play a role in NTSR.
Since few studies have investigated laccase family members, and their potential biological roles have not been completely understood. In A. thaliana, laccases are known to play a role in lignin biosynthesis, and 17 laccase members that have various expression patterns and functions have been identified. Some of these laccase genes are associated with lignin biosynthesis (Lu et al., 2013); however, the roles of the other laccase genes remain unknown (Liang et al., 2006; Berthet et al., 2011). Furthermore, many laccase family members have been identified in rice, but their functions are unclear. Notably, studies have indicated that a laccase family member is a target of miR397 (Jones-Rhoades and Bartel, 2004). MicroR397 (miR397) is conserved across most dicotyledon and monocotyledon species (Jones-Rhoades and Bartel, 2004). At present, studies on miR397 have focused on its role in abiotic stress response. For example, a study found that miR397 expression was up-regulated in A. thaliana by a range of abiotic stresses such as cold, drought, and high salinity (Sunkar and Zhu, 2004). Similarly, the expression of miR397 was induced in rice in response to drought stress (Zhao et al., 2007). However, after persistent drought treatment, the expression of miR397 was down-regulated (Zhou et al., 2010). Furthermore, miR397 was also found to be up-regulated under oxidative stress caused by copper deficiency (Abdel-Ghany and Pilon, 2008; Li et al., 2011). As herbicides such as fenoxaprop-P-ethyl can cause oxidative stress to plants, and the question arises as whether some miRNAs might be involved in herbicide resistance and endow herbicide NTSR.
In this study, we characterized miR397 expression in a susceptible versus three resistant B. syzigachne populations (involving both TSR and NTSR). We also utilized transgenic tobacco and rice plants to further characterize the role of miR397 in regulating plant resistance and tolerance to fenoxaprop-P-ethyl. Our results suggest that bsy-miR397 play a role in fenoxaprop-P-ethyl NTSR in B. syzigachne by down-regulating laccase expression, leading to changes in oxidase/peroxidase genes. To our knowledge, this is the first case of how down-regulation of a gene (laccase) can enhance NTSR. Our study also shows that down-regulation of laccase by miRNA has a causative role on certain oxidase/peroxidase genes.
Materials and Methods
Plant Material and Growth Conditions
Three fenoxaprop-P-ethyl-resistant B. syzigachne populations possessing both NTSR and TSR and one susceptible population (S) were used in this study (Pan et al., 2016). These populations were collected from the following locations: S from Feidong in Anhui Province, where fenoxaprop-P-ethyl was never applied; R1 from Xinlucun in Jiangsu Province with 10 years of fenoxaprop-P-ethyl use history; R2 from Lijiacun in Jiangsu Province, with 6 years of fenoxaprop-P-ethyl use; and R3 from Guocun in Jiangsu Province, with 5 years of fenoxaprop-P-ethyl use. These locations were more than 118 km apart from each other.
In our previous studies, we only compared the differences in gene expression between one resistant and one susceptible population, which might yield few “false positive” alleles that show differential expression between populations, but irrelevant to NTSR. Therefore, in the present study, we used three fenoxaprop-P-ethyl-resistant populations with different genetic backgrounds to minimize “false positives.”
Seeds were planted in pots filled with a 2:1 (wt/wt) mixture of sand and loam soil (pH 5.6; organic matter content 1.4%). All pots were placed in a greenhouse at 20°C/15°C day/night under a photoperiod of 12 h/12 h.
Tobacco (Nicotiana benthamiana) plants were grown in vermiculite containing Murashige and Skoog salt nutritional liquid in a growth chamber (light:dark period of 12:12 h at 25°C:18°C). Six-week-old tobacco plants were used for Agrobacterium tumefaciens-mediated transient expression as described (Chen et al., 2008).
The rice (Oryza sativa L.) wild type (WT) and miR397-overexpressing transgenic (OXmiR397) plants were kindly provided by Prof. Yueqin Chen from the Sun Yat-sen University (Zhang et al., 2013). The details about the rice plants were described (Zhang et al., 2013). Rice plants were grown in the culture solution (Zhang et al., 2013) supplemented with fenoxaprop-P-ethyl in a glasshouse with a day/night cycle of 12 h/12 h at 30°C/24°C.
Quantitative Reverse-Transcriptase Polymerase Chain Reaction of bsy-miR397 and bsy-Laccase in B. syzigachne
From the B. syzigachne mRNA sequence library (PRJNA290808) and miRNA sequence library (PRJNA290416) established in our laboratory, the B. syzigachne laccase gene (accession number: KP952245) and the B. syzigachne miR397 (sequence: 5′-ATTGAGTGCAGCGTTGATGAA-3′) were detected, and they were named as bsy-Laccase and bsy-miR397, respectively.
In total 300 plants from each of the three populations were cultivated to the tillering stage in 3-inch pots at day/night temperature of 20°C/15°C under a photoperiod of 12 h/12 h. Two tillers were collected from each plant: one for resistance/susceptibility analysis and the other for seed production. Resistance/susceptibility tested to fenoxaprop-P-ethyl was tested at the recommended field dose (62.0 g a.i.⋅ha-1), and survival assessed 3 weeks after treatment. The confirmed resistant tillers were placed in a pollen mask to produce progenies. In total, 15 susceptible and 285 resistant individuals were confirmed in R1 population; 15 susceptible and 285 resistant individuals in R2 population; and 16 susceptible and 284 resistant individuals in R3 population. The resistance tillers were self-pollinated in isolation to produce seeds of progeny populations. Thus, three resistance-enriched populations (R1, R2, and R3) were generated (Pan et al., 2016). With this method, three “purified” progeny R populations were obtained (thus minimizing genetic variation unrelated to fenoxaprop-P-ethyl resistance).
Plants from each population were cultivated up to the 3- to 4-leaf stage under the experimental conditions described above. Fenoxaprop-P-ethyl was applied at one-eighth of the recommended dose to allow survival of susceptible plants for gene expression analysis (Pan et al., 2016). The expression of candidate genes was measured in 30 untreated and 30 fenoxaprop-P-ethyl-treated plants for each fenoxaprop-P-ethyl dose at 24, 48, and 72 h. Total RNA was extracted using TRIzol (Invitrogen Inc., Carlsbad, CA, United States) following the manufacturer’s protocol. The quality and purity of RNA were assessed using the Nanodrop 2000 spectrophotometer (Thermo Scientific, Wilmington, DE, United States). Each RNA sample (10 μg) was subjected to reverse transcription using the Prime Script RT Reagent Kit (TaKaRa, Dalian, China) to produce cDNA. Small RNAs were reverse transcribed to cDNA using the Prime ScriptTM miRNA qPCR Starter Kit (Version 2.0, TaKaRa, Dalian, China). The absence of gDNA contamination was confirmed by lack of amplification of the reference gene glyceraldehyde-3-phosphate dehydrogenase (GAPDH) using the primers containing an intron sequence.
Real-time PCR was performed using the ABI-7500 Fast Real-Time PCR System (ABI, United States) by using the SYBR® Premix Ex TaqTM kit (TaKaRa, Japan).
The relative expression of genes to that of the control was calculated using the 2-ΔΔCT method (Livak and Schmittgen, 2001). In each experiment, qPCR was conducted on each single plant (creating 30 data points) and repeated at least twice (two technical replicates). Significant differences in expression levels were analyzed using Welch’s t-test (Zheng et al., 2013). Two threshold values—a significant result in the t-test (P < 0.05) and a two-fold change were used to determine either up- or down-regulation. Capsine phosphatase, GAPDH, and ubiquitin were used as internal controls. The qPCR primers were designed based on the bsy-Laccase and bsy-miR397 sequences. The primers used for qRT-PCR are listed in Supplementary Tables S1, S2. The reverse primer 5′-GTGCAGGGTCCGAGGT-3′ was used for bsy-miR397 amplification.
We also included three populations (possessing TSR and in which metabolic resistance might not be involved) for comparison. These three populations were JYDX-R, SHQP-R, and JYSC-R. ACCase sequencing results showed that all plants in JYDX-R contained the Ile-1781-Leu mutation, those in SHQP-R contained the Ile-2041-Asn mutation, and those in JYSC-R contained the Gly-2096-Ala mutation. Next, 50 randomly selected plants from each of these populations were treated with fenoxaprop-P-ethyl + piperonyl butoxide (PBO, a known P450 inhibitor), and 20 randomly selected plants were treated with fenoxaprop-P-ethyl alone. No phenotypic differences were observed between plants subjected to fenoxaprop-P-ethyl alone and fenoxaprop-P-ethyl + PBO. When the three populations were compared with the S population, no difference was found in the structural and expressions of the 15 genes related to metabolic resistance. Our previous studies showed that PBO can partially reverse fenoxaprop resistance and that the 15 genes played a role in metabolic resistance in the three resistant populations R1, R2, and R3 (Pan et al., 2016); thus, populations JYDX-R, SHQP-R, and JYSC-R likely exhibited only TSR. The expression profiles of bsy-miR397 and bsy-Laccase in JYDX-R, SHQP-R, and JYSC-R populations were analyzed as described above.
Laccase Extraction and Assay
Laccase was isolated using the protocol of Wang et al. (2004) with slight modifications. Leaf samples of the S, R1, R2, R3 and JYDX-R, SHQP-R, JYSC-R plants were harvested at the 3- to 4-leaf stage. Crude laccase was extracted from the leaf material (200 mg fresh weight) in 1 mL buffer (25 mM MOPS, 200 mM CaCl2, pH 7.0), followed by centrifugation (16,000 × g, 10 min). Ten microliters of crude enzyme (about 6 μg protein) was added to a 0.5-mL mixture, and the reaction was conducted at 30°C for 30 min. Coomassie brilliant blue method was used to quantify the (total) protein using bovine serum albumin as the standard. Laccase activity prior to and after fenoxaprop treatment (24, 48, and 72 h) was determined colorimetrically by measuring the oxidation rate of 2 mg/mL 2,2-azobis (3-ethylbenzothiazoline-6-sulphonic acid) at 420 nm. The test was performed twice with three replicates per treatment.
Co-expression of bsy-miR397 and bsy-Laccase in Tobacco
The bsy-miR397 and bsy-Laccase co-transformation in tobacco leaves was used to validate the interaction between bsy-miR397 and bsy-Laccase genes. A full-length (1,740 bp) cDNA of bsy-Laccase was RT-PCR amplified from B. syzigachne by using the primers listed in Supplementary Table S3, and the sequence-confirmed PCR fragment was inserted into the pEarleyGate vector 202 under the control of a 35S promoter (Earley et al., 2006). The amiR-bsy-miR397 was constructed using a previously described method (Warthmann et al., 2008). The primers for amiR-bsy-miR397 amplification are listed in Supplementary Table S3. A 21-mer sequence was used to replace the endogenous miRNA and miRNA∗ in the plasmid pRS300 (Schwab et al., 2006) designed using the Web MicroRNA Designer platform. The resulting amiRNA was cloned into pEarleyGate vector 201 under the control of a 35S promoter. All constructs were sequenced to confirm the intended construction and designs. Descriptions of the amiRNAs, their target sequences, and genes are listed in Supplementary Table S4.
The Agrobacterium strain GV3101 was used to transfect the recombinant vectors into tobacco leaf cells. The strains containing different recombinant vectors (35S:bsy-miR397, 35S:bsy-Laccase, and 35S:Os-miR164-control) were cultured to OD600 = 0.8 at 28°C in a shaker at 220 rpm. Each cultured sample was collected and diluted to OD600 = 0.5 with MgCl2 and acetosyringone. The 35S:bsy-laccase and 35S:bsy-miR397 samples were mixed in equal volumes, and the mixture was adjusted to OD600 = 1.0 and used to test the cleavage function of bsy-miR397. In the tobacco assay, laccase needs to be co-expressed with other non-effect miRNAs as control. As Os-miR164 has no effect on laccase, we co-expressed Os-miR164 and laccase to test our hypothesis. Subsequently, 100 μL of each treatment sample was used to infiltrate the tobacco leaves. The agrobacteria were infiltrated into the tobacco leaves, and treated leaves were harvested 2 days later. Total RNAs were extracted for qRT-PCR. Tobacco 18S rRNA expression was used as an internal standard for normalization. Primers used to detect the expression of bsy-Laccase and bsy-miR397 in these plants are listed in Supplementary Tables S1, S2.
Sensitivity to Fenoxaprop-P-ethyl and Other Herbicides in OXmiR397-Overexpressing Rice
Wild type and OXmiR397-overexpressing transgenic rice seeds were planted in pots containing commercial potting soil (Zhang et al., 2013) and maintained in a well-watered state in a greenhouse. When the seedlings reached the 3- to 4-leaf stage, fenoxaprop-P-ethyl was applied at the recommended field dose (62 g a.i.⋅ha-1) to the WT and OXmiR397 rice lines. Whether miR397/laccase is involved specifically in fenoxaprop-P-ethyl resistance was determined by including another ACCase-inhibiting herbicide haloxyfop-R-methyl (recommended field dose, 40.5 g a.i.⋅ha-1). Three weeks after treatment, the root length, plant height, and fresh weight were determined for each plant.
The target for OXmiR397 in rice is LOC_Os05g38420 (OsLAC) (Jeong et al., 2011; Zhang et al., 2013). The OsLAC expression and laccase activity were quantified in rice as described above. The primers were OsLAC-F (5′-GAGGAGGTGCCCATCATGTTC-3′) and OsLAC-R (5′-CCTTCAGCTTAAACGTGTCTTGG-3′) were used for quantification of OsLAC expression.
B. syzigachne Sensitivity to Fenoxaprop-P-ethyl Following Treatment with 20 mM CuSO4
Laccase expression has been shown to be induced by 20 mM CuSO4 (Zhu and Ding, 2003; Jin et al., 2008). This approach was also used to determine the role of miR397/laccase in fenoxaprop-P-ethyl resistance in B. syzigachne. Induction of the bsy-Laccase expression in B. syzigachne by 20 mM CuSO4 was confirmed by comparing the expression level of bsy-Laccase with and without CuSO4 treatment.
Seeds from the S and three B. syzigachne populations possessing both TSR and NTSR were germinated using the method described previously (Pan et al., 2015b). The 3-week-old (at the 3- to 4-leaf stage) plants were treated with fenoxaprop-P-ethyl alone, and 20 mM CuSO4 plus fenoxaprop-P-ethyl using a laboratory sprayer as described previously (Pan et al., 2015b). The experiments were conducted twice in a completely randomized design with four replicates per treatment. Herbicide application rates were selected around the GR50 value of each population. Fenoxaprop-P-ethyl was applied at 0, 5, 10, 20, 40, 80, and 160 g a.i. ha-1 to the susceptible population (S) and at 0, 80, 160, 320, 640, 1280, and 2560 g a.i. ha-1 to the resistant populations (R1, R2, and R3). CuSO4 was applied 1 h before fenoxaprop-P-ethyl application. Three weeks after treatment, the above-ground material was harvested, and fresh weights were determined.
The experiments with fenoxaprop-P-ethyl and 20 mM CuSO4 plus fenoxaprop-P-ethyl treatments in JYDX-R, SHQP-R, and JYSC-R populations were conducted as described above.
RNA-Seq Data Processing and Differentially Expressed Transcription Factor Analysis in B. syzigachne
MiR397 has been implicated in the network for lignin biosynthesis via putative interactions with several transcription factors (TFs) and laccases in Populus trichocarpa (Lu et al., 2013). The possible mechanism of miR397/laccase in regulating plant resistance to fenoxaprop-P-ethyl was assessed by constructing B. syzigachne RNA-seq libraries using RNA from the S population and three R populations (R1, R2, and R3) (Pan et al., 2016). The four libraries were assayed for quality and quantity and sequenced by Beijing BioMarker Technologies (Beijing, China). The mRNA-Seq libraries were constructed and sequence data were analyzed, assembled, and annotated as described by Pan et al. (2016). Gene names of specific TFs were assigned based on the best Basic Local Alignment Search Tool hit (highest score), excluding any uninformative descriptions.
The results of the comparison between S and R libraries suggested that RSEM could be used to quantify the reads identified as TFs (Li and Dewey, 2011). Transcript levels were quantified by counting reads per kilobase of exon model per million mapped reads (RPKM) (Mortazavi et al., 2008). The RPKM method of read density reflects the molar concentration of a transcript by normalizing for RNA length and the total number of reads. The EBSeq software was used to determine P-values for the samples with no biological replicates. EBSeq employs an empirical Bayes hierarchical model (Leng et al., 2013). A two-fold change, a P-value of <0.01, and a minimum RPKM of 2 in at least one of the treatments were used as the criteria to classify a response as up- or down-regulation. TFs were found to be consistently up- or down-regulated in R1, R2, and R3.
The downstream effect of laccase down-regulation on the up-regulated pathways that contribute to NTSR against fenoxaprop were further confirmed by conducting additional experiments that detected the expressions of these TFs and oxidase/peroxidase genes in WT and OXmiR397 rice. Primers used for detecting their expression are listed in Supplementary Table S5.
Results
Expression of bsy-miR397 and bsy-laccase in B. syzigachne
Quantitative PCR was used to analyze the expression of bsy-miR397 and bsy-Laccase in 30 plants each of the S, R1, R2, R3, and JYDX-R, SHQP-R, JYSC-R population. Expression of bsy-miR397 was significantly higher in the 30 plants each of the R1, R2, and R3 populations than in the S population, whereas the expression of bsy-Laccase was significantly higher in the S plants than in the R1, R2, and R3 plants (Figure 1). The expression of bsy-miR397 was 8–9 times higher in plants of the R1, R2, and R3 populations than in the S population (Figure 1). In contrast, the expression of bsy-Laccase was 8–9-times lower in the plants of the R1, R2, and R3 populations than in the S population (Figure 1). Notably, we found that in JYDX-R, SHQP-R, and JYSC-R plants that likely exhibited only TSR, the expression patterns of bsy-miR397 and bsy-Laccase were consistent with that in the S plants (Figure 1).
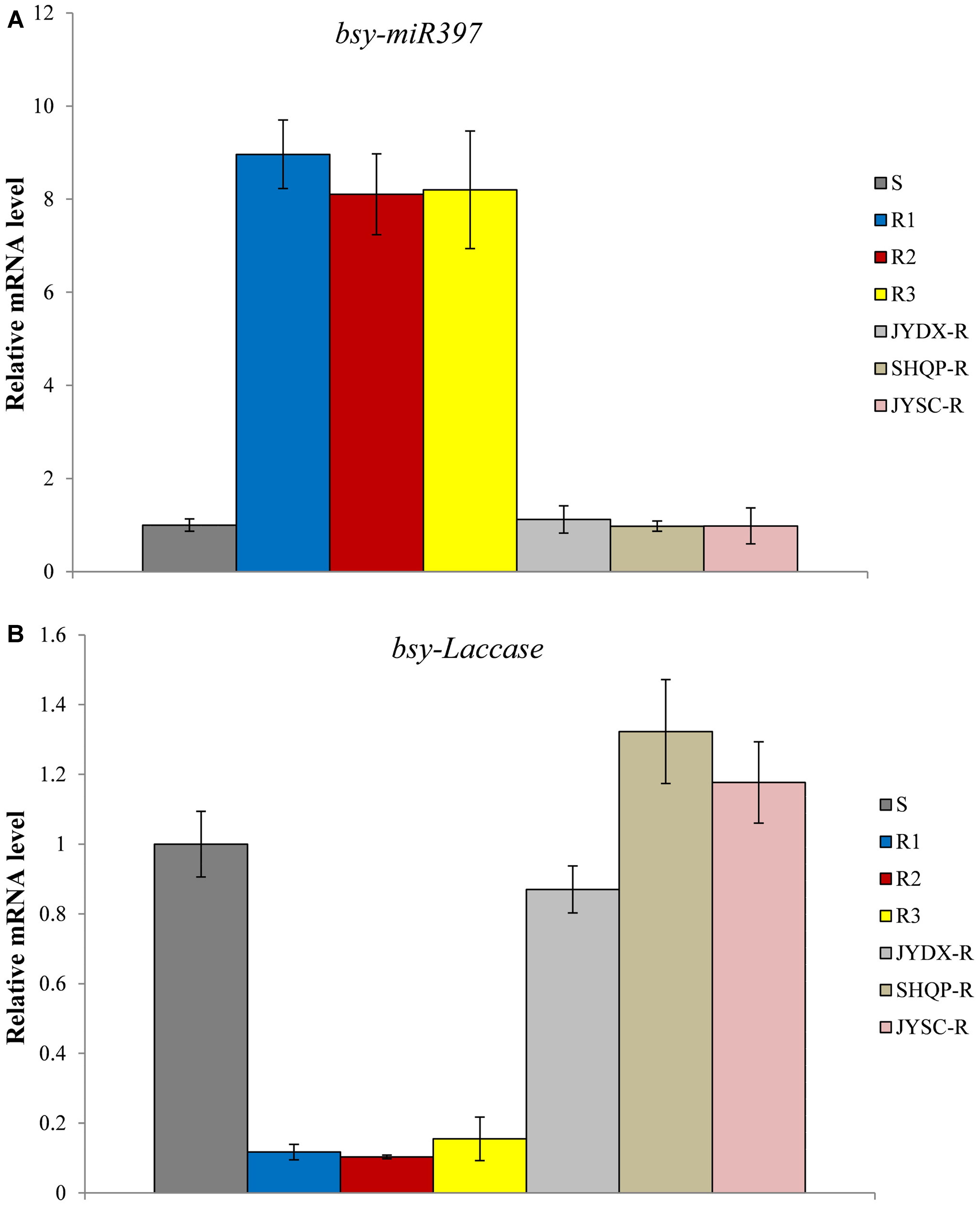
FIGURE 1. The relative expression levels of bsy-miR397 and bsy-Laccase in sensitive (S) and resistant (R) plants of Beckmannia syzigachne. (A) bsy-miR397, (B) bsy-Laccase. qPCR was conducted on each single plant (creating 30 data points) and repeated at least twice (two technical replicates). Data are the mean values of these replicates. The standard errors of the means are described by vertical bars. S, collected from where fenoxaprop-P-ethyl was never applied; R1, R2, and R3, three fenoxaprop-P-ethyl-resistant populations possessing both NTSR and TSR; JYDX-R, SHQP-R, and JYSC-R, fenoxaprop-P-ethyl-resistant populations exhibited only TSR.
The time-response expression profiles of bsy-miR397 and bsy-Laccase were determined in 30 plants each of the S, R1, R2, R3 and JYDX-R, SHQP-R, JYSC-R populations at 24, 48, and 72 h after fenoxaprop-P-ethyl treatment. In the S and JYDX-R, SHQP-R, JYSC-R plants, the expression of bsy-Laccase and bsy-miR397 remained unchanged (Figure 2). However, in the R1, R2, and R3 plants, after fenoxaprop-P-ethyl treatment, the expression of bsy-miR397 was up-regulated, whereas bsy-Laccase down-regulated (Figure 2). This was consistent with the expression of these two genes in the untreated R1, R2, and R3 plants. These data indicate a negative correlation between bsy-miR397 and bsy-Laccase expression both constitutively and in response to fenoxaprop-P-ethyl treatment in B. syzigachne R1, R2, and R3 populations.
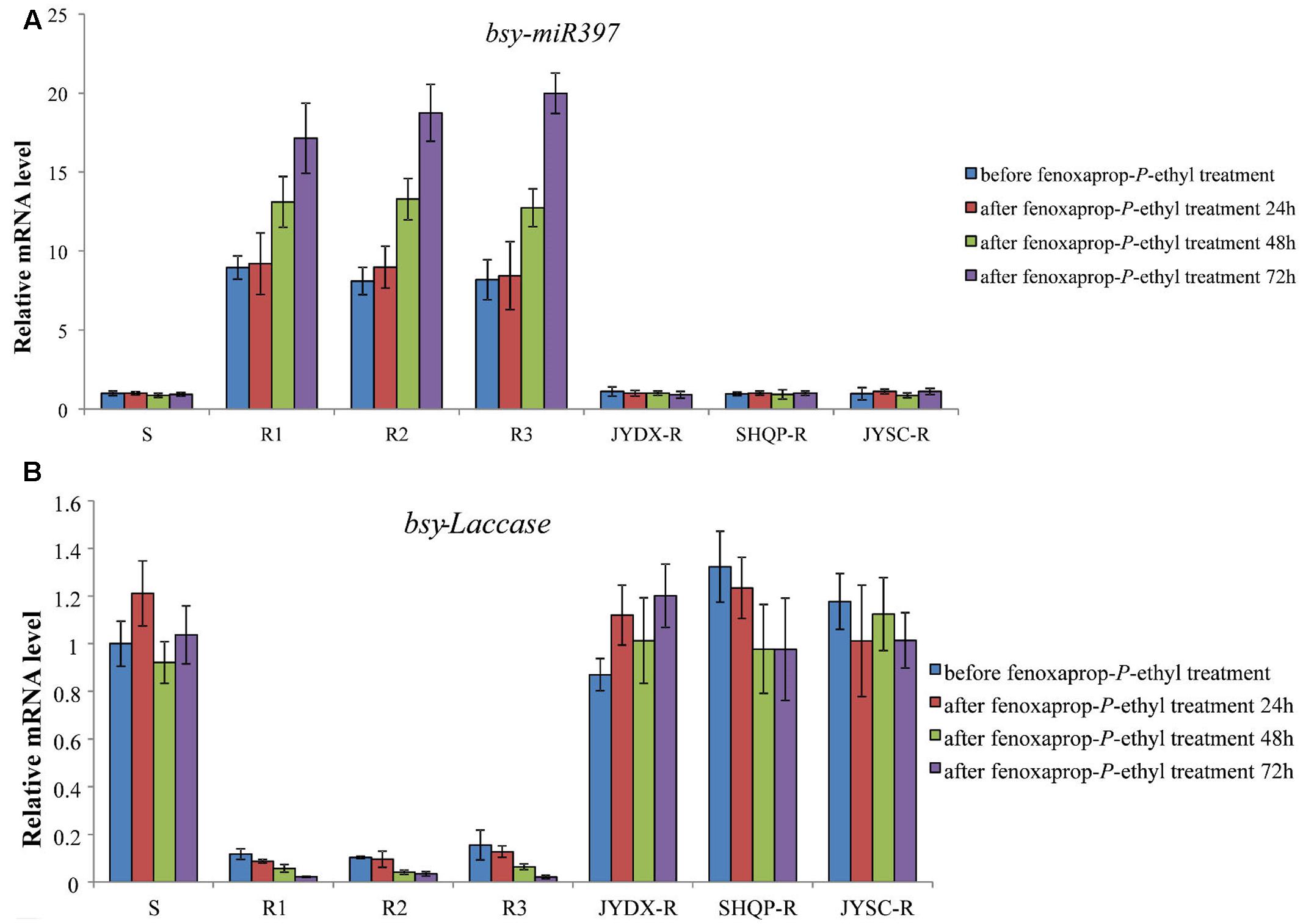
FIGURE 2. The relative expression patterns of bsy-miR397 and bsy-Laccase in sensitive (S) and resistant (R) plants of Beckmannia syzigachne before and after fenoxaprop treatment (24, 48, and 72 h). (A) bsy-miR397, (B) bsy-Laccase. qPCR was conducted on each single plant (creating 30 data points) and repeated at least twice (two technical replicates). Data are the mean values of these replicates. The standard errors of the means are described by vertical bars.
Laccase Activity in B. syzigachne
Consistent with bsy-Laccase expression in B. syzigachne, a higher level (5.8- to 8.6-fold) of laccase activity was detected in the S than in R1, R2, and R3 plants (P < 0.05; Figure 3A). These results are consistent with those for bsy-Laccase gene expression, suggesting that laccase might play a role in fenoxaprop-P-ethyl resistance of B. syzigachne.
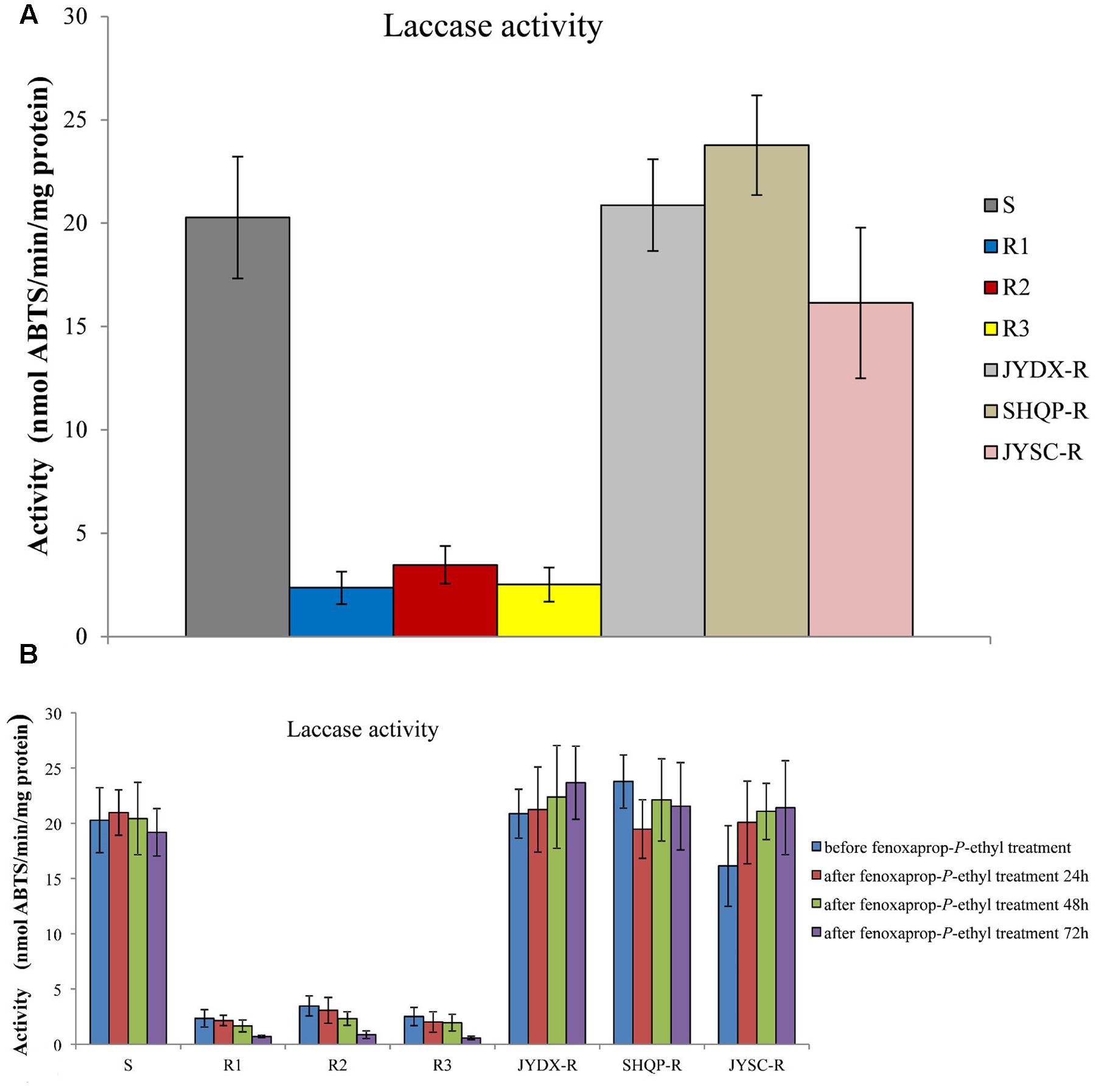
FIGURE 3. Laccase activities in sensitive (S) and resistant (R) plants of Beckmannia syzigachne were determined by monitoring the oxidation (nmol 2,2-azobis [3-ethylbenzothiazoline-6-sulphonic acid]⋅min-1⋅mg-1 protein) of ABTS at 420 nm. (A) Laccase activities before fenoxaprop treatment, (B) laccase activities before and after fenoxaprop treatment (24, 48, and 72 h). Data are the mean values of six biological replicates. The standard errors of the means are described by vertical bars.
However, after fenoxaprop-P-ethyl treatment, laccase activity in R1, R2, and R3 plants decreased relative to that in the untreated R1, R2, and R3 plants (Figure 3B), whereas in the S plants laccase activity did not change considerably. We found that in JYDX-R, SHQP-R, and JYSC-R plants, laccase activity were consistent with that in the S plants either before or after fenoxaprop-P-ethyl treatment (Figure 3). These results are also consistent with the bsy-Laccase expression pattern in S and R1, R2, and R3 plants after fenoxaprop-P-ethyl treatment.
Bsy-Laccase Expression Was Repressed by bsy-miR397 in Tobacco
In rice, the expression of laccase genes is known to be regulated by miRNA397 (Zhang et al., 2013). The target gene of bsy-miR397 is predicted to be bsy-Laccase from http://www.mirbase.org, and the 714- to 734-bp region on the bsy-Laccase gene might be a target site for bsy-miR397. Hence, we hypothesized that bsy-Laccase gene could be cleaved by bsy-miR397. Since co-expression in tobacco is one of the best ways to validate the interaction between an miRNA and its predicted target (Qi et al., 2012), we co-expressed the bsy-miR397 and bsy-Laccase genes in tobacco.
Quantitative RT-PCR analyses revealed that the bsy-Laccase expression in tobacco carrying 35S:bsy-Laccase/35S:bsy-miR397 was 3.6-fold lower than that in tobacco plants carrying only 35S:bsy-Laccase (Supplementary Figure S1). These results indicate that bsy-Laccase expression was repressed by bsy-miR397 in transformed tobacco carrying 35S:bsy-Laccase/35S:bsy-miR397, and bsy-Laccase might be the target gene of bsy-miR397.
Improved Tolerance to Fenoxaprop-P-ethyl in OXmiR397-Overexpressing Rice
As miR397 is conserved across most dicotyledon and monocotyledon species, and fenoxaprop-P-ethyl has no activity in broadleaf tobacco, we attempted to further investigate the role of miR397/laccase in fenoxaprop-P-ethyl resistance by using OXmiR397-overexpressing transgenic rice and the corresponding WT lines.
Rice OXmiR397 is known to regulate laccase. To determine if this regulation has an impact on herbicide tolerance, we treated WT and OXmiR397-overexpressing transgenic rice with the field rate of fenoxaprop-P-ethyl (62 g a.i. ha-1), with untreated rice plants as a control. Seven days after fenoxaprop-P-ethyl treatment, WT rice plants started to show phytotoxicity symptoms such as leaf withering and reduced plant height, whereas OXmiR397-overexpressing plants showed no visual damage. Three weeks after treatment, WT rice plants showed severe tissue damage, whereas OXmiR397-overexpressing plants remained largely healthy and green (Figure 4). In addition, OXmiR397-overexpressing plants exhibited significantly greater root length and plant height (Figure 4 and Table 1) as well as overall fresh weight than the WT rice (Figure 4 and Table 1). Six weeks after fenoxaprop-P-ethyl treatment, WT plants died, whereas OXmiR397-overexpressing plants only showed slight tissue damage (which occurred about a week later than the WT plants and never recovered). As expected, OXmiR397-overexpressing plants had lower levels of OsLAC expression and laccase activity than the WT plants (Figure 5). These results indicated that miR397/laccase regulation can also provide protection from fenoxaprop-P-ethyl damage in OXmiR397-overexpressing transgenic rice, suggesting that miR397/laccase might be involved in fenoxaprop-P-ethyl NTSR.
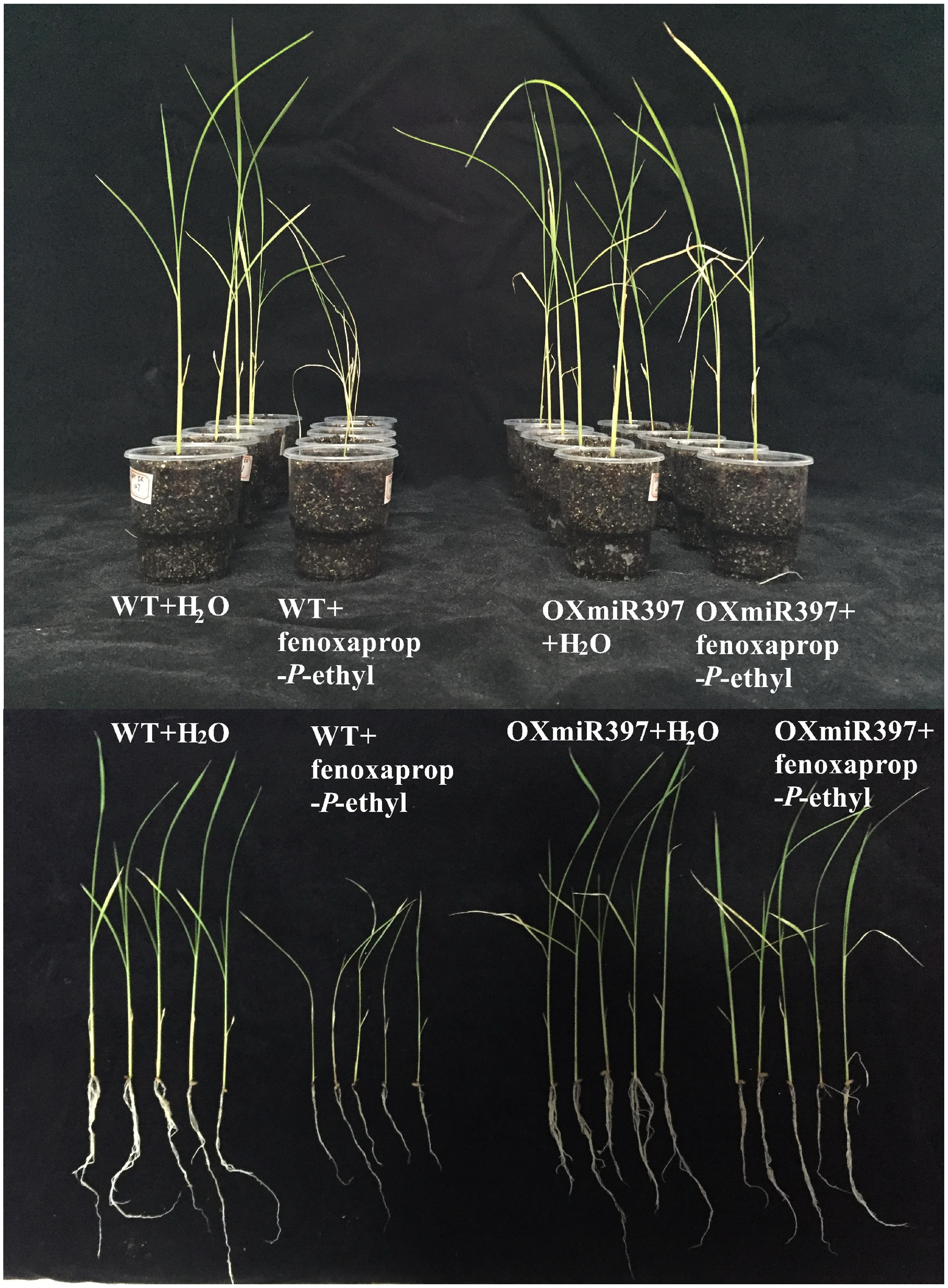
FIGURE 4. Overexpression of rice miR397 gene improves fenoxaprop-P-ethyl tolerance in rice. WT, wild type.
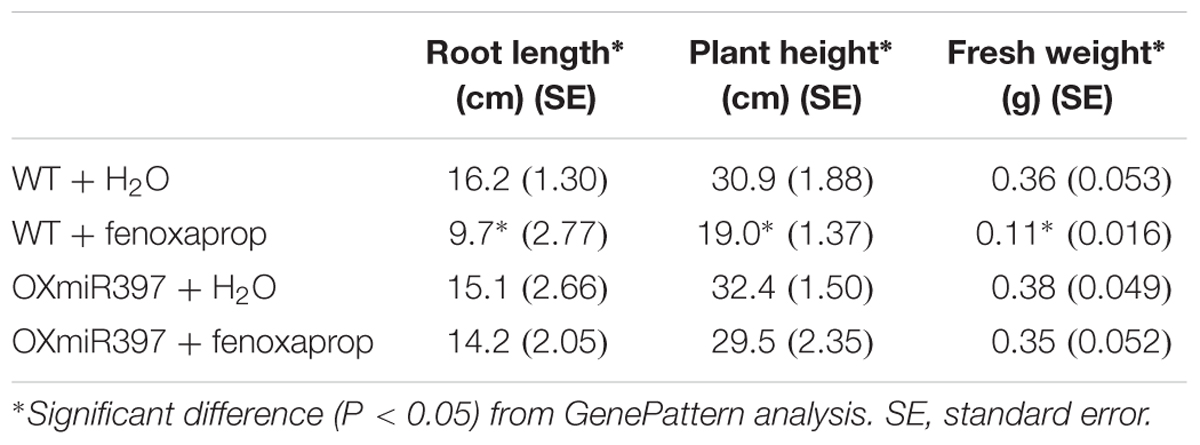
TABLE 1. Root length, plant height, and fresh weight in OXmiR397 overexpressing and WT rice plants treated with fenoxaprop (at the recommended field dose).
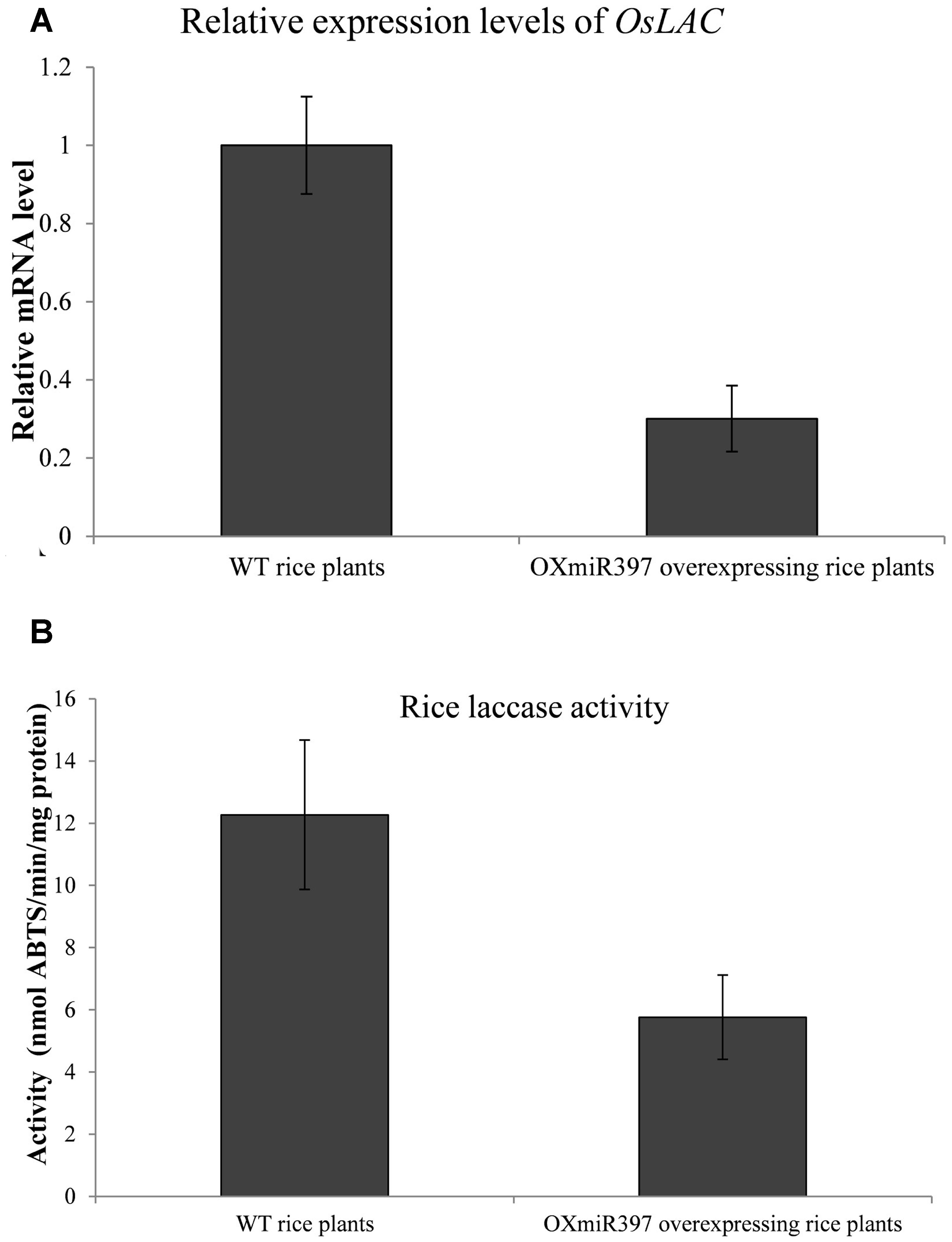
FIGURE 5. Relative expression levels of OsLAC mRNA (A) and laccase acitivity (B) in wild type (WT) and OXmiR397-overexpressing rice plants. qRT-PCR used three independent biological replicates and repeated at least twice (two technical replicates). Data are the mean values of six biological replicates. The standard errors of the means are described by vertical bars.
In contrast, 3 weeks after treatment with another ACCase herbicide haloxyfop-R-methyl at the recommended field dose, OXmiR397-overexpressing rice and WT plants all exhibited significantly smaller root length and plant height than that without haloxyfop-R-methyl treatment (Table 2). Six weeks after treatment, they all died. This result suggested that the miR397/laccase pathway might not be a general defense mechanism against all herbicides, but likely specific to fenoxaprop-P-ethyl.
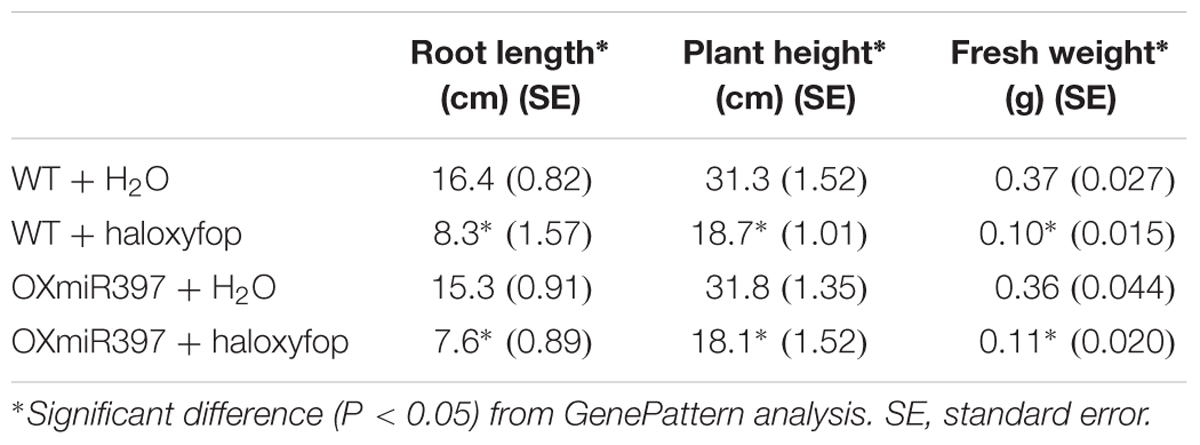
TABLE 2. Root length, plant height, and fresh weight in OXmiR397 overexpressing and WT rice plants treated with haloxyfop (at the recommended field dose).
Impact of 20 mM CuSO4 on Fenoxaprop-P-ethyl Resistance in B. syzigachne
In this experiment, 3-week-old (at the 3- to 4-leaf stage) B. syzigachne plants were used. Treatment with 20 mM CuSO4 alone did not have a visual effect on B. syzigachne plants (Table 3). However, bsy-Laccase expression was significantly induced 1 h after CuSO4 treatment in all populations (Figure 6). Fenoxaprop-P-ethyl treatment in combination with 20 mM CuSO4 increased herbicide toxicity in the three B. syzigachne R populations, with a slight (but significant) reduction of the GR50 (the effective rate of herbicide causing 50% inhibition in plant fresh weight) value (Table 3), whereas the GR50 value of the S population did not change significantly. These results indicate that the activation of bsy-Laccase by CuSO4 in fenoxaprop-P-ethyl-resistant B. syzigachne can have an impact on resistance.
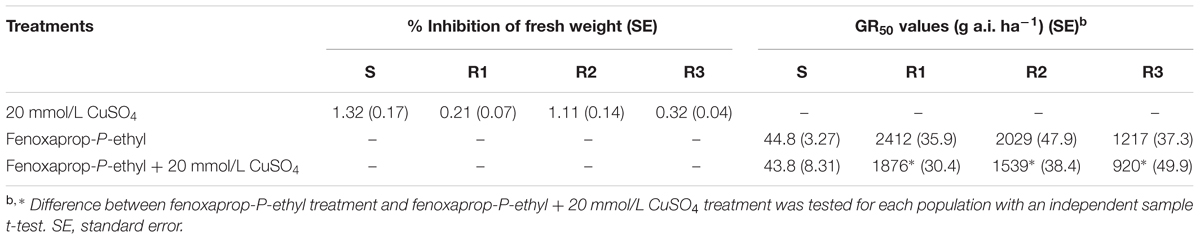
TABLE 3. Effects of fenoxaprop-P-ethyl and 20 mmol L-1 CuSO4 on growth of resistant R1, R2, and R3 Beckmannia syzigachne plants.
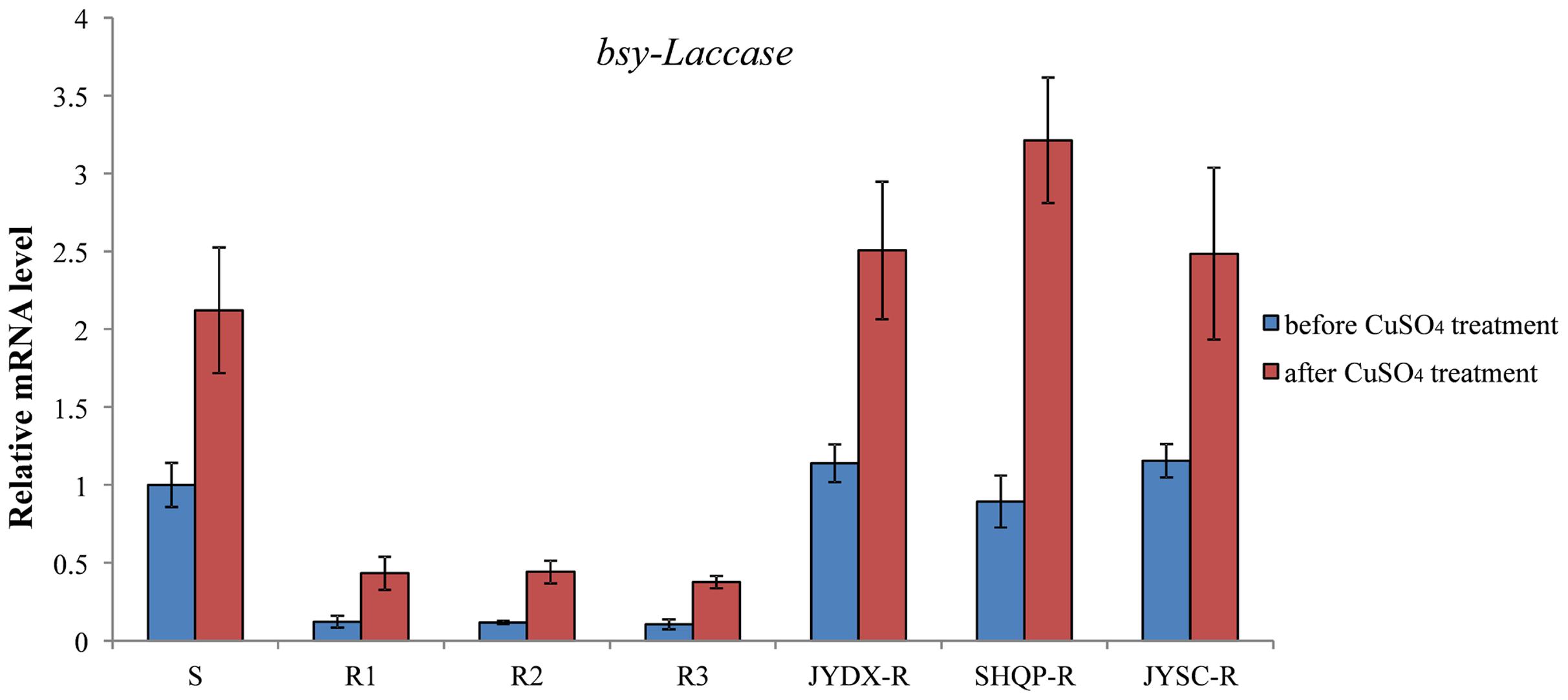
FIGURE 6. The expression level of bsy-Laccase before and after CuSO4 treatment in B. syzigachne. Data are the mean values of replicates. The standard errors of the means are described by vertical bars.
However, CuSO4 treatment did not increase fenoxaprop-P-ethyl toxicity in JYDX-R, SHQP-R, and JYSC-R populations which mainly exhibited TSR (Table 4), indicating that laccase might play a role in B. syzigachne R1, R2, and R3 populations.

TABLE 4. Effects of fenoxaprop-P-ethyl and 20 mmol L-1 CuSO4 on growth of JYDX-R, SHQP-R, JYSC-R Beckmannia syzigachne plants.
Differentially Expressed TF Analysis in B. syzigachne
From the RNA-seq libraries established in our previous study (Pan et al., 2016), we found 587 genes that were annotated as TFs. Of these, four TFs were consistently down-regulated in R1, R2, and R3, whereas the rest of them did not exhibit consistent changes in expression. The expression of two myeloblastosis (MYB) family genes (bsy-MYB39 and bsy-MYB2) and two auxin response factor (ARF) genes (bsy-ARF5 and bsy-ARF8) remained significantly lower in the R1, R2, and R3 plants than in the S plants (Table 5). In particular, the expression of bsy-MYB39, bsy-MYB2, bsy-ARF5, and bsy-ARF8 was about 3–6 times lower in the R than in the S plants (Table 5).

TABLE 5. Identification of TF (transcription factors) genes down-regulated in resistant (R1, R2, and R3) and susceptible (S) Beckmannia syzigachne plants using the RPKM method for fold-change.
To further confirm the downstream effect of laccase down-regulation on the up-regulated pathways that contributes to NTSR against fenoxaprop, we analyzed the expression of the four TFs and four oxidase/peroxidase genes in WT and OXmiR397 rice. The results showed that the expression of Os-peroxidase 66 and Os-ARF8 remained unchanged between WT and OXmiR397 rice. However, the expression levels of Os-ARF5, Os-MYB2, and Os-MYB39 were significantly lower in the OXmiR397 rice, whereas those of Os-l-ascorbate oxidase, Os-ubiquinol oxidase 1, and Os-peroxidase 1 were significantly higher (Figure 7). In particular, the expression of Os-MYB2 (Figure 7A), Os-MYB39 (Figure 7B), and Os-ARF5 (Figure 7C) was, respectively, 6.2, 3.5, and 3.0 times lower in OXmiR397 rice than in WT rice. In contrast, the expression of Os-l-ascorbate oxidase (Figure 7D), Os-ubiquinol oxidase 1 (Figure 7E), and Os-peroxidase (Figure 7F) was, respectively, 4.1-, 4.4-, and 2.2-fold higher in OXmiR397 rice than in WT rice.
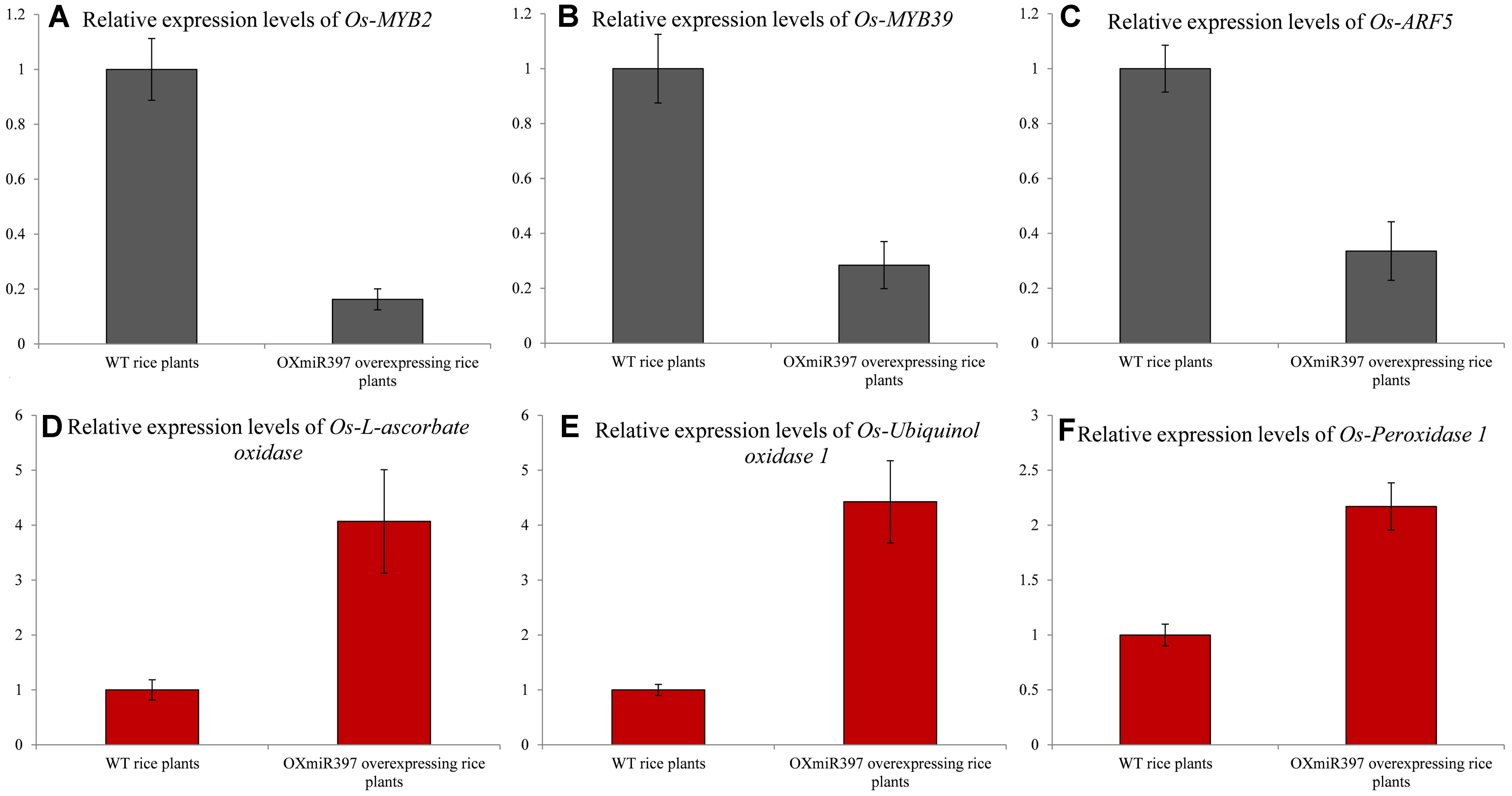
FIGURE 7. The relative expression levels of the three transcription factors and three oxidase/peroxidase genes consistent with B. syzigachne in wild type (WT) and OxmiR397 rice. (A) Os-MYB2, (B) Os-MYB39, (C) Os-ARF5, (D) Os-l-ascorbate oxidase, (E) Os-Ubiquinol oxidase 1, (F) Os-Peroxidase. qRT-PCR used three independent biological replicates and repeated at least twice (two technical replicates). Data are the mean values of six biological replicates. The standard errors of the means are described by vertical bars.
The relative expression levels of these three TFs and three oxidase/peroxidase genes are consistent with those in B. syzigachne, indicating that ARF5, MYB2, MYB39, l-ascorbate oxidase, ubiquinol oxidase 1, and peroxidase 1 might be involved in the regulatory network with bsy-miR397/bsy-Laccase.
Discussion
Several studies have revealed that miR397 has pivotal roles in plant responses to diverse abiotic stresses including H2O2, cold, dehydration, NaCl, and abscisic acid (Sunkar and Zhu, 2004; Luo et al., 2006). However, no previous studies have investigated the contribution or underlying molecular mechanisms of miR397 involvement in herbicide resistance. In this study, we experimentally confirmed that miR397 is likely involved in fenoxaprop resistance in B. syzigachne and in the improvement of fenoxaprop tolerance in rice via the negative regulation of laccases.
Laccases are known to act as targets of miR397 (Jones-Rhoades and Bartel, 2004). Bsy-Laccase was predicted to be a target gene of bsy-miR397, and our experiments performed using transient co-expression of bsy-Laccase and bsy-miR397 in tobacco confirmed this possibility (Supplementary Figure S1). It is worth to note that bsy-miR397 mediated suppression of bsy-Laccase gene in tobacco was only 3.6-fold. To increase the level of suppression to get increased NTSR, in the future we can try an inducible Gateway-compatible expression vector that allows tighter control of gene expression than previously designed inducible systems (Earley et al., 2006). This ‘double-lock’ inducible system remains sequestered in the cytoplasm until dexamethasone treatment, which allows the protein to move into the nucleus, catalyze the removal of the sequence blocking transcription by the 35S promoter, and thereby allow expression of the target gene (Joubès et al., 2004).
Laccases are four copper atom-containing glycoproteins that catalyze the oxidation of a suitable substrate molecule leading to the production of water and oligomers, including diphenol, dinaphthol, phenylenediamine, and anthranilate-derived metabolites (LaFayette et al., 1999). Cupric ions are present in the active sites of laccases. Cupric ions induce laccase activity by combining the free ω-carboxylic group anions of acidic amino acid residues around the active sites of laccases and affect the charge distribution during electron transfer (Zhu and Ding, 2003; Jin et al., 2008). Unlike for fungal laccases (Mayer and Staples, 2002), the roles of plant laccases are not yet completely understood, although they might be related to lignin biosynthesis (Ranocha et al., 2002) and copper homeostasis (Claus, 2004). They are required for the maintenance of copper homeostasis in many processes such as energy transduction and oxidative stress responses (Arredondo and Nunez, 2005). In turn, the down-regulation of laccases might limit some non-essential biological processes to allow the conservation of energy for defense purposes and to reserve required elements for the most essential functions under adverse stress conditions (Abdel-Ghany and Pilon, 2008). The activation of miR397 expression down-regulates laccase gene expression, suggesting that copper-responsive miRNAs reserve copper for more essential proteins such as cytochrome c oxidase (belonging to oxidases). Oxidases have been indicated to play an important role in NTSR for metabolizing herbicides (Délye, 2013).
The results of this study showed that the level of miR397 was higher in the resistant than in the susceptible B. syzigachne plants (Figures 1, 2). Furthermore, transgenic rice plants overexpressing miR397 showed normal growth, whereas the untransformed WT plants showed severe symptoms (e.g., dwarfism and etiolation; Figure 4), following fenoxaprop-P-ethyl treatment. In addition, 20 mM CuSO4 was used to activate bsy-Laccases in B. syzigachne (Figure 6); consequently, the fenoxaprop resistance level decreased significantly (Table 3) owing to the up-regulated expression of bsy-Laccase (Figure 6). These results are in accord with the hypothesis proposed above that laccase down-regulation improves fenoxaprop tolerance via copper homeostasis/energy conservation. The application of CuSO4 induces the expression of laccase via this mechanism, thereby reducing essential oxidase proteins and down-regulating their expression, and finally reducing tolerance. These findings suggest that bsy-miR397 and bsy-Laccase genes might play a role in fenoxaprop-P-ethyl resistance in B. syzigachne plants, and that increased levels of miR397 might be involved in the improvement of fenoxaprop-P-ethyl tolerance in rice.
Oxidases and peroxidases, such as those identified to be up-regulated in our previous RNA-seq analysis (Pan et al., 2016), might be involved in converting herbicide molecules into more hydrophilic metabolites, contributing to herbicide resistance (Délye, 2013). The relative abundance of laccases and peroxidases has been reported to be reciprocally regulated to mediate lignin polymerization in the Ptr-MIR397a regulatory networks, further contributing to the functional redundancy of enzymes for oxidative polymerization (Lu et al., 2013). Given that oxidases are important in NTSR, laccase and oxidases/peroxidases might also be reciprocally regulated to finally increase herbicide tolerance via the miR397 regulatory networks (Lu et al., 2013). In a previous RNA-seq analysis in the fenoxaprop-P-ethyl-resistant B. syzigachne populations used in the present study, we also found that two genes each in the oxidase gene family (ubiquinol oxidase 1 and l-ascorbate oxidase) and peroxidase family (peroxidase 1 and peroxidase 66) were constitutively up-regulated (Pan et al., 2016). In addition, four TFs (bsy-MYB39, bsy-MYB2, bsy-ARF5, and bsy-ARF8) were found to be consistently down-regulated in the resistant plants tested (R1, R2, and R3; Table 5). Previously, laccase has been implicated in the network for lignin biosynthesis by putative interactions with several TFs and peroxidases (Lu et al., 2013). In the present study, we also found that these TFs and oxidase/peroxidase genes were expressed in WT and OXmiR397-expressing rice. Their expression patterns are consistent with those in B. syzigachne, including ARF5, MYB2, MYB39, l-ascorbate oxidase, ubiquinol oxidase 1, and peroxidase 1 (Figure 7). As only miR397 was over-expressed in OXmiR397 rice, whereas all the other miRNAs showed the same expression as that in WT, these TFs and oxidase/peroxidase genes might participate in the miR397/laccase mechanism. Our findings indicated that the identified genes, including bsy-miR397, bsy-Laccase, and associated TFs and oxidases/peroxidases, might also form a regulatory network to detoxify fenoxaprop-P-ethyl in B. syzigachne.
Therefore, we hypothesized that in resistant B. syzigachne plants, bsy-miR397 might be part of a regulatory network mediating fenoxaprop-P-ethyl resistance. In resistant B. syzigachne, bsy-miR397 is expressed at a high level and thus down-regulates the abundance of laccases. This down-regulation of bsy-Laccase might regulate the downstream components of a network. Bsy-Laccase is implicated in such a network via the putative interactions with three TFs (bsy-MYB39, bsy-MYB2, and bsy-ARF5). As mentioned above, laccase down-regulation reserve energy for more essential oxidases proteins via copper homeostasis/energy conservation. These three TFs (bsy-MYB39, bsy-MYB2, and bsy-ARF5) might also control the up-regulation of three downstream pathway genes identified previously (ubiquinol oxidase 1, l-ascorbate oxidase, and peroxidase 1) (Pan et al., 2016). NTSR is largely conferred by an increase in the expression of oxidases/peroxidases to introduce an active moiety into herbicide molecules in Phase-2 metabolism (Délye, 2013). Therefore, the coordinated up-regulation of these three oxidase/peroxidase genes are likely involved in fenoxaprop-P-ethyl detoxification. The detected expression changes indicate the involvement of bsy-miR397/laccases and suggest bsy-miR397 as a regulator in fenoxaprop-P-ethyl-resistant B. syzigachne. However, all of the proposed interactions require further experimental validation. Notably, the effect is evident only in response to fenoxaprop-P-ethyl and not to haloxyfop-R-methyl, suggesting that the bsy-miR397/laccases regulation mechanism might not be a general stress response. This need further investigation in the future.
In China, fenoxaprop-P-ethyl is the most widely used and highly efficient herbicide for controlling grass weeds in wheat fields. Its extensive and continuous use, often as the sole method of weed control, has resulted in the evolution of fenoxaprop-P-ethyl resistance in weeds. Accordingly, fenoxaprop-P-ethyl-resistant B. syzigachne has become one of the most predominant and troublesome weeds in wheat fields in China and has been reported in several regions of the country (Li et al., 2013, 2014; Pan et al., 2015a,b,c; Tang et al., 2015). However, although two classes of GST genes have been reported to be involved in fenoxaprop-P-ethyl resistance in black-grass (A. myosuroides) and ryegrass (Lolium rigidum) populations (Cummins et al., 1999, 2013), our understanding of NTSR to fenoxaprop-P-ethyl is limited. Elevated levels of P450 and GST enzymes have been reported in several fenoxaprop-P-ethyl-resistant black-grass populations (Reade and Cobb, 2002; Letouze and Gasquez, 2003). In addition, fenoxaprop resistance in black grass has recently been suggested to be caused by the scavenging peroxidase activities of specific GST enzymes as well as by the concomitant production of protective flavonoids to counteract the free noxious radicals generated by the herbicide action (Cummins et al., 2013).
In the three fenoxaprop-P-ethyl-resistant B. syzigachne populations (R1, R2, and R3) identified in this study, the resistance mechanisms to fenoxaprop-P-ethyl are diverse involving both TSR and multiple NTSR mechanisms. TSR to fenoxaprop-P-ethyl in this species is due to point mutations in the ACCase CT domain (Pan et al., 2015a), as with the other three populations JYDX-R, SHQP-R, and JYSC-R used in the present study. However, NTSR also exists in populations R1, R2, and R3 and is more complex, as we have detected structural changes and altered expression levels of 15 genes encoding metabolizing enzymes (Pan et al., 2016). In addition, bsy-miR397 down-regulating laccase expression, as described in the present study, represents the first example of how down-regulation of a gene (laccase) can enhance NTSR in B. syzigachne. Knowledge of these resistance-regulating mechanisms is important for designing effective weed control strategies to manage and delay the onset of resistance to fenoxaprop-P-ethyl.
In summary, to our knowledge, this is the first report investigating how down-regulation of a gene (laccase) can enhance NTSR. We found that bsy-Laccase was inhibited by bsy-miR397 via transient expression in tobacco. We also found that the overexpression of miR397 correlated with fenoxaprop-P-ethyl resistance in B. syzigachne and improved fenoxaprop-P-ethyl tolerance in rice. Therefore, we propose that bsy-miR397 might play a role in NTSR to fenoxaprop-P-ethyl in B. syzigachne by down-regulating laccase expression, leading to associated changes in oxidase/peroxidase genes. The findings of this study thus indicated that NTSR might be driven by the manipulation of miR397/Laccase via the modulation of NTSR might be regulated by miR397/Laccase, and this may open a new avenue for understanding and managing complex NTSR in weedy plant species.
Author Contributions
LP, QY, and LD designed the experiments. LP and HZ performed the experimental work. LP, HZ, LB and LD performed the data analysis. LD supervised the research and manuscript preparation. All authors wrote and edited the manuscript.
Conflict of Interest Statement
The authors declare that the research was conducted in the absence of any commercial or financial relationships that could be construed as a potential conflict of interest.
Acknowledgments
This research was funded by National Natural Science Foundation of China (31572021) and Special Fund for Agro-scientific Research in the Public Interest (201303031). The authors thank Prof. Yueqin Chen (Sun Yat-sen University, China) for providing rice seeds.
Supplementary Material
The Supplementary Material for this article can be found online at: http://journal.frontiersin.org/article/10.3389/fpls.2017.00879/full#supplementary-material
References
Abdel-Ghany, S. E., and Pilon, M. (2008). MicroRNA-mediated systemic down-regulation of copper protein expression in response to low copper availability in Arabidopsis. J. Biol. Chem. 283, 15932–15945. doi: 10.1074/jbc.M801406200
Arredondo, M., and Nunez, M. T. (2005). Iron and copper metabolism. Mol. Aspects Med. 26, 313–327. doi: 10.1016/j.mam.2005.07.010
Berthet, S., Demont-Caulet, N., Pollet, B., Bidzinski, P., Cézard, L., Le Bris, P., et al. (2011). Disruption of Laccase4 and 17 results in tissue-specific alterations to lignification of Arabidopsis thaliana stems. Plant Cell 23, 1124–1137. doi: 10.1105/tpc.110.082792
Busi, R., Neve, P., and Powles, S. (2013). Evolved polygenic herbicide resistance in Lolium rigidum by low-dose herbicide selection within standing genetic variation. Evol. Appl. 6, 231–242. doi: 10.1111/j.1752-4571.2012.00282.x
Chen, H., Zou, Y., Shang, Y., Lin, H., Wang, Y., Cai, R., et al. (2008). Firefly luciferase complementation imaging assay for protein-protein interactions in plants. Plant Physiol. 146, 368–376. doi: 10.1104/pp.107.111740
Claus, H. (2004). Laccases: structure, reactions, distribution. Micron 35, 93–96. doi: 10.1016/j.micron.2003.10.029
Cummins, I., Cole, D. J., and Edwards, R. (1999). A role for glutathione transferases functioning as glutathione peroxidases in resistance to multiple herbicides in black-grass. Plant J. 18, 285–292. doi: 10.1046/j.1365-313X.1999.00452.x
Cummins, I., Wortley, D. J., Sabbadin, F., He, Z., Coxon, C. R., Straker, H. E., et al. (2013). Key role for a glutathione transferase in multiple-herbicide resistance in grass weeds. Proc. Natl. Acad. Sci. U.S.A. 110, 5812–5817. doi: 10.1073/pnas.1221179110
Délye, C. (2013). Unravelling the genetic bases of non-target-site-based resistance (NTSR) to herbicides: a major challenge for weed science in the forthcoming decade. Pest Manag. Sci. 69, 176–187. doi: 10.1002/ps.3318
Duhoux, A., Carrère, S., Gouzy, J., Bonin, L., and Délye, C. (2015). RNA-Seq analysis of rye-grass transcriptomic response to an herbicide inhibiting acetolactate-synthase identifies transcripts linked to non-target-site-based resistance. Plant Mol. Biol. 87, 473–487. doi: 10.1007/s11103-015-0292-293
Earley, K. W., Haag, J. R., Pontes, O., Opper, K., Juehne, T., Song, K., et al. (2006). Gateway-compatible vectors for plant functional genomics and proteomics. Plant J. 45, 616–629. doi: 10.1111/j.1365-313X.2005.02617.x
Gaines, T. A., Lorentz, L., Figge, A., Herrmann, J., Maiwald, F., Ott, M.-C., et al. (2014). RNA-Seq transcriptome analysis to identify genes involved in metabolism-based diclofop resistance in Lolium rigidum. Plant J. 78, 865–876. doi: 10.1111/tpj.12514
Gardin, J. A. C., Gouzy, J., Carrère, S., and Délye, C. (2015). ALOMYbase, a resource to investigate non-target-site-based resistance to herbicides inhibiting acetolactate-synthase (ALS) in the major grass weed Alopecurus myosuroides (black-grass). BMC Genomics 16:590. doi: 10.1186/s12864-015-1804-x
Jeong, D.-H., Park, S., Zhai, J., Gurazada, S. G. R., De Paoli, E., Meyers, B. C., et al. (2011). Massive analysis of rice small RNAs: mechanistic implications of regulated microRNAs and variants for differential target RNA cleavage. Plant Cell 23, 4185–4207. doi: 10.1105/tpc.111.089045
Jin, C., Wang, J., Wu, W., and Wang, Z. (2008). Effects of metal ions and EDTA on ROS free radical produced from Laccase-treated Bamboo Powder. J. Northeast For. Univ. 36, 26–27.
Jones-Rhoades, M. W., and Bartel, D. P. (2004). Computational identification of plant microRNAs and their targets, including a stress-induced miRNA. Mol. Cell 14, 787–799. doi: 10.1016/j.molcel.2004.05.027
Joubès, J., De Schutter, K., Verkest, A., Inzé, D., and De Veylder, L. (2004). Conditional, recombinase-mediated expression of genes in plant cell cultures. Plant J. 37, 889–896. doi: 10.1111/j.1365-313X.2004.02004.x
Khraiwesh, B., Zhu, J. K., and Zhu, J. (2012). Role of miRNAs and siRNAs in biotic and abiotic stress responses of plants. Biochim. Biophys. Acta 1819, 137–148. doi: 10.1016/j.bbagrm.2011.05.001
LaFayette, P. R., Eriksson, K. E. L., and Dean, J. F. D. (1999). Characterization and heterologous expression of laccase cDNAs from xylem tissues of yellow-poplar (Liriodendron tulipifera). Plant Mol. Biol. 40, 23–35. doi: 10.1023/a:1026437406859
Leng, N., Dawson, J. A., Thomson, J. A., Ruotti, V., Rissman, A. I., Smits, B. M. G., et al. (2013). EBSeq: an empirical Bayes hierarchical model for inference in RNA-seq experiments. Bioinformatics 29, 1035–1043. doi: 10.1093/bioinformatics/btt087
Letouze, A., and Gasquez, J. (2003). Enhanced activity of several herbicide-degrading enzymes: a suggested mechanism responsible for multiple resistance in blackgrass (Alopecurus myosuroides Huds.). Agronomie 23, 601–608. doi: 10.1051/agro:2003036
Li, B., and Dewey, C. N. (2011). RSEM: accurate transcript quantification from RNA-Seq data with or without a reference genome. BMC Bioinformatics 12:323. doi: 10.1186/1471-2105-12-323
Li, L., Bi, Y., Liu, W., Yuan, G., and Wang, J. (2013). Molecular basis for resistance to fenoxaprop-p-ethyl in American sloughgrass (Beckmannia syzigachne Steud.). Pestic. Biochem. Physiol. 105, 118–121. doi: 10.1016/j.pestbp.2012.12.007
Li, L., Du, L., Liu, W., Yuan, G., and Wang, J. (2014). Target-site mechanism of ACCase-inhibitors resistance in American sloughgrass (Beckmannia syzigachne Steud.) from China. Pestic. Biochem. Physiol. 110, 57–62. doi: 10.1016/j.pestbp.2014.03.001
Li, T., Li, H., Zhang, Y. X., and Liu, J. Y. (2011). Identification and analysis of seven H2O2-responsive miRNAs and 32 new miRNAs in the seedlings of rice (Oryza sativa L. ssp. indica). Nucleic Acids Res. 39, 2821–2833. doi: 10.1093/nar/gkq1047
Liang, M., Davis, E., Gardner, D., Cai, X., and Wu, Y. (2006). Involvement of AtLAC15 in lignin synthesis in seeds and in root elongation of Arabidopsis. Planta 224, 1185-1196. doi: 10.1007/s00425-006-0300-306
Livak, K. J., and Schmittgen, T. D. (2001). Analysis of relative gene expression data using Real-Time quantitative PCR and the 2-ΔΔCT method. Methods 25, 402–408. doi: 10.1006/meth.2001.1262
Llave, C., Xie, Z., Kasschau, K. D., and Carrington, J. C. (2002). Cleavage of scarecrow-like mRNA targets directed by a class of Arabidopsis miRNA. Science 297, 2053–2056. doi: 10.1126/science.1076311
Lu, S., Li, Q., Wei, H., Chang, M.-J., Tunlaya-Anukit, S., Kim, H., et al. (2013). Ptr-miR397a is a negative regulator of laccase genes affecting lignin content in Populus trichocarpa. Proc. Natl. Acad. Sci. U.S.A. 110, 10848–10853. doi: 10.1073/pnas.1308936110
Luo, Y. C., Zhou, H., Li, Y., Chen, J. Y., Yang, J.-H., Chen, Y. Q., et al. (2006). Rice embryogenic calli express a unique set of microRNAs, suggesting regulatory roles of microRNAs in plant post-embryogenic development. FEBS Lett. 580, 5111–5116. doi: 10.1016/j.febslet.2006.08.046
Mayer, A. M., and Staples, R. C. (2002). Laccase: new functions for an old enzyme. Phytochemistry 60, 551–565. doi: 10.1016/S0031-9422(02)00171-1
Mortazavi, A., Williams, B. A., McCue, K., Schaeffer, L., and Wold, B. (2008). Mapping and quantifying mammalian transcriptomes by RNA-Seq. Nat. Methods 5, 621–628. doi: 10.1038/nmeth.1226
Pan, L., Gao, H. T., Xia, W. W., Zhang, T., and Dong, L. Y. (2016). Establishing a herbicide-metabolizing enzyme library in Beckmannia syzigachne to identify genes associated with metabolic resistance. J. Exp. Bot. 67, 1745–1757. doi: 10.1093/jxb/erv565
Pan, L., Li, J., Xia, W. W., Zhang, D., and Dong, L. Y. (2015a). An effective method, composed of LAMP and dCAPS, to detect different mutations in fenoxaprop-P-ethyl-resistant American sloughgrass (Beckmannia syzigachne Steud.) populations. Pestic. Biochem. Physiol. 117, 1–8. doi: 10.1016/j.pestbp.2014.10.008
Pan, L., Li, J., Zhang, T., Zhang, D., and Dong, L. Y. (2015b). Cross-resistance patterns to acetyl coenzyme A carboxylase (ACCase) inhibitors associated with different ACCase mutations in Beckmannia syzigachne. Weed Res. 55, 609–620. doi: 10.1111/wre.12170
Pan, L., Li, J., Zhang, W. N., and Dong, L. (2015c). Detection of the I1781L mutation in fenoxaprop-p-ethyl-resistant American sloughgrass (Beckmannia syzigachne Steud.), based on the loop-mediated isothermal amplification method. Pest Manag. Sci. 71, 123–130. doi: 10.1002/ps.3777
Powles, S. B., and Yu, Q. (2010). Evolution in action: plants resistant to herbicides. Annu. Rev. Plant Biol. 61, 317–347. doi: 10.1146/annurev-arplant-042809-112119
Qi, Y., Wang, S., Shen, C., Zhang, S., Chen, Y., Xu, Y., et al. (2012). OsARF12, a transcription activator on auxin response gene, regulates root elongation and affects iron accumulation in rice (Oryza sativa). New Phytol. 193, 109–120. doi: 10.1111/j.1469-8137.2011.03910.x
Ranocha, P., Chabannes, M., Chamayou, S., Danoun, S., Jauneau, A., Boudet, A.-M., et al. (2002). Laccase down-regulation causes alterations in phenolic metabolism and cell wall structure in poplar. Plant Physiol. 129, 145–155. doi: 10.1104/pp.010988
Reade, J. P. H., and Cobb, A. H. (2002). New, quick tests for herbicide resistance in black-grass (Alopecurus myosuroides Huds) based on increased glutathione S-transferase activity and abundance. Pest Manag. Sci. 58, 26–32. doi: 10.1002/ps.411
Schwab, R., Ossowski, S., Riester, M., Warthmann, N., and Weigel, D. (2006). Highly specific gene silencing by artificial microRNAs in Arabidopsis. Plant Cell 18, 1121–1133. doi: 10.1105/tpc.105.039834
Sunkar, R., Li, Y. F., and Jagadeeswaran, G. (2012). Functions of microRNAs in plant stress responses. Trends Plant Sci. 17, 196–203. doi: 10.1016/j.tplants.2012.01.010
Sunkar, R., and Zhu, J. K. (2004). Novel and stress-regulated microRNAs and other small RNAs from Arabidopsis. Plant Cell 16, 2001–2019. doi: 10.1105/tpc.104.022830
Tang, W., Zhou, F., Zhang, Y., and Chen, J. (2015). Resistance of American sloughgrass (Bechmannia syzigachne) populations to ACCase-inhibiting herbicides involves three different target site mutations from China. Pestic. Biochem. Physiol. 124, 93–96. doi: 10.1016/j.pestbp.2015.05.003
Vaahtera, L., and Brosché, M. (2011). More than the sum of its parts – How to achieve a specific transcriptional response to abiotic stress. Plant Sci. 180, 421–430. doi: 10.1016/j.plantsci.2010.11.009
Wang, G. D., Li, Q. J., Luo, B., and Chen, X. Y. (2004). Ex planta phytoremediation of trichlorophenol and phenolic allelochemicals via an engineered secretory laccase. Nat. Biotech. 22, 893–897. doi: 10.1038/nbt982
Warthmann, N., Chen, H., Ossowski, S., Weigel, D., and Hervé, P. (2008). Highly specific gene silencing by artificial miRNAs in rice. PLoS ONE 3:e1829. doi: 10.1371/journal.pone.0001829
Yu, Q., and Powles, S. (2014). Metabolism-based herbicide resistance and cross-resistance in crop weeds: a threat to herbicide sustainability and global crop production. Plant Physiol. 166, 1106–1118. doi: 10.1104/pp.114.242750
Zhang, Y. C., Yu, Y., Wang, C. Y., Li, Z. Y., Liu, Q., Xu, J., et al. (2013). Overexpression of microRNA OsmiR397 improves rice yield by increasing grain size and promoting panicle branching. Nat. Biotechnol. 31, 848–852. doi: 10.1038/nbt.2646
Zhao, B., Liang, R., Ge, L., Li, W., Xiao, H., Lin, H., et al. (2007). Identification of drought-induced microRNAs in rice. Biochem. Bioph. Res. Co. 354, 585–590. doi: 10.1016/j.bbrc.2007.01.022
Zheng, L., Diamond, J. M., and Dentonz, D. L. (2013). Evaluation of whole effluent toxicity data characteristics and use of Welch’s T-test in the test of significant toxicity analysis. Environ. Toxicol. Chem. 32, 468–474. doi: 10.1002/etc.2075
Zhou, L., Liu, Y., Liu, Z., Kong, D., Duan, M., and Luo, L. (2010). Genome-wide identification and analysis of drought-responsive microRNAs in Oryza sativa. J. Exp. Bot. 61, 4157–4168. doi: 10.1093/jxb/erq237
Keywords: Beckmannia syzigachne, fenoxaprop-P-ethyl-resistance, non-target-site resistance, miR397, Laccase, metabolism
Citation: Pan L, Zhao H, Yu Q, Bai L and Dong L (2017) miR397/Laccase Gene Mediated Network Improves Tolerance to Fenoxaprop-P-ethyl in Beckmannia syzigachne and Oryza sativa. Front. Plant Sci. 8:879. doi: 10.3389/fpls.2017.00879
Received: 15 February 2017; Accepted: 10 May 2017;
Published: 23 May 2017.
Edited by:
Viswanathan Chinnusamy, Indian Agricultural Research Institute (ICAR), IndiaReviewed by:
Ratna Karan, University of Florida, United StatesRohit Joshi, Jawaharlal Nehru University, India
Copyright © 2017 Pan, Zhao, Yu, Bai and Dong. This is an open-access article distributed under the terms of the Creative Commons Attribution License (CC BY). The use, distribution or reproduction in other forums is permitted, provided the original author(s) or licensor are credited and that the original publication in this journal is cited, in accordance with accepted academic practice. No use, distribution or reproduction is permitted which does not comply with these terms.
*Correspondence: Liyao Dong, ZGx5QG5qYXUuZWR1LmNu